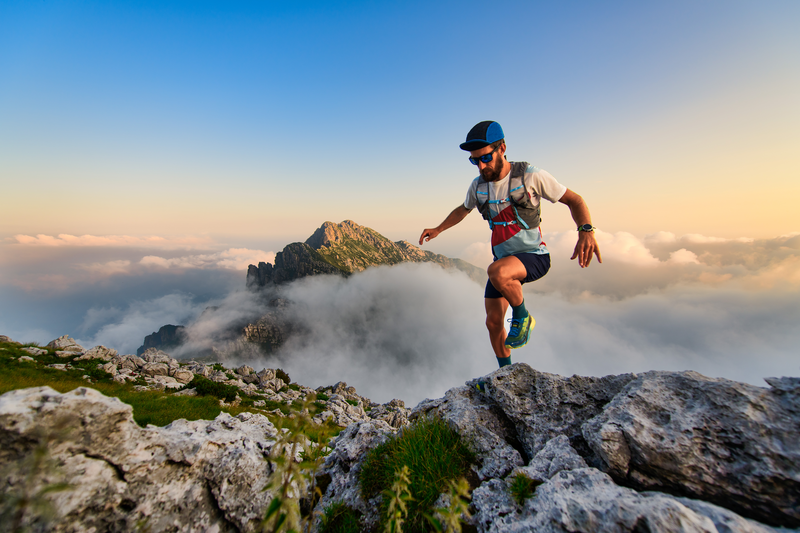
95% of researchers rate our articles as excellent or good
Learn more about the work of our research integrity team to safeguard the quality of each article we publish.
Find out more
REVIEW article
Front. Cell. Infect. Microbiol. , 24 October 2023
Sec. Intestinal Microbiome
Volume 13 - 2023 | https://doi.org/10.3389/fcimb.2023.1279172
This article is part of the Research Topic Recent Advances in Understanding the Role and Mechanisms of Gut Microbiota in Inflammatory Bowel Disease and Irritable Bowel Syndrome View all 5 articles
Inflammatory bowel disease (IBD) is a chronic inflammatory intestinal disease that affects more than 3.5 million people, with rising prevalence. It deeply affects patients’ daily life, increasing the burden on patients, families, and society. Presently, the etiology of IBD remains incompletely clarified, while emerging evidence has demonstrated that altered gut microbiota and decreased aryl hydrocarbon receptor (AHR) activity are closely associated with IBD. Furthermore, microbial metabolites are capable of AHR activation as AHR ligands, while the AHR, in turn, affects the microbiota through various pathways. In light of the complex connection among gut microbiota, the AHR, and IBD, it is urgent to review the latest research progress in this field. In this review, we describe the role of gut microbiota and AHR activation in IBD and discussed the crosstalk between gut microbiota and the AHR in the context of IBD. Taken as a whole, we propose new therapeutic strategies targeting the AHR–microbiota axis for IBD, even for other related diseases caused by AHR-microbiota dysbiosis.
As a gastrointestinal (GI) chronic, relapsing inflammatory disease, inflammatory bowel disease (IBD) can be divided into ulcerative colitis (UC) and Crohn’s disease (CD) according to clinical manifestations, endoscopic manifestations, and pathological results. It appears as abdominal pain, diarrhea, bloody stool, emaciation, and even extraintestinal symptoms (e.g., joint pain, and arthritis). It is characterized as relapsing and chronic, thus deeply affecting patients’ health, work, and daily life (Chang, 2020). In the late 20th century, IBD was considered a Western disease that mainly affected populations in North America and Europe, yet after entering the 21st century, the incidence and prevalence of IBD have risen worldwide, especially rapidly in the East (Mak et al., 2020). Although the etiology of IBD has not been fully elucidated, accumulating evidence revealed that the onset of IBD involves the complex interaction between genetic, environmental, microbial, and immune factors (Chang, 2020). Unfortunately, IBD remains incurable, which is greatly limited by the complex and unknown pathogenesis. Currently, the treatment for IBD includes the use of drugs and/or surgery. Regarding drug therapy, the suppression of the overactivated immunity and inflammation is the main drug action mechanism that non-steroidal drugs (NSAIDs), corticosteroids, immunosuppressants, and biological agents have been widely used for in the treatment of IBD (Nakase et al., 2021). However, the drug therapy of IBD is generally complicated, with obvious side effects, and poor tolerance which lead to about 15% of IBD patients worldwide being forced to undergo surgery each year (Le Berre et al., 2020) . Complex and limited therapeutic options further increase the physical and psychological burden of IBD patients instead.
Recently, increasing attention has been focused on the aryl hydrocarbon receptor (AHR) due to its role in the regulation of inflammation, immunity, intestinal barrier function, and intestinal microecology (Pernomian et al., 2020; Sun et al., 2020). The demonstration that the AHR modulates the differentiation and activity of Th1 and Th17 cell populations (Rodríguez-Sosa et al., 2005; Quintana et al., 2008; Quintana et al., 2012) further prompted us to examine the expression and role of the AHR in IBD. Reportedly, the AHR is highly expressed and highly activated in healthy intestinal epithelial cells (IECs) (de Vos et al., 2022) while lower levels of the AHR have been detected in IBD patients (Monteleone et al., 2011; Qiu and Zhou, 2013; Zhao et al., 2016). The pivotal role of the AHR in IBD has also been revealed by animal research (Furumatsu et al., 2011; Wang et al., 2018). Accordingly, AHR activity has been proposed as a potential therapeutic target for IBD. Several studies have confirmed that colitis can be alleviated by AHR ligands through activating the AHR (Hanieh, 2014; Lv et al., 2018a; Lv et al., 2018b; Yu et al., 2018; Marafini et al., 2019; Chen et al., 2020; Lin et al., 2023). However, most of the recognized exogenous synthetic AHR ligands have been associated with toxicity, such as 2,3,7,8-tetrachlorodibenzo-p-dioxin (TCDD) and polycyclic aromatic hydrocarbon (PAH) (Abudahab et al., 2023), which hamper the widespread application of AHR-ligand therapy and forces us to find non-toxic natural AHR ligands.
Compelling evidence has accumulated supporting the close association between gut microbiota and the development and maintenance of IBD (Lee and Chang, 2021). The human GI tract harbors an incredibly myriad microbiota that provide a platform for several biochemical metabolic reactions, which is considered a functional organ. Notably, an abundance of AHR ligands are accommodated in the GI tract, most of which are microbial metabolites (Pinto et al., 2023). The microbiota-derived AHR ligands include tryptophan metabolites, short-chain fatty acids (SCFAs), and phenolic microbial metabolites (Pernomian et al., 2020). In addition to the effects of the microbial community on AHR activity, the AHR could modulate the gut microbiota in turn (Pinto et al., 2023), that sufficient AHR ligands can rebalance the intestinal microecology and minimize mucosal inflammation to resist bacterial translocation (Esser, 2016). The mechanisms of AHR–microbiota interplay are multifactorial, including inflammatory response (Monteleone et al., 2013; Pernomian et al., 2020), immunologic balance (Monteleone et al., 2013; Pernomian et al., 2020), intestinal barrier integrity (Esser and Rannug, 2015), intestinal homeostasis (Qiu et al., 2012), and carcinogenesis (Murray et al., 2014).
In brief, accumulating evidence has demonstrated a crucial role for microbiota, as well as AHR activity in the pathogenesis of IBD. The research interest regarding the AHR–microbiota crosstalk in various diseases has been rising recently; the field has progressed rapidly and will undoubtedly continue to advance at a fast pace. In this review, we discuss the effects of gut microbiota, aryl hydrocarbon receptors, and their interactions on IBD depending on current evidence from clinical and animal experiments. More importantly, we aim to offer constructive strategies for the prevention and treatment of IBD via regulating the AHR–gut microbiota axis in the future.
The complex intestinal microecology, composed of trillions of diverse microorganisms that colonize the GI tract, is necessary for intestinal homeostasis (Arumugam et al., 2011). More than 1,000 microbial species residing in the GI tract have now been identified (Rajilić-Stojanović and de Vos, 2014). Firmicutes, Bacteroidetes, Actinobacteria, Fusobacteria, Proteobacteria, Verrucomicrobia, and Cyanobacteria are predominant divisions in the gut microenvironment, among which, Firmicutes and Bacteroidetes account for the majority of microbiota at the phyla level among healthy controls (HCs) (Gagliardi et al., 2018; Glassner et al., 2020). The gut microbiota is dynamic and widely involved in inflammation, immunity, metabolism, and other physiological processes, and exerts a significant influence on both the physical and mental health of individuals (Adak and Khan, 2019). With microbial qualitative or quantitative imbalance, namely, dysbiosis, various diseases can be triggered, such as cardiovascular diseases (Witkowski et al., 2020), autoimmune diseases (Christovich and Luo, 2022), gastrointestinal cancer (Meng et al., 2018), irritable bowel syndrome (IBS) (Canakis et al., 2020), and IBD (Lee and Chang, 2021).
Analyses of the composition of gut microbiota in IBD patients observed differences in specific intestinal bacterial species compared with HCs (Glassner et al., 2020; Haneishi et al., 2023). The Firmicutes/Bacteroidetes (F/B) ratio is widely considered to maintain intestinal homeostasis, which is widely decreased in IBD patients (Stojanov et al., 2020; Ma et al., 2021). Briefly, although the results vary among multiple studies, the consensus is toward dysbiosis in IBD mainly being characterized by the decreased beneficial microorganisms with anti-inflammatory capacities (e.g. Bacteroides, Bifidobacterium, Faecalibacterium prausnitzii, Suterella, Clostridium groups IV and XIVa, and Roseburia) (Mondot et al., 2011; Morgan et al., 2012; Quévrain et al., 2016; Zhou et al., 2018; Pittayanon et al., 2020; Zhou et al., 2021) together with increased bacteria with pro-inflammatory effects, such as Enterobacteriaceae (Escherichia coli and Fusobacterium (Palmela et al., 2018; Mirsepasi-Lauridsen et al., 2019)), Pasteurellaceae, Ruminococcus, and Veillonellaceae (Pittayanon et al., 2020). Helicobacter, Listeria, Klebsiella, Methanosphaera stadtmanae, Salmonella, and Yersinia were also demonstrated related to the onset of IBD (Wang et al., 2014). The abundance of SCFA-producing bacteria that possess anti-inflammatory abilities is also observed to be reduced in IBD, which consequently exacerbates symptoms of IBD (Liu et al., 2021; Haneishi et al., 2023). Other non-bacterial microbiota, such as archaeome, mycobiome, virome, and eukaryotic parasites, also change in IBD (Matijašić et al., 2020). Further, the microbial community also differs in UC and CD patients (Qin et al., 2010).
In addition to the specific taxa alterations, IBD patients are also characterized by a decreased total number of species and low microbial diversity (Mentella et al., 2020; Ma et al., 2021); even the disease activity and severity of IBD are associated with these alterations in the gut microbiota (Stojanov et al., 2020). Multiple genetic studies (especially genome-wide association research) have proposed the association between several IBD-related gene polymorphisms and microbial responses (Jostins et al., 2012). These findings all confirm the pivotal role of dysbiosis of gut microbiota in the pathophysiology of IBD. Microbiota have thus emerged as a potential target for IBD treatment that attracts increasing attention.
The AHR is a ligand-activated transcription factor, as well as a member of the basic helix-loop-helix/PER-ARNT-SIM (bHLH/PAS) family, ubiquitous in vertebrate cells (Kewley et al., 2004). It consists of an N-terminal bHLH domain, two PAS domains (PAS A and PAS B), and a C-terminal transactivation domain (TAD) where coactivators and corepressors interplay (Figure 1) (Stockinger et al., 2021). In the absence of ligands, the AHR resides in the cytoplasm in a transcriptionally inactive state as part of a complex with the chaperone proteins, known as two molecules of the heat shock protein 90 (HSP90), one molecule of the X-associated protein 2 (XAP2, also known as AIP), and one molecule of the HSP90 cochaperone p23 (Petrulis and Perdew, 2002). After ligand binding, the activated AHR translocates into the nucleus, where it dimerizes with its partner protein, the AHR nuclear translocator (ARNT), forming a functional transcription factor (Petrulis and Perdew, 2002). The AHR/ARNT complex then initiates the regulation of various target gene expressions by binding to the DNA of the entire genome, mainly the xenobiotic response element (XRE) (Quintana, 2013). The detailed process above can be seen in Figure 2.
Figure 1 Structures of the AHR. The AHR contains three major domains, the bHLH, the PAS, and the TAD. The bHLH domain located at the N-terminus is involved in interaction with chaperones, HSP90, and XAP2, dimerization with ARNT, and binding to DNA. The PAS domain includes the PAS-A and PAS-B, among which, the PAS-A is involved in dimerization with ARNT and interaction with HSP90 and XAP2, while the PAS-B mediates AHR interaction with ligands. The C-terminal located TAD consists of three subdomains, an acidic residue (glutamate/aspartate) rich subdomain, a glutamate-rich subdomain (Q-rich), and a P/S/T region rich in proline/serine/threonine residues that are responsible for interaction with cofactors and mediating transcriptional activation. Abbreviation: AHR, aryl hydrocarbon receptor; ARNT, aryl hydrocarbon receptor nuclear translocator; bHLH, basic helix-loop-helix; TAD, transactivation domain; PAS, period circadian protein (PER) Aryl Hydrocarbon Receptor Nuclear Translocator | single-minded protein (SIM); HSP90, heat shock protein 90; XAP2, X-associated protein 2.
