- 1State Key Laboratory of Biobased Material and Green Papermaking, Qilu University of Technology, Shandong Academy of Science, Jinan, China
- 2School of Bioengineering, Qilu University of Technology, Shandong Academy of Science, Jinan, China
- 3Research and Development Department(R&D), Shandong Ande Healthcare Apparatus Co., Ltd., Zibo, China
- 4School of Bioengineering, Shandong Polytechnic, Jinan, China
- 5Henan Province Key Laboratory of Water Pollution Control and Rehabilitation Technology, Henan University of Urban Construction, Pingdingshan, China
Hypertension is an increasingly pressing public health concern across the globe. It can be triggered by a variety of factors such as age and diet, as well as the stress of modern life. The traditional treatment of hypertension includes calcium ion blockers, angiotensin II receptor inhibitors and β-receptor blockers, but these drugs have at least some side effects. Recent studies have revealed that intestinal flora plays a vital role in maintaining and promoting human health. This is due to the type and amount of probiotics present in the flora. Probiotics can reduce hypertension symptoms through four mechanisms: regulating vascular oxidative stress, producing short-chain fatty acids, restoring endothelial cell function, and reducing inflammation. It has been reported that certain functional foods, using probiotics as their raw material, can modify the composition of intestinal flora, thus regulating hypertension symptoms. Consequently, utilizing the probiotic function of probiotics in conjunction with the properties of functional foods to treat hypertension is a novel, side-effect-free treatment method. This study seeks to summarize the various factors that contribute to hypertension, the mechanism of probiotics in mitigating hypertension, and the fermented functional foods with probiotic strains, in order to provide a basis for the development of functional foods which utilize probiotics as their raw material and may have the potential to reduce hypertension.
1 Introduction
With the rapid development of social economy, the daily dietary structure has changed significantly, leading to an increasing prevalence of excess food nutrition. That, as well as the accelerated pace of life and work, has caused the age composition of hypertension patients to tend towards younger (Rison et al., 2022). Poor eating habits, fat accumulation, irregular work and rest, environmental stimulation (Park et al., 2020), and family genetics can all contribute to hypertension (Arnett and Claas, 2018). Hypertension management usually requires long-term treatment with drug combinations to control the disease. However, the long-term use of anti-hypertensive drugs may have a significant side effect on the human body, such as depression caused by the long-term use of β-receptor blockers (Zhang et al., 2022). Furthermore, there are various restrictions on the use of blood pressure medications in patients with diabetes, chronic kidney disease, and pregnancy. For example, angiotensin-converting enzyme inhibitors (ACEs) and angiotensin-receptor inhibitors (ARBs) should be discontinued immediately in pregnant women with hypertension (Wei et al., 2021).
In recent years, the rapid development of sequencing technology has enabled us to further explore and study the role of human microbial communities in our physiological health (Gebrayel et al., 2022). A significant association has been established between the composition of the gut microbiome and cardiovascular diseases, such as hypertension (Lakshmanan et al., 2021). Over the past decade, a significant body of evidence has emerged to support the notion that the gut microbiome can regulate blood pressure. In the past five years, the field has progressed from association to causation, as research has revealed various mechanisms by which the gut microbiome can regulate blood pressure (O’Donnell et al., 2023). These studies have uncovered a variety of mechanisms by which gut microbes regulate hypertension, ranging from “why gut microbes can affect hypertension” to “how gut microbes affect hypertension”. This has further opened up possibilities for new treatments which could potentially prevent hypertension by regulating the gut microbiome.
The Food and Agriculture Organization of the United Nations (FAO) and the World Health Organization (WHO) define probiotics as “live microorganisms that, when consumed in sufficient quantities, have a beneficial effect on the host” (Jäger et al., 2019). In recent years, probiotics have gained public attention for their potential to promote human health. This has led to a rapid expansion of the global probiotics market, resulting in the application of probiotics being utilized not just in supplements, but also as raw materials for functional foods. Probiotics are able to affect the body in multiple ways, such as through the secretion of metabolites which can directly impact other microorganisms and regulate the host defense system (De Vos et al., 2022). Furthermore, they can help to prevent invading pathogens, as well as target digestive functions and chronic inflammation (Cristofori et al., 2021). Additionally, probiotics have been studied for their potential anti-cancer properties (Eslami et al., 2019). More recently, research has focused on the potential of probiotics as functional raw materials for hypertension prevention.
Granato et al. (2020) defines functional foods as industrially processed or natural foods that, when consumed regularly in effective levels as part of a varied diet, may have a positive impact on health in addition to essential nutrients. However, in order for any functional food to be widely used, a large number of randomized, double-blind, controlled clinical trials must first be conducted to prove its safety and functionality. Therefore, no fresh, unprocessed or processed food can be considered a functional food without the proper evidence. Generally, functional foods can be integrated into a daily diet and contain bioactive ingredients which can reduce the risk of multiple diseases. For those with hypertension, consuming functional foods based on probiotics – which have not been found to have any side effects – can help to maintain a normal blood pressure range (Granato et al., 2020). In this study, we provided a theoretical basis for the daily treatment and prevention of hypertension by introducing the functional foods containing probiotics.
2 Hypertension and gut microbes
2.1 Hypertension
Hypertension, characterized by elevated arterial blood pressure and persistent high blood pressure, can lead to stroke, heart disease, kidney disease, and other derived diseases. It can be divided into primary and secondary hypertension (Rivas et al., 2021). Essential hypertension is an elevation in blood pressure that is not caused by any other disease, whereas secondary hypertension is an increase in blood pressure caused by other diseases.
As shown in Figure 1, the coverage of hypertension is extensive, as different groups present with differing symptoms and types. Approximately 20-30% of childhood hypertension is primary, while 65-80% is secondary. Hypertensive syndrome of pregnancy, also known as pregnancy poisoning or pre-eclampsia, is unique to pregnant women and typically appears around 20 weeks gestation or within two weeks postpartum (Ives et al., 2020). Senile systolic hypertension, which refers to systolic blood pressure higher than normal levels and normal diastolic blood pressure in elderly individuals over 60 years old, is an independent condition and a risk factor for senile vascular diseases and stroke (Hu et al., 2013).
2.2 Intestinal microorganisms
The host microbiome is a complex ecosystem consisting of bacteria, eukaryotes, viruses, and archaea which coexist on tissue surfaces and within the body (Figure 2). These microbial communities play important roles in physiological activities such as digestion, metabolism, immune response, compound synthesis, toxin elimination, and even the pathogenesis of certain diseases (Gasaly et al., 2021). Intestinal microorganisms have been found to have a role in the occurrence and maintenance of hypertension. When researchers studied a model of spontaneous hypertension, they noted a series of changes in intestinal physiology, such as changes in intestinal microbes and the amount of tight junction proteins in the intestinal wall (Kim et al., 2018). The imbalance of intestinal microorganisms, combined with the increased inflammation, leads to abnormal sympathetic nerve and intestinal function, which can ultimately lead to the development of hypertension (Guyenet et al., 2020). Li et al. (2021) conducted bacterial genome analysis and fecal microbiome transplantation to find that an abnormal gut microbiota richness was significantly associated with hypertension. The research results of Liu et al. (2018) indicated that the number of Bifidobacterium and Bacteroides decreased, while the number of Eubacillus rectalis increased in the intestinal tract of patients with hypertension, and that the content of intestinal flora was correlated with blood pressure.
Hypertensive individuals and animals have been observed to have reduced bacterial diversity in their gut microbiome, disordered microbial structure and function, and the fermentation end products often altered (Li et al., 2021). This imbalance of intestinal flora and the change of metabolites are key factors in controlling blood pressure. With the development and widespread use of metagenomic sequencing and metabolomics, the relationship between metabolites of intestinal flora and human cardiovascular diseases has been brought to the attention of public. In particular, recent studies on metabolite have found that trimethylamino-N-oxide (TMAO) contributes to the promotion of atherosclerosis through the production of pro-inflammatory cytokines (TNF-α and IL-1B), the reduction of anti-inflammatory cytokines (IL-10), especially the excessive reaction of platelets led to the formation of thrombus (Livshits and Kalinkovich, 2019). Calderón-Pérez et al. (2020) studied gut microbes by targeting microbial metabolites short chain fatty acids (SCFA) and TMAO in plasma and stool. The results of their study showed that there were significant differences in the types and richness of intestinal flora between normal people and hypertensive patients.
Based on the microbiome and microbial metabolites, it is possible to distinguish individuals with hypertension from those without before treatment with drugs. Furthermore, this may be used to initially identify individuals with hypertension symptoms.
2.3 Probiotics and functional foods
Probiotics are live, non-pathogenic microorganisms that, when present in sufficient amounts, may confer health benefits on the host (Salminen et al., 2021). The application of probiotics is commonly achieved in the form of raw materials for functional foods (Rashidinejad et al., 2022). Functional foods are defined as food with specific nutritional and health-promoting functions, meant for certain people with the purpose of regulating body functions (Gul et al., 2016). These include foods for improving body constitution, preventing certain diseases (such as hypertension, diabetes, and coronary heart disease), and restoring health. However, functional foods differ from drugs in that they are not used for treatment, and cannot replace medication for patients. In addition, functional foods must be non-toxic or, at least, exhibit toxicity levels below the threshold set by modern toxicology, meaning that they pose no significant risk of toxic side effects with regular consumption. When compared to current antihypertensive drugs, functional foods can be used to relieve mild symptoms and prevent elevated blood pressure, thus alleviating or counteracting the disease without the risk of developing depression associated with β-receptor blockers (Yeo et al., 2009).
In recent years, probiotics have been proposed as an alternative to antimicrobials and as an adjunct therapy for various chronic diseases (Tsai et al., 2019). Microorganisms directly participate in the metabolic mechanisms of certain diseases, or produce metabolites that affect certain diseases (Hou et al., 2022), such as histone deacetylase inhibitor metabolites that modify cancer susceptibility and lesions. The metabolites produced by probiotics upon entering the body, commonly known as prebiotics, can have direct effects on atherosclerosis (Vourakis et al., 2021), hypertension (Muralitharan et al., 2020), heart failure (Kaye et al., 2020), Type-2 diabetes (Iatcu et al., 2021), and other diseases. Probiotics can directly or indirectly affect host physiology through the production of prebiotics, such as TMAO, SCFA, secondary bile acids and indoxyl sulfate. These metabolites can stimulate a variety of signaling pathways that control physiological processes.
Probiotics have become increasingly popular due to their beneficial properties as functional foods and are now frequently used in everyday life. Besides traditional frozen dairy products, bread and snack products provide new opportunities for the development of probiotics, such as probiotic nuts, probiotic wafer cookies launched in the Chinese market, and energy bars with probiotics added in the Australian market. In the past, probiotics were often fragile and had to be refrigerated, making them difficult to be incorporated into products other than dairy products. However, with the increasing demand, more and more tolerant probiotics have been screened out, such as adapting to extreme pH, high temperature, low temperature, or high pressure. This expands the possibilities for probiotics to be used in frozen foods, hot drinks, and snacks. The growing market of probiotic consumption further indicates people’s newfound understanding of probiotics. Japan, which was the first country to commercially utilize probiotics, has put a lot of emphasis on the probiotic market. Lactic acid drinks containing probiotics have been used in Japan since the 1970s, making it one of the largest consumers of probiotic products in the world. Meanwhile, some enterprises have also emerged in the probiotic market in other countries as listed in Table 1. These enterprises have a long history and rich experience in the production and processing of probiotics.
