- 1Clinical Medical Research Center, The Second Affiliated Hospital, Army Medical University, Chongqing, China
- 2Department of Pediatrics, Yunyang Women and Children’s Hospital (Yunyang Maternal and Child Health Hospital), Chongqing, China
- 3Department of Surgery, Yunyang Women and Children’s Hospital (Yunyang Maternal and Child Health Hospital), Chongqing, China
- 4The 3rd Affiliated Teaching Hospital of Xinjiang Medical University (Affiliated Tumor Hospital), Urumqi, China
- 5Sun Yat-sen University Cancer Center, State Key Laboratory of Oncology in South China and Collaborative Innovation Center for Cancer Medicine, Guangzhou, China
Parasitic diseases pose a significant threat to global public health, particularly in developing countries. Host genetic factors play a crucial role in determining susceptibility and resistance to infection. Recent advances in molecular and biological technologies have enabled significant breakthroughs in understanding the impact of host genes on parasite adaptation. In this comprehensive review, we analyze the host genetic factors that influence parasite adaptation, including hormones, nitric oxide, immune cells, cytokine gene polymorphisms, parasite-specific receptors, and metabolites. We also establish an interactive network to better illustrate the complex relationship between host genetic factors and parasite-host adaptation. Additionally, we discuss future directions and collaborative research priorities in the parasite-host adaptation field, including investigating the impact of host genes on the microbiome, developing more sophisticated models, identifying and characterizing parasite-specific receptors, utilizing patient-derived sera as diagnostic and therapeutic tools, and developing novel treatments and management strategies targeting specific host genetic factors. This review highlights the need for a comprehensive and systematic approach to investigating the underlying mechanisms of parasite-host adaptation, which requires interdisciplinary collaborations among biologists, geneticists, immunologists, and clinicians. By deepening our understanding of the complex interactions between host genetics and parasite adaptation, we can develop more effective and targeted interventions to prevent and treat parasitic diseases. Overall, this review provides a valuable resource for researchers and clinicians working in the parasitology field and offers insights into the future directions of this critical research area.
1 Introduction
Parasitic diseases are important among human infectious diseases. The prevalence of parasitic infection in tropical and subtropical regions is more severe owing to humidity and relatively lagging economic and sanitary conditions (Dejon-Agobe et al., 2022; Kalkman et al., 2022; Taylor et al., 2022); thus, parasitic infection remains a major public health problem in these regions. During the course of biological evolution, intricate relationships have been formed between organisms, among which the term symbiosis describes the phenomenon that two different organisms live together. Symbiosis can be further divided into commensalism, mutualism and parasitism. Parasitism means that two organisms live together, one of which, the parasite, benefits and the other, the host, suffers. Parasitism originates from contact between parasites and hosts. Once a host is infected, parasites trigger the defensive response of the host, but with different outcomes. First, hosts can expel all of the worms and acquire the ability to resist reinfection; second, hosts can eliminate only some worms, which is the case upon which most relationships between hosts and parasites are based; and third, hosts can fail to control the parasites effectively, leading to sustained parasite survival, the development of parasitism, and thus evident pathological changes, clinical symptoms and even death (Micucci et al., 2010; Lotter et al., 2013; Zhou et al., 2021). The outcomes of parasite-host adaptation are generally closely related to host genetic backgrounds, yet summaries in this field are lacking. In this article, the host adaptation of parasites and the potential mechanisms remarkably influenced by host genetic backgrounds are reviewed (Shen et al., 2020). It is expected that this review will provide new insights for the in-depth understanding of parasite-host adaptation as well as strategies to prevent and control parasitic infection in the future.
2 Main text
2.1 Literature search strategy
In this study, we reviewed scientific studies with no time restriction to identify studies focusing on the factors of host genetic backgrounds that affect parasite-host adaptation. A total of 19 parasites whose adaptation has been documented were included in this study, including protozoans, flagellates, sporozoans, trematodes, cestodes and nematodes. Search terms were the combinations of “Latin name for parasite”, “host”, “host susceptibility”, “host resistance”, and “host adaptation” using the online databases PubMed (https://www.ncbi.nlm.nih.gov/pubmed/) or Web of Science (http://apps.webofknowledge.com/UA_GeneralSearch_input.do?product=UA&search_mode=GeneralSearch&SID=5ChWtwlripdAqJRyqsq&preferencesSaved=). After removing duplicated articles, we screened the titles and abstracts of the articles according to the following inclusion criteria: (1) studies published in English and (2) articles describing the differences among parasite migration, development, survival and pathogenicity caused by host genetic backgrounds. The exclusion criteria were as follows: (1) studies that were duplicates of graduate theses; (2) studies of influencing factors with no significant differences in hosts of different genetic backgrounds; and (3) studies not describing or related to parasite-host adaptation.
2.2 Protozoan
2.2.1 Entamoeba histolytica
Entamoeba histolytica (E. histolytica) is the only intestinal amebiasis known to cause amebiasis in humans, characterized by intestinal damage and amoebic liver abscess. Humans are permissive hosts for E. histolytica. Monkeys, cats, dogs, and mice can also serve as incidental hosts but retain natural resistance to amoebae. Some rodent strains, such as C3H/Hej, CBA/J, Mongolian gerbils, and hamsters, develop amoebic liver abscesses similar to humans, while others, such as BALB/c, C57BL/6 and rats, are somewhat resistant to the development of amoebic liver abscesses, which is attributed to the increasing levels of nitric oxide (NO) and some higher levels of cytokines, such as IL-10 (Chadee and Meerovitch, 1984; Ivory et al., 2007; Olivos-Garcia et al., 2018). Mucin deficiency leading to the failure of mucin to cleave the amoebic caspase in some hosts might cause natural resistance. Studies have also confirmed that IL-10 deficiency reduces the synthesis of host colonic mucin MUC2. Therefore, IL-10-deficient mice with defective mucin synthesis are susceptible to amebic invasion of the epithelium (Ivory et al., 2007). In contrast, rats are completely resistant to amebiasis by virtue of the elimination of amebiasis through serum complement components at an early stage (the first 6 hours after infection) (Olivos-Garcia et al., 2018). In addition, the expression of inducible nitric oxide synthase (iNOS) stimulated by amoebae varies widely among the macrophages of different hosts. For example, iNOS is highly expressed by alveolar macrophages obtained from rats, but not in those obtained hamsters or humans, when stimulated by LPS or IFN-γ, thus participating in the elimination of amoeba (Ramirez-Emiliano et al., 2007). In the human population, amoeba infection is more common in men than in women (Groneberg et al., 2022). Similarly, E. histolytica activates a stronger immunopathological response and results in larger liver abscesses in male mice than in female mice. Male HIF-1α knockout (KO) mice show no difference from female mice in the size of amoebic liver abscesses as a consequence of the decrease in Th17 cells and cytokine IL-6 located downstream (Groneberg et al., 2022). Hence, HIF-1α-regulated Th17 cells and cytokines might be important factors determining the differences in immunopathology between males and females. Additionally, the host status of hormones, such as testosterone and leptin, may affect the resistance of the host to amebiasis. Testosterone shows an inhibitory effect on host resistance by suppressing IFN-γ secretion by natural killer T cells, while leptin enhances this secretion (Duggal et al., 2011; Lotter et al., 2013). Furthermore, as reported, MHC polymorphisms such as HLA-DRB1*03:0101 and HLA-DRB1*11:0101 in humans may be associated with higher susceptibility to amebiasis (Al-Khaliq and Mahdi, 2018). Altogether, these findings imply that host genetic background-related factors such as NO, IL-10, complement, hormone and MHC polymorphisms are involved in parasite-host adaptation.
2.3 Flagellates
2.3.1 Leishmania
Leishmaniosis is a zoonosis caused by Leishmania that is transmitted through the bite of female phlebotomine sandflies carrying the promastigote in their salivary glands. These parasites infect not only humans but also other vertebrates, including rodents, lagomorphs and mustelids (Villanueva-Saz et al., 2022). Leishmania braziliensis (L. braziliensis) is the agent of cutaneous leishmaniasis (CL), the most prevalent clinical form of leishmaniasis in Brazil. However, only a few infected people exhibit symptoms. The underlying mechanism varies but is genetically correlated. First, a single nucleotide polymorphism (SNP) is involved. As reported, people carrying the rs2275913 A SNP of the IL17A gene are more vulnerable to L. braziliensis infection than those carrying the rs2275913 G SNP (Goncalves de Albuquerque et al., 2019). For carriers of the rs16944 T/C SNP of the IL1B gene, those with the C/C genotype are more susceptible to CL (da Silva et al., 2019). The effects of cytokines on susceptibility may be opposite in mice with different genetic backgrounds. It was reported that IL-18 knockout 129×CD1 and DBA/1 genetic background mice showed higher susceptibility to Leishmania infection than wild-type (WT) mice, likely by suppressing the Th1 response. Conversely, the enforced Th1 response led the IL-18 knockout BALB/c background mice to exhibit much resistance to Leishmania infection(Wei et al., 2004) and only BALB/c background mice shows the response specific to IL-18 knockout while other background mice like 129×CD1, DBA/1 and C57BL/6(Clay et al., 2014) exhibit increased susceptibility to Leishmania infection. In addition, the resistance of hosts is also related to other cytokines, such as IL-1α, IL-10, IL-22 and IL-32(Schamber-Reis et al., 2013; Dos Santos et al., 2020). IL-1α is required for optimal IL-12-induced Th1 development in BALB/c but not C57BL/6 mice (Shibuya et al., 1998). The less IL-1α in response to L. major from BALB/c compared with C57BL/6 mice, providing partial explanation for the disparate outcomes of infection between these two mouse strains (Von Stebut et al., 2003). IL-22 is a member of the IL-10 family cytokines. The increased expression of the IL-10 was significantly associated with enhanced sensitivity to L. major infection in UNC93B1 mutant mice for the reason of the skewed expression pattern towards upregulation of IL-10 (Schamber-Reis et al., 2013). The genetic background of IL-32 variation in host can influence its susceptibility to Leishmania (Dos Santos et al., 2020). The IL32 rs4786370 genetic variation was associated with protection against ATL, while IL32 rs4349147 susceptibility to localized cutaneous and mucosal leishmaniasis.
2.3.2 Trypanosoma
Infectious Trypanosoma in humans includes Trypanosoma brucei gambiense (T. b. gambiense), Trypanosoma brucei rhodesiense (T. b. rhodesiense) and Trypanosoma cruzi (T. cruzi). T. b. gambiense and T. b. rhodesiense are prevalent in Africa and are transmitted by the Glossina palpalis and Glossina tsetse flies, respectively. The reservoirs of T. b. gambiense include humans, cattle, pigs, sheep and dogs, whereas those of T. b. rhodesiense comprise humans, sheep, cattle, lions and dogs (Alfituri et al., 2020). For the American subspecies, T. cruzi transmitted through the bite of the triatomine bug is virulent to humans and other mammals, including foxes, squirrels, anteaters, dogs and cats (Rosypal et al., 2014; Busselman and Hamer, 2022). Because T. cruzi is the most intensively studied Trypanosoma species, we mainly summarize the host adaptation of T. cruzi.
Genetic backgrounds play important roles in the susceptibility of hosts to T. cruzi. Mice with a BALB/c background are resistant to T. cruzi, while C57BL/6 mice are susceptible to T. cruzi. In detail, different mouse strains have distinct major histocompatibility complex (MHC) haplotypes, which affect their antigen presentation and recognition. As is known, C57BL/6 mice have the H-2b haplotype, which is more efficient in presenting peptides derived from intracellular pathogens, whereas BALB/c mice have the H-2d haplotype, which is more efficient in presenting peptides derived from extracellular pathogens. And this genetic background difference results in their different susceptibility to T. cruzi. Moreover, In B10 or DBA/1 mice, the H-2q haplotype is related to resistance, whereas the C3H genetic background is related to susceptibility (Powell and Wassom, 1993). It is reported that C57BL/6 mice produced higher levels of IFN-γ and lower levels of IL-4 than BALB/c mice, indicating a Th1 immune response(Bergersen et al., 2021). However, C57BL/6 shows more susceptible to T. cruzi than BALB/c mice, suggesting Th2 immunity likely plays a more important role in the elimination of T. cruzi. The polymorphism of cytokine genes, such as the IL-1, IL-4, IL-13, IL-17, IL-18, TNF-α and IFN-γ genes, may impact their expression and lead to diverse immune responses, resulting in different parasite adaptation and host susceptibility (Hiyama et al., 2001; Miyazaki et al., 2010; Chessler et al., 2011; Abad Dar and Holscher, 2018; Strauss et al., 2019). For example, the amplification of the IL-13 gene in mice increases the susceptibility to T. cruzi, elevates parasite parasitemia and impairs survival because of the enhanced activation of arginase, which promotes parasite replication (Abad Dar and Holscher, 2018). As demonstrated, arginase limits the production of NO and increases the level of polyamine (Abad Dar and Holscher, 2018), and NO enhances the resistance of the host to parasites, but polyamine provides materials for replication. Consistently, higher NO levels were observed in C57BL/6 mice during acute infection with T. cruzi, and iNOS KO mice showed a higher susceptibility to T. cruzi (Holscher et al., 1998). Furthermore, insufficient NO causes a higher susceptibility to T. cruzi in BALB/c mice than in XID mice, which is negatively related to excessive IL-10 secreted by B-1 cells (da Rocha et al., 2019). In addition, parasite-specific IgG and sex differences are also involved in parasite-host adaptation, but further investigation of the underlying mechanisms is needed (Perez et al., 2005; Micucci et al., 2010).
2.3.3 Trichomonas vaginalis
Trichomonas vaginalis (T. vaginalis) is a parasite infecting the human vagina and urinary tract, where it causes inflammation. Hitherto, it has been believed that T. vaginalis causes disease only in humans (Corbeil, 1995). Both men and women are susceptible to T. vaginalis, and infected individuals can be either symptomatic or asymptomatic. Males display stronger resistance to T. vaginalis, which might be attributed to pre-T cells, while estrogen helps T. vaginalis adhesion in females (Martinotti et al., 1988). Symptomatic patients show higher CP30 than asymptomatic patients among females (Yadav et al., 2007), whether it is the same among males remains further exploration. Furthermore, the elevation of IgM, IgG1 and IgG2b in patient urine samples was found to be related to more severe symptoms (Imam et al., 2007). In addition, the virulence of T. vaginalis could be modulated by MHC, the sensitivity of T cells and the activation of phagocytes in hosts (Caterina et al., 1996). A mouse strain harboring H-2d was shown to be susceptible to infection with T. vaginalis, but strains with H-2k were resistant (Caterina et al., 1996). Notably, the differences due to hormones, immunoglobulin and MHC depend on genetic divergence, which would affect the susceptibility of hosts. However, the direct evidences supporting genetic divergence-determined susceptibility remain more further investigations.