Figure 2 Summary of the possible mechanism of AHR activation in the onset of IBD under the context of dysbiosis. The AHR is expressed by epithelial cells, endothelial cells, immune cells, and neurons of the gut. Upon microbial AHR ligand triggering, AHR activity maintains intestinal homeostasis involving multi-factors, including modulation of intestinal barrier integrity, immune and inflammatory responses, intestinal peristalsis and vascular function, and gut microbiota. Once the intestinal microecology is disturbed, the deficient AHR activation will be triggered, which may lead to increased microbial translocation, bacterial overgrowth, pro-inflammatory cytokines production, IELs activation, vascular leakage, collagen synthesis, and fibrosis, and decreased production of anti-inflammatory cytokines, anti-microbial peptides, Treg cells, goblet cells and mucus, and intestinal barrier damage, along with restriction of IEC proliferation and T cell differentiation and migration. These explain the role of AHR activation in the pathogenesis of IBD. Abbreviation: AHR, aryl hydrocarbon receptor; ARNT; aryl hydrocarbon receptor nuclear translocator; ENS, enteric nervous system; HSP90, heat shock protein 90; IBD, Inflammatory bowel disease; IECs, intestinal epithelial cells; IELs, intraepithelial lymphocytes; ILCs, innate lymphoid cells; XAP2, X-associated protein 2; XRE, xenobiotic response element.
The AHR can exert different effects by binding various exogenous and endogenous ligands with different structural and physicochemical properties (Denison and Nagy, 2003). However, the AHR activity is tightly controlled by three different negative feedback loops: (1) proteasomal degradation of the AHR induced by ubiquitin ligase complex; (2) metabolism clearance of ligands by Cytochrome P450 1A1 (CYP1A1); and (3) the interruption of the AHR/ARNT complex by the AHR repressor (AHRR) (Lamas et al., 2018b). In addition to these canonical signaling pathways, non-canonical mechanisms have also been revealed. During non-canonical signaling pathways, the AHR can: (1) interact with nuclear factor kappa-B (NF-κB), thus decreasing the expression of Cyp1A1 and increasing the expression of cytokines and chemokines including B-cell-activating factor of the tumor necrosis factor family (BAFF), B-lymphocyte chemoattractant (BLC), CC-chemokine ligand 1(CCL1), and interferon responsive factor (IFR3); (2) bind to Kruppel-like factor 6 (KLF6), which mediates cell cycle control; (3) combine hypophosphorylated retinoblastoma protein (pRb) to block cell cycle progression; and (4) interplay with the estrogen receptor (ER) and facilitate the proteolysis of the ER (Lamas et al., 2018b).
Overall, the ligand-activated AHR could regulate various gene expressions to maintain healthy cell physiology. Indeed, AHR ligands can be divided into exogenous (industrial synthesis and dietary), endogenous (indole and tryptophan metabolites), and other ligands (some chemicals, such as caffeine, nicotine, and pyridines) (Lamas et al., 2018b). Nowadays, searching for AHR ligands could be a novel strategy against IBD.
After a genome-wide linkage analysis of a large Danish family, Eiberg, H. et al (Eiberg et al., 2023). found that the AHR gene on the 7p-tel region was a candidate susceptibility gene for UC. Another study from Southeast China found an association between CD and AHR gene polymorphism in a group of patients that the genotypes of AHR (rs2158041) may be a CD susceptibility gene (especially for ileal CD and stricture CD) (Wu et al., 2019). These studies reasoned that the AHR gene may be the candidate susceptible gene for IBD. Consistently, diminished levels of AHR were observed in both colonic mucosa (Monteleone et al., 2011; Qiu and Zhou, 2013; Zhao et al., 2016) and fecal samples (Lamas et al., 2016) from IBD patients compared with HCs. Among these studies, the gene and protein expression levels of the AHR decreased markedly in the inflamed mucosa of CD patients compared with uninflamed tissues of HCs, but not in UC patients (Monteleone et al., 2011; Qiu and Zhou, 2013; Zhao et al., 2016). Additionally, decreased endogenous AHR ligand levels were reported in IBD patients (Hao et al., 2013; Esser, 2016; Lamas et al., 2016; Rothhammer et al., 2016). Monteleone, I. et al (Monteleone et al., 2011). observed no difference in terms of AHR activation between UC and HCs and suggested that the reduced AHR activation in CD is not an epiphenomenon of persistent inflammation. They also proved that the immune cells of IBD patients tend to express low AHR levels, especially in CD patients. Specifically, AHR expression was decreased in CD3+, CD4+, CD56+, and CD25+ cells through flow cytometry analysis of the LP mononuclear cells (Monteleone et al., 2011). Li, J et al (Li et al., 2016). subsequently made similar findings that the AHR expression in group 3 innate lymphoid cells (ILC3s) was lower in the inflamed intestinal biopsies of CD patients compared with unaffected tissues. Interestingly, different results have also been published. Arsenescu, R., et al (Arsenescu et al., 2011). reported a different result, finding that the colonic expression of the AHR was elevated in CD patients compared with HCs. The reasons for this discrepancy are unclear, which may partly be due to the different methods of AHR assessment and the smoking status of the study population in that the dioxins contained in cigarettes could induce the AHR.
Therefore, diminished AHR activity may be related to the onset and related symptoms of IBD, which has also been proved in animal studies. In murine models, AHR-deficient mice (AHR−/−) were more sensitive to dextran sodium sulfate (DSS)-induced colitis (Monteleone et al., 2011; Basu et al., 2012; Chinen et al., 2015; Schiering et al., 2017), showed higher levels of pro-inflammatory cytokines than wild-type (WT) (namely AHR+/+) controls, and more severe colitis-related symptoms and clinical outcomes (Takamura et al., 2010; Arsenescu et al., 2011; Furumatsu et al., 2011; Metidji et al., 2018; Wang et al., 2018). Accordingly, AHR agonists are capable of ameliorating colitis in mice (Cervantes-Barragan et al., 2017; Aoki et al., 2018), while AHR antagonists enhanced the severity of colitis (Monteleone et al., 2011). All these suggest that the AHR may play an important role in IBD, and the mechanisms involved in its protective role in maintaining intestinal homeostasis require more research.
It has been reported that the AHR is highly expressed in healthy colon cells (mainly epithelial cells and immune cells in the lamina propria) (de Vos et al., 2022), while the loss of AHR activity is related to inflammatory intestinal disorders (Qiu and Zhou, 2013; Wu et al., 2019; de Vos et al., 2022). Here, we elaborate on the current insights into the mechanism of the AHR in intestinal inflammation, immunity, barrier, motility, and vascular homeostasis that may count for the initiation of IBD. The latent mechanism is shown in Figure 2.
Reportedly, AHR-deficient mice displayed impaired intestinal gut barriers (Di Meglio et al., 2014), which indicates the protective effects of the AHR in sustaining intestinal barrier integrity. The IECs form a physical and biochemical barrier as the first innate protection against pathogen invasion. The AHR is highly expressed in IECs (Stockinger et al., 2011; Stange and Veldhoen, 2013) and exerts barrier-protective effects after activation by natural ligands (Stockinger et al., 2021). Foremost, AHR signaling is necessary for local stem cells to differentiate into IECs (Metidji et al., 2018). Further, the AHR could control the bacterial load (Li et al., 2011) and is capable of goblet cells and mucus production (Yin et al., 2019) which, once lacking, can lead to bacterial translocation. Additionally, the AHR could modulate tight junction (TJ) proteins to maintain the intestinal barrier. The DSS-induced colitis mouse model is characterized by a significant loss of TJs proteins (ZO-1, claudin-1, and occludin), which could be markedly reversed by administration of 6-formylindolo [3,2-b] carbazole (FICZ) (Park et al., 2015; Yu et al., 2018), an endogenous AHR ligand (Rannug et al., 1995). Moreover, antioxidant urolithin A could main the gut barrier and function through the intersection of the AHR and nuclear factor erythroid 2-related factor 2 gene (Nrf2)/NF-κB pathways (Singh et al., 2019). IL-22, targeting IECs, has been shown to promote IECs proliferation (Han et al., 2022), induce antibacterial proteins, and enhance the mucus barrier (Sugimoto et al., 2008; Zenewicz et al., 2008). AHR activation ensures the production of IL-22 to exert a wide variety of beneficial effects on the intestinal barrier (Lee and Lee, 2010; Han et al., 2022). The AHR could also control the intraepithelial lymphocytes (IELs) which are associated with the gut barrier and inflammation development thus maintaining the barrier integrity (Li et al., 2011; Ji et al., 2015). Another mechanism by which the AHR protects the intestinal barrier is by reducing colonic inflammation. Consistently, AHR-deficient mice are more susceptible to pathogens (Kimura et al., 2008; Lee and Lee, 2010; Kiss et al., 2011; Lee et al., 2011). After the administration of AHR agonists, researchers observed that the activated AHR could offset the dysfunction of the intestinal barrier induced by tumor necrosis factor-a (TNF-a) and interferon-gamma (IFN-γ) (Yamada et al., 2016; Yu et al., 2018).
Apart from the improved intestinal permeability, researchers have also found reduced colonic inflammation (e.g., IL-6, IL-1β, TNF-α) and overall disease activity index (DAI) after urolithin A therapy which active the AHR in 2, 4, 6trinitrobenzenesulphonic acid (TNBS)-induced colitis models (Singh et al., 2019). The potential modulatory role of the AHR on intestinal inflammation may be through suppressing the expression of pro-inflammatory cytokines such as TNF-α, IFN-γ, IL-6, IL-12, IL-7, IL-1β, and IL-17 while promoting the expression of anti-inflammatory cytokines such as IL-10 and IL-22 (Qiu and Zhou, 2013; Hanieh, 2014; Wang et al., 2018; Pernomian et al., 2020). What is more, upon AHR triggering, the microbial translocation and collagen synthesis or fibrosis could be restricted by increased anti-microbial peptides (Pernomian et al., 2020). The prospective role of the AHR as an inhibitor of NOD-like receptor thermal protein domain associated protein 3 (NLRP3) inflammasome (linked to bowel inflammation) for IBD is also worth noting (Ngui et al., 2020). Of note, AHR activation induced high amounts of IL-22 produced by innate lymphoid cells (ILCs) and CD4+ T cells, which enhance the intestinal barrier and induce anti-microbial peptides, thus restricting bacterial translocation in dysbiosis and reduce intestinal inflammation (Marafini et al., 2019; Chen et al., 2020; Pernomian et al., 2020). Additionally, AHR activation may constrain T cell responses, contributing to inflammation control (Monteleone et al., 2011; Lamas et al., 2018b). Th17/Treg imbalance promotes the pathogenesis of IBD (Quintana et al., 2008). As such, AHR ligands, including TCDD (Singh et al., 2011) and 3, 3′-diindolylmethane (DIM) (Huang et al., 2013), could restore the Th17/Treg ratio to alleviate experimental colitis by inhibiting Th17 proliferation and inducing Treg differentiation. Meanwhile, Th17 cells-produced IL-17, which is involved in acute and chronic inflammation, could be negatively regulated by the activated AHR pathway (Hao et al., 2013; Chng et al., 2016; Ye et al., 2017). Takamura et al. (2010) proposed that the inhibition of colitis by the AHR may be due to the increased production of Prostaglandin E2 (PGE2) and that the inhibition of PGE2 reduced the inhibitory effects of the AHR on colitis. These confirmed the positive effect of AHR activation on intestinal inflammation. Consistently, the AHR agonist medication attenuates colitis in mice models (Benson and Shepherd, 2011; Monteleone et al., 2011; Huang et al., 2013).