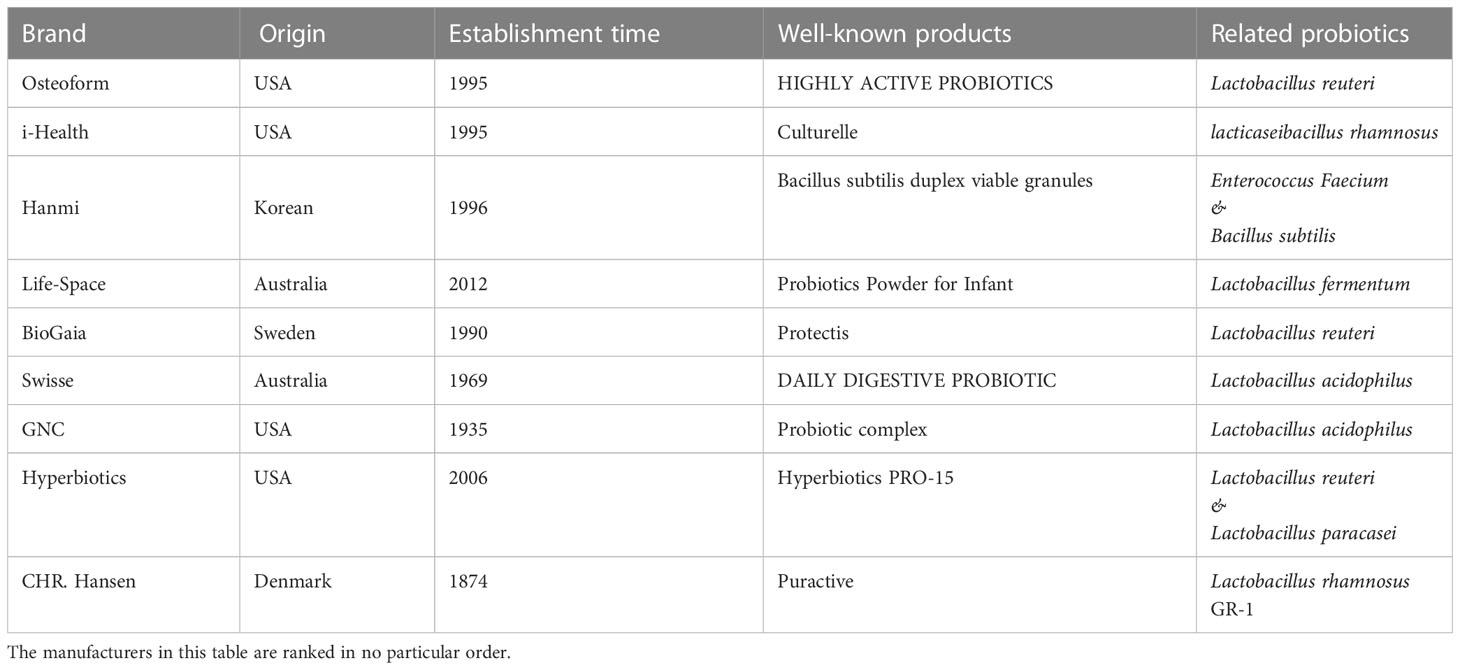
Table 1 The origin and establishment time of the representative probiotic product manufacturers, and their well-known products and the types of probiotics.
3 Causes of hypertension
3.1 Age
The incidence of hypertension was highest in individuals aged over 40 and increased with age (Wang et al., 2020). According to an analysis of Statistics Canada statistics, the incidence of high blood pressure is positively correlated with increasing age (DeGuire et al., 2019). A national survey on hypertension in France in 2015 found that hypertension was prevalent among individuals aged 18 to 74, with the incidence in men being slightly higher than that in women (Vallée et al., 2020). For middle-aged hypertensive patients aged 30 to 50 years, the manifestation was increased diastolic blood pressure, along with an increase in systolic blood pressure. Simple diastolic hypertension was more common in middle-aged men. Weight gain was associated with increased peripheral vascular resistance and normal cardiac output. For elderly hypertension patients aged over 65 years, simple systolic hypertension was the most common type (Briasoulis et al., 2014). Epidemiology has demonstrated that systolic blood pressure increases with age, while diastolic blood pressure gradually decreases after 55 years of age (Jennings et al., 2019).
3.2 Eating habit
People who consume excessive amounts of salt have been found to have an increased incidence of hypertension (He et al., 2020). High-sodium diets have been linked to changes in various proteins involved in calcium stabilization and heart muscle contraction, and excessive sodium intake has been associated with hypertension, stroke, and various cardiovascular diseases. Notably, the salt-sensitive population demonstrates an unusually sensitive renal response to salt intake. This is due to the abnormal overreaction of the sympathetic nervous system, coupled with weakened inhibition of the renin-angiotensin axis, which results in the blocking of nitric oxide (NO) synthesis by endothelial cells, leading to increased vascular resistance and hypertension in the salt-sensitive population. Poor dietary habits are a major cause of hypertension. A study on five dietary patterns for middle-aged and elderly people in the rural areas of Beijing, Northern China, revealed that an irrational dietary pattern characterized by high consumption of refined wheat, meat, poultry and alcoholic beverages was positively correlated with blood pressure (Li et al., 2023).
3.3 Obesity
Obesity is associated with an increased incidence of hypertension. As shown in Figure 3, this can be attributed to several changes in endocrine, inflammatory, and endothelial cell levels (Seravalle and Grassi, 2017; Xiao and Harrison, 2020; Cober et al., 2022; Fernandes-Rosa et al., 2023). Adolescent primary hypertension is largely driven by the increase in visceral fat deposition, which triggers the typical biological maturation and metabolic abnormal syndrome, and ultimately leads to the release of adrenaline (Manosroi and Williams, 2019). The mechanisms underlying obesity-related hypertension include the release of leptin, adipokines, and inflammatory factors, which promote vascular inflammation, damage endothelial function, and contribute to the proliferation, migration and foam cell formation of vascular smooth muscle cells (VSMC), platelet adhesion and aggregation, and atherosclerosis, resulting in elevated blood pressure (Supriya et al., 2018; Recinella et al., 2020).
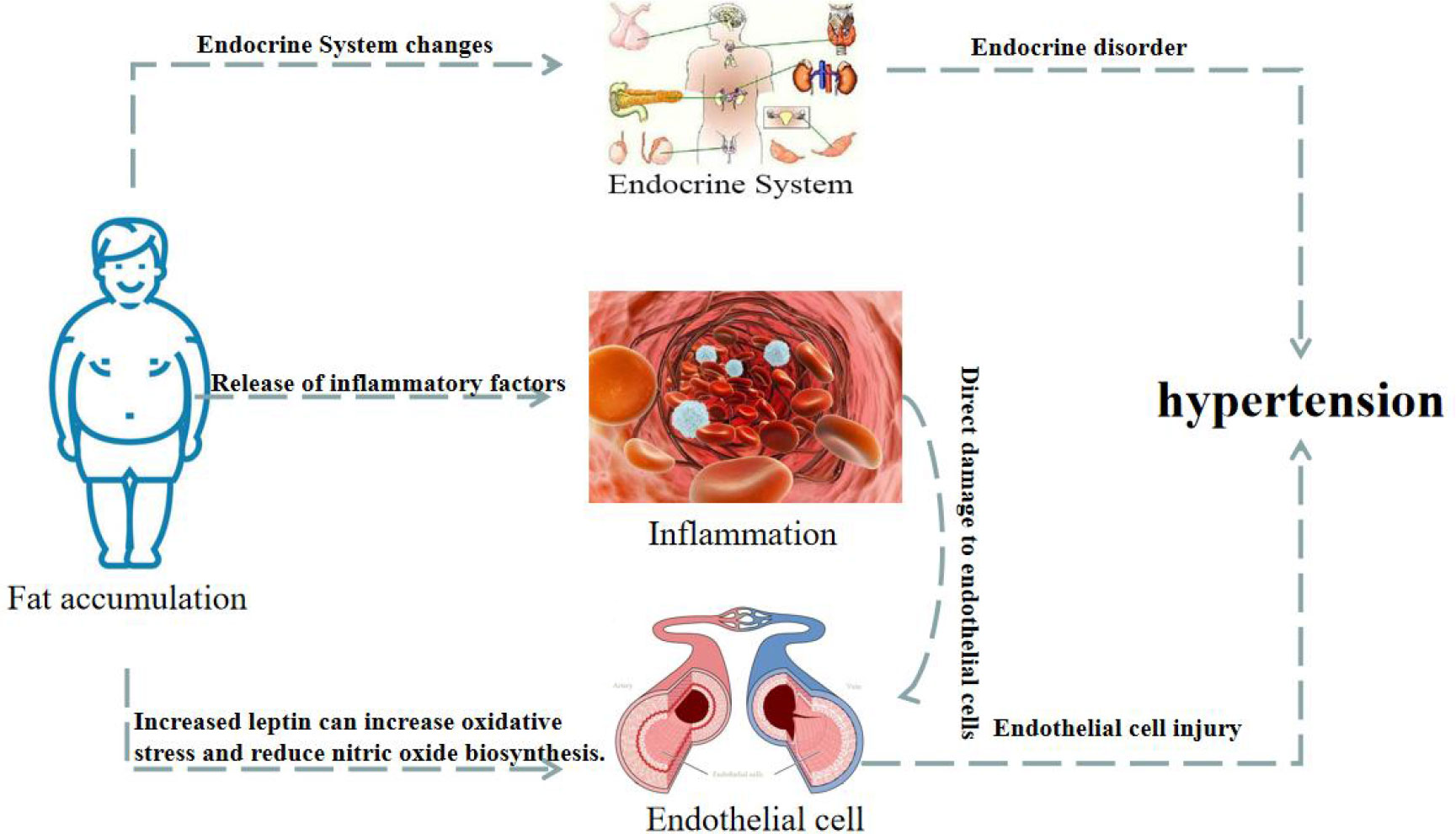
Figure 3 Changes of endocrine, vascular inflammation and endothelial cells in obese patients lead to hypertension.
Hyperleptinemia and hypoadiponectinemia are common among obese hypertensive patients (Perrotta et al., 2019; Gruber et al., 2021). Elevated leptin levels have been found to increase oxidative stress, reduce NO biosynthesis, and promote sodium reabsorption, resulting in increased arterial blood pressure (Bełtowski, 2006). On the other hand, adiponectin has been shown to have a protective effect on the cardiovascular system, increasing insulin sensitivity and inhibiting inflammation (Cohen et al., 2022). The plasma adiponectin levels were also found to be negatively correlated with blood pressure. Furthermore, most of the body is covered with fat outside of the blood vessels. This adipose tissue can release lipid-derived vasodilator factors to act on the potassium channels of the vascular smooth muscle, causing vasodilation. However, this lipid-derived vasodilation response is impaired in hypertension. In one study, adipose tissue and VSMC were co-cultured, and the expression of angiotension II (Ang II) receptor and RhoA and Rho kinases were found to be increased in the VSMC. These signaling molecules are known to play an important role in vascular remodeling and blood pressure regulation (Savoia et al., 2005). Moreover, when the area of the visceral fat was further increased, various metabolic disorders and cardio-renal vascular target organ damage began to emerge successively.
