- 1Department of Science and Education Division, Public Health Clinical Center of Chengdu, Chengdu, Sichuan, China
- 2Department of Clinical Laboratory Medicine, Chengdu Women’s and Children’s Central Hospital, School of Medicine, University of Electronic Science and Technology of China, Chengdu, China
Objectives: This study summarizes the epidemiological characteristics, species distribution, and drug sensitivity of clinical nontuberculous mycobacteria (NTM) isolates at the Public Health Clinical Center of Chengdu, China, from January 2017 to December 2022.
Methods: We retrospectively analyzed data from patients with clinically isolated NTM strains. Chi-square analysis assessed the rate of Mycobacterium strain isolation over 6 years.
Results: The number of samples tested for Mycobacterium tuberculosis (MTB) and/or NTM increased each year, while MTB detection decreased and NTM detection rose significantly each year (P=0.03). The average age of NTM patients was 51 ± 17.53 years, with a 14.1% HIV infection rate. The predominant isolates were Mycobacterium avium-intracellulare (MAC) and M. chelonae/M. abscessus, with 96.4% of cases being of Han ethnicity. Amikacin, moxifloxacin, and clarithromycin were effective against M. avium and M. intracellulare; linezolid, amikacin, and cefoxitin were effective against M. chelonae/M. abscessus. Over 90% of NTM cases originated from the respiratory tract.
Conclusion: The NTM isolation rate in Southwest China has risen in recent years, primarily among elderly patients with a high HIV co-infection rate. The main NTM isolates were MAC and M. chelonae/M. abscessus. Amikacin, moxifloxacin, clarithromycin, and linezolid exhibited strong antibacterial activity against SGM, while amikacin and linezolid displayed relatively better antibacterial activity against RGM. The prevalence of NTM infection may be positively associated with regional economic development and health conditions.
Introduction
Nontuberculous mycobacteria (NTM) are part of the Mycobacterium species, distinct from those in the M. tuberculosis complex or M. leprae, and typically act as opportunistic pathogens (Falkinham, 1996; Falkinham, 2002). This term encompasses around 200 distinct Mycobacterium species, with new species continually emerging (Zhou et al., 2020). In recent years, both in China and globally, NTM infections have been on the rise (Wang et al., 2019; Liu et al., 2021; Thornton et al., 2021). Among clinical laboratories in China, the Mycobacterium avium-intracellulare complex (MAC) and the M. chelonae/M. abscessus complex are the two most commonly encountered NTM complexes, and they are among the most drug-resistant species (Wang et al., 2016; Wang et al., 2019; Guo et al., 2021). However, limited studies have explored NTM drug susceptibility due to small sample sizes or restricted antibiotic types being tested. Therefore, this study aims to summarize NTM identification and drug sensitivity data from the largest sample size in a major central city in Southwest China. This information can provide valuable insights for the prevention and treatment of NTM diseases.
Methods
Study population and diagnostic criteria
This study included all patients with culture-positive NTM infections treated at the Public Health Clinical Center of Chengdu (PHCC) in Sichuan Province, China, from January 2017 to December 2022. During the 6-year study period, a total of 126,368 suspected mycobacterial infections were cultured using the BACTEC™ MGIT 960 System (Becton Dickinson & Co., NJ, USA), resulting in 26,510 Mycobacterium tuberculosis (MTB) culture-positive cases and 587 NTM culture-positive cases. Diagnosis and categorization of NTM-infected patients were based on the 2012 and 2020 NTM Diagnosis and Treatment Expert Consensus (Editorial Board of CSTB, 2016; Tuberculosis Branch of Chinese Medical Association, 2020), the Clinical Diagnosis and Treatment Guidelines for Tuberculosis in China (Chinese Medical Association, 2005), and the updated guidelines from the World Health Organization. Diagnosis of human immunodeficiency virus (HIV) followed the Chinese HIV and HIV Infection Diagnostic Criteria (WS293–2008) (From the Centers for Disease Control and prevention, 1993).
Bacterial strains culture, identification, and drug sensitivity
We employed the BACTEC™ MGIT 960 System for culturing mycobacteria. Extrapulmonary samples (such as pleural fluid, spinal fluid, and lymph nodes) were obtained through lumbar puncture, pleural tap, fine needle aspiration, lymph node biopsy, and other procedures (Wang et al., 2017). Initial identification of NTM bacteria primarily relied on the MPT 64 antigen detection (Colloidal Gold immunochromatography) or polymerase chain reaction (PCR) methods. Subsequently, P-nitrobenzoic acid (PNB) and thiophene-2-carboxylic acid hydrazide (TCH) were employed for NTM revalidation using the MicroDST ™ (Yinke AUTOBIO Diagnostics Co., Ltd, Zhuhai, China) approach (Cao et al., 2021). Further identification of NTM species/complexes was conducted using Genechip, following the manufacturer’s instructions (CapitalBio Corp., Chengdu, China). Strains that couldn’t be identified via genechip were subjected to analysis using matrix-assisted laser desorption/ionization time-of-flight mass spectrometry (Microflex LT; Bruker Daltonics, Bremen, Germany) or 16S rDNA sequencing.
