- 1Biosciences, Faculty of Health and Life Sciences, University of Exeter, Exeter, United Kingdom
- 2ISCA Diagnostics Ltd., Hatherly Laboratories, Exeter, United Kingdom
Mucoromycosis is a highly aggressive angio-invasive disease of humans caused by fungi in the zygomycete order, Mucorales. While Rhizopus arrhizus is the principal agent of mucoromycosis, other Mucorales fungi including Apophysomyces, Cunninghamella, Lichtheimia, Mucor, Rhizomucor and Syncephalastrum are able to cause life-threatening rhino-orbital-cerebral, pulmonary, gastro-intestinal and necrotising cutaneous infections in humans. Diagnosis of the disease currently relies on non-specific CT, lengthy and insensitive culture from invasive biopsy, and time-consuming histopathology of tissue samples. At present, there are no rapid antigen tests that detect Mucorales-specific biomarkers of infection, and which allow point-of-care diagnosis of mucoromycosis. Here, we report the development of an IgG2b monoclonal antibody (mAb), TG11, which binds to extracellular polysaccharide (EPS) antigens of between 20 kDa and 250 kDa secreted during hyphal growth of Mucorales fungi. The mAb is Mucorales-specific and does not cross-react with other yeasts and molds of clinical importance including Aspergillus, Candida, Cryptococcus, Fusarium, Lomentospora and Scedosporium species. Using the mAb, we have developed a Competitive lateral-flow device that allows rapid (30 min) detection of the EPS biomarker in human serum and bronchoalveolar lavage (BAL), with a limit of detection (LOD) in human serum of ~100 ng/mL serum (~224.7 pmol/L serum). The LFD therefore provides a potential novel opportunity for detection of mucoromycosis caused by different Mucorales species.
Introduction
Mucoromycosis (Borman and Johnson, 2023) is a highly destructive angio-invasive disease of humans caused by zygomycete fungi in the order Mucorales (Thornton, 2020), recently characterised as a high priority group in the World Health Organisation’s fungal priority pathogens list (WHO, 2022). The disease encompasses debilitating rhino-orbital-cerebral mucoromycosis (ROCM), and pulmonary, cutaneous, gastro-intestinal and disseminated infections (Petrikkos et al., 2012; Ganesan et al., 2019; Jeong et al., 2019; Thornton, 2023) which, prior to the COVID-19 pandemic, were typically seen in patients with haematological malignancies (Miller et al., 2020), in bone marrow and solid organ transplant recipients (Roden et al., 2005; Song et al., 2017; Miller et al., 2020; Skiada et al., 2020) and in individuals with poorly controlled diabetes mellitus (DM), a major independent risk factor for the disease (Corzo-León et al., 2018; Skiada et al., 2020; Thornton, 2023). However, during the second wave of the pandemic in India, there was a dramatic increase in ROCM in patients with severe SARS-CoV-2 infection, exacerbated by a high background prevalence of DM and the overuse of anti-inflammatory corticosteroids (John et al., 2021; Rodriguez-Morales et al., 2021; Sen et al., 2021). While Rhizopus arrhizus is the principal cause of mucoromycosis worldwide (Prakash and Chakrabarti, 2019; Davies and Thornton, 2022), and was responsible for a large number of cases of COVID-19-associated mucoromycosis (CAM) in India and other countries worldwide (Prakash and Chakrabarti, 2019; Prakash and Chakrabarti, 2021; Hoenigl et al., 2022), Mucorales fungi other than R. arrhizus are able to cause mucoromycosis, including species in the genera Apophysomyces, Cunninghamella, Lichtheimia, Mucor, Rhizomucor, Saksenaea, and Syncephalastrum (Álvarez et al., 2009; Gomes et al., 2011; Zaki et al., 2014; Jeong et al., 2019; Walther et al., 2019; Skiada et al., 2020; Thornton, 2023).
Mucoromycosis is associated with high rates of mortality, with an overall all-cause mortality rate of 54% (Roden et al., 2005). Furthermore, survivors of ROCM are often left with severe facial disfigurement due to the aggressive surgery needed to contain rapidly progressive infections (Sen et al., 2021). The disease is especially problematic in low- to middle-income countries (LMIC), where limited access to well-resourced and appropriately-equipped diagnostic facilities delays diagnosis and treatment (Rudramurthy et al., 2021; Thornton, 2023). There is therefore an urgent need for simple, rapid and accurate diagnostic tests for the disease that can be performed at point-of-care. Lateral-flow immunoassays are ideally suited to point-of-care detection of fungal infections in resource-limited settings (Thornton, 2020; Osaigbovo and Bongomin, 2021; Thornton, 2023), and might help to improve the speed and accuracy of mucoromycosis detection compared to insensitive and time-consuming culture and histopathology, the cornerstones of detection in LMIC countries (Rudramurthy et al., 2021; Thornton, 2023).
At present, there are no antigen biomarker tests which allow rapid, sensitive and specific detection of Mucorales species (Skiada et al., 2020; Lamoth, 2023), and their differentiation from other fungal pathogens such as Aspergillus, Candida, Cryptococcus, Fusarium and Scedosporium (Marques et al., 2011; Obradovic-Tomasev et al., 2014; Skiada et al., 2020; Marino et al., 2023). Despite this, we recently reported the development of a monoclonal antibody (mAb), KC9, specific to R. arrhizus, and its incorporation into a lateral-flow device (KC9-LFD) for rapid detection of an extracellular polysaccharide (EPS) biomarker of the pathogen in human serum and bronchoalveolar lavage (BAL) fluid (Davies and Thornton, 2022). While sensitive and simple to perform, the test detects R. arrhizus only, and so is unable to detect the other Mucorales fungi capable of causing mucoromycosis in humans.
In this paper, we report the development of a murine mAb, TG11, and a Competitive LFD for the detection of a Mucorales-specific EPS biomarker in human serum and BAL. We show that the pan-Mucorales test, when combined with a Cube reader, has a limit of detection (LOD) of ~100 ng/mL serum (~224.7 pmol/L serum). This is the first time, to the best of our knowledge, that a Mucorales-specific mAb has been developed and used in a rapid point-of-care test (POCT) for detection of these life-threatening pathogens.
Materials and methods
Ethics statement
Hybridoma work described in this study was conducted under a UK Home Office Project License, and was reviewed by the institution’s Animal Welfare Ethical Review Board (AWERB) for approval. The work was carried out in accordance with The Animals (Scientific Procedures) Act 1986 Directive 2010/63/EU, and followed all the Codes of Practice which reinforce this law, including all elements of housing, care, and euthanasia of the animals.
Fungal cultures
Fungi (Table 1) were cultured on malt extract agar (MEA; 70145, Sigma) or oatmeal agar (OA; O3506, Sigma). Media were autoclaved 121°C for 15 min prior to use, and fungi were grown at 30°C or 37°C. To induce sporulation in Apophysomyces spp., the fungi were grown on autoclaved Czapek Dox agar (CDA; 70185, Sigma) at 37°C.

Table 1 Details of the fungi used in this study and results of TG11-LFD and KC9-LFD tests of culture filtrates.
Production of hybridomas and screening by indirect ELISA
Extracellular polysaccharides (EPS) were prepared from 6-d-old culture fluids using the method described previously (Davies and Thornton, 2022). For hybridoma production, the immunogen comprised a 1 mg/mL solution of EPS from Lichtheimia corymbifera (strain CBS109940), with 6-week-old BALB/c white mice each given four intra-peritoneal injections (300 µL per injection) of immunogen at 2-week intervals, and a single booster injection 5 days before fusion. Hybridoma cells were produced by the method described elsewhere (Thornton, 2001), and monoclonal antibody (mAb)-producing clones identified in indirect ELISA tests by using 20 μg EPS/mL phosphate-buffered saline (PBS; 137 mM NaCl, 2.7 mM KCl, 8 mM Na2HPO4, 1.5 mM KH2PO4, pH 7.2) immobilised to the wells of Maxisorp microtiter plates (Nunc) at 50 µL/well. Wells containing immobilised antigen were incubated with 50 µL of mAb hybridoma tissue culture supernatant (TCS) for 1 h, after which wells were washed three times, for 5 min each, with PBST (PBS containing 0.05% (v/v) Tween-20). Goat anti-mouse polyvalent immunoglobulin (G, A, M) peroxidase conjugate (PA1-84388, Invitrogen), diluted 1:5000 in PBST, was added to the wells and incubated for a further hour. The plates were washed with PBST as described, given a final 5 min wash with PBS, and bound antibody visualised by incubating wells with tetramethyl benzidine (TMB) substrate solution for 30 min, after which reactions were stopped by the addition of 3 M H2SO4. Absorbance values were determined at 450 nm using a microplate reader (infinite F50, Tecan Austria GmbH). Control wells were incubated with tissue culture medium (TCM) containing 10% (v/v) fetal bovine serum (FBS; FCS-SA, Biosera) only. All incubation steps were performed at 23°C in sealed plastic bags. The threshold for detection of the antigen in ELISA was determined from control means (2 x TCM absorbance values). These values were consistently in the range of 0.050-0.100. Consequently, absorbance values ≥0.100 were considered as positive for the detection of antigen.
Determination of Ig class and sub-cloning procedure
The Ig class of mAbs was determined by using antigen-mediated indirect ELISA (Thornton, 2001). Wells of microtiter plates coated with 20 μg EPS/mL PBS were incubated successively with hybridoma TCS for 1 h, followed by goat anti-mouse IgG1, IgG2a, IgG2b, IgG3, IgM or IgA-specific antiserum (ISO-2, Sigma) diluted 1:3000 in PBST for 30 min, and rabbit anti-goat peroxidase conjugate (A5420, Sigma) diluted 1:1000 for a further 30 min. Bound antibody was visualised with TMB substrate as described. Hybridoma cell lines were sub-cloned three times by limiting dilution, and cell lines were grown in bulk in a non-selective medium, preserved by slowly freezing in FBS/dimethyl sulfoxide (92:8 v/v), and stored in liquid N2.
Antibody purification and enzyme conjugation
Hybridoma TCS of mAb TG11 was harvested by centrifugation at 2,147 x g for 40 min at 4°C, followed by filtration through a 0.8 μM cellulose acetate filter (10462240, GE Healthcare Life Sciences, UK). Culture supernatant was loaded onto a HiTrap Protein A column (17-0402-01, GE Healthcare Life Sciences) using a peristaltic pump P-1 (18-1110-91, GE Healthcare Life Sciences) with a low pulsation flow of 1 mL/min. Columns were equilibrated with 10 mL of PBS, and column-bound antibody was eluted with 5 mL of 0.1 M glycine-HCl buffer (pH 2.5) with a flow rate of 0.5 mL/min. The buffer of the purified antibody was exchanged to PBS using a disposable PD-10 desalting column (17-0851-01, GE Healthcare Life Sciences). Following purification, the antibody was sterile filtered with a 0.24 µm syringe filter (85037-574-44, Sartorius) and stored at 4°C. Antibody purity was confirmed by SDS-PAGE and gel staining using Coomassie Brilliant Blue R-250 dye (Thermo Fisher Scientific). Protein A-purified mAb TG11 was conjugated to horseradish peroxidase (HRP) for ELISA studies using a Lightning-Link horseradish peroxidase conjugation kit (701-0000; Bio-Techne Ltd.), or to alkaline phosphatase (AKP) for western blotting studies using a Lightning-Link alkaline phosphatase conjugation kit (702-0010; Bio-Techne Ltd.).
