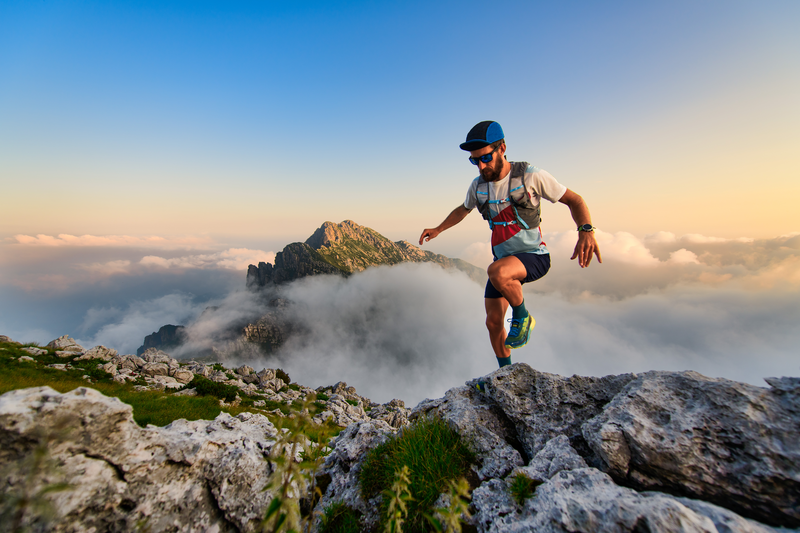
94% of researchers rate our articles as excellent or good
Learn more about the work of our research integrity team to safeguard the quality of each article we publish.
Find out more
MINI REVIEW article
Front. Cell. Infect. Microbiol. , 21 August 2023
Sec. Bacteria and Host
Volume 13 - 2023 | https://doi.org/10.3389/fcimb.2023.1250257
This article is part of the Research Topic Role of Microbes in Ocular Surface Health and Diseases View all 8 articles
Bacterial keratitis (bacterial infection of the cornea) is a major cause of vision loss worldwide. Given the rapid and aggressive nature of the disease, immediate broad-spectrum antibiotics are essential to adequately treat this disease. However, rising antibiotic resistance continues to accelerate, rendering many commonly used therapeutics increasingly ineffective. As such, there is a significant effort to understand the basic pathogenesis of common causative organisms implicated in keratitis in part, to fuel the development of novel therapies to treat this blinding disease. This review explores two common causes of bacterial keratitis, Staphylococcus aureus and Pseudomonas aeruginosa, with regards to the bacterial mediators of virulence as well as novel therapies on the horizon.
Bacterial keratitis (bacterial infection of the cornea) is a devastating disease responsible for up to 2 million annual cases of blindness globally (Whitcher and Srinivasan, 1997; Ung et al., 2019; Ting et al., 2021c). In the United States, prior studies have reported an incidence of bacterial keratitis of approximately 30/100,000 patients annually (Thylefors et al., 1995), though incidences as high as 700/100,000 have been described in developing nations (Whitcher and Srinivasan, 1997). Overall, ocular trauma remains the greatest risk for bacterial keratitis (Srinivasan et al., 1997; Upadhyay et al., 2001; Bialasiewicz et al., 2006; Ung et al., 2019), however in the United States and other developed nations, other important risk factors include contact lens wear, ocular surface disease, and previous corneal surgery (Erie et al., 1993; Bourcier et al., 2003; Keay et al., 2006).
While a wide variety of bacterial species have been associated with bacterial keratitis, the Gram-positive organism, Staphylococcus aureus, and Gram-negative organism, Pseudomonas aeruginosa, are among the most commonly identified etiologic agents (Ung et al., 2019; Gurnani and Kaur, 2022). Specifically, studies have reported a prevalence of S. aureus keratitis ranging from 8-36% among all cases of bacterial keratitis (Green et al., 2008; Ting et al., 2021c). Of note, the prevalence increases to 24-46% with the inclusion of closely related coagulase-negative Staphylococcal species (Tan et al., 2017; Ting et al., 2021c). Similarly, the prevalence of P. aeruginosa as causative organism in keratitis, has been reported as high as 10-39% among all cases of infectious keratitis in some centers (Forster, 1998; Varaprasathan et al., 2004; Ting et al., 2021b). Moreover, perhaps due to its propensity to form biofilms on contact lenses and cases, P. aeruginosa is a particularly important cause of contact lens-associated keratitis, accounting for nearly 70% of these cases in some series (Galentine et al., 1984; Schein et al., 1989; Cheng et al., 1996; Liesegang, 1997).
Given the clinical severity of bacterial keratitis, initial treatment requires immediate, broad-spectrum topical antibiotics. However, rising antibiotic resistance has rendered many currently available therapeutics ineffective, weakening our ability to adequately treat this blinding disease. For example, among clinical ocular S. aureus isolates, resistance to fluoroquinolones, a mainstay of treatment, has been steadily on the rise. Resistance rates as high as 40% have been reported for the third-generation fluoroquinolone, levofloxacin, and 35% for the fourth-generation fluoroquinolone, moxifloxacin (Peng et al., 2018; Thomas et al., 2021). When considering methicillin-resistant S. aureus (MRSA), these rates can soar as high as 82% and 73%, respectively (Marangon et al., 2004; Thomas et al., 2019).
Fortunately, among ocular P. aeruginosa isolates, circulating resistance has historically remained low in the United States. For example, previous reports of P. aeruginosa resistance have ranged from 4% to 17% towards moxifloxacin (Shalchi et al., 2011; Mei et al., 2022). However, in developing countries, resistance has been steadily rising. In India, resistance to fluoroquinolones dramatically rose from 19% to 52% in just over 2 years (Oldenburg et al., 2013; Hernandez-Camarena et al., 2015). Additionally, the appearance of multi-drug resistant P. aeruginosa keratitis isolates has been increasingly reported worldwide (Oldenburg et al., 2013; Hernandez-Camarena et al., 2015), including the recent 2023 outbreak of extreme multi-drug resistant P. aeruginosa infections associated with contaminated artificial tear solutions that led not only to serious ocular infections but several patient deaths (Shoji et al., 2023).
Given the severity and high prevalence of S. aureus and P. aeruginosa keratitis, coupled with rapidly rising resistance, there is substantial interest in understanding the underlying bacterial mechanisms driving disease to fuel the discovery of novel therapeutic approaches. As such, this review aims to describe the current literature of known and suspected virulence factors of both S. aureus and P. aeruginosa that mediate pathogenesis in infectious keratitis (Table 1). Additionally, the current state of antimicrobial drug discovery efforts in Ophthalmology is described, highlighting promising new therapies to treat this blinding disease (Table 2).
