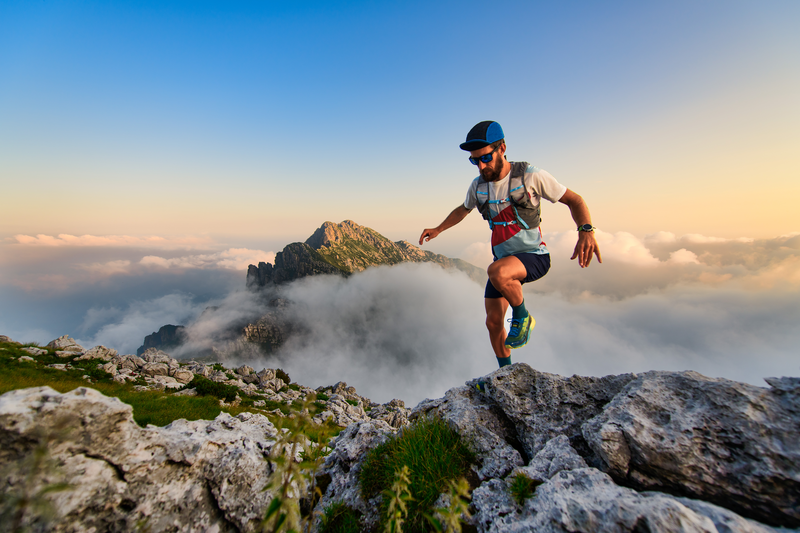
94% of researchers rate our articles as excellent or good
Learn more about the work of our research integrity team to safeguard the quality of each article we publish.
Find out more
ORIGINAL RESEARCH article
Front. Cell. Infect. Microbiol. , 20 February 2024
Sec. Clinical Microbiology
Volume 13 - 2023 | https://doi.org/10.3389/fcimb.2023.1220012
This article is part of the Research Topic Clinical metagenomics-based diagnostics for infectious diseases View all 25 articles
Background: Fungal co-infection is prevalent in critically ill patients with COVID-19. The conventional approach applied to fungal identification has relatively low sensitivity and is time-consuming. The metagenomic next-generation sequencing (mNGS) technology can simultaneously detect a variety of microorganisms, and is increasingly being used for the rapid detection and diagnosis of pathogens.
Methods: In this single-center retrospective study, we described the clinical presentation and outcomes of COVID-19 and mNGS positive for fungi in pulmonary critically ill patients during the outbreak of Omicron infection from December 2022 to January 2023.
Results: Among 43 COVID-19 patients with acute respiratory distress syndrome (ARDS) on a single intensive care unit (ICU), 10 were reported to be fungal positive using the mNGS test. The number of pathogenic microorganisms detected by mNGS was significantly higher than that via traditional methods, especially in the detection of fungi and viruses. Aspergillus infection was dominant, and most of these patients also had concurrent bacterial or viral infections. Probable or possible COVID-19-associated pulmonary aspergillosis (CAPA) was diagnosed in all 10 patients, and the prognosis was poor.
Conclusion: Patients with COVID-19 may be at increased risk of developing fungal infections as well as concurrent bacterial or viral infections, and mNGS can be a powerful tool in identifying these infections. Clinicians should be aware of the increased risk of fungal infections in COVID-19 patients, particularly those who have underlying immunocompromising conditions, and should monitor for early signs of infection.
The pandemic of an infectious disease named coronavirus disease 2019 (COVID-19) caused by severe acute respiratory syndrome coronavirus 2 (SARS-CoV-2) has become a worldwide threat (Shereen et al., 2020; Hu et al., 2021). COVID-19 infection has rapidly spread over China since December 2022 despite restrictive control measures by the government (The, 2023). As the infection continued to spread, severe pneumonia patients infected with SARS-CoV-2 requiring intensive care unit (ICU) admission gradually emerged.
The prevalence of fungal co-infection was reported in critically ill patients with COVID-19 and the mortality rate remains high (Bhatt et al., 2021; Seyedjavadi et al., 2022). A pre-Omicron outbreak retrospective observational study conducted in China revealed that 5.8% (3/52) of critically ill patients exhibited fungal co-infections (Yang et al., 2020). The identified infectious fungi included Aspergillus flavus, Aspergillus fumigatus, and Candida albicans. As far as our knowledge extends, following the emergence of the Omicron variant in China, substantial data encompassing a large sample size to characterize co-existing fungal infections in COVID-19 cases are currently lacking. Therefore, further clinical evidence in relation to the COVID-19 and fungal co-infection in critically ill patients is necessary. Rapid identification of pulmonary fungal infections facilitates the well-timed antifungal therapy. Despite the fact that microscopy and culture are the conventional approach applied to fungal identification, both methods have a relatively low sensitivity, and culture is a time-consuming process because of the long fungal growth time (Li, 2022). Histopathological diagnosis is still the gold standard for identifying invasive fungal infections (IFIs), despite it being a lengthy process (Mendonca et al., 2022). Immunological recognition of serum galactomannan and β-d-glucan antigen has significant auxiliary diagnostic efficacy, but there are many cofounding factors (Li, 2022). The metagenomic next-generation sequencing (mNGS) technology is a high-throughput DNA or RNA sequencing method that can simultaneously detect a variety of microorganisms, including bacteria, viruses, and fungi, and is widely used for the rapid detection and diagnosis of pathogens (Gu et al., 2021). Although mNGS has disadvantages such as its high cost, susceptibility to exogenous microbial contamination, and reduced efficiency in detecting thick-walled fungi, research has showcased its superior sensitivity and specificity in diagnosing invasive fungal diseases compared to traditional culture and histopathological methods (El-Kamand et al., 2019; Song et al., 2021; Wang et al., 2022). In certain circumstances, mNGS has a superior positive detection efficiency and can determine fungal pathogens that are difficult to diagnose via conventional diagnostic approaches (Zheng et al., 2021; Wu et al., 2023).