As expressed by different intestinal immune cells, including IELs, ILCs, Th17 cells, macrophages, dendritic cells (DCs), and neutrophils, the AHR is considered to participate in both innate and adaptive immune responses and regulates the immune homeostasis in the gut, which defends against infections (Qiu et al., 2013; Esser and Rannug, 2015; Lamas et al., 2018b). AHR ligands, including TCDD (Singh et al., 2011; Liu et al., 2020), FICZ (Qiu and Zhou, 2013; Hanieh, 2014; Wang et al., 2018), and Norisoboldine (NOR) (Lv et al., 2018a; Lv et al., 2018b), could ameliorate colitis symptoms by suppressing Th17 differentiation, leading to the increased IL-22 production, as well as decreased expression of IFN-γ and IL-17. NOR was also found to promote Treg cell differentiation by inhibiting Th1 differentiation (Tong et al., 2016; Lv et al., 2018b). Strikingly, the salient role of ligand-activated AHR in inducing tumor immune escape has been demonstrated by various studies (Ye et al., 2017; Cheong and Sun, 2018). Apart from the inhibited T cell responses by AHR activation (Kerkvliet et al., 1990), decreased lymphocyte accumulation in lymph nodes and the spleen (Fernandez-Salguero et al., 1995) and increased expression of pro-inflammatory cytokines (e.g., IL-12 and IFN-γ) in spleen cells (Rodríguez-Sosa et al., 2005) induced by AHR deficiency have also been reported. Kynurenine, another endogenous AHR ligand, is capable of regulating Treg cell functional maturation, combined with inhibiting inflammatory cytokine production thus altering the progression of inflammation and immunity balance (Mezrich et al., 2010; Díaz-Díaz et al., 2016). Another potent AHR agonist IPyA has exhibited remarkable anti-inflammatory effects in experimental colitis models through raised colonic IL10-positive T cells and lessening the pro-inflammatory Th1 cytokines (Aoki et al., 2018). Conversely, AHR antagonists elevated Th1 cytokines production and exacerbated the severity of TNBS-induced colitis in mice (Monteleone et al., 2011).
Surprisingly, the AHR expression and function in the enteric nervous system (ENS) were identified only 2 years ago (Stockinger et al., 2021). Adult mice studies showed that the sustained expression of the AHR in the ENS, which is restricted to colonic neurons, was found to be microbiota dependent (Obata et al., 2020). Serotonin, known as 5-hydroxytryptamine (5-HT), is in charge of GI motility, sensation, and secretion as a key neurotransmitter of the ENS (Spencer and Hu, 2020). Nevertheless, microbial metabolites promote 5-HT expression, in line with which, peristaltic defects were observed in germ-free mice due to the decreased 5-HT levels (Yano et al., 2015). However, by way of enhancing the induction of the AHR to Cyp1a1, 5-HT could influence the AHR activity in human IECs (Manzella et al., 2020). The AHR ENS neurons of mice showed delayed colonic transit, indicating colonic peristaltic activity is driven to some extent by ligand-dependent AHR activation (Obata et al., 2020). Obata, Y. et al. observed the expression of the AHR in the enteric neurons of mice treated with antibiotics partially restores intestinal motility (Obata et al., 2020). A previous dilated cardiomyopathy-related study showed that the promotion of peristalsis was partly attributed to AHR-mediated transcriptional upregulation of KCNJ12, a lipid-gated ion channel, which is responsible for encoding the primary subunit for IK1 (a key passage for cardiomyocytes). Recently, Zhizhu decoction has been proven to alleviate slow transit constipation by activating the AHR signaling pathway through gut microbiota (Wen et al., 2023). Interestingly, Vijay, A. et al. found that AHR activation affects nitrergic neuronal survival and delays intestinal motility in mice (Vijay et al., 2023). In brief, the AHR plays a regulatory role in intestinal motility, but the relevant mechanisms and studies are still limited and need to be further explored in the future.
Excessive angiogenesis is associated with the AHR in intestinal vascular homeostasis dysregulated repair responses, as a novel component of IBD pathogenesis (Pousa et al., 2008). Given the role of the AHR in IBD protection, along with growing evidence for the AHR in regulating vascular homeostasis, the function of the AHR in vascular remodeling and regeneration warrants further study. As reported, the involvement of laminar fluid shear stress represents a homeostatic function of AHR signaling in vascular tissue (Conway et al., 2009; Lano et al., 2020). The anti-inflammatory responses of AHR agonists in vascular tissue have been summarized previously (Bock, 2019). Recently, a study demonstrated for the first time that endothelial AHR ligand sensing is a key node in maintaining normal blood vessels of endothelial cell subtypes, thus regulating intestinal homeostasis (Wiggins et al., 2023). Further, they uncovered: 1) AHR ligands act directly on endothelial cells to promote quiescence and anti-inflammatory programs; 2) AHR facilitates vasculoprotective pathways in human endothelial cells (Wiggins et al., 2023). Although studies on the role of the AHR in the intestinal vasculature are limited, the AHR in endothelial cells has been presumed to potentially influence intestinal repair and regeneration upon injury, in which pro-inflammatory and angiogenic signals released by epithelial cells and immune cells at the injury site can trigger angiogenesis (Stockinger et al., 2021). Anti-inflammatory responses of AHR agonists in vascular tissue have been discussed in 2019 (Bock, 2019). In addition, the AHR in the vascular system is also involved in microbial defense (Stølevik et al., 2011).
Collectively, aside from the effect on the intestinal barrier, inflammation, immunity, motility, and vascular homeostasis, the AHR can also modulate the gut microbiota, which will be discussed in the next section.
The expression of the AHR and its target genes is lower in germ-free mice (Korecka et al., 2016). Also, caspase recruitment domain 9 (CARD9), one of the IBD susceptibility genes, links to AHR activation by interacting with the gut microbiota thus influencing the tryptophan (Trp) metabolism and IL-22 induction (Lamas et al., 2017). The microbiota from CARD9 knockout mice showed inhibited Trp metabolism, along with less production of AHR ligands IL-22, which eventually increased the risk of colitis (Rivas et al., 2011; Lamas et al., 2016; Lamas et al., 2017). Further, the microbiota of IBD patients with the risk allele of CARD9 also failed to adequately trigger the AHR activity, which may result in intestinal inflammation (Lamas et al., 2016). Hence, it is plausible to assume a relationship between the AHR and the gut microbiota in IBD.
As mentioned above, the AHR could control the bacterial load, induce anti-microbial peptides, and resist bacterial translocation (Takamura et al., 2010; Li et al., 2011; Esser, 2016; Marafini et al., 2019; Yin et al., 2019; Chen et al., 2020; Pernomian et al., 2020). Correspondingly, the diminished anti-microbial peptides induced by decreased AHR activity have been found rescued by FICZ treatment in mice (Wang et al., 2018). Through suppressing the neurogenic locus notch homolog protein 1 (Notch 1) signaling, the AHR can also produce more mucus to regulate the microbiome (Alvarado et al., 2019). Additionally, AHR expression has been shown to maintain specific ratios of various bacterial populations in the cecum, as well as modify the gut microbial community (Murray et al., 2016). For example, ILC3 from AHR-mediated IL-22 production was found to be critical in shaping the microbiota to mediate early colonization resistance to C. rodentium infection (Monteleone et al., 2011; Basu et al., 2012; Guo et al., 2015; Schiering et al., 2017). Furthermore, 2,3,7,8-tetrachlorodibenzofuran (TCDF) disrupted metabolism, altering the microbiota composition in mice by activating the AHR signaling (Zhang et al., 2015). Additionally, diets rich in AHR ligands influence the gut microbiota in mice. After feeding mice a diet lacking AHR ligands, researchers observed decreased abundance of Bacteroidetes, Actinobacteria, and Tenericutes in AHR-deficient mice, compared to WT mice (Korecka et al., 2016). They subsequently revealed that diets rich in AHR ligands upregulated the abundance of Firmicutes and downregulate the abundance of Bacteroidetes. The ability of the AHR to assess the relative abundance of bacterial communities has been described by several studies (Quintana et al., 2008; Kiss et al., 2011; Moura-Alves et al., 2014; Esser and Rannug, 2015; Moura-Alves et al., 2019). As a final note, delayed colonic transit induced by loss of the AHR in neurons leads to bacterial overgrowth in patients (Stockinger et al., 2021). Therefore, the AHR could modulate the gut microbiota (Pinto et al., 2023) and rebalance the intestinal microecology (Esser, 2016).
Both diminished levels of microbial AHR ligands and AHR activity were observed in fecal samples from IBD patients compared with HCs (Lamas et al., 2016). The role of the gut microbiota in providing AHR ligands has also been emphasized by many studies (Zelante et al., 2013; Hubbard et al., 2015; Lamas et al., 2016; Marinelli et al., 2019). Dong et al. (2020) observed alterations in AHR ligand production in germ-free mice. They identified both endogenous and microbiota-derived tryptophan metabolites present in mice cecal contents and human fecal samples, respectively, with the capacity to activate the AHR. These reveal the essential role of microbiota in AHR activity. Indeed, microbial derivatives including Trp metabolites and short-chain fatty acids (SCFAs) realized AHR activation. Precisely, Trp metabolites derived from microbiota represent an important source of endogenous AHR ligands (Sun et al., 2020), while SCFAs are essential for regulating the activation of the AHR induced by Trp-derived AHR ligands (Jin et al., 2017; Visekruna and Luu, 2021; Modoux et al., 2022). Several microbial Trp metabolites endowed with AHR agonist activity, including kynurenine, indole, serotonin, and tryptamine, are involved in the regulation of intestinal homeostasis (Zelante et al., 2013; Jin et al., 2014; Lamas et al., 2016; Liang et al., 2018; Kurata et al., 2019; Scott et al., 2020; Salminen, 2023). Moreover, a high-fat diet has been shown to alter the intestinal microbiome and its ability for metabolite production, such as tryptamine and indole-3-acetate (I3A), both of which are AHR agonists (Jin et al., 2014; Krishnan et al., 2018). Regarding microbiota-derived SCFAs, it not only increased the responsiveness and activation of the AHR (Jin et al., 2017; Marinelli et al., 2019) but also supported the growth of microorganisms with the capacity to metabolite Trp, which promotes AHR activity in B cells (Rosser et al., 2020). Reportedly, co-cultures of Lactobacillus acidophilus and Bacillus subtilis enhanced the mucosal barrier through bacterial SCFAs which upregulated the expression of the AHR and IL-22 (Xie et al., 2022). Gut microbiota-derived 3-phenylpropionic acid can also promote intestinal epithelial barrier function via AHR signaling (Hu et al., 2023).
To date, multiple bacteria species, including Bacteroides fragilis, Bacteroides thetaiotaomicron, Citrobacter sp (Chung et al., 1975)., Clostridia (Elsden et al., 1976), Clostridioides difficile (Lawson et al., 2016), Clostridium sporogenes (Dodd et al., 2017), and Lactobacillus reuteri (Natividad et al., 2018; Lamas et al., 2020; Bender et al., 2023) have been proved to produce the AHR agonist. Other microbial metabolites such as 2,8 dihydroxyquinoline have also been found to have capacity for AHR activation in human cells (Hubbard et al., 2019). Nevertheless, microorganisms may produce AHR antagonists, which could potentially affect AHR signaling locally and systemically in health and disease. A 2020 study examined the effect of 11 microbial tryptophan metabolites as AHR agonists/antagonists in detail (Vyhlídalová et al., 2020). Recently, both host and microbial-origin AHR ligands described as AHR agonists/antagonists have been summarized (Pinto et al., 2023), among which gut phenolic metabolite also exerts positive effects as an AHR modulator. Searching for AHR ligands could be a novel strategy against IBD (Pinto et al., 2023).
As discussed above, the AHR–microbiota axis in IBD has been brought to light. Both microbiota and AHR activity are deemed potential targets for IBD diagnosis and treatment. Nowadays, various therapies targeting the microbiome for IBD such as probiotics, antibiotics, fecal microbiota transplantation (FMT), defined enteral nutritional therapy (ENT), and gene manipulation have been discovered and demonstrated to have varying degrees of efficacy (Glassner et al., 2020). The positive effect of AHR agonists in colitis models has also been proved (Benson and Shepherd, 2011; Furumatsu et al., 2011; Monteleone et al., 2011; Singh et al., 2011; Huang et al., 2013; Qiu and Zhou, 2013; Hanieh, 2014; Ji et al., 2015; Park et al., 2015; Aoki et al., 2018; Lv et al., 2018a; Lv et al., 2018b; Wang et al., 2018; Yu et al., 2018; Marafini et al., 2019; Singh et al., 2019; Chen et al., 2020; Liu et al., 2020; Lin et al., 2023). However, both the markable immunotoxic effects and even carcinogenesis properties of most recognized exogenous synthetic AHR ligands have been elucidated (Murray et al., 2014; Stockinger et al., 2021; Abudahab et al., 2023; Chen et al., 2023), which limited clinical widespread application in IBD patients. Therefore, searching for non-toxic natural AHR ligands and exploring a series of potential mechanisms in the treatment of IBD has become a research hotspot in recent years.