Several key studies have begun to define the set of S. aureus virulence factors that are particularly important in promoting virulence in corneal infections. As described below, these include toxins such as α-, β- and γ-toxin, Panton-Valentine leucocidin (PVL), as well as fibronectin binding protein, collagen-adhesion binding protein, Staphopain A, and extracellular adherence protein (Eap), which mediate bacteria-host cell interactions. Additionally, the recent identification of Staphylococcal enterotoxins and their role in keratitis virulence will be discussed (Table 1).
The canonical pore-forming toxin, α-hemolysin toxin (hla), has been widely implicated in S. aureus pathogenesis in diverse physiologic sites as well as shown to promote disease severity in both rabbit and mouse models of keratitis (O'Callaghan et al., 1997). In a study by O'Callaghan et al., α-toxin-deficient strains of S. aureus resulted in less conjunctival inflammation and stromal ulceration in rabbit models of keratitis (O'Callaghan et al., 1997). Moreover, purified alpha-toxin applied topically to the rabbit ocular surface led to increased conjunctival inflammation, corneal edema, and iritis, further supporting the role of this virulence factor in keratitis pathogenesis (O'Callaghan et al., 1997). In a complementary study by Putra et al., α-toxin-deficient S. aureus strains demonstrated decreased ability to invade corneal epithelial cells in an in vitro corneal cell culture and resulted in improved wound healing in a murine model of keratitis (Putra et al., 2019).
β-toxin, a sphingomyelinase that lyses epithelial cell membranes, has also been shown to contribute to keratitis virulence. For example, Dajcs et al. found that in a rabbit keratitis model, infections from S. aureus strains deficient in both α- and β-toxins resulted in lower disease severity than strains deficient in α-toxin alone (Dajcs et al., 2002b). The addition of topical purified β-toxin has also been shown to induce mild conjunctival inflammation in rabbit eyes and scleral inflammation in intrastromal injections (O'Callaghan et al., 1997; Dajcs et al., 2002b). Moreover, infections with a β-toxin deficient mutant of S. aureus produced less ocular edema in rabbit models than the wild type strains (Dajcs et al., 2002b).
Another notable toxin includes γ-toxin, a pore-forming toxin comprised of two secreted proteins HlgB and either HlgA or HlgC. Using a rabbit model of keratitis, Dajcs et al. demonstrated that while bacterial counts were equivalent in animals infected with S. aureus strain Newman and its isogenic hlg-deficient mutant, markers of disease severity such as conjunctival injection and chemosis, corneal edema, corneal infiltrate, and hypopyon formation were significantly higher in the presence of γ-toxin (Dajcs et al., 2002a). Additionally, Bierdeman et al. demonstrated that direct injection of γ-toxin into rabbit corneas resulted in significant corneal infiltrates, iritis, fibrin formation in the anterior chamber as well as conjunctival injection (Bierdeman et al., 2017).
Panton-Valentine leucocidin (PVL) is a secreted two component-toxin comprised of the proteins LukF-PV and LukS-PV and is commonly associated with methicillin-resistant S. aureus (MRSA) strains. PVL is capable of binding complement pathway proteins and has been shown to have cytotoxic effects to a wide range of host immune cells such as neutrophils, macrophages and natural killer cells (Astley et al., 2019). In a recent study by Zaidi et al., PVL expression in MRSA isolates led to increased bacterial burden and cytotoxicity in both murine and human epithelial cell culture keratitis models (Zaidi et al., 2013). However, interestingly, there is still debate about the clinical importance of PVL as a more recent study on human subjects by Hsaio et al. found similar clinical features, including ulcer location, ulcer size and presence of hypopyon, as well as antibiotic susceptibility between PVL-positive and PVL-deficient strains (Hsiao et al., 2022). It should be noted that there are several other identified homologous protein components such as LukM, LukS-R, LukE, LukF-R and LukD that can combine with each other to form multiple unique toxins. While the roles of these variants have not been explicitly tested in keratitis, it is possible they may also play a role in promoting corneal disease.
Other S. aureus virulence factors that have been implicated in keratitis include fibronectin-binding protein, collagen-binding adhesion, Staphopain A, and Eap. Fibronectin-binding protein mediates attachment of bacteria to fibronectin molecules on the surface of corneal epithelial cells which is a precursor for host cellular invasion (Jett and Gilmore, 2002; Maurin et al., 2021). Specifically, Maurin et al. found that fibronectin-binding proteins were the principal driving factor for epithelial invasion, especially in injured epithelium (Maurin et al., 2021). Similarly, collagen-binding adhesion (coa) promotes bacterial attachment to the corneal surface, and in a rabbit model of keratitis, may also promote a more severe suppurative keratitis (Rhem et al., 2000). Additionally, the cysteine protease Staphopain A has been shown to promote S. aureus invasion into corneal epithelial cells by increasing fibronectin binding (Hume et al., 2020). Eap has also been found to promote corneal epithelial invasion in murine keratitis models through the inhibition of host neutrophil proteases (Hume et al., 2009; Kretschmer et al., 2021).
Recently, Staphylococcal enterotoxins, a set of secreted toxins well known for their role in S. aureus-mediated food poisoning as well as septic shock due to widespread nonspecific host T-cell activation, have been identified as playing a key role in keratitis virulence. For example, in a corneal epithelial cell model, S. aureus strains that encode and express one or more of the enterotoxins sed, sej, sek, seq, ser, or selX, resulted in widespread corneal cell cytotoxicity compared to those infecting strains without enterotoxins. Moreover, in a murine model of keratitis, an infecting strain that expressed enterotoxins Sed, Sej, Sek, Seq and Ser led to a 3-log increase in bacterial burden compared to the isogenic enterotoxin deletion mutant or a strain that naturally lacks enterotoxins (Johnson et al., 2023).
P. aeruginosa, similarly to S. aureus, has an impressive arsenal of virulence factors that can be leveraged to promote infection in specific physiologic niches. With regards to keratitis, several virulence factors have been described that specifically mediate disease in the cornea. As described below, the serine protease Pseudomonas protease IV (PIV), exotoxins exoS, exoU and exoT, P. aeruginosa small protease (PASP), Elastases, and extracellular factors such as lipopolysaccharide (LPS) are bacterial determinants that have an established link to keratitis (Table 1).
Protease IV has been shown to be a key virulence factor in corneal infections, able to evade the host immune response due to its intrinsic low immunogenicity (Thibodeaux et al., 2005) and promote the degradation of key host immune proteins such as complement, fibrinogen, plasminogen and immunoglobulin (Engel et al., 1998; Malloy et al., 2005). While PIV does not appear to impact the bacterial burden in a rabbit model of keratitis, disease severity as assessed by slit lamp exams was significantly higher in strains expressing PIV compared to an PIV-deficient mutant (Caballero et al., 2004). Moreover, plasmid-based expression of PIV in an otherwise PIV-negative Pseudomonas putida strain led to significant increase in corneal tissue damage and ocular inflammation (Traidej et al., 2003).