2.4 Sporozoan
2.4.1 Plasmodium
Plasmodium has a wide range of hosts, including humans, birds, reptiles, apes, monkeys, rodents, ungulates and other kinds of animals (Perkins, 2014; Templeton et al., 2016; Galen et al., 2018; Matta et al., 2018; Valkiunas et al., 2018). Currently, six species of Plasmodium have been proven to cause malaria in humans, namely, Plasmodium. falciparum (Pf), Plasmodium. vivax (Pv), Plasmodium. knowlesi (Pk), Plasmodium. malariae (Pm), and two subspecies of Plasmodium ovale (Po) (Sutherland et al., 2010). Among the Plasmodium species infectious to humans, Pf belongs to the subgenus Laverania, for which host selection is extremely strict. Pf infects only humans, whereas the others specifically infect apes such as chimpanzees or gorillas, which is determined by the variance in receptor-ligand binding during the adsorption of Plasmodium to erythrocytes, as widely recognized (Liu et al., 2016). Although genomics between species within the subgenus Laverania is relatively conserved, the genes encoding erythrocyte adsorption ligands, such as those of the Stero gene family and Rh gene family, are quite different (Otto et al., 2018; Plenderleith et al., 2018). Furthermore, EBA165 in the erythrocyte binding-like gene family is silenced in the Pf genome but expressed in other Laverania species. When Pf expresses EBA165, Pf gains the ability to infect apes rather than infect humans (Proto et al., 2019). On the other hand, the surface receptors of human and ape erythrocytes are distinct. Studies have shown that the Rh5 molecule of Pf is the only ligand present on all Pf strains that can bind to basigin (BSG, CD147) on the surface of erythrocytes (Crosnier et al., 2011). The affinity of Rh5 to human BSG is significantly higher than that of chimpanzees and gorillas for two key BSG sites, F27 and K191, thereby increasing the affinity for Rh5 (Forni et al., 2015). In addition, differences in the sialic acids on the surface of erythrocytes might affect the infection outcomes for humans and apes. In apes, Neu5Gc regulates the production of sialic acid. Neu5Ac cannot be converted into Neu5Gc in humans due to the lack of cytidine monophosphate N-acetylneuraminic acid hydroxylase, thus showing the differences in plasmodium adsorption to erythrocytes in different species (Muchmore et al., 1998; Hayakawa et al., 2001).
Hosts of Plasmodium can be divided into hosts with intermediate host adaptation and hosts with definitive host adaptation. Humans are a special intermediate host, which is mainly represented in the fact that humans in some populations are naturally resistant to malaria. The ligand-receptor pair composed of Duffy-binding protein (DBP-RII) on the surface of Pv and Duffy antigen receptor chemokine (DARC) on the surface of erythrocytes is an essential molecular basis for infection. The surfaces of the erythrocytes of most black people in West Africa lack DARC because of natural selection, so these individuals are naturally resistant to Pv (Chitnis and Sharma, 2008; de Sousa et al., 2014; Payne et al., 2017). Abnormal structures of erythrocytes, including those associated with the sickle cell (HbAS) and HbAC (HbAC) traits, could result in inhibitory effects on Pf, thus enhancing resistance in people throughout Africa harboring these two structures. However, the specific mechanism has not been fully elucidated (Kreuels et al., 2010; Goncalves et al., 2016). In addition, G6PD is essential to maintain the growth and development of Plasmodium (Usanga and Luzzatto, 1985). Therefore, populations with G6PD deficiency generally display enforced resistance to Plasmodium (Louicharoen et al., 2009). Definitive hosts of Plasmodium include mosquitoes, Hylemyia and midges (Hellgren et al., 2008; Martinez-de la Puente et al., 2011), and mosquitoes are the main definitive host, while only Anopheles mosquitoes can transmit Plasmodium as a result of ookinete immune evasion (Knockel et al., 2013). At the molecular mechanistic level, Pfs47 on the surface of Pf binding to P47Rec is specifically expressed in the stomach of Anopheles mosquitoes and mediates the immune escape of ookinetes, which is further confirmed by the blunted Pf infection upon P47Rec silencing (Molina-Cruz et al., 2020).
2.4.2 Toxoplasma gondii
Toxoplasma gondii (T. gondii) is an obligate intracellular and opportunistic parasite that parasitizes all karyocytes and mainly spreads by peroral infection and vertical transmission. The life history of T. gondii is divided into the sexual stage (enteral phase) and the asexual stage (parenteral period). Only cats and felines are definitive hosts for T. gondii (Frenkel et al., 1970) because the lack of ω-6-desaturase in cat intestinal epithelial cells leads to a high content of linoleic acid, which indicates that ω-6-desaturase can foster host adaptation by T. gondii. Consistently, the ω-6-desaturase inhibitor or a high linoleic acid diet was found to enable T. gondii to develop to the sexual stage in mice (Martorelli Di Genova et al., 2019).
The asexual part of T. gondii exhibits a flexible form of host selection, with hosts including all warm-blooded animals such as mice, rabbits, sheep, cattle and some cold-blooded animals (Dubey, 2008). As reported, the host adaptation of T. gondii depends on immune factors, NO and reactive oxygen species (ROS). The higher level of NO in rats is more resistant to T. gondii when than that in mice (Witola et al., 2017). However, recent studies have shown that the knockout of the iNOS gene enables stronger resistance against T. gondii in rats, which is possibly because the loss of iNOS frees the ROS system of the mononuclear phagocyte system. ROS have been well documented to participate in the elimination of pathogens; hence, the targeting of ROS is likely an effective strategy to kill T. gondii and to avoid the spread of this pathogen in rats (Wang et al., 2021).
2.4.3 Cryptosporidium
Cryptosporidium is an opportunistic intracellular parasite that parasitizes intestinal epithelial cells, and its life history consists of sexual and asexual generations. Upon infection, Cryptosporidium mainly causes gastrointestinal symptoms such as diarrhea. Cryptosporidium and its diverse subspecies can infect almost all organisms, including humans, cattle, sheep, pigs and other species (Xiao and Fayer, 2008). Infectious Cryptosporidium species to humans mainly include Cryptosporidium parvum (C. parvum) and Cryptosporidium hominis (C. hominis). In addition, several other Cryptosporidium species also infect patients with immunodeficiency diseases such as acquired immunodeficiency syndrome (AIDS) (Hunter and Nichols, 2002; Ahmadpour et al., 2020). As an opportunistic parasite, Cryptosporidium infection is closely related to an immunocompromised or immunodeficient status, and hosts display different susceptibilities according to HLAI/II types (Kirkpatrick et al., 2008). A high infection rate of Cryptosporidium is influenced by HLA DQB1*0301 and HLA B*15. In fact, infants are more susceptible to Cryptosporidium than adults (Hunter et al., 2004). Moreover, patients with immune deficiency related to T cells exhibit higher susceptibility to Cryptosporidium, which indicates that T-cell-mediated adaptive immunity probably plays a major role in parasite-host adaptation (Winfield, 1997). In the case of Cryptosporidium infection, T-cell-mediated adaptive immunity is believed to be important for host resistance (McDonald, 2000; McNair and Mead, 2013; Russler-Germain et al., 2021). The genetic background of the host can influence the T-cell response and subsequent parasite adaptation. Genetic variations in immune-related genes, including those involved in T-cell activation, cytokine production, and antigen presentation, can impact the effectiveness of the T-cell response against Cryptosporidium. For example, polymorphisms in genes (like SNPs of LTα, IFNβ1 and TNFα) can affect the production and function of cytokines, which in turn can influence the balance between Th1 and Th2 responses (Davhana et al., 2020; Kloch et al., 2023). However, a dearth of experimental studies at the genetic level exists for the majority of factors that have been unequivocally identified as influencing parasite host adaptation. Nevertheless, it is possible to engage in speculation regarding the genetic underpinnings of these influential factors and elucidate the association between the host gene corresponding to the factor and host adaptation. In contrast, B cells show no significant effect on host resistance to Cryptosporidium (Gomez Morales et al., 1996).
2.5 Trematodes
2.5.1 Clonorchis sinensis
The hosts of C. sinensis comprise the first intermediate host (freshwater snails), second intermediate hosts (freshwater fish and shrimp) and definitive hosts. More than 30 mammals, including humans, serve as definitive hosts (Li et al., 2018). Humans are generally susceptible to C. sinensis regardless of sex, age, or race. After infection, C. sinensis metacercariae migrate to and parasitize human intrahepatic bile ducts, where they develop into adults and lay eggs under the chemotaxis of cholic acid, suggesting that cholic acid affects the host adaptation of C. sinensis (Li et al., 2018). Furthermore, the susceptibility of hosts to C. sinensis is correlated with host immunity. C3H/HeN mice with higher levels of IgE, IFN-γ, IL-13 and Th1/Th2 immune responses are less susceptible than C57BL/6, BALB/C and ICR mice (Lee et al., 2006; Uddin et al., 2012). However, the development of C. sinensis is impaired in immunocompromised SCID and nude mice, which implies that immune factors probably exert dual functions in mouse strains with different genetic backgrounds (Yoon et al., 2001).
2.5.2 Fasciola hepatica
The hosts of Fasciola hepatica (F. hepatica) comprise the intermediate host (Lymnaeidae) and definitive hosts (diverse mammals, including humans). Differences in genetic background among intermediate hosts have notable influences on susceptibility to F. hepatica (Cruz-Reyes and Malek, 1987). Lymnaeidae includes Pseudosuccinea columella and Fossaria cubensis. Pseudosuccinea columella is more susceptible to F. hepatica than Fossaria cubensis, which is possibly the cause of the divergence in genetic background and size between snails (Cruz-Reyes and Malek, 1987). F. hepatica is a common parasite that parasitizes the intrahepatic bile ducts of the definitive host, including common hosts (cattle and sheep) and uncommon hosts (rodents, rabbits and humans). The adaptation of F. hepatica varies in different definitive hosts. In uncommon hosts, the egg size is smaller than that in common hosts, and the ability of eggs to develop into miracidia is weaker, thus leading to a reduction in their prevalence (Abrous et al., 1998). In addition, the resistance of definitive hosts to F. hepatica is closely associated with the adaptive immune response. The thymus, the major reservoir of T cells, is the contributing factor of host resistance to F. hepatica. As reported, infection with F. hepatica is lethal to congenitally athymic nude mice (Eriksen, 1980). Furthermore, the Th1 immune response promotes the elimination of hosts by F. hepatica (Pleasance et al., 2011). Consistently, indigenous thin-tailed sheep with early and locally occurring Th1 immune responses were found to exhibit stronger resistance to F. hepatica infection (Pleasance et al., 2011).
2.5.3 Schistosoma
The hosts of Schistosoma include intermediate and definitive hosts. The intermediate hosts comprise Oncomelania hupensis, Biomphalaria, Bulinus, Tricula aperta and the small Robert snail, while the definitive hosts include humans, cattle, pigs, dogs, sheep, rodents, monkeys and baboons. Based on the differences in resistance to Schistosoma, definitive hosts are classified into nonpermissive hosts and permissive hosts. Nonpermissive hosts with stronger resistance to Schistosoma include Microtus fortis, rats and buffalo (Yang et al., 2013; Shen et al., 2020), in which Schistosoma fails to develop to sexual maturity. Permissive hosts exhibiting less resistance to Schistosoma include mice, goats and humans, where the adult worms lay eggs and consequently develop hepatic lesions characterized by egg granulomas (Yang et al., 2013).
The intermediate hosts of S. mansoni mainly include the NMRI and BS-90 strains of B. glabrata. The NMRI strain is susceptible to S. mansoni, whereas the BS-90 strain is not (Knight et al., 1999). Resistance and susceptibility are closely related to some special genes. At the genome level, these genes are in the same linkage group with a short distance from each other, of which the genes including Prim1_910, Prim1_771, Prim6_1024 and Prim7_823 are related to resistance, while Prim24_524 is associated with susceptibility (Ittiprasert et al., 2013). At the phenotype level, the resistance of BS-90 strains to S. mansoni could be reversed by knocking down the fibrinogen-related protein FREP3 (Zhang et al., 2008; Hanington et al., 2012). The susceptibility of B. glabrata is also closely linked to the expression of HSP70 and HSP90 induced by mild heat shock (32 °C), leading to the stronger susceptibility of BS-90 strains to S. mansoni. In addition, the inhibition of HSP 90 reverses the susceptible phenotype of NMRI strains (Ittiprasert and Knight, 2012). Furthermore, Biomphalaria alexandrina serves as another intermediate host of S. mansoni with higher resistance that is likely attributable to SOD1 activity, levels of polymorphic mucin (SmPoMuc) and NO, determined by the genetic background (Bender et al., 2007). Environmental light can also modulate the susceptibility of B. glabrata to S. mansoni, but the underlying mechanisms remain elusive (Steinauer and Bonner, 2012).
The resistance and susceptibility phenotypes of definitive hosts are closely linked to immune factors, parasite-induced gene expression and hormone levels determined by the genetic background. Immune factors include humoral immunity components, cytokines and immune cells. At the humoral immunity level, the resistance of M. fortis to S. japonicum is dependent on serum albumin and IgG3 helping to eliminate worms (Jiang et al., 2010; Li et al., 2013), whereas in rats, this resistance depends on IgG2a and IgE (Shen et al., 2020). The application of purified albumin from M. fortis infecting mice exerts significant effects on worm load reduction, suggesting a promising target for therapy (Jiang et al., 2010; Li et al., 2013). In terms of cytokines, diverse inflammatory factors (including FN-γ, IL-1β, GM-CSF, M-CSF, MCP-1, VEGF, IL-2, IL-3, IL-4, IL-5, IL-10, IL-12, IL-13 and IL-17) are remarkedly upregulated in hosts upon infection (Hu et al., 2017). Further blockade of IL-4 and IL-13 was shown to notably elevate the worm burden of S. mansoni in rats, indicating that Th2-type cytokines enhance the resistance of nonpermissive hosts (Khalife et al., 2000). However, in permissive hosts, Th2 responses seem beneficial to parasites (Khalife et al., 2000). Regarding immune cells, eosinophils, macrophages and lymphocytes function to eliminate worms in M. fortis (Shen et al., 2020). Based on these findings, adoptive immunotherapy with bone marrow transplanted from M. fortis significantly inhibited the development of worms and reduced the number of worm eggs in immunocompromised mice, showing promising therapeutic efficiency. At the parasite-induced gene expression level, microRNAs, including miR-705 and miR-122, inhibit the development of S. japonicum by affecting lipid uptake, and high levels of iNOS in rats inhibit the development of S. japonicum (Shen et al., 2017). Significantly, the proteins Mf-HSP90a (Gong et al., 2010), Mf-KPNA2 (Cheng et al., 2011), and Mf-E77.43 (Wang et al., 2016) in M. fortis (Shen et al., 2017) and the serum in rats can directly kill S. japonicum (Wang et al., 2005), providing a candidate strategy for schistosomiasis treatment. Furthermore, the differences in hormone levels between males and females also affect the development of S. mansoni, as observed for testosterone in males, which enhances resistance and reduces the adult worm burden in hosts (Nakazawa et al., 1997).
2.5.4 Cestodes
2.5.4.1 Hymenolepis nana and Hymenolepis diminuta
The life cycle of Hymenolepis nana (H. nana) is analogous to that of Hymenolepis diminuta (H. diminuta). Mice and humans serve as their definitive hosts, in which adult worms parasitize the intestine. Similar to most parasites, NO presents obvious inhibition capacity to hymenolepis (Mahmoud and Habib, 2003). In addition, immune factors are also involved in the host adaptation of this parasite. As reported, thymectomized mice displayed a slower efficiency of worm elimination for hymenolepis (Andreassen et al., 1978), suggesting that the resistance of hosts presents certain immune-dependent characteristics (Palmas et al., 1993). In addition, cytokines (such as IL-5 and IL-13) and the inflammation-related transcription factor stat6 promote the expulsion of H. diminuta (McKay and Khan, 2003; Ryan and Oghumu, 2019). Moreover, immune cells, including mast cells and neutrophils, enforce the resistance of hosts to this parasite (Gonzalez et al., 2018).