Considering the AHR–microbiota axis of IBD, related therapies targeting the microbiota may achieve the remission of IBD targeting AHR activity. It has been proposed that insufficient microbial AHR agonists may contribute to the pathogenesis of IBD, metabolic syndrome, and other conditions (Lamas et al., 2016; Miani et al., 2018; Natividad et al., 2018). However, probiotics-produced AHR agonists have been successfully used to dampen inflammation in colitis. For instance, Akkermansia muciniphila, a promising probiotic, protects against colitis via activating AHR-Trp signaling (Gu et al., 2021a). Two Bifidobacterium bifidum strains, FL-276.1 and FL-228.1, were reported to ameliorate DSS-induced colitis by promoting the AHR pathway, which safeguards the barrier function (Cui et al., 2022; Cui et al., 2023). Similar results have also been reported in Lactobacillus rhamnosus (Luo et al., 2022; Niu et al., 2022), Bifidobacterium breve (Park et al., 2023), and co-cultures of Lactobacillus acidophilus and Bacillus subtilis (Xie et al., 2022). Additionally, Lactobacillus reuteri (Cervantes-Barragan et al., 2017; Özçam et al., 2019) and Lactobacillus bulgaricus strain OLL1181 (Perdew and Babbs, 1991; Takamura et al., 2010; Takamura et al., 2011) have been described as AHR activators both in mice models and human colonic samples. Other species including Propionibacterium freudenreichii ET-3, which is isolated from Swiss-type cheese, are also able to activate the AHR signaling, induced anti-microbial peptides, and improve DSS-induced colitis (Fukumoto et al., 2014). A recent study demonstrated three strains of Lactobacillus (L. murinus, L. reuteri, and L. taiwanensis) from feces of WT mice rescued impaired IL-22 production and high susceptibility to DSS of germ-free WT mice colonized by CARD9 deficiency microbiota (Lamas et al., 2016).
Beyond the probiotics, FMT also represents a novel therapy for IBD as an AHR promoter, yet is not fully clarified. After FMT from normal mice, the abundance of Bifidobacterium and Lactobacillus were augmented in colitis mice, along with the AHR expression and the levels of the anti-inflammatory cytokine, which revealed an association between the microorganisms and AHR activity, thus restoring the intestinal homeostasis and attenuated colitis (Wei et al., 2018). Withal, it is also possible to balance the gut microbiota through a diet rich in AHR ligands to relieve IBD patients (Daniel et al., 2014; Esser, 2016; Lamas et al., 2018a; Liang et al., 2018). After DSS gavage, both WT mice, which feed on a vegetable-free diet, and AHR-deficient mice displayed an increased abundance of Bacteroides and severe colitis (Li et al., 2011). However, these alterations can be attenuated by the adoption of vegetable-derived AHR ligands, which highlights the role of the AHR in the negative control of gut inflammation (Li et al., 2011). Aside from the reshaping of the gut microbiota, there are beneficial effects of long-lasting adherence to the Mediterranean diet for IBD patients also owing to the abundant specific components like phenolic metabolites, which are described as AHR agonists/antagonists thereby regulating AHR activity (Pinto et al., 2023).
Additionally, herbal medicine has shown potential efficacy for IBD regarding the AHR-microbiota axis. Berberine (Jing et al., 2021), Shenling Baizhu San (Lv et al., 2022), Gegen Qinlian decoction (GQD) (Wang et al., 2023), and baicalein (Li et al., 2022) can greatly prevent the intestinal barrier and inflammation in the animal colitis model induced by DSS by regulating the AHR–microbiota axis. Recently, a random-controlled 8-week trial showed the efficacy of natural indigo, a Chinese herb containing AHR ligands, for UC patients (Naganuma et al., 2018). The therapies targeting the AHR activity for IBD in the context of microbiota is shown in Figure 3.
Figure 3 Prospects in IBD therapy targeting the AHR–gut microbiota axis. Due to the altered AHR activity and microbiome in IBD, the detection of AHR activity and the microbiome may become a new method for the diagnosis of IBD in addition to endoscopy. Further, the dysbiosis of IBD patients can be reconstituted by diet, probiotics, FMT, and herbal medicine. Notably, the microbiome produced the tryptophan metabolites, short-chain fatty acids, and other metabolites that act as AHR agonists or inhibitors to regulate AHR activity. Thus, a restored healthy intestinal microbiome may upregulate the AHR activity based on the AHR–gut microbiota axis, thus alleviating IBD. Abbreviation: AHR, aryl hydrocarbon receptor; IBD, Inflammatory bowel disease; FMT, fecal microbiota transplantation.
The great potential of the AHR–microbiota axis in IBD is well discussed in the previous section. However, the emergence of the AHR–microbiota axis prompted us to expand insights into other GI diseases, and AHR activity-related diseases including Alzheimer’s disease (Salminen, 2023), arthritis (Rosser et al., 2020), cardiovascular disease (Paeslack et al., 2022), psoriasis (Qiao et al., 2022), and so on. Consistently, the positive effect of Lactobacillus rhamnosus GG in alcohol-associated liver disease (ALD) was associated with its bacterial AHR ligand-enriched exosome-like nanoparticles (ELNPs), which increased expression of Reg3 and Nrf2 thereby improving barrier function (Gu et al., 2021b). Additionally, Bacillus subtilis KC1 enhanced AHR activation thus alleviating lung injury (Chen et al., 2023). Bifidobacterium longum CCFM1029 reshaped the gut microbial composition in atopic dermatitis patients, and activated the AHR-mediated immune response, alleviating related symptoms (Fang et al., 2022). Moreover, the AHR activated by Lactobacillus reuteri helps alleviate Escherichia coli-induced mastitis in mice (Zhao et al., 2021). In addition to probiotics, supplementation with dietary AHR ligands could alleviate Escherichia coli-induced endometritis in mice (Zhao et al., 2022). Withal, tapinarof, a bacteria-derived AHR agonist, has recently been indicated to resolve skin inflammation in mice and humans (Smith et al., 2017). Likewise, quinolinic acid, an AHR agonist derived from skin microbiota, negatively regulates NLRP3 inflammasome through the AHR-Trp pathway in Psoriasis (Qiao et al., 2022). Furthermore, FMT upregulated the abundance of Lactobacillus (FMT vs Con; 84.98% vs 66.94%), which activated the AHR, thus increasing the expression of CYP1A2 and IL-22, maintaining Th17/Treg balance and immune homeostasis, improving chicken growth performance (Ma et al., 2023).
Herein, we speculated that the appropriate application of the microbiota-target therapies including diets, probiotics, herb medicine, and even FMT, maintains the homeostasis of the AHR activity and alleviates, treats, and prevents other diseases that are not limited to IBD.
Generally, as the potential mechanisms of IBD, there has been a recent surge in research interest towards gut microbiota and AHR activity, such as the AHR–microbiota axis. However, current research regarding the AHR–microbiota axis in IBD is limited, which may become the next research target. Notably, we described an in-depth insight into the potential therapeutic strategies for IBD but need clinical studies to be confirmed in the future. More than such, it also requires deeper knowledge in this field to expound on the modulation of the AHR–microbiota axis in human physiological homeostasis and other diseases.
J-JH: Conceptualization, Formal Analysis, Software, Visualization, Writing – original draft, Writing – review & editing. A-HM: Validation, Visualization, Writing – review & editing. Y-HQ: Conceptualization, Writing – review & editing.
The author(s) declare that no financial support was received for the research, authorship, and/or publication of this article.
The authors declare that the research was conducted in the absence of any commercial or financial relationships that could be construed as a potential conflict of interest.
All claims expressed in this article are solely those of the authors and do not necessarily represent those of their affiliated organizations, or those of the publisher, the editors and the reviewers. Any product that may be evaluated in this article, or claim that may be made by its manufacturer, is not guaranteed or endorsed by the publisher.
Abudahab, S., Price, E. T., Dozmorov, M. G., Deshpande, L. S., McClay, J. L. (2023). The aryl hydrocarbon receptor, epigenetics and the aging process. J. Nutr. Health Aging 27 (4), 291–300. doi: 10.1007/s12603-023-1908-1
Adak, A., Khan, M. R. (2019). An insight into gut microbiota and its functionalities. Cell Mol. Life Sci. 76 (3), 473–493. doi: 10.1007/s00018-018-2943-4
Alvarado, D. M., Chen, B., Iticovici, M., Thaker, A. I., Dai, N., VanDussen, K. L., et al. (2019). Epithelial indoleamine 2,3-dioxygenase 1 modulates aryl hydrocarbon receptor and notch signaling to increase differentiation of secretory cells and alter mucus-associated microbiota. Gastroenterology 157 (4), 1093–1108.e11. doi: 10.1053/j.gastro.2019.07.013
Aoki, R., Aoki-Yoshida, A., Suzuki, C., Takayama, Y. (2018). Indole-3-pyruvic acid, an aryl hydrocarbon receptor activator, suppresses experimental colitis in mice. J. Immunol. 201 (12), 3683–3693. doi: 10.4049/jimmunol.1701734
Arsenescu, R., Arsenescu, V., Zhong, J., Nasser, M., Melinte, R., Dingle, R. W., et al. (2011). Role of the xenobiotic receptor in inflammatory bowel disease. Inflammation Bowel Dis. 17 (5), 1149–1162. doi: 10.1002/ibd.21463
Arumugam, M., Raes, J., Pelletier, E., Le Paslier, D., Yamada, T., Mende, D. R., et al. (2011). Enterotypes of the human gut microbiome. Nature 473 (7346), 174–180. doi: 10.1038/nature09944
Basu, R., O'Quinn, D. B., Silberger, D. J., Schoeb, T. R., Fouser, L., Ouyang, W., et al. (2012). Th22 cells are an important source of IL-22 for host protection against enteropathogenic bacteria. Immunity 37 (6), 1061–1075. doi: 10.1016/j.immuni.2012.08.024
Bender, M. J., McPherson, A. C., Phelps, C. M., Pandey, S. P., Laughlin, C. R., Shapira, J. H., et al. (2023). Dietary tryptophan metabolite released by intratumoral Lactobacillus reuteri facilitates immune checkpoint inhibitor treatment. Cell 186 (9), 1846–1862.e26. doi: 10.1016/j.cell.2023.03.011
