- 1Center of Clinical Laboratory Medicine, Zhongda Hospital, Southeast University, Nanjing, Jiangsu, China
- 2Department of Laboratory Medicine, Medical School of Southeast University, Nanjing, Jiangsu, China
- 3Jiangsu Provincial Key Laboratory of Critical Care Medicine, Southeast University, Nanjing, Jiangsu, China
Treponema phagedenis, a human commensal spirochete, has been reported world-wide as a key factor in the pathogenesis of bovine digital dermatitis. Here we report a case of T. phagedenis sequence detection in the cerebrospinal fluid (CSF) of a patient. The patient was diagnosed with neurosyphilis, and T. phagedenis was detected as the only microorganism in his CSF by metagenomic sequencing. The patient went through a round of penicillin therapy previously (2.4 million units of Benzathine Penicillin intramuscularly once a week for three weeks) that did not resolve the symptoms; after the diagnosis of neurosyphilis he was treated with Penicillin G Sodium 4.0 million units q4h intravenous for 14 days then his symptoms resolved. To the best of our knowledge, T. phagedenis has never been reported to be detected in a human’s CSF before. This was also the first time it was detected by metagenomic next-generation sequencing. We propose that more etiological tests should be performed including culture and sequencing for more patients with syphilis, which will contribute to a deeper understanding of the pathogenicity of the spirochete.
1 Introduction
Treponema are Gram-negative, helically coiled, strictly anaerobic, or microaerophilic cells (Radolf, 1996). Fastidious growth requirements and the fragile nature of the species in the Treponema genus complicate the determination of taxonomic relationships among them and make their study difficult (Fouad, 2016; Everall and Leonor, 2017). Treponema have been frequently reported in humans, pigs, and cattle’s oral, rectal, and genital tracts with diverse roles in a variety of niches (Paster et al., 1998; Evans et al., 2012). There are 28 validated species in the genus according to the List of Prokaryotic Names with Standing in Nomenclature (Parte et al., 2020), including both commensal and pathogenic spirochetes. Pathogenic Treponema are host-associated and implicated in many diseases of humans and animals with a complicated relationship with disease etiology. Four species/subspecies of the genus are the agents of human invasive treponematoses: Treponema pallidum subsp. pallidum (syphilis), Treponema pallidum subsp. pertenue (endemic syphilis), Treponema pallidum subsp. pertenue (yaws) and Treponema carateum (pinta) (Miao and Fieldsteel, 1978; Miao and Fieldsteel, 1980). Treponema medium and Treponema denticola are implicated in the polymicrobial etiologies of human and canine periodontal disease (Umemoto et al., 1997; Dashper et al., 2011). Non-pathogenic Treponema may be part of the normal flora of the intestinal tract, the oral cavity, or the genital tract of humans and animals. For example, Treponema rectale has been reported to be isolated from the bovine rectum (Staton et al., 2017), while Treponema peruense is a commensal spirochete isolated from human feces (Belkhou et al., 2021), and Treponema ruminis has been isolated from the rumen of cows (Newbrook et al., 2017). T. phagedenis used to be isolated as well as other spirochetes from human skin lesions and the genital tract during the original pursuit of the syphilis agent (Noguchi, 1912; Fukunaga et al., 1992). T. phagedenis was well studied by comparison with T. pallidum and was considered non-pathogenic for human beings (Moskophidis and Müller, 1984; Thomas et al., 1988). Although the species ‘Treponema phagedenis’ has been known for more than 100 years, the name was not validly published until 2020. Kuhnert et al. proposed the valid species nomenclature on the base of phenotypic and genotypic features of T. phagedenis isolates from bovine and humans (Kuhnert et al., 2020). Their results also indicated that the sequence of T. phagedenis genomes originating from bovine and humans were highly conserved; only slight variations can distinguish isolates from different host sources. As an accepted key agent in the pathogenesis of bovine digital dermatitis, a widespread infectious foot condition of economic and animal welfare importance, T. phagedenis has been reported many times over the years (Rosander et al., 2011; Espiritu et al., 2020; Khemgaew et al., 2021). T. phagedenis has never been detected from the central nervous system (CNS) (using any method). We observed the first detection of T. phagedenis from a patient’s cerebrospinal fluid, and this was also the first time that the pathogen was detected by metagenomic next-generation sequencing (mNGS). The patient was clinically diagnosed with neurosyphilis (tabes dorsalis). T. phagedenis was the only microorganism identified in the patient’s CSF by mNGS.