3.4 Heredity
Hypertension is a complex polygenic disorder, with many genes influencing its development (Zhao et al., 2013). If both parents have high blood pressure, their children are at higher risk of developing hypertension (Weijmans et al., 2015). Hypertension may be inherited through two different mechanisms: gene dominant inheritance and polygene association inheritance (Stoll et al., 2018; Teixeira et al., 2018). Advances in molecular biology have offered further insight into the pathogenesis of hypertension in these conditions, elucidating how several risk genes and environmental factors interact with each other and ultimately lead to hypertension (Kawarazaki and Fujita, 2021). In recent years, genome-wide association studies have identified more than 100 single nucleotide polymorphisms associated with blood pressure phenotypes and mutations in multiple single genes that lead to diseases with elevated blood pressure, such as glucocorticoid-inhibiting aldosteronism and Gordon syndrome (Padmanabhan et al., 2017; Mabillard and Sayer, 2019; Zennaro et al., 2020). Mutations in some genes are also associated with increased blood pressure (Matsuo et al., 2004; Dungan et al., 2009; Zhang et al., 2015; Fan et al., 2017). Further research into the genetic and DNA expression of hypertension may provide new pathways for blood pressure regulation and new ideas for hypothesized drug targets for hypertension.
3.5 External environment
Studies have found that people who live in noisy train stations or downtown areas have a higher risk of hypertension compared to those who reside in quiet, rural areas (Lee et al., 2019). This is due to the increase in norepinephrine secretion and increased heart rate caused by noise, as well as the reduction in blood magnesium, a protective agent for heart muscle, leading to a higher incidence of coronary heart disease (Münzel et al., 2017). Experimental evidence has demonstrated that in exposed noise environments, the walls of capillaries are more prone to deformation, which slows down blood flow and can damage both the myocardial and vascular systems. Hence, noise and other living environment factors can also significantly increase the risk of developing hypertension (Münzel et al., 2014; Hahad et al., 2019). High altitude and cold living environments are additional risk factors for hypertension (Shen et al., 2017; Chen and Sun, 2017).
4 Probiotic adjuvant therapy for hypertension
4.1 Routine clinical treatment
Numerous clinical trial data currently support the use of medication or low doses of clothiazone, angiotensin receptor blockers, and patient-specific therapy to reduce blood pressure. However, the efficacy of these new treatment strategies for patients with diabetes, chronic kidney disease, pregnancy, and other conditions remains to be further discussed. For example, in hypertensive women, ACE inhibitors and ARBs may need to be discontinued during pregnancy, and other options such as methyldopa and labetalol may be worth considering (Lu et al., 2018). In addition, many kinds of antihypertensive drugs have been found to have many side effects. The side effects of some common antihypertensive drugs are summarized in Table 2 (Laurent, 2017).
4.2 Probiotics adjuvant therapy and the underlying mechanisms
Gut microbial richness and diversity have been linked to hypertension in both animals and humans, and dysregulation of the microbiome increases the risk of developing the condition (Yang et al., 2015; Li et al., 2017b). Clinical studies suggest that the use of probiotics can lead to moderate to significant reductions in blood pressure (Yuan et al., 2023). For instance, consuming beverages containing Lactobacillus plantarum for a period between 1-2 months has been found to reduce vascular systolic blood pressure, fibrinogen, and interleukin levels in healthy participants. Furthermore, several clinical trials for hypertension have reported that the use of Lactobacillus-fermented milk significantly reduced blood pressure (Aoyagi et al., 2017). In recent years, the mechanism of probiotics in hypertension has been the subject of many studies, and the impact of probiotics on blood pressure levels has been increasingly recognized. The studies related to probiotics in the treatment of hypertension are listed in Table 3. It is clear from these studies that the treatment of hypertension relies not only on a single probiotic, but also on a combination application of probiotics. Moreover, the rich types of functional foods also provide a variety of carriers for the probiotics. As shown in Figure 4, four probiotics are used as adjunctive therapy for hypertension and their mechanisms of action.
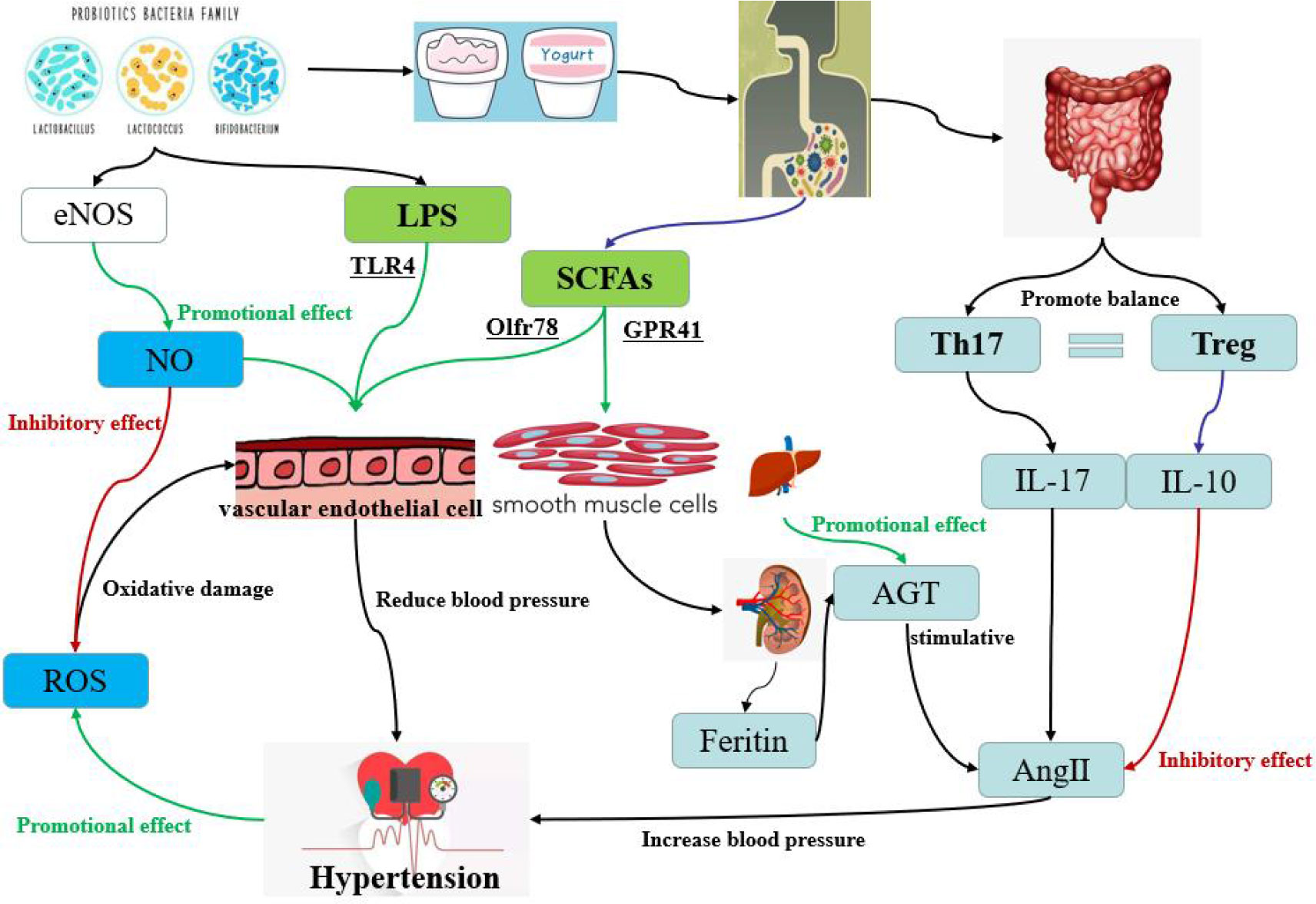
Figure 4 The different pathways of hypertension regulation by probiotics in yogurt, one of the common functional foods. The red pathway is inhibitory and the green pathway is accelerative. The pathways contain oxidative stress pathway, short-chain fatty acid pathway, dndothelial dysfunction pathway and bacterial LPS pathway.
4.2.1 Improvement of vascular oxidative stress
Oxidative stress is a pathological process in which the production of reactive oxygen species (ROS) is excessive or the antioxidant capacity of body is weakened, resulting in the accumulation of ROS and the oxidative damage of cells (Senoner and Dichtl, 2019). Hypertension has been closely linked to oxidative stress (Guzik and Touyz, 2017). When blood vessels are damaged, their systolic and diastolic dysfunction is a direct cause of abnormal blood pressure. Endothelial NO synthase (eNOS) is a key enzyme in the synthesis of NO by the vascular endothelium. The NO produced by eNOS can inhibit the production of ROS and protect the vascular endothelium (Kim et al., 2020). However, eNOS receptors are regulated by various proteins such as fovetin, calmodulin, heat shock protein and Ang II (Rochette et al., 2013). Friques et al. (2015) found that probiotic kefir can maintain blood pressure stability by restoring the balance between ROS and NO, but the treatment time for kefir must last for about 60 days. At the same time, Ang II can also activate the production of ROS (Montezano et al., 2014). Furthermore, in cases of hypertension, the further increase of ROS activity leads to endothelial dysfunction, smooth muscle cell proliferation, lipid oxidation, inflammation, and other occurrences, all of which contribute to vascular damage (Incalza et al., 2018). Wu et al. (2021) revealed for the first time the antioxidant and vascular remodeling-reducing effect of RND3 in hypertensive rats and its molecular mechanism. They found that RND3 regulated superoxide production, vascular smooth muscle cell migration and proliferation, and blood pressure vessel remodeling by inhibiting RICK1-NOX1/2 and mitochondrial superoxide signaling.
For the antihypertensive effect of the probiotic Lactobacillus fermentum CECT5716 (LC40) through the NO system, Robles-Vera et al. (2018) constructed a common model of hypertension (NG-nitro-L-arginine methyl ester added to drinking water). In this model, Bifidobacterium decreased significantly. After the addition of LC40 and NG-nitro-L-arginine methyl ester, Bifidobacterium did not decrease significantly, and Propionibacterium recovered to its normal level.The probiotic kefir mentioned earlier has a regulatory effect on the balance between NO and ROS, while Azizi et al. (2021) also provided a detailed summary of the therapeutic examples of this probiotic to date in his review, including kefir’s special functions of antioxidant and antihypertensive properties.