2.5.4.2 Echinococcus granulosus
The hosts of Echinococcus granulosus (E. granulosus) comprise intermediate hosts and definitive hosts. Artiodactyls (such as sheep, cattle, camels, pigs and deer), horses, kangaroos, rodents, primates and humans are intermediate hosts that can be infected by the hydatid cyst of E. granulosus and develop echinococcosis (Maillard et al., 2009; Otero-Abad and Torgerson, 2013; Kagendo et al., 2014). Dogs, wolves and jackals serve as the definitive hosts parasitized by the adult worms of E. granulosus, in which eggs are produced (Kagendo et al., 2014). Mongolian gerbils, Meriones Meridianus, and Lagurus act as alternative definitive hosts, where the development of worms is distinct from that in natural definitive hosts (Oku et al., 2002; Conchedda et al., 2006).
Regarding intermediate hosts, most infections with the hydatid cysts of E. granulosus result in multiorgan involvement. Specifically, in rodents, liver involvement is most common as a result of the existence of Kupffer cell-specific receptor (KCR), to which hydatid cyst laminated layer glycans directly bind (Fadden et al., 2003; Diaz et al., 2015). In contrast, large mammals, especially ruminants, mainly manifest extrahepatic organ involvement, which may be related to the larger diameter of lymphatic vessels in jejunal villi than that in nonruminant animals, leading to Echinococcus being more likely carried in lymph rather than in the portal circulation (Heath, 1971; Thompson, 2017). The immune factors established by the distinct genetic backgrounds of hosts result in distinct resistance to E. granulosus. When compared with BALB/c mice, C57BL/6 mice present lower vulnerability, which is attributed to the H-2-IAb haplotype T-cell epitope-mediated stronger immune responses to parasites (Miles et al., 2020; Garcia-Luna et al., 2021). Serum from C57BL/6 mice showed explicit therapeutic efficiency in BALB/c mice infected with E. granulosus, which is caused by IgG1 (Mourglia-Ettlin et al., 2016a). The pathogen associated with pattern receptor TLR4 also affects parasite-host adaptation. As reported, TLR4 SNPs and the heterozygous mutant Asp299Gly (A/G) genotype were closely associated with susceptibility to E. granulosus (Noori et al., 2018). In general, the Th1 response displays protective function against hydatid cysts, and the Th2 response shows a positive correlation with susceptibility (Siracusano et al., 2012). However, several critical cytokines (IL-4 and IL-10) of the Th2 response have opposite killing effects on protoscoleces by elevating the levels of NO, which enhances the cytotoxic effect in C57BL/6 mice upon infection (Amri et al., 2009; Mourglia-Ettlin et al., 2016b). In addition, the sex and age of the hosts are also closely related to worm resistance (Blancas Mosqueda et al., 2007; Mourglia-Ettlin et al., 2018).
2.5.4.3 Echinococcus multilocularis
The life cycle of E. multilocularis is similar to that of E. granulosus. The intermediate hosts of E. multilocularis include rodents, cattle, sheep and humans, where the worms fail to develop into sexual maturation (Weingartner et al., 2022). Animals such as foxes, dogs, wolves, badgers and cats serve as definitive hosts, in which adult worms lay eggs that are excreted with feces (Hansen et al., 2004; Karamon et al., 2019).
In general, E. multilocularis parasitizes the host liver in the form of alveolar cercariae and occasionally in other tissues, such as the brain and lungs (Maamri et al., 2022), since insulin in the liver can promote the development of E. multilocularis through the evolutionarily conserved insulin signaling pathway of worms (Hemer et al., 2014). The resistance of different rodents to E. multilocularis is not identical as a consequence of the distinct parasite-specific humoral and cellular immunity gradually established in various strains. Some rodent strains, for example, DBA/2 mice, show higher susceptibility to alveolar echinococcosis than C57BL/6 mice, whereas humans are generally more resistant than nonhuman primates (Nakao et al., 2011; Armua-Fernandez et al., 2016). In addition, other immune factors, including neutrophils and MHC polymorphisms, lower the susceptibility to and onset of echinococcosis in the hosts (Vuitton et al., 2006; Joekel et al., 2021). Hosts with HLA-DR3+ and HLA-DQ2+ haplotypes are more susceptible to progressive alveolar echinococcosis. In addition, the lack of iNOS enforces the resistance of mice, which is contradictory to most prior reports, and dexamethasone affects susceptibility by suppressing the immune response (Dai et al., 2003; Armua-Fernandez et al., 2016).
2.6 Nematodes
2.6.1 Trichuris trichiura Linnaeus
As the host of T. trichiura, humans are the only source of infection in which adult worms parasitize the cecum and lay eggs that are excreted into the natural environment and develop to the infectious stage in soil with suitable humidity and temperature (Bethony et al., 2006). After invasion, infective eggs migrate through the small intestine and intestinal lumen, eventually arrive at the cecum, develop into adult worms and cause trichuriasis in hosts (Bethony et al., 2006). Mouse models are the animal models most commonly exploited for trichuriasis investigation, and the divergent genetic backgrounds of mice lead to different susceptibilities due to MHC polymorphisms and immune factors (Else and Wakelin, 1988). The H-2k haplotype (BALB/k, B10.BR) mouse strains are more susceptible to T. trichiura, while H-2b (B10) or H-2q (B10.G) background strains are more resistant. Host sex also affects resistance to T. trichiura via a hormone-regulated immune response (Else and Wakelin, 1988). Dihydrotestosterone inhibits dendritic cell-mediated T-cell activation and suppresses Th2 responses through IL-8, and 17-β-estradiol enforces Th2 responses in an IL-4-dependent manner (Hepworth et al., 2010). Upon infection with T. trichiura, male IL-4-deficient BALB/c mice were found to exhibit chronic infection, while females displayed acute infection (Artis et al., 1999), suggesting that IL-4 plays a genetic-dependent role in the immune response of hosts induced by T. trichiura. In addition, another cytokine, IL-13, is reported to reduce worm burden (Hayes et al., 2007).
2.6.2 Hookworms
A total of 9 species of zoonotic hookworms have been found, among which Ancylostoma duodenale and Necator americanus are the main infection sources to humans. Others, such as Ancylostoma ceyloides (A. ceyloides), Ancylostoma canis (A. canis) and Ancylostoma brasiliensis (A. brasiliensis), also infect humans under certain circumstances. Hookworms manifest obvious infection diversity in hosts (Seguel and Gottdenker, 2017). Ancylostoma caninum, for example, is believed to parasitize only dogs and has been found to infect humans and cats in some cases (Bahgat et al., 1999; Kwon et al., 2003; Liu et al., 2013). The climate is also closely associated with infection. Epidemiological investigation has revealed that the prevalence of hookworm infection is much lower in June and winter than in the monsoon season (Croese, 1995).
Humans act as nonpermissive hosts of A. brasiliensis, and worms fail to develop into adults. Mouse models are the most commonly used models to study the host adaptation of A. brasiliensis. Studies have noted the important roles of Th2 immune responses in the host adaptation of A. brasiliensis. Th2-type cytokines such as IL-4 and IL-13 limit reproduction and survival and promote the elimination of worms (Urban et al., 1995; Gerbe et al., 2016; Symowski and Voehringer, 2019). The phospholipase A2 group 1b (pla2g1b) excreted by host epithelial cells induces Th2 immune responses to kill A. brasiliensis, while mice lacking pla2g1b fail to expel worms (Entwistle et al., 2017). The expression of pla2g1b in epithelial cells relies on the intestinal microbiota, adaptive immunity and coenzyme γ chain-dependent signaling. Due to the differences in genetic backgrounds, pla2g1b expression is distinctive among hosts, which is speculated to affect the host adaptation of A. brasiliensis (Entwistle et al., 2017). In addition, the inflammation-related transcription factor STAT6 enhances worm elimination by promoting hookworm capture and degradation in host mucosa (Ishiwata et al., 2002; Van Panhuys et al., 2013).
2.6.3 Strongyloides stercoralis
Strongyloides stercoralis (S. stercoralis) is a species of facultative parasites, and the life cycle includes free-living and parasitic generations. The hosts of parasitic generations comprise humans, dogs, cats, gerbils and mice (Lok, 2007; Bonne-Annee et al., 2011; Dantas-Torres and Otranto, 2014). S. stercoralis derived from humans can also infect dogs (Jaleta et al., 2017), resulting in chronic infection (Schad et al., 1984). Some hosts, including gerbils and C57BL/6J and SCID mice, show natural resistance to S. stercoralis infection by inhibiting the development of worms (Dawkins and Grove, 1982; Nolan et al., 1999).
The adults of S. stercoralis parasitize the small intestine of the host, and larvae can migrate into the main organs, such as the heart, liver, spleen and lungs, leading to strongyloidiasis. Before invasion, the larvae probably distinguish hosts and nonhosts by sensing host smell and skin temperature through the cGMP-gated cation channel subunit tax-4 (Bryant et al., 2018; Gang et al., 2020). In addition, the CO2 concentration and unique uridylic acid of skin also impact larval infection, but the molecular mechanism remains elusive (Safer et al., 2007). DAF-12 is a nematode-specific nuclear receptor and probably governs the development of S. stercoralis (Cheong et al., 2021), providing a therapeutic target for strongyloidiasis. As reported, treatment with Δ7-dafachronic acid significantly reduces worm burden by targeting DAF-12 (Patton et al., 2018). In terms of the host, the hormone level and immune factors exhibit effects on S. stercoralis infection. Recent studies have shown that endogenous cortisol mimics parasitic ecdysone and promotes the development of bacillary cercariae to filamentous cercariae, leading to persistent infection (Teixeira et al., 2016). Immunocompetent patients infected by S. stercoralis regularly present asymptomatic infections, while immunocompromised patients exhibit severe infections (Abanyie et al., 2015). The underlying mechanism might be impaired MHC II-mediated Th2 responses (Rodrigues et al., 2009).
2.6.4 Trichinella spiralis
Trichinella spiralis (T. spiralis) is one of the most frequent parasites of carnivorous mammals (Pozio, 2000; Scandrett et al., 2018). The adult worms infest the duodenum and jejunum of diverse hosts, where they lay newborn larvae that reach skeletal muscle with blood circulation and form infectious larval sacs. The life cycle of T. spiralis can be completed in the same host; thus, the host serves as both an intermediate host and a definitive host (Pozio, 2000; Scandrett et al., 2018). In view of different genetic backgrounds, different hosts infected by T. spiralis present distinct phenotypes, including cystic and noncystic foci (Pozio et al., 2004), suggesting that genetic factors affect pathogenicity. The development of T. spiralis in hosts is vulnerable to microenvironmental changes caused by immune and nonimmune factors (Huang et al., 2015). Eosinophils can promote larval growth by inhibiting local inflammation and enhancing nutrient absorption and metabolism (Huang et al., 2015). In contrast, intestinal mast cells promote the exclusion of worms from the gastrointestinal tract by increasing the permeability of intestinal epithelial cells (McDermott et al., 2003). Leptin reduction is involved in facilitating parasite expulsion by augmenting protective Th2 immune responses (Worthington et al., 2013). However, β-glucan enhances worm expulsion through the mucus layer independent of Th2 immunity (Jin et al., 2022).
2.6.5 Filaria
Filaria comprises multiple subspecies, of which nearly 8 infect humans, yet the parasitic sites and pathological outcomes vary greatly as a result of the genetic differences among hosts. Infected patients often present severe lymphadenitis or blindness. The hosts of filaria include intermediate and definitive hosts. The intermediate hosts of Filaria vary from mosquitoes, Simulium, and Tabanus to Culicoides (Brianti et al., 2012). Humans and some animals, such as dogs, foxes, wolves and cats, serve as definitive hosts (McNulty et al., 2013) and become infected when bitten by mosquitoes containing infective host-specific Filaria subspecies (Vakalis and Himonas, 1997). Generally, animal-specific Filaria fail to develop sexually and produce microfilariae in humans. This is likely attributed to the host immune response promoting worm elimination (Pampiglione et al., 1995).
In experimental animal models with distinct genetic backgrounds, the susceptibility and resistance to Filari also exhibit great differences. Litomosoides sigmodontis (L. sigmodontis) can develop into sexual maturity in BALB/c mice, and the infectious microfilariae can be released into the surrounding environment; however, in C57BL/6 mice, the worms are eliminated before microfilariae are released (Petit et al., 1992; Graham et al., 2005; Attout et al., 2008). One of the most likely causes is the stronger Th1/Th2 immune responses present in C57BL/6 mice (Babayan et al., 2003). In addition, C57BL/6 mice with MHC class-II and RAG2IL-2Rγ loss show impaired resistance to Filaria, suggesting that adaptive immunity plays a crucial role during L. sigmodontis infection (Hoffmann et al., 2001; Wiszniewsky et al., 2021). In line with these findings, cytokines (IL-4, IL-10, IL-17 and IFN-γ) and chemokines (CXCL12 and CCL17) tightly related to adaptive immunity have evident effects on the development, migration and survival of infective larvae (Hoffmann et al., 2001; Saeftel et al., 2001; Specht et al., 2011; Bouchery et al., 2012; Ritter et al., 2018). IL-4, IL-17, IFN-γ, and CXCL12 are required to repress filarial nematode development and survival, while IL-10 promotes the persistence of L. sigmodontis Mf in hosts; CCL17 controls filarial larval entry by inhibiting vascular permeability. Furthermore, the chemokine receptor CCR3 usually mediates the recruitment of innate immune cells, and BALB/c mice lacking CCR3 exhibit higher susceptibility to human-specific Loa loa castellani, indicating the critical role of innate immunity in the anti-infection abilities of L. sigmodontis (Fombad et al., 2019). Consistently, the accumulation of innate immune cell neutrophils in hosts significantly inhibits the motility and pathogenicity of L. sigmodontis (Frohberger et al., 2020). Moreover, L. sigmodontis was found to exploit coevolution with other pathogens, such as Wolbachia, to achieve immune escape and enforce host adaptation (Slatko et al., 2014; Lefoulon et al., 2016). The killing of Wolbachia with antibiotics favors Filaria elimination (Taylor et al., 2014), which is likely attributable to the perturbation of the host immune response by Wolbachia (Brattig, 2004).
2.6.6 Angiostrongylus cantonensis
Angiostrongylus cantonensis (A. cantonensis) remains one of the leading causes of eosinophilic meningoencephalitis worldwide. Infections often occur through the ingestion of food or contaminated water containing third-stage larvae (Zhou et al., 2019). The definitive hosts of A. cantonensis include permissive hosts (rats, tree shrews, dogs, meerkats, tree rats, etc.) and nonpermissive hosts (mice, humans, parrots, Mongolian gerbils, etc.) (Wallace and Rosen, 1969; Reece et al., 2013; Okano et al., 2014; Wei et al., 2014; Zhou et al., 2019). In permissive hosts, the worms develop into fourth-/fifth-stage larvae in the host brain, resulting in meningeal thickening and a severe inflammatory response. However, at 28 days after infection, the worms migrate from the brain to the lung, where they develop into adults and lay eggs along with mitigated pathological responses in the brain (Zhou et al., 2019). In nonpermissive hosts, the worms fail to leave the lungs and die in the brain, leading to severe eosinophilic meningoencephalitis (Zhou et al., 2021).