Production of antigen in vitro
For antigen production studies, fungi were grown in liquid YNB+G medium for 72 h at 30°C with shaking (100 rpm) using the method described previously (Davies and Thornton, 2022). Culture fluids were filtered through Miracloth, and then stored at -20°C prior to immunoassay by western blotting and direct ELISA.
For colony blots, MEA was inoculated centrally with 5 μL of a 103 spores/mL spore suspension of L. corymbifera (strain CBS109940) and incubated for 16 h at 30°C, after which the colony was overlayed with PVDF membrane (162-0175, Bio-Rad) for 8 h to bind extracellular antigens. The membrane was removed and discarded, the colony incubated for a further 16 h, and the blotting procedure repeated. The membrane was blocked and processed with TG11-AKP conjugate as described for western blotting.
Polyacrylamide gel electrophoresis and western blotting
Sodium-dodecyl-sulphate-polyacrylamide gel electrophoresis (SDS-PAGE) was carried out using 4–20% gradient polyacrylamide gels (4561094, Bio-Rad) under denaturing conditions. Antigens in EPS preparations and culture filtrates were separated electrophoretically at 165 V, and pre-stained markers (1610377, Bio-Rad) were used for molecular weight determinations. For western blotting, separated antigens were transferred electrophoretically onto a PVDF membrane for 2 h at 75 V, and the membrane was blocked for 16 h at 4°C in PBS containing 1% (w/v) BSA. Blocked membranes were incubated with TG11-AKP conjugate diluted 1:15,000 (v/v) in PBS containing 0.5% (w/v) BSA (PBSA) for 2 h at 23°C. Membranes were washed three times with PBS, once with PBST and bound antibody visualised by incubation in substrate solution. Reactions were stopped by immersing membranes in dH2O, and membranes were then air dried between sheets of Whatman filter paper.
Monoclonal antibodies JF5 (Thornton, 2008), MC3 (Morad et al., 2018), ED7 (Al-Maqtoofi and Thornton, 2016) and HG12 (Thornton, 2009) were used as controls to confirm the presence of extracellular immuno-reactive antigens in Aspergillus, Candida, Fusarium and Scedosporium culture filtrates and EPS preparations, respectively. The anti-glucuronoxylomannan (GXM) mAb 18B7 (MABF2069, Sigma) was used to confirm the presence of immuno-reactive capsular polysaccharide of Cryptococcus neoformans.
Direct ELISA
For direct ELISA, wells containing immobilised antigen were incubated with 50 µL of a 1:2000 (v/v) dilution of TG11-HRP conjugate in PBST for 1 h followed by TMB substrate solution for 30 min. All washing steps were as described for the indirect ELISA.
TG11 lateral-flow device
LFD configuration
The TG11 Competitive lateral-flow device (TG11-LFD) was manufactured by Lateral Dx (Alloa, Scotland, UK). The test consisted of Kenosha 75 mm backing card; 8950, 222, and 1281 conjugate, top and sample pads, respectively; and a CN95 (12 μm) nitrocellulose membrane. The test (T) line consisted of EPS from the L. corymbifera strain, CBS109940, at a concentration of 0.5 mg/mL, while the internal test control (C) line consisted of goat anti-mouse IgG (Arista Biologicals) at a concentration of 0.25 mg/mL.
LFD specificity
Specificity of the TG11-LFD was determined using purified EPS preparations and with filtrates from 72-h-old YNB+G shake cultures of mucoralean and non-mucoralean yeasts and molds of clinical importance.
For EPS, 100 μL of running buffer (PBS containing 0.1% (v/v) Tween-20) containing 50 μg/mL of EPS was added to the TG11-LFD, and T and C line intensities were recorded after 30 min as artificial units (a.u.) using a Cube reader (Davies and Thornton, 2022). The threshold value for test positivity using EPS was determined from the negative control (running buffer only), which was consistently ≥400 a.u. Consequently, a T line value below the threshold value (400 a.u.) showed a positive test result.
For culture filtrates, samples were mixed 1:10 (v/v) with running buffer and 100 μL was added to the TG11-LFD. The intensities of the T and C lines were recorded after 30 min as artificial units (a.u.) as described. The threshold value for test positivity was determined from the T line values of culture filtrates for non-Mucorales yeasts and molds, which were consistently ≥400 a.u. Consequently, a T line value below the threshold value (400 a.u.) showed a positive TG11-LFD test result. The Rhizopus arrhizus-specific KC9-LFD (Davies and Thornton, 2022) was used for specificity comparisons, with the threshold value for test positivity determined from the T line values of culture filtrates from fungi other than R. arrhizus. These values were consistently ≥700 a.u. Consequently, a T line value below the threshold value (700 a.u.) showed a positive KC9-LFD test result.
LFD tests with human serum and BAL
Normal serum from healthy AB blood group males (H6914, Sigma) was spiked with purified EPS from the L. corymbifera strain, CBS109940, and was stored at -20°C prior to use. On thawing, the serum was mixed 1:1 (v/v) with citrate-dextrose solution (ACD; 22 g/L sodium citrate (C3434, Sigma), 7.3 g/L citric acid (C0759, Sigma) and 24.5 g/L D-(+)-glucose, pH~5.0), heated at 100°C for 5 min in a heating block, and then centrifuged for 5 min at 14,000 x g. The clear supernatant was mixed 1:1 (v/v) with serum running buffer (SRB; PBS containing 0.05% (v/v) Tween-20 and 0.05% (v/v) Triton X-305). One hundred-μL was added to the TG11-LFD, and the intensities of the T and C lines were recorded after 30 min as artificial units (a.u.) using the Cube reader.
Normal BAL from a healthy 59-year-old male (HUMANBAL-0101312, BioIVT) was spiked with purified EPS from the Rhizopus arrhizus var. arrhizus strain, CBS112.07, and was stored at -20°C prior to use. On thawing, the BAL was mixed 1:10 (v/v) with SRB and the resultant 100 μL containing 100 μg EPS/mL was added to the TG11-LFD. Normal BAL mixed 1:10 (v/v) with SRB, and SRB alone, acted as negative controls, with the intensities of the T and C lines recorded after 30 min as artificial units (a.u.) using the Cube reader.
Statistical analysis
Numerical data were analysed using a Student’s t-test (independent, two-tailed) to determine statistical significance.
Results
Production of hybridomas and mAb isotyping
A single hybridoma fusion was performed, and 420 hybridoma cell lines were tested in indirect ELISA tests for recognition of the immunogen. Ten cell lines produced EPS-reactive antibodies of the immunoglobulin classes G2b (IgG2b) or M (IgM). The cell line TG11 (an IgG2b) was selected for further evaluation due to its isotype and broad recognition of Mucorales fungi, but lack of cross-reactivity with non-Mucorales fungi.
Production of antigen in vitro
A study of antigen production by Lichtheimia corymbifera, strain CBS109940, in YNB+G shake culture showed that growth of the pathogen plateaued after 72 h (Figure 1A). Immunoassay of culture filtrates showed that the TG11 antigen was secreted into the culture medium and was first detectable by ELISA (Figure 1B), western blot (Figure 1C) and TG11-LFD (Figures 1D, E) 48 h post-inoculation. In colony immuno-blots, extracellular production of the TG11 antigen was associated with the growing edge of the colony (Figure 1F).
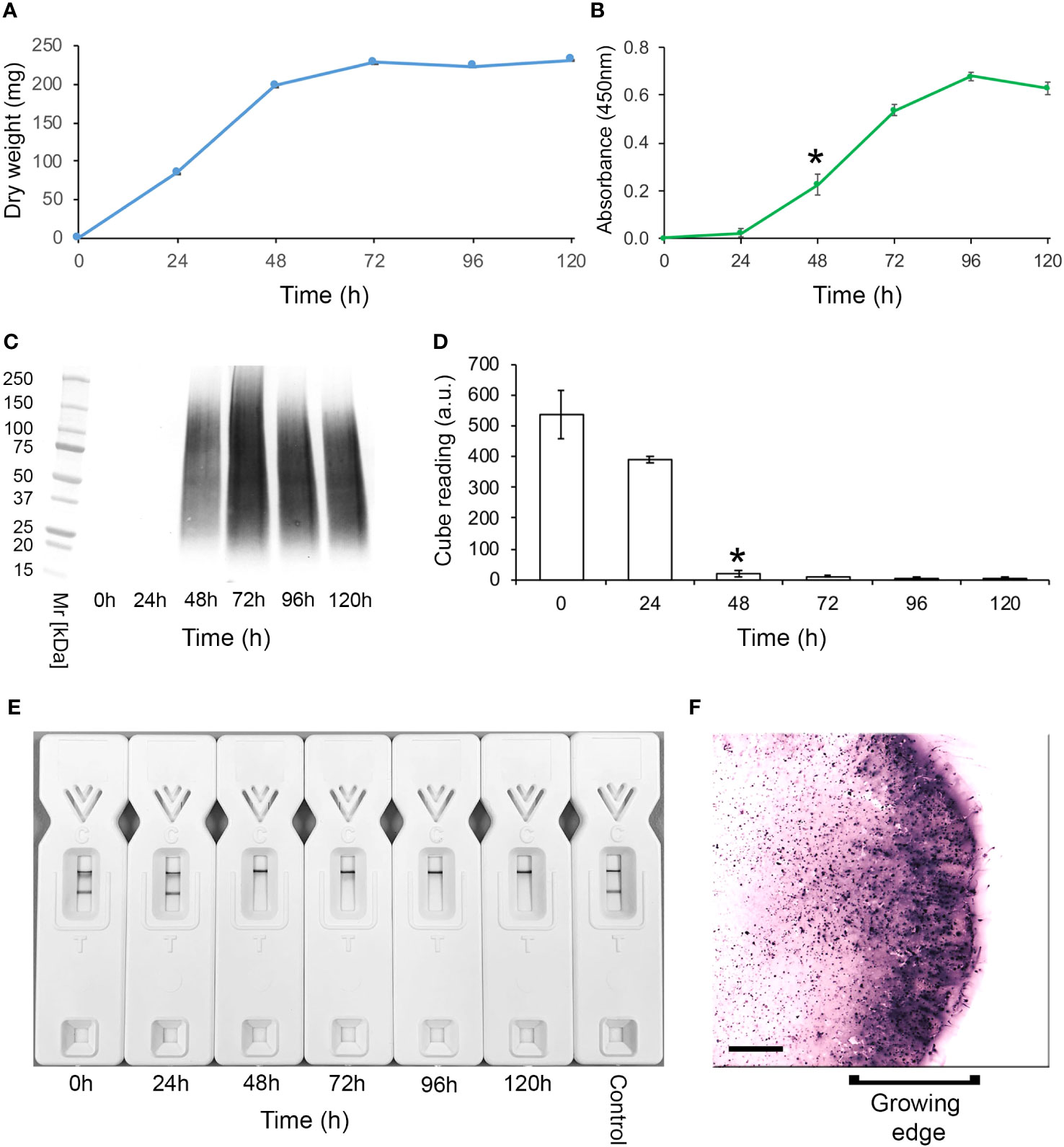
Figure 1 Production of the TG11 antigen by Lichtheimia corymbifera CBS109940. (A) Dry weights of the pathogen over a 5-day experimental period. (B) ELISA and (C) western blots of culture filtrates using mAb TG11, with a significant (Student’s t-test [p < 0.05]) increase in ELISA absorbance value at 48 h (indicated by *) compared to t = 0 h. (D) TG11-LFD test results using the culture filtrates, with test (T) line intensities measured as artificial units (a.u) using a Cube reader. There is a significant (Student’s t-test [p < 0.05]) reduction in T line intensity at 48 h (indicated by *) and thereafter, corresponding to the displacement of T lines in LFD tests at 48h, 72h, 96h and 120h (E). The control (E) comprised running buffer (PBS containing 0.1% (v/v) Tween-20) only. Data points (A, B) and bars (D) are the means of 3 replicates ± SE. All LFD tests had control (C) line scores of >600 a.u. using the Cube reader. (F) Colony immunoblot showing extracellular production of the TG11 antigen. Note the intense immuno-staining of extracellular antigen produced at the growing edge of the colony during active growth of the pathogen. Scale bar = 1cm.