Two other significant P. aeruginosa keratitis virulence factors are exotoxin S (exoS), which is implicated in invasive infections, and exotoxin U (exoU), which is associated with acute host cytotoxicity (Fleiszig et al., 1997; Karthikeyan et al., 2013; Kandasamy et al., 2020). Both proteins are secreted by the P. aeruginosa Type III secretion system and generally speaking, isolates either encode either exoS or exoU. While some studies have found no difference in the prevalence of these strains in keratitis isolates (Lomholt et al., 2001), others have found a propensity for exoU-positive strains in cases of keratitis, particularly in patients with contact lens-associated disease (Winstanley et al., 2005; Choy et al., 2008; Stewart et al., 2011). Moreover, the large-scale Steroids for Corneal Ulcers Trial (SCUT) found that those patients infected with exoU-positive strains had larger corneal infiltrates and presented with poorer visual acuity compared to patients infected with exoS-positive strains (Borkar et al., 2013). Two other effector proteins secreted by the P. aeruginosa Type III secretion system include ExoT, which is a closely related protein to ExoS, and ExoY, an adenylate cyclase. While ExoY appears to have limited influence in keratitis virulence, ExoT has been shown to promote P. aeruginosa disease severity as well as mitigate neutrophil-mediated bacterial killing in a murine model of keratitis (Sun et al., 2012). Of note, the Type III secretion system itself is a critical requirement for corneal disease, as strains that do not assemble this secretion system at all result in attenuated clinical disease severity in mice (Sun et al., 2012).
P. aeruginosa small protease (PASP) is a serine protease that causes collagen digestion, thereby promoting corneal epithelial and stromal ulceration (Marquart et al., 2005; Tang et al., 2013). Moreover, purified PASP injection into the cornea resulted in a significant polymorphonucleated cell stromal infiltrate (Tang et al., 2009). In both a corneal scratch and intrastromal injection rabbit model of keratitis, while the overall bacterial load remained unchanged, PASP-deficient mutants resulted in reduced disease severity as measured by slit-lamp exam scores, and infected eyes demonstrated less inflammation and tissue damage as measured by histology (Tang et al., 2013). Elastase (LasB) is another example of a metalloproteinase that can disrupt corneal extracellular matrix proteins and, in some models, has been shown to promote P. aeruginosa keratitis virulence (Dart and Seal, 1988; Fleiszig and Evans, 2002; Thibodeaux et al., 2007). The presence of Elastase was also significantly correlated with hypopyon formation and larger corneal ulcer size in human patients with P. aeruginosa keratitis (Oka et al, 2015). However, the exact role of LasB, as well as the related elastase, LasA, in corneal virulence is not entirely clear, as several studies have demonstrated that isogenic deletion mutants of lasB and lasA did not result in disease attenuation in a mouse model of keratitis (White et al., 2001; Hobden, 2002).
Extracellular surface factors, including pili and polysaccharide capsule component LPS, are also implicated in promoting P. aeruginosa keratitis disease severity through potent host immune stimulation (Fukuda et al., 2017). For example, injection of LPS into the rabbit corneal stroma led to rapid infiltration of neutrophils as well as corneal ulceration (Howes et al., 1982b; Schultz et al., 1997). Additionally, LPS may also have a role in promoting P. aeruginosa adherence to corneal epithelial cells as well as subsequent bacterial internalization (Zaidi, 1996).
As rising antibiotic resistance threatens our current therapeutic arsenal, there is an urgent need for novel antimicrobial therapeutics. While pharmaceutical companies have largely abandoned antimicrobial drug development in favor of more long-term, lucrative therapeutics, academic centers continue to innovate, seeking to leverage the expanding knowledge of bacterial pathogenesis to develop new treatment strategies. As described below, there are several novel therapeutics on the horizon to treat bacterial keratitis including novel broad-spectrum antibiotic drug combinations, host defense peptides, monoclonal antibodies, and non-pharmaceutical approaches such as corneal cross linking, bacteriophage and plasma therapies.
One strategy in the field of antimicrobial drug discovery is to repurpose existing therapeutics in new combinations to elicit improved antimicrobial activity. To that end, the combination of polymyxin B-trimethoprim (PT) + rifampin has recently been shown to display broad-spectrum, synergistic antimicrobial activity, with rapid in vitro bactericidal activity and anti-biofilm activity, the latter of which may have particular importance given the propensity of bacteria to establish biofilms on contact lenses (Chojnacki et al., 2019a; Chojnacki et al., 2019b). The potent activity of this combination has also been shown to effectively eradicate large sets of S. aureus and P. aeruginosa ocular clinical isolates from around the world, including those strains displaying multi-drug resistance in in vitro studies, demonstrating the ability to PT + rifampin to overcome relevant circulating isolates (Laskey et al., 2020; Mei et al., 2022). Importantly, PT + rifampin has been shown to eradicate in vivo S. aureus and P. aeruginosa keratitis infections in a murine model. For example, following infection with a clinical keratitis isolate of S. aureus with known fluoroquinolone-resistance, topical treatment with PT + rifampin four times daily for 72 hours led to complete eradication of disease in 7 out of 10 animals, compared to a less than 2-log reduction in bacterial burden following treatment with topical moxifloxacin, a current gold standard of treatment (Chojnacki et al., 2019b). Given the resistance to fluoroquinolones is on the rise, this data suggests that PT + rifampin may be a viable alternative for the treatment of keratitis.
An additional approach in drug discovery is to exploit naturally produced antimicrobial host immune compounds. For example, human-derived host defense peptides (HDPs), are a class of proteins produced by a variety of immune cells and are well known for their broad-spectrum antimicrobial activity via direct bacterial cell lysis. While naturally occurring HDPs have established limitations such as weak stability and host toxicity, several groups have sought to modify these compounds to improve compatibility and efficacy. For example, a synthetic, hybridized HDP comprised of cathelicidin and human beta defensin-2 components has been shown to have potent antimicrobial activity towards S. aureus in in vitro studies, a low propensity to drive resistance, and a modest reduction in bacterial burden in a S. aureus model of keratitis (Ting et al., 2021a).