Although it is generally acknowledged that A. cantonensis fails to move from the cerebrum to pulmonary tissue in nonpermissive hosts such as humans, parrots and Mongolian gerbils, sporadic cases with worms present in the lungs are still observed. In humans, the worms were observed in both the brain and lungs of a male infant infected with A. cantonensis (Lindo et al., 2004). In addition, the worms of A. cantonensis are also found in the lungs of parrots and Mongolian gerbils (Reece et al., 2013; Wei et al., 2014). Such examples reflect the fact that some key factors determine the host adaptation of A. cantonensis. In addition, the perturbation of these factors might affect the migration and development of worms in hosts. When fifth-stage larvae from the brains of the permissive host rats were transferred into the brains of the nonpermissive hosts rabbits or mice, no worms could migrate to the lungs (Ko, 1979), suggesting that the genetic backgrounds of hosts likely determine worm migration. In mice without thymuses or eosinophils, A. cantonensis larvae acquire the ability to migrate from the brain to the lungs but fail to develop sexually, indicating that larval migration in hosts occurs in an immunity-dependent manner, yet development relies on other factors (Yoshimura et al., 1982; Sasaki et al., 1993).
3 Conclusions
Parasites remain one of the most threatening pathogens to humans worldwide. A promising strategy to control parasitosis is to intervene in deleterious parasite-host adaptation, which comprises parasite migration, development, survival and pathogenicity in hosts as well as host susceptibility and resistance to parasites. As a consequence of the distinct genetic backgrounds of hosts, after infection, parasite-host adaptations vary and result in different outcomes. In this article, parasite-host adaptation closely correlated with host genetic background-related factors and the underlying mechanisms were reviewed. In summary, various genetically related factors, including hormones, NO, immune cells, cytokines, genetic polymorphisms and metabolites, affect parasite-host adaptation (Figure 1), among which most factors function by modulating host immune responses. Thus, we have established an interaction network of host genetic background- and immune-related factors involved in parasite resistance to better depict the underlying mechanisms (Figure 2). Importantly, the host genetic background generally determines the differences in immune factors. The genetic background of the C57BL/6 mouse strain leads these mice to possess stronger Th1 response components and thus commonly a higher resistance to parasites than BALB/c mice. In contrast, the genetic background of the BALB/c mouse strain leads these mice to present a stronger Th2 response than C57BK/6 mice and often exhibit susceptibility to parasites. In addition, host genetic backgrounds also influence hormone levels, which could affect parasite resistance in immune-dependent or immune-independent ways (Figure 2; Table 1). The majority of immune-related factors exert explicit antiparasite (Th1, NK, B cells, DC, etc.) or proparasite (Treg and insulin) functions apart from NO, eosinophil, and Th2 response components. In hosts with distinct genetic backgrounds, a lack of NO is positively or negatively correlated with parasite resistance (Table 2), eosinophils play dual roles in the expulsion and development of parasites, and Th2 cells and related cytokines exhibit contradictory functions (Tables 3, 4). For nonpermissive hosts, the Th2 response regularly promotes parasite elimination, while the Th2 response is beneficial for parasite survival (Tables 3, 4). Furthermore, genetic polymorphisms (mainly MHC polymorphisms) and serum immunoglobulin and complement levels display obvious correlations with host susceptibility and resistance (Table 5). Excitingly, sera derived from patients with parasitosis have shown diagnostic and therapeutic potential. In addition, the existence of parasite-specific receptors on host cells confers infection susceptibility, host-derived metabolites can favor the survival and development of larvae, and some hosts display responses to environmentally induced factors to affect susceptibility (Table 6).
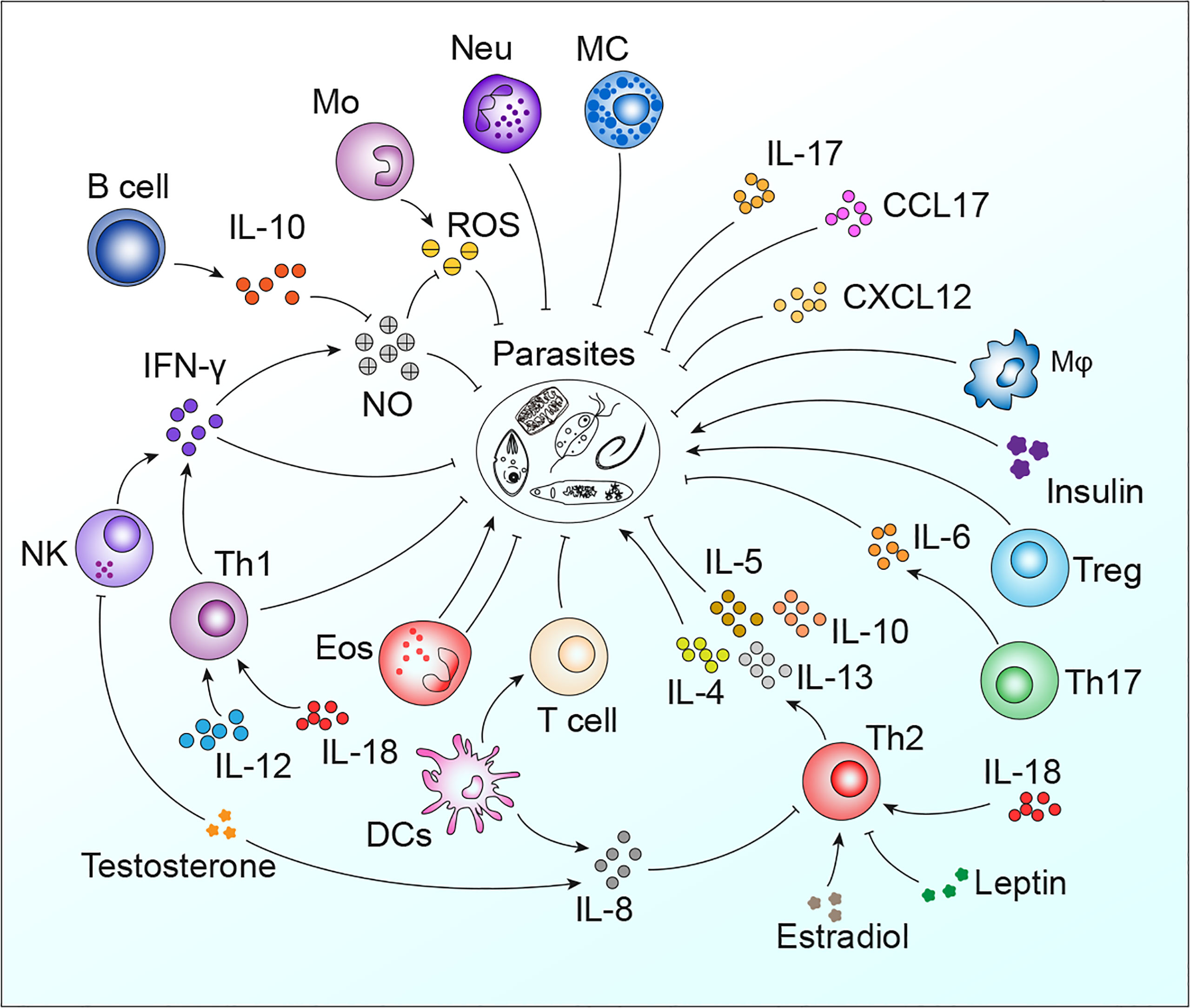
Figure 2 The interaction network of host genetic background- and immune-related factors involved in parasite resistance. Mφ, macrophages; Neu, neutrophils; Mo, monocytes; MC, mast cells.
To further explore the impact of parasite-specific receptors and host metabolites on parasite adaptation, in future studies, a combination of molecular and biochemical approaches can be used. CRISPR/Cas9 technology can be used to knock out or overexpress specific host receptors and investigate their impact on parasitic infection. Metabolomics can also be employed to identify host-derived metabolites that affect parasite growth and development. Furthermore, the utilization of patient-derived serum for the diagnosis and treatment of parasitic diseases can be a promising strategy. Investigating the specific antibodies and immune molecules present in patient serum that contribute to parasite resistance can aid in the development of targeted therapies for parasitic diseases.
To further understand the complex mechanisms underlying parasite adaptation, in future studies, a multidisciplinary approach, integrating genetics, immunology, biochemistry, and ecology, can be employed. Combining genetic studies with ecological studies can provide insights into how host-parasite coevolution shapes the genetic diversity of both hosts and parasites. In addition, the integration of immunological and biochemical studies can elucidate the molecular mechanisms underlying the impact of host immune cells and molecules on parasite adaptation. Furthermore, the study of the impact of parasite adaptation on host physiological and behavioral traits can provide insights into the broader ecological consequences of parasitic infections. Investigations on the impact of parasite adaptation on host microbiota can also provide insights into the complex interactions among parasites, hosts and the microbiome.
In summary, through an understanding of the underlying mechanisms of parasite-host adaptation from the perspective of host genetic backgrounds, it is expected that promising candidate targets to block the survival and development as well as the pathogenicity of parasites will be obtained. Interventions targeting key factors involved in host adaptation, enhancing the antiparasite immune response, exploiting patient-derived serum, blocking parasite-specific receptors, or interfering with the development and survival of parasites through inhibitors and diet restrictions, could ultimately achieve further advances in parasitosis control and therapy and deepen the understanding of the adaptive differences among parasites in different hosts.
Author contributions
HZ and JL conceived this study and revised the manuscript. CY, LZ, LT, and YD undertook the literature review and drafted the manuscript. All authors contributed to the article and approved the submitted version.
Funding
This study was supported by the National Natural Science Foundation of Chongqing (Grant No. 2023NSCQ-MSX1039) and the Project of Key Technology Innovation Special of Key Diseases of the Chongqing Science and Technology Bureau (Grant No. cstc2020jscx-msxm0165).
Conflict of interest
The authors declare that the research was conducted in the absence of any commercial or financial relationships that could be construed as a potential conflict of interest.
Publisher’s note
All claims expressed in this article are solely those of the authors and do not necessarily represent those of their affiliated organizations, or those of the publisher, the editors and the reviewers. Any product that may be evaluated in this article, or claim that may be made by its manufacturer, is not guaranteed or endorsed by the publisher.
Glossary
References
Abad Dar, M., Holscher, C. (2018). Arginase-1 is responsible for IL-13-mediated susceptibility to trypanosoma cruzi infection. Front. Immunol. 9. doi: 10.3389/fimmu.2018.02790
Abanyie, F. A., Gray, E. B., Delli Carpini, K. W., Yanofsky, A., McAuliffe, I., Rana, M., et al. (2015). Donor-derived Strongyloides stercoralis infection in solid organ transplant recipients in the United States 2009-2013. Am. J. Transplant. 15 (5), 1369–1375. doi: 10.1111/ajt.13137
Abrous, M., Comes, A. M., Gasnier, N., Rondelaud, D., Dreyfuss, G., Chauvin, A., et al. (1998). Morphological variability in Fasciola hepatica eggs in ruminants, rodents and lagomorphs. J. Helminthol 72 (4), 313–317. doi: 10.1017/s0022149x00016667
Ahmadpour, E., Safarpour, H., Xiao, L., Zarean, M., Hatam-Nahavandi, K., Barac, A., et al. (2020). Cryptosporidiosis in HIV-positive patients and related risk factors: A systematic review and meta-analysis. Parasite 27, 27. doi: 10.1051/parasite/2020025
Alfituri, O. A., Quintana, J. F., MacLeod, A., Garside, P., Benson, R. A., Brewer, J. M., et al. (2020). To the skin and beyond: the immune response to African trypanosomes as they enter and exit the vertebrate host. Front. Immunol. 11. doi: 10.3389/fimmu.2020.01250
Al-Khaliq, I. M. A., Mahdi, B. M. (2018). Association between Entamoeba histolytica infection and human leukocyte antigen HLA- DRB1. Ann. Med. Surg. (Lond) 36, 71–74. doi: 10.1016/j.amsu.2018.10.019
Amri, M., Mezioug, D., Touil-Boukoffa, C. (2009). Involvement of IL-10 and IL-4 in evasion strategies of Echinococcus granulosus to host immune response. Eur. Cytokine Netw. 20 (2), 63–68. doi: 10.1684/ecn.2009.0154
Andreassen, J., Hindsbo, O., Ruitenberg, E. J. (1978). Hymenolepis diminuta infections in congenitally athymic (nude) mice: worm kinetics and intestinal histopathology. Immunology 34 (1), 105–113.
Armua-Fernandez, M. T., Joekel, D., Schweiger, A., Eichenberger, R. M., Matsumoto, J., Deplazes, P. (2016). Successful intestinal Echinococcus multilocularis oncosphere invasion and subsequent hepatic metacestode establishment in resistant RccHan:WIST rats after pharmacological immunosuppression. Parasitology 143 (10), 1252–1260. doi: 10.1017/S0031182016000809
Artis, D., Humphreys, N. E., Bancroft, A. J., Rothwell, N. J., Potten, C. S., Grencis, R. K. (1999). Tumor necrosis factor alpha is a critical component of interleukin 13-mediated protective T helper cell type 2 responses during helminth infection. J. Exp. Med. 190 (7), 953–962. doi: 10.1084/jem.190.7.953
Attout, T., Martin, C., Babayan, S. A., Kozek, W. J., Bazzocchi, C., Oudet, F., et al. (2008). Pleural cellular reaction to the filarial infection Litomosoides sigmodontis is determined by the moulting process, the worm alteration, and the host strain. Parasitol. Int. 57 (2), 201–211. doi: 10.1016/j.parint.2008.01.001
Babayan, S., Ungeheuer, M. N., Martin, C., Attout, T., Belnoue, E., Snounou, G., et al. (2003). Resistance and susceptibility to filarial infection with Litomosoides sigmodontis are associated with early differences in parasite development and in localized immune reactions. Infect. Immun. 71 (12), 6820–6829. doi: 10.1128/IAI.71.12.6820-6829.2003
Bahgat, M. A., El Gindy, A. E., Mahmoud, L. A., Hegab, M. H., Shahin, A. M. (1999). Evaluation of the role of Ancylostoma caninum in humans as a cause of acute and recurrent abdominal pain. J. Egypt Soc. Parasitol. 29 (3), 873–882.
Bender, R. C., Goodall, C. P., Blouin, M. S., Bayne, C. J. (2007). Variation in expression of Biomphalaria glabrata SOD1: a potential controlling factor in susceptibility/resistance to Schistosoma mansoni. Dev. Comp. Immunol. 31 (9), 874–878. doi: 10.1016/j.dci.2006.12.005
Bergersen, K. V., Barnes, A., Worth, D., David, C., Wilson, E. H. (2021). Targeted transcriptomic analysis of C57BL/6 and BALB/c mice during progressive chronic toxoplasma gondii infection reveals changes in host and parasite gene expression relating to neuropathology and resolution. Front. Cell Infect. Microbiol. 11. doi: 10.3389/fcimb.2021.645778
Bethony, J., Brooker, S., Albonico, M., Geiger, S. M., Loukas, A., Diemert, D., et al. (2006). Soil-transmitted helminth infections: ascariasis, trichuriasis, and hookworm. Lancet 367 (9521), 1521–1532. doi: 10.1016/S0140-6736(06)68653-4
Blancas Mosqueda, M., Herrera Esparza, R., Rodriguez Padilla, C., Tavizon Garcia, J. P., Mercado Reyes, M., Badillo Almaraz, V., et al. (2007). Gender as a factor of susceptibility to infection in experimental hydatidosis. Rev. Latinoam Microbiol. 49 (1-2), 31–37.