2 Case report
The patient was a 62-year-old man with a 1.5 year history of numbness in both lower limbs and a positive serological test result for syphilis for six months. In March 2021, he experienced anesthesia and a sore sensation in both lower limbs without any apparent cause, and the feeling worsened after bending and long walks. After 8 months, he felt weakness in both lower legs after prolonged walking. After 12 months, he attended our hospital for gradually increasing difficulty in walking and pain in both lower limbs. He was diagnosed with lumbar spinal stenosis and lumbar disc herniation by the orthopedics department. In a routine examination, his blood tests for rapid plasma reagin (RPR) test (1:4) as well as the T. pallidum particle agglutination assay (TPPA) were positive in serum. The human immunodeficiency virus (HIV) antibodies were negative (routine commercial assay). Lumbar puncture was recommended to him to exclude neurosyphilis, but he was discharged and did not follow the medical advice of going to dermatology for further treatment. After 17 months, he received 2.4 million units/week intramuscular injections of Benzathine Penicillin in his local hospital for three weeks with no significant improvement.
18 months later, he visited our hospital again. Physical examination revealed reduced pain sensation in his lower extremities and no obvious skin rash was observed. On motor examination he had positive results in the heel-knee-tibia test and Romberg’s test. Laboratory investigation showed positive serum results of RPR test (1:2) and TPPA test, normal results of antinuclear antibody, rheumatoid factor, and serum tumor markers. Nuclear magnetic resonance imaging (MRI) of the thoracic spine and cranial brain showed a slightly higher signal at the level of the posterior cord of the spinal cord in the T3-6 segment and a widening of the left parietal sulcus, which had little change compared with the results of examination 6 months previously (Figure 1).
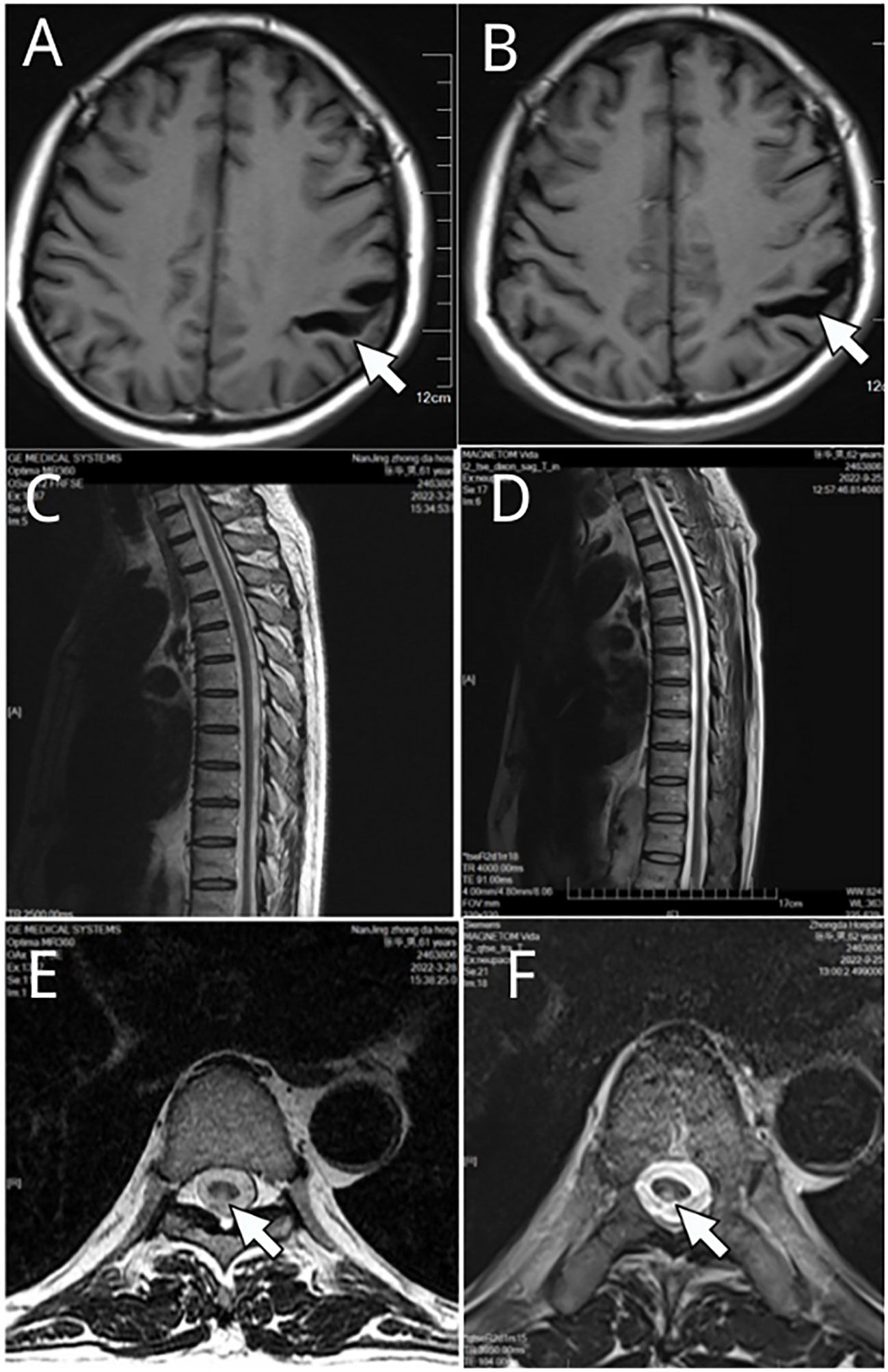
Figure 1 Nuclear magnetic resonance imaging (MRI) of the thoracic spine and cranial brain. (A, C, E) were results in Mar 2022, and (B, D, F) were examination results in Sep. 2022. A widening of the left parietal sulcus was observed in (A, B) (white arrow indicated). Slightly higher signal at the level of the posterior cord of the spinal cord (E, F) white arrow indicated) in the T3-6 segment (C, D) are shown.