Specificity of mAb TG11 in western blotting, LFD and ELISA immunoassay formats
Culture filtrates
Western blotting studies of 72-h-old culture filtrates showed that mAb TG11 is specific to Mucorales fungi, binding to antigens with molecular weights of between 25 kDa to 250 kDa (Figures 2A-F), and with an additional immuno-reactive antigen of ~25 kDa in strains of Rhizopus arrhizus var. arrhizus (Figure 1B), Mucor circinelloides CBS124429 (Figure 1E) and Mucor indicus CBS120.08 (Figure 1E). A single immuno-reactive antigen of ~20kDa was evident in culture filtrates of Rhizopus homothallicus CBS336.62 (Figure 2D). There was weak binding with culture filtrates from Rhizopus oligosporus strain Tempeh (Figure 2D), Apophysomyces elegans CBS477.78 (Figure 2F) and Apophysomyces variabilis CBS658.93 (Figure 2F), and no binding to antigens in culture filtrates of Apophysomyces ossiformis CBS125533 or Apophysomyces mexicanus CBS136361 (Figure 1F). There was no cross-reaction of mAb TG11 with antigens in culture filtrates from the unrelated molds Aspergillus fumigatus strain Af293 and Aspergillus flavus strain 114B (Figure 2C), the yeast Cryptococcus neoformans CBS8710 (Figure 2D), the molds Fusarium oxysporum CBS167.30 and Scedosporium aurantiacum CBS121926 (Figure 2D), the yeast Candida albicans strain SC5314 (Figure 2E), and the mold Aspergillus terreus CBS601.65 (Figure 2F), despite the presence of extracellular immuno-reactive antigens in the culture filtrates of these fungi (Supplementary Figure 1).
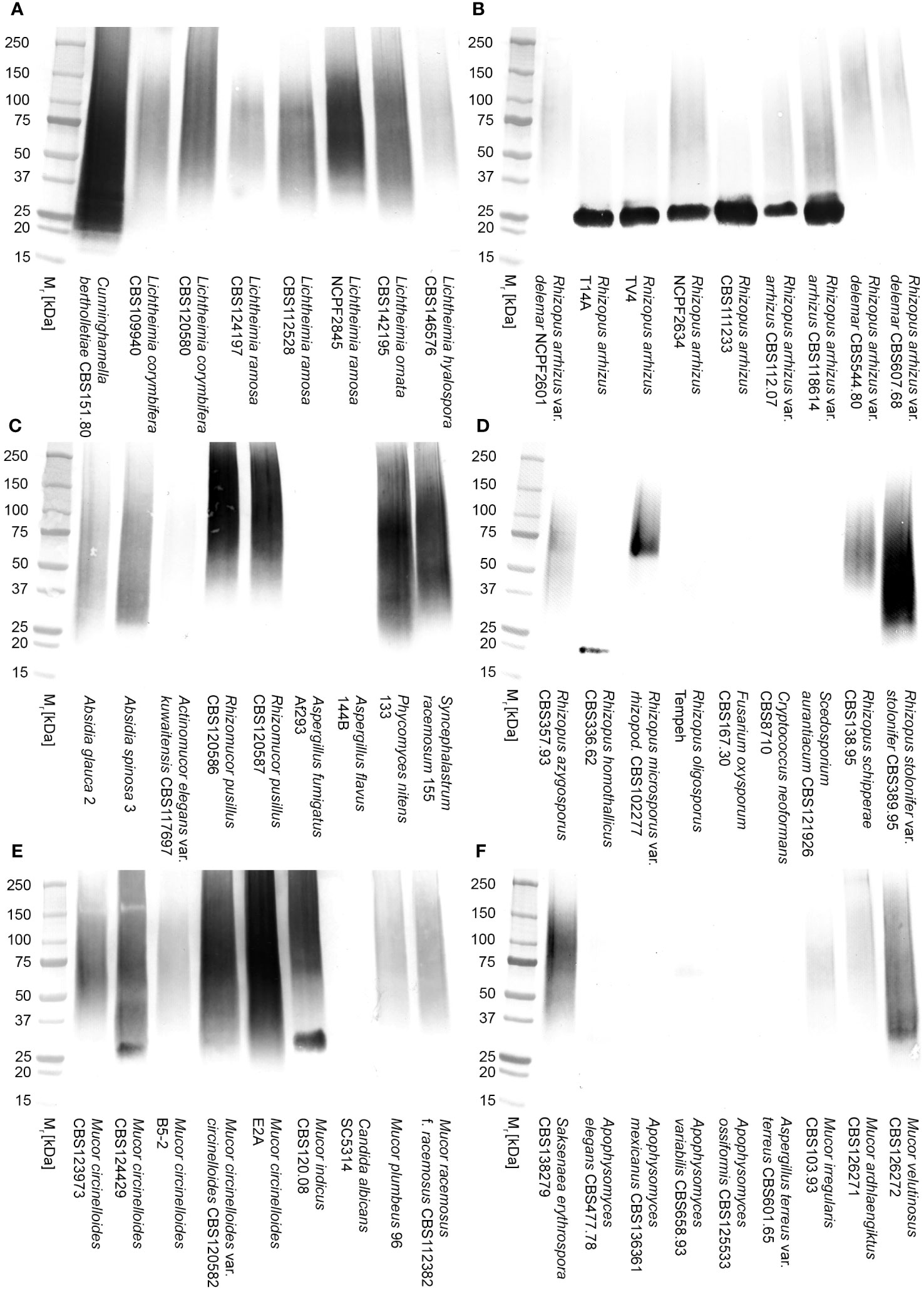
Figure 2 Western blots of culture filtrates from Mucorales fungi and from unrelated yeasts and molds of clinical importance using mAb TG11 (A–F). The corresponding results for TG11-LFD and KC9-LFD tests of culture filtrates are shown in Table 1.
In comparison to the KC9-LFD (Davies and Thornton, 2022), which was specific to antigens in culture filtrates of the Rhizopus arrhizus strains only (Table 1), the TG11-LFD is pan-Mucorales-specific, recognising antigens in culture filtrates from all of the Mucorales fungi tested (Table 1). There was displacement of the test (T) lines in all Mucorales TG11-LFD tests, with Cube reader values below the threshold value for test positivity (400 a.u.). There was no cross-reactivity of the TG11-LFD test with non-Mucorales yeasts and molds (Table 1), with a.u. values exceeding the 400 a.u. threshold value.
Extracellular polysaccharides
In direct ELISA studies, mAb TG11 reacted with EPS preparations from all of the Mucorales fungi tested (Figure 3A), with absorbance values for all species exceeding the threshold value of 0.100 for test positivity. The sensitivity of the ELISA using EPS from the L. corymbifera strain CBS109940 was 109.8 pmol/L, with a range of 439.3 pmol/L to 54.9 pmol/L for the other Mucorales species tested. Western blotting studies with the EPS samples (Figures 3B, C) showed strong reactivity of mAb TG11 with EPS from Lichtheimia spp., Cunninghamella bertholletiae CBS151.80, Rhizomucor pusillus CBS102587, and Apophysomyces variabilis CBS658.93. Despite weaker reactions of mAb TG11 with the Mucor and Rhizopus EPS preparations in western blots (Figures 3B, C), EPS from all of the Mucorales fungi gave strong TG11-LFD test results (Figure 3D), with T line values below the threshold value (400 a.u.) for test positivity.
