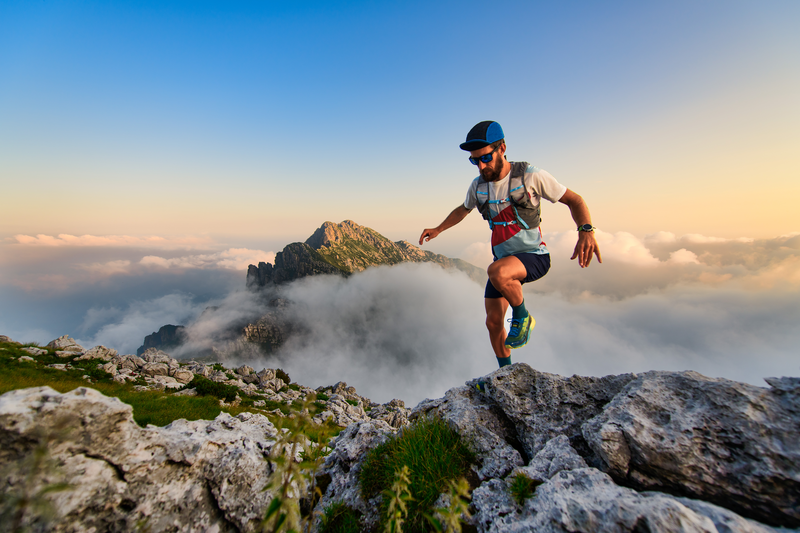
94% of researchers rate our articles as excellent or good
Learn more about the work of our research integrity team to safeguard the quality of each article we publish.
Find out more
ORIGINAL RESEARCH article
Front. Cell. Infect. Microbiol. , 19 December 2023
Sec. Antibiotic Resistance and New Antimicrobial drugs
Volume 13 - 2023 | https://doi.org/10.3389/fcimb.2023.1282320
This article is part of the Research Topic Synergistic Combinatorial Treatments to Overcome Antibiotic Resistance View all 6 articles
Introduction: Candida albicans is the primary cause of systemic candidiasis, which is involved in high morbidity and mortality. Drug resistance exacerbates these problems. In addition, there are limited antifungal drugs available. In order to solve these problems, combination therapy has aroused great interest. Teriflunomide is an immunosuppressant. In the present work, we aimed to identify whether teriflunomide can reverse the resistance of Candida albicans in the presence of sub-inhibitory concentrations of fluconazole in vitro and in vivo.
Methods: Seven Candida albicans isolates were used in this study. Susceptibility of Candida albicans in vitro to the drugs was determined using a checkerboard microdilution assay in accordance with the recommendations of the Clinical and Laboratory Standards Institute. The effects of drugs on biofilm biomass of Candida albicans were determined by crystal violet staining. The development ability of Candida albicans hyphae was performed using a modified broth microdilution method. Galleria mellonella was used for testing the in vivo efficacy of the combination therapies.
Results: We found that the combination of teriflunomide (64 µg/mL) and fluconazole (0.5-1 µg/mL) has a significant synergistic effect in all resistant Candida albicans isolates (n=4). Also, this drug combination could inhibit the immature biofilm biomass and hyphae formation of resistant Candida albicans. Galleria mellonella was used for testing the in vivo efficacy of this combination therapies. As for the Galleria mellonella larvae infected by resistant Candida albicans, teriflunomide (1.6 µg/larvae) combined with fluconazole (1.6 µg/larvae) significantly increased their survival rates, and reduced the fungal burden, as well as damage of tissue in comparison to that in the control group or drug monotherapy group.
Conclusion: These results expand our knowledge about the antifungal potential of teriflunomide as an adjuvant of existing antifungal drugs, and also open new perspectives in the treatment of resistant Candida albicans based on repurposing clinically available nonantifungal drugs.
Invasive Candida bloodstream infections remain the most frequent life-threatening fungal disease, with Candida albicans (C. albicans) isolates accounting for 70% to 80% of the Candida isolates isolated from infected patients (Chin et al., 2016). Indeed, C. albicans bloodstream infections cause a mortality rate more than 40% (Pfaller and Diekema, 2007; Gow and Yadav, 2017; Robbins et al., 2017). Moreover, the broad and irrational utilization of azole antifungals, especially fluconazole, has led to the emergence azole-resistant clinical isolates of C. albicans (Kohli et al., 2002). Owing to the limited number of available antifungal drugs in clinic, there is a need for alternative approaches or developing new antifungal drugs against resistant C. albicans infections.