A similar study has explored the efficacy of brilacidin, a synthetic mimetic of defensins, compounds naturally produced by innate immune cells that exhibits immunomodulatory activities as well as antibacterial effects via a variety of mechanism such as depolarizing bacterial cell membranes, neutralizing toxins, and inhibition of cell wall synthesis (Kowalski et al., 2016). Kowalski et al. demonstrated in vitro efficacy of brilacidin towards both S. aureus and Staphylococcus epidermidis, and, in a rabbit model of MRSA keratitis, brilacidin displayed equivalent efficacy compared to vancomycin with no evidence of toxicity. Of note, in this model, the efficacy of brilacidin required an ulcerated epithelium, suggesting there may be poor penetrance of this compound with an intact ocular surface (Kowalski et al., 2016).
Another proposed small peptide with a potential role in treating infectious keratitis is thymosin beta 4, a small, ubiquitously found natural protein that has been shown to reduce inflammation in the cornea and enhance bacterial killing in P. aeruginosa-associated keratitis (Sosne and Berger, 2023). Currently, thymosin beta 4 is being actively investigated in the treatment of dry eye disease due to its anti-inflammatory and pro-wound healing properties. However, as an adjunctive therapy with ciprofloxacin, thymosin beta 4 improved inflammatory infiltrates and enhanced bacterial killing in a P. aeruginosa murine keratitis model compared to a ciprofloxacin-only treated group. Similarly, studies have explored the use of the plant-derived compound wedelolactone as an adjunctive therapy to antibiotics to mediate inflammatory injury in P. aeruginosa keratitis. Wedelolactone exhibits diverse antioxidant and anti-inflammatory properties and is thought to mitigate non-canonical programmed cell death. As an adjunctive therapy with ciprofloxacin, wedelolactone resulted in reduced disease severity and attenuated immune response in a rat model of P. aeruginosa keratitis (Xu et al., 2021).
The use of monoclonal antibodies has also been explored as a potential treatment of infectious keratitis. For example, a human monoclonal antibody Fab fragment to S. aureus α-toxin has been evaluated in a rabbit model of S. aureus keratitis, demonstrating that this approach can decrease corneal ulceration, chemosis and iritis (Caballero et al., 2015). Similarly, a monoclonal antibody that targets Pseudomonas exotoxins and type III secretion proteins was shown to be non-toxic to the ocular surface in mice as well as mitigated signs of infection (Hebert et al., 2020).
Other novel therapeutic approaches include the use of non-pharmaceutical approaches such as photoactivated chromophore corneal collagen cross-linking (PACK-CXL), bacteriophage therapy, and plasma therapy. Corneal cross-linking is well-known for its application in slowing the progression of corneal ectatic disorders. However, it has also been appreciated that the free-radicals generated from the combination of ultraviolet-A light and the photosensitizing agent, riboflavin, may have antimicrobial activity as well. Over the past 10 years, PACK-CXL has been used to treat advanced corneal melts, including those with infectious origins, both as a standalone treatment but also in conjunction with traditional antimicrobial therapies with moderate success (Papaioannou et al., 2016; Tabibian et al., 2016; Lee et al., 2021; Barac et al., 2022). While this technology is currently used in cases that have failed conventional treatment, with optimization of both the light source and photosensitizing agent, there may be broader applications in the future.
Bacteriophages are viruses that naturally infect and kill specific bacterial species, making their use in the treatment of human disease appealing. While a major limitation of bacteriophage therapy is the narrow spectrum of activity of individual phages, there have been recent studies demonstrating efficacy in select cases of bacterial keratitis. Fukuda et al. have shown that a single-dose administration of a bacteriophage targeting the P. aeruginosa strain PA33 was sufficient to reduce disease severity and the host immune response (Fukuda et al., 2012). Additionally, there is also a recent case report of a human patient with recalcitrant S. aureus infection successfully treated with phage therapy (Fadlallah et al., 2015).
Finally, cold plasma is an ionized gas that already has established applications in medicine given its anti-inflammatory and wound healing effects. Recently this technology has been tested for its antibacterial activity as well as for safety on corneal limbal epithelial cells, suggesting there may be some promise in this novel technology for the treatment of keratitis (Reitberger et al., 2018).
Infectious keratitis is a blinding disease and current therapeutic options are failing due to the relentless increase in antibiotic resistance. To combat circulating and emerging drug-resistant strains, new antimicrobial therapies are desperately needed. This review highlights both the basic pathogenesis of two common causes of keratitis, S. aureus and P. aeruginosa in corneal infections as well as the leading edge of new therapies in the pipeline. An increasing knowledge of the bacterial drivers of infections can fuel future discoveries to both directly target the pathogen and mitigate the damaging host response.
RW edited the manuscript and SS conducted literature review. Both RW and SS contributed to the manuscript writing. All authors contributed to the article and approved the submitted version.
RW was supported by a Research to Prevent Blindness Career Development Award. SS was supported by a Research to Prevent Blindness Departmental Unrestricted Grant.
The authors declare that the research was conducted in the absence of any commercial or financial relationships that could be construed as a potential conflict of interest.
All claims expressed in this article are solely those of the authors and do not necessarily represent those of their affiliated organizations, or those of the publisher, the editors and the reviewers. Any product that may be evaluated in this article, or claim that may be made by its manufacturer, is not guaranteed or endorsed by the publisher.