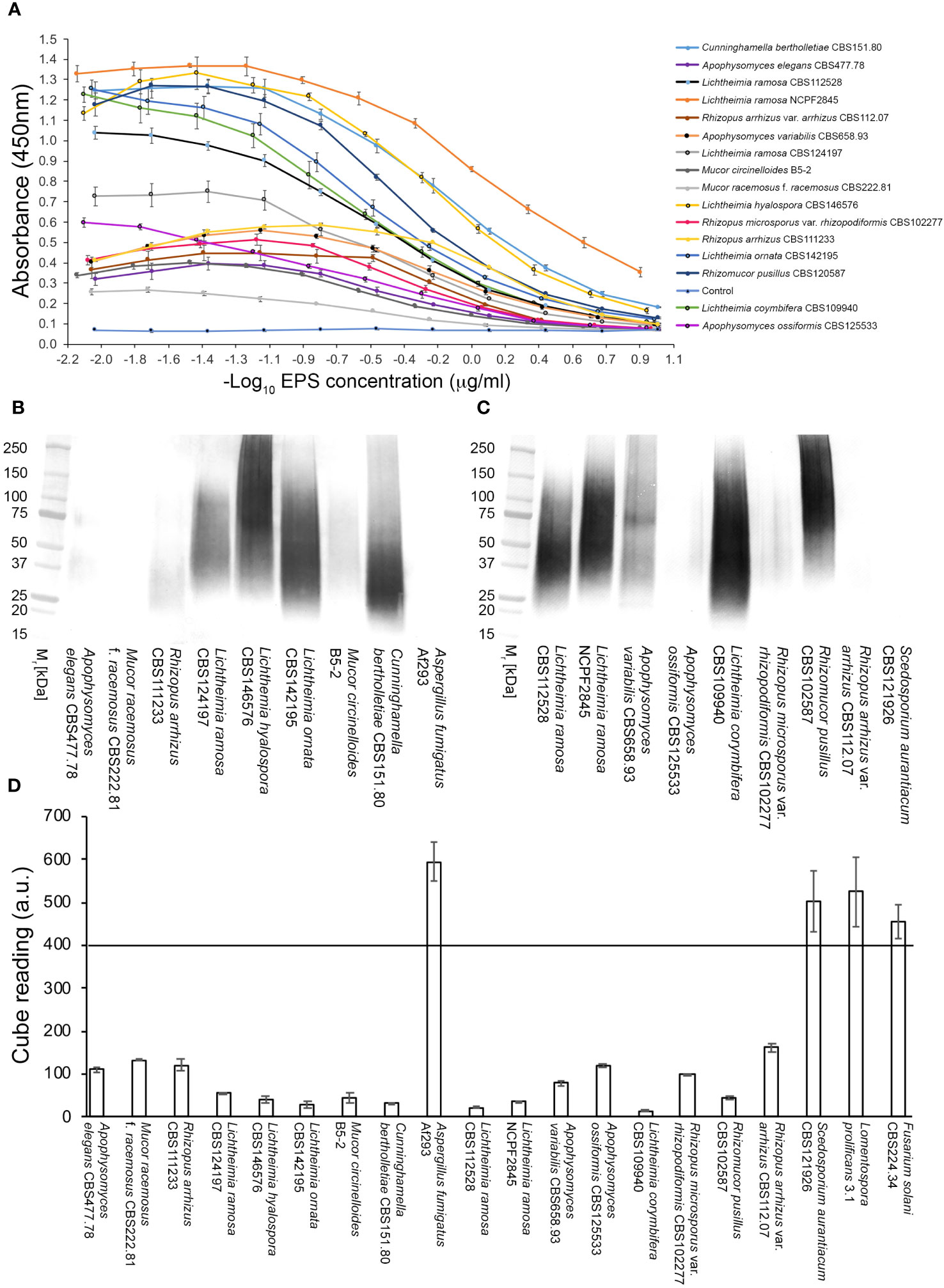
Figure 3 Reactivity of mAb TG11 with extracellular polysaccharide (EPS) from Mucorales spp. (A) ELISA of EPS samples from Mucorales species. The absorbance values of the negative control (phosphate buffered saline only) were consistently ≤0.100. Consequently, a threshold absorbance value of 0.100 was used for ELISA positivity. (B, C) Western blots of EPS samples (20 μg EPS/lane) from Mucorales spp. and from the unrelated human-pathogenic molds Aspergillus fumigatus Af293 and Scedosporium aurantiacum CBS121926. (D) Results of TG11-LFD tests using EPS samples (50 μg EPS/mL running buffer (PBS containing 0.1% (v/v) Tween-20)) from Mucorales spp. and from the unrelated molds A. fumigatus Af293, S. aurantiacum CBS121926, Fusarium solani CBS224.34, and Lomentospora prolificans strain 3.1. The threshold T line value for test positivity is ≤400 a.u. Values above this threshold show a negative LFD test result, while values below the threshold show a positive LFD test result. Data points (A) and bars (D) are the means of 2 replicates ± SE. All LFD tests (D) had control (C) line scores of >600 a.u. using the Cube reader.
There was no cross-reaction of mAb TG11 with EPS samples from the unrelated mold pathogens in either the western blotting (Figures 3B, C), LFD (Figures 3D, 4B) or ELISA (Figure 4A) immunoassay formats, despite the presence of immuno-reactive antigens in these EPS preparations (Figure 4C).
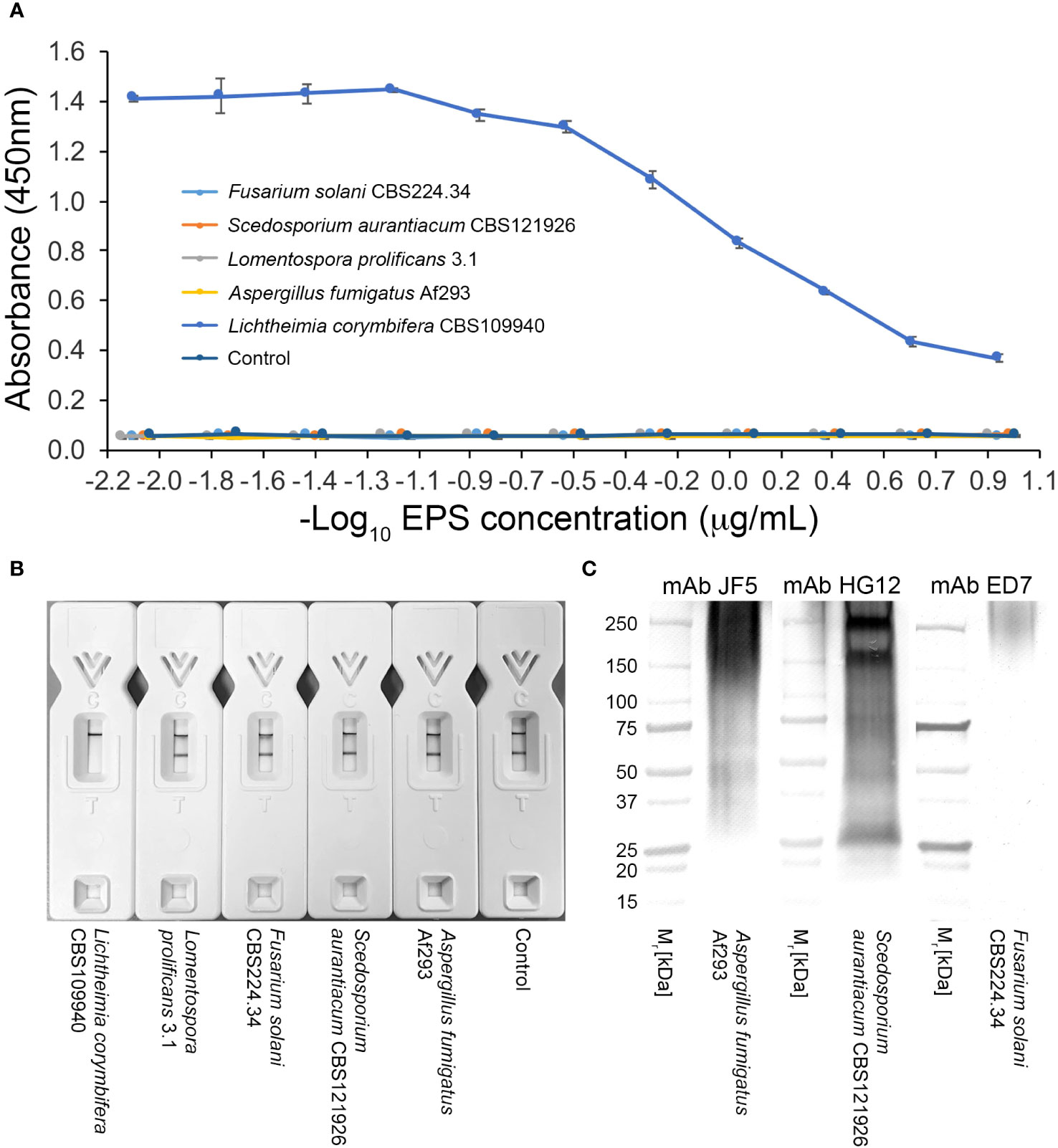
Figure 4 Specificity of mAb TG11. (A) ELISA of EPS samples from unrelated molds of clinical importance. Note the lack of cross-reactivity of mAb TG11 with EPS from the unrelated molds A fumigatus Af293, S. aurantiacum CBS121926, Fusarium solani CBS224.34 and Lomentospora prolificans strain 3.1, compared to the positive control (Lichthemia corymbifera CBS109940). Data points are the means of 2 replicates ± SE, and the negative control consisted of phosphate buffered saline only. (B) Visual appraisal of TG11-LFD specificity using EPS samples (50 μg EPS/mL running buffer (PBS containing 0.1% (v/v) Tween-20)) from L. corymbifera CBS109940 and the unrelated molds. Note the complete displacement of the test (T) line with L. corymbifera EPS compared to the unrelated molds and negative control (running buffer only). Using the Cube reader, all LFD tests had control (C) line scores of >600 a.u. (C) Western blots of EPS preparations (10 μg EPS/lane) from the human-pathogenic molds A fumigatus Af293, S. aurantiacum CBS121926 and Fusarium solani CBS224.34, showing the presence of antigens reactive with the Aspergillus-specific mAb JF5 (Thornton, 2008), Scedosporium-specific mAb HG12 (Thornton, 2009), and Fusarium-specific mAb ED7 (Al-Maqtoofi and Thornton, 2016), respectively.
Limit of detection (LOD) in human serum and compatibility with human BAL
Using the Cube reader, the LOD of the TG11-LFD using human serum spiked with EPS from L. corymbifera, strain CBS109940, was shown to be ~100 ng EPS/mL serum (~224.7 pmol/L serum)(Figure 5A). The TG11-LFD is compatible with human BAL, showing a significant reduction in T line values using BAL spiked with EPS from the Rhizopus arrhizus var. arrhizus, strain CBS112.07, compared to the T line values for normal BAL and SRB only (Figures 5B, C).