On day 3 of the admission, the patient received a lumbar puncture and the pressure of CSF was 115 mmH2O. CSF laboratory examination indicated elevated levels of protein (738.5 mg/L) and IgG index (0.89), positive results of RPR test (1:1) and TPPA test. The level of glucose, chlorides, and cells of CSF was normal. Metagenomic next-generation sequencing (MGISEQ 2000 platform, MGI Tech CO., Ltd, ShenZhen, China) of the CSF was done. Sequencing of the sample generated a total of 45469988 reads, of which 79.27% were human DNA sequences. High-quality sequencing data were obtained by filtering out low-quality reads using FastP. To eliminate human genome contamination, the reads were aligned to the GRch38 human reference genome using the Burrows-Wheeler-Alignment Tool (BWA). Among the remaining 2481482 reads, 2067412 reads were mapped to the T. phagedenis genome (CP054692.1 T. phagedenis strain KS 1 chromosome complete genome, BWA) and the number of reads stringently mapped (SMRN) to the genome in species level was 1988852. Almost all of the remaining sequence reads mapped to some environmental bacterial genomes. However no nucleic acid sequence of the reads was mapped to the T. pallidum genome, any viral genomes, any fungi genomes, and any parasite genomes. T. phagedenis was detected as the only microorganism in the CSF by metagenomic sequencing and the genome coverage rate of which was 88.53% (3329235 bp/3760559bp). The data presented in the study are deposited in the China National Center for Bioinformation (CNCB) repository, accession number CRA011061.
The patient had been given Penicillin G Sodium 4.0 million units q4h intravenous treatment for 14 days and his symptoms showed dramatic improvement. The route of transmission of the patient’s infection remains unclear. We only know that the patient’s wife had positive results of the serum RPR test (1:2) and TPPA test. After treatment, the patient was discharged from the hospital and was given Benzathine Penicillin 2.4 million units intramuscularly once a week for three weeks. The patient was followed up for one month and his symptoms had resolved. The clinical process of diagnosis and treatment of the patient is shown in the flow chart of the timeline (Figure 2).