In this single-center retrospective study, we described the clinical presentation and outcomes of COVID-19 and mNGS positive for fungi in pulmonary critically ill patients during the outbreak of Omicron infection from December 2022 to January 2023.
This is a retrospective single-center cohort study in China. Patients who were admitted to the ICU at the First Affiliated Hospital of Zhengzhou University between 29 December 2022 and 23 January 2023 were reviewed. All adult patients (aged > 18 years) who were diagnosed with COVID-19 and acute respiratory distress syndrome (ARDS), and who had undergone mNGS testing at least once, were screened. Furthermore, patients positive for fungi via mNGS testing were included in our study.
Demographic, epidemiological, clinical, laboratory, treatment, and outcome data were retrieved from electronic medical records utilizing a standardized data collection form. All data were examined by two physicians (CH and SC), and a third researcher (YW) resolved contradictions between the two reviewers, if any.
Methods for laboratory identification of SARS-CoV-2 infection have been described previously (Huang et al., 2020a). Briefly, the clinical laboratory of the First Affiliated Hospital of Zhengzhou University was responsible for SARS-CoV-2 detection in respiratory specimens using real-time RT-PCR or the antigen rapid detection test. SARS-CoV-2 and other pathogen detection in bronchoalveolar lavage fluid (BALF) or peripheral blood (PB) by mNGS was performed by the clinical laboratory of the First Affiliated Hospital of Zhengzhou University or Hangzhou Matridx Biotechnology Co., Ltd., Zhejiang, China. Initial data from the mNGS upon admission to the ICU were collected for analysis if the patient had multiple mNGS tests.
Results of the first examination after admission to ICU of routine blood examinations, procalcitonin (PCT), C-reactive protein (CRP), interleukin-6 (IL-6), N-terminal pro-brain natriuretic peptide (BNP), creatinine (Cr), urea, prothrombin time (PT), activated partial thromboplastin time (APTT), d-dimer, glucose (Glu), alanine aminotransferase (ALT), total bilirubin (TBIL), lactic acid (Lac), high-sensitivity cardiac troponin T (TNT), high-sensitivity cardiac troponin I (TNI), creatine kinase-MB (CK-MB), lymphocyte immunoassay, galactomannan, β-D-glucan, and microbial culture of PB, BALF, and sputum were collected for further analysis. Variables might be unavailable in some circumstances. Chest x-ray or CT scan was also performed for all patients. Frequency of examinations was determined by the managing physician. SOFA score, CURB-65 score, and APACHE II score were collected upon admission to the ICU.
Nucleic acid extraction, library preparation, and sequencing: For the BALF, cell walls of microbes were broken by vortex with glass beads. For the blood, the plasma was separated. DNA was extracted using the TIANamp micro DNA kit (DP316; Tiangen Biotech, Beijing, China) following the manufacturer’s operation manual. Total RNA was extracted with a QIAamp® Viral RNA Kit (Qiagen) and ribosomal RNA was removed using a Ribo-Zero rRNA Removal Kit (Illumina). cDNA was generated using reverse transcriptase, random hexamer primers, and dNTPs (Thermo Fisher). Libraries were constructed for the DNA and cDNA samples using a Nextera XT DNA Library Prep Kit (Illumina, San Diego, CA). The library was purified and the fragments were selected by the magnetic beads. The library was quality assessed by the Qubit dsDNA HS Assay kit followed by the High Sensitivity DNA kit (Agilent) on an Agilent 2100 Bioanalyzer. The size of the qualified library was 300 to 500 bp without adapters and PCR dimers, and the concentration of the library was greater than 1 ng/mL. Library pools were then loaded onto an Illumina Nextseq 500CN sequencer for 75 cycles of single-end sequencing to generate approximately 20 million reads for each library. The internal control, named UMSI (unique molecular spiked-in), was added to the sample before the DNA extraction. The sequence of UMSI varied in different samples. Each mNGS assay run included an external negative control that ran in parallel with clinical samples. During analysis, the contamination between samples can be found if the UMSI sequence was the same or the reads of some pathogens in the external control were very high.
Bioinformatics analyses: High-quality sequencing data were generated by removing low-quality and short (length < 40 bp) reads, followed by computational subtraction of human host sequences mapped to the human reference genome (hg38 and YH sequences) using Burrows–Wheeler Alignment. After the removal of low-complexity reads, the remaining data were classified by simultaneously aligning them to four microbial genome databases, namely, viruses, bacteria, fungi, and parasites. The classification reference databases were downloaded and optimized from public databases such as NCBI, EBI, or Genbank. In the end, the multi-parameters of species in microbial genome databases were calculated and exported, and professionals with microbiology and clinical backgrounds interpreted the results.
ARDS was diagnosed in accordance with the Berlin definition (Force et al., 2012). For the diagnosis of COVID-19-associated invasive pulmonary aspergillosis (CAPA), the modified ECMM/ISHAM 2020 consensus criteria were adopted (Fortun et al., 2023):
Probable CAPA: Compatible clinical and imaging manifestations (pulmonary infiltrate or nodules preferably documented by chest CT or cavitating infiltrate), along with microbiological isolation of Aspergillus in BALF, galactomannan in BALF > 1, or galactomannan in serum > 0.5.
Possible CAPA: Compatible clinical and imaging manifestations, along with microbiological isolation of Aspergillus in respiratory specimens in sputum. Possible pulmonary CAPA requires pulmonary infiltrates, well-circumscribed lesions(s) or nodules, preferably documented by chest CT, or cavitating infiltrate that cannot be attributed to another cause. In patients with bilateral, ground-glass opacities or other COVID-19-related manifestations, significant radiological changes as described above and confirmed by a specialized radiologist are necessary to consider the possibility of CAPA.
The study was conducted in accordance with the ethical principles of Good Clinical Practice and the Declaration of Helsinki. The study was approved by the ethics committee of the First Affiliated Hospital of Zhengzhou University (Identifier: 2023-KY-0139-001). This study was registered in chictr.org/cn (registry number: ChiCTR2300070926).