The Clinical and Laboratory Standards Institute (CLSI) recommends the microplate dilution method for in vitro drug sensitivity testing of some Slow-Growing Mycobacteria (SGMs) and Rapid-Growing Mycobacteria (RGMs). However, the exact Minimum Inhibitory Concentration (MIC) values for the strains require comprehensive evaluation and adjustment for clinical practice (Clinical and Laboratory Standards Institute (CLSI), [[NoYear]]). In this study, drug resistance tests (DST) for culture-positive NTM isolates were conducted using MicroDST ™ (Yinke AUTOBIO Diagnostics Co., Ltd, Zhuhai, China). The MIC was defined as the lowest drug concentration inhibiting visible growth of the tested isolates. MIC breakpoints and sensitivity/resistance determinations were interpreted following reagent instructions, and the protocol was executed in accordance with the manufacturer’s recommendations (Wang et al., 2020). We employed a total of 13 antimicrobial agents in this study, including rifampicin (1, 4, 6, 16 μg/mL), clarithromycin (0.5, 4, 16, 64 μg/mL), imipenem/cilastatin (IPM/CS) (0.5, 4, 16, 64 μg/mL), linezolid (0.5, 2, 8, 32 μg/mL), amikacin (1, 4, 16, 64 μg/mL), ethambutol (2.5, 5, 10, 20 μg/mL), and rifabutin (0.5, 2, 8, 32–10 μg/mL), at four concentrations. Cefoxitin (4, 16, 32, 40, 64, 80, 128, 160 μg/mL), tobramycin (0.5, 1, 2, 4, 8, 16, 32, 64 μg/mL), moxifloxacin (0.125, 0.25, 0.5, 1, 2, 4, 8, 16 μg/mL), doxycycline (0.5, 1, 4, 8, 16, 32, 64, 128 μg/mL), minocycline (0.5, 1, 4, 8, 16, 32, 64, 128 μg/mL), and sulfamethoxazole (8, 16, 32, 64, 80, 128, 160, 256 μg/mL) were employed at eight concentrations. Bold and italicized values in the aforementioned drug concentrations represent the resistance breakpoint for each drug. For NTM from the same case, site, and type, the DST results from the initial culture were considered. Monitoring was conducted using control strains H37Rv (ATCC 25618) and M. smegmatis (CGMCC 1.2621).
Laboratory quality control
External quality assessment (EQA) was carried out for smear, culture, and DST at the Innovation Alliance for TB Diagnosis and Treatment in Beijing, China. Additionally, a blinded retesting of approximately 10% of isolates from the study laboratory was conducted by a specialized Centers for Disease Control and Prevention.
Statistical analysis
Data were analyzed using SPSS Statistics Client 19.0 (SPSS Inc., IL, USA). Normally distributed measurement data were presented as means, while categorical variables were expressed as numbers and percentages. Chi-square (χ2) analysis was employed to assess variations in the Mycobacterium strain rate, age, and sex ratio over five years. Statistical significance was set at P < 0.05.
Ethics approval and consent to participate
This study received approval from the Ethics Committee of PHCC (Approval No. 2017Y025). All patient information used in this study was routinely collected through the mandatory notification system. The requirement for informed consent was waived by the ethics committee.
Results
Demographic and clinical characteristics
From January 2017 to December 2022, a total of 126,368 non-repeated clinical specimens with suspected mycobacterial infections were cultured at PHCC. Among these specimens, 26,510 (21.0%) tested positive in culture. MTB was detected in 25,923 (97.8%) positive samples, while NTM was found in 587 (2.2%) samples. Among all NTM cases, 13 were co-infected with both MTB and NTM (Figure 1; Table 1). Over the 6-year study period, a significant increase was observed in the number of samples tested for MTB and/or NTM each year, corresponding to a significant annual rise in NTM detection (χ2 = 18.01, P=0.03; Figure 1). The mean age of the 587 NTM patients was 51 ± 17.53 years (range: 13–88 years). Within this subgroup, 349/587 (59.5%) were males, and 238/587 (40.5%) were females, with a male-to-female ratio of 1.5. There was no significant difference in the male-to-female ratio over the 6-year period (χ2 = 0.71, P>0.05; Figure 2). The majority of NTM-infected cases were middle-aged and elderly patients, and the proportion of each age group showed no significant difference over the 6 years (χ2 = 11.16, P>0.05; as shown in Figure 2; Table 1).
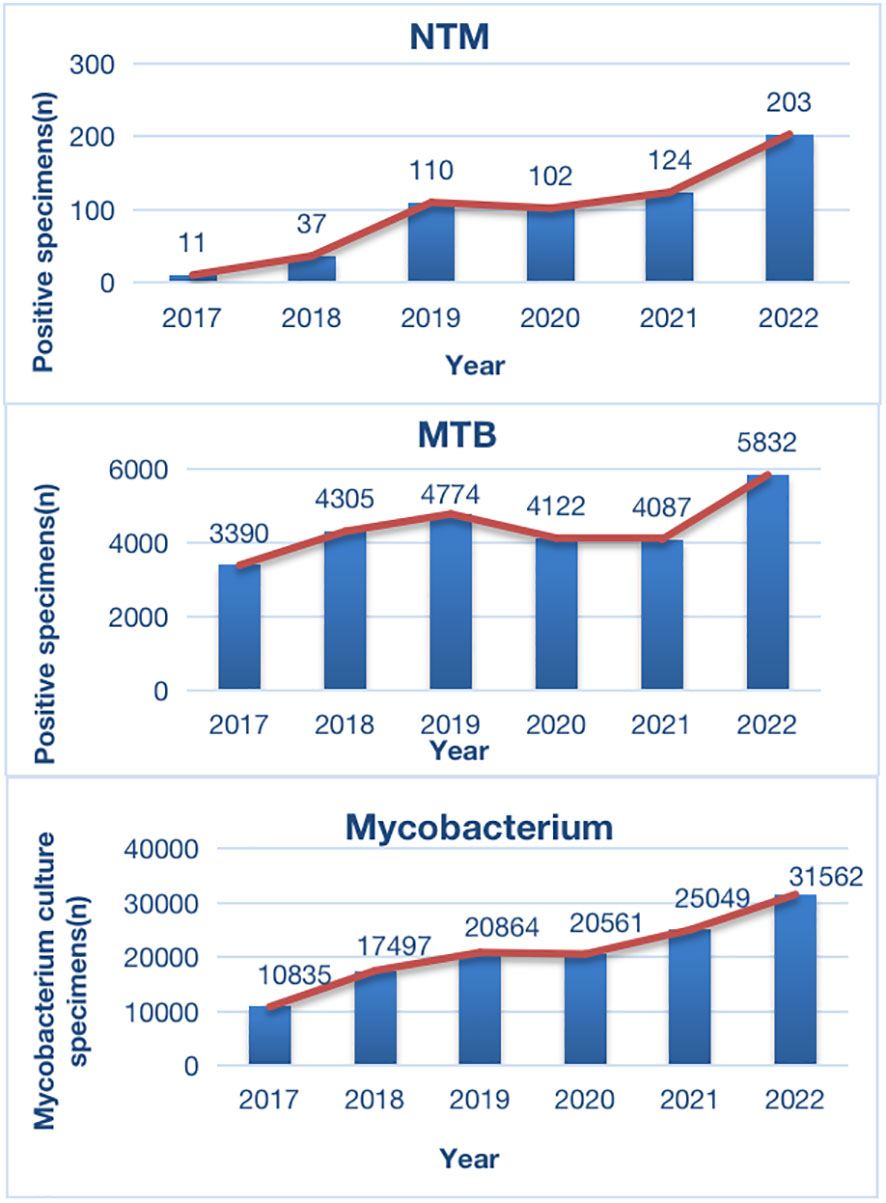
Figure 1 Distribution of Positive Specimens in Mycobacterium Culture During 2017-2021. MTB, Mycobacterium tuberculosis; NTM, non-tuberculous mycobacteria.