4.2.2 Production of short chain fatty acids
SCFAs play an integral role in both health maintenance and disease development. SCFAs have been shown to protect the heart and blood vessels from vascular damage (Bartolomaeus et al., 2019). Composed of organic fatty acids with a carbon atomic number between 2 and 6, SCFAs are consisted primarily of acetic acid, propionic acid, butyric acid, isobutyric acid, isovaleric acid and valeric acid. SCFAs not only provide energy as a nutrient, but also have important physiological regulatory roles, such as controlling cell proliferation and differentiation, apoptosis, immune response, nutrient absorption and lipid metabolism. Robles-Vera et al. (2020b) fed LC40 and Bifidobacterium brevis CECT7263 (BMF) directly to mice. Chronic treatment with LC40 or BFM increases the bacteria that produce butyrate and prevents an increase in blood pressure in SHRs. Oral therapy with either butyrate or acetate also prevents an increase in blood pressure and F/B ratio, while restoring the balance of type 17 helper T cells (Th17) and regulatory T cells (Tregs) in the MLN.
The prevalence of hypertension is largely linked to the intake of foods with different fiber content. What we eat every day has a great impact on the structure and metabolism of gut microbes, such as metabolites such as TMAO and SCFAs, which are key factors in gastrointestinal diseases, cancers, and cardiovascular diseases, particularly hypertension (Verhaar et al., 2020). The SCFAs produced by microbial fermentation of dietary fiber and resistant starch in the cecum and colon, can reduce the risk of ecological disorders in patients with hereditary hypertension and prevent the development of hypertension (Robles-Vera et al., 2020a). SCFAs pass the epithelial mucosal barrier in the form of ionization through 1:1 exchange with bicarbonate, hydrogen-coupled monocarboxylate transporter 1 (MCT-1), MCT-2, MCT-4, and sodium-coupled monocarboxylate transporter 1 (SMCT-1). It performs its physiological role through two mechanisms: the first is to acetylate lysine residues by inhibiting histone deacetylases (HDACs) and promote the binding of transcription factors to promoter regions to regulate gene expression (Luu et al., 2020); the second is to perform its biological function by binding to G protein coupled receptors (GPCRs) (Parada Venegas et al., 2019).
Four types of GPCRs have been identified that are activated by SCFAs, including GPR41/FFAR3, GPR43/FFAR2, GPR109A, and Olfr78 (Pluznick et al., 2013; Kimura et al., 2020; Li et al., 2020). These receptors are widely expressed in intestinal epithelial cells, adipocytes, immune system, vascular smooth muscle cells, and vascular endothelial cells. It has been found that SCFAs are mainly involved in the regulation of blood pressure through Olfr78 and GPR41 (Pluznick et al., 2013; Pluznick, 2017). Olfr78 is mainly distributed in the smooth muscle cells of renal entry arterioles and peripheral resistance vessels, and GPR41 is distributed in the endothelial cells of blood vessels. Pluznick et al. (2013) have discovered that Olfr78 is expressed in the kidneys and mediates renin secretion in response to SCFAs, specifically in response to propionate. Specifically, renin can play a promoting role in the generation of Ang II in plasma (Patel et al., 2017). Mice deprived of Olfr78 were particularly sensitive to a large and rapid drop in blood pressure when given propionate, suggesting that the normal function of Olfr78 is to raise blood pressure and counter the hypotensive effects of SCFAs. In contrast, mice lacking the GPR41 coding gene did not respond to propionate hypotension, and this dose resulted in a strong hypotensive response in wild-type mice, suggesting that GPR41 was involved in lowering blood pressure in response to propionate. Natarajan et al. (2016) confirmed that GPR41 can reduce blood pressure by affecting vascular tension. Reducing the gut microbiome of Olfr78 knockout mice by giving them antibiotics led to an increase in their blood pressure, suggesting that propionate produced by the gut microbiome regulates blood pressure through the Olfr78 receptor (Pluznick, 2014). Bartolomaeus et al. (2019) also found that long-term administration of propionate could alleviate endothelial dysfunction and thus inhibit blood pressure fluctuation.
4.2.3 Repair of endothelial cell dysfunction
Vascular endothelial cell (VEC) dysfunction is closely related to hypertension, as it plays an important role in the occurrence and progression of hypertension. Furthermore, hypertension itself can exacerbate VEC dysfunction, resulting in a vicious cycle. VECs are a continuous layer of flat cells that line the interior and exterior walls of the blood vessels, providing a smooth surface for blood flow and maintaining normal blood flow. Recent studies have revealed that VECs also have both endocrine and paracrine functions, secreting various vasoactive substances, such as vasodilator factors and contractile factors, that help maintain vascular wall tension (Oruc et al., 2017).
VECs are metabolically active cells, and hypertension stimulates their secretion of various physiological regulatory factors, the most important of which are the regulation of shear stress and pulsatile blood flow. Systolic blood pressure reflects the shear stress of blood flow on the vascular wall, while pulse pressure reflects both shear stress and the pulsatility of blood flow, both of which contribute to regulating VEC secretion and leading to endothelial dysfunction (Tesauro et al., 2017). Additionally, the ischemia and hypoxia caused by vasospasm and contraction, which are common in hypertension, further damage endothelial function and lead to changes that are characterized by endothelial dependence and weakened diastolic response (Lüscher et al., 1993). Hypertension stimulates VECs to produce and release a series of endothelial factors, causing smooth muscle cell (SMC) proliferation and hypertrophy, intima collagen deposition, and thickening of the vessel walls, thus increasing peripheral vascular resistance and further aggravating hypertension (Schober and Zernecke, 2007).
Rashid et al. (2014) have evaluated the potential of probiotics to resist hypertension in rats. This study ultimately showed that the consumption of probiotic VSL3 solution can prevent the endothelial dysfunction of Mesentery artery in rats, presumably by reducing bacterial migration and local angiotensin system activity, thereby improving oxidative stress and preventing hypertension. Malik et al. (2018)conducted a six-week clinical study using probiotic Lactobacillus plantarum 299v as a supplement and found that this probiotic can improve endothelial dysfunction and also reduce systemic inflammation. Toral et al. (2014) tested the probiotic Lactobacillus coryniformis CECT5711 to reduce inflammation in mice, and found that long-term feeding of probiotics can also improve endothelial dysfunction and vascular oxidative stress.
4.2.4 Reduction of vascular inflammation
Chronic inflammatory response is closely related to the occurrence and development of vascular diseases (Pugh and Dhaun, 2021). And a long-term low-activity micro-inflammatory state of the body is the most prominent sign of dysfunction of vascular endothelial cells (Engin, 2017). Current studies have shown that long-term hypertension in patients can lead to damage of vascular endothelial function, subsequently leading to more serious cardiovascular and cerebrovascular diseases (Sitia et al., 2010). Animal studies demonstrated elevated levels of inflammatory factors in the plasma and walls of blood vessels in hypertensive animals (Murray et al., 2021). Epidemiological and clinical trials have shown that plasma inflammatory factor levels in hypertensive patients are significantly higher than those in people with normal blood pressure (Yi et al., 2022). Atherosclerosis, a type of chronic inflammation of blood vessels, is linked to hypertension, with some studies suggesting that inflammation and hypertension can both influence each other. Inflammation can promote the onset of hypertension by altering the biological activity of NO and reducing endotheloid-dependent vasodilator factors, leading to increased expression of C-reactive protein, tumor necrosis factor, cell adhesion molecules, chemokines, growth factors, endothelin (ET), angiotensin, and other inflammatory factors (Soehnlein and Libby, 2021; Mahdavi-Roshan et al., 2022). Vascular endothelial cells are the direct and primary site of damage in vascular inflammation, and the occurrence of endothelial dysfunction will further lead to the worsening of vascular inflammation. In a review article, Grylls et al. (2021) introduced that the action mechanism of microbiome on host hypertension may be the effect of its component lipopolysaccharide (LPS). Vasodilation, vascular inflammation, and hypertension were improved after probiotics supplementation, with these changes being attributed to LPS activation of endothelial toll-like receptor 4 (TLR4) in probiotics (Lin et al., 2006; Suadoni, 2021). At the same time, a series of signaling pathways associated with endothelial cells, including oxidase- ROS, eNOS-NO, and NADPH oxidase pathways, as well as vascular inflammatory signaling pathways involving mitogen activated protein kinase (MAPK) and nuclear factor kappaB (NF-kB), were also activated simultaneously.
Long-term use of LC40 could treat and prevent intestinal flora disorders, and restore the balance of altered helper Th17 and Tregs in mesenteric lymph nodes (MLN) (Toral et al., 2018). Th17 cells produce interleukin-17 (IL-17), which has powerful pro-inflammatory effects, and the IL-17 content in the plasma of hypertensive patients remains at a high level (Zhang et al., 2019). Moreover, IL-17 is associated with Ang II, which is an important mediator of Ang II-induced hypertension (Basile et al., 2021). In contrast to Th17, Tregs regulate hypertension (Barhoumi et al., 2011). The anti-inflammatory factor IL-10, produced by Tregs, can effectively improve hypertension. Kassan et al. (2011) provided evidence that Tregs played a protective role through IL-10, demonstrating its ability to slightly improve the endothelium-dependent vasodilator response to hypertension. Tinsley et al. (2010) found that daily intraperitoneal injection of recombinant IL-10 restored normal blood pressure in pregnant rats with pregnancy-induced hypertension. Notably, IL-10 is known to have a vascular protection function, which can limit the vascular injury caused by Ang II (Barhoumi et al., 2011).
5 Future outlook
Increasing evidence suggests that probiotics play a critical role in regulating blood pressure, and may become a major force in the prevention and treatment of hypertension. This is because probiotics possess the function which is lack in clinical drugs for hypertension. A growing of researches suggest that regular consumption of probiotics, contained in yogurt, fermented milk and cheese, and in probiotic supplements, can help reduce hypertension and maintain healthy blood pressure levels as part of a healthy lifestyle. The treatment of probiotics is based on the principle of coordination and has certain specificity. Although it does not have the immediate effect of common hypertension drugs, probiotics can treat hypertension for a long time without side effects. Probiotics act beneficially by improving the microbial balance of the gut, promoting diversity, stability, and remodeling of gut microbiota, regulating the body’s inflammatory state, and enhancing the intestinal mucosal barrier function. However, there is no quick fix - research suggests that it takes a minimum of eight weeks of continuous probiotic consumption to reduce blood pressure, indicating that hypertension can be managed through diet, but it requires a long-term commitment. Nevertheless, this does not mean that probiotics can not play a role in preventing hypertension. In conclusion, using probiotics in the long-term prevention and treatment of hypertension provides a new, feasible idea for reducing the incidence of hypertension. Additionally, paying attention to dietary habits and living a healthy lifestyle are also measures to prevent and treat hypertension. The occurrence of hypertension is multi-factorial and multi-angle, but probiotics can regulate various mechanisms of hypertension through the gut microbiota, providing a new measure for hypertension.
Author contributions
ZC: writing—original draft preparation, writing—review and editing, validation. WL: methodology; software. JL: methodology; software. JD: methodology. FG: methodology; software. DZ: methodology. TW: Conceptualization, validation, writing—review and editing, supervision, funding acquisition. ZX: Conceptualization, validation, writing—review and editing, supervision, funding acquisition. All authors contributed to the article and approved the submitted version.
