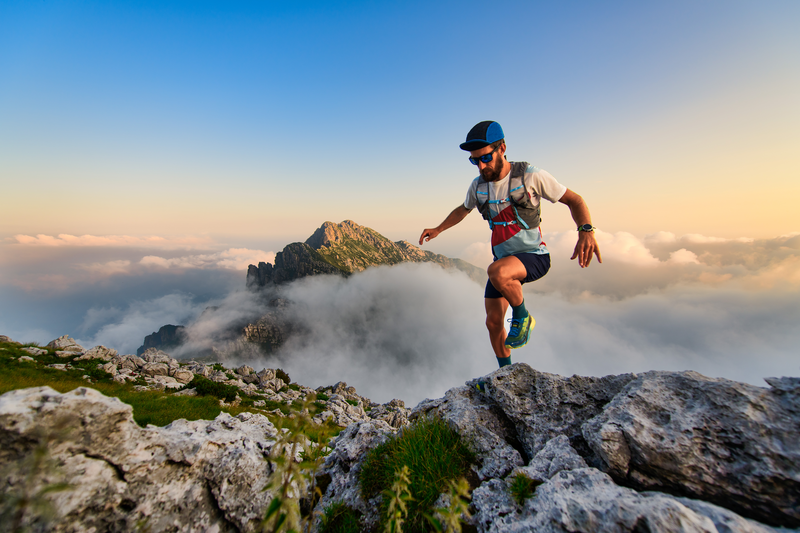
95% of researchers rate our articles as excellent or good
Learn more about the work of our research integrity team to safeguard the quality of each article we publish.
Find out more
ORIGINAL RESEARCH article
Front. Cell. Infect. Microbiol. , 15 August 2023
Sec. Antibiotic Resistance and New Antimicrobial drugs
Volume 13 - 2023 | https://doi.org/10.3389/fcimb.2023.1225341
This article is part of the Research Topic Novel antibiotics or compounds against non-tuberculous mycobacteria View all 5 articles
Objective: To evaluate contezolid (MRX-I) antibacterial activity against Mycobacterium abscessus in vitro and in vivo and to assess whether MRX-I treatment can prolong survival of infected zebrafish.
Methods: MRX-I inhibitory activity against M. abscessus in vitro was assessed by injecting MRX-I into zebrafish infected with green fluorescent protein-labelled M. abscessus. Thereafter, infected zebrafish were treated with azithromycin (AZM), linezolid (LZD) or MRX-I then maximum tolerated concentrations (MTCs) of drugs were determined based on M. abscessus growth inhibition using one-way ANOVA. Linear trend analysis of CFU counts and fluorescence intensities (mean ± SE values) was performed to detect linear relationships between MRX-I, AZM and LZD concentrations and these parameters.
Results: MRX-I anti-M. abscessus minimum inhibitory concentration (MIC) and MTC were 16 μg/mL and 15.6 μg/mL, respectively. MRX-I MTC-treated zebrafish fluorescence intensities were significantly lower than respective LZD group intensities (whole-body: 439040 ± 3647 vs. 509184 ± 23064, p < 0.01); head: 74147 ± 2175 vs. 95996 ± 8054, p < 0.05). As MRX-I concentration was increased from 0.488 μg/mL to 15.6 μg/mL, zebrafish whole-body, head and heart fluorescence intensities decreased. Statistically insignificant differences between the MRX-I MTC group survival rate (78.33%) vs. corresponding rates of the 62.5 μg/mL-treated AZM MTC group (88.33%, p > 0.05) and the 15.6 μg/mL-treated LZD MTC group (76.67%, p > 0.05) were observed.
Conclusion: MRX-I effectively inhibited M. abscessus growth and prolonged zebrafish survival when administered to M. abscessus-infected zebrafish, thus demonstrating that MRX-I holds promise as a clinical treatment for human M. abscessus infections.
Incidence and associated mortality rates of nontuberculous mycobacterial (NTM) lung disease are increasing worldwide (Cowman et al., 2019), thus highlighting the urgent need for improved NTM disease management. NTM is classified into fast-growing and slow-growing mycobacterial groups, with Mycobacterium abscessus the most commonly isolated fast-growing mycobacterial species associated with human NTM infections. According to 2020 NTM treatment guidelines prepared by the American Thoracic Society (ATS), the European Respiratory Society (ERS), the European Society of Clinical Microbiology and Infectious Diseases (ESCMID) and the Infectious Diseases Society of America (IDSA) (Daley et al., 2020). Moreover, the studies (Choi et al., 2012; Nie et al., 2014) have shown that azithromycin(AZM) has more antibacterial effect on Mycobacterium abscess both in vivo and in vitro, and it is not easy to develop resistance to macrolides. a multi-drug regimen containing macrolides is strongly recommended for the treatment of M. abscessus infection-associated lung disease. Nevertheless, the choice of effective therapeutic drugs is limited by the natural resistance of M. abscessus to commonly administered antibiotics (Nessar et al., 2012).