Benson, J. M., Shepherd, D. M. (2011). Aryl hydrocarbon receptor activation by TCDD reduces inflammation associated with Crohn's disease. Toxicol. Sci. 120 (1), 68–78. doi: 10.1093/toxsci/kfq360
Bock, K. W. (2019). Human AHR functions in vascular tissue: Pro- and anti-inflammatory responses of AHR agonists in atherosclerosis. Biochem. Pharmacol. 159, 116–120. doi: 10.1016/j.bcp.2018.11.021
Canakis, A., Haroon, M., Weber, H. C. (2020). Irritable bowel syndrome and gut microbiota. Curr. Opin. Endocrinol. Diabetes Obes. 27 (1), 28–35. doi: 10.1097/MED.0000000000000523
Cervantes-Barragan, L., Chai, J. N., Tianero, M. D., Di Luccia, B., Ahern, P. P., Merriman, J., et al. (2017). Lactobacillus reuteri induces gut intraepithelial CD4(+)CD8αα(+) T cells. Science 357 (6353), 806–810. doi: 10.1126/science.aah5825
Chang, J. T. (2020). Pathophysiology of inflammatory bowel diseases. N Engl. J. Med. 383 (27), 2652–2664. doi: 10.1056/NEJMra2002697
Chen, J., Haller, C. A., Jernigan, F. E., Koerner, S. K., Wong, D. J., Wang, Y., et al. (2020). Modulation of lymphocyte-mediated tissue repair by rational design of heterocyclic aryl hydrocarbon receptor agonists. Sci. Adv. 6 (3), eaay8230. doi: 10.1126/sciadv.aay8230
Chen, X., Ishfaq, M., Hu, F., Zheng, Q., Hu, X., Wang, J., et al. (2023). Bacillus subtilis KC1 prevents Mycoplasma gallisepticum-induced lung injury by enhancing intestinal Bifidobacterium animalis and regulating indole metabolism in chickens. Poult Sci. 102 (8), 102824. doi: 10.1016/j.psj.2023.102824
Chen, Y., Wang, Y., Fu, Y., Yin, Y., Xu, K. (2023). Modulating AHR function offers exciting therapeutic potential in gut immunity and inflammation. Cell Biosci. 13 (1), 85. doi: 10.1186/s13578-023-01046-y
Cheong, J. E., Sun, L. (2018). Targeting the IDO1/TDO2-KYN-ahR pathway for cancer immunotherapy - challenges and opportunities. Trends Pharmacol. Sci. 39 (3), 307–325. doi: 10.1016/j.tips.2017.11.007
Chinen, I., Nakahama, T., Kimura, A., Nguyen, N. T., Takemori, H., Kumagai, A., et al. (2015). The aryl hydrocarbon receptor/microRNA-212/132 axis in T cells regulates IL-10 production to maintain intestinal homeostasis. Int. Immunol. 27 (8), 405–415. doi: 10.1093/intimm/dxv015
Chng, S. H., Kundu, P., Dominguez-Brauer, C., Teo, W. L., Kawajiri, K., Fujii-Kuriyama, Y., et al. (2016). Ablating the aryl hydrocarbon receptor (AhR) in CD11c+ cells perturbs intestinal epithelium development and intestinal immunity. Sci. Rep. 6, 23820. doi: 10.1038/srep23820
Christovich, A., Luo, X. M. (2022). Gut microbiota, leaky gut, and autoimmune diseases. Front. Immunol. 13, 946248. doi: 10.3389/fimmu.2022.946248
Chung, K. T., Anderson, G. M., Fulk, G. E. (1975). Formation of indoleacetic acid by intestinal anaerobes. J. Bacteriol 124 (1), 573–575. doi: 10.1128/jb.124.1.573-575.1975
Conway, D. E., Sakurai, Y., Weiss, D., Vega, J. D., Taylor, W. R., Jo, H., et al. (2009). Expression of CYP1A1 and CYP1B1 in human endothelial cells: regulation by fluid shear stress. Cardiovasc. Res. 81 (4), 669–677. doi: 10.1093/cvr/cvn360
Cui, Q. Y., Zhang, Z., Tian, X., Liang, X., Lu, Y., Shi, Y., et al. (2022). Bifidobacterium bifidum relieved DSS-induced colitis in mice potentially by activating the aryl hydrocarbon receptor. Food Funct. 13 (9), 5115–5123. doi: 10.1039/D1FO04219J
Cui, Q., Tian, X. Y., Liang, X., Zhang, Z., Wang, R., Zhou, Y., et al. (2023). Bifidobacterium bifidum Ameliorates DSS-Induced Colitis in Mice by Regulating AHR/NRF2/NLRP3 Inflammasome Pathways through Indole-3-lactic Acid Production. J. Agric. Food Chem. 71 (4), 1970–1981. doi: 10.1021/acs.jafc.2c06894
Daniel, H., Gholami, A. M., Berry, D., Desmarchelier, C., Hahne, H., Loh, G., et al. (2014). High-fat diet alters gut microbiota physiology in mice. Isme J. 8 (2), 295–308. doi: 10.1038/ismej.2013.155
Denison, M. S., Nagy, S. R. (2003). Activation of the aryl hydrocarbon receptor by structurally diverse exogenous and endogenous chemicals. Annu. Rev. Pharmacol. Toxicol. 43, 309–334. doi: 10.1146/annurev.pharmtox.43.100901.135828
de Vos, W. M., Tilg, H., Van Hul, M., Cani, P.D.. (2022). Gut microbiome and health: mechanistic insights. Gut 71 (5), 1020–1032. doi: 10.1136/gutjnl-2021-326789
Díaz-Díaz, C. J., Ronnqklqiv-Kelly, S. M., Nukaya, M., Geiger, P. G., Balbo, S., Dator, R., et al. (2016). The aryl hydrocarbon receptor is a repressor of inflammation-associated colorectal tumorigenesis in mouse. Ann. Surg. 264 (3), 429–436. doi: 10.1097/SLA.0000000000001874
Di Meglio, P., Duarte, J. H., Ahlfors, H., Owens, N. D., Li, Y., Villanova, F., et al. (2014). Activation of the aryl hydrocarbon receptor dampens the severity of inflammatory skin conditions. Immunity 40 (6), 989–1001. doi: 10.1016/j.immuni.2014.04.019
Dodd, D., Spitzer, M. H., Van Treuren, W., Merrill, B. D., Hryckowian, A. J., Higginbottom, S. K., et al. (2017). A gut bacterial pathway metabolizes aromatic amino acids into nine circulating metabolites. Nature 551 (7682), 648–652. doi: 10.1038/nature24661
Dong, F., Hao, F., Murray, I. A., Smith, P. B., Koo, I., Tindall, A. M., et al. (2020). Intestinal microbiota-derived tryptophan metabolites are predictive of Ah receptor activity. Gut Microbes 12 (1), 1–24. doi: 10.1080/19490976.2020.1788899
Eiberg, H., Olsson, J. B., Bak, M., Bang-Berthelsen, C. H., Troelsen, J. T., Hansen, L. (2023). A family with ulcerative colitis maps to 7p21.1 and comprises a region with regulatory activity for the aryl hydrocarbon receptor gene. Eur. J. Hum. Genet. doi: 10.1038/s41431-023-01298-9
Elsden, S. R., Hilton, M. G., Waller, J. M. (1976). The end products of the metabolism of aromatic amino acids by Clostridia. Arch. Microbiol. 107 (3), 283–288. doi: 10.1007/BF00425340
Esser, C. (2016). The aryl hydrocarbon receptor in immunity: tools and potential. Methods Mol. Biol. 1371, 239–257. doi: 10.1007/978-1-4939-3139-2_16
Esser, C., Rannug, A. (2015). The aryl hydrocarbon receptor in barrier organ physiology, immunology, and toxicology. Pharmacol. Rev. 67 (2), 259–279. doi: 10.1124/pr.114.009001
Fang, Z., Pan, T., Li, L., Wang, H., Zhu, J., Zhang, H., et al. (2022). Bifidobacterium longum mediated tryptophan metabolism to improve atopic dermatitis via the gut-skin axis. Gut Microbes 14 (1), 2044723. doi: 10.1080/19490976.2022.2044723
Fernandez-Salguero, P., Pineau, T., Hilbert, D. M., McPhail, T., Lee, S. S., Kimura, S., et al. (1995). Immune system impairment and hepatic fibrosis in mice lacking the dioxin-binding Ah receptor. Science 268 (5211), 722–726. doi: 10.1126/science.7732381
Fukumoto, S., Toshimitsu, T., Matsuoka, S., Maruyama, A., Oh-Oka, K., Takamura, T., et al. (2014). Identification of a probiotic bacteria-derived activator of the aryl hydrocarbon receptor that inhibits colitis. Immunol. Cell Biol. 92 (5), 460–465. doi: 10.1038/icb.2014.2
Furumatsu, K., Nishiumi, S., Kawano, Y., Ooi, M., Yoshie, T., Shiomi, Y., et al. (2011). A role of the aryl hydrocarbon receptor in attenuation of colitis. Dig Dis. Sci. 56 (9), 2532–2544. doi: 10.1007/s10620-011-1643-9
Gagliardi, A., Totino, V., Cacciotti, F., Iebba, V., Neroni, B., Bonfiglio, G., et al. (2018). Rebuilding the gut microbiota ecosystem. Int. J. Environ. Res. Public Health 15 (8). doi: 10.3390/ijerph15081679
Glassner, K. L., Abraham, B. P., Quigley, E. M. M. (2020). The microbiome and inflammatory bowel disease. J. Allergy Clin. Immunol. 145 (1), 16–27. doi: 10.1016/j.jaci.2019.11.003
Gu, Z., Li, F., Liu, Y., Jiang, M., Zhang, L., He, L., et al. (2021a). Akkermansia muciniphila and its outer protein Amuc_1100 regulates tryptophan metabolism in colitis. Food Funct. 12 (20), 10184–10195. doi: 10.1039/D1FO02172A
Gu, Z., Pei, W., Shen, Y., Wang, L., Zhu, J., Zhang, Y., et al. (2021b). Exosome-like nanoparticles from lactobacillus rhamnosusGG protect against alcohol-associated liver disease through intestinal aryl hydrocarbon receptor in mice. Hepatol. Commun. 5 (5), 846–864. doi: 10.1002/hep4.1679
Guo, X., Liang, Y., Zhang, Y., Lasorella, A., Kee, B. L., Fu, Y. X. (2015). Innate Lymphoid Cells Control Early Colonization Resistance against Intestinal Pathogens through ID2-Dependent Regulation of the Microbiota. Immunity 42 (4), 731–743. doi: 10.1016/j.immuni.2015.03.012
Han, H., Davidson, L. A., Fan, Y. Y., Landrock, K. K., Jayaraman, A., Safq, S. H., et al. (2022). Loss of aryl hydrocarbon receptor suppresses the response of colonic epithelial cells to IL22 signaling by upregulating SOCS3. Am. J. Physiol. Gastrointest Liver Physiol. 322 (1), G93–g106. doi: 10.1152/ajpgi.00074.2021
Haneishi, Y., Furuya, Y., Hasqgawa, M., Picarqlli, A., Rossi, M., Miyamoto, J. (2023). Inflammatory bowel diseases and gut microbiota. Int. J. Mol. Sci. 24 (4). doi: 10.3390/ijms24043817
Hanieh, H. (2014). Toward understanding the role of aryl hydrocarbon receptor in the immune system: current progress and future trends. BioMed. Res. Int 2014 p, 520763. doi: 10.1155/2014/520763
Hao, K., Zhou, Q., Chqn, W., Jia, W., Zhqng, J., Kang, J., et al. (2013). Possible role of the 'IDO-AhR axis' in maternal-foetal tolerance. Cell Biol. Int. 37 (2), 105–108. doi: 10.1002/cbin.10023
Hu, J., Chqn, J., Xu, X., Hou, Q., Rqn, J., Yan, X. (2023). Gut microbiota-derived 3-phenylpropionic acid promotes intestinal epithelial barrier function via AhR signaling. Microbiome 11 (1), 102. doi: 10.1186/s40168-023-01551-9
Huang, Z., Jiang, Y., Yang, Y., Shao, J., Sun, X., Chqn, J., et al. (2013). 3,3'-Diindolylmethane alleviates oxazolone-induced colitis through Th2/Th17 suppression and Treg induction. Mol. Immunol. 53 (4), 335–344. doi: 10.1016/j.molimm.2012.09.007
Hubbard, T. D., Liu, Q., Murray, I. A., Dong, F., Millqr, C., 3rd, Smith, P. B., et al. (2019). Microbiota metabolism promotes synthesis of the human ah receptor agonist 2,8-dihydroxyquinoline. J. Proteome Res. 18 (4), 1715–1724. doi: 10.1021/acs.jproteome.8b00946
Hubbard, T. D., Murray, I. A., Perdew, G. H. (2015). Indole and tryptophan metabolism: endogenous and dietary routes to ah receptor activation. Drug Metab. Dispos 43 (10), 1522–1535. doi: 10.1124/dmd.115.064246