A total of 43 successive ARDS patients with COVID-19 (34 male and 9 female patients; median age, 60 years [IQR 44–76]) were detected. Of the 43 patients, 10 patients who tested positive for fungi via mNGS were included (Table 1).
Table 1 Characteristics of pulmonary critically ill patients with COVID-19 and positive for fungi by metagenomic analysis.
Patient #1: A 41-year-old man was admitted to the ICU because of severe ARDS with a Horowitz Index of 79 mm Hg. Nasopharyngeal swab PCR test showed that he was positive for SARS-CoV-2. He had a medical history of hypertension and type 2 diabetes. He underwent right kidney transplantation in 2003; tacrolimus, mycophenolate, and steroids were administered for immunosuppression therapy. Chest CT showed bilateral ground-glass opacities with mist-like high-density shadows (Supplementary Video S1; Figure 1A). PB was positive for A. fumigatus, and BALF was positive for A. fumigatus, Aspergillus niger, Pneumocystis jeroveci, Enterococcus faecium, Leuconostoc lactis, and Tropheryma whipplei, via the mNGS test. BALF culture tested positive for A. flavus. Intravenous voriconazole and inhalational amphotericin B were utilized for antifungal treatment. Sulfamethoxazole was administered as anti-pneumocystis prophylaxis. Meropenem and linezolid were adopted for antibacterial therapy. Penciclovir was adopted for antiviral therapy. Azvudine and Paxlovid were sequentially used for anti-COVID-19 therapy. Steroids were used to treat pneumonia, and prone positioning was also utilized. Because of acute renal failure, continuous renal replacement therapy (CRRT) was started.
Figure 1 Thoracic CT-scan/x-ray of patients with COVID-19-associated pulmonary fungal infection. (A) Patient #1: Combined bilateral ground-glass opacities accompanied by mist-like shadows of high density. (B) Patient #2: Bilateral reductions in transparency with the presence of multiple nodular shadows of high density, accompanied by consolidations. (C) Patient #3: Bilateral multiple ground-glass opacities and patchy shadows. (D) Patient #4: The bronchovascular shadows bilaterally increased and blurred, accompanied by multiple patchy, cord-like, and round-like high-density shadows, as well as consolidations. (E) Patient #5: Bilateral ground-glass opacities present along with multiple patchy, cord-like high-density shadows. (F) Patient #6: Bilateral ground-glass opacities with patchy, cord-like high-density shadows, as well as cavities in both upper lung lobes. In addition, there was evidence of hydropneumothorax causing the right lung to collapse. (G) Patient #7: Bilateral ground-glass opacities with multiple small nodules and patchy, cord-like high-density shadows. Additionally, there were multiple cavities and consolidations observed in the right lung lobe. (H) Patient #8: Bilateral thickening of the lung texture and the presence of small exudation shadows. (I) Patient #9: Bilateral bronchovascular shadows that appeared increased and blurred, along with the presence of multiple exudations. (J) Patient #10: Bilateral increased thickening of the lung texture, with evidence of pleural effusion and atelectasis on the left side, as well as pneumothorax on the right side.
Patient #2: A 55-year-old man was transferred to the ICU due to ARDS with a Horowitz Index of 97 mm Hg. Antigen rapid detection test revealed that he was SARS-CoV-2 positive (24 days before ICU admission). He had a medical history of acute kidney injury. He underwent liver transplantation owing to hepatitis B cirrhosis; tacrolimus and mycophenolate were administered for immunosuppression therapy. Chest CT showed bilateral decreased transparency and multiple nodular high-density shadows, with pulmonary consolidation (Supplementary Video S2; Figure 1B). A. flavus/Aspergillus oryzae, Klebsiella pneumoniae, and Acinetobacter baumannii were detected in BALF; A. fumigatus, K. pneumoniae, human alphaherpesvirus 1, and Epstein–Barr virus were detected in PB, via the mNGS test. A. baumannii was grown in sputum and BALF culture. E. faecium and K. pneumoniae were detected in PB culture. Intravenous voriconazole and inhalational amphotericin B were used for antifungal therapy. Ceftazidime, avibactam sodium, and polymyxin B were administered for antibacterial therapy. Penciclovir was used for antiviral therapy. Paxlovid was adopted to treat COVID-19. Steroids and prone positioning were also commenced. Owing to acute renal failure, CRRT was initiated.
Patient #3: A 62-year-old woman was transferred to the ICU by virtue of ARDS and having tested positive for SARS-CoV-2 via PCR. Her Horowitz Index was 70 mm Hg. Previous medical history suggested hypertension and nephritic syndrome. Mycophenolate was taken regularly for 2 years. Chest CT scan exhibited multiple ground-glass opacities and patchy shadows in both lungs (Supplementary Video S3; Figure 1C). A. fumigatus and A. flavus were both detected in BALF via mNGS and culture. Ceftizoxime was adopted for empirical treatment after ICU admission. Intravenous voriconazole was started after testing positive for Aspergillus. Azvudine and Paxlovid were successively commenced. Prone positioning was also put to use.
Patient #4: A 54-year-old man was admitted to the ICU because of mild ARDS (Horowitz Index of 229 mm Hg), coma, acute cerebral infarction, cerebral hernia, and septic shock. PCR test showed that he was positive for SARS-CoV-2. Previous medical history suggested hypertension, type 2 diabetes, and liver abscess after percutaneous drainage. Chest CT showed bilateral increased and blurred bronchovascular shadows with multiple patchy, cord-like, and round-like high-density shadows, as well as consolidations (Supplementary Video S4; Figure 1D). A. fumigatus, K. pneumoniae, Streptococcus pneumoniae, A. baumannii, E. faecium, human alphaherpesvirus 1, and Epstein–Barr virus were detected in BALF via mNGS analysis. Sputum culture grew A. baumannii, and drainage fluid of liver abscess culture grew K. pneumoniae. Intravenous voriconazole was commenced. Meropenem, ceftazidime, and avibactam sodium were utilized for antibacterial therapy.