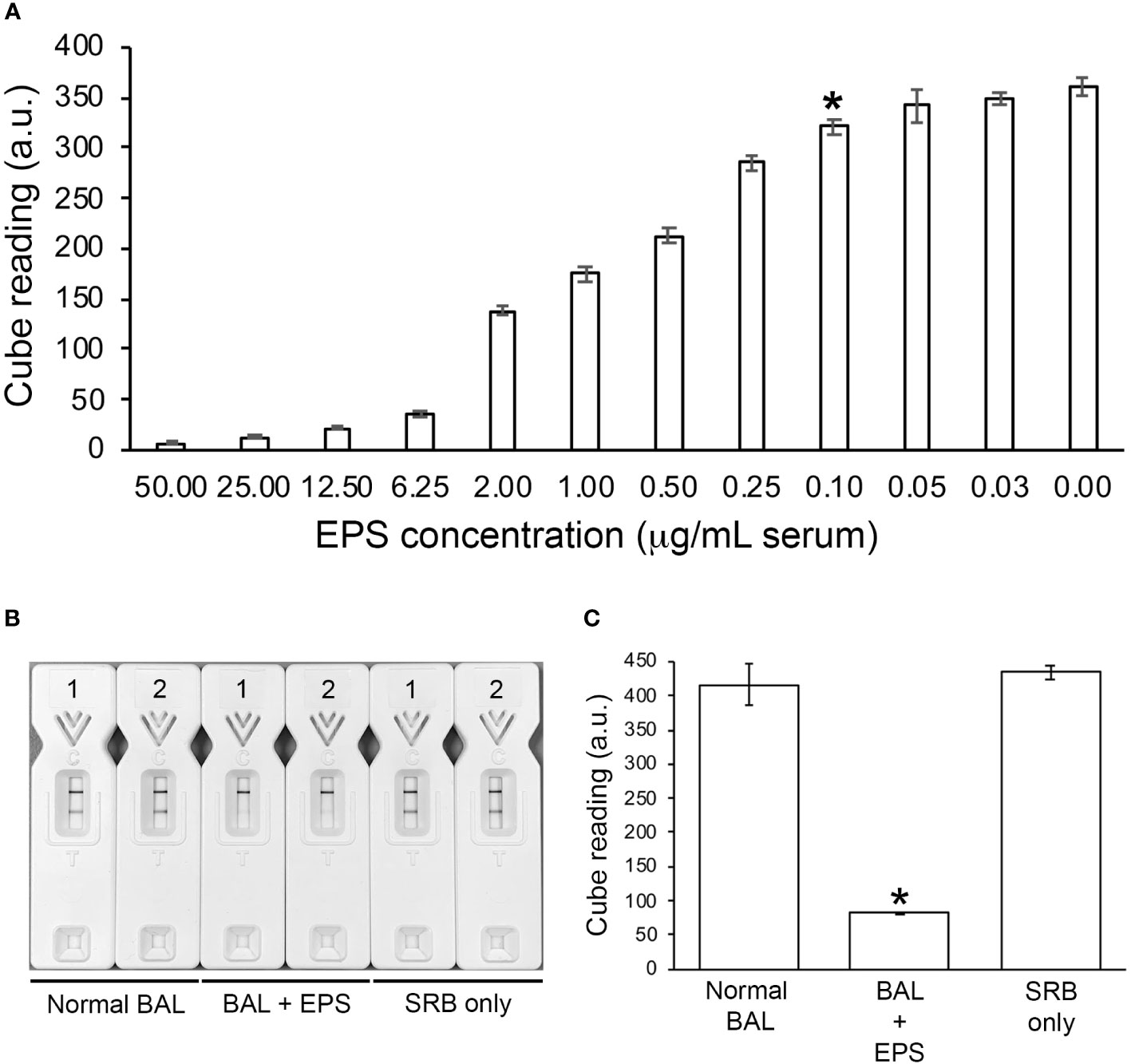
Figure 5 TG11-LFD tests with human serum and BAL. (A) Sensitivity of the TG11-LFD using L. corymbifera CBS109940 EPS diluted into human serum. Using the Cube reader, there were sequential decreases in test (T) line intensities with increase in EPS concentrations between 0.03 μg and 50.00 μg EPS/mL serum, with a significant reduction (Student’s t-test [p < 0.05]) at 0.10 μg EPS/mL serum (indicated by *) and thereafter compared to the control (normal serum only [0.00 μg EPS/mL serum]). Based on this result, the limit of detection (LOD) of the TG11-LFD is determined to be ~100 ng EPS/mL serum (~224.7 pmol/L serum). Data points are the means of 3 replicates ± SE, and all LFD tests had control (C) line scores of >600 a.u. (B) Visual appraisal of TG11-LFD compatibility with human BAL. Two replicate LFD tests with BAL samples spiked with 100 μg/mL EPS (BAL+EPS) from Rhizopus arrhizus var. arrhizus CBS112.07 are shown alongside replicate tests with normal BAL and serum running buffer (SRB) only. (C) Cube readings (a.u.) for the replicate LFD tests shown in (B). Each value is the mean a.u. of the 2 replicate tests ± SE, and all LFD tests had control (C) line scores of >600 a.u. using the Cube reader. There is a significant (Student’s t-test [p < 0.05]) reduction (indicated by *) in the T line intensities of the BAL+EPS tests compared to both of the controls (normal BAL and SRB only), but no significant difference between the T line intensities of the two controls.
Discussion
In this paper, we describe the development and characterisation of a murine IgG2b monoclonal antibody (mAb), TG11, raised against an extracellular polysaccharide (EPS) antigen from Lichtheimia corymbifera, and its incorporation into a Mucorales-specific lateral-flow device (TG11-LFD) for detection of the EPS biomarker in human serum and BAL.
The antigen bound by mAb TG11 is secreted into culture medium following germination of sporangiospores, and is associated with active hyphal growth in colony immunoblots. Using a combination of immunoassay tests (ELISA, western blotting and LFD) of crude culture filtrates and purified EPS from Mucorales species and from unrelated molds and yeasts of clinical importance, we have shown that the mAb and the TG11-LFD are Mucorales-specific. Differences in reactivity of the mAb to antigens in crude culture filtrates and to purified EPS were observed in western blotting studies (for example, weak recognition of A. variabilis antigen in crude culture filtrate, but strong recognition of purified A. variabilis EPS). The reason for this discrepancy is currently unknown, but may be related to lower concentrations of antigen in the crude filtrates. Despite this, there was consistent recognition of antigens in both sources by the TG11-LFD, the intended clinical immunoassay format. All Mucorales fungi were detected, including the most common causes of mucoromycosis worldwide, namely Lichtheimia spp., Mucor circinelloides, Rhizopus arrhizus, and Rhizopus microsporus var. rhizopodiformis (Roden et al., 2005; Gomes et al., 2011; Skiada et al., 2011; Laternier et al., 2012; Zaki et al., 2014; Prakash and Chakrabarti, 2019; Skiada et al., 2020; Radotra and Challa, 2022; Özbek et al., 2023; Pham et al., 2023; Yang et al., 2023), and also rarer, emerging, or more regionally-prevalent causes of the disease (Gomes et al., 2011; Skiada et al., 2020) including Actinomucor (Tully et al., 2009), Apophysomyces (Chander et al., 2015), Cunninghamella (Mita et al., 2022), other Mucor spp. (Deja et al., 2006; Álvarez et al., 2011; Lu et al., 2013; Chander et al., 2018), Rhizomucor (Chander et al., 2018; Schober et al., 2021), other Rhizopus spp. (Anstead et al., 1999; Kokkayil et al., 2017; Chander et al., 2018; Kanwar et al., 2021), Sakseneae (Chander et al., 2017), and Syncephalastrum (Irshad et al., 2020). Importantly, mAb TG11 does not cross-react with other fungal pathogens which have been encountered in mixed infections with Mucorales spp., including Aspergillus spp. (Arndt et al., 2009; Bergantim et al., 2013; Henn et al., 2014; Obradovic-Tomasev et al., 2014; Pandey et al., 2019; Anita et al., 2021; Bellanger et al., 2021; Benhadid-Brahmi et al., 2022; Buil et al., 2021; Johnson et al., 2021; Moorthy et al., 2021; Paul et al. (2022); Ramírez-Hinojosa et al., 2021; Singh et al., 2021; Zayet et al., 2021; Rahna et al., 2022; Suresh et al., 2022; Tabarsi et al., 2022; Teng et al., 2022; Zhan et al., 2022; Murray et al., 2023; Sardeshmukh et al., 2023), Fusarium spp. (De Almeida Junior et al., 2015; Marino et al., 2023), Candida albicans (Obradovic-Tomasev et al., 2014; Pandey et al., 2019; Jawanda et al., 2022), Cryptococcus neoformans (Henn et al., 2014; Gupta et al., 2023), Lomentospora prolificans (Erami et al., 2023), and Scedosporium apiospermum (Shand et al., 2004; Marques et al., 2011; Song et al., 2022; Kanaujia et al., 2023).
The pan-Mucorales-specificity of mAb TG11 makes it a suitable candidate for incorporation into a rapid LFD test for detection of the Mucorales-specific EPS biomarker. While a pan-Mucorales mitochondrial rnl (encoding for large-subunit-ribosomal-RNA) gene has been shown to be a novel molecular marker for Mucorales fungi (Caramalho et al., 2019), and a Mucorales-specific IgM mAb (WSSA-RA-1) which binds to intracellular cytoplasmic antigens of between 14 kDa and 110 kDa has previously been reported and used in the immunohistochemical detection of bovine mucoromycosis (Jensen et al., 1996), this is the first time, to the best of our knowledge, that a pan-Mucorales-specific mAb which binds to an extracellular antigenic Mucorales biomarker has been reported. Previously, we described the development of a Competitive LFD for the serological detection of Rhizopus arrhizus, the principal global agent of mucoromycosis in humans. The species-specific LFD employs a mAb, KC9, which binds to a single epitope present within a 15 kDa extracellular EPS antigen (Davies and Thornton, 2022). We have found that mAb TG11 similarly binds to a single epitope within larger 25 kDa to 250 kDa Mucorales antigens, necessitating its use in a Competitive lateral-flow immunoassay format. The Competitive immunoassay format is ideally suited to mAbs which bind to single epitopes, with Competitive LFDs finding widespread applicability in human medicine, environmental sciences, agriculture, and veterinary medicine, for the detection of pathogens, hormones, enzymes, chemicals, narcotic drugs, toxins, and pollutants (Andryukov, 2020; Davies and Thornton, 2022; Khelifa et al., 2022).
The Competitive TG11-LFD test described here relies on soluble antigen in the test sample (buffer, serum or BAL) displacing binding of the gold-conjugated TG11 mAb to purified L. corymbifera EPS present in the test (T) line; the response is therefore negatively correlated to the analyte concentration (the more analyte present, the weaker the signal, with no analyte giving the strongest signal). An advantage of the Competitive LFD format is that it does not suffer from false-negative prozone effects caused by high concentrations of the target antigen as seen in Sandwich LFD formats. Indeed, the cryptococcal antigen (CrAg) semiquantitative (SQ) lateral-flow assay now comprises a Competitive test line to counteract prozone effects (Tadeo et al., 2021).
Current detection of mucoromycosis relies on insensitive and time-consuming culture of fungi from biopsy samples, and on sophisticated laboratory-based PCR, MALDI-TOF, or enzyme-linked immunospot (ELISpot) tests (Lackner et al., 2021; Lamoth, 2023; Thornton, 2023). Detection of mucoromycosis is not possible using the pan-fungal 1→3-β-D-glucan (BDG) test, since Mucorales fungi lack this carbohydrate in their cell walls. Nevertheless, the BDG test and Aspergillus-specific ELISA and LFD tests can be used to rule out aspergillosis, the most common differential diagnosis associated with mucoromycosis. The simplicity, speed, and low cost of LFDs makes them ideally suited to the detection of infectious diseases in low- to middle-income countries (Osaigbovo and Bongomin, 2021), and may aid in the point-of-care detection of mucoromycosis (Thornton, 2023). Currently, no mAb-based serodiagnostic LFD test exists for the specific detection of all infectious Mucorales fungi. A mAb (2DA6) and a lateral-flow immunoassay (LFIA) have been developed that recognise Mucorales species, but the mAb lacks specificity, cross-reacting with an epitope on α-1,6 mannans conserved among human pathogenic yeasts and filamentous fungi including Candida albicans and the angio-invasive moulds Aspergillus, Fusarium, and Scedosporium (Burnham-Marusich et al., 2018). Despite this, the LFIA was able to detect cell wall fucomannan in BALf, serum, and urine samples from diabetic ketoacidotic and neutropenic mice following intratracheal challenge with Rhizopus delemar, Lichtheimia corymbifera, Mucor circinelloides and Cunninghamella bertholletiae (Orne et al., 2018), demonstrating the utility of carbohydrate biomarkers in the diagnosis of mucoromycosis, and their detection using lateral-flow technology.