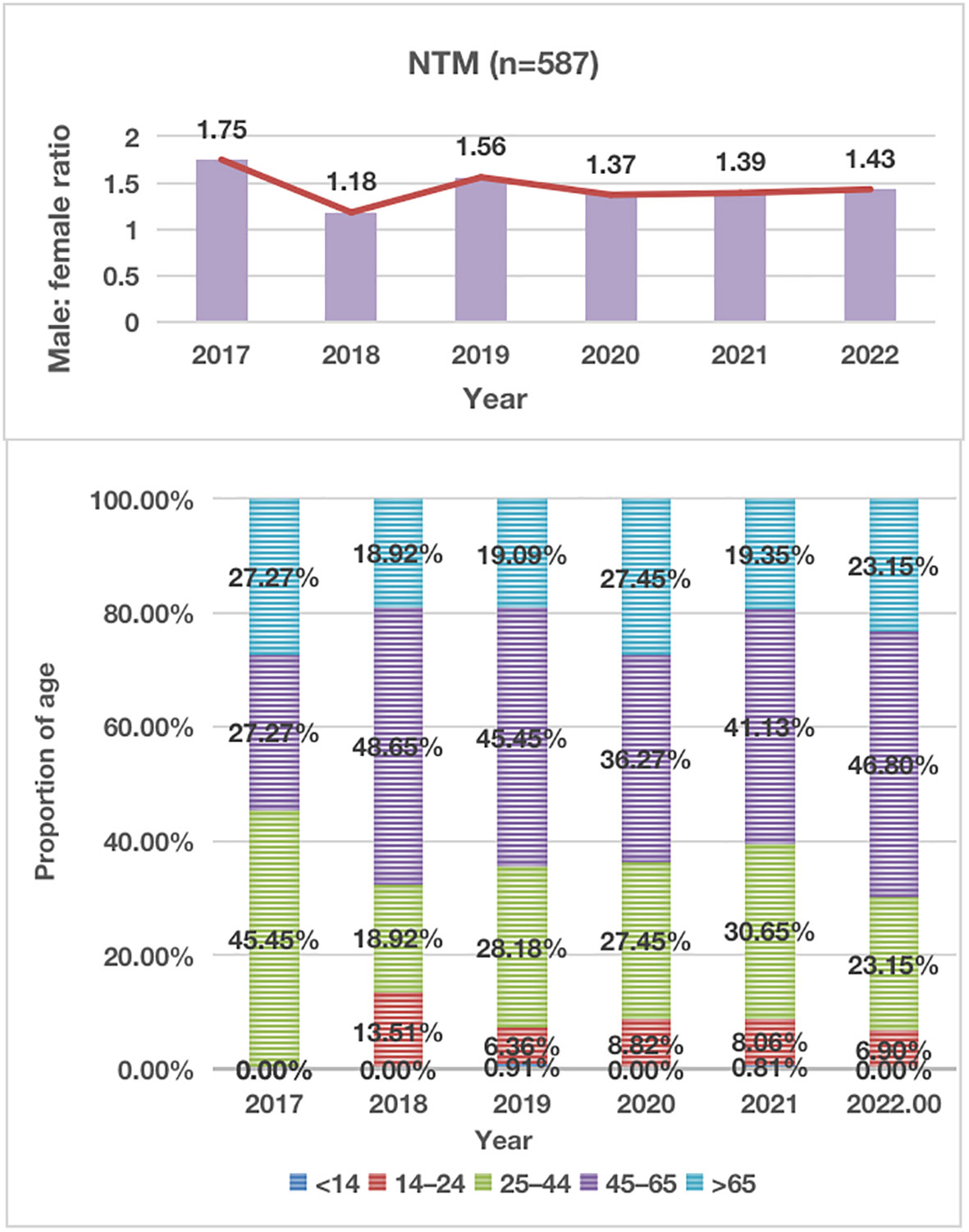
Figure 2 Distribution of Male-to-Female Ratio and Proportion of Age in NTM Patients During 2017-2021. NTM, nontuberculous mycobacteria.