Patient #5: A 53-year-old man presented with ARDS with a Horowitz Index of 99 mm Hg. Antigen rapid detection test suggested that he was SARS-CoV-2 positive. He was diagnosed with thymoma 20 days ago and was treated with oxaliplatin + cyclophosphamide + doxorubicine chemotherapy. Chest CT demonstrated bilateral ground-glass opacities and multiple patchy, cord-like high-density shadows (Supplementary Video S5; Figure 1E). A. fumigatus, A. baumannii, and human betaherpesvirus 5 were detected in BALF via the mNGS test. Imipenem, cilastatin sodium, and teicoplanin were adopted for antibacterial treatment. Intravenous voriconazole was started. On account of acute renal failure, CRRT was commenced. Owing to unsustainability of circulation, venoarterial (V-A) extracorporeal membrane oxygenation (ECMO) was put into use.
Patient #6: A 44-year-old woman was transferred to the ICU as a result of ARDS with a Horowitz Index of 117 mm Hg. PCR test suggested SARS-CoV-2 infection. She had a medical history of hypertension and uremia. She received kidney transplantation 10 years ago and was regularly taking tacrolimus and mycophenolate for antirejection therapy. Chest CT revealed ground-glass opacities with patchy, cord-like high-density shadows in both lungs, and cavity in the both upper lung lobes, as well as hydropneumothorax with a collapsed right lung (Supplementary Video S6; Figure 1F). A. flavus, A. fumigatus, E. faecium, Acinetobacter pittii, and Torque teno virus were detected in BALF via the mNGS test. Serum β-D-glucan also had a positive result. Intravenous voriconazole was utilized for antifungal therapy. Biapenem and linezolid were adopted for antibacterial therapy. Ganciclovir was used for antiviral therapy. Paxlovid was used for anti-COVID-19 treatment. Steroids were also started to treat pneumonia.
Patient #7: A 31-year-old man was admitted to the ICU because of mild ARDS (Horowitz Index: 79 mm Hg) and acute pancreatitis. PCR test showed that he was positive for SARS-CoV-2. Chest CT showed bilateral ground-glass opacities with multiple small nodules, patchy, cord-like high-density shadows, as well as multiple cavities and consolidations in the right lung lobe (Supplementary Video S7; Figure 1G). BALF was positive for A. flavus/A. oryzae, Rhizopus oryzae, Escherichia coli, and K. pneumoniae, via the mNGS test. A. baumannii was grown in sputum culture. E. coli and A. flavus were grown in BALF culture. Elevated galactomannan was seen in serum and BALF. Elevated serum β-D-glucan was also detected. Intravenous isavuconazole and inhalational amphotericin B were used for antifungal therapy. Imipenem, cilastatin sodium, ceftazidime, avibactam sodium, and teicoplanin were utilized for antibacterial treatment. Despite prone positioning, the patient required venovenous (V-V) ECMO supportive treatment to restore and maintain circulation stabilization.
Patient #8: A 76-year-old man was admitted to our ICU. He was intubated and developed severe ARDS with a Horowitz Index of 73 mm Hg. Nasopharyngeal swab PCR test showed that he was positive for SARS-CoV-2. He had a medical history of coronary atherosclerotic heart disease after percutaneous transluminal coronary intervention, liver cirrhosis, hypersplenism, old cerebral infarction, type 2 diabetes, and hypertension. X-ray radiography showed a combination of bilateral thickening of the lung texture and small exudation shadows (Figure 1H). The prehospital CT report suggested bilateral inferior pulmonary interstitial fibrosis and infection (no images available). A. flavus/A. oryzae, A. fumigatus, and A. baumannii were detected in BALF via the mNGS test. Elevated galactomannan in serum and BALF was detected. A. baumannii was detected in sputum and BALF culture. Intravenous voriconazole was used for antifungal treatment. Moxalactam or cefoperazone sodium and sulbactam sodium were used for antibacterial therapy. Azvudine or Paxlovid was separately adopted for anti-COVID-19 therapy. Steroids and prone positioning were also utilized. The patient eventually died of respiratory failure and respiratory cardiac arrest (Patient #1, Table 1).
Patient #9: A 73-year-old man presented with ARDS with a Horowitz Index of 48 mm Hg. PCR test revealed that he was SARS-CoV-2 positive. Chest x-ray imaged bilateral increased and blurred bronchovascular shadows, with multiple exudation (Figure 1I). Chest CT from the local hospital suggested bilateral ground-glass opacities (no images available). Azvudine and Paxlovid were already administered as anti-COVID-19 treatment at the local hospital. A. flavus, Aspergillus terreus, Clavispora lusitaniae, K. pneumoniae, and E. faecium were detected in BALF via mNGS. K. pneumoniae was grown in BALF and E. faecium was grown in PB. Cefoperazone sodium and sulbactam sodium were used for empirical treatment after ICU admission. The patient died of respiratory failure and malignant arrhythmias before the microbiological results became available.
Patient #10: An 83-year-old man was transferred to our ICU because of severe ARDS (Horowitz Index: 94 mm Hg) and cardiopulmonary arrest after cardiopulmonary resuscitation. PCR test suggested that he was SARS-CoV-2 positive. He had a medical history of hypertension and coronary heart disease. Chest x-ray radiography showed bilateral increased thickening of the lung texture, with pleural effusion and atelectasis on the left side, as well as pneumothorax on the right side (Figure 1J). BALF mNGS test revealed positive results for A. fumigatus and C. albicans. C. albicans was also detected in PB culture. V-V ECMO was performed after cardiopulmonary resuscitation. CRRT was adopted for renal replacement and toxin clearance. Imipenem and cilastatin sodium were utilized for empirical treatment after ICU admission. The patient died of respiratory failure and multiple organ dysfunction syndrome before antifungal therapy.