Astley, R., Miller, F. C., Mursalin, M. H., Coburn, P. S., Callegan, M. C. (2019). An eye on staphylococcus aureus toxins: roles in ocular damage and inflammation. Toxins (Basel) 11. doi: 10.3390/toxins11060356
Barac, I. R., Artamonov, A. R., Balta, G., Dinu, V., Mehedintu, C., Bobirca, A., et al. (2022). Photoactivated chromophore corneal collagen cross-linking for infectious keratitis (Pack-cxl)-A comprehensive review of diagnostic and prognostic factors involved in therapeutic indications and contraindications. J. Pers. Med. 12. doi: 10.3390/jpm12111907
Bialasiewicz, A., Shenoy, R., Thakral, A., Al-Muniri, A. A., Shenoy, U., Al-Mughairi, Z. (2006). Microbial keratitis: A 4 year study of risk factors and traditional/complementary medicine in Oman. Ophthalmologe 103, 682–687. doi: 10.1007/s00347-006-1363-2
Bierdeman, M. A., Torres, A. M., Caballero, A. R., Tang, A., O'callaghan, R. J. (2017). Reactions with antisera and pathological effects of staphylococcus aureus gamma-toxin in the cornea. Curr. Eye Res. 42, 1100–1107. doi: 10.1080/02713683.2017.1279636
Borkar, D. S., Fleiszig, S. M., Leong, C., Lalitha, P., Srinivasan, M., Ghanekar, A. A., et al. (2013). Association between cytotoxic and invasive Pseudomonas aeruginosa and clinical outcomes in bacterial keratitis. JAMA Ophthalmol. 131, 147–153. doi: 10.1001/jamaophthalmol.2013.778
Bourcier, T., Thomas, F., Borderie, V., Chaumeil, C., Laroche, L. (2003). Bacterial keratitis: predisposing factors, clinical and microbiological review of 300 cases. Br. J. Ophthalmol. 87, 834–838. doi: 10.1136/bjo.87.7.834
Caballero, A. R., Foletti, D. L., Bierdeman, M. A., Tang, A., Arana, A. M., Hasa-Moreno, A., et al. (2015). Effectiveness of alpha-toxin fab monoclonal antibody therapy in limiting the pathology of staphylococcus aureus keratitis. Ocul Immunol. Inflammation 23, 297–303. doi: 10.3109/09273948.2014.920035
Caballero, A., Thibodeaux, B., Marquart, M., Traidej, M., O'Callaghan, R. (2004). Pseudomonas keratitis: protease iv gene conservation, distribution, and production relative to virulence and other Pseudomonas proteases. Invest. Ophthalmol. Visual Sci. 45, 522–530. doi: 10.1167/iovs.03-1050
Cheng, K., Smyth, R. L., Govan, J. R., Doherty, C., Winstanley, C., Denning, N., et al. (1996). Spread of beta-lactam-resistant Pseudomonas aeruginosa in A cystic fibrosis clinic. Lancet 348, 639–642. doi: 10.1016/S0140-6736(96)05169-0
Chojnacki, M., Philbrick, A., Scherzi, T., Pecora, N., Dunman, P. M., Wozniak, R. A. F. (2019a). A novel, broad-spectrum antimicrobial combination for the treatment of Pseudomonas aeruginosa corneal infections. Antimicrob. Agents Chemother. 63. doi: 10.1128/AAC.00777-19
Chojnacki, M., Philbrick, A., Wucher, B., Reed, J. N., Tomaras, A., Dunman, P. M., et al. (2019b). Development of A broad-spectrum antimicrobial combination for the treatment of staphylococcus aureus and Pseudomonas aeruginosa corneal infections. Antimicrob. Agents Chemother. 63. doi: 10.1128/AAC.01929-18
Choy, M. H., Stapleton, F., Willcox, M. D. P., Zhu, H. (2008). Comparison of virulence factors in Pseudomonas aeruginosa strains isolated from contact lens- and non-contact lens-related keratitis. J. Med. Microbiol. 57, 1539–1546. doi: 10.1099/jmm.0.2008/003723-0
Dajcs, J. J., Austin, M. S., Sloop, G. D., Moreau, J. M., Hume, E. B., Thompson, H. W., et al. (2002a). Corneal pathogenesis of staphylococcus aureus strain newman. Invest. Ophthalmol. Vis. Sci. 43, 1109–1115.
Dajcs, J. J., Thibodeaux, B. A., Girgis, D. O., O'callaghan, R. J. (2002b). Corneal virulence of staphylococcus aureus in an experimental model of keratitis. DNA Cell Biol. 21, 375–382. doi: 10.1089/10445490260099656
Dart, J. K., Seal, D. V. (1988). Pathogenesis and therapy of Pseudomonas aeruginosa keratitis. Eye (Lond) 2 Suppl, S46–S55. doi: 10.1038/eye.1988.133
Engel, L. S., Hill, J. M., Caballero, A. R., Green, L. C., O'callaghan, R. J. (1998). Protease iv, A unique extracellular protease and virulence factor from Pseudomonas aeruginosa. J. Biol. Chem. 273, 16792–16797. doi: 10.1074/jbc.273.27.16792
Erie, J. C., Nevitt, M. P., Hodge, D. O., Ballard, D. J. (1993). Incidence of ulcerative keratitis in A defined population from 1950 through 1988. Arch. Ophthalmol. 111, 1665–1671. doi: 10.1001/archopht.1993.01090120087027
Fadlallah, A., Chelala, E., Legeais, J. M. (2015). Corneal infection therapy with topical bacteriophage administration. Open Ophthalmol. J. 9, 167–168. doi: 10.2174/1874364101509010167
Fleiszig, S. M., Evans, D. J. (2002). The pathogenesis of bacterial keratitis: studies with Pseudomonas aeruginosa. Clin. Exp. Optom. 85, 271–278. doi: 10.1111/j.1444-0938.2002.tb03082.x
Fleiszig, S. M., Wiener-Kronish, J. P., Miyazaki, H., Vallas, V., Mostov, K. E., Kanada, D., et al. (1997). Pseudomonas aeruginosa-mediated cytotoxicity and invasion correlate with distinct genotypes at the loci encoding exoenzyme S. Infect. Immun. 65, 579–586. doi: 10.1128/iai.65.2.579-586.1997
Forster, R. K. (1998). Conrad berens lecture. The management of infectious keratitis as we approach the 21st century. Clao J. 24, 175–180.
Fukuda, T., Bouchi, R., Terashima, M., Sasahara, Y., Asakawa, M., Takeuchi, T., et al. (2017). Ipragliflozin reduces epicardial fat accumulation in non-obese type 2 diabetic patients with visceral obesity: A pilot study. Diabetes Ther. 8, 851–861. doi: 10.1007/s13300-017-0279-y
Fukuda, K., Ishida, W., Uchiyama, J., Rashel, M., Kato, S., Morita, T., et al. (2012). Pseudomonas aeruginosa keratitis in mice: effects of topical bacteriophage kpp12 administration. PloS One 7, e47742. doi: 10.1371/journal.pone.0047742
Galentine, P. G., Cohen, E. J., Laibson, P. R., Adams, C. P., Michaud, R., Arentsen, J. J. (1984). Corneal ulcers associated with contact lens wear. Arch. Ophthalmol. 102, 891–894. doi: 10.1001/archopht.1984.01040030711025
Green, M., Apel, A., Stapleton, F. (2008). Risk factors and causative organisms in microbial keratitis. Cornea 27, 22–27. doi: 10.1097/ICO.0b013e318156caf2
Gurnani, B., Kaur, K. (2022). “Bacterial keratitis,” in Statpearls (Treasure Island (Fl): StatPearls Publishing).
Hebert, W., Digiandomenico, A., Zegans, M. (2020). Multifunctional monoclonal antibody targeting Pseudomonas aeruginosa keratitis in mice. Vaccines (Basel) 8. doi: 10.3390/vaccines8040638
Hernandez-Camarena, J. C., Graue-Hernandez, E. O., Ortiz-Casas, M., Ramirez-MIranda, A., Navas, A., Pedro-Aguilar, L., et al. (2015). Trends in microbiological and antibiotic sensitivity patterns in infectious keratitis: 10-year experience in Mexico city. Cornea 34, 778–785. doi: 10.1097/ICO.0000000000000428
Hobden, J. A. (2002). Pseudomonas aeruginosa proteases and corneal virulence. DNA Cell Biol. 21, 391–396. doi: 10.1089/10445490260099674
Howes, E. L., Jr., Char, D. H., Christensen, M. (1982b). Aqueous immune complexes in immunogenic uveitis. Invest. Ophthalmol. Vis. Sci. 23, 715–718.