Twenty-one (3.6%) patients belonged to ethnic minorities. Among the 587 patients, 93 (15.8%) had at least one co-infectious disease: HIV infection in 83 (14.1%), diabetes mellitus in 29 (4.9%), hepatitis B virus in 12 (2.0%), and hypertension in 9 (1.5%), syphilis in 3 (0.5%), and hepatitis E virus in 1 (0.2%). Additionally, 99 (16.8%) patients experienced at least one adverse drug reaction: liver dysfunction in 82 (14.0%), hyperuricemia in 24 (4.1%), leucopenia in 19 (3.2%), thrombocytopenia in 8 (1.4%), and drug eruption in 7 (1.2%) patients.
NTM species identification
The DNA microarray chip identified NTM species as follows: M. avium in 154 (26.2%), M. chelonae/M. abscessus in 151 (25.7%), M. intracellulare in 145 (24.7%), M. kansasii in 37 (6.3%), M. fortuitum in 19 (3.2%), M. scrofulaceum in 15 (2.6%), M. gordonae in 13 (2.2%), M. lentiflavum in 10 (1.7%), and mixed infections of MTB and NTM in 13 (2.2%). Additionally, 30 specimens initially identified as other Mycobacterium spp. by the Genechip (CapitalBio Corporation) included M. szulgai, M. malmoense, M. terrae, M. peregrinum, M. margueri, M. phlei, M. septicum, M. marseillense, M. shigaense, M. xenopi, M. simiae, and M. lentil, identified through 16S rDNA sequencing or matrix-assisted laser desorption/ionization time-of-flight mass spectrometry systems. Moreover, a significant rise in NTM detection was observed from 2017 to 2022 (Table 2; Figure 3).
Drug resistance of the NTM species
Out of the 587 patients with NTM disease, antimicrobial susceptibility testing was conducted for 113, as depicted in Table 3. Among the Slowly Growing Mycobacteria (SGM), amikacin (high-level resistance ≥ 64 μg/mL) demonstrated the highest activity against M. avium, with a resistance rate of 2/29 (6.9%). Moxifloxacin (high-level resistance ≥ 8 μg/mL) exhibited the highest activity against M. intracellulare, with a resistance rate of 2/27 (7.4%). Clarithromycin (high-level resistance ≥ 16 μg/mL) displayed effective antibacterial effects on both M. avium and M. intracellulare, with drug resistance rates of 5/29 (17.2%) and 6/27 (22.2%), respectively. In this study, all six strains of M. kansasii were completely sensitive to clarithromycin, linezolid (high-level resistance ≥ 32 μg/mL), amikacin, and moxifloxacin. Eight strains of M. gordonae showed complete sensitivity to rifampicin (high-level resistance ≥ 4 μg/mL), clarithromycin, linezolid, amikacin, cefoxitin (high-level resistance ≥ 80 μg/mL), and moxifloxacin. Linezolid, amikacin, tobramycin (high-level resistance ≥ 16 μg/mL), and moxifloxacin also exhibited strong antibacterial activity against M. scrofulaceum.
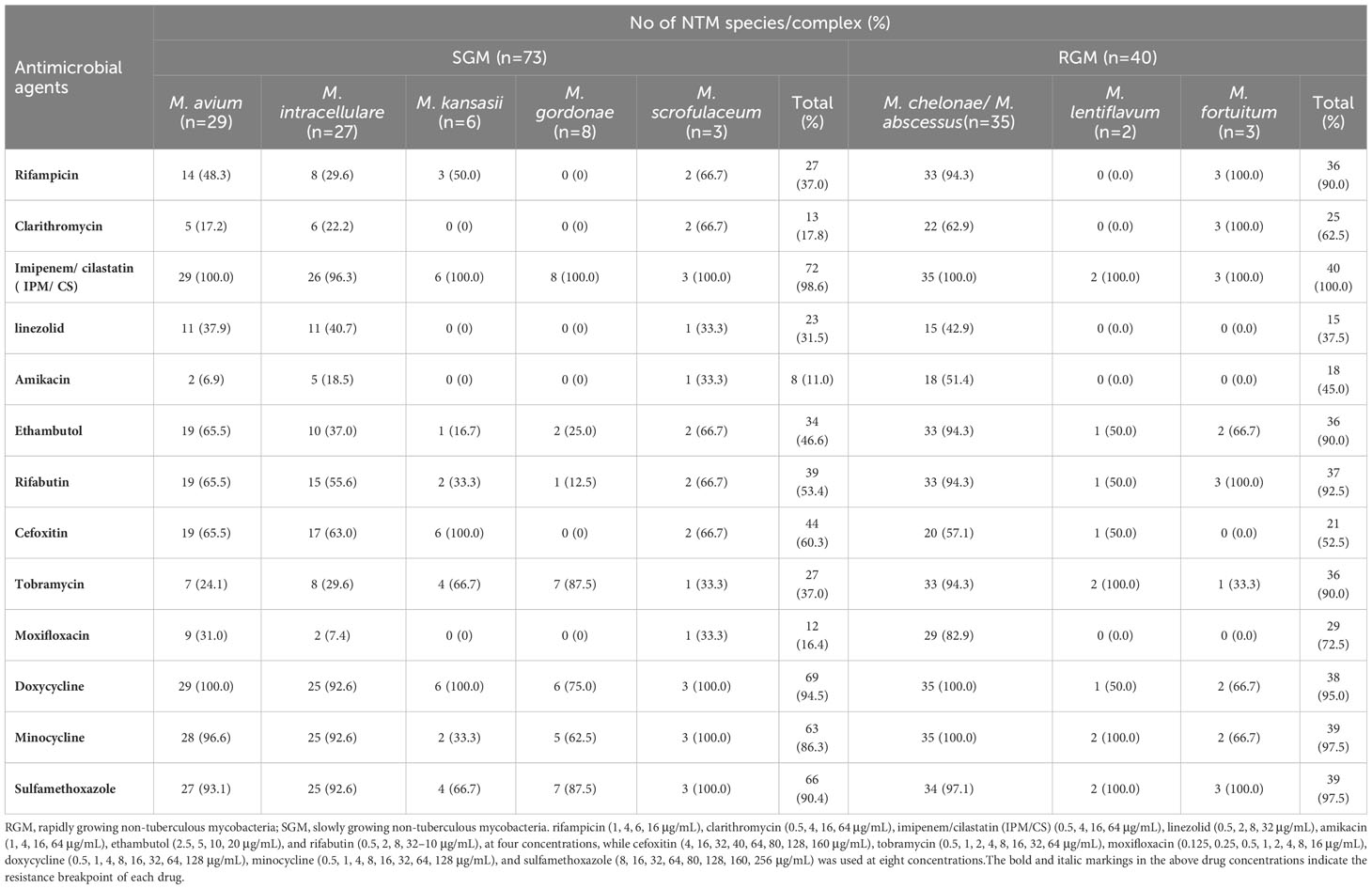
Table 3 Number of Nontuberculous Mycobacteria clinical strains resistant to drugs in vitro experiments.