The development of new drugs is an expensive and time-consuming project (Khanna, 2012; Wang et al., 2016). Only one antifungal drug (isavuconazole) was newly licensed in the last ten years (Van Daele et al., 2019). The lack of new antifungal drugs and the continual buildup of resistance mechanisms by C. albicans require new strategies with satisfying antifungal efficacy (Fisher et al., 2022). Combination drug therapy may be an effective way to overcome resistant fungi. In fact, drug combinations have already been used to treat many diseases, such as cancer, HIV or cardiovascular disease (Lehar et al., 2009; Ahmad et al., 2015; Shrestha et al., 2015). Combining a new antifungal agent with a known antifungal drug, with a different or similar mechanism of action, would represent a novel therapeutic approach, which may circumvent the drug resistance problem. To date, there have been many studies of FDA-approved drugs used in combination with existing antifungal drugs to overcome resistant C. albicans, the rationale being that the safety of FDA-approved drugs has been studied and their properties are well known (Li et al., 2019b; Rajasekharan et al., 2019; Zhang et al., 2020).
Teriflunomide, a dihydrowhey acid dehydrogenase inhibitor, is an FDA-approved immunomodulator with low cytotoxicity, and used to treat relapse-remission multiple sclerosis in the clinic (Wiese et al., 2013; Scott, 2019; Barua et al., 2023; Lang et al., 2023). Up to now, no study on the antifungal activity of teriflunomide has so far been reported. Inspired by the antifungal effects of other immunomodulators, such as tacrolimus and ciclosporin A, we attempt to explore whether teriflunomide can be used in combination with azole antifungal drugs to combat resistant C. albicans. Fluconazole is an azole antifungal drug with low toxicity and high bioavailability. We aimed to identify whether teriflunomide could potentially enhance its antifungal activity. For this purpose, the synergistic antifungal activity of fluconazole combined with teriflunomide on C. albicans were investigated in vitro and in vivo. Furthermore, we also attempted to explore the potential antifungal mechanism for this drug combination.
Seven isolates of C. albicans (SC5314, CA4, CA8, CA10, CA16, CA103 and CA137) were used in this study, which were isolated from the blood of patients and kindly provided by Professor Shujuan Sun (Shandong Provincial Qianfoshan Hospital, Jinan, China). C. albicans ATCC 10231 was used as a reference strain of the study. All isolates were taken from previously established stocks. Before all experiments, isolates were routinely inoculated twice in yeast extract-peptone-dextrose agar medium overnight at 35°C. Teriflunomide (Dalian Meilun Biotech Co., Ltd, Dalian, China) was dissolved in ethyl alcohol at a concentration of 54, 000 μg/mL, whereas fluconazole (Dalian Meilun Biotech Co., Ltd, Dalian, China) was dissolved in sterilized water at a concentration of 5, 120 μg/mL. To avoid affecting the outcomes of experiments, the proportion of ethyl alcohol was less than 1% of the whole test volume, according to the Clinical and Laboratory Standards Institute M27-A3 (CLSI M27-A3) (Rex et al., 2008). This study was approved by the Scientific Research Ethics Committee of Shandong Provincial Maternal and Child Health Hospital (No. 2023-083).
Susceptibility of planktonic cells of C. albicans to the drugs was determined using a checkerboard microdilution assay in accordance with the recommendations of CLSI M27-A3 (Rex et al., 2008). The minimal inhibitory concentrations (MIC) of teriflunomide and fluconazole against C. albicans strains were determined by broth microdilution method as described previously (Mo et al., 2020). Yeast with a final concentration of 1 ×103 cells/mL was inoculated in the RPMI 1640 liquid medium with serial (2 ×) dilutions of each drug on 96-well flat bottom plates. After incubation at 35 °C for 24 h, MIC was visually determined as the lowest concentration of drug that reduced ≥50% growth in comparison to growth control without drug treatment (Ernst et al., 2002; Rex et al., 2008). Fractional inhibitory concentration (FIC) indexes (FICI) model was applied for the calculation of each combination using an equation, FICA + FICB = FICI, where FICA is the MIC of fluconazole in combination divided by the MIC of fluconazole alone, and FICB is the MIC of teriflunomide in combination divided by the MIC of teriflunomide alone. The results were defined as FICI ≤ 0.5 for synergism, FICI > 4.0 for antagonism, and 0.5 < FICI ≤ 4.0 for no interaction (Odds, 2003).