Howes, E. L., Cruse, V. K., Kwok, M. T. (1982a). Mononuclear cells in the corneal response to endotoxin. Invest. Ophthalmol. Vis. Sci. 22, 494–501.
Hsiao, C. H., Kang, E. Y., Yeh, L. K., Ma, D. H. K., Chen, H. C., Hung, K. H., et al. (2022). Staphylococcus aureus keratitis in Taiwan: genotyping, antibiotic susceptibility, and clinical features. Int. J. Mol. Sci. 23. doi: 10.3390/ijms231911703
Hume, E. B., Cole, N., Khan, S., Walsh, B. J., Willcox, M. D. (2020). The role of staphopain A in staphylococcus aureus keratitis. Exp. Eye Res. 193, 107994. doi: 10.1016/j.exer.2020.107994
Hume, E. B., Khan, S., Cole, N., Willcox, M. (2009). Investigating specific virulence factors involved in staphylococcus aureus keratitis. Invest. Ophthalmol. Visual Sci. 50, 3457–3457.
Jett, B. D., Gilmore, M. S. (2002). Internalization of staphylococcus aureus by human corneal epithelial cells: role of bacterial fibronectin-binding protein and host cell factors. Infect. Immun. 70, 4697–4700. doi: 10.1128/IAI.70.8.4697-4700.2002
Johnson, W. L., Sohn, M., Woeller, C. F., Wozniak, R. A. F. (2023). Staphylococcal enterotoxins promote virulence in bacterial keratitis. Invest. Ophthalmol. Vis. Sci. 64, 5. doi: 10.1167/iovs.64.5.5
Kandasamy, K., Thirumalmuthu, K., Prajna, N. V., Lalitha, P., Mohankumar, V., Devarajan, B. (2020). Comparative genomics of ocular Pseudomonas aeruginosa strains from keratitis patients with different clinical outcomes. Genomics 112, 4769–4776. doi: 10.1016/j.ygeno.2020.08.032
Karthikeyan, R. S., Priya, J. L., Leal, S. M., Jr., Toska, J., Rietsch, A., Prajna, V., et al. (2013). Host response and bacterial virulence factor expression in Pseudomonas aeruginosa and streptococcus pneumoniae corneal ulcers. PloS One 8, e64867. doi: 10.1371/journal.pone.0064867
Keay, L., Edwards, K., Naduvilath, T., Taylor, H. R., Snibson, G. R., Forde, K., et al. (2006). Microbial keratitis predisposing factors and morbidity. Ophthalmology 113, 109–116. doi: 10.1016/j.ophtha.2005.08.013
Kowalski, R. P., Romanowski, E. G., Yates, K. A., Mah, F. S. (2016). An independent evaluation of A novel peptide mimetic, brilacidin (Pmx30063), for ocular anti-infective. J. Ocul Pharmacol. Ther. 32, 23–27. doi: 10.1089/jop.2015.0098
Kretschmer, D., Breitmeyer, R., Gekeler, C., Lebtig, M., Schlatterer, K., Nega, M., et al. (2021). Staphylococcus aureus depends on eap proteins for preventing degradation of its phenol-soluble modulin toxins by neutrophil serine proteases. Front. Immunol. 12, 701093. doi: 10.3389/fimmu.2021.701093
Laskey, E., Chen, Y., Sohn, M. B., Gruber, E., Chojnacki, M., Wozniak, R. A. F. (2020). Efficacy of A novel ophthalmic antimicrobial drug combination toward A large panel of staphylococcus aureus clinical ocular isolates from around the world. Cornea 39, 1278–1284. doi: 10.1097/ICO.0000000000002414
Lee, J. W., Somerville, T., Kaye, S. B., Romano, V. (2021). Staphylococcus aureus keratitis: incidence, pathophysiology, risk factors and novel strategies for treatment. J. Clin. Med. 10. doi: 10.3390/jcm10040758
Liesegang, T. J. (1997). Contact lens-related microbial keratitis: part I: epidemiology. Cornea 16, 125–131.
Lomholt, J. A., Poulsen, K., Kilian, M. (2001). Epidemic population structure of Pseudomonas aeruginosa: evidence for A clone that is pathogenic to the eye and that has A distinct combination of virulence factors. Infect. Immun. 69, 6284–6295. doi: 10.1128/IAI.69.10.6284-6295.2001
Malloy, J. L., Veldhuizen, R. A., Thibodeaux, B. A., O'callaghan, R. J., Wright, J. R. (2005). Pseudomonas aeruginosa protease iv degrades surfactant proteins and inhibits surfactant host defense and biophysical functions. Am. J. Physiol. Lung Cell Mol. Physiol. 288, L409–L418. doi: 10.1152/ajplung.00322.2004
Marangon, F. B., Miller, D., Muallem, M. S., Romano, A. C., Alfonso, E. C. (2004). Ciprofloxacin and levofloxacin resistance among methicillin-sensitive staphylococcus aureus isolates from keratitis and conjunctivitis. Am. J. Ophthalmol. 137, 453–458. doi: 10.1016/j.ajo.2003.10.026
Marquart, M. E., Caballero, A. R., Chomnawang, M., Thibodeaux, B. A., Twining, S. S., O'callaghan, R. J. (2005). Identification of A novel secreted protease from Pseudomonas aeruginosa that causes corneal erosions. Invest. Ophthalmol. Vis. Sci. 46, 3761–3768. doi: 10.1167/iovs.04-1483
Maurin, C., Courrier, E., He, Z., Rigaill, J., Josse, J., Laurent, F., et al. (2021). Key role of staphylococcal fibronectin-binding proteins during the initial stage of staphylococcus aureus keratitis in humans. Front. Cell Infect. Microbiol. 11, 745659. doi: 10.3389/fcimb.2021.745659
Mei, J. A., Johnson, W., Kinn, B., Laskey, E., Nolin, L., Bhamare, P., et al. (2022). Antimicrobial activity of A triple antibiotic combination toward ocular Pseudomonas aeruginosa clinical isolates. Transl. Vis. Sci. Technol. 11, 26. doi: 10.1167/tvst.11.5.26
O'Callaghan, R. J., Callegan, M. C., Moreau, J. M., Green, L. C., Foster, T. J., Hartford, O. M., et al. (1997). Specific roles of alpha-toxin and beta-toxin during staphylococcus aureus corneal infection. Infect. Immun. 65, 1571–1578. doi: 10.1128/iai.65.5.1571-1578.1997
Oka, N., Suzuki, T., Ishikawa, E., Yamaguchi, S., Hayashi, N., Gotoh, N., et al. (2015). Relationship of virulence factors and clinical features in keratitis caused by Pseudomonas aeruginosa. Invest. Ophthalmol. Vis. Sci. 56, 6892–6898. doi: 10.1167/iovs.15-17556