Linezolid (LZD), an oxazolidinone antibiotic that inhibits bacterial protein synthesis by binding to 30S and 50S ribosomal subunit rRNAs (Vinh and Rubinstein, 2009), was recommended as a treatment for M. abscessus-induced lung disease by the American Chest Association in 2007 and the British Chest Association in 2017 (Griffith et al., 2007; Haworth et al., 2017). In fact, LZD works well as a drug-resistant tuberculosis (TB) treatment (Conradie et al., 2020), as well as a treatment for improving rates of conversion of sputum M. abscessus-positive cultures to negative culture status for patients with pulmonary M. abscessus infection (Li et al., 2019). However, despite its efficacy, LZD use has been clinically limited, since the drug can trigger severe bone marrow suppression as a serious safety issue (Hashemian et al., 2018). Therefore, safer drugs are needed for TB and NTM disease treatment. Contezolid (MRX-I) is a novel oxazolidinone antibiotic with the chemical name (S)-5-[(isoxazole-3-arylamino)methyl]-3-[2,3,5-trifluoro-4-[4-oxo-3,4-dihydropyridine-1(2H)-yl]phenyl]oxazolidine-2-one (Gordeev and Yuan, 2014) that provides the same anti-M. tuberculosis effect as LZD (Shoen et al., 2018). Nonetheless, despite the fact that MRX-I treatment is associated with markedly reduced rates of bone marrow suppression and monoamine oxidase inhibition (MAOI) rates than those reported for LZD (Cowman et al., 2016), few studies have investigated M. abscessus susceptibility and resistance to MRX-I, prompting this study. Here, antibacterial effects of MRX-I treatment were assessed both in vitro and in vivo using the M. abscessus standard strain. Furthermore, the efficacy of the drug for prolonging survival was also assessed using M. abscessus-infected zebrafish as an in vivo bacterial infection model.
AZM and LZD are both purchased by Beijing Solarbio Science & Technology Co., Ltd.(Beijing, China), and MRX-I is provided by Shanghai Micurx Pharmaceutical Co., Ltd. (Shanghai, China). Both drugs were dissolved in dimethyl sulfoxide (DMSO), and the drug solution was prepared according to the suggestions provided by the Institute of Clinical and Laboratory Standards (CLSI) (Woods et al., 2011). The standard strain of M. abscessus ATCC 19977 was cultured on solid Lowenstein-Jensen medium at 37°C for 4-6 days. The MICs of AZM, LZD and MRX-I were determined by adding drugs to M. abscessus cultured in 96-well plates according to the recommended CLSI broth microdilution method. The broth was diluted twice, and both the concentrations of AZM, LZD and MRX-I ranged from 0.5-256μg/mL. A bacterial inoculum with turbidity equivalent to 0.5 McFarland standard dilution of 1∶200 was prepared for each strain. The MIC of M. abscessus was determined after 3 days of culture at 37°C together with antibiotics. Thereafter, 70 μl of Alamar Blue solution (Sirotec, 20μL Alamar Blue+50μL 5% Tween 80) was added to each well, and then the plates were incubated for another 24 hours. The color change from blue to pink indicates bacterial growth (Cowman et al., 2016). MIC is defined as the lowest drug concentration that no color change, that is, the lowest concentration that can inhibit the visible growth of the test isolate. Explain the results of drug sensitivity test (DST) according to the breakpoint recommended by CLSI.