A hallmark of mucoromycosis is extensive angio-invasion (Spellberg et al., 2005; Ibrahim et al., 2012; Skiada et al., 2020), which presents an opportunity for serological detection of circulating EPS biomarker. We therefore investigated the compatibility of the TG11-LFD with human serum as a minimally-invasive biofluid for biomarker detection. When combined with a simple serum pre-treatment step, we were able to detect the EPS biomarker in spiked serum samples. Furthermore, using a Cube reader, we were able to determine the limit of detection (LOD) of L. corymbifera EPS as ~100 ng/mL serum (~224.7 pmol/L serum), which is within the range of sensitivities for Competitive lateral-flow immunoassays (Di Nardo et al., 2021). The relevance of this LOD has yet to be determined with samples from patients with ROCM, pulmonary, cutaneous or disseminated mucoromycosis, but compatibility of the TG11-LFD with human serum and BAL provides an opportunity for clinical evaluation of the test in different disease backgrounds (e.g. neutropenia, ketoacidosis, diabetes). When combined with the serum- and BAL-compatible R. arrhizus-specific LFD test (Davies and Thornton, 2022), the possibility exists for comprehensive point-of-care detection of all clinically-relevant Mucorales species.
Data availability statement
The raw data supporting the conclusions of this article will be made available by the authors, without undue reservation.
Ethics statement
Hybridoma work described in this study was conducted under a UK Home Office Project License, and was re-viewed by the institution’s Animal Welfare Ethical Review Board (AWERB) for approval. The work was carried out in accordance with The Animals (Scientific Procedures) Act 1986 Directive 2010/63/EU, and followed all the Codes of Practice which reinforce this law, including all elements of housing, care, and euthanasia of the animals. The study was conducted in accordance with the local legislation and institutional requirements.
Author contributions
CRT: Conceptualization, Data curation, Formal Analysis, Funding acquisition, Investigation, Methodology, Project administration, Resources, Supervision, Validation, Visualization, Writing – original draft, Writing – review & editing. GED: Conceptualization, Data curation, Formal Analysis, Investigation, Methodology, Supervision, Visualization, Writing – review & editing. LD: Formal Analysis, Investigation, Writing – review & editing.
Funding
The author(s) declare financial support was received for the research, authorship, and/or publication of this article. The study was funded by ISCA Diagnostics Ltd. (Grant: A pan-Mucorales Lateral-Flow Device). The funder had no role in the study design, data collection and analysis, decision to publish, or preparation of the manuscript.
Conflict of interest
CRT is a Director of ISCA Diagnostics Ltd. GED is an employee of ISCA Diagnostics Ltd.
The remaining author declares that the research was conducted in the absence of any commercial or financial relationships that could be construed as a potential conflict of interest.
Publisher’s note
All claims expressed in this article are solely those of the authors and do not necessarily represent those of their affiliated organizations, or those of the publisher, the editors and the reviewers. Any product that may be evaluated in this article, or claim that may be made by its manufacturer, is not guaranteed or endorsed by the publisher.
Supplementary material
The Supplementary Material for this article can be found online at: https://www.frontiersin.org/articles/10.3389/fcimb.2023.1305662/full#supplementary-material
Supplementary Figure 1 | Western blots of culture filtrates from non-Mucorales yeasts and molds of clinical importance showing the presence of (A) Aspergillus extracellular antigens reactive with the Aspergillus-specific mAb JF5 (Thornton, 2008), (B) Scedosporium extracellular antigens reactive with the Scedosporium-specific mAb HG12 (Thornton, 2009), (C) Candida albicans extracellular antigens reactive with the Candida-specific mAb MC3 (Morad et al., 2018), (D) Fusarium extracellular antigens reactive with the Fusarium-specific mAb ED7 (Al-Maqtoofi and Thornton, 2016), and (E) Cryptococcus neoformans capsular polysaccharide reactive with the anti-glucuronoxylomannan (GXM) mAb 18B7.
References
Al-Maqtoofi, M., Thornton, C. R. (2016). Detection of human pathogenic Fusarium species in hospital and communal sink biofilms by using a highly specific monoclonal antibody. Environ. Microbiol. 18, 3620–3634. doi: 10.1111/1462-2920.13233
Álvarez, E., Cano, J., Stchigel, A. M., Sutton, D. A., Fothergill, A. W., Salas, V., et al. (2011). Two new species of Mucor from clinical samples. Med. Mycol. 49, 62–72. doi: 10.3109/13693786.2010.499521
Álvarez, E., Sutton, D. A., Cano, J., Fothergill, W. W., Stchigel, A., Rinaldi, M. G., et al. (2009). The spectrum of zygomycete species identified in clinically significant specimens in the United States. J. Clin. Microbiol. 47, 1650–1656. doi: 10.1128/JCM.00036-09
Andryukov, B. G. (2020). Six decades of lateral flow immunoassay: from determining metabolic markers to diagnosing COVID-19. AIMS Microbiol. 6, 280–304. doi: 10.3934/microbiol.2020018
Anita, A., Kumar, S., Kumari, N., Rajpal, K., Kumar, S., Singh, R.K. (2021). A case series of mucormycosis and aspergillus coinfection in post-COVID-19 patient with uncontrolled diabetic. Indian Journal of Case Reports. 7, 420–423. doi: 10.32677/ijcr.v7i10.3054
Anstead, G. M., Sutton, D. A., Thompson, E. H., Weitzman, I., Otto, R. A., Ahuja, S. K. (1999). Disseminated zygomycosis due to Rhizopus schipperae after heatstroke. J. Clin. Microbiol. 37, 2656–2662. doi: 10.1128/JCM.37.8.2656-2662.1999
Arndt, S., Aschendorff, A., Echternach, M., Daemmrich, T. D., Maier, W. (2009). Rhino-orbital-cerebral mucoromycosis and aspergillosis: differential diagnosis and treatment. Eur. Arch. Otorhinolaryngol. 266, 71–76. doi: 10.1007/s00405-008-0692-y
Bellanger, A.-P., Navellou, J.-C., Lepiller, Q., Brion, A., Brunel, A.-S., Millon., L., et al. (2021). Mixed mold infection with Aspergillus fumigatus and Rhizopus microsporus in a severe acute respiratory syndrome Coronavirus 2 (SARS-CoV-2) patient. Infect. Dis. Now 51, 633–635. doi: 10.1016/j.idnow.2021.01.010
Benhadid-Brahmi, Y., Hamane, S., Soyer, B., Mebazaa, A., Alanio, A., Chousterman, B., et al. (2022). COVID-19-associated mixed mold infections: a case report of aspergillosis and mucormycosis and a literature review. J. Med. Mycol. 32, 101231. doi: 10.1016/j.mycmed.2021.101231
Bergantim, R., Rios, E., Trigo, F., Guimarães, J. E. (2013). Invasive coinfection with Aspergillus and Mucor in a patient with acute myeloid leukemia. Clin. Drug Investig. 33, S51–S55. doi: 10.1007/s40261-012-0022-4
Borman, A., Johnson, E. M. (2023). Name changes for fungi of medical importance 2020 to 2021. J. Clin. Microbiol 61, e00330-22. doi: 10.1128/jcm.00330-22
Buil, J. B., van Zanten, A. R. H., Bentvelsen, R. G., Rijpstra, T. A., Goorhuis, B., van der Voort, S., et al. (2021). Case series of four secondary mucormycosis infections in COVID-19 patients, the Netherlands, December 2020 to May 2021. Euro Surveill. 26, 2100510. doi: 10.2807/1560-7917.ES.2021.26.23.2100510
Burnham-Marusich, A. R., Hubbard, B., Kvam, A. J., Gates-Hollingsworth, M., Green, H. R., Soukup, E., et al. (2018). Conservation of mannan synthesis in fungi of the zygomycota and ascomycota reveals a broad diagnostic target. mSphere 3, e00094–e00018. doi: 10.1128/mSphere.00094-18
Chander, J., Kaur, M., Singla, N., Punia, R. P. S., Singhal, S. K., Attri, A. K., et al. (2018). Mucoromycosis: battle with the deadly enemy over a five-year period in India. J. Fungi 4, 46. doi: 10.3390/jof4020046
Chander, J., Singla, N., Kaur, M., Punia, R. S., Attri, A., Alastruey-Izquierdo, A., et al. (2017). Saksenaea erythrospora, an emerging mucoralean fungus causing severe necrotizing skin and soft tissue infections – a study from a tertiary care hospital in north India. Infect. Dis. 49, 170–177. doi: 10.1080/23744235.2016.1239027
Chander, J., Stchigel, A. M., Alastruey-Izquierdo, A., Jayant, M., Bala, K., Rani, H., et al. (2015). Fungal necrotizing fasciitis, an emerging infectious disease caused by Apophysomyces (Mucorales). Rev. Iberoam Micol. 32, 93–98. doi: 10.1016/j.riam.2014.06.005
Corzo-León, D. E., Chora-Hernández, L. D., Rodríguez-Zulueta, A. P., Walsh, T. J. (2018). Diabetes mellitus as the major risk factor for mucoromycosis in Mexico: epidemiology, diagnosis, and outcomes of reported cases. Med. Mycol 56, 29–43. doi: 10.1093/mmy/myx017
Davies, G. E., Thornton, C. R. (2022). Development of a monoclonal antibody and a serodiagnostic lateral-flow device specific to Rhizopus arrhizus (syn. R. oryzae), the principal global agent of mucoromycosis in humans. J. Fungi 8, 756. doi: 10.3390/jof8070756
De Almeida Júnior, J. N., Ibrahim, K. Y., Del Negro, G. M. B., Bezerra, E. D., Duarte Neto, A. N., Batista, M. V., et al. (2015). Rhizopus arrhizus and Fusarium solani concomitant infection in an immunocompromised host. Mycopathologia 181, 125–129. doi: 10.1007/s11046-015-9936-8