3 Discussion
In this case the nucleotide reads of T. phagedenis in the sequencing data were assembled into 3012 scaffolds (T. phagedenis ZD2201, CNCB available in Supplementary Materials 1, 2) and the phylogenetic tree was constructed by KSNP3 (Gardner et al., 2015) based on the reference genome sequencing data of the genus Treponema available in the NCBI (National Center for Biotechnology Information) database (Figure 3). The phylogenetic tree revealed a clear separation among the Treponema species, with all strains of T. phagedenis forming a distinct lineage. The human isolates of T. phagedenis seemed to form a cluster on the phylogenetic tree that was separated from the bovine isolates. The result was in agreement with that of Clegg et al. (Clegg et al., 2016), who reported that T. phagedenis human isolates were as diverse as animal strains and were separated from the animal strains by Multi-Locus Sequence Typing (MLST) analysis.
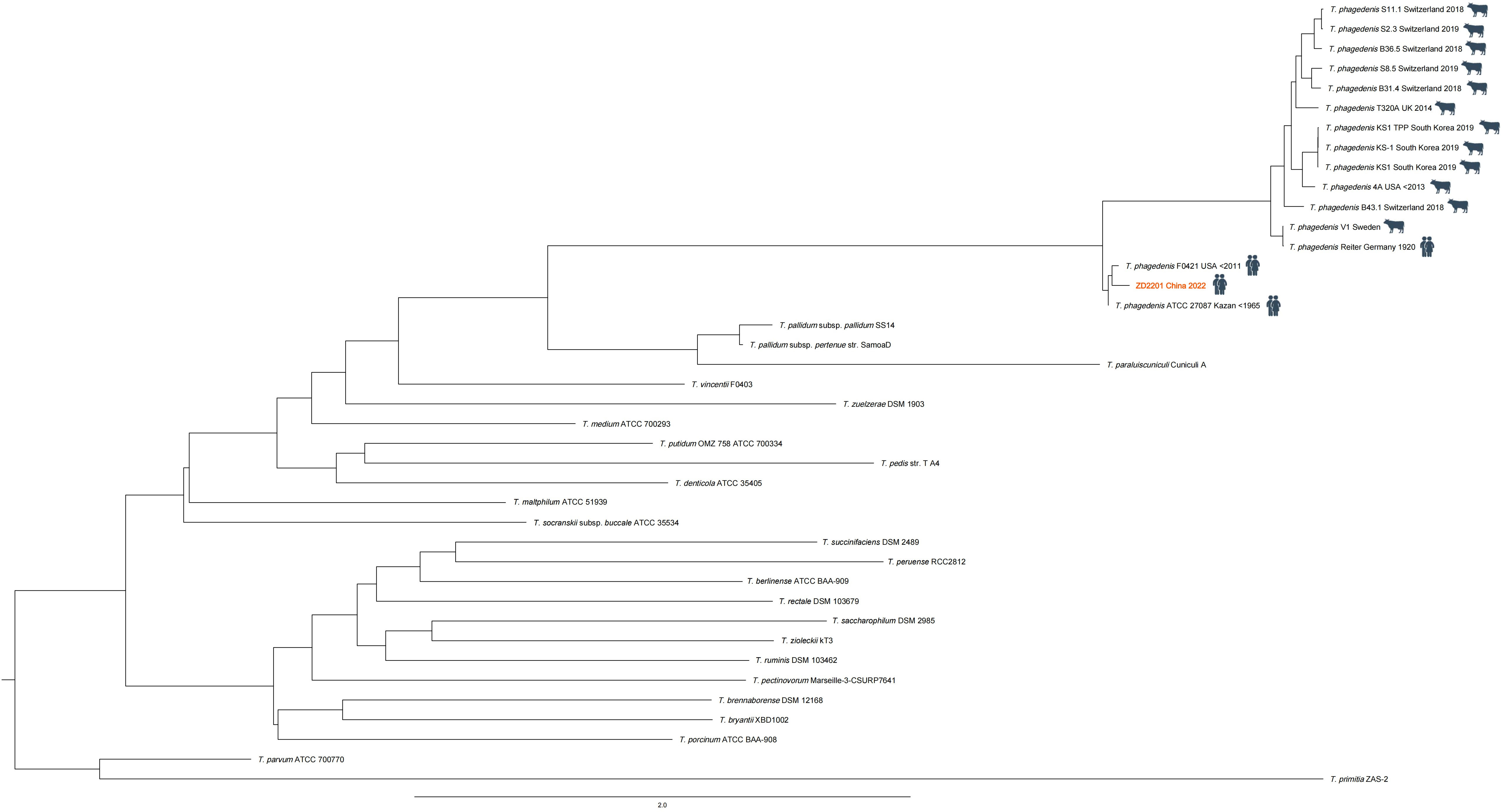
Figure 3 Phylogenetic tree constructed (by KSNP3) based on the whole genome sequencing data of the genus Treponema. Treponema phagedenis F0421 (NZ_AEFH00000000.1) was the closest relative of the T.phagedenis ZD2201 (the red font).