Regarding the Rapidly Growing Mycobacteria (RGM), linezolid, amikacin, and cefoxitin were the most effective agents against M. chelonae/M. abscessus. Rifampicin, clarithromycin, linezolid, amikacin, and moxifloxacin showed complete sensitivity in two strains of M. lentiflavum, while linezolid, amikacin, cefoxitin, and moxifloxacin demonstrated complete sensitivity in three strains of M. fortuitum.
NTM species distribution from different specimen types
Among the 113 NTM strains tested for drug sensitivity in this study, over 90% were isolated from respiratory tract samples [sputum 90/113 (79.6%), bronchoalveolar lavage fluid 12/113 (10.6%)]. Cerebrospinal fluid contributed 7/113 (6.2%) samples, while lymph nodes, the digestive tract, and pleural effusion each provided 2/113 (1.8%), 1/113 (0.9%), and 1/113 (0.9%) samples, respectively. The primary NTM strains isolated from these six tissue sources were M. chelonae/M. abscessus and MAC.
Discussion
According to recent reports from China and other countries, there has been a gradual increase in the incidence of NTM infection and laboratory isolation rates (Wang et al., 2016; Wang et al., 2019; Guo et al., 2021; Liu et al., 2021; Thornton et al., 2021). NTMs are inherently resistant to many anti-TB drugs (ATDs), and treatment plans depend on factors such as the NTM species, infection site, and the severity of the infection (Lee et al., 2015; Daley et al., 2020; Gopalaswamy et al., 2020). The prevalence of NTM strains varies among different regions and populations. For instance, the most common NTM isolates reported in various countries include M. kansasii and MAC in Poland (Przybylski et al., 2023), MAC and M. gordonae in Italy (Giannoni et al., 2023), M. fortuitum, and M. simiae in Iran (Tarashi et al., 2023), and MAC, M. abscessus, and M. kansasii in Turkey (Babalik et al., 2023). In Switzerland, common NTM isolates include M. avium and M. gordonae (Vongthilath-Moeung et al., 2022). However, information regarding NTM isolates and drug resistance profiles in southwest China has been scarce. Therefore, accurately understanding the NTM epidemic and drug resistance situation is crucial for early differential diagnosis and treatment of TB and NTM diseases.
In southwest China, there has been limited data on NTM infections. Our preliminary study discussed only a small sample of NTM-infected individuals in Southwest China, while a few studies have reported on large samples of clinically NTM-infected individuals and the dynamic sensitivity of NTM to multiple antibiotics in China and worldwide (Jeong et al., 2017; Zhou et al., 2020; Maya et al., 2022). In this study, we conducted a systematic analysis of NTM clinical infection cases in Southwest China over the past six years. The results indicated a rising trend in the number of patients visiting PHCC for mycobacteria culture evaluation each year from 2017 to 2022. This trend may be attributed to increased public awareness of healthcare in recent years and the expanding reach of PHCC in Southwest China. However, in 2020, there was a slight decline in this trend, likely due to the initial COVID-19 pandemic control measures, which resulted in reduced public mobility and hospital visits. Nevertheless, the trend resumed its upward trajectory after 2021. Moreover, among mycobacteria-positive cultures, the count of MTB cases increased from 2017 to 2019 and then gradually decreased until 2020. This trend is consistent with the recent control measures for TB, which have led to decreasing incidence and mortality rates each year (World Health Organization, 2021). Notably, the number of isolated clinical NTM cases showed a continuous upward trend from 2017 to 2022, rising from 11 cases in 2017 to 203 cases in 2022. This finding aligns with reports of increasing NTM infection cases worldwide and the observation that laboratory isolation rates have been on the rise each year (López-Roa et al., 2020).
In this study, NTM infections were primarily observed in middle-aged and elderly individuals, with those aged over 45 accounting for 65.5% of the cases. This is in contrast to our previous studies, where the majority of MTB infections were in middle-aged and young individuals (Wang et al., 2017; López-Roa et al., 2020; Wang et al., 2020). The co-infection rate of acquired immune deficiency syndrome (AIDS) with NTM cases was 14.1%, slightly higher than the 11.5% reported in our previous study (Wang et al., 2019). This increase may be linked to the rising incidence of AIDS cases in southwest China in recent years (Zhang et al., 2015; Wang et al., 2016; Yang et al., 2018). Additionally, some NTM cases were complicated by varying degrees of diabetes and hypertension, indicating that middle-aged and elderly patients with underlying pulmonary diseases, HIV, and compromised immunity are risk factors for NTM infections.
In our previous study, we observed that MTB infections accounted for a significant proportion of ethnic minorities, particularly among children with TBM in Southwest China (Wang et al., 2020). However, in this study, 96.4% of NTM-infected patients were of Han ethnicity from central cities, with minority groups representing a small subset of the infected individuals. This pattern contrasts with the MTB-infected population model in southwest China, suggesting a positive correlation between NTM infection and the regional level of economic development and sanitation conditions.