This study was approved by the Ethics Committee of Beijing Chest Hospital, a hospital affiliated with Capital Medical University (2021-020). The wild-type zebrafish AB strain was maintained through natural paired mating to generate zebrafish progeny that were raised in water at 28 °C. Meanwhile, the smooth (S) morphology M. abscessus standard strain (ATCC19977) was incubated for 5 to 7 days at 30 °C in Middlebrook 7H9 broth (Becton Dickinson) containing 10% OADC (Becton Dickinson) and 0.05% Tween 80 (Sigma-Aldrich). Mid-log-phase M. abscessus cultures were centrifuged then the pellets were washed and cells were resuspended in phosphate buffered saline (PBS) containing 0.05% Tween 80. Next, the bacterial suspension was homogenised and sonicated then the tube containing dispersed cells was left upright for 5 to 10 min while bacterial cells settled to the bottom of the tube. The bacteria were then collected and resuspended in a smaller volume of PBS and labelled with the green fluorescent dye DIO (Thermo Fisher Scientific, USA or Hill Technology Co., Ltd., China). Labelled bacteria were next introduced into wild-type zebrafish at 2 days post-fertilisation (dpf) by micro-intravenous injection of about 3.6 × 103 colony-forming units (CFUs) into the tail of each zebrafish in order to establish a zebrafish M. abscessus infection model. Zebrafish were anesthetized using 3-aminobenzoic acid ethyl ester methanesulfonate (C9H11NO2·CH4O3S, MESAB). The MESAB was prepared by mixing MESAB and Na2HPO4·12H2O in a total mass ratio of 1:5 to make a 4 mg/mL solution, which was stored at 4°C. For use, it was diluted with standard dilution water, with a final anesthetic concentration of 0.16 mg/mL. Then the zebrafish were injected bacteria by intravenous microinjection with each fish receiving approximately 3.6×10³colony forming units (CFUs)of the transplant to establish the zebrafish model of M. abscessus infection (Guo et al., 2020; Nie et al., 2020).
Zebrafish collected at 3 dpf under the microscope were randomly allocated to wells of 6-well plates (30 zebrafish per well). Meanwhile, azithromycin (AZM), LZD and MRX-I were prepared in dimethylsulfoxide (DMSO, Shanghai Aladdin Biochemical Technology Co., Ltd.) as initial 20.0 mg/mL stock solutions that were stored at -20 °C. For MTC determinations, drugs were diluted in water then were added to wells containing zebrafish to generate dilutions in wells of 6-well plates at final concentrations for AZM (62.5 μg/mL, 125 μg/mL, 250 μg/mL, 500 μg/mL, 1000 μg/mL), for LZD (15.6 μg/mL, 31.2 μg/mL, 62.5 μg/mL, 125 μg/mL, 250 μg/mL) and for MRX-I (15.6 μg/mL, 31.2 μg/mL, 62.5 μg/mL, 125 μg/mL, 250 μg/mL). The blank control group (zebrafish without injected M. abscessus and without drug dosing) and the model group (the negative control group of zebrafish injected with M. abscessus without drug dosing) were prepared at the same time using the same methods used to prepare the abovementioned drug-treated samples. After plates were incubated for 48 h at 35 °C, MTCs were determined as based on the highest concentration of each drug that did not cause zebrafish death.
According to previously reported experimental procedures, 3 dpf zebrafish were selected under the microscope then were randomly assigned to 6-well plates (30 zebrafish/well). Initial AZM, LZD and MRX-I stock solutions were diluted in water to generate working solutions of AZM (62.5 μg/mL), of LZD (15.6 μg/mL) and of MRX-I (0.488 μg/mL, 0.977 μg/mL, 1.95 μg/mL, 3.91 μg/mL, 7.81 μg/mL, 15.6 μg/mL). The control group and the model group were concurrently set up. All control and experimental samples were prepared in the same final volume of 3 mL/well. After treatment of zebrafish at 35 °C for 48 h, 10 zebrafish were randomly selected from each experimental group to photograph. The zebrafish were anesthetized and transferred onto methyl cellulose using a Pasteur pipette. An electrically controlled, continuously variable magnification fluorescence microscope (AZ100, Nikon, Japan) equipped with a green fluorescence channel was used at a magnification of 30x. Image processing software (NIS-Elements D 3.20) was used to analyse and collect fluorescence-based data. The efficacy of each drug, as based on inhibition of M. abscessus growth in zebrafish, was evaluated as based on fluorescence intensity of zebrafish whole-body, head and heart tissues.
3 dpf zebrafish were selected under the microscope and randomly assigned to 50-mL beakers (cups) with 60 zebrafish (experimental group) in a 20-mL volume per cup. Initial AZM, LZD and MRX-I stock solutions were diluted to generate a 62.5-μg/mL AZM MTC solution, a 15.6-μg/mL LZD MTC solution and MRX-I solutions at concentrations of 0.488 μg/mL, 0.977 μg/mL, 1.95 μg/mL, 3.91 μg/mL, 7.81 μg/mL and 15.6 μg/mL (MTC). Concurrently, control group and model group samples were set up in 20-mL volumes in cups then all control and experimental group cups were incubated at 35 °C. Numbers of zebrafish deaths for all groups were recorded every day and dead zebrafish were removed daily. At experiment completion, data were statistically analysed to calculate survival rates of zebrafish in each experimental and control group.