Unfortunately, since the patient’s CSF was not cultured anaerobically and there was no opportunity to have a second lumbar puncture it was not possible to culture T. phagedenis in this case and the pathogenicity of T. phagedenis cannot be determined. Due to the fastidiousness and almost non-culturable characteristics of T. pallidum subsp. pallidum (without tissue culture cells) (Edmondson and Norris, 2021), since there are reports now of culture, albeit difficult and not suitable for routine lab testing, etiological diagnosis of syphilis relies on syphilis serology tests, dark field microscopic examination for spirochetes and molecular biology detection techniques. The term “neurosyphilis” refers to infection of the CNS by T. pallidum, subsp. pallidum. Neurosyphilis can occur at any time after initial infection. T. phagedenis strain ATCC 27087 was isolated from a case of syphilis (Kuhnert et al., 2020) which may support the rare association of T. phagedenis and syphilis-like diseases shown by the current study. The laboratory diagnosis of neurosyphilis is based on abnormal results of serum and CSF serologic tests and on elevations in the CSF white-cell count and protein level (Ropper, 2019). However, antigen cross reactivity between species of Treponema has been reported. In 1990, Luther and colleagues reported that there was antigenic cross-reactivity between Borrelia burgdorferi, Borrelia recurrentis, T. pallidum, and T. phagedenis, although none of the borrelial immune sera tested were reactive in the Treponema pallidum Hemagglutination Assay (TPHA), anti-T. phagedenis immune serum showed a weak reaction in the TPHA (Luther and Moskophidis, 1990). In 1991, Yelton et al. found that T. phagedenis encodes and expresses homologues of the T. pallidum TmpA and TmpB proteins (Yelton et al., 1991).
Of course, despite the detection of T. phagedenis sequences in the patient’s CSF, the case has many limitations, and whether T. phagedenis is the key agent of the patient’s CNS infection has not been elucidated, and the pathogenicity of T. phagedenis remains unclear. Etiological tests including culture and sequencing are recommended for more patients with syphilis.
Data availability statement
The datasets presented in this study can be found in online repositories. The names of the repository/repositories and accession number(s) can be found in the article/Supplementary Material.
Ethics statement
Written informed consent was obtained from the individual(s) for the publication of any potentially identifiable images or data included in this article. Written informed consent was obtained from the participant/patient(s) for the publication of this case report.
Author contributions
WX collected and analyzed the data. CZ and WG took clinical care of the patient and organized the patient’s information. GW provided supervision. CY drafted the manuscript. All authors approved the final version.
Funding
This work was supported by grants from the National Science and Technology Major Project (No. 2020ZX09201015), Jiangsu Provincial Medical Key Laboratory (JSDW202240), and Jiangsu Provincial Key Laboratory of Critical Care Medicine (JSKLCCM202202015).
Conflict of interest
The authors declare that the research was conducted in the absence of any commercial or financial relationships that could be construed as a potential conflict of interest.
Publisher's note
All claims expressed in this article are solely those of the authors and do not necessarily represent those of their affiliated organizations, or those of the publisher, the editors and the reviewers. Any product that may be evaluated in this article, or claim that may be made by its manufacturer, is not guaranteed or endorsed by the publisher.