Among the 587 NTM cases in this study, the top three isolated strains were M. avium, M. chelonae/M. abscessus, and M. intracellulare, followed by M. kansasii. These clinical strains mirrored the domestic epidemic trend (Jing et al., 2012; Wu et al., 2014; Tan et al., 2018; Liu et al., 2019). Among the 113 cases that underwent in vitro drug sensitivity testing, 90.3% of the strains originated from the respiratory tract, followed by cerebrospinal fluid, lymph nodes, the gastrointestinal tract, and pleural effusion. Intriguingly, MAC and M. chelonae/M. abscessus were the predominant strains in various tissues, followed by M. kansasii, M. fortuitum, M. scrofulaceum, M. gordoniae, and M. lentiflavum.
NTM naturally exhibits resistance to various anti-TB drugs, and its clinical isolation rate is relatively low. Furthermore, the emergence of NTM resistance poses a significant challenge in clinical treatment. Currently, research on NTM drug resistance is limited, with only a few reports on the sensitivity of NTM to various antibiotics in China, often based on small sample sizes or focused on a single antibiotic (Lan et al., 2011; Li et al., 2017). In this study, we conducted comprehensive in vitro sensitivity tests on 113 NTM strains with 13 antibiotics. The results revealed that clarithromycin exhibited significant antibacterial efficacy against M. avium and M. intracellulare, with drug resistance rates of 17.2% and 22.2%, respectively. The average drug resistance rate among SGM was 17.8%, while for RGM, it was 62.5%. Aminoglycosides are commonly used and effective drugs for NTM treatment. In our study, amikacin demonstrated potent antibacterial effects on M. avium and M. intracellulare, with low drug resistance rates of 6.9% and 18.5%, respectively. The antibacterial activity of amikacin against MAC was superior to that of clarithromycin. Notably, amikacin also displayed good antibacterial efficacy against both SGM and RGM, with drug resistance rates of 13.7% and 45.0%, respectively. In addition to its effectiveness against MAC, tobramycin’s antibacterial activity against M. chelonae/M. abscessus was notable at 94.3%, although it differed from amikacin at 51.4%. This discrepancy may be attributed to variations in ethnicity, geographical regions, or clinical isolates. While aminoglycosides demonstrate potent antibacterial activity against NTM, their prolonged use can lead to hepatorenal toxicity and ototoxicity. Therefore, these drugs should be used judiciously in clinical practice. Additionally, in this study, the fluoroquinolone moxifloxacin exhibited robust antibacterial activity against SGM but showed weaker effectiveness against RGM.
Conclusions
The isolation rate of NTM in southwest China has shown an increasing trend in recent years. The majority of infected cases involve elderly patients, and there has been an elevated proportion of individuals with HIV infection. The predominant clinical isolates are MAC and M. chelonae/M. abscessus, followed by M. kansasii and M. fortuitum. Among the tested antibiotics, amikacin, moxifloxacin, clarithromycin, and linezolid demonstrated effective antibacterial activity against SGM, whereas linezolid and amikacin exhibited relatively better antibacterial activity against RGM. The incidence of NTM infection may be positively correlated with the level of regional economic development and healthcare conditions.
Limitations
One significant limitation of this study is the extended treatment cycle required for NTM infections (Lee et al., 2015; Daley et al., 2020; Gopalaswamy et al., 2020), often exceeding one year or even longer. Consequently, many cases experience issues such as loss to follow-up, poor treatment outcomes, and a high recurrence rate. Although follow-up observations are ongoing for some cases to assess treatment efficacy, the data collection for these cases is incomplete. Future research should aim to provide more comprehensive and valuable reference information for clinical use.
Data availability statement
The original contributions presented in the study are included in the article/supplementary material, further inquiries can be directed to the corresponding author/s.
Ethics statement
The studies involving humans were approved by This study was approved by the Ethics Committee of PHCC [2017Y] 025. The studies were conducted in accordance with the local legislation and institutional requirements. Written informed consent for participation was not required from the participants or the participants’ legal guardians/next of kin in accordance with the national legislation and institutional requirements.
Author contributions
DW: Conceptualization, Data curation, Funding acquisition, Resources, Supervision, Writing – original draft, Writing – review & editing. HL: Data curation, Investigation, Resources, Writing – review & editing. YZ: Data curation, Investigation, Supervision, Writing – review & editing. YX: Data curation, Investigation, Writing – review & editing. YL: Data curation, Project administration, Supervision, Writing – original draft, Writing – review & editing.
Funding
The author(s) declare financial support was received for the research, authorship, and/or publication of this article. This study was supported by 2023 Chengdu Medical Research Project, Sichuan, China (202311013240) (to DMW).
Conflict of interest
The authors declare that the research was conducted in the absence of any commercial or financial relationships that could be construed as a potential conflict of interest.
Publisher’s note
All claims expressed in this article are solely those of the authors and do not necessarily represent those of their affiliated organizations, or those of the publisher, the editors and the reviewers. Any product that may be evaluated in this article, or claim that may be made by its manufacturer, is not guaranteed or endorsed by the publisher.
References
Babalik, A., Koç, E. N., Sekerbey, H. G., Dönmez, G. E., Balikci, A., Kilicaslan, Z. (2023). Nontuberculous mycobacteria isolation from sputum specimens: A retrospective analysis of 1061 cases. Int. J. Mycobacteriol. 12 (1), 55–65. doi: 10.4103/ijmy.ijmy_10_23
Cao, X. J., Li, Y. P., Wang, J. Y., Zhou, J., Guo, X. G. (2021). MPT64 assays for the rapid detection of Mycobacterium tuberculosis. BMC Infect. Dis. 21 (1), 336. doi: 10.1186/s12879-021-06022-w
Clinical and Laboratory Standards Institute (CLSI) (2015). Susceptibility Testing of Mycobacteria, Nocardia spp., and Other Aerobic Actinomycetes. 3rd Edition (USA: CLSI guideline. M24).