Funding
This work was financially supported by the Key Research and Development Program of Shandong Province (Grant No. 2020CXGC010602), the Science and Technology Support Plan for Young People in Colleges and Universities of Shandong Province (Grant No. 2020KJE005), Key innovation Project of Qilu University of Technology (Shandong Academy of Sciences) (2022JBZ01-06), the Basic Research of Pilot Project for the Integration of Science, Education and Industry, Qilu University of Technology, Shandong Academy of Sciences (Grant No. 2022PY074), and the Open Fund Project of Henan Province Key Laboratory of Water Pollution Control and Rehabilitation Technology (Grant No. CJSP2022004).
Conflict of interest
Author WL is employed by Shandong Ande Healthcare Apparatus Co., Ltd.
The remaining authors declare that the research was conducted in the absence of any commercial or financial relationships that could be construed as a potential conflict of interest.
Publisher’s note
All claims expressed in this article are solely those of the authors and do not necessarily represent those of their affiliated organizations, or those of the publisher, the editors and the reviewers. Any product that may be evaluated in this article, or claim that may be made by its manufacturer, is not guaranteed or endorsed by the publisher.
References
Adams, C., Sawh, F., Green-Johnson, J. M., Jones Taggart, H., Strap, J. L. (2020). Characterization of casein-derived peptide bioactivity: differential effects on angiotensin-converting enzyme inhibition and cytokine and nitric oxide production. J. Dairy Sci. 103, 5805–5815. doi: 10.3168/jds.2019-17976
Ahrén, I. L., Xu, J., Önning, G., Olsson, C., Ahrné, S., Molin, G. (2015). Antihypertensive activity of blueberries fermented by lactobacillus plantarum DSM 15313 and effects on the gut microbiota in healthy rats. Clin. Nutr. 34, 719–726. doi: 10.1016/j.clnu.2014.08.009
Aihara, K., Kajimoto, O., Hirata, H., Takahashi, R., Nakamura, Y. (2005). Effect of powdered fermented milk with lactobacillus helveticus on subjects with high-normal blood pressure or mild hypertension. J. Am. Coll. Nutr. 24, 257–265. doi: 10.1080/07315724.2005.10719473
Amorim, F. G., Coitinho, L. B., Dias, A. T., Friques, A. G. F., Monteiro, B. L., Rezende, L. C. D., et al. (2019). Identification of new bioactive peptides from kefir milk through proteopeptidomics: bioprospection of antihypertensive molecules. Food Chem. 282, 109–119. doi: 10.1016/j.foodchem.2019.01.010
Aoyagi, Y., Park, S., Matsubara, S., Honda, Y., Amamoto, R., Kushiro, A., et al. (2017). Habitual intake of fermented milk products containing lactobacillus casei strain shirota and a reduced risk of hypertension in older people. Benef Microbes 8, 23–29. doi: 10.3920/bm2016.0135
Arnett, D. K., Claas, S. A. (2018). Omics of blood pressure and hypertension. Circ. Res. 122, 1409–1419. doi: 10.1161/circresaha.118.311342
Azizi, N. F., Kumar, M. R., Yeap, S. K., Abdullah, J. O., Khalid, M., Omar, A. R., et al. (2021). Kefir and its biological activities. Foods (Basel Switzerland) 10 (6), 1210. doi: 10.3390/foods10061210
Barhoumi, T., Kasal, D. A., Li, M. W., Shbat, L., Laurant, P., Neves, M. F., et al. (2011). T Regulatory lymphocytes prevent angiotensin II-induced hypertension and vascular injury. Hypertension 57, 469–476. doi: 10.1161/hypertensionaha.110.162941
Bartolomaeus, H., Balogh, A., Yakoub, M., Homann, S., Markó, L., Höges, S., et al. (2019). Short-chain fatty acid propionate protects from hypertensive cardiovascular damage. Circulation 139, 1407–1421. doi: 10.1161/circulationaha.118.036652
Basile, D. P., Abais-Battad, J. M., Mattson, D. L. (2021). Contribution of Th17 cells to tissue injury in hypertension. Curr. Opin. Nephrol. Hypertens. 30, 151–158. doi: 10.1097/mnh.0000000000000680
Bełtowski, J. (2006). Role of leptin in blood pressure regulation and arterial hypertension. J. hypertension 24 (5), 789–801. doi: 10.1097/01.hjh.0000222743.06584.66
Briasoulis, A., Agarwal, V., Tousoulis, D., Stefanadis, C. (2014). Effects of antihypertensive treatment in patients over 65 years of age: a meta-analysis of randomised controlled studies. Heart 100, 317–323. doi: 10.1136/heartjnl-2013-304111
Calderón-Pérez, L., Gosalbes, M. J., Yuste, S., Valls, R. M., Pedret, A., Llauradó, E., et al. (2020). Gut metagenomic and short chain fatty acids signature in hypertension: a cross-sectional study. Sci. Rep. 10, 6436. doi: 10.1038/s41598-020-63475-w
Chen, Y., Liu, W., Xue, J., Yang, J., Chen, X., Shao, Y., et al. (2014). Angiotensin-converting enzyme inhibitory activity of lactobacillus helveticus strains from traditional fermented dairy foods and antihypertensive effect of fermented milk of strain H9. J. Dairy Sci. 97, 6680–6692. doi: 10.3168/jds.2014-7962
Chen, P. G., Sun, Z. (2017). AAV delivery of endothelin-1 shRNA attenuates cold-induced hypertension. Hum. Gene Ther. 28, 190–199. doi: 10.1089/hum.2016.047
Cober, N. D., Vandenbroek, M. M., Ormiston, M. L., Stewart, D. J. (2022). Evolving concepts in endothelial pathobiology of pulmonary arterial hypertension. Hypertension 79, 1580–1590. doi: 10.1161/hypertensionaha.122.18261
Cohen, K. E., Katunaric, B., Senthilkumar, G., Mcintosh, J. J., Freed, J. K. (2022). Vascular endothelial adiponectin signaling across the life span. Am. J. Physiol. Heart Circ. Physiol. 322, H57–h65. doi: 10.1152/ajpheart.00533.2021
Cristofori, F., Dargenio, V. N., Dargenio, C., Miniello, V. L., Barone, M., Francavilla, R. (2021). Anti-inflammatory and immunomodulatory effects of probiotics in gut inflammation: a door to the body. Front. Immunol. 12. doi: 10.3389/fimmu.2021.578386
De Almeida Silva, M., Mowry, F. E., Peaden, S. C., Andrade, T. U., Biancardi, V. C. (2020). Kefir ameliorates hypertension via gut-brain mechanisms in spontaneously hypertensive rats. J. Nutr. Biochem. 77, 108318. doi: 10.1016/j.jnutbio.2019.108318
DeGuire, J., Clarke, J., Rouleau, K., Roy, J., Bushnik, T. (2019). Blood pressure and hypertension. Health Rep. 30 (2), 14–21. doi: 10.25318/82-003-x201900200002
De Vos, W. M., Tilg, H., Van Hul, M., Cani, P. D. (2022). Gut microbiome and health: mechanistic insights. Gut 71, 1020–1032. doi: 10.1136/gutjnl-2021-326789
Dungan, J. R., Conley, Y. P., Langaee, T. Y., Johnson, J. A., Kneipp, S. M., Hess, P. J., et al. (2009). Altered beta-2 adrenergic receptor gene expression in human clinical hypertension. Biol. Res. Nurs. 11, 17–26. doi: 10.1177/1099800409332538
Elkhtab, E., El-Alfy, M., Shenana, M., Mohamed, A., Yousef, A. E. (2017). New potentially antihypertensive peptides liberated in milk during fermentation with selected lactic acid bacteria and kombucha cultures. J. Dairy Sci. 100, 9508–9520. doi: 10.3168/jds.2017-13150
Engin, A. (2017). Endothelial dysfunction in obesity. Adv. Exp. Med. Biol. 960, 345–379. doi: 10.1007/978-3-319-48382-5_15
Eslami, M., Yousefi, B., Kokhaei, P., Hemati, M., Nejad, Z. R., Arabkari, V., et al. (2019). Importance of probiotics in the prevention and treatment of colorectal cancer. J. Cell Physiol. 234, 17127–17143. doi: 10.1002/jcp.28473
Fan, W., Qu, X., Li, J., Wang, X., Bai, Y., Cao, Q., et al. (2017). Associations between polymorphisms of the ADIPOQ gene and hypertension risk: a systematic and meta-analysis. Sci. Rep. 7, 41683. doi: 10.1038/srep41683
Fernandes-Rosa, F. L., Boulkroun, S., Fedlaoui, B., Hureaux, M., Travers-Allard, S., Drossart, T., et al. (2023). New advances in endocrine hypertension: from genes to biomarkers. Kidney Int. 103, 485–500. doi: 10.1016/j.kint.2022.12.021
Friques, A. G., Arpini, C. M., Kalil, I. C., Gava, A. L., Leal, M. A., Porto, M. L., et al. (2015). Chronic administration of the probiotic kefir improves the endothelial function in spontaneously hypertensive rats. J. Trans. Med. 13, 390. doi: 10.1186/s12967-015-0759-7
Gasaly, N., De Vos, P., Hermoso, M. A. (2021). Impact of bacterial metabolites on gut barrier function and host immunity: a focus on bacterial metabolism and its relevance for intestinal inflammation. Front. Immunol. 12. doi: 10.3389/fimmu.2021.658354
Gebrayel, P., Nicco, C., Al Khodor, S., Bilinski, J., Caselli, E., Comelli, E. M., et al. (2022). Microbiota medicine: towards clinical revolution. J. Transl. Med. 20, 111. doi: 10.1186/s12967-022-03296-9
Glazunova, O. A., Moiseenko, K. V., Savinova, O. S., Fedorova, T. V. (2022). In vitro and In vivo antihypertensive effect of milk fermented with different strains of common starter lactic acid bacteria. Nutrients 14(24), 5357. doi: 10.3390/nu14245357
Gonzalez-Gonzalez, C., Gibson, T., Jauregi, P. (2013). Novel probiotic-fermented milk with angiotensin I-converting enzyme inhibitory peptides produced by bifidobacterium bifidum MF 20/5. Int. J. Food Microbiol. 167, 131–137. doi: 10.1016/j.ijfoodmicro.2013.09.002
Granato, D., Barba, F. J., Bursać Kovačević, D., Lorenzo, J. M., Cruz, A. G., Putnik, P. (2020). Functional foods: product development, technological trends, efficacy testing, and safety. Annu. Rev. Food Sci. Technol. 11, 93–118. doi: 10.1146/annurev-food-032519-051708