SPSS 26.0 was used to statistically analyse all data obtained in this study. One-way ANOVA was used for intergroup comparisons of CFU counts and fluorescence intensity expressed as mean ± SE values. For comparisons between two groups, t or t’ tests were used to compare normally distributed data from independent samples and non-parametric tests were used to compare data with non-normal distributions. One-way ANOVA analysis was chosen to compare normally distributed data with equal variance among multiple groups; otherwise non-parametric tests were used. Linearity between MRX-I concentration and fluorescence intensity was assessed using a linear trend analysis method. Kaplan-Meier survival analysis was performed using the log-rank test to visualise survival rates of zebrafish treated with different MRX-I concentrations. Intergroup differences with p values of <0.05 were considered statistically significant.
MICs of AZM, LZD and MRX-I were determined according to their effects on growth of the M. abscessus standard strain in zebrafish. The MIC of MRX-I for inhibition of growth of the M. abscessus standard strain was 16 μg/mL, and the MICs of AZM and LZD were 0.5µg/mL and 8μg/mL, respectively.
Table 1 presents results related to efficacies of AZM, LZD and MRX-I against M. abscessus infection. The AZM MTC was 62.5 µg/mL and MTCs of LZD and MRX-I were both 15.6 µg/mL. Treatment of zebrafish with AZM at 2 times the MTC led to deterioration of zebrafish health; treatment with 8 times the MTC led to signs of cardiac congestion accompanied by body bending; treatment with 16 times the MTC led to zebrafish mortality approaching the rate of 33.33%. At the LZD MTC of 15.6 μg/mL, no notable zebrafish health differences were observed as compared to that of the model group, while LZD treatment of zebrafish at 2 times the MTC resulted in a dramatic increase in mortality rate to 23.33%. Similarly, treatment with MRX-I led to similar trends as those observed for LZD; at the MRX-I MTC of 15.6 μg/mL, no significant changes were observed in zebrafish phenotype as compared with that of the model group, while treatment with 2 times the MRX-I MTC led to a marked increase in mortality rate.
Fluorescence intensities of zebrafish whole-body, head and heart tissues of AZM MTC, LZD MTC and MRX-I MTC groups were lower than that of the model group (Table 2). The LZD MTC group whole-body fluorescence intensity exceeded that of the MRX-I MTC group (509184 ± 23064 vs. 439040 ± 3647, p < 0.01). Comparisons of effects of different MRX-I concentrations revealed that the whole-body fluorescence intensity of the MRX-I MTC group was only lower than fluorescence intensities of the 1/32, 1/16 and 1/4 MTC MRX-I groups (439040 ± 3647 vs. 524203 ± 31487, P < 0.01; 439040 ± 3647 vs. 505230 ± 16923, p < 0.01; 439040 ± 3647 vs. 487036 ± 11374, P < 0.01, respectively), with zebrafish whole-body fluorescence intensity decreasing with increasing MRX-I concentration (p = 0.004). Zebrafish head fluorescence intensities were lower in the MRX-I MTC group than in the MRX-I 1/32 MTC group (74147 ± 2175 vs. 105710 ± 10573, P < 0.05), the MRX-I 1/8 MTC group (74147 ± 2175 vs. 93451 ± 5928, P < 0.01) and the MRX-I 1/4 MTC group (74147 ± 2175 vs. 83397 ± 3571, P < 0.05), while the head fluorescence intensity of the LZD MTC group was higher than that of the MRX-I MTC group (95996 ± 8054 vs. 74147 ± 2175, P < 0.05). As for whole-body fluorescence intensity, head fluorescence intensity decreased with increasing MRX-I concentration (p = 0.001). By contrast, the MRX-I MTC group zebrafish heart fluorescence intensity was lower than corresponding intensities of the MRX-I 1/16 MTC and 1/8 MTC groups (10011 ± 852 vs. 20258 ± 4171, P < 0.05; 10011 ± 852 vs. 14733 ± 1663, P < 0.05, respectively), with zebrafish cardiac fluorescence intensity increasing linearly with increasing MRX-I concentration (p = 0.005). Figure 1 shows zebrafish fluorescence intensity distributions for different tissues of zebrafish treated with different concentrations of the three drugs.Increased WX-081 concentration was associated with lower bacterial burdens in both whole-body, head and heart of zebrafish as determined by bacterial CFU (Figure 2).
Table 2 The efficacy of AZM, LZD, and MRX-I against Mycobacterium Abscess infection in zebrafish in vivo.