Daley, C. L., Iaccarino, J. M., Lange, C., Cambau, E., Wallace, R. J., Jr, Andrejak, C., et al. (2020). Treatment of nontuberculous mycobacterial pulmonary disease: an official ATS/ERS/ESCMID/IDSA clinical practice guideline. Clin. Infect. Dis. 71 (4), e1–e36. doi: 10.1093/cid/ciaa241
Editorial Board of CSTB (2016). Nontuberculous mycosis diagnosis and treatment expert consensus. Chin. J. Tuberc Respir. 39, 438–443. doi: 10.3760/cma.j.issn.1001-0939.2012.08.006. Chin J Tuberc Respir Dis.
Falkinham, J. O., 3rd (1996). Epidemiology of infection by nontuberculous mycobacteria. Clin. Microbiol. Rev. 9 (2), 177–215. doi: 10.1128/CMR.9.2.177
Falkinham, J. O., 3rd (2002). Nontuberculous mycobacteria in the environment. Clin. Chest Med. 23 (3), 529–551. doi: 10.1016/s0272-5231(02)00014-x
From the Centers for Disease Control and prevention (1993). 1993 revised classifification system for HIV infection and expanded surveillance case defifinition for AIDS among adolescents and adults. JAMA 269, 460.
Giannoni, F., Lanni, A., Iacobino, A., Fattorini, L. (2023). Italian Multicentre Study on Nontuberculous Mycobacteria (IMS-NTM); members of the IMS-NTM laboratory network. Epidemiology and drug susceptibility of nontuberculous mycobacteria (NTM) in Italy in 2016-2020. Ann. Ist Super Sanita. 59 (2), 132–138. doi: 10.4415/ANN_23_02_06
Gopalaswamy, R., Shanmugam, S., Mondal, R., Subbian, S. (2020). Of tuberculosis and non-tuberculous mycobacterial infections - a comparative analysis of epidemiology, diagnosis and treatment. J. BioMed. Sci. 27 (1), 74. doi: 10.1186/s12929-020-00667-6
Guo, Q., Wei, J., Zou, W., Li, Q., Qian, X., Zhu, Z. (2021). Antimicrobial susceptibility profiles of Mycobacterium abscessus complex isolates from respiratory specimens in Shanghai, China. J. Glob Antimicrob. Resist. 25, 72–76. doi: 10.1016/j.jgar.2021.02.024
Jeong, S. H., Kim, S. Y., Huh, H. J., Ki, C. S., Lee, N. Y., Kang, C. I., et al. (2017). Mycobacteriological characteristics and treatment outcomes in extrapulmonary Mycobacterium abscessus complex infections. Int. J. Infect. Dis. 60, 49–56. doi: 10.1016/j.ijid.2017.05.007
Jing, H., Wang, H., Wang, Y., Deng, Y., Li, X., Liu, Z., et al. (2012). Prevalence of nontuberculous mycobacteria infection, China, 2004-2009. Emerg. Infect. Dis. 18 (3), 527–528. doi: 10.3201/eid1803.110175
Lan, R., Yang, C., Lan, L., Ou, J., Qiao, K., Liu, F., et al. (2011). Mycobacterium tuberculosis and non-tuberculous mycobacteria isolates from HIV-infected patients in Guangxi, China. Int. J. Tuberc Lung Dis. 15 (12), 1669–1675. doi: 10.5588/ijtld.11.0036
Lee, M. R., Sheng, W. H., Hung, C. C., Yu, C. J., Lee, L. N., Hsueh, P. R. (2015). Mycobacterium abscessus complex infections in humans. Emerg. Infect. Dis. 21 (9), 1638–1646. doi: 10.3201/2109.141634
Li, G., Pang, H., Guo, Q., Huang, M., Tan, Y., Li, C., et al. (2017). Antimicrobial susceptibility and MIC distribution of 41 drugs against clinical isolates from China and reference strains of nontuberculous mycobacteria. Int. J. Antimicrob. Agents. 49 (3), 364–374. doi: 10.1016/j.ijantimicag.2016.10.024
Liu, C. F., Song, Y. M., He, W. C., Liu, D. X., He, P., Bao, J. J., et al. (2021). Nontuberculous mycobacteria in China: incidence and antimicrobial resistance spectrum from a nationwide survey. Infect. Dis. Poverty. 10 (1), 59. doi: 10.1186/s40249-021-00844-1
Liu, S., Gao, X., Zhu, J., Chen, J., Yang, H., He, L. (2019). Nontuberculous mycobacteria pulmonary disease: A retrospective analysis. Zhong Nan Da Xue Xue Bao Yi Xue Ban. 44 (4), 432–436. doi: 10.11817/j.issn.1672-7347.2019.04.014
López-Roa, P., Aznar, E., Cacho, J., Cogollos-Agruña, R., Domingo, D., García-Arata, M. I., et al. (2020). Epidemiology of Non-Tuberculous Mycobacteria isolated from clinical specimens in Madrid, Spain, from 2013 to 2017. Eur. J. Clin. Microbiol. Infect. Dis. 39 (6), 1089–1094. doi: 10.1007/s10096-020-03826-7
Maya, T. G., Komba, E. V., Mensah, G. I., Mbelele, P. M., Mpagama, S. G., Mfinanga, S. G., et al. (2022). Drug susceptibility profiles and factors associated with non-tuberculous mycobacteria species circulating among patients diagnosed with pulmonary tuberculosis in Tanzania. PloS One 17 (3), e0265358. doi: 10.1371/journal.pone.0265358
Przybylski, G., Bukowski, J., Kowalska, W., Pilaczyńska-Cemel, M., Krawiecka, D. (2023). Trends from the last decade with nontuberculous mycobacteria lung disease (NTM-LD): clinicians' Perspectives in regional center of pulmonology in Bydgoszcz, Poland. Pathogens 12 (8), 988. doi: 10.3390/pathogens12080988