Gruber, T., Pan, C., Contreras, R. E., Wiedemann, T., Morgan, D. A., Skowronski, A. A., et al. (2021). Obesity-associated hyperleptinemia alters the gliovascular interface of the hypothalamus to promote hypertension. Cell Metab. 33, 1155–1170.e1110. doi: 10.1016/j.cmet.2021.04.007
Grylls, A., Seidler, K., Neil, J. (2021). Link between microbiota and hypertension: focus on LPS/TLR4 pathway in endothelial dysfunction and vascular inflammation, and therapeutic implication of probiotics. BioMed. Pharmacother. 137, 111334. doi: 10.1016/j.biopha.2021.111334
Gul, K., Singh, A. K., Jabeen, R. (2016). Nutraceuticals and functional foods: the foods for the future world. Crit. Rev. Food Sci. Nutr. 56, 2617–2627. doi: 10.1080/10408398.2014.903384
Guyenet, P. G., Stornetta, R. L., Souza, G., Abbott, S. B. G., Brooks, V. L. (2020). Neuronal networks in hypertension: recent advances. Hypertension 76, 300–311. doi: 10.1161/hypertensionaha.120.14521
Guzik, T. J., Touyz, R. M. (2017). Oxidative stress, inflammation, and vascular aging in hypertension. Hypertension 70, 660–667. doi: 10.1161/hypertensionaha.117.07802
Hahad, O., Kröller-Schön, S., Daiber, A., Münzel, T. (2019). The cardiovascular effects of noise. Dtsch Arztebl Int. 116, 245–250. doi: 10.3238/arztebl.2019.0245
He, F. J., Tan, M., Ma, Y., Macgregor, G. A. (2020). Salt reduction to prevent hypertension and cardiovascular disease: JACC state-of-the-Art review. J. Am. Coll. Cardiol. 75, 632–647. doi: 10.1016/j.jacc.2019.11.055
Hou, H., Chen, D., Zhang, K., Zhang, W., Liu, T., Wang, S., et al. (2022). Gut microbiota-derived short-chain fatty acids and colorectal cancer: ready for clinical translation? Cancer Lett. 526, 225–235. doi: 10.1016/j.canlet.2021.11.027
Hu, J., Zhu, F., Xie, J., Cheng, X., Chen, G., Tai, H., et al. (2013). Structural and functional changes of the coronary arteries in elderly senile patients with essential hypertension. Mol. Med. Rep. 8 (5), 1385–1389. doi: 10.3892/mmr.2013.1681
Iatcu, C. O., Steen, A., Covasa, M. (2021). Gut microbiota and complications of type-2 diabetes. Nutrients 14(1), 166. doi: 10.3390/nu14010166
Incalza, M. A., D'Oria, R., Natalicchio, A., Perrini, S., Laviola, L., Giorgino, F. (2018). Oxidative stress and reactive oxygen species in endothelial dysfunction associated with cardiovascular and metabolic diseases. Vasc. Pharmacol. 100, 1–19. doi: 10.1016/j.vph.2017.05.005
Ives, C. W., Sinkey, R., Rajapreyar, I., Tita, A. T. N., Oparil, S. (2020). Preeclampsia-pathophysiology and clinical presentations: JACC state-of-the-Art review. J. Am. Coll. Cardiol. 76, 1690–1702. doi: 10.1016/j.jacc.2020.08.014
Jäger, R., Mohr, A. E., Carpenter, K. C., Kerksick, C. M., Purpura, M., Moussa, A., et al. (2019). International society of sports nutrition position stand: probiotics. J. Int. Soc. Sports Nutr. 16, 62. doi: 10.1186/s12970-019-0329-0
Jennings, A., Berendsen, A. M., De Groot, L., Feskens, E. J. M., Brzozowska, A., Sicinska, E., et al. (2019). Mediterranean-Style diet improves systolic blood pressure and arterial stiffness in older adults. Hypertension 73, 578–586. doi: 10.1161/hypertensionaha.118.12259
Kassan, M., Galan, M., Partyka, M., Trebak, M., Matrougui, K. (2011). Interleukin-10 released by CD4(+)CD25(+) natural regulatory T cells improves microvascular endothelial function through inhibition of NADPH oxidase activity in hypertensive mice. Arterioscler. Thromb. Vasc. Biol. 31, 2534–2542. doi: 10.1161/atvbaha.111.233262
Kawarazaki, W., Fujita, T. (2021). Kidney and epigenetic mechanisms of salt-sensitive hypertension. Nat. Rev. Nephrol. 17, 350–363. doi: 10.1038/s41581-021-00399-2
Kaye, D. M., Shihata, W. A., Jama, H. A., Tsyganov, K., Ziemann, M., Kiriazis, H., et al. (2020). Deficiency of prebiotic fiber and insufficient signaling through gut metabolite-sensing receptors leads to cardiovascular disease. Circulation 141, 1393–1403. doi: 10.1161/circulationaha.119.043081
Kim, S., Goel, R., Kumar, A., Qi, Y., Lobaton, G., Hosaka, K., et al. (2018). Imbalance of gut microbiome and intestinal epithelial barrier dysfunction in patients with high blood pressure. Clin. Sci. (Lond) 132, 701–718. doi: 10.1042/cs20180087
Kim, E. D., Lee, H. S., Kim, K. T., Paik, H. D. (2021). Antioxidant and angiotensin-converting enzyme (ACE) inhibitory activities of yogurt supplemented with Lactiplantibacillus plantarum NK181 and Lactobacillus delbrueckii KU200171 and sensory evaluation. Foods 10(10), 2324. doi: 10.3390/foods10102324
Kim, D. H., Meza, C. A., Clarke, H., Kim, J. S., Hickner, R. C. (2020). Vitamin d and endothelial function. Nutrients 12(2), 575. doi: 10.3390/nu12020575
Kim, J., Yoon, Y. W., Kim, M. S., Lee, M. H., Kim, G. A., Bae, K., et al. (2022). Gamma-aminobutyric acid fermentation in MRS-based medium by the fructophilic Lactiplantibacillus plantarum Y7. Food Sci. Biotechnol. 31, 333–341. doi: 10.1007/s10068-022-01035-w
Kimura, I., Ichimura, A., Ohue-Kitano, R., Igarashi, M. (2020). Free fatty acid receptors in health and disease. Physiol. Rev. 100 (1), 171–210. doi: 10.1152/physrev.00041.2018
Lakshmanan, A. P., Shatat, I. F., Zaidan, S., Jacob, S., Bangarusamy, D. K., Al-Abduljabbar, S., et al. (2021). Bifidobacterium reduction is associated with high blood pressure in children with type 1 diabetes mellitus. BioMed. Pharmacother. 140, 111736. doi: 10.1016/j.biopha.2021.111736
Laurent, S. (2017). Antihypertensive drugs. Pharmacol. Res. 124, 116–125. doi: 10.1016/j.phrs.2017.07.026
Lee, P. J., Park, S. H., Jeong, J. H., Choung, T., Kim, K. Y. (2019). Association between transportation noise and blood pressure in adults living in multi-storey residential buildings. Environ. Int. 132, 105101. doi: 10.1016/j.envint.2019.105101
Li, Y. J., Chen, X., Kwan, T. K., Loh, Y. W., Singer, J., Liu, Y., et al. (2020). Dietary fiber protects against diabetic nephropathy through short-chain fatty acid-mediated activation of G protein-coupled receptors GPR43 and GPR109A. J. Am. Soc. Nephrol. JASN 31 (6), 1267–1281. doi: 10.1681/ASN.2019101029
Li, C., Li, Y., Wang, N., Ge, Z., Shi, Z., Wang, J., et al. (2023). The increased risk of hypertension caused by irrational dietary pattern may be associated with Th17 cell in the middle-aged and elderly rural residents of Beijing city, northern China: a 1:1 matched case-control study. Nutrients 15(2), 290. doi: 10.3390/nu15020290
Li, J., Yang, X., Zhou, X., Cai, J. (2021). The role and mechanism of intestinal flora in blood pressure regulation and hypertension development. Antioxid Redox Signal 34, 811–830. doi: 10.1089/ars.2020.8104
Li, J., Zhao, F., Wang, Y., Chen, J., Tao, J., Tian, G., et al. (2017). Gut microbiota dysbiosis contributes to the development of hypertension. Microbiome 5, 14. doi: 10.1186/s40168-016-0222-x
Lin, F. Y., Chen, Y. H., Tasi, J. S., Chen, J. W., Yang, T. L., Wang, H. J., et al. (2006). Endotoxin induces toll-like receptor 4 expression in vascular smooth muscle cells via NADPH oxidase activation and mitogen-activated protein kinase signaling pathways. Arterioscler. Thromb. Vasc. Biol. 26, 2630–2637. doi: 10.1161/01.ATV.0000247259.01257.b3
Lin, P. P., Hsieh, Y. M., Kuo, W. W., Lin, Y. M., Yeh, Y. L., Lin, C. C., et al. (2013a). Probiotic-fermented purple sweet potato yogurt activates compensatory IGF-IR/PI3K/Akt survival pathways and attenuates cardiac apoptosis in the hearts of spontaneously hypertensive rats. Int. J. Mol. Med. 32, 1319–1328. doi: 10.3892/ijmm.2013.1524
Lin, P. P., Hsieh, Y. M., Kuo, W. W., Lin, Y. M., Yeh, Y. L., Lin, C. C., et al. (2013b). Suppression of TLR-4-related inflammatory pathway and anti-fibrosis effects of probiotic-fermented purple sweet potato yogurt in hearts of spontaneously hypertensive rats. Chin. J. Physiol. 56, 174–183. doi: 10.4077/cjp.2013.Bab118
Liu, J., An, N., Ma, C., Li, X., Zhang, J., Zhu, W., et al. (2018). Correlation analysis of intestinal flora with hypertension. Exp. Ther. Med. 16, 2325–2330. doi: 10.3892/etm.2018.6500
Livshits, G., Kalinkovich, A. (2019). Inflammaging as a common ground for the development and maintenance of sarcopenia, obesity, cardiomyopathy and dysbiosis. Ageing Res. Rev. 56, 100980. doi: 10.1016/j.arr.2019.100980
Lu, Y., Chen, R., Cai, J., Huang, Z., Yuan, H. (2018). The management of hypertension in women planning for pregnancy. Br. Med. Bull. 128, 75–84. doi: 10.1093/bmb/ldy035
Lüscher, T. F., Tanner, F. C., Tschudi, M. R., Noll, G. (1993). Endothelial dysfunction in coronary artery disease. Annu. Rev. Med. 44, 395–418. doi: 10.1146/annurev.me.44.020193.002143
Luu, M., Monning, H., Visekruna, A. (2020). Exploring the molecular mechanisms underlying the protective effects of microbial SCFAs on intestinal tolerance and food allergy. Front. Immunol. 11, 1225. doi: 10.3389/fimmu.2020.01225
Mabillard, H., Sayer, J. A. (2019). The molecular genetics of Gordon syndrome. Genes (Basel) 10(12), 986. doi: 10.3390/genes10120986