Ji, T., Xu, C., Sun, L., Yu, M., Pqng, K., Qiu, Y., et al. (2015). Aryl hydrocarbon receptor activation down-regulates IL-7 and reduces inflammation in a mouse model of DSS-induced colitis. Dig Dis. Sci. 60 (7), 1958–1966. doi: 10.1007/s10620-015-3632-x
Jin, U. H., Cheng, Y., Park, H., Davidson, L. A., Callaway, E. S., Chapkin, R. S., et al. (2014). Microbiome-derived tryptophan metabolites and their aryl hydrocarbon receptor-dependent agonist and antagonist activities. Mol. Pharmacol. 85 (5), 777–788. doi: 10.1124/mol.113.091165
Jin, U. H., Lee, SO, Sridharan, G, Lee, K, Davidson, LA, Jayaraman, A, et al. (2017). Short chain fatty acids enhance aryl hydrocarbon (Ah) responsiveness in mouse colonocytes and caco-2 human colon cancer cells. Sci. Rep. 7 (1), 10163. doi: 10.1038/s41598-017-10824-x
Jing, W., Dong, S., Luo, X., Liu, J., WQi , B., Du, W., et al. (2021). Berberine improves colitis by triggering AhR activation by microbial tryptophan catabolites. Pharmacol. Res. 164, 105358. doi: 10.1016/j.phrs.2020.105358
Jostins, L., Ripke, S., Weersma, R. K., Duerr, R. H., McGovern, D. P., Hui, K. Y., et al. (2012). Host-microbe interactions have shaped the genetic architecture of inflammatory bowel disease. Nature 491 (7422), 119–124. doi: 10.1038/nature11582
Kerkvliet, N. I., Baecher-Steppan, L., Smith, B. B., Youngberg, J. A., Henderson, M. C., Buhler, D. R., et al. (1990). Role of the Ah locus in suppression of cytotoxic T lymphocyte activity by halogenated aromatic hydrocarbons (PCBs and TCDD): structure-activity relationships and effects in C57Bl/6 mice congenic at the Ah locus. Fundam Appl. Toxicol. 14 (3), 532–541. doi: 10.1016/0272-0590(90)90257-K
Kewley, R. J., Whitelaw, M. L., Chapman-Smith, A. (2004). The mammalian basic helix-loop-helix/PAS family of transcriptional regulators. Int. J. Biochem. Cell Biol. 36 (2), 189–204. doi: 10.1016/S1357-2725(03)00211-5
Kimura, A., Naka, T., Nohara, K., Fujii-Kuriyama, Y., Kishimoto, T. (2008). Aryl hydrocarbon receptor regulates Stat1 activation and participates in the development of Th17 cells. Proc. Natl. Acad. Sci. U.S.A. 105 (28), 9721–9726. doi: 10.1073/pnas.0804231105
Kiss, E. A., Vonarbourg, C., Kopfmann, S., Hobeika, E., Finke, D., Esser, C., et al. (2011). Natural aryl hydrocarbon receptor ligands control organogenesis of intestinal lymphoid follicles. Science 334 (6062), 1561–1565. doi: 10.1126/science.1214914
Korecka, A., Dona, A., Lahiri, S., Tett, A. J., Al-Asmakh, M., Braniste, V., et al. (2016). Bidirectional communication between the Aryl hydrocarbon Receptor (AhR) and the microbiome tunes host metabolism. NPJ Biofilms Microbiomes 2, 16014. doi: 10.1038/npjbiofilms.2016.14
Krishnan, S., Ding, Y., Saedi, N., Choi, M., Sridharan, G. V., Sherr, D. H., et al. (2018). Gut microbiota-derived tryptophan metabolites modulate inflammatory response in hepatocytes and macrophages. Cell Rep. 23 (4), 1099–1111. doi: 10.1016/j.celrep.2018.03.109
Kurata, K., Kawahara, H., Nishimura, K., Jisaka, M., Yokota, K., Shimizu, H. (2019). Skatole regulates intestinal epithelial cellular functions through activating aryl hydrocarbon receptors and p38. Biochem. Biophys. Res. Commun. 510 (4), 649–655. doi: 10.1016/j.bbrc.2019.01.122
Lamas, B., Richard, M. L., Leducq, V., Pham, H. P., Michel, M. L., Da Costa, G., et al. (2016). CARD9 impacts colitis by altering gut microbiota metabolism of tryptophan into aryl hydrocarbon receptor ligands. Nat. Med. 22 (6), 598–605. doi: 10.1038/nm.4102
Lamas, B., Michel, M. L., Waldschmitt, N., Pham, H. P., Zacharioudaki, V., Dupraz, L., et al. (2018a). Card9 mediates susceptibility to intestinal pathogens through microbiota modulation and control of bacterial virulence. Gut 67 (10), 1836–1844. doi: 10.1136/gutjnl-2017-314195
Lamas, B., Hernandez-Galan, L., Galipeau, H. J., Constante, M., Clarizio, A., Jury, J., et al. (2020). Aryl hydrocarbon receptor ligand production by the gut microbiota is decreased in celiac disease leading to intestinal inflammation. Sci. Transl. Med. 12 (566). doi: 10.1126/scitranslmed.aba0624
Lamas, B., Natividad, J. M., Sokol, H. (2018b). Aryl hydrocarbon receptor and intestinal immunity. Mucosal Immunol. 11 (4), 1024–1038. doi: 10.1038/s41385-018-0019-2
Lamas, B., Richard, M. L., Sokol, H. (2017). Caspase recruitment domain 9, microbiota, and tryptophan metabolism: dangerous liaisons in inflammatory bowel diseases. Curr. Opin. Clin. Nutr. Metab. Care 20 (4), 243–247. doi: 10.1097/MCO.0000000000000382
Lano, G., Laforêt, M., Von Kotze, C., Perrin, J., Addi, T., Brunet, P., et al. (2020). Aryl hydrocarbon receptor activation and tissue factor induction by fluid shear stress and indoxyl sulfate in endothelial cells. Int. J. Mol. Sci. 21 (7). doi: 10.3390/ijms21072392
Lawson, P. A., Citron, D. M., Tyrrell, K. L., Finegold, S. M. (2016). Reclassification of Clostridium difficile as Clostridioides difficile (Hall and O'Toole 1935) Prévot 1938. Anaerobe 40, 95–99. doi: 10.1016/j.anaerobe.2016.06.008
Le Berre, C., Ananthakrishnan, A. N., Danese, S., Singh, S., Peyrin-Biroulet, L. (2020). Ulcerative colitis and crohn's disease have similar burden and goals for treatment. Clin. Gastroenterol. Hepatol. 18 (1), 14–23. doi: 10.1016/j.cgh.2019.07.005
Lee, M., Chang, E. B. (2021). Inflammatory bowel diseases (IBD) and the microbiome-searching the crime scene for clues. Gastroenterology 160 (2), 524–537. doi: 10.1053/j.gastro.2020.09.056
Lee, J. S., Cella, M., McDonald, K. G., Garlanda, C., Kennedy, G. D., Nukaya, M., et al. (2011). AHR drives the development of gut ILC22 cells and postnatal lymphoid tissues via pathways dependent on and independent of Notch. Nat. Immunol. 13 (2), 144–151. doi: 10.1038/ni.2187
Lee, J. H., Lee, J. (2010). Indole as an intercellular signal in microbial communities. FEMS Microbiol. Rev. 34 (4), 426–444. doi: 10.1111/j.1574-6976.2009.00204.x
Li, J., Doty, A., Glover, S. C. (2016). Aryl hydrocarbon receptor signaling involves in the human intestinal ILC3/ILC1 conversion in the inflamed terminal ileum of Crohn's disease patients. Inflammation Cell Signal 3 (3). doi: 10.14800/ics.1404
Li, Y., Innocentin, S., Withers, D. R., Roberts, N. A., Gallagher, A. R., Grigorieva, E. F., et al. (2011). Exogenous stimuli maintain intraepithelial lymphocytes via aryl hydrocarbon receptor activation. Cell 147 (3), 629–640. doi: 10.1016/j.cell.2011.09.025
Li, Y. Y., Wang, X. J., Su, Y. L., Wang, Q., Huang, S. W., Pan, Z. F., et al. (2022). Baicalein ameliorates ulcerative colitis by improving intestinal epithelial barrier via AhR/IL-22 pathway in ILC3s. Acta Pharmacol. Sin. 43 (6), 1495–1507. doi: 10.1038/s41401-021-00781-7
Liang, H., Dai, Z., Liu, N., Ji, Y., Chen, J., Zhang, Y., et al. (2018). Dietary L-tryptophan modulates the structural and functional composition of the intestinal microbiome in weaned piglets. Front. Microbiol. 9, 1736. doi: 10.3389/fmicb.2018.01736
Lin, L., Liu, Y., Chen, L., Dai, Y., Xia, Y.. (2023). Discovery of norisoboldine analogue III(11) as a novel and potent aryl hydrocarbon receptor agonist for the treatment of ulcerative colitis. J. Med. Chem. 66 (10), 6869–6888. doi: 10.1021/acs.jmedchem.3c00287
Liu, C., Li, Y., Chen, Y., Huang, S., Wang, X., Luo, S., et al. (2020). Baicalein restores the balance of th17/treg cells via aryl hydrocarbon receptor to attenuate colitis. Mediators Inflamm 2020 p, 5918587. doi: 10.1155/2020/5918587
Liu, S., Zhao, W., Lan, P., Mou, X.. (2021). The microbiome in inflammatory bowel diseases: from pathogenesis to therapy. Protein Cell 12 (5), 331–345. doi: 10.1007/s13238-020-00745-3
Luo, M., Luo, D., Liu, J., Wang, H., Liu, X., Yang, M., et al. (2022). Ameliorative effect of the probiotic peptide against benzo(α)pyrene-induced inflammatory damages in enterocytes. Int. Immunopharmacol 112, 109255. doi: 10.1016/j.intimp.2022.109255
Lv, Q., Ma, Y. M., Huang, J. Y., He, S. Q., Li, S. P., Lin, J., et al. (2018a). Norisoboldine, a natural aryl hydrocarbon receptor agonist, alleviates TNBS-induced colitis in mice, by inhibiting the activation of NLRP3 inflammasome. Chin. J. Nat. Med. 16 (3), 161–174. doi: 10.1016/S1875-5364(18)30044-X
Lv, Q., Wang, K., Qiao, S., Yang, L., Xin, Y., Dai, Y., et al. (2018b). Norisoboldine, a natural AhR agonist, promotes Treg differentiation and attenuates colitis via targeting glycolysis and subsequent NAD(+)/SIRT1/SUV39H1/H3K9me3 signaling pathway. Cell Death Dis. 9 (3), 258. doi: 10.1038/s41419-018-0297-3
Lv, W. J., Ma, Y. M., Huang, J. Y., He, S. Q., Li, S. P., Lin, J., et al. (2022). Polysaccharides derived from Shenling Baizhu San improve colitis via modulating tryptophan metabolism in mice. Int. J. Biol. Macromol 222 (Pt A), 1127–1136. doi: 10.1016/j.ijbiomac.2022.09.246
Ma, Y., Zhang, Y., Xiang, J., Xiang, S., Zhao, Y., Xiao, M., et al. (2021). Metagenome analysis of intestinal bacteria in healthy people, patients with inflammatory bowel disease and colorectal cancer. Front. Cell Infect. Microbiol. 11, 599734. doi: 10.3389/fcimb.2021.599734
Ma, Z., Akhtar, M., Pan, H., Liu, Q., Chen, Y., Zhou, X., et al. (2023). Fecal microbiota transplantation improves chicken growth performance by balancing jejunal Th17/Treg cells. Microbiome 11 (1), 137. doi: 10.1186/s40168-023-01569-z
Mak, W. Y., Zhao, M., Ng, S. C., Burisch, J.. (2020). The epidemiology of inflammatory bowel disease: East meets west. J. Gastroenterol. Hepatol. 35 (3), 380–389. doi: 10.1111/jgh.14872
Manzella, C. R., Ackerman, M., Singhal, M., Ticho, A. L., Ceh, J., Alrefai, W. A., et al. (2020). Serotonin modulates ahR activation by interfering with CYP1A1-mediated clearance of ahR ligands. Cell Physiol. Biochem. 54 (1), 126–141. doi: 10.33594/000000209
Marafini, I., Di Fusco, D., Dinallo, V., Franzè, E., Stolfi, C., Sica, G., et al. (2019). NPD-0414-2 and NPD-0414-24, two chemical entities designed as aryl hydrocarbon receptor (AhR) ligands, inhibit gut inflammatory signals. Front. Pharmacol. 10, 380. doi: 10.3389/fphar.2019.00380
Marinelli, L., Martin-Gallausiaux, C., Bourhis, J. M., Béguet-Crespel, F., Blottière, H. M., Lapaque, N. (2019). Identification of the novel role of butyrate as AhR ligand in human intestinal epithelial cells. Sci. Rep. 9 (1), 643.
