- 1GSK Vaccines Institute for Global Health (GVGH) S.r.l., Siena, Italy
- 2Department of Life Sciences, Imperial College London, London, United Kingdom
Shigella is a major global pathogen and the etiological agent of shigellosis, a diarrheal disease that primarily affects low- and middle-income countries. Shigellosis is characterized by a complex, multistep pathogenesis during which bacteria use multiple invasion proteins to manipulate and invade the intestinal epithelium. Antibodies, especially against the O-antigen and some invasion proteins, play a protective role as titres against specific antigens inversely correlate with disease severity; however, the context of antibody action during pathogenesis remains to be elucidated, especially with Shigella being mostly an intracellular pathogen. In the absence of a correlate of protection, functional assays rebuilding salient moments of Shigella pathogenesis can improve our understanding of the role of protective antibodies in blocking infection and disease. In vitro assays are important tools to build correlates of protection. Only recently animal models to recapitulate human pathogenesis, often not in full, have been established. This review aims to discuss in vitro assays to evaluate the functionality of anti-Shigella antibodies in polyclonal sera in light of the multistep and multifaced Shigella infection process. Indeed, measurement of antibody level alone may limit the evaluation of full vaccine potential. Serum bactericidal assay (SBA), and other functional assays such as opsonophagocytic killing assays (OPKA), and adhesion/invasion inhibition assays (AIA), are instead physiologically relevant and may provide important information regarding the role played by these effector mechanisms in protective immunity. Ultimately, the review aims at providing scientists in the field with new points of view regarding the significance of functional assays of choice which may be more representative of immune-mediated protection mechanisms.
Introduction
Shigellosis is a diarrhoeal disease caused by a bacterial infection of the colonic epithelium that causes great morbidity and mortality, with >200.000 global annual deaths especially in Africa and Asia (Khalil et al., 2018; Libby et al., 2023). Children younger than 5 years, in particular if not breastfed and malnourished, and adults older than 70 are at higher risk of severe disease and death (World Health Organization, 2005; Khalil et al., 2018). Shigellae spp. are reported to be highly invasive in humans, their only natural host; indeed ingesting as little as a few dozen of bacteria is sufficient to cause disease (DuPont et al., 1989). Transmission typically occurs via the faecal-oral route by exposure to infected feces, via person-to-person contact, ingestion of contaminated food and water, and contact with contamined fomites (Carayol and Tran Van Nhieu, 2013; Kotloff et al., 2018). In the last decades sexual contact has become an important transmission route of infection at the origin of several outbreaks in high-income countries (Kotloff et al., 2018; Charles et al., 2022). Typical treatment forsees the use of first-line and second-line antibiotic cycles (Kotloff et al., 2018), and anti-microbial resistance is rising dramatically (Organization, W.H, 2017).
The etiological agents of shigellosis are four Gram-negative Shigella species, S. flexneri, S. sonnei, S. dysenteriae, and S. boydii, typically classified in serotypes depending on the structure of the O-antigen (OAg), the polysaccharidic portion of the lipopolysaccharide (LPS) (Liu et al., 2008). Shigella shares extended genomic relatedness with E. coli (Lan and Reeves, 2002). During evolution, Shigella acquired essential virulence genes via an extra invasion plasmid encoding for factors enhancing its invasive capacity (Yang et al., 2005). Among these, the structural components, regulators, and effectors of the type three secretion system (T3SS) are essential for host infection, generating a needle-like structure that mediates cell entry (Yang et al., 2005).
Available seroepidemiological studies indicate that S. flexneri and S. sonnei are the most prevalent species, and according to the latest available data five S. flexeneri serotypes alone (2a, 6, 3a, 2b, and 1b) are responsible for more than half of all infections in low- and middle-income countries (LMICs) (Kotloff et al., 1999; Livio et al., 2014). Those, together with S. sonnei could count for the vast majority of Shigella cases worldwide (Livio et al., 2014). Anyhow, current numbers are probably underestimated, and studies are urgently needed, especially differentiated by region (Baker and The, 2018).
The important global burden of shigellosis, together with the emergence of antibiotic resistant Shigella prompts the urgent development of new preventive and treatment measures (Lefevre et al., 2023). Shigella vaccine discovery has been prioritized by the immunization, vaccines, and biologicals department of the World Health Organization (WHO), with the rationale that vaccination would decrease morbidity and mortality associated to shigellosis, as well as the use of antibiotics inducing antimicrobial resistance (AMR) (World Health Organization, 2017). Despite prolonged efforts, no licenced vaccine against shigellosis is currently available, and vaccine candidates demonstrated only partial protection against single serotypes (Mel et al., 1968; Passwell et al., 2010; Pasetti et al., 2020; MacLennan et al., 2022).