Mahdavi-Roshan, M., Salari, A., Kheirkhah, J., Ghorbani, Z. (2022). The effects of probiotics on inflammation, endothelial dysfunction, and atherosclerosis progression: a mechanistic overview. Heart Lung Circ. 31, e45–e71. doi: 10.1016/j.hlc.2021.09.006
Malik, M., Suboc, T. M., Tyagi, S., Salzman, N., Wang, J., Ying, R., et al. (2018). Lactobacillus plantarum 299v supplementation improves vascular endothelial function and reduces inflammatory biomarkers in men with stable coronary artery disease. Circ. Res. 123 (9), 1091–1102. doi: 10.1161/CIRCRESAHA.118.313565
Manosroi, W., Williams, G. H. (2019). Genetics of human primary hypertension: focus on hormonal mechanisms. Endocr. Rev. 40, 825–856. doi: 10.1210/er.2018-00071
Matsuo, A., Katsuya, T., Ishikawa, K., Sugimoto, K., Iwashima, Y., Yamamoto, K., et al. (2004). G2736A polymorphism of thiazide-sensitive Na-cl cotransporter gene predisposes to hypertension in young women. J. Hypertens. 22, 2123–2127. doi: 10.1097/00004872-200411000-00014
Montezano, A. C., Nguyen Dinh Cat, A., Rios, F. J., Touyz, R. M. (2014). Angiotensin II and vascular injury. Curr. hypertension Rep. 16 (6), 431. doi: 10.1007/s11906-014-0431-2
Moreno-Montoro, M., Olalla-Herrera, M., Rufián-Henares, J., Martínez, R. G., Miralles, B., Bergillos, T., et al. (2017). Antioxidant, ACE-inhibitory and antimicrobial activity of fermented goat milk: activity and physicochemical property relationship of the peptide components. Food Funct. 8, 2783–2791. doi: 10.1039/c7fo00666g
Münzel, T., Gori, T., Babisch, W., Basner, M. (2014). Cardiovascular effects of environmental noise exposure. Eur. Heart J. 35 (13), 829–836. doi: 10.1093/eurheartj/ehu030
Münzel, T., Daiber, A., Steven, S., Tran, L. P., Ullmann, E., Kossmann, S., et al. (2017). Effects of noise on vascular function, oxidative stress, and inflammation: mechanistic insight from studies in mice. Eur. Heart J. 38, 2838–2849. doi: 10.1093/eurheartj/ehx081
Muralitharan, R. R., Jama, H. A., Xie, L., Peh, A., Snelson, M., Marques, F. Z. (2020). Microbial peer pressure: the role of the gut microbiota in hypertension and its complications. Hypertension 76, 1674–1687. doi: 10.1161/hypertensionaha.120.14473
Murray, E. C., Nosalski, R., MacRitchie, N., Tomaszewski, M., Maffia, P., Harrison, D. G., et al. (2021). Therapeutic targeting of inflammation in hypertension: from novel mechanisms to translational perspective. Cardiovasc. Res. 117 (13), 2589–2609. doi: 10.1093/cvr/cvab330
Natarajan, N., Hori, D., Flavahan, S., Steppan, J., Flavahan, N. A., Berkowitz, D. E., et al. (2016). Microbial short chain fatty acid metabolites lower blood pressure via endothelial G protein-coupled receptor 41. Physiol. Genomics 48 (11), 826–834. doi: 10.1152/physiolgenomics.00089.2016
O’Donnell, J. A., Zheng, T., Meric, G., Marques, F. Z. (2023). The gut microbiome and hypertension. Nat. Rev. Nephrol. 19 (3), 153–167. doi: 10.1038/s41581-022-00654-0
Obaroakpo, J. U., Liu, L., Zhang, S., Lu, J., Pang, X., Lv, J. (2019). α-glucosidase and ACE dual inhibitory protein hydrolysates and peptide fractions of sprouted quinoa yoghurt beverages inoculated with lactobacillus casei. Food Chem. 299, 124985. doi: 10.1016/j.foodchem.2019.124985
Oruc, C. U., Akpinar, Y. E., Amikishiyev, S., Uzum, A. K., Salmaslioglu, A., Gurdol, F., et al. (2017). Hypovitaminosis d is associated with endothelial dysfunction in patients with metabolic syndrome. Curr. Vasc. Pharmacol. 15, 152–157. doi: 10.2174/1570161114666161003093443
Padmanabhan, S., Aman, A., Dominiczak, A. F. (2017). Genomics of hypertension. Pharmacol. Res. 121, 219–229. doi: 10.1016/j.phrs.2017.04.031
Parada Venegas, D., de la Fuente, M. K., Landskron, G., González, M. J., Quera, R., Dijkstra, G., et al. (2019). Short chain fatty acids (SCFAs)-mediated gut epithelial and immune regulation and its relevance for inflammatory bowel diseases. Front. Immunol. 10. doi: 10.3389/fimmu.2019.00277
Park, S., Kario, K., Chia, Y. C., Turana, Y., Chen, C. H., Buranakitjaroen, P., et al. (2020). The influence of the ambient temperature on blood pressure and how it will affect the epidemiology of hypertension in Asia. J. Clin. Hypertens. (Greenwich) 22, 438–444. doi: 10.1111/jch.13762
Patel, S., Rauf, A., Khan, H., Abu-Izneid, T. (2017). Renin-angiotensin-aldosterone (RAAS): the ubiquitous system for homeostasis and pathologies. Biomedicine pharmacotherapy = Biomedecine pharmacotherapie 94, 317–325. doi: 10.1016/j.biopha.2017.07.091
Pereira, Á. M. S., De Farias, D. R. B., De Queiroz, B. B., Nobre, M. S. C., Cavalcanti, M. T., Salles, H. O., et al. (2019). Influence of a Co-culture of streptococcus thermophilus and lactobacillus casei on the proteolysis and ACE-inhibitory activity of a beverage based on reconstituted goat whey powder. Probiotics Antimicrob. Proteins 11, 273–282. doi: 10.1007/s12602-017-9362-y
Perrotta, F., Nigro, E., Mollica, M., Costigliola, A., D'agnano, V., Daniele, A., et al. (2019). Pulmonary hypertension and obesity: focus on adiponectin. Int. J. Mol. Sci. 20(4), 912. doi: 10.3390/ijms20040912
Pluznick, J. (2014). A novel SCFA receptor, the microbiota, and blood pressure regulation. Gut Microbes 5, 202–207. doi: 10.4161/gmic.27492
Pluznick, J. L. (2017). Microbial short-chain fatty acids and blood pressure regulation. Curr. Hypertens. Rep. 19, 25. doi: 10.1007/s11906-017-0722-5
Pluznick, J. L., Protzko, R. J., Gevorgyan, H., Peterlin, Z., Sipos, A., Han, J., et al. (2013). Olfactory receptor responding to gut microbiota-derived signals plays a role in renin secretion and blood pressure regulation. Proc. Natl. Acad. Sci. U.S.A. 110, 4410–4415. doi: 10.1073/pnas.1215927110
Pugh, D., Dhaun, N. (2021). Hypertension and vascular inflammation: another piece of the genetic puzzle. Hypertension 77, 190–192. doi: 10.1161/hypertensionaha.120.16420
Rashid, S. K., Idris-Khodja, N., Auger, C., Alhosin, M., Boehm, N., Oswald-Mammosser, M., et al. (2014). Probiotics (VSL#3) prevent endothelial dysfunction in rats with portal hypertension: role of the angiotensin system. PloS One 9, e97458. doi: 10.1371/journal.pone.0097458
Rashidinejad, A., Bahrami, A., Rehman, A., Rezaei, A., Babazadeh, A., Singh, H., et al. (2022). Co-Encapsulation of probiotics with prebiotics and their application in functional/synbiotic dairy products. Crit. Rev. Food Sci. Nutr. 62, 2470–2494. doi: 10.1080/10408398.2020.1854169
Recinella, L., Orlando, G., Ferrante, C., Chiavaroli, A., Brunetti, L., Leone, S. (2020). Adipokines: new potential therapeutic target for obesity and metabolic, rheumatic, and cardiovascular diseases. Front. Physiol. 11. doi: 10.3389/fphys.2020.578966
Rison, S. C., Carvalho, C., Rull, G., Robson, J. (2022). Investigating hypertension in younger patients. Bmj 376, e067924. doi: 10.1136/bmj-2021-067924
Rivas, A. M., Pena, C., Kopel, J., Dennis, J. A., Nugent, K. (2021). Hypertension and hyperthyroidism: association and pathogenesis. Am. J. Med. Sci. 361, 3–7. doi: 10.1016/j.amjms.2020.08.012
Robles-Vera, I., de la Visitación, N., Toral, M., Sánchez, M., Romero, M., Gómez-Guzmán, M., et al. (2020a). Probiotic bifidobacterium breve prevents DOCA-salt hypertension. FASEB J. 34, 13626–13640. doi: 10.1096/fj.202001532R
Robles-Vera, I., Toral, M., de la Visitación, N., Sánchez, M., Gómez-Guzmán, M., Romero, M., et al. (2020b). Probiotics prevent dysbiosis and the rise in blood pressure in genetic hypertension: role of short-chain fatty acids. Mol. Nutr. Food Res. 64, e1900616. doi: 10.1002/mnfr.201900616
Robles-Vera, I., Toral, M., de la Visitación, N., Sánchez, M., Romero, M., Olivares, M., et al. (2018). The probiotic lactobacillus fermentum prevents dysbiosis and vascular oxidative stress in rats with hypertension induced by chronic nitric oxide blockade. Mol. Nutr. Food Res. 62, e1800298. doi: 10.1002/mnfr.201800298
Rochette, L., Lorin, J., Zeller, M., Guilland, J. C., Lorgis, L., Cottin, Y., et al. (2013). Nitric oxide synthase inhibition and oxidative stress in cardiovascular diseases: possible therapeutic targets? Pharmacol. Ther. 140, 239–257. doi: 10.1016/j.pharmthera.2013.07.004
Rui, X., Wen, D., Li, W., Chen, X., Jiang, M., Dong, M. (2015). Enrichment of ACE inhibitory peptides in navy bean (Phaseolus vulgaris) using lactic acid bacteria. Food Funct. 6, 622–629. doi: 10.1039/c4fo00730a
Salminen, S., Collado, M. C., Endo, A., Hill, C., Lebeer, S., Quigley, E. M. M., et al. (2021). The international scientific association of probiotics and prebiotics (ISAPP) consensus statement on the definition and scope of postbiotics. Nat. Rev. Gastroenterol. Hepatol. 18, 649–667. doi: 10.1038/s41575-021-00440-6
Savoia, C., Tabet, F., Yao, G., Schiffrin, E. L., Touyz, R. M. (2005). Negative regulation of RhoA/Rho kinase by angiotensin II type 2 receptor in vascular smooth muscle cells: role in angiotensin II-induced vasodilation in stroke-prone spontaneously hypertensive rats. J. Hypertens. 23, 1037–1045. doi: 10.1097/01.hjh.0000166845.49850.39
Schober, A., Zernecke, A. (2007). Chemokines in vascular remodeling. Thromb. haemostasis 97 (5), 730–737.