Among the 10 patients, 6 patients died, with all fatalities attributed to pulmonary co-infections caused by COVID-19 and subsequent multi-organ dysfunction, while the remaining 4 patients survived following treatment. Detailed patient characteristics are given in Table 1. Hematological test results for the 10 patients are presented in Table 2.
Patients with ARDS induced by SARS-CoV-2 infection are prone to fungal infections despite having no previous immunodeficiency conditions (Buil et al., 2020; Koehler et al., 2020). Among 43 COVID-19 patients with moderate to severe ARDS with or without underlying immunocompromising factors on a single ICU, 10 were reported to be fungal positive using the mNGS test.
In the past few years, mNGS has been increasingly utilized in clinical diagnosis and has remarkable practical application value in the diagnosis of pathogenic microbial infection (Gu et al., 2019; Duan et al., 2021; Tang et al., 2021). In our study, the number of pathogenic microorganisms detected by mNGS was significantly higher than that by traditional methods, especially in the detection of fungi and viruses, although some studies report that there was no significant difference in the specificity between mNGS and conventional methods (Chen et al., 2020b; Huang et al., 2020b). The combined application of mNGS and traditional methods may provide robust backup in the diagnosis of pulmonary pathogenic microorganism infections. Metagenomic sequencing is a powerful tool in identifying newly emerging, uncommon, and unexpected pathogens. In certain circumstances, it can even be a life-saving test and the most dependable way to detect disease-causing microorganisms (Wilson et al., 2014).
For patients with pulmonary infections, PB and sputum specimens are more readily obtainable. In our center, traditional culturing and immunological testing remain the preferred diagnostic methods. However, the overall diagnostic efficiency is relatively low, often necessitating repeated testing to yield positive results or with consistently negative results, particularly in cases where empirical antibiotic treatment has been administered. BALF is highly sensitive for pulmonary infection detection and is the preferred specimen type, particularly in critically ill patients requiring fiberoptic bronchoscopy. BALF can be used for microbial culture, biochemical testing, cytological examination, parasitological examination, and mNGS testing. In this study, mNGS test results were obtained for the first time after ICU admission, and in most cases, BALF specimens were predominantly used. Therefore, the time of mNGS specimen submission typically lags behind that of conventional culturing and immunological testing.
Our objective is quite clear: we aim to utilize mNGS technology for early and rapid screening of potential pathogenic microorganisms, thereby providing specific guidance for further antimicrobial treatment. In the analysis of these 10 cases, it is evident that COVID-19 patients have a significantly high occurrence of concurrent infections with other pathogenic microorganisms, and there is also a high probability of detecting fungi. However, it is not possible to diagnose IFIs solely through mNGS testing, as positive fungal detection in BALF samples may also indicate colonization. The gold standard for IFI diagnosis is histopathological biopsy. Nevertheless, patient conditions often render this approach unrealistic, and as such, we did not pursue it. COVID-19 patients who develop IFI exhibit poor treatment responsiveness and high mortality rates (Baddley et al., 2021; Fortun et al., 2023). Consequently, when we detected fungi in BALF through mNGS, antifungal treatment for the patients was subsequently initiated. However, owing to the limited number of cases, it is challenging to determine whether this combined anti-infective therapy decisively contributed to the patients’ ultimate recovery. Given this limitation, it may be necessary to expand the sample size in future mNGS-related studies. Additionally, this highlights a shortcoming of mNGS, as it can detect a wide array of pathogenic species but cannot distinguish between pathogenic, colonizing, and contaminating microorganisms. Following pathogen detection, it is imperative to integrate other infection-related indicators in patients, such as blood PCT, CRP, BALF galactomannan, β-D-glucan, BALF cultures, and chest imaging, among others, to comprehensively evaluate the patient’s infection status and thereby guide treatment decisions. Ultimately, treatment effectiveness should be assessed based on the patient’s response to therapy.
There is a growing body of evidence suggesting that patients, especially those admitted to the ICU with COVID-19, may be at increased risk of developing fungal infections, such as aspergillosis, mucormycosis, candidiasis, and cryptococcosis (Amin et al., 2021; Doman and Banyai, 2022; Hoenigl et al., 2022). This association is likely due to several factors, including the immunocompromised effects of COVID-19, the use of corticosteroids and other immunomodulatory therapies in the treatment of severe COVID-19, and the use of invasive medical procedures such as mechanical ventilation, which can increase the risk of fungal colonization and infection (Baddley et al., 2021; Ezeokoli et al., 2021). Aspergillus infection was dominant in our study, as revealed by mNGS analysis indicating that all 10 patients were infected with or had co-infections involving Aspergillus. Likewise, some previous studies also documented a notable rise in the occurrence of aspergillosis among COVID-19 patients who were critically ill (Chen et al., 2020a; Koehler et al., 2020; Chong and Neu, 2021). It is important for clinicians to be aware of the increased risk of fungal infections in COVID-19 patients, particularly those who have underlying immunocompromising conditions, and to monitor for early signs of infection. Treatment of fungal infections in these patients may require aggressive antifungal therapy and invasive intervention in some cases. The results of mNGS and conventional diagnostic methods suggest that most of these patients also had concurrent bacterial or viral infections. Elevated inflammatory marks, such as white blood cell count, CRP, PCT, or IL-6, also suggest the possibility of concurrent infection. These patients progressed rapidly and had a poor prognosis; empirical use of antibiotics or antivirals before obtaining microbiological results may be reasonable.
The incidence of COVID-19-associated CAPA among patients who have been admitted to the ICU has been documented to vary from 19.6% to 33.3% (Fortun et al., 2023). Patients with CAPA had poorer prognoses, as evidenced by higher ordinal disease severity scores and longer recovery times, with mortality rates estimated to be approximately 50%, despite the widespread use of antifungals (Chong and Neu, 2021). In our study, of the 10 fungal-positive patients by mNGS testing, probable CAPA was diagnosed in 6 patients and possible CAPA was diagnosed in the remaining 4 patients. It is suggested that the results of mNGS testing and conventional diagnostic methods are largely consistent.