Oldenburg, C. E., Lalitha, P., Srinivasan, M., Rajaraman, R., Ravindran, M., Mascarenhas, J., et al. (2013). Emerging moxifloxacin resistance in Pseudomonas aeruginosa keratitis isolates in south India. Ophthalmic Epidemiol. 20, 155–158. doi: 10.3109/09286586.2013.790978
Papaioannou, L., Miligkos, M., Papathanassiou, M. (2016). Corneal collagen cross-linking for infectious keratitis: A systematic review and meta-analysis. Cornea 35, 62–71. doi: 10.1097/ICO.0000000000000644
Peng, M. Y., Cevallos, V., Mcleod, S. D., Lietman, T. M., Rose-Nussbaumer, J. (2018). Bacterial keratitis: isolated organisms and antibiotic resistance patterns in san francisco. Cornea 37, 84–87. doi: 10.1097/ICO.0000000000001417
Putra, I., Rabiee, B., Anwar, K. N., Gidfar, S., Shen, X., Babalooee, M., et al. (2019). Staphylococcus aureus alpha-hemolysin impairs corneal epithelial wound healing and promotes intracellular bacterial invasion. Exp. Eye Res. 181, 263–270. doi: 10.1016/j.exer.2019.02.019
Reitberger, H. H., Czugala, M., Chow, C., Mohr, A., Burkovski, A., Gruenert, A. K., et al. (2018). Argon cold plasma-A novel tool to treat therapy-resistant corneal infections. Am. J. Ophthalmol. 190, 150–163. doi: 10.1016/j.ajo.2018.03.025
Rhem, M. N., Lech, E. M., Patti, J. M., Mcdevitt, D., Hook, M., Jones, D. B., et al. (2000). The collagen-binding adhesin is A virulence factor in staphylococcus aureus keratitis. Infect. Immun. 68, 3776–3779. doi: 10.1128/IAI.68.6.3776-3779.2000
Schein, O. D., Ormerod, L. D., Barraquer, E., Alfonso, E., Egan, K. M., Paton, B. G., et al. (1989). Microbiology of contact lens—Related keratitis. Cornea 8, 281–285. doi: 10.1097/00003226-198912000-00011
Schultz, C. L., Morck, D. W., Mckay, S. G., Olson, M. E., Buret, A. (1997). Lipopolysaccharide induced acute red eye and corneal ulcers. Exp. Eye Res. 64, 3–9. doi: 10.1006/exer.1996.0190
Shalchi, Z., Gurbaxani, A., Baker, M., Nash, J. (2011). Antibiotic resistance in microbial keratitis: ten-year experience of corneal scrapes in the United Kingdom. Ophthalmology 118, 2161–2165. doi: 10.1016/j.ophtha.2011.04.021
Shoji, M. K., Gutkind, N. E., Meyer, B. I., Yusuf, R., Sengillo, J. D., Amescua, G., et al. (2023). Multidrug-resistant Pseudomonas aeruginosa keratitis associated with artificial tear use. JAMA Ophthalmol. 141, 499–500. doi: 10.1001/jamaophthalmol.2023.1109
Sosne, G., Berger, E. A. (2023). Thymosin beta 4: A potential novel adjunct treatment for bacterial keratitis. Int. Immunopharmacol 118, 109953. doi: 10.1016/j.intimp.2023.109953
Srinivasan, M., Gonzales, C. A., George, C., Cevallos, V., Mascarenhas, J. M., Asokan, B., et al. (1997). Epidemiology and aetiological diagnosis of corneal ulceration in Madurai, South India. Br. J. Ophthalmol. 81, 965–971. doi: 10.1136/bjo.81.11.965
Stewart, R. M., Wiehlmann, L., Ashelford, K. E., Preston, S. J., Frimmersdorf, E., Campbell, B. J., et al. (2011). Genetic characterization indicates that A specific subpopulation of Pseudomonas aeruginosa is associated with keratitis infections. J. Clin. Microbiol. 49, 993–1003. doi: 10.1128/JCM.02036-10
Sun, Y., Karmakar, M., Taylor, P. R., Rietsch, A., Pearlman, E. (2012). Exos and exot adp ribosyltransferase activities mediate Pseudomonas aeruginosa keratitis by promoting neutrophil apoptosis and bacterial survival. J. Immunol. 188, 1884–1895. doi: 10.4049/jimmunol.1102148
Tabibian, D., Mazzotta, C., Hafezi, F. (2016). Pack-cxl: corneal cross-linking in infectious keratitis. Eye Vis. (Lond) 3, 11. doi: 10.1186/s40662-016-0042-x
Tan, S. Z., Walkden, A., Au, L., Fullwood, C., Hamilton, A., Qamruddin, A., et al. (2017). Twelve-year analysis of microbial keratitis trends at A uk tertiary hospital. Eye (Lond) 31, 1229–1236. doi: 10.1038/eye.2017.55
Tang, A., Caballero, A. R., Marquart, M. E., O'callaghan, R. J. (2013). Pseudomonas aeruginosa small protease (Pasp), A keratitis virulence factor. Invest. Ophthalmol. Vis. Sci. 54, 2821–2828. doi: 10.1167/iovs.13-11788
Tang, A., Marquart, M. E., Fratkin, J. D., Mccormick, C. C., Caballero, A. R., Gatlin, H. P., et al. (2009). Properties of pasp: A Pseudomonas protease capable of mediating corneal erosions. Invest. Ophthalmol. Vis. Sci. 50, 3794–3801. doi: 10.1167/iovs.08-3107
Thibodeaux, B. A., Caballero, A. R., Dajcs, J. J., Marquart, M. E., Engel, L. S., O'callaghan, R. J. (2005). Pseudomonas aeruginosa protease iv: A corneal virulence factor of low immunogenicity. Ocul Immunol. Inflammation 13, 169–182. doi: 10.1080/09273940490518937
Thibodeaux, B. A., Caballero, A. R., Marquart, M. E., Tommassen, J., O'callaghan, R. J. (2007). Corneal virulence of Pseudomonas aeruginosa elastase B and alkaline protease produced by Pseudomonas putida. Curr. Eye Res. 32, 373–386. doi: 10.1080/02713680701244181
Thomas, R. K., Melton, R., Asbell, P. A. (2019). Antibiotic resistance among ocular pathogens: current trends from the armor surveillance study, (2009-2016). Clin. Optom (Auckl) 11, 15–26. doi: 10.2147/OPTO.S189115
Thomas, R. K., Melton, R., Vollmer, P. M., Asbell, P. A. (2021). In vitro antibiotic resistance among bacteria from the cornea in the antibiotic resistance monitoring in ocular microorganisms surveillance study. Optom. Vis. Sci. 98, 1113–1121. doi: 10.1097/OPX.0000000000001768
Thylefors, B., Negrel, A. D., Pararajasegaram, R., Dadzie, K. Y. (1995). Global data on blindness. Bull. World Health Organ 73, 115–121.