Bonne-Annee, S., Hess, J. A., Abraham, D. (2011). Innate and adaptive immunity to the nematode Strongyloides stercoralis in a mouse model. Immunol. Res. 51 (2-3), 205–214. doi: 10.1007/s12026-011-8258-2
Bouchery, T., Denece, G., Attout, T., Ehrhardt, K., Lhermitte-Vallarino, N., Hachet-Haas, M., et al. (2012). The chemokine CXCL12 is essential for the clearance of the filaria Litomosoides sigmodontis in resistant mice. PLoS One 7 (4), e34971. doi: 10.1371/journal.pone.0034971
Brattig, N. W. (2004). Pathogenesis and host responses in human onchocerciasis: impact of Onchocerca filariae and Wolbachia endobacteria. Microbes Infect. 6 (1), 113–128. doi: 10.1016/j.micinf.2003.11.003
Brianti, E., Otranto, D., Dantas-Torres, F., Weigl, S., Latrofa, M. S., Gaglio, G., et al. (2012). Rhipicephalus sanguineus (Ixodida, Ixodidae) as intermediate host of a canine neglected filarial species with dermal microfilariae. Vet. Parasitol. 183 (3-4), 330–337. doi: 10.1016/j.vetpar.2011.07.031
Bryant, A. S., Ruiz, F., Gang, S. S., Castelletto, M. L., Lopez, J. B., Hallem, E. A. (2018). A critical role for thermosensation in host seeking by skin-penetrating nematodes. Curr. Biol. 28(14), 2338–2347 e2336. doi: 10.1016/j.cub.2018.05.063
Busselman, R. E., Hamer, S. A. (2022). Chagas disease ecology in the United States: recent advances in understanding trypanosoma cruzi transmission among triatomines, wildlife, and domestic animals and a quantitative synthesis of vector-host interactions. Annu. Rev. Anim. Biosci. 10, 325–348. doi: 10.1146/annurev-animal-013120-043949
Caterina, P., Warton, A., Papadimitriou, J. M., Ashman, R. B. (1996). Relationship of the virulence of Trichomonas vaginalis and the major histocompatibility complex in murine trichomonad infection. Parasitol. Res. 82 (7), 628–633. doi: 10.1007/s004360050176
Chadee, K., Meerovitch, E. (1984). The Mongolian gerbil (Meriones unguiculatus) as an experimental host for Entamoeba histolytica. Am. J. Trop. Med. Hyg 33 (1), 47–54. doi: 10.4269/ajtmh.1984.33.47
Cheng, G., Gong, Q., Gai, N., Xiong, D. H., Yu, Y. J., Zeng, Q. R., et al. (2011). Karyopherin alpha 2 (KPNA2) is associated with the natural resistance to Schistosoma Japanicum infection in Microtus fortis. BioMed. Pharmacother. 65 (3), 230–237. doi: 10.1016/j.biopha.2011.02.012
Cheong, M. C., Wang, Z., Jaleta, T. G., Li, X., Lok, J. B., Kliewer, S. A., et al. (2021). Identification of a nuclear receptor/coactivator developmental signaling pathway in the nematode parasite Strongyloides stercoralis. Proc. Natl. Acad. Sci. U.S.A. 118 (8), e2021864118. doi: 10.1073/pnas.2021864118
Chessler, A. D., Caradonna, K. L., Da'dara, A., Burleigh, B. A. (2011). Type I interferons increase host susceptibility to Trypanosoma cruzi infection. Infect. Immun. 79 (5), 2112–2119. doi: 10.1128/IAI.01176-10
Chitnis, C. E., Sharma, A. (2008). Targeting the Plasmodium vivax Duffy-binding protein. Trends Parasitol. 24 (1), 29–34. doi: 10.1016/j.pt.2007.10.004
Clay, G. M., Sutterwala, F. S., Wilson, M. E. (2014). NLR proteins and parasitic disease. Immunol. Res. 59 (1-3), 142–152. doi: 10.1007/s12026-014-8544-x
Conchedda, M., Gabriele, F., Bortoletti, G. (2006). Development and sexual maturation of Echinococcus granulosus adult worms in the alternative definitive host, Mongolian gerbil (Meriones unguiculatus). Acta Trop. 97 (2), 119–125. doi: 10.1016/j.actatropica.2005.08.009
Corbeil, L. B. (1995). Use of an animal model of trichomoniasis as a basis for understanding this disease in women. Clin. Infect. Dis. 21 Suppl 2, S158–S161. doi: 10.1093/clinids/21.supplement_2.s158
Croese, J. (1995). Seasonal influence on human enteric infection by Ancylostoma caninum. Am. J. Trop. Med. Hyg 53 (2), 158–161. doi: 10.4269/ajtmh.1995.53.158
Crosnier, C., Bustamante, L. Y., Bartholdson, S. J., Bei, A. K., Theron, M., Uchikawa, M., et al. (2011). Basigin is a receptor essential for erythrocyte invasion by Plasmodium falciparum. Nature 480 (7378), 534–537. doi: 10.1038/nature10606
Cruz-Reyes, A., Malek, E. A. (1987). Suitability of six lymnaeid snails for infection with Fasciola hepatica. Vet. Parasitol. 24 (3-4), 203–210. doi: 10.1016/0304-4017(87)90041-0
Dai, W. J., Waldvogel, A., Jungi, T., Stettler, M., Gottstein, B. (2003). Inducible nitric oxide synthase deficiency in mice increases resistance to chronic infection with Echinococcus multilocularis. Immunology 108 (2), 238–244. doi: 10.1046/j.1365-2567.2003.01567.x
Dantas-Torres, F., Otranto, D. (2014). Dogs, cats, parasites, and humans in Brazil: opening the black box. Parasit Vectors 7, 22. doi: 10.1186/1756-3305-7-22
da Rocha, R., LaRocque-de-Freitas, I. F., Arcanjo, A. F., Logullo, J., Nunes, M. P., Freire-de-Lima, C. G., et al. (2019). B-1 cells may drive macrophages susceptibility to trypanosoma cruzi infection. Front. Microbiol. 10. doi: 10.3389/fmicb.2019.01598
da Silva, G. A. V., de Mesquita, T. G. R., de Souza Encarnacao, H. V., do Espirito Santo Junior, J., da Costa Sabino, K., de Aguiar Neres, I., et al. (2019). A polymorphism in the IL1B gene (rs16944 T/C) is associated with cutaneous leishmaniasis caused by Leishmania guyanensis and plasma cytokine interleukin receptor antagonist. Cytokine 123, 154788. doi: 10.1016/j.cyto.2019.154788
Davhana, N. C., ElBakri, A. K., Bessong, P. O., Samie, A. (2020). Association of genetic polymorphism at tumor necrosis factor-alpha gene promoter - 1031T/C and parasitic infections among children in Northern South Africa. Heliyon 6 (10), e05129. doi: 10.1016/j.heliyon.2020.e05129
Dawkins, H. J., Grove, D. I. (1982). Attempts to establish infections with Strongyloides stercoralis in mice and other laboratory animals. J. Helminthol 56 (1), 23–26. doi: 10.1017/s0022149x00034957
Dejon-Agobe, J. C., Edoa, J. R., Adegnika, A. A., Grobusch, M. P. (2022). Schistosomiasis in Gabon from 2000 to 2021 - A review. Acta Trop. 228, 106317. doi: 10.1016/j.actatropica.2022.106317
de Sousa, T. N., Kano, F. S., de Brito, C. F., Carvalho, L. H. (2014). The Duffy binding protein as a key target for a Plasmodium vivax vaccine: lessons from the Brazilian Amazon. Mem Inst Oswaldo Cruz 109 (5), 608–617. doi: 10.1590/0074-0276130592
Diaz, A., Fernandez, C., Pittini, A., Seoane, P. I., Allen, J. E., Casaravilla, C. (2015). The laminated layer: Recent advances and insights into Echinococcus biology and evolution. Exp. Parasitol. 158, 23–30. doi: 10.1016/j.exppara.2015.03.019
Dos Santos, J. C., Leite Quixabeira, V. B., Teodoro Silva, M. V., Damen, M., Schraa, K., Jaeger, M., et al. (2020). Genetic variation in Interleukin-32 influence the immune response against New World Leishmania species and susceptibility to American Tegumentary Leishmaniasis. PLoS Negl. Trop. Dis. 14 (2), e0008029. doi: 10.1371/journal.pntd.0008029
Dubey, J. P. (2008). The history of Toxoplasma gondii–the first 100 years. J. Eukaryot Microbiol. 55 (6), 467–475. doi: 10.1111/j.1550-7408.2008.00345.x
Duggal, P., Guo, X., Haque, R., Peterson, K. M., Ricklefs, S., Mondal, D., et al. (2011). A mutation in the leptin receptor is associated with Entamoeba histolytica infection in children. J. Clin. Invest. 121 (3), 1191–1198. doi: 10.1172/JCI45294
Dupont, C. D., Christian, D. A., Hunter, C. A. (2012). Immune response and immunopathology during toxoplasmosis. Semin. Immunopathol. 34, 793–813. doi: 10.1007/s00281-012-0339-3
Else, K., Wakelin, D. (1988). The effects of H-2 and non-H-2 genes on the expulsion of the nematode Trichuris muris from inbred and congenic mice. Parasitology 96 (Pt 3), 543–550. doi: 10.1017/s0031182000080173
Entwistle, L. J., Pelly, V. S., Coomes, S. M., Kannan, Y., Perez-Lloret, J., Czieso, S., et al. (2017). Epithelial-cell-derived phospholipase A2 group 1B is an endogenous anthelmintic. Cell Host Microbe 22 (4), 484–493 e485. doi: 10.1016/j.chom.2017.09.006
Eriksen, L. (1980). Fasciola hepatica: influence of thymus function on the course of infection in mice. Nord Vet. Med. 32 (6), 243–254.
Fadden, A. J., Holt, O. J., Drickamer, K. (2003). Molecular characterization of the rat Kupffer cell glycoprotein receptor. Glycobiology 13 (7), 529–537. doi: 10.1093/glycob/cwg068
Fombad, F. F., Njouendou, A. J., Ndongmo, P. C., Ritter, M., Chunda, V. C., Metuge, H. M., et al. (2019). Effect of flubendazole on developing stages of Loa loa in vitro and in vivo: a new approach for screening filaricidal agents. Parasit Vectors 12 (1), 14. doi: 10.1186/s13071-018-3282-x
Forni, D., Pontremoli, C., Cagliani, R., Pozzoli, U., Clerici, M., Sironi, M. (2015). Positive selection underlies the species-specific binding of Plasmodium falciparum RH5 to human basigin. Mol. Ecol. 24 (18), 4711–4722. doi: 10.1111/mec.13354
Frenkel, J. K., Dubey, J. P., Miller, N. L. (1970). Toxoplasma gondii in cats: fecal stages identified as coccidian oocysts. Science 167 (3919), 893–896. doi: 10.1126/science.167.3919.893
Frohberger, S. J., Fercoq, F., Neumann, A. L., Surendar, J., Stamminger, W., Ehrens, A., et al. (2020). S100A8/S100A9 deficiency increases neutrophil activation and protective immune responses against invading infective L3 larvae of the filarial nematode Litomosoides sigmodontis. PLoS Negl. Trop. Dis. 14 (2), e0008119. doi: 10.1371/journal.pntd.0008119
Galen, S. C., Borner, J., Martinsen, E. S., Schaer, J., Austin, C. C., West, C. J., et al. (2018). The polyphyly of Plasmodium: comprehensive phylogenetic analyses of the malaria parasites (order Haemosporida) reveal widespread taxonomic conflict. R Soc. Open Sci. 5 (5), 171780. doi: 10.1098/rsos.171780
Gang, S. S., Castelletto, M. L., Yang, E., Ruiz, F., Brown, T. M., Bryant, A. S., et al. (2020). Chemosensory mechanisms of host seeking and infectivity in skin-penetrating nematodes. Proc. Natl. Acad. Sci. U.S.A. 117 (30), 17913–17923. doi: 10.1073/pnas.1909710117
Garcia-Luna, J., Magnone, J., Miles, S., Lopez-Santurio, C., Dematteis, S., Mourglia-Ettlin, G. (2021). Polyreactive antibodies as potential humoral biomarkers of host resistance to cystic echinococcosis. Parasite Immunol. 43 (3), e12802. doi: 10.1111/pim.12802
Gerbe, F., Sidot, E., Smyth, D. J., Ohmoto, M., Matsumoto, I., Dardalhon, V., et al. (2016). Intestinal epithelial tuft cells initiate type 2 mucosal immunity to helminth parasites. Nature 529 (7585), 226–230. doi: 10.1038/nature16527
Gomez Morales, M. A., Ausiello, C. M., Guarino, A., Urbani, F., Spagnuolo, M. I., Pignata, C., et al. (1996). Severe, protracted intestinal cryptosporidiosis associated with interferon gamma deficiency: pediatric case report. Clin. Infect. Dis. 22 (5), 848–850. doi: 10.1093/clinids/22.5.848
Goncalves, B. P., Gupta, S., Penman, B. S. (2016). Sickle haemoglobin, haemoglobin C and malaria mortality feedbacks. Malar J. 15, 26. doi: 10.1186/s12936-015-1077-5
Goncalves de Albuquerque, S. D. C., da Costa Oliveira, C. N., Vaitkevicius-Antao, V., Silva, A. C., Luna, C. F., de Lorena, V. M. B., et al. (2019). Study of association of the rs2275913 IL-17A single nucleotide polymorphism and susceptibility to cutaneous leishmaniasis caused by Leishmania Braziliensis. Cytokine 123, 154784. doi: 10.1016/j.cyto.2019.154784
Gong, Q., Cheng, G., Qin, Z. Q., Xiong, D. H., Yu, Y. J., Zeng, Q. R., et al. (2010). Identification of the resistance of a novel molecule heat shock protein 90alpha (HSP90alpha) in Microtus fortis to Schistosoma japonicum infection. Acta Trop. 115 (3), 220–226. doi: 10.1016/j.actatropica.2010.03.007
Gonzalez, M. I., Lopes, F., McKay, D. M., Reyes, J. L. (2018). Mast cell deficiency in mice results in biomass overgrowth and delayed expulsion of the rat tapeworm Hymenolepis diminuta. Biosci. Rep. 38 (6), BSR20180687. doi: 10.1042/BSR20180687
Graham, A. L., Taylor, M. D., Le Goff, L., Lamb, T. J., Magennis, M., Allen, J. E. (2005). Quantitative appraisal of murine filariasis confirms host strain differences but reveals that BALB/c females are more susceptible than males to Litomosoides sigmodontis. Microbes Infect. 7 (4), 612–618. doi: 10.1016/j.micinf.2004.12.019