Matijašić, M., Meštrović, T., Paljetak, H., Perić, M., Barešić, A., Verbanac, D.. (2020). Gut microbiota beyond bacteria-mycobiome, virome, archaeome, and eukaryotic parasites in IBD. Int. J. Mol. Sci. 21 (8). doi: 10.3390/ijms21082668
Meng, C., Bai, C., Brown, T. D., Hood, L. E., Tian, Q. (2018). Human gut microbiota and gastrointestinal cancer. Genomics Proteomics Bioinf. 16 (1), 33–49. doi: 10.1016/j.gpb.2017.06.002
Mentella, M. C., Scaldaferri, F., Pizzoferrato, M., Gasbarrini, A., Miggiano, G. A.D.. (2020). Nutrition, IBD and gut microbiota: A review. Nutrients 12 (4). doi: 10.3390/nu12040944
Metidji, A., Omenetti, S., Crotta, S., Li, Y., Nye, E., Ross, E., et al. (2018). The environmental sensor AHR protects from inflammatory damage by maintaining intestinal stem cell homeostasis and barrier integrity. Immunity 49 (2), 353–362.e5. doi: 10.1016/j.immuni.2018.07.010
Mezrich, J. D., Fechner, J. H., Zhang, X., Johnson, B. P., Burlingham, W. J., Bradfield, C.A. (2010). An interaction between kynurenine and the aryl hydrocarbon receptor can generate regulatory T cells. J. Immunol. 185 (6), 3190–3198. doi: 10.4049/jimmunol.0903670
Miani, M., Le Naour, J., Waeckel-Enée, E., Verma, S. C., Straube, M., Emond, P., et al. (2018). Gut microbiota-stimulated innate lymphoid cells support β-defensin 14 expression in pancreatic endocrine cells, preventing autoimmune diabetes. Cell Metab. 28 (4), 557–572.e6. doi: 10.1016/j.cmet.2018.06.012
Mirsepasi-Lauridsen, H. C., Vallance, B. A., Krogfelt, K. A., Petersen, A. M.. (2019). Escherichia coli pathobionts associated with inflammatory bowel disease. Clin. Microbiol. Rev. 32 (2). doi: 10.1128/CMR.00060-18
Modoux, M., Rolhion, N., Lefevre, J. H., Oeuvray, C., Nádvorník, P., Illes, P., et al. (2022). Butyrate acts through HDAC inhibition to enhance aryl hydrocarbon receptor activation by gut microbiota-derived ligands. Gut Microbes 14 (1), 2105637. doi: 10.1080/19490976.2022.2105637
Mondot, S., Kang, S., Furet, J. P., Aguirre de Carcer, D., McSweeney, C., Morrison, M., et al. (2011). Highlighting new phylogenetic specificities of Crohn's disease microbiota. Inflammation Bowel Dis. 17 (1), 185–192. doi: 10.1002/ibd.21436
Monteleone, I., Rizzo, A., Sarra, M., Sica, G., Sileri, P., Biancone, L., et al. (2011). Aryl hydrocarbon receptor-induced signals up-regulate IL-22 production and inhibit inflammation in the gastrointestinal tract. Gastroenterology 141 (1), 237–248. doi: 10.1053/j.gastro.2011.04.007
Monteleone, I., Pallone, F., Monteleone, G. (2013). Aryl hydrocarbon receptor and colitis. Semin. Immunopathol. 35 (6), 671–675. doi: 10.1007/s00281-013-0396-2
Morgan, X. C., Tickle, T. L., Sokol, H., Gevers, D., Devaney, K. L., Ward, D. V., et al. (2012). Dysfunction of the intestinal microbiome in inflammatory bowel disease and treatment. Genome Biol. 13 (9), R79. doi: 10.1186/gb-2012-13-9-r79
Moura-Alves, P., Faé, K., Houthuys, E., Dorhoi, A., Kreuchwig, A., Furkert, J., et al. (2014). AhR sensing of bacterial pigments regulates antibacterial defence. Nature 512 (7515), 387–392. doi: 10.1038/nature13684
Moura-Alves, P., Puyskens, A., Stinn, A., Klemm, M., Guhlich-Bornhof, U., Dorhoi, A., et al. (2019). Host monitoring of quorum sensing during Pseudomonas aeruginosa infection. Science 366 (6472). doi: 10.1126/science.aaw1629
Murray, I. A., Nichols, R. G., Zhang, L., Patterson, A. D., Perdew, G. H.. (2016). Expression of the aryl hydrocarbon receptor contributes to the establishment of intestinal microbial community structure in mice. Sci. Rep. 6, 33969. doi: 10.1038/srep33969
Murray, I. A., Patterson, A. D., Perdew, G. H. (2014). Aryl hydrocarbon receptor ligands in cancer: friend and foe. Nat. Rev. Cancer 14 (12), 801–814. doi: 10.1038/nrc3846
Naganuma, M., Patterson, A. D., Perdew, G. H. (2018). Efficacy of indigo naturalis in a multicenter randomized controlled trial of patients with ulcerative colitis. Gastroenterology 154 (4), 935–947. doi: 10.1053/j.gastro.2017.11.024
Nakase, H., Uchino, M., Shinzaki, S., Matsuura, M., Matsuoka, K., Kobayashi, T., et al. (2021). Evidence-based clinical practice guidelines for inflammatory bowel disease 2020. J. Gastroenterol. 56 (6), 489–526. doi: 10.1007/s00535-021-01784-1
Natividad, J. M., Agus, A., Planchais, J., Lamas, B., Jarry, A. C., Martin, R., et al. (2018). Impaired aryl hydrocarbon receptor ligand production by the gut microbiota is a key factor in metabolic syndrome. Cell Metab. 28 (5), 737–749.e4. doi: 10.1016/j.cmet.2018.07.001
Ngui, I. Q. H., Perera, A. P., Eri, R. (2020). Does NLRP3 inflammasome and aryl hydrocarbon receptor play an interlinked role in bowel inflammation and colitis-associated colorectal cancer? Molecules 25 (10). doi: 10.3390/molecules25102427
Niu, H., Zhou, X., Gong, P., Jiao, Y., Zhang, J., Wu, Y., et al. (2022). Effect of lactobacillus rhamnosus MN-431 producing indole derivatives on complementary feeding-induced diarrhea rat pups through the enhancement of the intestinal barrier function. Mol. Nutr. Food Res. 66 (2), e2100619. doi: 10.1002/mnfr.202100619
Obata, Y., Castaño, Á., Boeing, S., Bon-Frauches, A. C., Fung, C., Fallesen, T., et al. (2020). Neuronal programming by microbiota regulates intestinal physiology. Nature 578 (7794), 284–289. doi: 10.1038/s41586-020-1975-8
Özçam, M., Tocmo, R., Oh, J. H., Afrazi, A., Mezrich, J. D., Roos, S., et al. (2019). Gut symbionts lactobacillus reuteri R2lc and 2010 encode a polyketide synthase cluster that activates the mammalian aryl hydrocarbon receptor. Appl. Environ. Microbiol. 85 (10). doi: 10.1128/aem.01661-18
Paeslack, N., Mimmler, M., Becker, S., Gao, Z., Khuu, M. P., Mann, A., et al. (2022). Microbiota-derived tryptophan metabolites in vascular inflammation and cardiovascular disease. Amino Acids 54 (10), 1339–1356. doi: 10.1007/s00726-022-03161-5
Palmela, C., Chevarin, C., Xu, Z., Torres, J., Sevrin, G., Hirten, R., et al. (2018). Adherent-invasive Escherichia coli in inflammatory bowel disease. Gut 67 (3), 574–587. doi: 10.1136/gutjnl-2017-314903
Park, S. L., Justiniano, R., Williams, J. D., Cabello, C. M., Qiao, S., Wondrak, G. T., et al. (2015). The tryptophan-derived endogenous aryl hydrocarbon receptor ligand 6-formylindolo[3,2-b]Carbazole is a nanomolar UVA photosensitizer in epidermal keratinocytes. J. Invest. Dermatol. 135 (6), 1649–1658. doi: 10.1038/jid.2014.503
Park, I. S., Kim, J. H., Yu, J., Shin, Y., Kim, K., Kim, T. I., et al. (2023). Bifidobacterium breve CBT BR3 is effective at relieving intestinal inflammation by augmenting goblet cell regeneration. J. Gastroenterol. Hepatol. doi: 10.1111/jgh.16209
Perdew, G. H., Babbs, C. F. (1991). Production of Ah receptor ligands in rat fecal suspensions containing tryptophan or indole-3-carbinol. Nutr. Cancer 16 (3-4), 209–218. doi: 10.1080/01635589109514159
Pernomian, L., Duarte-Silva, M., de Barros Cardoso, C. R. (2020). The aryl hydrocarbon receptor (AHR) as a potential target for the control of intestinal inflammation: insights from an immune and bacteria sensor receptor. Clin. Rev. Allergy Immunol. 59 (3), 382–390. doi: 10.1007/s12016-020-08789-3
Petrulis, J. R., Perdew, G. H. (2002). The role of chaperone proteins in the aryl hydrocarbon receptor core complex. Chem. Biol. Interact. 141 (1-2), 25–40. doi: 10.1016/S0009-2797(02)00064-9
Pinto, C. J. G., Ávila-Gálvez, M., Lian, Y., Moura-Alves, P., Nunes Dos Santos, C. (2023). Targeting the aryl hydrocarbon receptor by gut phenolic metabolites: A strategy towards gut inflammation. Redox Biol. 61, 102622. doi: 10.1016/j.redox.2023.102622
Pittayanon, R., Lau, J. T., Leontiadis, G. I., Tse, F., Yuan, Y., Surette, M., et al. (2020). Differences in gut microbiota in patients with vs without inflammatory bowel diseases: A systematic review. Gastroenterology 158 (4), 930–946.e1. doi: 10.1053/j.gastro.2019.11.294
Pousa, I. D., Maté, J., Gisbert, J. P. (2008). Angiogenesis in inflammatory bowel disease. Eur. J. Clin. Invest. 38 (2), 73–81. doi: 10.1111/j.1365-2362.2007.01914.x
Qiao, P., Zhang, C., Yu, J., Shao, S., Zhang, J., Fang, H., et al. (2022). Quinolinic acid, a tryptophan metabolite of the skin microbiota, negatively regulates NLRP3 inflammasome through ahR in psoriasis. J. Invest. Dermatol. 142 (8), 2184–2193.e6. doi: 10.1016/j.jid.2022.01.010
Qin, J., Li, R., Raes, J., Arumugam, M., Burgdorf, K. S., Manichanh, C., et al. (2010). A human gut microbial gene catalogue established by metagenomic sequencing. Nature 464 (7285), 59–65. doi: 10.1038/nature08821
Qiu, J., Heller, J. J., Guo, X., Chen, Z. M., Fish, K., Fu, Y. X., et al. (2012). The aryl hydrocarbon receptor regulates gut immunity through modulation of innate lymphoid cells. Immunity 36 (1), 92–104. doi: 10.1016/j.immuni.2011.11.011
Qiu, J., Guo, X., Chen, Z. M., He, L., Sonnenberg, G. F., Artis, D., et al. (2013). Group 3 innate lymphoid cells inhibit T-cell-mediated intestinal inflammation through aryl hydrocarbon receptor signaling and regulation of microflora. Immunity 39 (2), 386–399. doi: 10.1016/j.immuni.2013.08.002
Qiu, J., Zhou, L. (2013). Aryl hydrocarbon receptor promotes RORγt+ group 3 ILCs and controls intestinal immunity and inflammation. Semin. Immunopathol. 35 (6), 657–670. doi: 10.1007/s00281-013-0393-5
Quévrain, E., Maubert, M. A., Michon, C., Chain, F., Marquant, R., Tailhades, J., et al. (2016). Identification of an anti-inflammatory protein from Faecalibacterium prausnitzii, a commensal bacterium deficient in Crohn's disease. Gut 65 (3), 415–425. doi: 10.1136/gutjnl-2014-307649
Quintana, F. J. (2013). Regulation of central nervous system autoimmunity by the aryl hydrocarbon receptor. Semin. Immunopathol. 35 (6), 627–635. doi: 10.1007/s00281-013-0397-1
Quintana, F. J., Basso, A. S., Iglesias, A. H., Korn, T., Farez, M. F., Bettelli, E., et al. (2008). Control of T(reg) and T(H)17 cell differentiation by the aryl hydrocarbon receptor. Nature 453 (7191), 65–71. doi: 10.1007/s00281-013-0397-1
Quintana, F. J., Jin, H., Burns, E. J., Nadeau, M., Yeste, A., Kumar, D., et al. (2012). Aiolos promotes TH17 differentiation by directly silencing Il2 expression. Nat. Immunol. 13 (8), 770–777. doi: 10.1038/ni.2363
Rajilić-Stojanović, M., de Vos, W. M. (2014). The first 1000 cultured species of the human gastrointestinal microbiota. FEMS Microbiol. Rev. 38 (5), 996–1047. doi: 10.1111/1574-6976.12075
Rannug, U., Rannug, A., Sjöberg, U., Li, H., Westerholm, R., Bergman, J. (1995). Structure elucidation of two tryptophan-derived, high affinity Ah receptor ligands. Chem. Biol. 2 (12), 841–845. doi: 10.1016/1074-5521(95)90090-X
Rivas, M. A., Beaudoin, M., Gardet, A., Stevens, C., Sharma, Y., Zhang, C. K., et al. (2011). Deep resequencing of GWAS loci identifies independent rare variants associated with inflammatory bowel disease. Nat. Genet. 43 (11), 1066–1073. doi: 10.1038/ng.952
Rodríguez-Sosa, M., Elizondo, G., López-Durán, R. M., Rivera, I., Gonzalez, F. J., Vega, L., et al. (2005). Over-production of IFN-gamma and IL-12 in ahR-null mice. FEBS Lett. 579 (28), 6403–6410. doi: 10.1016/j.febslet.2005.10.023
Rosser, E. C., Piper, C. J.M., Matei, D. E., Blair, P. A., Rendeiro, A. F., Orford, M., et al. (2020). Microbiota-derived metabolites suppress arthritis by amplifying aryl-hydrocarbon receptor activation in regulatory B cells. Cell Metab. 31 (4), 837–851.e10. doi: 10.1016/j.cmet.2020.03.003
Rothhammer, V., Mascanfroni, I. D., Bunse, L., Takenaka, M. C., Kenison, J. E., Mayo, L., et al. (2016). Type I interferons and microbial metabolites of tryptophan modulate astrocyte activity and central nervous system inflammation via the aryl hydrocarbon receptor. Nat. Med. 22 (6), 586–597. doi: 10.1038/nm.4106
Salminen, A. (2023). Activation of aryl hydrocarbon receptor (AhR) in Alzheimer's disease: role of tryptophan metabolites generated by gut host-microbiota. J. Mol. Med. (Berl) 101 (3), 201–222. doi: 10.1007/s00109-023-02289-5