To further assess the synergistic antifungal mechanisms, we tested the effect of teriflunomide in combination with fluconazole against the biofilm biomass of resistant C. albicans (CA10). Living and dead fungal cells were stained with crystal violet (Petrachi et al., 2017). The total biomass of biofilms was assessed using crystal violet staining, with slight modifications (Manoharan et al., 2017). Cell suspensions were adjusted to reach a concentration of 1 × 106 CFU per mL in RPMI 1640 medium. Then, 200 μL of cell suspensions was transferred into 96-well flat bottom plates and incubated for different times (4 h, 24 h) at 37°C. After incubation, non-adhered cells were removed by washing thrice with sterile PBS, and then 200 μL of desired concentration of drugs in RPMI 1640 medium was added to each well of 96-well flat bottom plates. The plates were incubated at 37°C for 24 h to evaluate the effect of drugs on biofilm biomass by crystal violet (0.1%) staining. Briefly, wells were washed twice with sterile PBS to remove all non-adherent cells and then stained with crystal violet for 5 min. The wells were washed three times with sterile PBS. After drying, 120 μL of 95% ethanol was added into each well. After 10 min of 95% ethanol treatment, 100 μL of suspension from each well was transferred to a new 96-well flat bottom plate and the optical density (OD) values at 570 nm was measured using a microplate reader (Epoch2, Agilen BioTek Co., Ltd., USA). Each experiment was performed at least three times.
Yeast-to-hyphae phenotype switching is a characteristic pathogenic trait of C. albicans (Boyce and Andrianopoulos, 2015). The hyphal development ability was performed using a modified broth microdilution method (Chang et al., 2012). Both 100 μL of C. albicans (CA10) cell suspensions (2 × 10 5 CFU per mL) and 100 μL of desired concentration of drugs in RPMI 1640 medium was simultaneously added to each well of 96-well flat bottom plates. Wells containing C. albicans and RPMI 1640 alone served as controls. The plates were incubated at 35°C for 4 h. After incubation, cells were observed for morphological transition under a fluorescent microscope (ECLIPSE Ts2, Nikon Instruments, Japan). Each experiment was performed at least three times.
Galleria mellonella (G. mellonella) larvae were used as an invertebrate infection model to evaluate the in vivo interactions between fluconazole and teriflunomide, as described previously with some modifications (Li et al., 2019a). Four groups of eighteen randomly chosen larvae with a similar weight (ca. 0.20 g) and injected with 10 μL of a yeast (CA10) suspension (1 × 108 CFU/mL) via the last left proleg. After incubation at 35 °C for 2 h, each larva was injected with 10 μL of sterile PBS, fluconazole (160 μg/mL), teriflunomide (160 μg/mL), or fluconazole (160 μg/mL) plus teriflunomide (160 μg/mL) via the last right proleg. All larvae were incubated at 35 °C in the dark, and the numbers of G. mellonella that survived were recorded daily for a period of 4 days. The larvae not responding to touch were considered dead. The survival curves were plotted by the Kaplan-Meier method using SPSS 22 software. Each experiment was performed at least three times.
For histological study, four groups of eighteen randomly chosen larvae with a similar weight (ca. 0.20 g) and the experimental procedures were the same as described above. Three larvae incubated for two days were randomly selected from each group and cut into sections (7 μm). Sections stained with periodic acid Schiff (PAS) stain were observed using the digital slice scanning system (Precice 510, Unicmedical equipment Co., Ltd., China). Each experiment was performed at least three times.
The combination of teriflunomide and fluconazole was found to have a significant synergistic effect against all resistant C. albicans isolates (100%) in this study (Table 1). When fluconazole was combined with teriflunomide (64 µg/mL), the MIC ranges of fluconazole decreased from >512 µg/mL to 0.5-1 µg/mL, suggesting a strong synergy for the combination of teriflunomide and fluconazole against resistant C. albicans. No synergistic effect was found for this drug combination against susceptible C. albicans isolates (Table 1).
Crystal violet staining is often used for the determination of yeast biofilm biomass, mainly because of its high detection accuracy for a large number of biofilms (Kulisova et al., 2023). Figure 1 showed the effect of teriflunomide in combination with fluconazole on the immature or mature biofilm biomass of resistant C. albicans. Compared with the control group, fluconazole group alone, or teriflunomide group alone respectively, the biomass of immature biofilm (4 h) was significantly inhibited by this drug combination (Figure 1A). However, compared with the other three groups, the biomass of mature biofilm (24 h) was not significantly inhibited by this drug combination (Figure 1B).
Figure 1 Teriflunomide in combination with fluconazole inhibited the biofilm biomass. (A) immature biofilm (4 h); (B) mature biofilm (24 h).