Tan, Y., Su, B., Shu, W., Cai, X., Kuang, S., Kuang, H., et al. (2018). Epidemiology of pulmonary disease due to nontuberculous mycobacteria in Southern China, 2013-2016. BMC Pulm Med. 18 (1), 168. doi: 10.1186/s12890-018-0728-z
Tarashi, S., Sakhaee, F., Masoumi, M., Ghazanfari Jajin, M., Siadat, S. D., Fateh, A. (2023). Molecular epidemiology of nontuberculous mycobacteria isolated from tuberculosis-suspected patients. AMB Express. 13 (1), 49. doi: 10.1186/s13568-023-01557-4
Thornton, C. S., Mellett, M., Jarand, J., Barss, L., Field, S. K., Fisher, D. A. (2021). The respiratory microbiome and nontuberculous mycobacteria: an emerging concern in human health. Eur. Respir. Rev. 30 (160), 200299. doi: 10.1183/16000617.0299-2020
Tuberculosis Branch of Chinese Medical Association (2020). Guidelines for diagnosis and treatment of nontuberculous mycobacteriosis (2020 edition). Chin. J. Tuberc Respir. Dis. 43 (11), 918–946. doi: 10.3760/cMA.j.cn112147-20200508-00570
Vongthilath-Moeung, R., Plojoux, J., Poncet, A., Renzi, G., Veziris, N., Schrenzel, J., et al. (2022). Nontuberculous mycobacteria under scrutiny in the Geneva area (2015-2020). Respiration 101 (4), 367–375. doi: 10.1159/000520033
Wang, D. M., Li, Q. F., Zhu, M., Wu, G. H., Li, X., Xu, Y. H., et al. (2020). Epidemiological, clinical characteristics and drug resistance situation of culture-confirmed children TBM in southwest of China: a 6-year retrospective study. BMC Infect. Dis. 20 (1), 318. doi: 10.1186/s12879-020-05041-3
Wang, D. M., Li, Q. F., Zhu, M., Xu, Y. H., Luo, J., Li, Y. J., et al. (2017). Analysis of infection and drug-resistance in 6 107 cases of extrapulmonary tuberculosis in Chengdu area. Zhonghua Jie He Hu Xi Za Zhi 40, 592–595. doi: 10.3760/cma.j.issn.1001-0939.2017.08.010
Wang, D. M., Liao, Y., Li, Q. F., Zhu, M., Wu, G. H., Xu, Y. H., et al. (2019). Drug resistance and pathogenic spectrum of patients coinfected with nontuberculous mycobacteria and human-immunodeficiency virus in Chengdu, China. Chin. Med. J. (Engl) 132 (11), 1293–1297. doi: 10.1097/CM9.0000000000000235
Wang, D. M., Zhu, M., Xu, Y. H., Luo, J., Li, Y. J., Liu, X. D., et al. (2016). Clinical relevance and drug resistance of nontuberculous mycobacteria, Sichuan area in Chinese. Chin. J. Infect. Dis. 34, 520–523. doi: 10.3760/cma.j.issn.1000-6680.2016.09.002
Wang, Y., Yang, Y., Shi, X., Mao, S., Shi, N., Hui, X. (2016). The spatial distribution pattern of human immunodefificiency virus/acquired immune defificiency syndrome in China. Geospat Health 11, 414. doi: 10.4081/gh.2016.414
Wu, J., Zhang, Y., Li, J., Lin, S., Wang, L., Jiang, Y., et al. (2014). Increase in nontuberculous mycobacteria isolated in Shanghai, China: results from a population-based study. PLoS One 9 (10), e109736. doi: 10.1371/journal.pone.0109736
Yang, S., Chiu, A. P. Y., Lin, Q., Zeng, Z., Li, Y., Zhang, Y., et al. (2018). HIV epidemics in Shenzhen and Chongqing, China. PLoS One 13, e192849. doi: 10.1371/journal.pone.0192849
Zhang, L., Chow, E. P., Su, S., Yiu, W. L., Zhang, X., Iu, K. I., et al. (2015). A systematic review and meta-analysis of the prevalence, trends, and geographical distribution of HIV among Chinese female sex workers (2000–2011): implications for preventing sexually transmitted HIV. Int. J. Infect. Dis. 39, 76–86. doi: 10.1016/j.ijid.2015.08.014
Keywords: epidemiology, TB, drug resistance, NTM, clinical characteristics
Citation: Wang D-M, Liu H, Zheng Y-L, Xu Y-H and Liao Y (2023) Epidemiology of Nontuberculous Mycobacteria in Tuberculosis suspects, Southwest of China, 2017-2022. Front. Cell. Infect. Microbiol. 13:1282902. doi: 10.3389/fcimb.2023.1282902
Received: 25 August 2023; Accepted: 17 October 2023;
Published: 31 October 2023.
Edited by:
Sanket Kaushik, Amity University Jaipur, IndiaReviewed by:
Anna Malgorzata Brzostek, Polish Academy of Sciences, PolandXuejiao Hu, Guangdong Provincial People’s Hospital, China
Copyright © 2023 Wang, Liu, Zheng, Xu and Liao. This is an open-access article distributed under the terms of the Creative Commons Attribution License (CC BY). The use, distribution or reproduction in other forums is permitted, provided the original author(s) and the copyright owner(s) are credited and that the original publication in this journal is cited, in accordance with accepted academic practice. No use, distribution or reproduction is permitted which does not comply with these terms.
*Correspondence: Yi Liao, 1191063186@qq.com