Pathogenesis of shigellosis
Shigella infects the colonic epithelium, causing ulcers in the mucosa, and acute inflammation with recruitment of inflammatory cells (Mathan and Mathan, 1991; Perdomo et al., 1994; Schroeder and Hilbi, 2008; Marteyn et al., 2012; Kotloff et al., 2018). The molecular pathogenesis of shigellosis is a complex multistep process, still not fully elucidated today. Shigella evolved great tolerance to the extreme conditions of the gastrointestinal tract, and integrates an array of environmental cues, e.g., bile, to increase its survival and prime its invasive phenotype (Marteyn et al., 2012; Sistrunk et al., 2016; Nickerson et al., 2017; Tinevez et al., 2019; Chiang et al., 2021). To complete its infection cycle and spread in the environment, Shigella needs to gain access to the intracellular compartment at the colonic level. The primary route of entry of Shigella was identified in microfold cells (M cells), specialized cells that constitutively translocate material from the intestinal lumen to the basolateral side, to be sampled by the underlying organized gut-associated lymphoid tissue (Wassef et al., 1989; Mathan and Mathan, 1991; Perdomo et al., 1994; Sansonetti et al., 1996). Shigella reaching the subepithelial compartment escape macrophage phagocytosis and interacts with the basolateral surface of the epithelium, triggering its reuptake (Sansonetti et al., 1996; Cossart and Sansonetti, 2004). The idea of Shigella directly invading enterocytes has been traditionally neglected, since Shigella is not very efficient in infecting epithelia in both in vitro (Mounier et al., 1992) and in vivo models (Perdomo et al., 1994). However, a recent study using an Intestine-Chip model, which mimics the mechanical forces of shear stress and peristalsis found in the human intestine, demonstrated that low bacterial doses can significantly infect epithelial cells from the apical side. These findings challenge the idea that M cells are the only entry point for Shigella, and suggest current epithelial models could be oversimplified and not representative (Grassart et al., 2019).
It has been reported that adhesion of Shigella is regulated by environmental conditions, such as the presence of bile salts, which need to be reproduced in in vitro models during investigation (Faherty et al., 2012; Sistrunk et al., 2016; Chanin et al., 2019). Furthermore, the role of both classical (Chanin et al., 2019) and new adhesins has been described, such as IcsA (Brotcke Zumsteg et al., 2014), MaM (Mahmoud et al., 2016), and T3SS-secreted OspE1/OspE2 proteins (Faherty et al., 2012), but the regulation of their adhesion is T3SS-dependent and their deletion does not completely abrogate adhesion to cells (Faherty et al., 2012; Brotcke Zumsteg et al., 2014). It was proposed that Shigella could enhance it adhesion by binding host molecules present in the intestinal mucosa to mediate the connection to the epithelium, such as the host defence peptide human Enteric α-Defensin (HED5). Interestingly, this hypothesis could partially explain Shigella human host specificity (Xu et al., 2018). The adhesins required for Shigella attachment to the colonic epithelium may serve as ideal targets for vaccine development, but so far none of these targets has been tested in clinic as a vaccine.
Upon cell entry Shigella escapes the endosome and spreads to neighbouring epithelial cells, minimizing exposure to the extracellular milieu and immune cells (Wassef et al., 1989; Rey et al., 2020). During the cytosolic phase, Shigella replicates and spreads to adjacent epithelial cells via an action-based motility mechanism mediated by the outer membrane protein IcsA (or VirG) (Fukumatsu et al., 2012). Briefly, Shigella takes control of the host’s cytoskeleton, polymerizing actin filaments at one pole to propel itself (Bernardini et al., 1989). The push on the cell membranes forms outward pseudopodia-containing bacteria that extend into neighbouring cells. Recipient cells eventually engulf double-membraned compartments and release the bacterium in the cytosol (Fukumatsu et al., 2012).
Historically the induction of uptake by cells and endosome escape has been described to be mediated by the T3SS. The T3SS is a needle-like structure that pierces the host cell membrane and delivers effectors such as invasion plasmid antigen (Ipa) proteins in the host cell cytoplasm to modulate cell function and favour invasion. The extracellular domains of the needle and needle tip complex (Muthuramalingam et al., 2021) are involved in host-sensing and may represent important targets for antibodies. Briefly, the needle body is composed of MxiH, and a pentamer of IpaD sits on top of the needle to avoid premature translocation (Epler et al., 2012; Muthuramalingam et al., 2021). Upon environmental cues identified in bile salts and particularly deoxycholate, IpaD induces recruitment of IpaB to the needle tip complex (Olive et al., 2007; Stensrud et al., 2008; Dickenson et al., 2011; Barta et al., 2012; Bernard et al., 2017). Host cell sensing is probably mediated by IpaD and IpaB regulation, as IpaB deletion mutants lose their ability to invade, but it is not elucidated yet (High et al., 1992).
LPS mediates immune evasion, as it protects the bacterium from both extracellular and intracellular factors, such as extreme acidity of the gastric environment (Martinic et al., 2011), and protects from complement-mediated killing and phagocytosis by reducing opsonization (Lindberg et al., 1991; Fudała et al., 2003; Caboni et al., 2015). By modifying the length and structure of its LPS, Shigella modulates accessibility to surface proteins and thus immune sensing (Morona et al., 2003; Edwards-Jones et al., 2004; West et al., 2005; Paciello et al., 2013). The role of LPS as modulator of bacterial pathogenesis was further expanded in S. sonnei, which produces a polysaccharidic capsule with structural analogy to LPS OAg (Caboni et al., 2015). The presence of the capsule was found to greatly decrease the ability of S. sonnei to invade HeLa cells by masking relevant critical virulence proteins for invasion e.g., IpaB. On the other hand, the capsule increases resistance to complement-mediated killing and the ability to spread in a rabbit model (Caboni et al., 2015). Whether LPS has a specific role in the invasion process is not fully clarified. Studies in in vitro models suggest that LPS may not be necessary for cell entry as ΔOAg strains of S. sonnei and S. flexneri are routinely -used to invade HeLa and other cell types (Okamura and Nakaya, 1977). Work on polarized epithelia showed that LPS may enhance adherence and invasion via the basolateral membrane (Kohler et al., 2002). This is in line with the observation that mutants lacking the OAg are less virulent than their wild-type counterparts, as shown in a guinea pig model of keratoconjunctivitis (Okamura and Nakaya, 1977). Overall, the fact that naturally acquired protection against Shigella is serotype specific testifies the fundamental role of LPS as a fitness factor of Shigella (Cohen et al., 2019).