Liquid RPMI 1640 medium is known to induce morphological transition of C. albicans. To determine the effect of teriflunomide alone or in combination with fluconazole on the yeast-to-hypha morphological transition of resistant C. albicans, CA10 cells and drugs were placed in liquid RPMI 1640 medium. C. albicans in the control group without drug treatment revealed the formation of long true hyphae (Figures 2A–C). The teriflunomide and fluconazole alone group showed the similar amounts of hyphae as the control group (Figures 2D–I). Interestingly, hyphae were scarcely observed in the group of teriflunomide (64 µg/mL) in combination with fluconazole (1 µg/mL), indicating this drug combination could inhibit the yeast-to-hypha morphological transition of resistant C. albicans (Figures 2J–L).
Figure 2 Teriflunomide in combination with fluconazole inhibited yeast-to-hypha morphological transition. (A–C): control; (D–F): fluconazole (1 µg/mL); (G–I): teriflunomide (64 µg/mL); (J–L): teriflunomide (64 µg/mL) in combination with fluconazole (1 µg/mL).
In the in vivo experiment, eighteen randomly chosen G. mellonella larvae in each group were injected with the CA10 suspension, and after 2 h of infection, the larvae were treated with drugs. As can been seen from Figure 3, teriflunomide combined with fluconazole kept the larvae free from CA10 infections and resulted in significantly higher survival of the larvae over a 4-day infection. In brief, the mean survival rate of larvae on the 2nd-4th day in the control group, fluconazole group and teriflunomide group was 31-50%, 35-70% and 33-69% respectively (Supplementary Table 1). Notably, the survival rate of larvae on 2nd-4th in the drug combination group was 69-87%, which was significantly higher than that of the control group or drug monotherapy group (p < 0.05) (Supplementary Table 1). Data from any one experiment were shown in Figure 3, which indicated that the combination of teriflunomide and fluconazole significantly increased the survival rates of infected larvae.
Figure 3 Teriflunomide in combination with fluconazole improved the survival rates of G. mellonella after infection. A log-rank test for these curves was conducted and the p value was 0.024.
Regarding observation of histological sections (Figure 4), the infected tissues showed black areas after PAS staining, and the black areas contained clustered yeast cells and hyphae. As can been seen from Figure 4, black areas in the fluconazole-monotherapy group and teriflunomide-monotherapy group as well as the control group were numerous and large, while those in drug combination group were obviously much fewer and smaller. These observations suggested that teriflunomide combined with fluconazole significantly reduced the fungal burden and tissue damage of the resistant C. albicans to the larvae in comparison with that in the control group or drug monotherapy group.
Figure 4 Teriflunomide in combination with fluconazole reduced the fungal burden and damage in tissues of G. mellonella after infection. (A–C): control; (D–F): fluconazole; (G–I): teriflunomide; (J–L): teriflunomide in combination with fluconazole.
In the last decades, C. albicans has served as the leading causal agent of life-threatening invasive infections with mortality rates approaching 40% despite treatment (Chen et al., 2020). Besides the high mortality, resistance of C. albicans to conventional antifungal drugs is also the paramount concern in the field of medical mycology. The emergence of drug-resistant isolates of C. albicans has created a higher risk for clinical infections and is a growing concern. Because none of the current antifungals have all the characteristics of the ideal antifungals, and the discovery rate of new antifungals is declining, the study of drug combination against drug-resistant fungal infections is receiving increasing attention (Kaneko et al., 2013; Wong et al., 2014). In recent years, there has been increasing interest in repurposing FDA-approved drugs because their clinical and toxicological properties are already known, thus reducing the cost and time of drug development. Many FDA-approved drugs from pharmacologically distinct families have been proved to have antifungal potentials against resistant C. albicans, such as aripiprazole, ambroxol hydrochloride, and ribavirin (Li et al., 2019b; Rajasekharan et al., 2019; Zhang et al., 2020). Inspired by these findings, we evaluated the interaction of teriflunomide, an FDA-approved immunomodulator with low cytotoxicity, and fluconazole against resistant C. albicans. In this study, no synergistic effect was found for this drug combination against the susceptible C. albicans isolates (Table 1). Nevertheless, we first found that teriflunomide can reverse the resistance of C. albicans to fluconazole in vitro (Table 1). The combination of teriflunomide (64 µg/mL) and fluconazole (0.5-1 µg/mL) was found to have strong synergistic effects against planktonic cells of four resistant C. albicans isolates, demonstrating the antifungal potentials of this drug combination.