Senoner, T., Dichtl, W. (2019). Oxidative stress in cardiovascular diseases: still a therapeutic target? Nutrients 11 (9), 2090. doi: 10.3390/nu11092090
Seravalle, G., Grassi, G. (2017). Obesity and hypertension. Pharmacol. Res. 122, 1–7. doi: 10.1016/j.phrs.2017.05.013
Shen, Y., Chang, C., Zhang, J., Jiang, Y., Ni, B., Wang, Y. (2017). Prevalence and risk factors associated with hypertension and prehypertension in a working population at high altitude in China: a cross-sectional study. Environ. Health Prev. Med. 22, 19. doi: 10.1186/s12199-017-0634-7
Shu, G., Shi, X., Chen, H., Ji, Z., Meng, J. (2019). Optimization of nutrient composition for producing ACE inhibitory peptides from goat milk fermented by lactobacillus bulgaricus LB6. Probiotics Antimicrob. Proteins 11, 723–729. doi: 10.1007/s12602-018-9410-2
Sitia, S., Tomasoni, L., Atzeni, F., Ambrosio, G., Cordiano, C., Catapano, A., et al. (2010). From endothelial dysfunction to atherosclerosis. Autoimmun. Rev. 9 (12), 830–834. doi: 10.1016/j.autrev.2010.07.016
Soehnlein, O., Libby, P. (2021). Targeting inflammation in atherosclerosis - from experimental insights to the clinic. Nat. Rev. Drug Discovery 20, 589–610. doi: 10.1038/s41573-021-00198-1
Solanki, D., Sakure, A., Prakash, S., Hati, S. (2022). Characterization of angiotensin I-converting enzyme (ACE) inhibitory peptides produced in fermented camel milk (Indian breed) by lactobacillus acidophilus NCDC-15. J. Food Sci. Technol. 59, 3567–3577. doi: 10.1007/s13197-022-05357-9
Stoll, S., Wang, C., Qiu, H. (2018). DNA Methylation and histone modification in hypertension. Int. J. Mol. Sci. 19(4), 1174. doi: 10.3390/ijms19041174
Suadoni, M. T. (2021). Correspondence on 'A. Grylls, K. Seidler, J. Neil, link between microbiota and hypertension: focus on LPS/TLR4 pathway in endothelial dysfunction and vascular inflammation, and therapeutic implication of probiotics'. BioMed. Pharmacother. 138, 111432. doi: 10.1016/j.biopha.2021.111432
Supriya, R., Yung, B. Y., Yu, A. P., Lee, P. H., Lai, C. W., Cheng, K. K., et al. (2018). Adipokine profiling in adult women with central obesity and hypertension. Front. Physiol. 9. doi: 10.3389/fphys.2018.00294
Teixeira, S. K., Pereira, A. C., Krieger, J. E. (2018). Genetics of resistant hypertension: the missing heritability and opportunities. Curr. Hypertens. Rep. 20, 48. doi: 10.1007/s11906-018-0852-4
Tesauro, M., Mauriello, A., Rovella, V., Annicchiarico-Petruzzelli, M., Cardillo, C., Melino, G., et al. (2017). Arterial ageing: from endothelial dysfunction to vascular calcification. J. Internal Med. 281 (5), 471–482. doi: 10.1111/joim.12605
Tinsley, J. H., South, S., Chiasson, V. L., Mitchell, B. M. (2010). Interleukin-10 reduces inflammation, endothelial dysfunction, and blood pressure in hypertensive pregnant rats. Am. J. Physiol. Regul. Integr. Comp. Physiol. 298, R713–R719. doi: 10.1152/ajpregu.00712.2009
Toral, M., Gómez-Guzmán, M., Jiménez, R., Romero, M., Sánchez, M., Utrilla, M. P., et al. (2014). The probiotic Lactobacillus coryniformis CECT5711 reduces the vascular pro-oxidant and pro-inflammatory status in obese mice. Clin. Sci. (London Engl. 1979) 127 (1), 33–45. doi: 10.1042/CS20130339
Toral, M., Romero, M., Rodríguez-Nogales, A., Jiménez, R., Robles-Vera, I., Algieri, F., et al. (2018). Lactobacillus fermentum improves tacrolimus-induced hypertension by restoring vascular redox state and improving eNOS coupling. Mol. Nutr. Food Res. 62 (14), e1800033. doi: 10.1002/mnfr.201800033
Tsai, Y. L., Lin, T. L., Chang, C. J., Wu, T. R., Lai, W. F., Lu, C. C., et al. (2019). Probiotics, prebiotics and amelioration of diseases. J. BioMed. Sci. 26, 3. doi: 10.1186/s12929-018-0493-6
Vallée, A., Gabet, A., Grave, C., Sorbets, E., Blacher, J., Olié, V. (2020). Patterns of hypertension management in France in 2015: the ESTEBAN survey. J. Clin. Hypertens. (Greenwich) 22, 663–672. doi: 10.1111/jch.13834
Verhaar, B. J. H., Prodan, A., Nieuwdorp, M., Muller, M. (2020). Gut microbiota in hypertension and atherosclerosis: a review. Nutrients 12(10), 2982. doi: 10.3390/nu12102982
Vourakis, M., Mayer, G., Rousseau, G. (2021). The role of gut microbiota on cholesterol metabolism in atherosclerosis. Int. J. Mol. Sci. 22(15), 8074. doi: 10.3390/ijms22158074
Wang, X. L., Chen, W. J., Jin, R., Xu, X., Wei, J., Huang, H., et al. (2023). Engineered probiotics clostridium butyricum-pMTL007-GLP-1 improves blood pressure via producing GLP-1 and modulating gut microbiota in spontaneous hypertension rat models. Microb. Biotechnol. 16, 799–812. doi: 10.1111/1751-7915.14196
Wang, C., Yuan, Y., Zheng, M., Pan, A., Wang, M., Zhao, M., et al. (2020). Association of age of onset of hypertension with cardiovascular diseases and mortality. J. Am. Coll. Cardiol. 75, 2921–2930. doi: 10.1016/j.jacc.2020.04.038
Wei, Q., Zhang, L., Duan, M. F., Wang, Y. M., Huang, N., Song, C. R. (2021). Use of angiotensin II receptor blocker during pregnancy: a case report. Med. (Baltimore) 100, e24304. doi: 10.1097/md.0000000000024304
Weijmans, M., van der Graaf, Y., De Borst, G. J., Asselbergs, F. W., Cramer, M. J., Algra, A., et al. (2015). The relation between the presence of cardiovascular disease and vascular risk factors in offspring and the occurrence of new vascular events in their parents already at high vascular risk. Am. Heart J. 170, 744–752.e742. doi: 10.1016/j.ahj.2015.06.023
Wu, N., Zheng, F., Li, N., Han, Y., Xiong, X. Q., Wang, J. J., et al. (2021). RND3 attenuates oxidative stress and vascular remodeling in spontaneously hypertensive rat via inhibiting ROCK1 signaling. Redox Biol. 48, 102204. doi: 10.1016/j.redox.2021.102204
Xiao, L., Harrison, D. G. (2020). Inflammation in hypertension. Can. J. Cardiol. 36, 635–647. doi: 10.1016/j.cjca.2020.01.013
Yang, T., Santisteban, M. M., Rodriguez, V., Li, E., Ahmari, N., Carvajal, J. M., et al. (2015). Gut dysbiosis is linked to hypertension. Hypertension 65, 1331–1340. doi: 10.1161/hypertensionaha.115.05315
Yeo, S. K., Liong, M. T. (2010). Angiotensin I-converting enzyme inhibitory activity and bioconversion of isoflavones by probiotics in soymilk supplemented with prebiotics. Int. J. Food Sci. Nutr. 61, 161–181. doi: 10.3109/09637480903348122
Yeo, S. K., Ooi, L. G., Lim, T. J., Liong, M. T. (2009). Antihypertensive properties of plant-based prebiotics. Int. J. Mol. Sci. 10 (8), 3517–3530. doi: 10.3390/ijms10083517
Yi, Y., Qu, T., Shi, A., Pang, Z., Zhao, Y., Li, P., et al. (2022). Relationship between inflammatory cells level and longer duration of hypertension in Chinese community residents. Clin. Exp. hypertension (New York N.Y. 1993) 44 (7), 619–626. doi: 10.1080/10641963.2022.2100411
Yuan, L., Li, Y., Chen, M., Xue, L., Wang, J., Ding, Y., et al. (2023). Effects of probiotics on hypertension. Appl. Microbiol. Biotechnol. 107, 1107–1117. doi: 10.1007/s00253-023-12369-8
Yuan, L., Li, Y., Chen, M., Xue, L., Wang, J., Ding, Y., et al. (2022). Antihypertensive activity of milk fermented by lactiplantibacillus plantarum SR37-3 and SR61-2 in l-NAME-Induced hypertensive rats. Foods 11(15), 2332. doi: 10.3390/foods11152332
Zennaro, M. C., Boulkroun, S., Fernandes-Rosa, F. L. (2020). Pathogenesis and treatment of primary aldosteronism. Nat. Rev. Endocrinol. 16, 578–589. doi: 10.1038/s41574-020-0382-4
Zhang, L., Bao, Y., Tao, S., Zhao, Y., Liu, M. (2022). The association between cardiovascular drugs and depression/anxiety in patients with cardiovascular disease: a meta-analysis. Pharmacol. Res. 175, 106024. doi: 10.1016/j.phrs.2021.106024
Zhang, Y., Wang, L., Zhou, X., Geng, J., Li, X. (2019). The immunomodulatory mechanism of brain injury induced by hyperhomocysteinemia in spontaneously hypertensive rats. J. Cell. Biochem. 120 (6), 9421–9429. doi: 10.1002/jcb.28217
Zhang, X., Zhao, H., Zhang, J., Han, D., Zheng, Y., Guo, X., et al. (2015). Gene environment interaction of GALNT2 and APOE gene with hypertension in the Chinese han population. BioMed. Mater Eng. 26 Suppl 1, S1977–S1983. doi: 10.3233/bme-151501
Keywords: probiotics, functional foods, hypertension, mechanisms, intestinal flora
Citation: Chen Z, Liang W, Liang J, Dou J, Guo F, Zhang D, Xu Z and Wang T (2023) Probiotics: functional food ingredients with the potential to reduce hypertension. Front. Cell. Infect. Microbiol. 13:1220877. doi: 10.3389/fcimb.2023.1220877
Received: 11 May 2023; Accepted: 15 June 2023;
Published: 03 July 2023.
Edited by:
Zhendong Cai, Ningbo University, ChinaReviewed by:
Zhang Chenchen, Yangzhou University, ChinaZhilan Sun, Jiangsu Academy of Agricultural Sciences (JAAS), China
Copyright © 2023 Chen, Liang, Liang, Dou, Guo, Zhang, Xu and Wang. This is an open-access article distributed under the terms of the Creative Commons Attribution License (CC BY). The use, distribution or reproduction in other forums is permitted, provided the original author(s) and the copyright owner(s) are credited and that the original publication in this journal is cited, in accordance with accepted academic practice. No use, distribution or reproduction is permitted which does not comply with these terms.
*Correspondence: Zhenshang Xu, eHV6aGVuc2hhbmdAMTI2LmNvbQ==; Ting Wang, dGluZ3dhbmdfOTdAaG90bWFpbC5jb20=