Ting, D. S. J., Goh, E. T. L., Mayandi, V., Busoy, J. M. F., Aung, T. T., Periayah, M. H., et al. (2021a). Hybrid derivative of cathelicidin and human beta defensin-2 against gram-positive bacteria: A novel approach for the treatment of bacterial keratitis. Sci. Rep. 11, 18304. doi: 10.1038/s41598-021-97821-3
Ting, D. S. J., Ho, C. S., Cairns, J., Elsahn, A., Al-Aqaba, M., Boswell, T., et al. (2021b). 12-year analysis of incidence, microbiological profiles and in vitro antimicrobial susceptibility of infectious keratitis: the nottingham infectious keratitis study. Br. J. Ophthalmol. 105, 328–333. doi: 10.1136/bjophthalmol-2020-316128
Ting, D. S. J., Ho, C. S., Deshmukh, R., Said, D. G., Dua, H. S. (2021c). Infectious keratitis: an update on epidemiology, causative microorganisms, risk factors, and antimicrobial resistance. Eye (Lond) 35, 1084–1101. doi: 10.1038/s41433-020-01339-3
Traidej, M., Caballero, A. R., Marquart, M. E., Thibodeaux, B. A., O'callaghan, R. J. (2003). Molecular analysis of Pseudomonas aeruginosa protease iv expressed in Pseudomonas putida. Invest. Ophthalmol. Vis. Sci. 44, 190–196. doi: 10.1167/iovs.02-0458
Ung, L., Bispo, P. J. M., Shanbhag, S. S., Gilmore, M. S., Chodosh, J. (2019). The persistent dilemma of microbial keratitis: global burden, diagnosis, and antimicrobial resistance. Surv Ophthalmol. 64, 255–271. doi: 10.1016/j.survophthal.2018.12.003
Upadhyay, M. P., Karmacharya, P. C., Koirala, S., Shah, D. N., Shakya, S., Shrestha, J. K., et al. (2001). The bhaktapur eye study: ocular trauma and antibiotic prophylaxis for the prevention of corneal ulceration in Nepal. Br. J. Ophthalmol. 85, 388–392. doi: 10.1136/bjo.85.4.388
Varaprasathan, G., Miller, K., Lietman, T., Whitcher, J. P., Cevallos, V., Okumoto, M., et al. (2004). Trends in the etiology of infectious corneal ulcers at the F. I. Proctor foundation. Cornea 23, 360–364. doi: 10.1097/00003226-200405000-00009
Whitcher, J. P., Srinivasan, M. (1997). Corneal ulceration in the developing world–A silent epidemic. Br. J. Ophthalmol. 81, 622–623. doi: 10.1136/bjo.81.8.622
White, C. D., Alionte, L. G., Cannon, B. M., Caballero, A. R., O'callaghan, R. J., Hobden, J. A. (2001). Corneal virulence of lasa protease–deficient Pseudomonas aeruginosa pao1. Cornea 20, 643–646. doi: 10.1097/00003226-200108000-00017
Winstanley, C., Kaye, S. B., Neal, T. J., Chilton, H. J., Miksch, S., Hart, C. A., et al. (2005). Genotypic and phenotypic characteristics of Pseudomonas aeruginosa isolates associated with ulcerative keratitis. J. Med. Microbiol. 54, 519–526. doi: 10.1099/jmm.0.46005-0
Xu, S., Liu, X., Liu, X., Shi, Y., Jin, X., Zhang, N., et al. (2021). Wedelolactone ameliorates Pseudomonas aeruginosa-induced inflammation and corneal injury by suppressing caspase-4/5/11/gsdmd-mediated non-canonical pyroptosis. Exp. Eye Res. 211, 108750. doi: 10.1016/j.exer.2021.108750
Zaidi, T. E. A. (1996). Lipopolysaccharide outer core is A ligand for corneal cell binding and ingestion of Pseudomonas aeruginosa. Iovs Arvo J. 37, 976–986.
Zaidi, T. S., Fleiszig, S. M., Preston, M. J., Goldberg, J. B., Pier, G. B. (1996). Lipopolysaccharide outer core is A ligand for corneal cell binding and ingestion of Pseudomonas aeruginosa. Invest. Ophthalmol. Vis. Sci. 37, 976–986.
Keywords: keratitis, bacterial virulence factors, antibiotic drug development, microbial drug resistance, Staphyloccocus aureus, Pseudomonas aeruginosa
Citation: Shah S and Wozniak RAF (2023) Staphylococcus aureus and Pseudomonas aeruginosa infectious keratitis: key bacterial mechanisms that mediate pathogenesis and emerging therapeutics. Front. Cell. Infect. Microbiol. 13:1250257. doi: 10.3389/fcimb.2023.1250257
Received: 29 June 2023; Accepted: 04 August 2023;
Published: 21 August 2023.
Edited by:
Poonam Mudgil, Western Sydney University, AustraliaReviewed by:
Ashok Kumar, Wayne State University, United StatesCopyright © 2023 Shah and Wozniak. This is an open-access article distributed under the terms of the Creative Commons Attribution License (CC BY). The use, distribution or reproduction in other forums is permitted, provided the original author(s) and the copyright owner(s) are credited and that the original publication in this journal is cited, in accordance with accepted academic practice. No use, distribution or reproduction is permitted which does not comply with these terms.
*Correspondence: Rachel A. F. Wozniak, cmFjaGVsX3dvem5pYWtAdXJtYy5yb2NoZXN0ZXIuZWR1
Disclaimer: All claims expressed in this article are solely those of the authors and do not necessarily represent those of their affiliated organizations, or those of the publisher, the editors and the reviewers. Any product that may be evaluated in this article or claim that may be made by its manufacturer is not guaranteed or endorsed by the publisher.
Research integrity at Frontiers
Learn more about the work of our research integrity team to safeguard the quality of each article we publish.