Deja, M., Wolf, S., Weber-Carstens, S., Lehmann, T.-N., Adler, A., Ruhnke, M., et al. (2006). Gastrointestinal zygomycosis caused by Mucor indicus in a patient with acute traumatic brain injury. Med. Mycol. 44, 683–687. doi: 10.1080/13693780600803888
Di Nardo, F., Chiarello, M., Cavalera, S., Baggiani, C., Anfossi, L. (2021). Ten years of lateral flow immunoassay technique applications: trends, challenges and future perspectives. Sensors 21, 5185. doi: 10.3390/s21155185
Erami, M., Mirhendi, H., Momen-Heravi, M., Sharif, A., Hashemi Hezaveh, S. J., Matini, A. H., et al. (2023). Case report: COVID-19-associated mucoromycosis co-infection with Lomentospora prolificans: the first case and review on multiple co-infections during COVID-19 pandemic. Front. Med. 10, 1078970. doi: 10.3389/fmed.2023.1078970
Ganesan, A., Shaikh, F., Bradley, W., Blyth, D. M., Bennett, D., Petfield, J. L., et al. (2019). Classification of trauma-associated invasive fungal infections to support wound treatment decisions. Emerg. Infect. Dis. 25, 1639–1647. doi: 10.3201/eid2509.190168
Gomes, M. Z. R., Lewis, R. E., Kontoyiannis, D. P. (2011). Mucoromycosis caused by unusual mucormycetes, non-Rhizopus, -Mucor, and Lichtheimia species. Clin. Microbiol. Rev. 24, 411–445. doi: 10.1128/CMR.00056-10
Gupta, P., Kaur, H., Rompally, S., Muthu, V., Kollabathula, A., Sharma, A., et al. (2023). Disseminated cryptococcosis and pulmonary mucoromycosis: dealing with dual mycoses in a renal transplant patient. Mycopathologia 188, 163–167. doi: 10.1007/s11046-022-00700-2
Henn, A., Mellon, G., Henry, B., Roos-Weil, D., Jauréguiberry, S., Mordant, P., et al. (2014). Disseminated cryptococcosis, invasive aspergillosis, and mucoromycosis in a patient treated with alemtuzumab for chronic lymphocytic leukaemia. Scand. J. Infect. Dis. 46, 231–234. doi: 10.3109/00365548.2013.866269
Hoenigl, M., Seidel, D., Carvalho, A., Rudramurthy, S. M., Arastehfar, A., Gangneux, J.-P., et al. (2022). The emergence of COVID-19 associated mucoromycosis: a review of cases from 18 countries. Lancet Microbe 3, e543–e552. doi: 10.1016/S2666-5247(21)00237-8
Ibrahim, A. S., Spellberg, B., Walsh, T. J., Kontoyiannis, D. P. (2012). Pathogenesis of mucoromycosis. Clin. Infect. Dis. 54, S16–S22. doi: 10.1093/cid/cir865
Irshad, M., Nasir, N., Hashmi, U. H., Farooqi, J., Faisal, S. (2020). Invasive pulmonary infection by Synephalastrum species: two case reports and review of literature. IDCases 21, e00913. doi: 10.1016/j.idcr.2020.e00913
Jawanda, M. K., Narula, R., Gupta, S., Sharma, V., Sidhu, S. K., Kaur, N. (2022). Mixed infections (Mucormycosis, Actinomycosis and Candidiasis) leading to maxillary osteomyelitis in a diabetic mellitus patient in post COVID phase: First case report. Acta Med. 64, 218–223. doi: 10.14712/18059694.2022.5
Jensen, H. E., Aalbæk, B., Lind, P., Krogh, H. V. (1996). Immunohistochemical diagnosis of systemic bovine zygomycosis by murine monoclonal antibodies. Vet. Pathol. 33, 176–183. doi: 10.1177/030098589603300207
Jeong, W., Keighley, C., Wolfe, R., Lee, W. L., Slavin, M. A., Kong, D. C. M., et al. (2019). The epidemiology and clinical manifestations of mucoromycosis: a systematic review and meta-analysis of case reports. Clin. Microbiol. Infect. 25, 26–34. doi: 10.1016/j.cmi.2018.07.011
John, T. M., Jacob, C. N., Kontoyiannis, D. P. (2021). When uncontrolled diabetes mellitus and severe COVID-19 converge: the perfect storm for mucoromycosis. J. Fungi 7, 298. doi: 10.3390/jof7040298
Johnson, A. K., Ghazarian, Z., Cendrowski, K. D., Persichino, J. G. (2021). Pulmonary aspergillosis and mucoromycosis in a patient with COVID-19. Med. Mycol. Case Rep. 32, 64–67. doi: 10.1016/j.mmcr.2021.03.006
Kanaujia, R., Muthu, V., Singh, S., Rudramurthy, S. M., Kaur, H. (2023). Rapidly progressive lung coinfection due to Rhizopus and Scedosporium in a diabetic marijuana smoker. Clin. Microbiol. Infect. 29, 51–53. doi: 10.1016/j.cmi.2022.05.012
Kanwar, A., Jordan, A., Olewiler, S., Wehberg, S., Cortes, M., Jackson, B. R. (2021). A fatal case of Rhizopus azygosporus pneumonia following COVID-19. J. Fungi 7, 174. doi: 10.3390/jof7030174
Khelifa, L., Hu, Y., Jiang, N., Yetisen, A. K. (2022). Lateral flow assays for hormones. Lab. Chip 22, 2451. doi: 10.1039/D1LC00960E
Kokkayil, P., Pandey, M., Agarwal, R., Kale, P., Singh, G., Xess, I. (2017). Rhizopus homothallicus causing invasive infections: series of three cases from a single centre in north India. Mycopathologia 182, 921–926. doi: 10.1007/s11046-017-0153-5
Lackner, N., Posch, W., Lass-Flörl, C. (2021). Microbiological and molecular diagnosis of mucoromycosis: from old to new. Microorganisms 9, 1518. doi: 10.3390/microorganisms9071518
Lamoth, F. (2023). Novel approaches in the management of mucoromycosis. Curr. Fungal Infect. Rep. 17, 98–107. doi: 10.1007/s12281-023-00463-3
Laternier, F., Dannaoui, E., Morizot, G., Elie, C., Garcia-Hermoso, D., Huerre, M., et al. (2012). French Mycosis Study Group. A global analysis of mucoromycosis in France: the RetroZygo study, (2005-2007). Clin. Infect. Dis. 54, S35–S43. doi: 10.1093/cid/cir880
Lu, X.-L., Najafzadeh, M. J., Dolatabadi, S., Ran, Y.-P., Gerrits van den Ende, A. H. G., Shen, Y.-N., et al. (2013). Taxonomy and epidemiology of Mucor irregularis, agent of chronic cutaneous mucoromycosis. Persoonia 30, 48–56. doi: 10.3767/003158513X665539
Marino, A., Calvo, M., Trovato, L., Scalia, G., Gussio, M., Consoli, U. (2023). Mucorales/Fusarium mixed infection in hematologic patient with COVID-19 complications: an unfortunate combination. Pathogens 12, 304. doi: 10.3390/pathogens12020304
Marques, D. S., Vaz, C. P., Branca, R., Campilho, F., Lamelas, C., Afonso, L. P., et al. (2011). Rhizomucor and Scedosporium infection post hematopoietic stem-cell transplant. Case Rep. Med. 2011, 830769. doi: 10.1155/2011/830769
Miller, M. A., Molina, K. C., Gutman, J. A., Scherger, S., Lum, J. M., Mossad, S. B., et al. (2020). Mucoromycosis in hematopoietic cell transplant recipients and in patients with hematological Malignancies in the era of new antifungal agents. Open Forum Infect. Dis. 8, ofaa646. doi: 10.1093/ofid/ofaa646
Mita, A., Hirano, S., Uehara, T., Uehara, K., Ohno, Y., Kubota, K., et al. (2022). Fatal disseminated mucoromycosis due to Cunninghamella bertholletiae infection after ABO-incompatible living donor liver transplantation: a case report. Surg. Case Rep. 8, 164. doi: 10.1186/s40792-022-01516-4
Morad, H. O. J., Wild, A.-M., Wiehr, S., Davies, G., Maurer, A., Pichler, B. J., et al. (2018). Pre-clinical imaging of invasive candidiasis using immunoPET/MR. Front. Microbiol. 9, 1996. doi: 10.3389/fmicb.2018.01996
Moorthy, A., Gaikwad, R., Krishna, S., Hegde, R., Tripathi, K., Kale, P. G., et al. (2021). SARS-CoV-2, uncontrolled diabetes and corticosteroids—an unholy trinity in invasive fungal infections of the maxillofacial region? A retrospective multi-centric analysis. J. Maxillofac. Oral. Surg. 20, 418–425. doi: 10.1007/s12663-021-01532-1
Murray, J., Lu, Z. A., Miller, K., Meadows, A., Totten, M., Zhang, S. X. (2023). Dual disseminated aspergillosis and mucoromycosis diagnosed at autopsy: a case report of two cases of coinfection and a review of the literature. J. Fungi 9, 357. doi: 10.3390/jof9030357
Obradovic-Tomasev, M., Popovic, A., Vuckovic, N., Jovanovic, M. (2014). Mixed fungal infection (Aspergillus, Mucor, and Candida) of severe hand injury. Case Rep. Infect. Dis. 2014, 954186. doi: 10.1155/2014/954186
Orne, C., Burnham-Marusich, A., Baldin, C., Gebremariam, T., Ibrahim, A., Kvam, A., et al. (2018). Cell wall fucomannan is a biomarker for diagnosis of invasive murine mucoromycosis. 28th European Congress of Clinical Microbiology and Infectious Disease (ECCMID) 1. Available online at: https://www.escmid.org/escmid_publications/escmid_elibrary/material/?mid=62799 [Google Scholar]
Osaigbovo, I. I., Bongomin, F. (2021). Point of care tests for invasive fungal infections: a blue print for increasing availability in Africa. Ther. Adv. Infect. Dis. 8, 20499361211034266. doi: 10.1177/20499361211034266
Özbek, L., Topçu, U., Manay, M., Esen, B. H., Bektas, S. N., Aydin, S., et al. (2023). COVID-19-associated mucoromycosis: a systematic review and meta-analysis of 958 cases. Clin. Microbiol. Infect. 29, 722–731. doi: 10.1016/j.cmi.2023.03.008
Pandey, D., Agarwal, M., Chadha, S., Aggarwal, D. (2019). Mixed opportunistic infection with Mucor, Aspergillus and Candida in oculo-rhino-cerebral mycosis: an uncommon case. J. Acad. Clin. Microbiol. 21, 47–49. doi: 10.4103/jacm.jacm_2_19
Paul, M., Sasidharan, J., Taneja, J., Chatterjee, K., Abbas, S. Z., Chowdury, V., et al. (2022). Invasive mucoromycosis and aspergillosis coinfection associated with post-COVID-19 pneumonia in a tertiary care hospital. J. Med. Mycol. 63, 59–64. doi: 10.3314/mmj.21-00019
Petrikkos, G., Skiada, A., Lortholary, O., Roilides, E., Walsh, T. J., Kontoyiannis, D. P. (2012). Epidemiology and clinical manifestations of mucoromycosis. Clin. Infect. Dis. 54, S23–S34. doi: 10.1093/cid/cir866