The role of antibodies in fighting shigellosis
The use of antibody-based biologics against Shigella is supported by epidemiological studies showing that the natural immunity of adults and children living in Shigella-endemic countries protects from disease or reduces severity (Ndungo and Pasetti, 2020). Placentally-transferred IgG shields infants from infection during the first months of life (Thompson et al., 2016; Chisenga et al., 2021; Ndungo et al., 2021). High levels of Shigella-specific secretory IgA (sIgA) against virulence plasmid-associated antigens in maternal milk confer partial protection (reviewed in (McGuire et al., 2020)), and even predicted symptom status in a cohort of Shigella-infected infants (Hayani et al., 1992). Its proposed mechanism of action was investigated in a rabbit ileal loop infection model and was attributed to the ability of the antibodies to reduce the invasion (“immune exclusion”) of the colonic epithelium and to the downregulation of the detrimental inflammatory response (Boullier et al., 2009).
LPS, the most abundant surface antigen of Shigella, has been identified as potential protective antigen during epidemiological as well as clinical studies (Cohen et al., 2019). Evidence has been accumulated that serum IgG antibodies against Shigella LPS can be a mechanistic immunological correlate of protection (Plotkin, 2020). Recently a re-analyses of serologic and vaccine efficacy data from two trials with Shigella sonnei glycoconjugate in adults and children aged 1–4 years in Israel confirmed the association between anti–S. sonnei LPS antibodies with vaccine efficacy (Cohen et al., 2023).
Subunit vaccine candidates (extensively reviewed in (MacLennan et al., 2022)) are based on different technologies to display OAg to the immune system, ranging from classical conjugates (Taylor et al., 1993), to synthetic carbohydrates conjugated to carrier protein TT (Cohen et al., 2021), to bioconjugates to recombinant exotoxin A of Pseudomonas aeruginosa (rEPA) (Clarkson et al., 2021; Martin and Alaimo, 2022), to delivery system represented by outer membrane vesicles (called Generalized Modules for Membrane Antigens - GMMA) purified from genetically modified Shigella (Micoli et al., 2022). Unfortunately, because of Shigella OAg hypervariability, a combination of OAg from different serotypes is needed to induce broad protection against shigellosis, despite a certain level of cross-reactivity among S. flexneri OAg, which could result in cross-protection (Citiulo et al., 2021). Antibodies against Shigella proteins, including virulence factors such as Ipa, are also present in the serum of infected subjects (Oaks et al., 1986; Pasetti et al., 2020). Compared to hypervariable LPS, protein antigens present the advantage of being more conserved across Shigella spp. High anti-T3SS protein titres were found in cord blood of infants and naturally infected people, suggesting a protective role (Ndungo et al., 2018; Pasetti et al., 2020; Ndungo et al., 2021). Vaccine approaches targeting Shigella proteins have been attempted e.g., against outer membrane protease IcsP (Kim et al., 2015), however in vaccines currently in clinical development protein antigens are combined with LPS (e.g., containing IpaB and IpaD proteins complexed with S. flexneri 2a LPS (Turbyfill et al., 2022)).
Serological assays for Shigella
This review is focused on the dissection of the systemic humoral response against Shigella by the use of serological assays to quantify antigen-specific antibody levels and their functionality. Beyond quantification of antibodies, functional assays are serological methods that enable to measure in vitro the ability of antibodies to neutralize or inhibit key effector functions of the pathogenicity of Shigella. The dissection of mucosal humoral response is out of the scope of this review, nonetheless, some serological assays described below (e.g., binding assays, adhesion/invasion inhibition assay) could be applied to secretions of the gastrointestinal tract, such as feces or rectal swabs, to provide information about mucosal antibodies.
The most widely used assay to determine the specificity of antibody binding to single antigens and quantify their abundance in serum or other samples is represented by the enzyme linked immunosorbent assay (ELISA). Briefly, antigen-coated plates are incubated with the sera of interest and antibody binding is revealed though binding of a secondary antibody directed against the host species of the primary antibody, conjugated with enzymes (e.g., alkaline phosphatase) to amplify the signal with the substrate of interest. The ELISA is very simple, can be automated to increase throughput and operator independency, and can be easily transferred to different labs including those with low level of technology. Flow cytometry is instead commonly used to assess serum binding to whole Shigella instead of single antigens (Mancini et al., 2023).
Multiplex technologies (e.g., Luminex or MesoScale) offer the extra option to interrogate up to dozens of antigens simultaneously, enabling the operator to investigate quantity, specificity, and cross-reactivity of the serum against several antigens in parallel. Throughput wise, ELISA and Luminex assays can be developed in 384-well plates format, granting a high throughput with low quantity of sample needed to perform the assay. Considering the high number of Shigella OAg types, multiplexed immunoassays provide the most convenient and sample-saving method to screen for multiple OAg in naturally infected as well as vaccinated individuals. A 6-plex panel containing IpaB, IpaC, IpaD and purified LPS from S. sonnei, S. flexneri 2a, and S. dysenteriae has been published where Luminex titers were confirmed to correlate with ELISA (Kaminski et al., 2013).
Antigen expression/extraction, purification, and modification for coating or coupling purposes inevitably introduce changes compared to natural antigens. Among the different key antigens important for shigellosis pathogenesis, LPS (containing the serotypical OAg) purified by bacteria using hot phenol extraction was chosen as a consensus coating antigen in immunological assays to determine antibody titres against different serotypes of Shigella (Hayani et al., 1992).