To clarify the synergistic effects of this drug combination against resistant C. albicans, further exploration of the underlying mechanisms is quite needed. Many mechanisms seem to play important roles in the development of C. albicans resistance. Among them, over-expression of efflux pumps, biofilm, and hypha are the most important research hotspots. So far, several drug combinations have been proved to exert their synergistic antifungal effects against resistant C. albicans by inhibiting the over-expression of efflux pumps (Usai et al., 2019; Lu et al., 2020). What’s more, biofilm formation of C. albicans is among the culprits of its resistance, with multiple studies reporting up to a 1000-fold greater drug resistance in biofilm-forming cells compared with non-biofilm cells in vitro (Tobudic et al., 2012). Many available antifungal drugs against C. albicans are capable of controlling the growth of planktonic cells alone, resulting in their invalidation in controlling biofilms in vivo, which eventually may lead to the therapeutic failure in a clinical scenario (Taff et al., 2013). Different C. albicans morphotypes differentially elicit host immune responses and the production of cytokines. Hyphae, which represent an important phase of C. albicans in the disease process, can cause tissue damage by invading mucosal epithelial cells (Chen et al., 2020). The formation of hyphae ultimately forms biofilms, which protect sessile yeast cells from antifungal drugs and may induce new infections. While drug resistance mechanisms in the commensal human pathogen C. albicans are continually evolving, hyphae are still one of the most important factors associated with drug resistance. Therefore, exploring better therapeutic therapies to combat the hyphae of C. albicans appears to be particularly vital. Therefore, we further studied the efflux pump activity, biofilm biomass, and hyphae formation of resistant C. albicans isolates with the treatment of this drug combination. The present study found that this drug combination has no impact on the activity of efflux pump activity (Supplementary Figure 1). However, teriflunomide in combination with fluconazole can synergistically inhibit the biomass of resistant C. albicans immature biofilm (4 h) (Figure 1A). Our results in this study also indicated that teriflunomide in combined with fluconazole exerts the synergistic effects against resistant C. albicans by inhibiting its hyphae formation (Figure 2). Furthermore, this study not only provides a theoretical basis for the identification of targets in candidiasis treatment, but also gives some reference to the study of novel antifungal drugs.
G. mellonella is a convenient in vivo model for assessing the activity and toxicity of antimicrobial agents and for studying the immune response to pathogens and provide results similar to those from mammals (Brennan et al., 2002; Kavanagh and Sheehan, 2018; Jemel et al., 2020). G. mellonella larvae are now widely used in academia and their use can assist in the identification and evaluation of novel antimicrobial agents. G. mellonella larvae are inexpensive to purchase and house, easy to inoculate, generate results within 24–48 h and their use is not restricted by legal or ethical considerations. In this study, the in vivo antifungal effects of teriflunomide combined with fluconazole was evaluated by using the model of G. mellonella. The determination of survival curve showed that teriflunomide combined with fluconazole was very effective in protecting larvae from fatal infection by resistant C. albicans (Figure 3). In addition, histological examination plays an important role in studying the virulence of infection. In this study, it showed that the virulence was related to the degree of tissue damage. Resistant C. albicans produced hyphae and induced serious tissue damage in larvae. Many infected black areas and clusters of yeast cells were also observed. After the treatment of drug combination, fewer clustered yeast cells and hyphae were observed (Figure 4). The good efficacy of the combination therapies of teriflunomide combined with fluconazole on G. mellonella infected by resistant C. albicans was confirmed.
In conclusion, our findings demonstrated a potential use of this drug combination in prevention or early treatment of resistant C. albicans infections. Inhibition the biomass of immature biofilm and hyphae formation provided an explanation of the synergistic mechanisms for this drug combination. Although teriflunomide has a certain degree of side effects in clinical use, with the modification of the structure of teriflunomide, this combination or their analogues may become a new alternative way to treat resistant C. albicans infections. Besides, this study on the repurposing of teriflunomide could also serve as an example to inspire the reapplication of other FDA-approved drugs in the antifungal field.
The original contributions presented in the study are included in the article/Supplementary Material. Further inquiries can be directed to the corresponding authors.
This study was approved by the Scientific Research Ethics Committee of Shandong Provincial Maternal and Child Health Hospital (No.2023-083).
XL: Conceptualization, Data curation, Methodology, Writing – original draft. BK: Conceptualization, Data curation, Writing – original draft. YS: Conceptualization, Data curation, Writing – original draft. FS: Conceptualization, Writing – original draft. HY: Conceptualization, Writing – review & editing. SZ: Conceptualization, Writing – review & editing.