Lymphocyte analysis serves as a means to evaluate the immune system function, which includes the number and proportion of different types of lymphocytes in the blood (Luo et al., 2019). Lymphocyte analysis is of great significance for the diagnosis and prognosis evaluation of COVID-19 infection. COVID-19 infection may result in a decrease in lymphocyte count, especially CD8+ T, CD4+ T, and natural killer (NK) cells, which may lead to an impaired immune response to pathogens and aggravate the disease (Carsetti et al., 2020; Diao et al., 2020). Dysregulation of lymphocyte subsets may also be one of the reasons for the occurrence of dangerous and uncontrollable inflammatory response, namely, “cytokine storm”, in COVID-19 patients (Ghasemzadeh et al., 2022; Zanza et al., 2022). Some studies have found that the abnormal distribution of lymphocyte subpopulations may be associated with the severity of COVID-19 infection (Tan et al., 2020; Cantenys-Molina et al., 2021; Liu et al., 2021). We retrospectively reviewed the proportions and counts of T cells from the 10 patients (Table 2). Apart from one patient with thymoma (Patient #5), the T, CD4+ T, CD8+ T, and NK cell proportions in the other nine patients were mostly within the normal range or close to the normal range. However, the T, CD4+ T, CD8+ T, and NK cell counts of these patients were significantly decreased. These findings are consistent with literature reports, indicating that the immune system of patients with ARDS complicated by COVID-19 infection is significantly impaired. The lymphocyte ratio and count in blood routine examination also confirmed the immune system damage in these patients.
The SOFA scoring system has been widely applied in clinical research and critical care fields, especially in evaluating the condition and prognosis of patients with severe infections and ARDS. In our study, all 10 patients had a SOFA score greater than 2 upon admission to the ICU, indicating a high incidence of sepsis in patients with COVID-19 and ARDS. The CRB-65 score is used to assess the severity of community-acquired pneumonia and can help guide clinical decision-making for hospitalization and predict mortality. Interestingly, among the 10 patients, 8 patients had a CURB-65 score of less than 3 upon ICU admission. This suggests that for COVID-19 patients with ARDS, the CURB-65 score may need to be dynamically evaluated after admission. The Acute Physiology and Chronic Health Evaluation (APACHE) II score is a widely used clinical prediction tool that assesses the severity of critical illness in patients admitted to ICUs. Of the 10 patients, 6 patients had an APACHE II score greater than 15 upon ICU admission, indicating the severity of the disease.
In addition to respiratory symptoms, COVID-19 has been reported to cause damage to other organs, including the heart, liver, kidney, and the nervous system (Jain, 2020). Our study also supports the findings mentioned above. For example, some patients required renal replacement therapy or ECMO support (Table 1), while others exhibited abnormal coagulation, liver, kidney, or cardiac function indicators (Table 2). These extra-pulmonary manifestations highlight the systemic nature of COVID-19 and the need for comprehensive clinical management.
This study also has some limitations: (1) the sample size is relatively small, and (2) the data came from a single center during the outbreak of Omicron infection. Our findings need to be confirmed in clinical trials to elucidate the role of mNGS and COVID-19 in pulmonary critically ill patients in the future.
It is possible for COVID-19 patients to have a higher chance of developing fungal infections, along with simultaneous viral or bacterial infections. The utilization of mNGS can aid in detecting these infections effectively. Healthcare providers must be mindful of the heightened risk of fungal infections among COVID-19 patients, especially those with existing immunocompromised states. Early infection signs should be closely monitored.
The raw data supporting the conclusions of this article will be made available by the authors, without undue reservation.
The studies involving humans were approved by Ethic committee of the First Affiliated Hospital of Zhengzhou University. The studies were conducted in accordance with the local legislation and institutional requirements. The ethics committee/institutional review board waived the requirement of written informed consent for participation from the participants or the participants’ legal guardians/next of kin because (1) The research involves risks no greater than minimal risk, exclusively involves retrospective data collection, and does not interfere with clinical diagnosis and treatment; (2) Waiving informed consent will not adversely affect the rights or benefits of the subjects; (3) Patients have been discharged, and some have died. Without waiving informed consent, the research cannot proceed.
Conceptualization, WG and MF; methodology, CH and SC; validation, RM, YS, and YL; data curation, YW; writing-original draft preparation, CH and RM; writing-review and editing, SC, YS, and YL; supervision, WG and MF. All authors contributed to the article and approved the submitted version.
The author(s) declare financial support was received for the research, authorship, and/or publication of this article. This study was supported by the “Joint Construction Project of Medical Science and Technology of Henan Province” (No. LHGJ20220316) and the “Liver and Biliary Mutual Aid Fund of Henan Province Charity Federation” (No. GDXZ2023004).
The authors thank Huifang Sun for technical assistance.
The authors declare that the research was conducted in the absence of any commercial or financial relationships that could be construed as a potential conflict of interest.
All claims expressed in this article are solely those of the authors and do not necessarily represent those of their affiliated organizations, or those of the publisher, the editors and the reviewers. Any product that may be evaluated in this article, or claim that may be made by its manufacturer, is not guaranteed or endorsed by the publisher.