Pham, D., Howard-Jones, A. R., Sparks, R., Stefani, M., Sivalingham, V., Halliday, C. L., et al. (2023). Epidemiology, modern diagnostics, and the management of mucorales infections. J. Fungi 9, 659. doi: 10.3390/jof9060659
Prakash, H., Chakrabarti, A. (2019). Global epidemiology of mucoromycosis. J. Fungi 5, 26. doi: 10.3390/jof5010026
Prakash, H., Chakrabarti, A. (2021). Epidemiology of mucoromycosis in India. Microorganisms 9, 523. doi: 10.3390/microorganisms9030523
Radotra, B., Challa, S. (2022). Pathogenesis and pathology of COVID-associated mucoromycosis: what is new and why. Curr. Fungal Infect. Rep. 16, 206–220. doi: 10.1007/s12281-022-00443-z
Rahna, P., Chandra, S., Padma, B. P., Ranjini, K. (2022). Mixed infections of rhino-orbital-cerebral mucoromycosis and aspergillosis with culture proven Aspergillus endophthalmitis in post COVID patient – a case report. Indian J. Clin. Exp. Ophthalmol. 8, 163–165. doi: 10.18231/j.ijceo.2022.030
Ramírez-Hinojosa, J. P., Medrano-Ahumada, S., Arenas, R., Bravo-Escobar, A., Paraguirre-Martínez, S., Xicohtencatl-Cortes, J., et al. (2021). Fungal invasive co-infection due to Aspergillus fumigatus and Rhizopus arrhizus: a rhino-orbital presentation. J. Fungi 7, 1096. doi: 10.3390/jof7121096
Roden, M. M., Zaoutis, T. E., Buchanan, W. L., Knudsen, T. A., Sarkisova, T. A., Schaufele, R. L., et al. (2005). Epidemiology and outcome of zygomycosis: a review of 929 reported cases. Clin. Infect. Dis. 41, 634–653. doi: 10.1086/432579
Rodriguez-Morales, A. J., Sah, R., Millan-Oñate, J., Gonzalez, A., Montenegro-Idrogo, J. J., Scherger, S., et al. (2021). COVID-19 associated mucoromycosis: the urgent need to reconsider the indiscriminate use of immunosuppressive drugs. Ther. Adv. Infect. Dis. 8, 1–5. doi: 10.1177/20499361211027065
Rudramurthy, S. M., Hoenigl, M., Meis, J. F., Cornely, O. A., Muthu, V., Gangneux, J. P., et al. (2021). ECMM/ISHAM recommendations for clinical management of COVID-19 associated mucoromycosis in low- and middle-income countries. Mycoses 64, 1028–1037. doi: 10.1111/myc.13335
Sardeshmukh, A. A., Kshirsagar, R. A., Prasannakumar, P., Nair, V. S., Jha, G. K., Warade, P. (2023). Mucoromycosis and aspergillosis infection following maxillofacial trauma: case report. Indian J. Otolaryngol Head Neck Surg. 75, 1245–1249. doi: 10.1007/s12070-023-03492-z
Schober, S., Stanchi, K. M. C., Riecker, A., Pfeiffer, M., Tsiflikas, I., Wiegand, G., et al. (2021). Fulminant Rhizomucor pusillus mucormycosis during anti-leukemic treatment with blinatumomab in a child: a case report and review of the literature. Med. Mycol. Case Rep. 32, 4–9. doi: 10.1016/j.mmcr.2020.12.002
Sen, M., Honavar, S. G., Bansal, R., Sengupta, S., Rao, R., Kim, U., et al. (2021). Epidemiology, clinical profile, management, and outcome of COVID-19-associated rhino-orbital-cerebral mucoromycosis in 2826 patients in India – collaborative OPAI-IJO study on mucoromycosis in COVID-19 (COSMIC), Report 1. Indian J. Ophthalmol. 69, 1670–1692. doi: 10.4103/ijo.IJO_1565_21
Shand, J. M., Albrecht, R. M., Burnett, H. F., Miyake, A. (2004). Invasive fungal infection of the midfacial and orbital complex due to Scedosporium apiospermum and mucoromycosis. J. Oral. Maxillofac. Surg. 62, 231–234. doi: 10.1016/j.joms.2003.04.013
Singh, A., Mohanty, A., Jha, S., Gupta, P., Kaistha, N. (2021). Concomitant mucoromycosis with aspergillosis in patients with uncontrolled diabetes mellitus: a case series. J. Clin. Diagn. Res. 15, DR01–DR03. doi: 10.7860/JCDR/2021/47912.14507
Skiada, A., Pagano, L., Groll, A., Zimmerli, S., Dupont, B., Lagrou, K., et al. (2011). Zygomycosis in Europe: analysis of 230 cases accrued by the registry of the European Confederation of Medical Mycology (ECMM) Working Group on Zygomycosis between 2005 and 2007. Clin. Microbiol. Infect. 17, 1859–1867. doi: 10.1111/j.1469-0691.2010.03456.x
Skiada, A., Pavlaes, I., Drogari-Apiranthitou, M. (2020). Epidemiology and diagnosis of mucoromycosis: an update. J. Fungi 6, 265. doi: 10.3390/jof6040265
Song, Y., Qiao, J., Giovanni, G., Liu, G., Yang, H., Wu, J., et al. (2017). Mucoromycosis in renal transplant recipients: review of 174 reported cases. BMC Infect. Dis. 17, 283. doi: 10.1186/s12879-017-2381-1
Song, Y., Zhou, M., Gong, Q., Guo, J. (2022). Scedosporium apiospermum and Lichtheimia corymbifera co-infection due to inhalation of biogas in immunocompetent patients: a case series. Infect. Drug Resist. 15, 6423–6430. doi: 10.2147/IDR.S388166
Spellberg, B., Edwards, J., Ibrahim, A. (2005). Novel perspectives on mucoromycosis: pathophysiology, presentation, and management. Clin. Microbiol. Rev. 18, 556–569. doi: 10.1128/CMR.18.3.556-569.2005
Suresh, A., Joshi, A., Desai, A. K., Juturu, U., Kurian, D. J., Jain, P., et al. (2022). Covid-19 associated fungal osteomyelitis of jaws and sinuses: an experience driven management protocol. Med. Mycol. 60, myab082. doi: 10.1093/mmy/myab082
Tabarsi, P., Sharifynia, S., Pourabdollah Toutkaboni, M., Abtahian, Z., Rahdar, M., Mirahmadian, A. S., et al. (2022). Coinfection by Aspergillus and Mucoraceae species in two cases of acute rhinosinusitis as a complication of COVID-19. Case Rep. Med. 2022, 1–6. doi: 10.1155/2022/8114388
Tadeo, K. K., Nimwesiga, A., Kwizera, R., Apeduno, L., Martyn, E., Okirwoth, M., et al. (2021). Evaluation of the diagnostic performance of a semiquantitative cryptococcal antigen point-of-care assay among HIV-infected persons with cryptococcal meningitis. J. Clin. Microbiol. 59, e00860–e00821. doi: 10.1128/JCM.00860-21
Teng, P., Han, X., Zhang, S., Wei, D., Wang, Y., Liu, D., et al. (2022). Mixed invasive pulmonary Mucor and Aspergillus infection: a case report and literature review. Chin. Med. J. 135, 854–856. doi: 10.1097/CM9.0000000000001839
Thornton, C. R. (2001). “Immunological methods for fungi,” in Molecular and Cellular Biology of Filamentous Fungi, A Practical Approach. Ed. Talbot, N. J. (Oxford: University Press), 227–256.
Thornton, C. R. (2008). Development of an immunochromatographic lateral-flow device for rapid serodiagnosis of invasive aspergillosis. Clin. Vaccine Immunol. 15, 1095–1105. doi: 10.1128/CVI.00068-08
Thornton, C. R. (2009). Tracking the emerging human pathogen Pseudallescheria boydii by using highly specific monoclonal antibodies. Clin. Vaccine Immunol. 16, 756–764. doi: 10.1128/CVI.00061-09
Thornton, C. R. (2020). Detection of the ‘big five’ mold killers of humans: Aspergillus, Fusarium, Lomentospora, Scedosporium and Mucormycetes. Adv. Appl. Microbiol. 110, 1–61. doi: 10.1016/bs.aambs.2019.10.003
Thornton, C. R. (2023). The potential for rapid antigen testing for mucoromycosis in the context of COVID-19. Expert Rev. Mol. Diagn. doi: 10.1080/14737159.2023.2233906
Tully, C. C., Romanelli, A. M., Sutton, D. A., Wickes, B. L., Hospenthal, D. R. (2009). Fatal Actinomucor elegans var. Kuwaitensis infection following combat trauma. J. Clin. Microbiol. 47, 3394–3399. doi: 10.1128/JCM.00797-09
Walther, G., Wagner, L., Kurzai, O. (2019). Updates on the taxonomy of Mucorales with an emphasis on clinically important taxa. J. Fungi 5, 106. doi: 10.3390/jof5040106
WHO (2022). WHO fungal priority pathogens list to guide research, development and public health action (Geneva: World Health Organization). License: CC BY-NC-SA 3.0 IGO.
Yang, N., Zhang, L., Feng, S. (2023). Clinical features and treatment progress of invasive mucoromycosis in patients with hematological Malignancies. J. Fungi 9, 592. doi: 10.3390/jof9050592
Zaki, S. M., Elkholy, I. M., Elkady, N. A., Abdel-Ghany, K. (2014). Mucoromycosis in Cairo, Egypt: review of 10 reported cases. Med. Mycol. 52, 73–80. doi: 10.3109/13693786.2013.809629
Zayet, S., Zaghdoudi, A., Ammari, L., Kilani, B., Benaissa, H. T. (2021). Cerebro-rhino-orbital mucormycosis and aspergillosis coinfection in a patient with diabetes mellitus: a case report. IDCases. 23, e01022. doi: 10.1016/j.idcr.2020.e01022
Keywords: Mucorales, mucoromycosis, monoclonal antibody, biomarker, lateral-flow test
Citation: Thornton CR, Davies GE and Dougherty L (2023) Development of a monoclonal antibody and a lateral-flow device for the rapid detection of a Mucorales-specific biomarker. Front. Cell. Infect. Microbiol. 13:1305662. doi: 10.3389/fcimb.2023.1305662
Received: 02 October 2023; Accepted: 20 November 2023;
Published: 08 December 2023.
Edited by:
Georgios Chamilos, University of Crete, GreeceReviewed by:
Fazal Shirazi, Pfizer, United StatesShakti Singh, University of California, Los Angeles, United States
Copyright © 2023 Thornton, Davies and Dougherty. This is an open-access article distributed under the terms of the Creative Commons Attribution License (CC BY). The use, distribution or reproduction in other forums is permitted, provided the original author(s) and the copyright owner(s) are credited and that the original publication in this journal is cited, in accordance with accepted academic practice. No use, distribution or reproduction is permitted which does not comply with these terms.
*Correspondence: Christopher R. Thornton, Yy5yLnRob3JudG9uQGV4ZXRlci5hYy51aw==
†These authors have contributed equally to this work