Figure 1 Fluorescence intensity distribution of different drugs in zebrafish. Green spots are Mycobacterium abscessus. The red dotted box represents the head analysis area. The yellow dotted box represents the heart analysis area. (A) Control; (B) Model; (C) 62.5μg/mL AZM; (D) 15.6μg/mL LZD; (E) 0.488μg/mL- MRX-I; (F) 0.977μg/mL-MRX-I; (G) 1.95μg/mL-MRX-I; (H) 3.91 μg/mL- MRX-I; (I) 7.81μg/mL- MRX-I; (J) 15.6 μg/mL-MRX-I.
Figure 2 Therapeutic effect of different concentrations of WX-081 on M. abscessus infection in zebrafish whole-body, head and heart. With the increase of MRX-I concentration, the level of CFU in zebrafish gradually decreased, and the linear trend of each group was P < 0.05.
The survival rate of zebrafish in the MRX-I MTC group was 78.33%, a rate that was not significantly different from survival rates of MRX-I 1/16, 1/8, 1/4 and 1/2 MTC groups (Table 3). In addition, the survival rate of the MRX-I MTC group was not significantly different from that of the AZM MTC group that was treated with 62.5 μg/mL (78.33% vs. 88.33%, p > 0.05) and that of the LZD MTC group treated with 15.6 μg/mL (78.88% vs. 76.67%, p > 0.05). As compared with the model group survival rate, no significant difference in LZD and MRX-I MTC (15.6 μg/mL) group survival rates were observed (66.67% vs. 76.67%, p > 0.05; 66.67% vs. 78.33%, p > 0.05, respectively), although MRX-I 1/32, 1/16 and 1/8 MTC group survival rates exceeded that of the model group (95.00% vs. 66.67%, p < 0.001; 90.00% vs. 66.67%, p < 0.01; 85.00% vs. 66.67%, p < 0.05, respectively). Figure 3 shows zebrafish survival curves for different concentrations of AZM, LZD and MRX-I.
Zebrafish, a small (<4-cm-long) freshwater fish, is used as an animal model, due to similarities of its innate and acquired immune systems with those of mammals that render it more advantageous for researching human diseases than invertebrate models (e.g., Drosophila melanogaster, nematodes (van der Sar et al., 2004)). In recent years, an increasing number of studies (Bernut et al., 2014; Dupont et al., 2017; Lefebvre et al., 2017; Winters et al., 2022) have employed the M. abscessus-infected zebrafish model to assess antibacterial activities of drugs in vivo.
Macrolide drugs are one of the reliable drugs to treat M. abscessus infection, but it is also easy for M. abscessu to produce macrolide drug-acquired mutations (Richard et al., 2020; Griffith and Daley, 2022). LZD is the first oxazolidinone used in humans, and it has anti-mycobacterium tuberculosis activity in vitro and in vivo (Shoen et al., 2018), meanwhile,it is also an effective drug for treating M. abscessus infection (Zhang et al., 2018), however, due to drug adverse reactions, its clinical practice is limited (Shoen et al., 2018). Here we explored the antibacterial activity of MRX-I, a new oxazolidinone antibiotic, against M. abscessus in vitro and in vivo. Previously, the in vitro activity of MRX-I against M. abscessus was studied by Guo et al., who demonstrated that in vitro MRX-I MICs within the range of 0.25 to 64 mg/L could serve as indicators of antibacterial activity against M. abscessus. However, no in vivo studies conducted using animal models infected with M. abscessus have been reported, prompting this study. Here we report the first in vivo investigation of the antibacterial activity of MRX-I, as assessed based on the drug’s effect in prolonging survival of M. abscessus-infected zebrafish. Our results revealed that the MRX-I MIC based on inhibition of M. abscessus growth in zebrafish was 16 μg/mL and the MRX-I MTC was 15.6 μg/mL. In addition, based on the fact that the main pathogenic subspecies isolated from human pathogenic M. abscessus strains are S-type/Smooth-type (Nie et al., 2014), as the concentration of MTX concentration was increased from 0.488 μg/mL to the MTC, whole-body, head and heart S-type M. abscessus fluorescence intensities gradually decreased. MRX-I and LZD showed similar anti-mycobacterium tuberculosis ability in mouse model (Shoen et al., 2018). However, in zebrafish model, when both MRX-I and LZD were in MTC concentration, the whole body fluorescence intensity of MRX-I group was lower than that of LZD group (439040 ± 3647 vs. 509184 ± 23064, p < 0.01), indicating that the anti-mycobacterium abscess activity of MRX-I might be better than LZD.