The Supplementary Material for this article can be found online at: https://www.frontiersin.org/articles/10.3389/fcimb.2023.1220012/full#supplementary-material
Amin, A., Vartanian, A., Poladian, N., Voloshko, A., Yegiazaryan, A., Al-Kassir, A. L., et al. (2021). Root causes of fungal coinfections in COVID-19 infected patients. Infect. Dis. Rep. 13, 1018–1035. doi: 10.3390/idr13040093
Baddley, J. W., Thompson, G. R., 3rd, Chen, S. C., White, P. L., Johnson, M. D., Nguyen, M. H., et al. (2021). Coronavirus disease 2019-associated invasive fungal infection. Open Forum Infect. Dis. 8, ofab510. doi: 10.1093/ofid/ofab510
Bhatt, K., Agolli, A., Patel, M. H., Garimella, R., Devi, M., Garcia, E., et al. (2021). High mortality co-infections of COVID-19 patients: mucormycosis and other fungal infections. Discoveries (Craiova) 9, e126. doi: 10.15190/d.2021.5
Buil, J. B., Meijer, E. F. J., Denning, D. W., Verweij, P. E., Meis, J. F. (2020). Burden of serious fungal infections in the Netherlands. Mycoses 63, 625–631. doi: 10.1111/myc.13089
Cantenys-Molina, S., Fernandez-Cruz, E., Francos, P., Lopez Bernaldo De Quiros, J. C., Munoz, P., Gil-Herrera, J. (2021). Lymphocyte subsets early predict mortality in a large series of hospitalized COVID-19 patients in Spain. Clin. Exp. Immunol. 203, 424–432. doi: 10.1111/cei.13547
Carsetti, R., Zaffina, S., Piano Mortari, E., Terreri, S., Corrente, F., Capponi, C., et al. (2020). Different innate and adaptive immune responses to SARS-CoV-2 infection of asymptomatic, mild, and severe cases. Front. Immunol. 11, 610300. doi: 10.3389/fimmu.2020.610300
Chen, N., Zhou, M., Dong, X., Qu, J., Gong, F., Han, Y., et al. (2020a). Epidemiological and clinical characteristics of 99 cases of 2019 novel coronavirus pneumonia in Wuhan, China: a descriptive study. Lancet 395, 507–513. doi: 10.1016/S0140-6736(20)30211-7
Chen, X., Ding, S., Lei, C., Qin, J., Guo, T., Yang, D., et al. (2020b). Blood and bronchoalveolar lavage fluid metagenomic next-generation sequencing in pneumonia. Can. J. Infect. Dis. Med. Microbiol. 2020, 6839103. doi: 10.1155/2020/6839103
Chong, W. H., Neu, K. P. (2021). Incidence, diagnosis and outcomes of COVID-19-associated pulmonary aspergillosis (CAPA): a systematic review. J. Hosp Infect. 113, 115–129. doi: 10.1016/j.jhin.2021.04.012
Diao, B., Wang, C., Tan, Y., Chen, X., Liu, Y., Ning, L., et al. (2020). Reduction and functional exhaustion of T cells in patients with coronavirus disease 2019 (COVID-19). Front. Immunol. 11, 827. doi: 10.3389/fimmu.2020.00827
Doman, M., Banyai, K. (2022). COVID-19-associated fungal infections: an urgent need for alternative therapeutic approach? Front. Microbiol. 13, 919501. doi: 10.3389/fmicb.2022.919501
Duan, H., Li, X., Mei, A., Li, P., Liu, Y., Li, X., et al. (2021). The diagnostic value of metagenomic next rectanglegeneration sequencing in infectious diseases. BMC Infect. Dis. 21, 62. doi: 10.1186/s12879-020-05746-5
El-Kamand, S., Papanicolaou, A., Morton, C. O. (2019). The use of whole genome and next-generation sequencing in the diagnosis of invasive fungal disease. Curr. Fungal Infection Rep. 13, 284–291. doi: 10.1007/s12281-019-00363-5
Ezeokoli, O. T., Gcilitshana, O., Pohl, C. H. (2021). Risk factors for fungal co-infections in critically ill COVID-19 patients, with a focus on immunosuppressants. J. Fungi (Basel) 7, 545. doi: 10.3390/jof7070545
Force, A. D. T., Ranieri, V. M., Rubenfeld, G. D., Thompson, B. T., Ferguson, N. D., Caldwell, E., et al. (2012). Acute respiratory distress syndrome: the Berlin Definition. JAMA 307, 2526–2533. doi: 10.1001/jama.2012.5669
Fortun, J., Mateos, M., de la Pedrosa, E. G., Soriano, C., Pestana, D., Palacios, J., et al. (2023). Invasive pulmonary aspergillosis in patients with and without SARS-CoV-2 infection. J. Fungi (Basel) 9, 130. doi: 10.3390/jof9020130
Ghasemzadeh, M., Ghasemzadeh, A., Hosseini, E. (2022). Exhausted NK cells and cytokine storms in COVID-19: Whether NK cell therapy could be a therapeutic choice. Hum. Immunol. 83, 86–98. doi: 10.1016/j.humimm.2021.09.004
Gu, W., Deng, X., Lee, M., Sucu, Y. D., Arevalo, S., Stryke, D., et al. (2021). Rapid pathogen detection by metagenomic next-generation sequencing of infected body fluids. Nat. Med. 27, 115–124. doi: 10.1038/s41591-020-1105-z
Gu, W., Miller, S., Chiu, C. Y. (2019). Clinical metagenomic next-generation sequencing for pathogen detection. Annu. Rev. Pathol. 14, 319–338. doi: 10.1146/annurev-pathmechdis-012418-012751
Hoenigl, M., Seidel, D., Sprute, R., Cunha, C., Oliverio, M., Goldman, G. H., et al. (2022). COVID-19-associated fungal infections. Nat. Microbiol. 7, 1127–1140. doi: 10.1038/s41564-022-01172-2
Hu, B., Guo, H., Zhou, P., Shi, Z. L. (2021). Characteristics of SARS-CoV-2 and COVID-19. Nat. Rev. Microbiol. 19, 141–154. doi: 10.1038/s41579-020-00459-7
Huang, C., Wang, Y., Li, X., Ren, L., Zhao, J., Hu, Y., et al. (2020a). Clinical features of patients infected with 2019 novel coronavirus in Wuhan, China. Lancet 395, 497–506. doi: 10.1016/S0140-6736(20)30183-5
Huang, J., Jiang, E., Yang, D., Wei, J., Zhao, M., Feng, J., et al. (2020b). Metagenomic next-generation sequencing versus traditional pathogen detection in the diagnosis of peripheral pulmonary infectious lesions. Infect. Drug Resist. 13, 567–576. doi: 10.2147/IDR.S235182