Groneberg, M., Hoenow, S., Marggraff, C., Fehling, H., Metwally, N. G., Hansen, C., et al. (2022). HIF-1alpha modulates sex-specific Th17/Treg responses during hepatic amoebiasis. J. Hepatol. 76 (1), 160–173. doi: 10.1016/j.jhep.2021.09.020
Hanington, P. C., Forys, M. A., Loker, E. S. (2012). A somatically diversified defense factor, FREP3, is a determinant of snail resistance to schistosome infection. PLoS Negl. Trop. Dis. 6 (3), e1591. doi: 10.1371/journal.pntd.0001591
Hansen, F., Jeltsch, F., Tackmann, K., Staubach, C., Thulke, H. H. (2004). Processes leading to a spatial aggregation of Echinococcus multilocularis in its natural intermediate host Microtus arvalis. Int. J. Parasitol. 34 (1), 37–44. doi: 10.1016/j.ijpara.2003.10.003
Hayakawa, T., Satta, Y., Gagneux, P., Varki, A., Takahata, N. (2001). Alu-mediated inactivation of the human CMP- N-acetylneuraminic acid hydroxylase gene. Proc. Natl. Acad. Sci. U.S.A. 98 (20), 11399–11404. doi: 10.1073/pnas.191268198
Hayes, K. S., Bancroft, A. J., Grencis, R. K. (2007). The role of TNF-alpha in Trichuris muris infection I: influence of TNF-alpha receptor usage, gender and IL-13. Parasite Immunol. 29 (11), 575–582. doi: 10.1111/j.1365-3024.2007.00979.x
Heath, D. D. (1971). The migration of oncospheres of Taenia pisiformis, T. serialis and Echinococcus granulosus within the intermediate host. Int. J. Parasitol. 1 (2), 145–152. doi: 10.1016/0020-7519(71)90008-7
Hellgren, O., Bensch, S., Malmqvist, B. (2008). Bird hosts, blood parasites and their vectors–associations uncovered by molecular analyses of blackfly blood meals. Mol. Ecol. 17 (6), 1605–1613. doi: 10.1111/j.1365-294X.2007.03680.x
Hemer, S., Konrad, C., Spiliotis, M., Koziol, U., Schaack, D., Forster, S., et al. (2014). Host insulin stimulates Echinococcus multilocularis insulin signalling pathways and larval development. BMC Biol. 12, 5. doi: 10.1186/1741-7007-12-5
Hepworth, M. R., Hardman, M. J., Grencis, R. K. (2010). The role of sex hormones in the development of Th2 immunity in a gender-biased model of Trichuris muris infection. Eur. J. Immunol. 40 (2), 406–416. doi: 10.1002/eji.200939589
Hiyama, K., Hamano, S., Nakamura, T., Nomoto, K., Tada, I. (2001). IL-4 reduces resistance of mice to Trypanosoma cruzi infection. Parasitol. Res. 87 (4), 269–274. doi: 10.1007/pl00008577
Hoffmann, W. H., Pfaff, A. W., Schulz-Key, H., Soboslay, P. T. (2001). Determinants for resistance and susceptibility to microfilaraemia in Litomosoides sigmodontis filariasis. Parasitology 122 (Pt 6), 641–649. doi: 10.1017/s0031182001007892
Holscher, C., Kohler, G., Muller, U., Mossmann, H., Schaub, G. A., Brombacher, F. (1998). Defective nitric oxide effector functions lead to extreme susceptibility of Trypanosoma cruzi-infected mice deficient in gamma interferon receptor or inducible nitric oxide synthase. Infect. Immun. 66 (3), 1208–1215. doi: 10.1128/IAI.66.3.1208-1215.1998
Hu, Y., Sun, L., Yuan, Z., Xu, Y., Cao, J. (2017). High throughput data analyses of the immune characteristics of Microtus fortis infected with Schistosoma japonicum. Sci. Rep. 7 (1), 11311. doi: 10.1038/s41598-017-11532-2
Huang, L., Beiting, D. P., Gebreselassie, N. G., Gagliardo, L. F., Ruyechan, M. C., Lee, N. A., et al. (2015). Eosinophils and IL-4 support nematode growth coincident with an innate response to tissue injury. PLoS Pathog. 11 (12), e1005347. doi: 10.1371/journal.ppat.1005347
Hunter, P. R., Hughes, S., Woodhouse, S., Raj, N., Syed, Q., Chalmers, R. M., et al. (2004). Health sequelae of human cryptosporidiosis in immunocompetent patients. Clin. Infect. Dis. 39 (4), 504–510. doi: 10.1086/422649
Hunter, P. R., Nichols, G. (2002). Epidemiology and clinical features of Cryptosporidium infection in immunocompromised patients. Clin. Microbiol. Rev. 15 (1), 145–154. doi: 10.1128/CMR.15.1.145-154.2002
Imam, N. F., Eassa, A. H., Shoeib, E. Y., Abo-Raia, G. Y. (2007). Antibody isotypes in urethral swabs of symptomatic and asymptomatic men infected with Trichomonas vaginalis. J. Egypt Soc. Parasitol. 37 (3), 977–988.
Ishiwata, K., Nakao, H., Nakamura-Uchiyama, F., Nawa, Y. (2002). Immune-mediated damage is not essential for the expulsion of Nippostrongylus brasiliensis adult worms from the small intestine of mice. Parasite Immunol. 24 (7), 381–386. doi: 10.1046/j.1365-3024.2002.00472.x
Ittiprasert, W., Knight, M. (2012). Reversing the resistance phenotype of the Biomphalaria glabrata snail host Schistosoma mansoni infection by temperature modulation. PLoS Pathog. 8 (4), e1002677. doi: 10.1371/journal.ppat.1002677
Ittiprasert, W., Miller, A., Su, X. Z., Mu, J., Bhusudsawang, G., Ukoskit, K., et al. (2013). Identification and characterisation of functional expressed sequence tags-derived simple sequence repeat (eSSR) markers for genetic linkage mapping of Schistosoma mansoni juvenile resistance and susceptibility loci in Biomphalaria glabrata. Int. J. Parasitol. 43 (8), 669–677. doi: 10.1016/j.ijpara.2013.03.007
Ivory, C., Kammanadiminti, S., Chadee, K. (2007). Innate resistance to Entamoeba histolytica in murine models. Trends Parasitol. 23 (2), 46–48. doi: 10.1016/j.pt.2006.12.006
Jaleta, T. G., Zhou, S., Bemm, F. M., Schar, F., Khieu, V., Muth, S., et al. (2017). Different but overlapping populations of Strongyloides stercoralis in dogs and humans-Dogs as a possible source for zoonotic strongyloidiasis. PLoS Negl. Trop. Dis. 11 (8), e0005752. doi: 10.1371/journal.pntd.0005752
Jiang, W., Hong, Y., Peng, J., Fu, Z., Feng, X., Liu, J., et al. (2010). Study on differences in the pathology, T cell subsets and gene expression in susceptible and non-susceptible hosts infected with Schistosoma japonicum. PLoS One 5 (10), e13494. doi: 10.1371/journal.pone.0013494
Jin, X., Liu, Y., Wang, J., Wang, X., Tang, B., Liu, M., et al. (2022). beta-Glucan-triggered Akkermansia muciniphila expansion facilitates the expulsion of intestinal helminth via TLR2 in mice. Carbohydr Polym 275, 118719. doi: 10.1016/j.carbpol.2021.118719
Joekel, D. E., Nur, S., Monne Rodriguez, J., Kronenberg, P. A., Kipar, A., LeibundGut-Landmann, S., et al. (2021). Agranulocytosis leads to intestinal Echinococcus multilocularis oncosphere invasion and hepatic metacestode development in naturally resistant Wistar rats. Parasitology 148 (1), 53–62. doi: 10.1017/S0031182020002012
Kagendo, D., Magambo, J., Agola, E. L., Njenga, S. M., Zeyhle, E., Mulinge, E., et al. (2014). A survey for Echinococcus spp. of carnivores in six wildlife conservation areas in Kenya. Parasitol. Int. 63 (4), 604–611. doi: 10.1016/j.parint.2014.04.001
Kalkman, L. C., Hanscheid, T., Krishna, S., Grobusch, M. P. (2022). Fluid therapy for severe malaria. Lancet Infect. Dis. 22 (6), e160–e170. doi: 10.1016/S1473-3099(21)00471-0
Karamon, J., Sroka, J., Dabrowska, J., Bilska-Zajac, E., Zdybel, J., Kochanowski, M., et al. (2019). First report of Echinococcus multilocularis in cats in Poland: a monitoring study in cats and dogs from a rural area and animal shelter in a highly endemic region. Parasit Vectors 12 (1), 313. doi: 10.1186/s13071-019-3573-x
Khalife, J., Cetre, C., Pierrot, C., Capron, M. (2000). Mechanisms of resistance to S. mansoni infection: the rat model. Parasitol. Int. 49 (4), 339–345. doi: 10.1016/s1383-5769(00)00059-3
Kirkpatrick, B. D., Haque, R., Duggal, P., Mondal, D., Larsson, C., Peterson, K., et al. (2008). Association between Cryptosporidium infection and human leukocyte antigen class I and class II alleles. J. Infect. Dis. 197 (3), 474–478. doi: 10.1086/525284
Kloch, A., Mierzejewska, E. J., Welc-Faleciak, R., Bajer, A., Biedrzycka, A. (2023). Cytokine gene polymorphism and parasite susceptibility in free-living rodents: Importance of non-coding variants. PLoS One 18 (1), e0258009. doi: 10.1371/journal.pone.0258009
Knight, M., Miller, A. N., Patterson, C. N., Rowe, C. G., Michaels, G., Carr, D., et al. (1999). The identification of markers segregating with resistance to Schistosoma mansoni infection in the snail Biomphalaria glabrata. Proc. Natl. Acad. Sci. U.S.A. 96 (4), 1510–1515. doi: 10.1073/pnas.96.4.1510
Knockel, J., Molina-Cruz, A., Fischer, E., Muratova, O., Haile, A., Barillas-Mury, C., et al. (2013). An impossible journey? The development of Plasmodium falciparum NF54 in Culex quinquefasciatus. PLoS One 8 (5), e63387. doi: 10.1371/journal.pone.0063387
Ko, R. C. (1979). Host-parasite relationship of Angiostrongylus cantonensis. 1. Intracranial transplantation into various hosts. J. Helminthol 53 (2), 121–126. doi: 10.1017/s0022149x00005824
Kreuels, B., Kreuzberg, C., Kobbe, R., Ayim-Akonor, M., Apiah-Thompson, P., Thompson, B., et al. (2010). Differing effects of HbS and HbC traits on uncomplicated falciparum malaria, anemia, and child growth. Blood 115 (22), 4551–4558. doi: 10.1182/blood-2009-09-241844
Kwon, I. H., Kim, H. S., Lee, J. H., Choi, M. H., Chai, J. Y., Nakamura-Uchiyama, F., et al. (2003). A serologically diagnosed human case of cutaneous larva migrans caused by Ancylostoma caninum. Korean J. Parasitol. 41 (4), 233–237. doi: 10.3347/kjp.2003.41.4.233
Lee, J. S., Kim, I. S., Sohn, W. M., Lee, J., Yong, T. S. (2006). A DNA vaccine encoding a fatty acid-binding protein of Clonorchis sinensis induces protective immune response in Sprague-Dawley rats. Scand. J. Immunol. 63 (3), 169–176. doi: 10.1111/j.1365-3083.2006.01721.x
Lefoulon, E., Bain, O., Makepeace, B. L., d'Haese, C., Uni, S., Martin, C., et al. (2016). Breakdown of coevolution between symbiotic bacteria Wolbachia and their filarial hosts. PeerJ 4, e1840. doi: 10.7717/peerj.1840
Li, R., Wu, G. J., Xiong, D. H., Gong, Q., Yu, R. J., Hu, W. X. (2013). A Microtus fortis protein, serum albumin, is a novel inhibitor of Schistosoma japonicum schistosomula. Mem Inst Oswaldo Cruz 108 (7), 865–872. doi: 10.1590/0074-0276130659
Li, S., Yoo, W. G., Song, J. H., Kim, T. I., Hong, S. J. (2018). Bile acids drive chemotaxis of Clonorchis sinensis juveniles to the bile duct. PLoS Negl. Trop. Dis. 12 (10), e0006818. doi: 10.1371/journal.pntd.0006818
Lindo, J. F., Escoffery, C. T., Reid, B., Codrington, G., Cunningham-Myrie, C., Eberhard, M. L. (2004). Fatal autochthonous eosinophilic meningitis in a Jamaican child caused by Angiostrongylus cantonensis. Am. J. Trop. Med. Hyg 70 (4), 425–428. doi: 10.4269/ajtmh.2004.70.425
Liu, W., Sundararaman, S. A., Loy, D. E., Learn, G. H., Li, Y., Plenderleith, L. J., et al. (2016). Multigenomic delineation of plasmodium species of the laverania subgenus infecting wild-living chimpanzees and gorillas. Genome Biol. Evol. 8 (6), 1929–1939. doi: 10.1093/gbe/evw128
Liu, Y., Zheng, G., Alsarakibi, M., Zhang, X., Hu, W., Lu, P., et al. (2013). Molecular identification of Ancylostoma caninum isolated from cats in southern China based on complete ITS sequence. BioMed. Res. Int. 2013, 868050. doi: 10.1155/2013/868050
Lok, J. B. (2007). Strongyloides stercoralis: a model for translational research on parasitic nematode biology. WormBook, 1–18. doi: 10.1895/wormbook.1.134.1
Lotter, H., Helk, E., Bernin, H., Jacobs, T., Prehn, C., Adamski, J., et al. (2013). Testosterone increases susceptibility to amebic liver abscess in mice and mediates inhibition of IFNgamma secretion in natural killer T cells. PLoS One 8 (2), e55694. doi: 10.1371/journal.pone.0055694
Louicharoen, C., Patin, E., Paul, R., Nuchprayoon, I., Witoonpanich, B., Peerapittayamongkol, C., et al. (2009). Positively selected G6PD-Mahidol mutation reduces Plasmodium vivax density in Southeast Asians. Science 326 (5959), 1546–1549. doi: 10.1126/science.1178849
Maamri, K., Cherif, I., Trifa, A., Nessib, N., Elkahla, G., Darmoul, M. (2022). Hydatid cyst in the third ventricle of the brain: case report of an exceptionally rare condition. Childs Nerv Syst. 38 (10), 1845–1846. doi: 10.1007/s00381-022-05460-4
Mahmoud, M. S., Habib, F. S. (2003). Role of nitric oxide in host defense against Hymenolepis nana infection. J. Egypt Soc. Parasitol. 33 (2), 485–496.