Supplementary material
The Supplementary Material for this article can be found online at: https://www.frontiersin.org/articles/10.3389/fcimb.2023.1218049/full#supplementary-material
References
Belkhou, C., Tadeo, R. T., Bacigalupe, R., Valles-Colomer, M., Chaffron, S., Joossens, M., et al. (2021). Treponema Peruense sp. nov., a commensal spirochaete isolated from human faeces. Int. J. System. Evol. Microbiol. 71 (10). doi: 10.1099/ijsem.0.005050
Clegg, S. R., Carter, S. D., Birtles, R. J., Brown, J. M., Hart, C. A., Evans, N. J. (2016). Multilocus sequence typing of pathogenic treponemes isolated from cloven-hoofed animals and comparison to treponemes isolated from humans. Appl. Environ. Microbiol. 82 (15), 4523–4536. doi: 10.1128/AEM.00025-16
Dashper, S., Seers, C., Tan, K., Reynolds, E. (2011). Virulence factors of the oral spirochete Treponema denticola. J. Dental Res. 90 (6), 691–703. doi: 10.1177/0022034510385242
Edmondson, D. G., Norris, S. J. (2021). In vitro cultivation of the syphilis spirochete Treponema pallidum. Curr. Protoc. 1 (2), e44. doi: 10.5187/jast.2020.62.6.948
Espiritu, H. M., Mamuad, L. L., Jin, S., Kim, S., Kwon, S., Lee, S., et al (2020). Genotypic and Phenotypic Characterization of Treponema phagedenis from Bovine Digital Dermatitis. Microorganisms 8 (10), 1520. doi: 10.3390/microorganisms8101520
Evans, N. J., Timofte, D., Isherwood, D. R., Brown, J. M., Williams, J. M., Sherlock, K., et al. (2012). Host and environmental reservoirs of infection for bovine digital dermatitis treponemes. Vet. Microbiol. 156 (1-2), 102–109. doi: 10.1016/j.vetmic.2011.09.029
Everall, I., Leonor, S. B. (2017). Bringing Treponema into the spotlight. Nat. Rev. Microbiol. 15 (4), 196–196. doi: 10.1038/nrmicro.2017.23
Fouad, A. F. (2016). Treponema spp. shown to be important pathogens in primary endodontic infections. J. Evidence-Based Dental Pract. 16 (1), 50–52. doi: 10.1016/j.jebdp.2016.01.007
Fukunaga, M., Okuzako, N., Mifuchi, I., Arimitsu, Y., Seki, M. (1992). Organization of the ribosomal RNA genes in Treponema phagedenis and Treponema pallidum. Microbiol. Immunol. 36 (2), 161–167. doi: 10.1111/j.1348-0421.1992.tb01653.x
Gardner, S. N., Slezak, T., Hall, B. G. (2015). kSNP3. 0: SNP detection and phylogenetic analysis of genomes without genome alignment or reference genome. Bioinformatics 31 (17), 2877–2878. doi: 10.1093/bioinformatics/btv271
Khemgaew, R., Omachi, M., Takesada, T., Vetchapitak, T., Sato, H., Taniguchi, T., et al. (2021). Transcellular penetration of Treponema phagedenis isolated from papillomatous digital dermatitis in polarized normal human epidermal keratinocytes in vitro. J. Vet. Med. Sci. 83 (6), 889–897. doi: 10.1292/jvms.21-0034
Kuhnert, P., Brodard, I., Alsaaod, M., Steiner, A., Stoffel, M. H., Jores, J. (2020). Treponema phagedenis (ex Noguchi 1912) Brumpt1922 sp. nov., nom. rev., isolated from bovine digital dermatitis. Int. J. System. Evol. Microbiol. 70 (3), 2115–2123. doi: 10.1099/ijsem.0.004027
Luther, B., Moskophidis, M. (1990). Antigenic cross-reactivity between Borrelia burgdorferi, Borrelia recurrentis, Treponema pallidum, and Treponema phagedenis. Zentralblatt für Bakteriol. 274 (2), 214–226. doi: 10.1016/S0934-8840(11)80104-0
Miao, R., Fieldsteel, A. H. (1978). Genetics of Treponema: relationship between Treponema pallidum and five cultivable treponemes. J. Bacteriol. 133 (1), 101–107. doi: 10.1128/jb.133.1.101-107.1978
Miao, R. M., Fieldsteel, A. H. (1980). Genetic relationship between Treponema pallidum and Treponema pertenue, two noncultivable human pathogens. J. Bacteriol. 141 (1), 427–429. doi: 10.1128/JB.141.1.427-429.1980