Notably, the survival rate of 4-9 dpf zebrafish treated with the MRX-I MTC was 78%, a rate that was lower than survival rates of the 1/32 MTC group and not statistically significantly different from survival rates of groups treated with other MRX-I concentrations. Moreover, fluorescence intensities of zebrafish whole-body, head and heart tissues were found to increase in a linear manner with increasing MRX-I concentration within the range of 0.488 μg/mL to 15.6 μg/mL. Therefore, a lower zebrafish fluorescence intensity reflected a stronger inhibitory effect on M. abscessus growth. Furthermore, the survival of 4-9 dpf zebrafish decreased from 95.00% to 78.33% as the concentration of MRX-I was increased from 0.488 μg/mL to the MTC. Taken together, the abovementioned results obtained using the M. abscessus-infected zebrafish model demonstrated that MRX-I may inhibite in vivo growth of M. abscessus.
Importantly, LZD can penetrate the blood-brain barrier and thus has good activity against intracranial infections. In order to verify whether MRX-I also has good intracranial antibacterial activity, head bacterial counts were specifically studied here. Our results revealed that in vivo bacterial counts in zebrafish heads decreased as MRX concentration was increased. Moreover, we investigated MRX-I effects on head fluorescence intensity of M. abscessus within zebrafish heads, whereby analysis of head fluorescence results revealed a lower head fluorescence intensity of the MRX-I MTC group as compared to those of the model group (p < 0.001) and groups treated with MRX-I at 1/8 (p < 0.01) and 1/4 (p < 0.05) of the MTC. Furthermore, as the drug concentration was gradually increased, head fluorescence intensity gradually decreased and the head bacterial count decreased linearly with increasing drug concentration, thus suggesting that MRX-I may reduce the bacterial load in zebrafish. Similarly, results of a previous study had suggested that LZD freely entered the cerebrospinal fluid of pulmonary TB patients with intact blood-brain barrier function (Nau et al., 2010). A case report of MRX-I in the treatment of tuberculous meningitis (Guo et al., 2023) shows that MRX-I can penetrate into cerebrospinal fluid well, as similar results of the current study indicating that MRX-I can act on M. abscessus in zebrafish head tissues. Nonetheless, clinical studies are needed to determine whether MRX-I can serve as an effective treatment for central nervous system TB cases.
This study had several limitations. First, here we used a zebrafish model of bacterial infection to assess MRX-I bacteriostasis against a single standard M. abscessus strain without studying the drug’s effects on other M. abscessus complex strains, including M. abscessus subspecies massiliense and bolletii. Second, the number of clinical isolates of M. abscessus analysed in this study was limited and thus should expanded in future studies to provide more reliable real-world data to help clinicians choose the most effective and safe drug regimens for patient treatment.
In conclusion, MRX-I (contelozid) exerts an inhibitory effect on M. abscessus growth and tends to prolong survival time of M. abscessus-infected zebrafish. Thus, results presented here should provide significantly relevant information to guide the clinical application of contezolid as a treatment for M. abscessus infection.
The original contributions presented in the study are included in the article/supplementary material. Further inquiries can be directed to the corresponding author.
The animal study was reviewed and approved by the Ethics Committee of Beijing Chest Hospital, Capital Medical University.
SG and WN contributed to the experimental operation and article writing. LL, LS, YY, RG contributed to the experimental operation. NC contributed to the revision of the article. All authors contributed to the article and approved the submitted version.
This study was funded by National Natural Science Foundation of China [8210002], Beijing Health Technologies Promotion Program [BHTPP2022083] and Training Fund for Open Projects at Clinical Institutes and Departments of Capital Medical University [CCMU2022ZKYXZ003].
We thank the sample bank of Beijing Chest Hospital Capital Medical University / Beijing Tuberculosis and Thoracic Tumor Research Institute for providing isolates, Shanghai MicuRx Co., Ltd. for providing Contezolid, and Nanjing Hunter Biotech Laboratory's Xu Yiqiao and Cao Huizhuo for providing Zebrafish infected animal models.
The authors declare that the research was conducted in the absence of any commercial or financial relationships that could be construed as a potential conflict of interest.
All claims expressed in this article are solely those of the authors and do not necessarily represent those of their affiliated organizations, or those of the publisher, the editors and the reviewers. Any product that may be evaluated in this article, or claim that may be made by its manufacturer, is not guaranteed or endorsed by the publisher.