Maillard, S., Gottstein, B., Haag, K. L., Ma, S., Colovic, I., Benchikh-Elfegoun, M. C., et al. (2009). The EmsB tandemly repeated multilocus microsatellite: a new tool to investigate genetic diversity of Echinococcus granulosus sensu lato. J. Clin. Microbiol. 47 (11), 3608–3616. doi: 10.1128/JCM.00938-09
Martinez-de la Puente, J., Martinez, J., Rivero-de Aguilar, J., Herrero, J., Merino, S. (2011). On the specificity of avian blood parasites: revealing specific and generalist relationships between haemosporidians and biting midges. Mol. Ecol. 20 (15), 3275–3287. doi: 10.1111/j.1365-294X.2011.05136.x
Martinotti, M. G., Musso, T., Savoia, D. (1988). Influence of gender in pathogenesis of trichomoniasis in congenitally athymic (nude) mice. Genitourin Med. 64 (1), 18–21. doi: 10.1136/sti.64.1.18
Martorelli Di Genova, B., Wilson, S. K., Dubey, J. P., Knoll, L. J. (2019). Intestinal delta-6-desaturase activity determines host range for Toxoplasma sexual reproduction. PLoS Biol. 17 (8), e3000364. doi: 10.1371/journal.pbio.3000364
Matta, N. E., Gonzalez, L. P., Pacheco, M. A., Escalante, A. A., Moreno, A. M., Gonzalez, A. D., et al. (2018). Plasmodium parasites in reptiles from the Colombia Orinoco-Amazon basin: a re-description of Plasmodium kentropyxi Lainson R, Landau I, Paperna I 2001 and Plasmodium carmelinoi Lainson R, Franco CM, da Matta R 2010. Parasitol. Res. 117 (5), 1357–1370. doi: 10.1007/s00436-018-5815-9
McDermott, J. R., Bartram, R. E., Knight, P. A., Miller, H. R., Garrod, D. R., Grencis, R. K. (2003). Mast cells disrupt epithelial barrier function during enteric nematode infection. Proc. Natl. Acad. Sci. U.S.A. 100 (13), 7761–7766. doi: 10.1073/pnas.1231488100
McDonald, V. (2000). Host cell-mediated responses to infection with Cryptosporidium. Parasite Immunol. 22 (12), 597–604. doi: 10.1046/j.1365-3024.2000.00343.x
McKay, D. M., Khan, W. I. (2003). STAT-6 is an absolute requirement for murine rejection of Hymenolepis diminuta. J. Parasitol. 89 (1), 188–189. doi: 10.1645/0022-3395(2003)089[0188:SIAARF]2.0.CO;2
McNair, N. N., Mead, J. R. (2013). CD4+ effector and memory cell populations protect against Cryptosporidium parvum infection. Microbes Infect. 15 (8-9), 599–606. doi: 10.1016/j.micinf.2013.04.009
McNulty, S. N., Mitreva, M., Weil, G. J., Fischer, P. U. (2013). Inter and intra-specific diversity of parasites that cause lymphatic filariasis. Infect. Genet. Evol. 14, 137–146. doi: 10.1016/j.meegid.2012.11.002
Micucci, L. R., Bazan, P. C., Fauro, R., Baez, A., Lo Presti, M. S., Triquel, M. F., et al. (2010). [Importance of host sex in the development of trypanosoma cruzi infection]. Rev. Fac Cien Med. Univ Nac Cordoba 67 (2), 73–76.
Miles, S., Magnone, J., Cyrklaff, M., Arbildi, P., Frischknecht, F., Dematteis, S., et al. (2020). Linking murine resistance to secondary cystic echinococcosis with antibody responses targeting Echinococcus granulosus tegumental antigens. Immunobiology 225 (3), 151916. doi: 10.1016/j.imbio.2020.151916
Miyazaki, Y., Hamano, S., Wang, S., Shimanoe, Y., Iwakura, Y., Yoshida, H. (2010). IL-17 is necessary for host protection against acute-phase Trypanosoma cruzi infection. J. Immunol. 185 (2), 1150–1157. doi: 10.4049/jimmunol.0900047
Molina-Cruz, A., Canepa, G. E., Alves, E. S. T. L., Williams, A. E., Nagyal, S., Yenkoidiok-Douti, L., et al. (2020). Plasmodium falciparum evades immunity of anopheline mosquitoes by interacting with a Pfs47 midgut receptor. Proc. Natl. Acad. Sci. U.S.A. 117 (5), 2597–2605. doi: 10.1073/pnas.1917042117
Mourglia-Ettlin, G., Cucher, M., Arbildi, P., Rosenzvit, M., Dematteis, S. (2016a). Natural and induced antibodies contribute to differential susceptibility to secondary cystic echinococcosis of Balb/c and C57Bl/6 mice. Immunobiology 221 (1), 103–115. doi: 10.1016/j.imbio.2015.07.016
Mourglia-Ettlin, G., Merlino, A., Capurro, R., Dematteis, S. (2016b). Susceptibility and resistance to Echinococcus granulosus infection: Associations between mouse strains and early peritoneal immune responses. Immunobiology 221 (3), 418–426. doi: 10.1016/j.imbio.2015.11.012
Mourglia-Ettlin, G., Miles, S., Hernandez, A., Dematteis, S. (2018). Ageing impairs parasite-specific antibody responses in cystic echinococcosis. Parasite Immunol. 40 (1), e12505. doi: 10.1111/pim.12505
Muchmore, E. A., Diaz, S., Varki, A. (1998). A structural difference between the cell surfaces of humans and the great apes. Am. J. Phys. Anthropol 107 (2), 187–198. doi: 10.1002/(SICI)1096-8644(199810)107:2<187::AID-AJPA5>3.0.CO;2-S
Nakao, R., Kameda, Y., Kouguchi, H., Matsumoto, J., Dang, Z., Simon, A. Y., et al. (2011). Identification of genetic loci affecting the establishment and development of Echinococcus multilocularis larvae in mice. Int. J. Parasitol. 41 (11), 1121–1128. doi: 10.1016/j.ijpara.2011.06.007
Nakazawa, M., Fantappie, M. R., Freeman, G. L., Jr., Eloi-Santos, S., Olsen, N. J., Kovacs, W. J., et al. (1997). Schistosoma mansoni: susceptibility differences between male and female mice can be mediated by testosterone during early infection. Exp. Parasitol. 85 (3), 233–240. doi: 10.1006/expr.1997.4148
Nolan, T. J., Bhopale, V. M., SChad, G. A. (1999). Strongyloides stercoralis: oral transfer of parasitic adult worms produces infection in mice and infection with subsequent autoinfection in gerbils. Int. J. Parasitol. 29 (7), 1047–1051. doi: 10.1016/s0020-7519(99)00068-5
Noori, J., Spotin, A., Ahmadpour, E., Mahami-Oskouei, M., Sadeghi-Bazargani, H., Kazemi, T., et al. (2018). The potential role of toll-like receptor 4 Asp299Gly polymorphism and its association with recurrent cystic echinococcosis in postoperative patients. Parasitol. Res. 117 (6), 1717–1727. doi: 10.1007/s00436-018-5850-6
Okano, T., Haga, A., Mizuno, E., Onuma, M., Nakaya, Y., Nagamine, T. (2014). Angiostrongylus cantonensis (Nematoda: Metastrongylidae) in the Ryukyu Islands tree rat (Diplothrix legata). J. Wildl Dis. 50 (2), 322–325. doi: 10.7589/2013-03-050
Oku, Y., Wei, J., Chai, J. J., Osman, I., Wei, J., Liao, L. F., et al. (2002). Meriones meridianus and Lagurus lagurus as alternative definitive hosts of Echinococcus multilocularis and E. granulosus. Exp. Anim. 51 (1), 27–32. doi: 10.1538/expanim.51.27
Olivos-Garcia, A., Nequiz, M., Liceaga, S., Mendoza, E., Zuniga, P., Cortes, A., et al. (2018). Complement is a rat natural resistance factor to amoebic liver infection. Biosci. Rep. 38 (5), BSR20180713. doi: 10.1042/BSR20180713
Otero-Abad, B., Torgerson, P. R. (2013). A systematic review of the epidemiology of echinococcosis in domestic and wild animals. PLoS Negl. Trop. Dis. 7 (6), e2249. doi: 10.1371/journal.pntd.0002249
Otto, T. D., Gilabert, A., Crellen, T., Bohme, U., Arnathau, C., Sanders, M., et al. (2018). Genomes of all known members of a Plasmodium subgenus reveal paths to virulent human malaria. Nat. Microbiol. 3 (6), 687–697. doi: 10.1038/s41564-018-0162-2
Palmas, C., Ecca, A. R., Gabriele, F. (1993). Specific cross-immunity between Hymenolepis nana and H. diminuta: effects of transfer of immunity with homologous and heterologous immune mesenteric lymph node cells in BALB/c mice. Parassitologia 35 (1-3), 73–76.
Pampiglione, S., Canestri Trotti, G., Rivasi, F. (1995). Human dirofilariasis due to Dirofilaria (Nochtiella) repens: a review of world literature. Parassitologia 37 (2-3), 149–193.
Patton, J. B., Bonne-Annee, S., Deckman, J., Hess, J. A., Torigian, A., Nolan, T. J., et al. (2018). Methylprednisolone acetate induces, and Delta7-dafachronic acid suppresses, Strongyloides stercoralis hyperinfection in NSG mice. Proc. Natl. Acad. Sci. U.S.A. 115 (1), 204–209. doi: 10.1073/pnas.1712235114
Payne, R. O., Silk, S. E., Elias, S. C., Milne, K. H., Rawlinson, T. A., Llewellyn, D., et al. (2017). Human vaccination against Plasmodium vivax Duffy-binding protein induces strain-transcending antibodies. JCI Insight 2 (12), e93683. doi: 10.1172/jci.insight.93683
Perez, A. R., Tamae-Kakazu, M., Pascutti, M. F., Roggero, E., Serra, E., Revelli, S., et al. (2005). Deficient control of Trypanosoma cruzi infection in C57BL/6 mice is related to a delayed specific IgG response and increased macrophage production of pro-inflammatory cytokines. Life Sci. 77 (16), 1945–1959. doi: 10.1016/j.lfs.2005.01.025
Perkins, S. L. (2014). Malaria's many mates: past, present, and future of the systematics of the order Haemosporida. J. Parasitol. 100 (1), 11–25. doi: 10.1645/13-362.1
Petit, G., Diagne, M., Marechal, P., Owen, D., Taylor, D., Bain, O. (1992). Maturation of the filaria Litomosoides sigmodontis in BALB/c mice; comparative susceptibility of nine other inbred strains. Ann. Parasitol. Hum. Comp. 67 (5), 144–150. doi: 10.1051/parasite/1992675144
Pleasance, J., Wiedosari, E., Raadsma, H. W., Meeusen, E., Piedrafita, D. (2011). Resistance to liver fluke infection in the natural sheep host is correlated with a type-1 cytokine response. Parasite Immunol. 33 (9), 495–505. doi: 10.1111/j.1365-3024.2011.01305.x
Plenderleith, L. J., Liu, W., MacLean, O. A., Li, Y., Loy, D. E., Sundararaman, S. A., et al. (2018). Adaptive evolution of RH5 in ape plasmodium species of the laverania subgenus. mBio 9 (1), e02237-17. doi: 10.1128/mBio.02237-17
Powell, M. R., Wassom, D. L. (1993). Host genetics and resistance to acute Trypanosoma cruzi infection in mice. I. Antibody isotype profiles. Parasite Immunol. 15 (4), 215–221. doi: 10.1111/j.1365-3024.1993.tb00603.x
Pozio, E. (2000). Factors affecting the flow among domestic, synanthropic and sylvatic cycles of Trichinella. Vet. Parasitol. 93 (3-4), 241–262. doi: 10.1016/s0304-4017(00)00344-7
Pozio, E., Marucci, G., Casulli, A., Sacchi, L., Mukaratirwa, S., Foggin, C. M., et al. (2004). Trichinella papuae and Trichinella Zimbabwensis induce infection in experimentally infected varans, caimans, pythons and turtles. Parasitology 128 (Pt 3), 333–342. doi: 10.1017/s0031182003004542
Proto, W. R., Siegel, S. V., Dankwa, S., Liu, W., Kemp, A., Marsden, S., et al. (2019). Adaptation of Plasmodium falciparum to humans involved the loss of an ape-specific erythrocyte invasion ligand. Nat. Commun. 10 (1), 4512. doi: 10.1038/s41467-019-12294-3
Ramirez-Emiliano, J., Flores-Villavicencio, L. L., Segovia, J., Arias-Negrete, S. (2007). [Nitric oxide participation during amoebic liver abscess development]. Medicina (B Aires) 67 (2), 167–176.