Moskophidis, M., Müller, F. (1984). Molecular characterization of glycoprotein antigens on surface of Treponema pallidum: comparison with nonpathogenic Treponema phagedenis biotype Reiter. Infect. Immun. 46 (3), 867–869. doi: 10.1128/iai.46.3.867-869.1984
Newbrook, K., Staton, G. J., Clegg, S. R., Birtles, R. J., Carter, S. D., Evans, N. J. (2017). Treponema ruminis sp. nov., a spirochaete isolated from the bovine rumen. Int. J. System. Evol. Microbiol. 67 (5), 1349–1354. doi: 10.1099/ijsem.0.001812
Noguchi, H. (1912). Pure cultivation of Spirochaeta phagedenis (new species), a spiral organism found in phagedenic lesions on human external genitalia. J. Exp. Med. 16 (3), 261–261. doi: 10.1084/jem.16.3.261
Parte, A. C., Carbasse, J. S., Meier-Kolthoff, J. P., Reimer, L. C., Goeker, M. (2020). List of Prokaryotic names with Standing in Nomenclature (LPSN) moves to the DSMZ. Int. J. System. Evol. Microbiol. 70 (11), 5607–5612. doi: 10.1099/ijsem.0.004332
Paster, B. J., Dewhirst, F. E., Coleman, B. C., Lau, C. N., Ericson, R. L. (1998). Phylogenetic analysis of cultivable oral treponemes from the Smibert collection. Int. J. System. Bacteriol. 48, 713–722. doi: 10.1099/00207713-48-3-713
Radolf, J. D. (1996). “Chapter 36 Treponema,” in Medical Microbiology, 4th ed. Ed. Baron, S. (Galveston (TX: University of Texas Medical Branch at Galveston Copyright © 1996, The University of Texas Medical Branch at Galveston).
Ropper, A. H. (2019). Neurosyphilis. New Engl. J. Med. 381 (14), 1358–1363. doi: 10.1056/NEJMra1906228
Rosander, A., Guss, B., Frykberg, L., Björkman, C., Näslund, K., Pringle, M. (2011). Identification of immunogenic proteins in Treponema phagedenis-like strain V1 from digital dermatitis lesions by phage display. Vet. Microbiol. 153 (3-4), 315–322. doi: 10.1016/j.vetmic.2011.06.005
Staton, G. J., Newbrook, K., Clegg, S. R., Birtles, R. J., Evans, N. J., Carter, S. D. (2017). Treponema rectale sp. nov., a spirochete isolated from the bovine rectum. Int. J. System. Evol. Microbiol. 67 (7), 2470–2475. doi: 10.1099/ijsem.0.002051
Thomas, D. D., Navab, M., Haake, D. A., Fogelman, A. M., Miller, J. N., Lovett, M. A. (1988). Treponema pallidum invades intercellular junctions of endothelial cell monolayers. Proc. Natl. Acad. Sci. 85 (10), 3608–3612. doi: 10.1073/pnas.85.10.3608
Umemoto, T., Nakazawa, F., Hoshino, E., Okada, K., Fukunaga, M., Namikawa, I. (1997). Treponema medium sp. nov., isolated from human subgingival dental plaque. Int. J. System. Bacteriol. 47 (1), 67–72. doi: 10.1099/00207713-47-1-67
Keywords: Treponema phagedenis, cerebrospinal fluid, neurosyphilis, case report, etiological tests
Citation: Yuan C, Xu W, Zhao C, Gao W and Wu G (2023) Case Report: Detection of Treponema phagedenis in cerebrospinal fluid of a neurosyphilis patient by metagenomic next-generation sequencing. Front. Cell. Infect. Microbiol. 13:1218049. doi: 10.3389/fcimb.2023.1218049
Received: 06 May 2023; Accepted: 11 August 2023;
Published: 29 August 2023.
Edited by:
Giuseppe Celenza, University of L’Aquila, ItalyReviewed by:
Michael John Calcutt, University of Missouri, United StatesCasey Chen, University of Southern California, United States
Copyright © 2023 Yuan, Xu, Zhao, Gao and Wu. This is an open-access article distributed under the terms of the Creative Commons Attribution License (CC BY). The use, distribution or reproduction in other forums is permitted, provided the original author(s) and the copyright owner(s) are credited and that the original publication in this journal is cited, in accordance with accepted academic practice. No use, distribution or reproduction is permitted which does not comply with these terms.
*Correspondence: Guoqiu Wu, MTAxMDA4NDA0QHNldS5lZHUuY24=
†These authors share first authorship