Bernut, A., Le Moigne, V., Lesne, T., Lutfalla, G., Herrmann, J. L., Kremer, L. (2014). In vivo assessment of drug efficacy against mycobacterium abscessus using the embryonic zebrafish test system. Antimicrob. Agents Chemother. 58 (7), 4054–4063. doi: 10.1128/AAC.00142-14
Choi, G. E., Shin, S. J., Won, C. J., Min, K. N., Oh, T., Hahn, M. Y., et al. (2012). Macrolide treatment for Mycobacterium abscessus and Mycobacterium massiliense infection and inducible resistance. Am. J. Respir. Crit. Care Med. 186 (9), 917–925. doi: 10.1164/rccm.201111-2005OC
Conradie, F., Diacon, A. H., Ngubane, N., Howell, P., Everitt, D., Crook, A. M., et al. (2020). Treatment of highly drug-resistant pulmonary tuberculosis. N. Engl. J. Med. 382 (10), 893–902. doi: 10.1056/NEJMoa1901814
Cowman, S., Burns, K., Benson, S., Wilson, R., Loebinger, M. R. (2016). The antimicrobial susceptibility of non-tuberculous mycobacteria. J. Infect. 72 (3), 324–331. doi: 10.1016/j.jinf.2015.12.007
Cowman, S., van Ingen, J., Griffith, D. E., Loebinger, M. R.. (2019). Non-tuberculous mycobacterial pulmonary disease. Eur. Respir. J. 54 (1), 1900250. doi: 10.1183/13993003.00250-2019
Daley, C. L., Iaccarino, J. M., Lange, C., Cambau, E., Wallace, R. J., Andrejak, C., et al. (2020). Treatment of nontuberculous mycobacterial pulmonary disease: an official ATS/ERS/ESCMID/IDSA clinical practice guideline. Clin. Infect. Dis. 71 (4), 905–913. doi: 10.1093/cid/ciaa1125
Dupont, C., Viljoen, A., Thomas, S., Roquet-Banères, F., Herrmann, J. L., Pethe, K., et al. (2017). Bedaquiline inhibits the ATP synthase in Mycobacterium abscessus and is effective in infected zebrafish. Antimicrob. Agents Chemother. 61 (11), e01225–17. doi: 10.1128/AAC.01225-17
Gordeev, M. F., Yuan, Z. Y. Y. (2014). New potent antibacterial oxazolidinone (MRX-I) with an improved class safety profile. J. Med. Chem. 57 (11), 4487–4497. doi: 10.1021/jm401931e
Griffith, D. E., Aksamit, T., Brown-Elliott, B. A., Catanzaro, A., Daley, C., Gordin, F., et al. (2007). An official ATS/IDSA statement: diagnosis, treatment, and prevention of nontuberculous mycobacterial diseases. Am. J. Respir. Crit. Care Med. 175 (4), 367–416. doi: 10.1164/rccm.200604-571ST
Griffith, D. E., Daley, C. L. (2022). Treatment of Mycobacterium abscessus pulmonary disease. Chest 161 (1), 64–75. doi: 10.1016/j.chest.2021.07.035
Guo, Y., Fan, Y., Pei, X. (2020). Fangjihuangqi Decoction inhibits MDA-MB-231 cell invasion in vitro and decreases tumor growth and metastasis in triple-negative breast cancer xenografts tumor zebrafish model. Cancer Med. 9 (7), 2564–2578. doi: 10.1002/cam4.2894
Guo, W., Hu, M., Xu, N., Shangguan, Y., Xia, J., Hu, W., et al. (2023). The concentration of contezolid in cerebrospinal fluid and serum in a patient with tuberculous meningoencephalitis: A case report. Int. J. Antimicrob. Agents 62 (2), 106875. doi: 10.1016/j.ijantimicag.2023.106875
Hashemian, S. M. R., Farhadi, T., Ganjparvar, M. (2018). Linezolid: a review of its properties, function, and use in critical care. Drug Des. Devel. Ther. 12, 1759–1767. doi: 10.2147/DDDT.S164515
Haworth, C. S., Banks, J., Capstick, T., Fisher, A. J., Gorsuch, T., Laurenson, I. F., et al. (2017). British Thoracic Society guidelines for the management of non-tuberculous mycobacterial pulmonary disease (NTM-PD). Thorax 72 (Suppl 2), ii1–ii64. doi: 10.1136/thoraxjnl-2017-210927
Lefebvre, A. L., Le Moigne, V., Bernut, A., Veckerlé, C., Compain, F., Herrmann, J. L., et al. (2017). Inhibition of the β-lactamase BlaMab by avibactam improves the in vitro and in vivo efficacy of imipenem against mycobacterium abscessus. Antimicrob. Agents Chemother. 61 (4), e02440–16. doi: 10.1128/AAC.02440-16
Li, H., Tong, L., Wang, J., Liang, Q., Zhang, Y., Chu, N., et al. (2019). An intensified regimen containing linezolid could improve treatment response in Mycobacterium abscessus lung disease. BioMed. Res. Int. 2019, 8631563. doi: 10.1155/2019/8631563