Additional analyses better define the class/subclasses profile and the affinity/avidity of the antibodies. With antibodies being complex molecules, functionality results from the contribution of Fab-related attributes (e.g., targeted epitope, affinity, and avidity) as well as Fc-mediated effector functions (e.g., interaction with immune cell receptors, host cell-signalling, antigen presentation, initiation of the complement cascade). Therefore, functional assays should be integrated with assays designed to characterize and dissect above-mentioned relevant attributes of the immune response. Antibody subclasses in particular could predict at least in part antibody functionality, as the effector function is regulated at the level of the tail region of immune-complexed antibodies by protein differences and glycosylation (Wang and Ravetch, 2019). Experts in the field agree that the humoral response deserves a more in-depth characterization through the use, among others, of functional assays, usually serum bactericidal assay (SBA) or opsonophagocytic killing assay (OPKA) (Kaminski et al., 2019).
Functional assays in shigellosis
To evaluate the protection induced by vaccine formulations following active and/or passive immunization at the preclinical level, challenge models in animals are often used. Since Shigella is a human-restricted pathogen, animal infection models currently developed to assess the potential protective efficacy of candidate vaccines do not mirror well human infections (Lederer et al., 2005; Brunner et al., 2019). Proposed small animal challenges include guinea pig model (intrarectal route) (Shim et al., 2007), keratoconjunctivitis in guinea pigs, rabbits, or mice (Sereny test) (Sereny, 1957), rabbit ligated ileal loop model (injection) (Sansonetti et al., 1983; Wassef et al., 1989; West et al., 2005; Medeiros et al., 2020), mouse pulmonary model (intranasal route) (Mallett et al., 1993), and mouse peritoneal model (Yang et al., 2014). The group of R. Guerrant developed a murine model of diarrhoea that allowed the oral administration of bacteria, but with a considerable inoculum of 108 bacteria per mouse (PH et al., 2019; Medeiros et al., 2020). Finally, Mitchell and colleagues developed an oral infection mouse model in NAIP–NLRC4 inflammasome deficient mouse that become susceptible to shigellosis, however infection is only possible prior to clearance of the microbial flora via streptomycin treatment, possibly altering the first steps of the invasion process (Mitchell et al., 2020). Eventually, testing candidate Shigella vaccines in humans seems the most valuable way to assess their efficacy, by controlled human infection models (CHIMs) (Frenck et al., 2020; Frenck et al., 2021; Talaat et al., 2021). However, CHIMs are usually conducted on naïve, adult subjects rather than in infants in LMICs, thus their immune reponse might not be representative of the target population. Upon identifying a protective immune response, this must be dissected not only qualitatively, but also functionally to identify surrogates of protection. This evaluation must necessarily rely on functional assays (McArthur et al., 2017).
In vitro assays evaluating antibody functionality are key to better characterize the features of the elicited humoral response, aiding vaccine development. In contrast to methodologies evaluating antibody quantity, or simply binding to bacteria like flow cytometry, functional assays mimic the ability of the host immune response to counteract infection mechanisms. Indeed, different effector functions of antibodies might act in specific niches of the host or certain steps of the lifecycle of the infection, with a pathogen that can modulate the expression of antigens as a mechanism to infect and in turn evade the host response.
Functional assays can be also aimed at measuring antibody-mediated immunological effector functions, such as phagocyte-mediated bacterial killing or complement-mediated lysis. The correlation between the results of these functional assays and favourable clinical outcomes in Shigella CHIMs or clinical trials has been reviewed extensively by Ndungo & Pasetti (Ndungo and Pasetti, 2020) and by the group of G. Alter (Bernshtein et al., 2022). Here we present an overview of the functional assays used in Shigella vaccine development. To facilitate the reading, in Figure 1 we report a simplified protocol for each main group of functional assays described below: serum bactericidal assay (SBA), opsonophagocytic killing assay (OPKA), and adhesion/invasion inhibition assay (AIA). In Figure 2 we represent the physiological relevance of each functional assay.