The author(s) declare financial support was received for the research, authorship, and/or publication of this article. This work was supported by Shandong Province Natural Science Foundation [Grant no. ZR2020QH365], Key Research and Development Plan of Shandong Province [Grant no. 2019GSF108204], Research fund of China Maternal and Child Health Research Association [Grant no. 2023CAMCHS003A11], and the special fund research topic of Shandong Provincial Maternal and Child Health Care Hospital [Grant no. YJKY20220-35]. All these funding organizations provided funds for the purchase of laboratory supplies for our study but had no role in the design of the study and the collection, analysis and interpretation of the data.
The authors declare that the research was conducted in the absence of any commercial or financial relationships that could be construed as a potential conflict of interest.
All claims expressed in this article are solely those of the authors and do not necessarily represent those of their affiliated organizations, or those of the publisher, the editors and the reviewers. Any product that may be evaluated in this article, or claim that may be made by its manufacturer, is not guaranteed or endorsed by the publisher.
The Supplementary Material for this article can be found online at: https://www.frontiersin.org/articles/10.3389/fcimb.2023.1282320/full#supplementary-material
Ahmad, A., Wani, M. Y., Khan, A., Manzoor, N., Molepo, J. (2015). Synergistic Interactions of Eugenol-tosylate and Its Congeners with Fluconazole against Candida albicans. PloS One 10, e0145053. doi: 10.1371/journal.pone.0145053
Barua, S., Kaltenboeck, B., Juan, Y. C., Bird, R. C., Wang, C. (2023). Comparative evaluation of GS-441524, teriflunomide, ruxolitinib, molnupiravir, ritonavir, and nirmatrelvir for in vitro antiviral activity against feline infectious peritonitis virus. Vet. Sci. 10, 513. doi: 10.3390/vetsci10080513
Boyce, K. J., Andrianopoulos, A. (2015). Fungal dimorphism: the switch from hyphae to yeast is a specialized morphogenetic adaptation allowing colonization of a host. FEMS Microbiol. Rev. 39, 797–811. doi: 10.1093/femsre/fuv035
Brennan, M., Thomas, D. Y., Whiteway, M., Kavanagh, K. (2002). Correlation between virulence of Candida albicans mutants in mice and Galleria mellonella larvae. FEMS Immunol. Med. Microbiol. 34, 153–157. doi: 10.1111/j.1574-695X.2002.tb00617.x
Chang, W., Li, Y., Zhang, L., Cheng, A., Lou, H. (2012). Retigeric acid B attenuates the virulence of Candida albicans via inhibiting adenylyl cyclase activity targeted by enhanced farnesol production. PloS One 7, e41624. doi: 10.1371/journal.pone.0041624
Chen, H., Zhou, X., Ren, B., Cheng, L. (2020). The regulation of hyphae growth in Candida albicans. Virulence 11, 337–348. doi: 10.1080/21505594.2020.1748930
Chin, V. K., Lee, T. Y., Rusliza, B., Chong, P. P. (2016). Dissecting Candida albicans infection from the perspective of C. albicans virulence and omics approaches on host-pathogen interaction: a review. Int. J. Mol. Sci. 17, 1643. doi: 10.3390/ijms17101643.