Nau, R., Sorgel, F., Eiffert, H. (2010). Penetration of drugs through the blood-cerebrospinal fluid/blood-brain barrier for treatment of central nervous system infections. Clin. Microbiol. Rev. 23 (4), 858–883. doi: 10.1128/CMR.00007-10
Nessar, R., Cambau, E., Reyrat, J. M., Murray, A., Gicquel, B.. (2012). Mycobacterium abscessus: a new antibiotic nightmare. J. Antimicrob. Chemother. 67 (4), 810–818. doi: 10.1093/jac/dkr578
Nie, W., Duan, H., Huang, H., Lu, Y., Bi, D., Chu, N., et al. (2014). Species identification of Mycobacterium abscessus subsp. abscessus and Mycobacterium abscessus subsp. bolletii using rpoB and hsp65, and susceptibility testing to eight antibiotics. Int. J. Infect. Dis. 25, 170–174. doi: 10.1016/j.ijid.2014.02.014
Nie, W. J., Xie, Z. Y., Gao, S., Teng, T. L., Zhou, W. Q., Shang, Y. Y., et al. (2020). Efficacy of Moxifloxacin against Mycobacterium abscessus in Zebrafish Model in vivo. BioMed. Environ. Sci. 33 (5), 350–358. doi: 10.3967/bes2020.047
Richard, M., Gutierrez, A. V., Kremer, L. (2020). Dissecting erm(41)-Mediated Macrolide-Inducible Resistance in Mycobacterium abscessus. Antimicrob. Agents Chemother. 64 (2), e01879–19. doi: 10.1128/AAC.01879-19
Shoen, C., DeStefano, M., Hafkin, B., Cynamon, M.. (2018). In vitro and in vivo activities of contezolid (MRX-I) against mycobacterium tuberculosis. Antimicrob. Agents Chemother. 62 (8), e00493–18. doi: 10.1128/AAC.00493-18
van der Sar, A. M., Appelmelk, B. J., Vandenbroucke-Grauls, C. M., Bitter, W.. (2004). A star with stripes: zebrafish as an infection model. Trends Microbiol. 12 (10), 451–457. doi: 10.1016/j.tim.2004.08.001
Vinh, D. C., Rubinstein, E. (2009). Linezolid: a review of safety and tolerability. J. Infect. 59, S59–S74. doi: 10.1016/S0163-4453(09)60009-8
Winters, C. G., Basnet, R. M., FaasuaMalie, P. E., Shallom, S. J., Zelazny, A. M., Gupta, S., et al. (2022). Disulfiram is effective against drug-resistant mycobacterium abscessus in a zebrafish embryo infection model. Antimicrob. Agents Chemother. 66 (11), e0053922. doi: 10.1128/aac.00539-22
Woods, G. L., Brown-Elliott, B. A., Conville, P. S., Desmond, E. P., Hall, G. S., Lin, G., et al. (2011). Susceptibility testing of Mycobacteria, Nocardiae, and other Aerobic Actinomycetes [Internet]. 2nd ed. Wayne (PA): Clinical and Laboratory Standards Institute; 2011 Mar. Report No.: M24-A2.
Keywords: Mycobacterium abscessus, contezolid, zebrafish, activity, in vivo
Citation: Gao S, Nie W, Liu L, Su L, You Y, Geng R and Chu N (2023) Antibacterial activity of the novel oxazolidinone contezolid (MRX-I) against Mycobacterium abscessus. Front. Cell. Infect. Microbiol. 13:1225341. doi: 10.3389/fcimb.2023.1225341
Received: 19 May 2023; Accepted: 18 July 2023;
Published: 15 August 2023.
Edited by:
Ling Shen, College of Medicine Chicago, United StatesReviewed by:
Jiang Pi, Guangdong Meidcal University, ChinaCopyright © 2023 Gao, Nie, Liu, Su, You, Geng and Chu. This is an open-access article distributed under the terms of the Creative Commons Attribution License (CC BY). The use, distribution or reproduction in other forums is permitted, provided the original author(s) and the copyright owner(s) are credited and that the original publication in this journal is cited, in accordance with accepted academic practice. No use, distribution or reproduction is permitted which does not comply with these terms.
*Correspondence: Naihui Chu, Y2h1bmFpaHVpMTk5NEBzaW5hLmNvbQ==
†These authors have contributed equally to this work
Disclaimer: All claims expressed in this article are solely those of the authors and do not necessarily represent those of their affiliated organizations, or those of the publisher, the editors and the reviewers. Any product that may be evaluated in this article or claim that may be made by its manufacturer is not guaranteed or endorsed by the publisher.
Research integrity at Frontiers
Learn more about the work of our research integrity team to safeguard the quality of each article we publish.