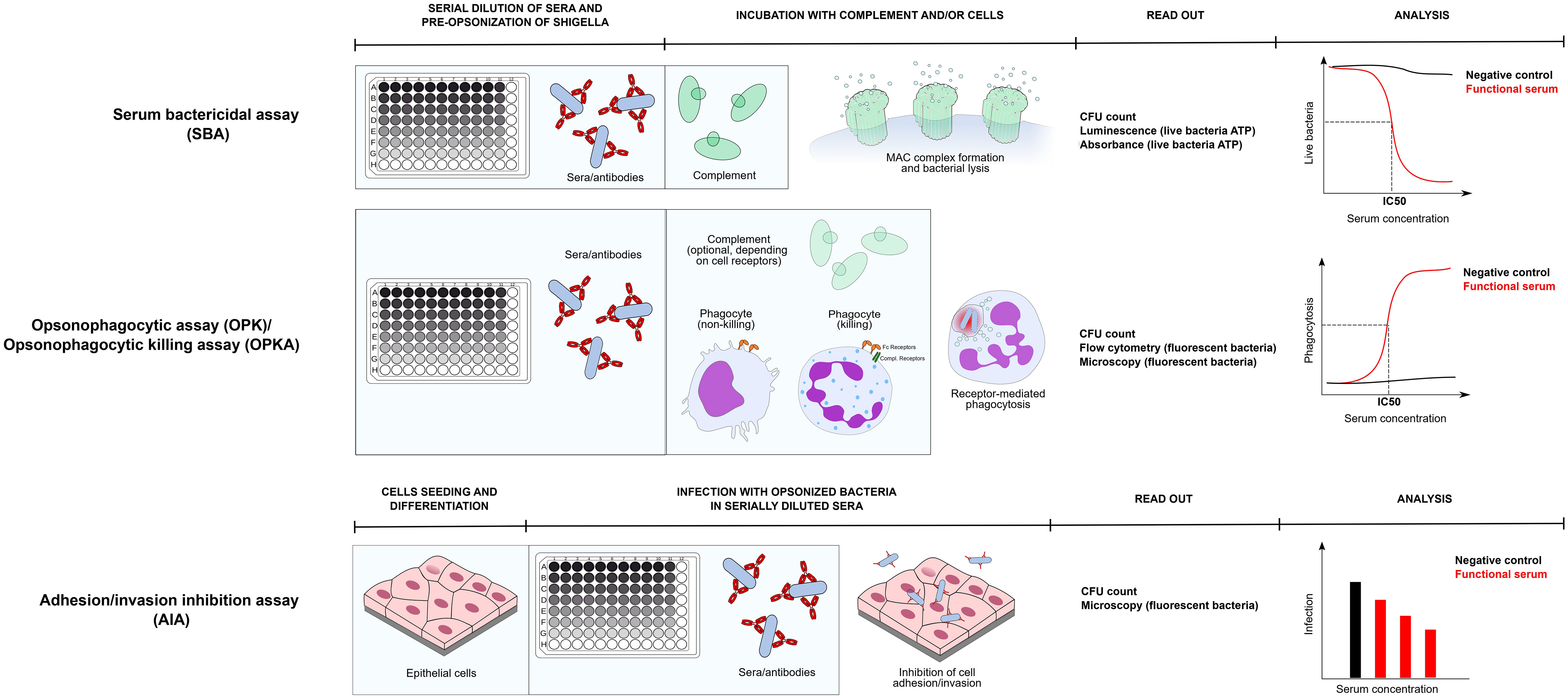
Figure 1 Assay protocol of three main functional assays to dissect antibody responses against Shigella: SBA, OPKA, AIA. Protocols of each assay is represented in its main steps. All assays are assumed to be prepared in a 96 well format. Serum Bactericidal Assay - During SBA and OPA/OPKA sera are first serially diluted in the plate and incubated with bacteria (preopsonization phase). Subsequently an exogenous complement source is added and incubated for a time ranging from 15 minutes to 3 hours. During SBA bacterial lysis occurs, which can be verified by means of CFU count or by quantifying ATP of live bacteria via luminometric or colorimetric methods. Bactericidal activity of serum is represented as the quantification live bacteria plotted against serum concentration and the 50% inhibitory concentration is calculated. Opsonophagocytosis assay/Opsonophagocytic killing assay - In OPA/OPKA opsonized bacteria with serially diluted sera are incubated together with phagocytic cells. Depending on the phagocytic receptors profile of the phagocyte (Fc receptors + complement receptors), complement may or may not be included. Depending on the cell type and its differentiation stage, phagocytes only uptake bacteria, or also kill them in the phagolysosome. Uptake and clearance of bacteria are commonly monitored by CFU count, usually upon cell lysis, or by monitoring of engulfed fluorescent bacteria by flow cytometry and/or fluorescence miscroscopy. Quantification of phagocytosis is plotted against serum concentration and similarly to SBA an IC50 value is extrapolated. Adhesion/invasion inhibition assay – the epithelial cell model of choice is prepared, possibly in a 96 well plate format. Subsequently, preopsonized bacteria are added to the cells for infection and excess bacteria are removed by washing. Adhesion/invasion are evaluated by CFU count or by fluorescence microscopy and values are usually represented as the number of infecting bacteria or percentage of infecting bacteria compared to negative control against serum concentration.
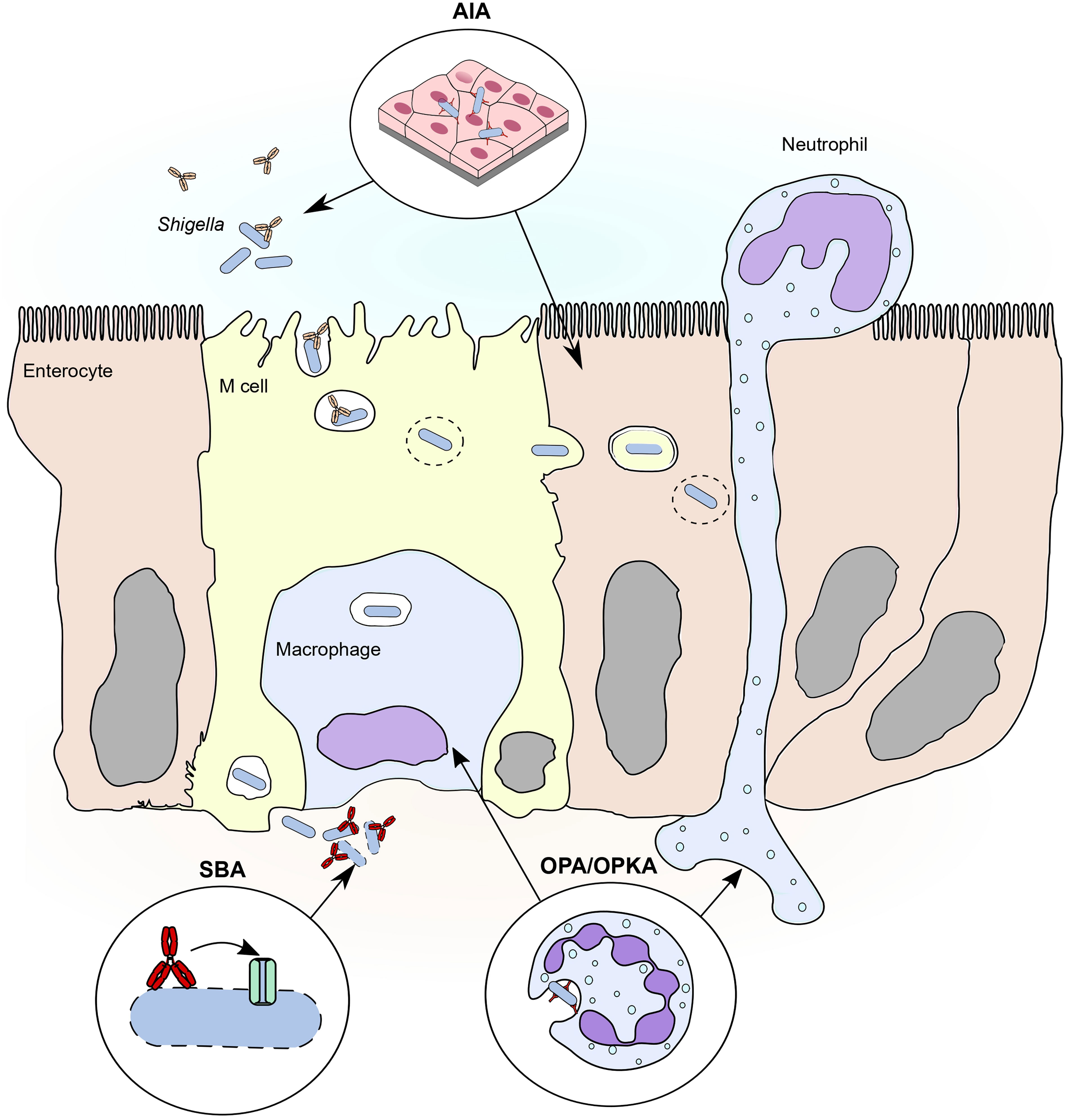
Figure 2 Major functional assays to evaluate humoral response against shigellosis in relation to steps of the pathogenesis. Three main functional assay approximate three important steps of the pathogenesis of shigellosis. 1) Adhesion/invasion inhibition assay (AIA). The adhesion/invasion inhibition assay reproduces the first phase of infection, in which Shigella approaches the colonic epithelium from the intestinal lumen, possibly via engulfed by M cell endosomes. Adhesion assays evaluate the role of mucosal antibodies in neutralizing relevant invasion factors that mediate contact of Shigella with M cells and/or epithelial cells. Invasion cells also evaluate the role of antibodies in inhibiting endosome escape and the spreading of Shigella from cell to cell in the epithelium. 