Koehler, P., Cornely, O. A., Bottiger, B. W., Dusse, F., Eichenauer, D. A., Fuchs, F., et al. (2020). COVID-19 associated pulmonary aspergillosis. Mycoses 63, 528–534. doi: 10.1111/myc.13096
Li, H. (2022). Editorial: mNGS for fungal pulmonary infection diagnostics. Front. Cell Infect. Microbiol. 12, 864163. doi: 10.3389/fcimb.2022.864163
Liu, G., Jiang, X., Zeng, X., Pan, Y., Xu, H. (2021). Analysis of lymphocyte subpopulations and cytokines in COVID-19-associated pneumonia and community-acquired pneumonia. J. Immunol. Res. 2021, 6657894. doi: 10.1155/2021/6657894
Luo, Y., Xie, Y., Zhang, W., Lin, Q., Tang, G., Wu, S., et al. (2019). Combination of lymphocyte number and function in evaluating host immunity. Aging (Albany NY) 11, 12685–12707. doi: 10.18632/aging.102595
Mendonca, A., Santos, H., Franco-Duarte, R., Sampaio, P. (2022). Fungal infections diagnosis - Past, present and future. Res. Microbiol. 173, 103915. doi: 10.1016/j.resmic.2021.103915
Seyedjavadi, S. S., Bagheri, P., Nasiri, M. J., Razzaghi-Abyaneh, M., Goudarzi, M. (2022). Fungal infection in co-infected patients with COVID-19: an overview of case reports/case series and systematic review. Front. Microbiol. 13, 888452. doi: 10.3389/fmicb.2022.888452
Shereen, M. A., Khan, S., Kazmi, A., Bashir, N., Siddique, R. (2020). COVID-19 infection: Origin, transmission, and characteristics of human coronaviruses. J. Adv. Res. 24, 91–98. doi: 10.1016/j.jare.2020.03.005
Song, N., Li, X., Liu, W. J. J. O. L., Medicine, P. (2021). Metagenomic next-generation sequencing (mNGS) for diagnosis of invasive fungal infectious diseases: a narrative review. Transl. Cancer Res. 10, 966–976. doi: 10.21037/jlpm-21-25
Tan, L., Wang, Q., Zhang, D., Ding, J., Huang, Q., Tang, Y. Q., et al. (2020). Lymphopenia predicts disease severity of COVID-19: a descriptive and predictive study. Signal Transduct Target Ther. 5, 33. doi: 10.1038/s41392-020-0148-4
Tang, W., Zhang, Y., Luo, C., Zhou, L., Zhang, Z., Tang, X., et al. (2021). Clinical application of metagenomic next-generation sequencing for suspected infections in patients with primary immunodeficiency disease. Front. Immunol. 12, 696403. doi: 10.3389/fimmu.2021.696403
Wang, C., You, Z., Fu, J., Chen, S., Bai, D., Zhao, H., et al. (2022). Application of metagenomic next-generation sequencing in the diagnosis of pulmonary invasive fungal disease. Front. Cell Infect. Microbiol. 12, 949505. doi: 10.3389/fcimb.2022.949505
Wilson, M. R., Naccache, S. N., Samayoa, E., Biagtan, M., Bashir, H., Yu, G., et al. (2014). Actionable diagnosis of neuroleptospirosis by next-generation sequencing. N Engl. J. Med. 370, 2408–2417. doi: 10.1056/NEJMoa1401268
Wu, S., Song, J., Hu, F., Li, H., Tian, Y., Cheng, W., et al. (2023). Pneumonia during the COVID-19 pandemic in hubei province, China: a case report of mucormycosis. Clin. Lab. 69. doi: 10.7754/Clin.Lab.2022.220905
Yang, X., Yu, Y., Xu, J., Shu, H., Xia, J., Liu, H., et al. (2020). Clinical course and outcomes of critically ill patients with SARS-CoV-2 pneumonia in Wuhan, China: a single-centered, retrospective, observational study. Lancet Respir. Med. 8, 475–481. doi: 10.1016/S2213-2600(20)30079-5
Zanza, C., Romenskaya, T., Manetti, A. C., Franceschi, F., La Russa, R., Bertozzi, G., et al. (2022). Cytokine storm in COVID-19: immunopathogenesis and therapy. Medicina (Kaunas) 58, 144. doi: 10.3390/medicina58020144
Keywords: COVID-19, SARS-CoV-2, aspergillosis, mNGS, CAPA
Citation: Huang C, Chang S, Ma R, Shang Y, Li Y, Wang Y, Feng M and Guo W (2024) COVID-19 in pulmonary critically ill patients: metagenomic identification of fungi and characterization of pathogenic microorganisms. Front. Cell. Infect. Microbiol. 13:1220012. doi: 10.3389/fcimb.2023.1220012
Received: 09 May 2023; Accepted: 31 December 2023;
Published: 20 February 2024.
Edited by:
Pushpanathan Muthuirulan, Harvard University, United StatesReviewed by:
Carolina Henritta Pohl, University of the Free State, South AfricaCopyright © 2024 Huang, Chang, Ma, Shang, Li, Wang, Feng and Guo. This is an open-access article distributed under the terms of the Creative Commons Attribution License (CC BY). The use, distribution or reproduction in other forums is permitted, provided the original author(s) and the copyright owner(s) are credited and that the original publication in this journal is cited, in accordance with accepted academic practice. No use, distribution or reproduction is permitted which does not comply with these terms.
*Correspondence: Min Feng, ZmVuZ21pbjYxMkBzaW5hLmNvbQ==; Wenzhi Guo, Z3Vvd3o2NkAxNjMuY29t
†These authors have contributed equally to this work
Disclaimer: All claims expressed in this article are solely those of the authors and do not necessarily represent those of their affiliated organizations, or those of the publisher, the editors and the reviewers. Any product that may be evaluated in this article or claim that may be made by its manufacturer is not guaranteed or endorsed by the publisher.
Research integrity at Frontiers
Learn more about the work of our research integrity team to safeguard the quality of each article we publish.