Schiering, C., Wincent, E., Metidji, A., Iseppon, A., Li, Y., Potocnik, A. J., et al. (2017). Feedback control of AHR signalling regulates intestinal immunity. Nature 542 (7640), 242–245. doi: 10.1038/nature21080
Scott, S. A., Fu, J., Chang, P. V. (2020). Microbial tryptophan metabolites regulate gut barrier function via the aryl hydrocarbon receptor. Proc. Natl. Acad. Sci. U.S.A. 117 (32), 19376–19387. doi: 10.1073/pnas.2000047117
Singh, N. P., Singh, U. P., Singh, B., Price, R. L., Nagarkatti, M., Nagarkatti, P. S.. (2011). Activation of aryl hydrocarbon receptor (AhR) leads to reciprocal epigenetic regulation of FoxP3 and IL-17 expression and amelioration of experimental colitis. PloS One 6 (8), e23522. doi: 10.1371/journal.pone.0023522
Singh, R., Chandrashekharappa, S., Bodduluri, S. R., Baby, B. V., Hegde, B., Kotla, N. G., et al. (2019). Enhancement of the gut barrier integrity by a microbial metabolite through the Nrf2 pathway. Nat. Commun. 10 (1), 89. doi: 10.1038/s41467-018-07859-7
Smith, S. H., Jayawickreme, C., Rickard, D. J., Nicodeme, E., Bui, T., Simmons, C., et al. (2017). Tapinarof is a natural ahR agonist that resolves skin inflammation in mice and humans. J. Invest. Dermatol. 137 (10), 2110–2119. doi: 10.1016/j.jid.2017.05.004
Spencer, N. J., Hu, H. (2020). Enteric nervous system: sensory transduction, neural circuits and gastrointestinal motility. Nat. Rev. Gastroenterol. Hepatol. 17 (6), 338–351. doi: 10.1038/s41575-020-0271-2
Stange, J., Veldhoen, M. (2013). The aryl hydrocarbon receptor in innate T cell immunity. Semin. Immunopathol. 35 (6), 645–655. doi: 10.1007/s00281-013-0389-1
Stockinger, B., Hirota, K., Duarte, J., Veldhoen, M.. (2011). External influences on the immune system via activation of the aryl hydrocarbon receptor. Semin. Immunol. 23 (2), 99–105. doi: 10.1016/j.smim.2011.01.008
Stockinger, B., Shah, K., Wincent, E. (2021). AHR in the intestinal microenvironment: safeguarding barrier function. Nat. Rev. Gastroenterol. Hepatol. 18 (8), 559–570. doi: 10.1038/s41575-021-00430-8
Stojanov, S., Berlec, A., Štrukelj, B. (2020). The influence of probiotics on the firmicutes/bacteroidetes ratio in the treatment of obesity and inflammatory bowel disease. Microorganisms 8 (11). doi: 10.3390/microorganisms8111715
Stølevik, S. B., Nygaard, U. C., Namork, E., Haugen, M., Kvalem, H. E., Meltzer, H. M., et al. (2011). Prenatal exposure to polychlorinated biphenyls and dioxins is associated with increased risk of wheeze and infections in infants. Food Chem. Toxicol. 49 (8), 1843–1848. doi: 10.1016/j.fct.2011.05.002
Sugimoto, K., Ogawa, A., Mizoguchi, E., Shimomura, Y., Andoh, A., Bhan, A. K., et al. (2008). IL-22 ameliorates intestinal inflammation in a mouse model of ulcerative colitis. J. Clin. Invest. 118 (2), 534–544. doi: 10.1172/JCI33194
Sun, M., Ma, N., He, T., Johnston, L. J., Ma, X. (2020). Tryptophan (Trp) modulates gut homeostasis via aryl hydrocarbon receptor (AhR). Crit. Rev. Food Sci. Nutr. 60 (10), 1760–1768. doi: 10.1080/10408398.2019.1598334
Takamura, T., Harama, D., Fukumoto, S., Nakamura, Y., Shimokawa, N., Ishimaru, K., et al. (2010). Activation of the aryl hydrocarbon receptor pathway may ameliorate dextran sodium sulfate-induced colitis in mice. Immunol. Cell Biol. 88 (6), 685–689. doi: 10.1038/icb.2010.35
Takamura, T., Harama, D., Matsuoka, S., Shimokawa, N., Nakamura, Y., Okumura, K., et al. (2011). Lactobacillus bulgaricus OLL1181 activates the aryl hydrocarbon receptor pathway and inhibits colitis. Immunol. Cell Biol. 89 (7), 817–822. doi: 10.1038/icb.2010.165
Tong, B., Yuan, X., Dou, Y., Wu, X., Chou, G., Wang, Z., et al. (2016). Norisoboldine, an isoquinoline alkaloid, acts as an aryl hydrocarbon receptor ligand to induce intestinal Treg cells and thereby attenuate arthritis. Int. J. Biochem. Cell Biol. 75, 63–73. doi: 10.1016/j.biocel.2016.03.014
Vijay, A., Boyle, N. R., Kumar, S. M., Perdew, G. H., Srinivasan, S., Patterson, A. D. (2023). Aryl hydrocarbon receptor activation affects nitrergic neuronal survival and delays intestinal motility in mice. Toxicol. Sci. 192 (1), 117–128. doi: 10.1093/toxsci/kfad014
Visekruna, A., Luu, M. (2021). The role of short-chain fatty acids and bile acids in intestinal and liver function, inflammation, and carcinogenesis. Front. Cell Dev. Biol. 9, 703218. doi: 10.3389/fcell.2021.703218
Vyhlídalová, B., Krasulová, K., Pečinková, P., Marcalíková, A., Vrzal, R., Zemánková, L., et al. (2020). Gut microbial catabolites of tryptophan are ligands and agonists of the aryl hydrocarbon receptor: A detailed characterization. Int. J. Mol. Sci. 21 (7). doi: 10.3390/ijms21072614
Wang, Z. K., Yang, Y. S., Chen, Y., Yuan, J., Sun, G., Peng, L. H., et al. (2014). Intestinal microbiota pathogenesis and fecal microbiota transplantation for inflammatory bowel disease. World J. Gastroenterol. 20 (40), 14805–14820. doi: 10.3748/wjg.v20.i40.14805
Wang, Q., Yang, K., Han, B., Sheng, B., Yin, J., Pu, A., et al. (2018). Aryl hydrocarbon receptor inhibits inflammation in DSS−induced colitis via the MK2/p−MK2/TTP pathway. Int. J. Mol. Med. 41 (2), 868–876. doi: 10.3892/ijmm.2017.3262
Wang, J., Wang, P., Tian, H., Tian, F., Zhang, Y., Zhang, L., et al. (2018). Aryl hydrocarbon receptor/IL-22/Stat3 signaling pathway is involved in the modulation of intestinal mucosa antimicrobial molecules by commensal microbiota in mice. Innate Immun. 24 (5), 297–306. doi: 10.1177/1753425918785016
Wang, X., Huang, S., Zhang, M., Su, Y., Pan, Z., Liang, J., et al. (2023). Gegen Qinlian decoction activates AhR/IL-22 to repair intestinal barrier by modulating gut microbiota-related tryptophan metabolism in ulcerative colitis mice. J. Ethnopharmacol 302 (Pt B), 115919. doi: 10.1016/j.jep.2022.115919
Wei, Y. L., Chen, Y. Q., Gong, H., Li, N., Wu, K. Q., Hu, W., et al. (2018). Fecal microbiota transplantation ameliorates experimentally induced colitis in mice by upregulating ahR. Front. Microbiol. 9, 1921. doi: 10.3389/fmicb.2018.01921
Wen, Y., Zhan, Y., Tang, S., Liu, F., Wu, R., Kong, P., et al. (2023). Zhizhu decoction alleviates slow transit constipation by regulating aryl hydrocarbon receptor through gut microbiota. Pharm. Biol. 61 (1), 111–124. doi: 10.1080/13880209.2022.2157020
Wiggins, B. G., Wang, Y. F., Burke, A., Grunberg, N., Vlachaki Walker, J. M., Dore, M., et al. (2023). Endothelial sensing of AHR ligands regulates intestinal homeostasis. Nature. doi: 10.1038/s41586-023-06508-4
Witkowski, M., Weeks, T. L., Hazen, S. L. (2020). Gut microbiota and cardiovascular disease. Circ. Res. 127 (4), 553–570. doi: 10.1161/CIRCRESAHA.120.316242
Wu, C. Q., Lin, Q. R., Ying, S. J., Luo, J. K., Hong, W. J., Lin, Z. J., et al. (2019). Association of crohn's disease with aryl hydrocarbon receptor gene polymorphisms in patients from southeast China. Immunol. Invest. 48 (8), 809–821. doi: 10.1080/08820139.2019.1569677
Xie, Z., Li, M., Qian, M., Yang, Z., Han, X.. (2022). Co-Cultures of Lactobacillus acidophilus and Bacillus subtilis Enhance Mucosal Barrier by Modulating Gut Microbiota-Derived Short-Chain Fatty Acids. Nutrients 14 (21). doi: 10.3390/nu14214475
Yamada, T., Horimoto, H., Kameyama, T., Hayakawa, S., Yamato, H., Dazai, M., et al. (2016). Constitutive aryl hydrocarbon receptor signaling constrains type I interferon-mediated antiviral innate defense. Nat. Immunol. 17 (6), 687–694. doi: 10.1038/ni.3422
Yano, J. M., Yu, K., Donaldson, G. P., Shastri, G. G., Ann, P., Ma, L., et al. (2015). Indigenous bacteria from the gut microbiota regulate host serotonin biosynthesis. Cell 161 (2), 264–276. doi: 10.1016/j.cell.2015.02.047
Ye, J., Qiu, J., Bostick, J. W., Ueda, A., Schjerven, H., Li, S., et al. (2017). The aryl hydrocarbon receptor preferentially marks and promotes gut regulatory T cells. Cell Rep. 21 (8), 2277–2290. doi: 10.1016/j.celrep.2017.10.114
Yin, J., Yang, K., Zhou, C., Xu, P., Xiao, W.Yang H., et al. (2019). Aryl hydrocarbon receptor activation alleviates dextran sodium sulfate-induced colitis through enhancing the differentiation of goblet cells. Biochem. Biophys. Res. Commun. 514 (1), 180–186. doi: 10.1016/j.bbrc.2019.04.136
Yu, M., Wang, Q., Ma, Y., Li, L., Yu, K., Zhang, Z., et al. (2018). Aryl hydrocarbon receptor activation modulates intestinal epithelial barrier function by maintaining tight junction integrity. Int. J. Biol. Sci. 14 (1), 69–77. doi: 10.7150/ijbs.22259
Zelante, T., Iannitti, R. G., Cunha, C., De Luca, A., Giovannini, G., Pieraccini, G., et al. (2013). Tryptophan catabolites from microbiota engage aryl hydrocarbon receptor and balance mucosal reactivity via interleukin-22. Immunity 39 (2), 372–385. doi: 10.1016/j.immuni.2013.08.003
Zenewicz, L. A., Yancopoulos, G. D., Valenzuela, D. M., Murphy, A. J., Stevens, S., Flavell, R. A. (2008). Innate and adaptive interleukin-22 protects mice from inflammatory bowel disease. Immunity 29 (6), 947–957. doi: 10.1016/j.immuni.2008.11.003
Zhang, L., Nichols, R. G., Correll, J., Murray, I. A., Tanaka, N., Smith, P. B., et al. (2015). Persistent organic pollutants modify gut microbiota-host metabolic homeostasis in mice through aryl hydrocarbon receptor activation. Environ. Health Perspect. 123 (7), 679–688. doi: 10.1289/ehp.1409055
Zhao, Y., Ma, T., Chen, W., Chen, Y., Li, M., Ren, L., et al. (2016). MicroRNA-124 promotes intestinal inflammation by targeting aryl hydrocarbon receptor in crohn's disease. J. Crohns Colitis 10 (6), 703–712. doi: 10.1093/ecco-jcc/jjw010
Zhao, C., Hu, X., Bao, L., Wu, K., Feng, L., Qiu, M., et al. (2021). Aryl hydrocarbon receptor activation by Lactobacillus reuteri tryptophan metabolism alleviates Escherichia coli-induced mastitis in mice. PloS Pathog. 17 (7), e1009774. doi: 10.1371/journal.ppat.1009774
Zhao, C., Bao, L., Qiu, M., Feng, L., Chen, L., Liu, Z., et al. (2022). Dietary tryptophan-mediated aryl hydrocarbon receptor activation by the gut microbiota alleviates escherichia coli-induced endometritis in mice. Microbiol. Spectr. 10 (4), e0081122. doi: 10.1128/spectrum.00811-22
Zhou, L., Zhang, M., Wang, Y., Dorfman, R. G., Liu, H., Yu, T., et al. (2018). Faecalibacterium prausnitzii produces butyrate to maintain th17/treg balance and to ameliorate colorectal colitis by inhibiting histone deacetylase 1. Inflammation Bowel Dis. 24 (9), 1926–1940. doi: 10.1093/ibd/izy182
Keywords: inflammatory bowel disease, aryl hydrocarbon receptor, gut microbiota, interplay, therapy
Citation: Hou J-J, Ma A-H and Qin Y-H (2023) Activation of the aryl hydrocarbon receptor in inflammatory bowel disease: insights from gut microbiota. Front. Cell. Infect. Microbiol. 13:1279172. doi: 10.3389/fcimb.2023.1279172
Received: 17 August 2023; Accepted: 09 October 2023;
Published: 24 October 2023.
Edited by:
Abbas Yadegar, Shahid Beheshti University of Medical Sciences, IranReviewed by:
Zhi Liu, Huazhong University of Science and Technology, ChinaCopyright © 2023 Hou, Ma and Qin. This is an open-access article distributed under the terms of the Creative Commons Attribution License (CC BY). The use, distribution or reproduction in other forums is permitted, provided the original author(s) and the copyright owner(s) are credited and that the original publication in this journal is cited, in accordance with accepted academic practice. No use, distribution or reproduction is permitted which does not comply with these terms.
*Correspondence: Yue-Hua Qin, cWluc3dlZXRAc2luYS5jb20=
Disclaimer: All claims expressed in this article are solely those of the authors and do not necessarily represent those of their affiliated organizations, or those of the publisher, the editors and the reviewers. Any product that may be evaluated in this article or claim that may be made by its manufacturer is not guaranteed or endorsed by the publisher.
Research integrity at Frontiers
Learn more about the work of our research integrity team to safeguard the quality of each article we publish.