2) Serum bactericidal assay (SBA). Serum bactericidal assay reproduces the conditions in which Shigella, once reached the basolateral side of the epithelium, is opsonized by complement proteins. Complement opsonins deposit on the membrane forming a pore that eventually lyses the bacterium, possibly controlling spreading and reinfection from the basolateral side. Antibodies enhance compement deposition, thus enhancing complement-mediated lysis of Shigella and their contribution can be investigated by serum bactericidal assay. 3) Opsonophagocytic killing assay (OPKA). During infection Shigella interacts with two major phagocytic cell types: neutrophils, recalled to the site of infection from the from the circulation, and resident macrophages. Opsonophagocytic killing assays approximate the interaction between phagocytes and Shigella and predict the role of antibodies in inducing efficient uptake and clearance of the bacteria.
Serum bactericidal assays
Serum bactericidal assays (SBA) evaluate the functionality of opsonizing antibodies by measuring complement-mediated lysis of Gram-negative bacteria such as Shigella spp. Antibodies of relevant subclasses binding the bacterial surface spark the classical and alternative pathways of complement activation, depositing complement elements that culminate into the assembly of a lytic pore (BOX1). SBA is the most widely functional assay used to measure the functionality of antibodies against Gram-negative bacteria by vaccine developers (Holst et al., 2003; Borrow et al., 2005; Plotkin and Gilbert, 2012; Boyd et al., 2014; Rossi et al., 2020; Micoli et al., 2021). Indeed, SBA is routinely used to dissect the humoral response against Shigella spp., either from naturally exposed individuals, challenged, or vaccinated patients (Kaminski et al., 2019; Bernshtein et al., 2022; Kapulu et al., 2022). High SBA titres have been associated with some degree of protection from moderate to severe disease in several studies, suggesting that SBA may be a functional correlate of protection recapitulating a positive role of antibodies during the pathogenesis of Shigella (Shimanovich et al., 2017; Clarkson et al., 2020). Bactericidal activity is mainly attributed to anti-LPS/OAg antibodies, probably since LPS provides abundant and repetitive surface antigens that favour antibody hexamerization and thus complement deposition (Rossi et al., 2020; Mancini et al., 2022).
The role of bactericidal activity per se in the context of the pathogenesis of shigellosis remains to be elucidated as the infection usually remains localized in the colonic mucosa and extra-intestinal complications are rare (Kotloff et al., 2018). Bacterial lysis can only occur beneath the epithelium where the extracellular matrix is relatively rich in complement and serum antibodies. SBA titres could therefore provide a useful indication of how protective antibodies could enhance Shigella complement sensitivity and ability to prevent reinfection from the basolateral side. The intestinal lumen could also be a disregarded setting of complement-mediated lysis since IgM and IgG and at least a part of complement elements was found on the intestinal surface (Sina et al., 2018; Chen et al., 2020). How SBA titres could reflect mucosal immunity in this regard is a matter of investigation.