Ernst, E. J., Roling, E. E., Petzold, C. R., Keele, D. J., Klepser, M. E. (2002). In vitro activity of micafungin (FK-463) against Candida spp.: microdilution, time-kill, and postantifungal-effect studies. Antimicrob. Agents Chemother. 46, 3846–3853. doi: 10.1128/AAC.46.12.3846-3853.2002
Fisher, M. C., Alastruey-Izquierdo, A., Berman, J., Bicanic, T., Bignell, E. M., Bowyer, P., et al. (2022). Tackling the emerging threat of antifungal resistance to human health. Nat. Rev. Microbiol. 20, 557–571. doi: 10.1038/s41579-022-00720-1
Gow, N., Yadav, B. (2017). Microbe Profile: Candida albicans: a shape-changing, opportunistic pathogenic fungus of humans. Microbiol. (Reading) 163, 1145–1147. doi: 10.1099/mic.0.000499
Jemel, S., Guillot, J., Kallel, K., Botterel, F., Dannaoui, E. (2020). Galleria mellonella for the evaluation of antifungal efficacy against medically important fungi, a narrative review. Microorganisms 8, 390. doi: 10.3390/microorganisms8030390
Kaneko, Y., Fukazawa, H., Ohno, H., Miyazaki, Y. (2013). Combinatory effect of fluconazole and FDA-approved drugs against Candida albicans. J. Infect. Chemother. 19, 1141–1145. doi: 10.1007/s10156-013-0639-0
Kavanagh, K., Sheehan, G. (2018). The use of Galleria mellonella larvae to identify novel antimicrobial agents against fungal species of medical interest. J. Fungi (Basel) 4, 113. doi: 10.3390/jof4030113
Khanna, I. (2012). Drug discovery in pharmaceutical industry: productivity challenges and trends. Drug Discovery Today 17, 1088–1102. doi: 10.1016/j.drudis.2012.05.007
Kohli, A., Smriti, N. F. N., Mukhopadhyay, K., Rattan, A., Prasad, R. (2002). In vitro low-level resistance to azoles in Candida albicans is associated with changes in membrane lipid fluidity and asymmetry. Antimicrob. Agents Chemother. 46, 1046–1052. doi: 10.1128/AAC.46.4.1046-1052.2002
Kulisova, M., Matatkova, O., Branyik, T., Zelenka, J., Drabova, L., Kolouchova, I. J. (2023). Detection of microscopic filamentous fungal biofilms - choosing the suitable methodology. J. Microbiol. Methods 205, 106676. doi: 10.1016/j.mimet.2023.106676
Lang, P., Geertsen, S. S., Lublin, A. L., Potter, M. C., Gladysheva, T., Gregory, J. S., et al. (2023). In vitro evaluation of the activity of teriflunomide against SARS-CoV-2 and the human coronaviruses 229E and OC43. Biochem. Biophys. Rep. 33, 101395. doi: 10.1016/j.bbrep.2022.101395
Lehar, J., Krueger, A. S., Avery, W., Heilbut, A. M., Johansen, L. M., Price, E. R., et al. (2009). Synergistic drug combinations tend to improve therapeutically relevant selectivity. Nat. Biotechnol. 27, 659–666. doi: 10.1038/nbt.1549
Li, X., Wu, X., Gao, Y., Hao, L. (2019a). Synergistic effects and mechanisms of combined treatment with harmine hydrochloride and azoles for resistant candida albicans. Front. Microbiol. 10 2295. doi: 10.3389/fmicb.2019.02295
Li, X., Wu, X., Gao, Y., Hao, L., Sun, S. (2019b). Apoptosis-linked antifungal effect of ambroxol hydrochloride by cystolic calcium concentration disturbance in resistant Candida albicans. Sci. China Life Sci. 62, 1601–1604. doi: 10.1007/s11427-018-9830-0
Lu, M., Yan, H., Yu, C., Yuan, L., Sun, S. (2020). Proton pump inhibitors act synergistically with fluconazole against resistant Candida albicans. Sci. Rep. 10, 498. doi: 10.1038/s41598-019-57174-4
Manoharan, R. K., Lee, J. H., Kim, Y. G., Lee, J. (2017). Alizarin and chrysazin inhibit biofilm and hyphal formation by candida albicans. Front. Cell Infect. Microbiol. 7, 447. doi: 10.3389/fcimb.2017.00447
Mo, F., Ma, J., Yang, X., Zhang, P., Li, Q., Zhang, J. (2020). In vitro and in vivo effects of the combination of myricetin and miconazole nitrate incorporated to thermosensitive hydrogels, on C. albicans biofilms. Phytomedicine 71, 153223. doi: 10.1016/j.phymed.2020.153223
Odds, F. C. (2003). Synergy, antagonism, and what the chequerboard puts between them. J. Antimicrob. Chemother. 52, 1. doi: 10.1093/jac/dkg301
Petrachi, T., Resca, E., Piccinno, M. S., Biagi, F., Strusi, V., Dominici, M., et al. (2017). An alternative approach to investigate biofilm in medical devices: A feasibility study. Int. J. Environ. Res. Public Health 14, 1587. doi: 10.3390/ijerph14121587
Pfaller, M. A., Diekema, D. J. (2007). Epidemiology of invasive candidiasis: a persistent public health problem. Clin. Microbiol. Rev. 20, 133–163. doi: 10.1128/CMR.00029-06
Rajasekharan, S. K., Lee, J. H., Lee, J. (2019). Aripiprazole repurposed as an inhibitor of biofilm formation and sterol biosynthesis in multidrug-resistant Candida albicans. Int. J. Antimicrob. Agents 54, 518–523. doi: 10.1016/j.ijantimicag.2019.05.016
Rex, J. H., Alexander, B. D., Andes, D., Arthington-Skaggs, B., Brown, S. D., Chaturvedi, V., et al. (2008). Reference method for broth dilution antifungal susceptibility testing of yeasts; Approved Standard—Third Edition. CLSI document M27-A3. Wayne, PA: Clinical and Laboratory Standards Institute.