Reece, R. L., Perry, R. A., Spratt, D. M. (2013). Neuroangiostrongyliasis due to Angiostrongylus cantonensis in gang-gang cockatoos (Callocephalon fimbriatum). Aust. Vet. J. 91 (11), 477–481. doi: 10.1111/avj.12116
Ritter, M., Krupp, V., Wiszniewsky, K., Wiszniewsky, A., Katawa, G., Tamadaho, R. S. E., et al. (2018). Absence of IL-17A in Litomosoides sigmodontis-infected mice influences worm development and drives elevated filarial-specific IFN-gamma. Parasitol. Res. 117 (8), 2665–2675. doi: 10.1007/s00436-018-5959-7
Rodrigues, R. M., Silva, N. M., Goncalves, A. L., Cardoso, C. R., Alves, R., Goncalves, F. A., et al. (2009). Major histocompatibility complex (MHC) class II but not MHC class I molecules are required for efficient control of Strongyloides venezuelensis infection in mice. Immunology 128 (1 Suppl), e432–e441. doi: 10.1111/j.1365-2567.2008.02995.x
Rosypal, A. C., Smith, T., Alexander, A., Weaver, M., Stewart, R., Houston, A., et al. (2014). Serologic survey of antibodies to Trypanosoma cruzi in coyotes and red foxes from Pennsylvania and Tennessee. J. Zoo Wildl Med. 45 (4), 991–993. doi: 10.1638/2014-0117.1
Russler-Germain, E. V., Jung, J., Miller, A. T., Young, S., Yi, J., Wehmeier, A., et al. (2021). Commensal Cryptosporidium colonization elicits a cDC1-dependent Th1 response that promotes intestinal homeostasis and limits other infections. Immunity 54 (11), 2547–2564.e2547. doi: 10.1016/j.immuni.2021.10.002
Ryan, N. M., Oghumu, S. (2019). Role of mast cells in the generation of a T-helper type 2 dominated anti-helminthic immune response. Biosci. Rep. 39 (2), BSR20181771. doi: 10.1042/BSR20181771
Saeftel, M., Volkmann, L., Korten, S., Brattig, N., Al-Qaoud, K., Fleischer, B., et al. (2001). Lack of interferon-gamma confers impaired neutrophil granulocyte function and imparts prolonged survival of adult filarial worms in murine filariasis. Microbes Infect. 3 (3), 203–213. doi: 10.1016/s1286-4579(01)01372-7
Safer, D., Brenes, M., Dunipace, S., SChad, G. (2007). Urocanic acid is a major chemoattractant for the skin-penetrating parasitic nematode Strongyloides stercoralis. Proc. Natl. Acad. Sci. U.S.A. 104 (5), 1627–1630. doi: 10.1073/pnas.0610193104
Sasaki, O., Sugaya, H., Ishida, K., Yoshimura, K. (1993). Ablation of eosinophils with anti-IL-5 antibody enhances the survival of intracranial worms of Angiostrongylus cantonensis in the mouse. Parasite Immunol. 15 (6), 349–354. doi: 10.1111/j.1365-3024.1993.tb00619.x
Scandrett, B., Konecsni, K., Lalonde, L., Boireau, P., Vallee, I. (2018). Detection of natural Trichinella murrelli and Trichinella spiralis infections in horses by routine post-slaughter food safety testing. Food Waterborne Parasitol. 11, 1–5. doi: 10.1016/j.fawpar.2018.06.001
Schad, G. A., Hellman, M. E., Muncey, D. W. (1984). Strongyloides stercoralis: hyperinfection in immunosuppressed dogs. Exp. Parasitol. 57 (3), 287–296. doi: 10.1016/0014-4894(84)90103-6
Schamber-Reis, B. L., Petritus, P. M., Caetano, B. C., Martinez, E. R., Okuda, K., Golenbock, D., et al. (2013). UNC93B1 and nucleic acid-sensing Toll-like receptors mediate host resistance to infection with Leishmania major. J. Biol. Chem. 288 (10), 7127–7136. doi: 10.1074/jbc.M112.407684
Seguel, M., Gottdenker, N. (2017). The diversity and impact of hookworm infections in wildlife. Int. J. Parasitol. Parasites Wildl 6 (3), 177–194. doi: 10.1016/j.ijppaw.2017.03.007
Shen, J., Lai, D. H., Wilson, R. A., Chen, Y. F., Wang, L. F., Yu, Z. L., et al. (2017). Nitric oxide blocks the development of the human parasite Schistosoma japonicum. Proc. Natl. Acad. Sci. U.S.A. 114 (38), 10214–10219. doi: 10.1073/pnas.1708578114
Shen, J., Xiang, S., Peng, M., Zhou, Z., Wu, Z. (2020). Mechanisms of Resistance to Schistosoma japonicum Infection in Microtus fortis, the Natural Non-permissive Host. Front. Microbiol. 11. doi: 10.3389/fmicb.2020.02092
Shibuya, K., Robinson, D., Zonin, F., Hartley, S. B., Macatonia, S. E., Somoza, C., et al. (1998). IL-1 alpha and TNF-alpha are required for IL-12-induced development of Th1 cells producing high levels of IFN-gamma in BALB/c but not C57BL/6 mice. J. Immunol. 160 (4), 1708–1716. doi: 10.4049/jimmunol.160.4.1708
Siracusano, A., Delunardo, F., Teggi, A., Ortona, E. (2012). Host-parasite relationship in cystic echinococcosis: an evolving story. Clin. Dev. Immunol. 2012, 639362. doi: 10.1155/2012/639362
Slatko, B. E., Luck, A. N., Dobson, S. L., Foster, J. M. (2014). Wolbachia endosymbionts and human disease control. Mol. Biochem. Parasitol. 195 (2), 88–95. doi: 10.1016/j.molbiopara.2014.07.004
Specht, S., Frank, J. K., Alferink, J., Dubben, B., Layland, L. E., Denece, G., et al. (2011). CCL17 controls mast cells for the defense against filarial larval entry. J. Immunol. 186 (8), 4845–4852. doi: 10.4049/jimmunol.1000612
Steinauer, M. L., Bonner, K. M. (2012). Host susceptibility is altered by light intensity after exposure to parasites. J. Parasitol. 98 (5), 1052–1054. doi: 10.1645/GE-3109.1
Strauss, M., Acosta-Herrera, M., Alcaraz, A., Casares-Marfil, D., Bosch-Nicolau, P., Lo Presti, M. S., et al. (2019). Association of IL18 genetic polymorphisms with Chagas disease in Latin American populations. PLoS Negl. Trop. Dis. 13 (11), e0007859. doi: 10.1371/journal.pntd.0007859
Sutherland, C. J., Tanomsing, N., Nolder, D., Oguike, M., Jennison, C., Pukrittayakamee, S., et al. (2010). Two nonrecombining sympatric forms of the human malaria parasite Plasmodium ovale occur globally. J. Infect. Dis. 201 (10), 1544–1550. doi: 10.1086/652240
Symowski, C., Voehringer, D. (2019). Th2 cell-derived IL-4/IL-13 promote ILC2 accumulation in the lung by ILC2-intrinsic STAT6 signaling in mice. Eur. J. Immunol. 49 (9), 1421–1432. doi: 10.1002/eji.201948161
Taylor, M. J., Hoerauf, A., Townson, S., Slatko, B. E., Ward, S. A. (2014). Anti-Wolbachia drug discovery and development: safe macrofilaricides for onchocerciasis and lymphatic filariasis. Parasitology 141 (1), 119–127. doi: 10.1017/S0031182013001108
Taylor, M., Thomas, R., Oliver, S., Garner, P. (2022). Community views on mass drug administration for filariasis: a qualitative evidence synthesis. Cochrane Database Syst. Rev. 2, CD013638. doi: 10.1002/14651858.CD013638.pub2
Teixeira, M. C., Pacheco, F. T., Souza, J. N., Silva, M. L., Ines, E. J., Soares, N. M. (2016). Strongyloides stercoralis infection in alcoholic patients. BioMed. Res. Int. 2016, 4872473. doi: 10.1155/2016/4872473
Templeton, T. J., Asada, M., Jiratanh, M., Ishikawa, S. A., Tiawsirisup, S., Sivakumar, T., et al. (2016). Ungulate malaria parasites. Sci. Rep. 6, 23230. doi: 10.1038/srep23230
Thompson, R. C. (2017). Biology and systematics of echinococcus. Adv. Parasitol. 95, 65–109. doi: 10.1016/bs.apar.2016.07.001
Uddin, M. H., Li, S., Bae, Y. M., Choi, M. H., Hong, S. T. (2012). Strain variation in the susceptibility and immune response to Clonorchis sinensis infection in mice. Parasitol. Int. 61 (1), 118–123. doi: 10.1016/j.parint.2011.07.002
Urban, J. F., Jr., Maliszewski, C. R., Madden, K. B., Katona, I. M., Finkelman, F. D. (1995). IL-4 treatment can cure established gastrointestinal nematode infections in immunocompetent and immunodeficient mice. J. Immunol. 154 (9), 4675–4684. doi: 10.4049/jimmunol.154.9.4675
Usanga, E. A., Luzzatto, L. (1985). Adaptation of Plasmodium falciparum to glucose 6-phosphate dehydrogenase-deficient host red cells by production of parasite-encoded enzyme. Nature 313 (6005), 793–795. doi: 10.1038/313793a0
Vakalis, N. C., Himonas, C. A. (1997). Human and canine dirofilariasis in Greece. Parassitologia 39 (4), 389–391.
Valkiunas, G., Ilgunas, M., Bukauskaite, D., Fragner, K., Weissenbock, H., Atkinson, C. T., et al. (2018). Characterization of Plasmodium relictum, a cosmopolitan agent of avian malaria. Malar J. 17 (1), 184. doi: 10.1186/s12936-018-2325-2
Van Panhuys, N., Camberis, M., Yamada, M., Tegoshi, T., Arizono, N., Le Gros, G. (2013). Mucosal trapping and degradation of Nippostrongylus brasiliensis occurs in the absence of STAT6. Parasitology 140 (7), 833–843. doi: 10.1017/S0031182012002260
Villanueva-Saz, S., Giner, J., Marteles, D., Verde, M., Yzuel, A., Riera, C., et al. (2022). Leishmaniosis caused by Leishmania infantum in ferrets: Update review. Vet. Anim. Sci. 15, 100229. doi: 10.1016/j.vas.2021.100229
Von Stebut, E., Ehrchen, J. M., Belkaid, Y., Kostka, S. L., Molle, K., Knop, J., et al. (2003). Interleukin 1alpha promotes Th1 differentiation and inhibits disease progression in Leishmania major-susceptible BALB/c mice. J. Exp. Med. 198 (2), 191–199. doi: 10.1084/jem.20030159
Vuitton, D. A., Zhang, S. L., Yang, Y., Godot, V., Beurton, I., Mantion, G., et al. (2006). Survival strategy of Echinococcus multilocularis in the human host. Parasitol. Int. 55 Suppl, S51–S55. doi: 10.1016/j.parint.2005.11.007
Wallace, G. D., Rosen, L. (1969). Studies on eosinophilic meningitis. V. Molluscan hosts of Angiostrongylus cantonensis on Pacific Islands. Am. J. Trop. Med. Hyg 18 (2), 206–216.
Wang, H. M., Xiong, D. H., Luo, S. Q., Yang, J., Qian, Y. J., Qin, Z. Q. (2016). [Resistance of rAAV2-MfE77.43-Transferred Mice to Schistosoma japonicum Infection]. Zhongguo Ji Sheng Chong Xue Yu Ji Sheng Chong Bing Za Zhi 34 (4), 297–302.
Wang, M., Yi, X. Y., Li, X. P., Zhou, D. M., Larry, M., Zeng, X. F. (2005). Phage displaying peptides mimic schistosoma antigenic epitopes selected by rat natural antibodies and protective immunity induced by their immunization in mice. World J. Gastroenterol. 11 (19), 2960–2966. doi: 10.3748/wjg.v11.i19.2960
Wang, Z. J., Yu, S. M., Gao, J. M., Zhang, P., Hide, G., Yamamoto, M., et al. (2021). High resistance to Toxoplasma gondii infection in inducible nitric oxide synthase knockout rats. iScience 24 (11), 103280. doi: 10.1016/j.isci.2021.103280
Wei, Y., Hong, Q., Chen, D., Liang, C., Liu, H., Luo, X., et al. (2014). Permissibility of Mongolian gerbil for Angiostrongylus cantonensis infection and utility of this animal model for anthelmintic studies. Parasitol. Res. 113 (5), 1687–1693. doi: 10.1007/s00436-014-3813-0
Wei, X. Q., Niedbala, W., Xu, D., Luo, Z. X., Pollock, K. G., Brewer, J. M. (2004). Host genetic background determines whether IL-18 deficiency results in increased susceptibility or resistance to murine Leishmania major infection. Immunol. Lett. 94 (1-2), 35–37. doi: 10.1016/j.imlet.2004.04.001
Weingartner, M., Stucheli, S., Jebbawi, F., Gottstein, B., Beldi, G., Lundstrom-Stadelmann, B., et al. (2022). Albendazole reduces hepatic inflammation and endoplasmic reticulum-stress in a mouse model of chronic Echinococcus multilocularis infection. PLoS Negl. Trop. Dis. 16 (1), e0009192. doi: 10.1371/journal.pntd.0009192
Winfield, J. B (1997). Primary immunodeficiency diseases. Report of a WHO scientific group. Clin. Exp. Immunol. 109 Suppl 1, 1–28. doi: 10.1046/j.1365-2249.1997.00439.x
Wiszniewsky, A., Layland, L. E., Arndts, K., Wadephul, L. M., Tamadaho, R. S. E., Borrero-Wolff, D., et al. (2021). Adoptive transfer of immune cells into RAG2IL-2Rgamma-deficient mice during litomosoides sigmodontis infection: A novel approach to investigate filarial-specific immune responses. Front. Immunol. 12. doi: 10.3389/fimmu.2021.777860
Witola, W. H., Kim, C. Y., Zhang, X. (2017). Inherent oxidative stress in the Lewis rat is associated with resistance to toxoplasmosis. Infect. Immun. 85 (10), e00289-17. doi: 10.1128/IAI.00289-17
Worthington, J. J., Samuelson, L. C., Grencis, R. K., McLaughlin, J. T. (2013). Adaptive immunity alters distinct host feeding pathways during nematode induced inflammation, a novel mechanism in parasite expulsion. PLoS Pathog. 9 (1), e1003122. doi: 10.1371/journal.ppat.1003122
Xiao, L., Fayer, R. (2008). Molecular characterisation of species and genotypes of Cryptosporidium and Giardia and assessment of zoonotic transmission. Int. J. Parasitol. 38 (11), 1239–1255. doi: 10.1016/j.ijpara.2008.03.006
Yadav, M., Dubey, M. L., Gupta, I., Bhatti, G., Malla, N. (2007). Cysteine proteinase 30 in clinical isolates of T. vaginalis from symptomatic and asymptomatic infected women. Exp. Parasitol. 116 (4), 399–406. doi: 10.1016/j.exppara.2007.02.007
Yang, J., Hong, Y., Yuan, C., Fu, Z., Shi, Y., Zhang, M., et al. (2013). Microarray analysis of gene expression profiles of Schistosoma japonicum derived from less-susceptible host water buffalo and susceptible host goat. PLoS One 8 (8), e70367. doi: 10.1371/journal.pone.0070367
Yoon, B. I., Choi, Y. K., Kim, D. Y., Hyun, B. H., Joo, K. H., Rim, H. J., et al. (2001). Infectivity and pathological changes in murine clonorchiasis: comparison in immunocompetent and immunodeficient mice. J. Vet. Med. Sci. 63 (4), 421–425. doi: 10.1292/jvms.63.421
Yoshimura, K., Sato, K., Uchida, K., Oya, H. (1982). The course of Angiostrongylus cantonensis infection in athymic nude and neonatally thymectomized mice. Z Parasitenkd 67 (2), 217–226. doi: 10.1007/BF00928117
Zhang, S. M., Nian, H., Zeng, Y., Dejong, R. J. (2008). Fibrinogen-bearing protein genes in the snail Biomphalaria glabrata: characterization of two novel genes and expression studies during ontogenesis and trematode infection. Dev. Comp. Immunol. 32 (10), 1119–1130. doi: 10.1016/j.dci.2008.03.001
Zhou, H., Chen, Z., Limpanont, Y., Hu, Y., Ma, Y., Huang, P., et al. (2019). Necroptosis and Caspase-2-Mediated Apoptosis of Astrocytes and Neurons, but Not Microglia, of Rat Hippocampus and Parenchyma Caused by Angiostrongylus cantonensis Infection. Front. Microbiol. 10. doi: 10.3389/fmicb.2019.03126
Zhou, H., Zhou, M., Hu, Y., Limpanon, Y., Ma, Y., Huang, P., et al. (2021). TNF-alpha triggers RIP1/FADD/caspase-8-mediated apoptosis of astrocytes and RIP3/MLKL-mediated necroptosis of neurons induced by angiostrongylus cantonensis infection. Cell Mol. Neurobiol. 42 (6), 1841–1857. doi: 10.1007/s10571-021-01063-w
Keywords: parasite, host, adaptation, genetic background, immune response
Citation: Ye C, Zhang L, Tang L, Duan Y, Liu J and Zhou H (2023) Host genetic backgrounds: the key to determining parasite-host adaptation. Front. Cell. Infect. Microbiol. 13:1228206. doi: 10.3389/fcimb.2023.1228206
Received: 24 May 2023; Accepted: 20 July 2023;
Published: 10 August 2023.
Edited by:
Shahid Karim, University of Southern Mississippi, United StatesReviewed by:
Brajesh Kumar Singh, Brown University, United StatesMohammad Kashif, National Institute of Allergy and Infectious Diseases (NIH), United States
Copyright © 2023 Ye, Zhang, Tang, Duan, Liu and Zhou. This is an open-access article distributed under the terms of the Creative Commons Attribution License (CC BY). The use, distribution or reproduction in other forums is permitted, provided the original author(s) and the copyright owner(s) are credited and that the original publication in this journal is cited, in accordance with accepted academic practice. No use, distribution or reproduction is permitted which does not comply with these terms.
*Correspondence: Ji Liu, bGl1amk2QHN5c3VjYy5vcmcuY24=; Hongli Zhou, emhvdWhsaTdAbWFpbDIuc3lzdS5lZHUuY24=
†These authors have contributed equally to this work