The assay protocol forsees that bacteria are incubated with a source of opsonizing antibodies of interest, together with an exogenous complement source, most commonly baby rabbit complement (BRC) (Figure 1). Antibodies to be tested activate complement, leading to the lysis of bacteria. The outcome of the assay is usually represented as a 50% inhibitory concentration (IC50), which indicates the concentration of antibodies or serum required to kill half of total bacteria compared to the negative control. Several improvements to the classical assay have been achieved, mostly aimed at decreasing the duration of the assay and enhancing its throughput, including the miniaturization of the assay to a 384-well format (Aruta et al., 2021) and the simplification of readouts compared to plating and counting surviving bacterial colony forming units (CFU) (Nahm et al., 2018). Alternative methods based on colorimetric, luminometric and/or fluorometric readouts have converted SBA to a one-day assay. These readouts are usually derived from reactions with metabolic reagents by the live bacteria. Necchi et al. developed a luminescence-based SBA (L-SBA) protocol which detects adenosine triphosphate (ATP) production by live bacteria (Necchi et al., 2018) and the assay has been optimised and characterised (Rossi et al., 2020; Mancini et al., 2022) to analyse clinical samples from multiple studies (Frenck et al., 2021; Micoli et al., 2021; Kapulu et al., 2022). Resazurin (also known as alamarBlue) offers an alternative colorimetric and fluorometric endpoint (Romero-Steiner et al., 2004). Limitations of the assay include the fact that assay conditions need to be adjusted to each Shigella strain used depending on its intrinsic complement sensitivity, i.e., how much bacteria are lysed by spontaneous complement activation.
Box 1: classical pathway of complement activation
Complement activation culminates with the formation of pores on bacterial membranes called membrane attack complexes (MAC), ultimately lysing, and killing sensitive bacteria (Doorduijn et al., 2019).
IgM and IgG antibodies bound to epitopes on bacterial surfaces can spark local complement deposition via the classical pathway (Strasser et al., 2019; Chen et al., 2020). The cooperation of surface-bound antibodies is a prerequisite for efficient complement activation. Indeed, while IgM is already in pentameric form, pentamerization/hexamerization of IgGs determines conformational changes of their Fc portions that allow the docking of the C1 complement complex (Diebolder et al., 2014). C1 complexes comprises serine proteases C1r and C1s that start the enzymatic cascade of complement humoral proteins (Gaboriaud et al., 2014; Mortensen et al., 2017). C1s cleaves C4 into soluble C4a and the complement opsonin C4b. Surface-bound C4b-C1s2 complex cleaves C2 into C2a and C2b. C2a becomes incorporated in a complex C4bC2a, referred to as the C3 convertase, which cleaves C3 into surface-bound C3b, the central protein of the complement cascade and a phagocytic opsonin. C3b deposition quickly self-amplifies via the alternative pathway of complement activation, gaining thousands of C3b molecules on the bacterial surface in minutes. C3b and its cleavage product iC3b interact with phagocytic receptors on phagocytic cells to mediate internalization and killing. C3b interacts with both C3 convertases to form the C5 convertases (C4b2a3b/C3bBb3b), which convert soluble C5 into the anaphylatoxin C5a and C5b, the first component of the MAC complex (Heesterbeek et al., 2019; Doorduijn et al., 2020). C5b quickly interacts with C6 and then C7, the first proteins spanning the lipid bilayer. C5b-7 then recruits C8 and 18 copies of C9 forming the MAC complex, and is assembled from five complement proteins (C5b, C6, C7, C8 and multiple C9 proteins) (Heesterbeek et al., 2019; Doorduijn et al., 2020).
Phagocytosis and killing assays (OPA/OPK)
Opsonophagocytosis assays (OPA) and opsonophagocytic killing assays (OPKA) measure the ability of antibodies to mediate the internalization and/or killing of Shigella by phagocytes. Phagocytosis assays of Shigella not only offer a way to measure the ability of antibodies to engage effector immune cells but could provide clues on the pathogenesis of the disease. During infection, Shigella interacts with both resident subepithelial macrophages underneath Peyer’s patches and recruited polymorphonuclear cells (PMNs). Both cell types sense the opsonized bacteria via phagocytic receptors - Fcγ receptors (FcγR) and complement receptors (CR1 and CR3) - and eventually phagocytose and clear the pathogen (Perdomo et al., 1994; Sansonetti et al., 1996; Sansonetti, 2001; Schroeder and Hilbi, 2008). However, phagocytosis may represent a double-edged sword, as excessive inflammation amplified by phagocytic cells may have a negative outcome for the host. Indeed, Shigella manipulates the inflammatory response inducing pyroptosis of subepithelial macrophages with the release of tissue-damaging pro-inflammatory cytokines (Clerc et al., 1987; Sansonetti, 2001; Ashida et al., 2021) and necrosis of PMNs with the release of toxic granule contents, contributing to the destruction of the epithelial barrier (Francois et al., 2000). Furthermore, it was proposed that PMNs recruited to the site of infection to the lamina propria could transmigrate through the epithelial layer offering an additional route of entry as well as a destructive inflammatory milieu. The suppression of PMNs recruitment, together with the dampening of the associated pro-inflammatory cytokines in vivo, preserved epithelial integrity (Perdomo et al., 1994). These aspects of the pathogenesis of shigellosis encourage setting up and using phagocytosis assays to verify not only the uptake, but also the prompt and effective clearance of Shigella, and whether the presence of antibodies can modulate the outcome in favour of clearance and contain collateral damage.