Robbins, N., Caplan, T., Cowen, L. E. (2017). Molecular evolution of antifungal drug resistance. Annu. Rev. Microbiol. 71, 753–775. doi: 10.1146/annurev-micro-030117-020345
Scott, L. J. (2019). Teriflunomide: A review in relapsing-remitting multiple sclerosis. Drugs 79, 875–886. doi: 10.1007/s40265-019-01135-8
Shrestha, S. K., Fosso, M. Y., Garneau-Tsodikova, S. (2015). A combination approach to treating fungal infections. Sci. Rep. 5, 17070. doi: 10.1038/srep17070
Taff, H. T., Mitchell, K. F., Edward, J. A., Andes, D. R. (2013). Mechanisms of Candida biofilm drug resistance. Future Microbiol. 8, 1325–1337. doi: 10.2217/fmb.13.101
Tobudic, S., Kratzer, C., Lassnigg, A., Presterl, E. (2012). Antifungal susceptibility of Candida albicans in biofilms. Mycoses 55, 199–204. doi: 10.1111/j.1439-0507.2011.02076.x
Usai, D., Donadu, M., Bua, A., Molicotti, P., Zanetti, S., Piras, S., et al. (2019). Enhancement of antimicrobial activity of pump inhibitors associating drugs. J. Infect. Dev. Ctries 13, 162–164. doi: 10.3855/jidc.11102
Van Daele, R., Spriet, I., Wauters, J., Maertens, J., Mercier, T., Van Hecke, S., et al. (2019). Antifungal drugs: What brings the future? Med. Mycol 57, S328–S343. doi: 10.1093/mmy/myz012
Wang, C. H., Yu, J., Cai, Y. X., Zhu, P. P., Liu, C. Y., Zhao, A. C., et al. (2016). Characterization and functional analysis of 4-coumarate:CoA ligase genes in mul-berry. PloS One 11, e0155814. doi: 10.1371/journal.pone.0155814
Wiese, M. D., Rowland, A., Polasek, T. M., Sorich, M. J., O’doherty, C. (2013). Pharmacokinetic evaluation of teriflunomide for the treatment of multiple sclerosis. Expert Opin. Drug Metab. Toxicol. 9, 1025–1035. doi: 10.1517/17425255.2013.800483
Wong, S. S. W., Samaranayake, L. P., Seneviratne, C. J. (2014). In pursuit of the ideal antifungal agent for Candida infections: high-throughput screening of small molecules. Drug Discovery Today 19, 1721–1730. doi: 10.1016/j.drudis.2014.06.009
Keywords: teriflunomide, Candida albicans, fluconazole, drug combination, Galleria mellonella
Citation: Li X, Kong B, Sun Y, Sun F, Yang H and Zheng S (2023) Synergistic potential of teriflunomide with fluconazole against resistant Candida albicans in vitro and in vivo. Front. Cell. Infect. Microbiol. 13:1282320. doi: 10.3389/fcimb.2023.1282320
Received: 24 August 2023; Accepted: 05 December 2023;
Published: 19 December 2023.
Edited by:
Javier Alberto Garza Cervantes, Autonomous University of Nuevo León, MexicoReviewed by:
Julio Sempere, Carlos III Health Institute (ISCIII), SpainCopyright © 2023 Li, Kong, Sun, Sun, Yang and Zheng. This is an open-access article distributed under the terms of the Creative Commons Attribution License (CC BY). The use, distribution or reproduction in other forums is permitted, provided the original author(s) and the copyright owner(s) are credited and that the original publication in this journal is cited, in accordance with accepted academic practice. No use, distribution or reproduction is permitted which does not comply with these terms.
*Correspondence: Huijun Yang, bHd5aGpAMTYzLmNvbQ==; Shicun Zheng, c2N6aGVuZzA3MDFAMTYzLmNvbQ==
†These authors have contributed equally to this work
Disclaimer: All claims expressed in this article are solely those of the authors and do not necessarily represent those of their affiliated organizations, or those of the publisher, the editors and the reviewers. Any product that may be evaluated in this article or claim that may be made by its manufacturer is not guaranteed or endorsed by the publisher.
Research integrity at Frontiers
Learn more about the work of our research integrity team to safeguard the quality of each article we publish.