In a phagocytosis assay protocol, phagocytic cells are incubated with opsonized Shigella for the appropriate time. Internalization and/or killing of bacteria (or surrogates of bacteria) are then evaluated via multiple possible readouts, including CFU counting of colonies (Shimanovich et al., 2017; Ndungo et al., 2021), or flow cytometry-based (Bernshtein et al., 2022) (Figure 1). Depending on the combination of cell types and opsonins used, the assay provides different indications. Non-differentiated cells like THP-1 monocytes are not able to kill and only provide indication of antibody-mediated internalization of targets. In contrast, by using more specialized cells (e.g., primary neutrophils, differentiated HL-60, differentiated THP-1 macrophages) it is possible to evaluate killing efficiency of serum (Butler et al., 2019; Mancini et al., 2023). Complement is commonly added to the assay to evaluate concerted FcγRs- and CR-mediated uptake and killing (although it is not always necessary to induce internalization and/or killing) (Mancini et al., 2023).
OPKA were already used to evaluate the functionality of clinical samples. A classical OPKA with differentiated HL-60 was first established to evaluate killing of Shigella opsonized with serum from vaccinated and challenged patients in the presence of BRC, to eventually correlate functional antibody activity and protection (Shimanovich et al., 2017). The same assay was applied to measure the functional response induced by protective maternal blood compared to the cord blood of the corresponding baby (Ndungo et al., 2021). Both OPA and OPKA were inserted in the system serology panel of functional assays, with the difference that OPKA was performed with primary human neutrophils instead of differentiated HL-60. Flow cytometry-based OPA with THP-1 monocytes and neutrophils from whole blood were used to evaluate opsonophagocytosis of beads coated with antigens of interest, and not whole Shigella (Bernshtein et al., 2022) Interestingly, while SBA and OPKA results usually correlated with each other and with respect to symptoms severity, OPA assays with specific antigens provided new insights, as contrarily to SBA and OPKA titers, OAg- and IpaB-antibodies negatively correlated with symptoms severity (Bernshtein et al., 2022). Finally, classical OPKA was used by our group to evaluate the immune response of mice immunized with candidate GMMA-based vaccine (Mancini et al., 2023).
OPA and OPKA present several difficulties, among which the tuning of complement and cell conditions, which must be finely adjusted to appreciate phagocytosis-derived killing and not serum bactericidal assay. Furthermore, when flow cytometry or fluorescence microscopy are used to acquire the experiment conditions to achieve sufficient fluorescent of each bacterial strain must be optimized.
Adhesion/invasion inhibition assays
Invasion assays have been extensively used to identify targets mediating host-pathogen interaction and define the invasion of host cells mainly through T3SS (Skoudy et al., 2000). IpaB was described to interact with HeLa surface receptor CD44 and cholesterol in the lipid rafts, while the IpaB-CD complex binds to integrin α5β1, anchoring the bacterium on the cell surface and facilitating the creation of the pore on by the T3SS (Yang et al., 2015).
Adhesion/invasion inhibition assays (AIA) were used to demonstrate the ability of antibodies, especially against Ipa proteins, to reduce virulence. Generalizing the assay protocol, in vitro cell models of variable complexity (discussed below), usually organized in a 96-well plate format, are infected with a defined number of live bacteria opsonized in solution with the antibodies of interest (Figure 1). Incubation time is adjusted depending on the desired readout: a few minutes to better appreciate adhesion, to a few hours to better appreciate invasion. Usually, excess bacteria are then rinsed off the cells by repeated washing steps. Finally, adhesion/invasion rates of bacteria can be evaluated against non-opsonized controls by two main methods: either CFU count of total or internal bacteria (upon gentamycin treatment of cells to kill external bacteria), or by immunofluorescent microscopy, possibly with a counterstain to distinguish internalized and external bacteria.
Animal anti-sera and monoclonal antibodies against IpaD (Sierocki et al., 2021), IpaB (Mills et al., 1988; Shen et al., 2010; Li et al., 2022) and IpaC (Mills et al., 1988) were demonstrated to reduce the ability of virulent bacteria to infect in in vitro cell models. These works are in line with studies in animal models in which mice immunized with IpaB and IpaD developed a protective immune response (both IgG and IgA) upon challenge (Martinez-Becerra et al., 2012), therefore demonstrating in vivo the relevance of anti-Ipa antibodies. However, neutralization of Ipa proteins could be impaired by factors such as environmental cues influencing translocation from the cytosol to the bacterial surface, transient accessibility of Ipa in the tip complex to antibody binding (Skoudy et al., 2000).
In addition, invasion assays were used to assess the importance of OAg, not only in the context of SBA, but during the infection in general. Indeed, Chowers and colleagues showed that sera from children immunized with S. flexneri 2a or S. sonnei OAg fraction of LPS inhibit the invasion of the homologous strain on the epithelial cells Caco-2 (Chowers et al., 2007). Caboni et al. also demonstrated that the presence of the OAg-homologous capsule of S. sonnei impairs invasion of HeLa cells (Caboni et al., 2015).
More complex models of differentiated epithelia include the co-culture of Caco-2 and RajiB cells (a human lymphoid cell line), to produce a mature intestinal follicle-associated epithelium with transcytosing M cells (Beloqui et al., 2017; Rey et al., 2020). This model was used to propose the M cell-to-enterocyte pathway as the major dissemination pathway for Shigella to avoid the basolateral compartment (Rey et al., 2020). 2D human intestinal enteroids (HIE) from primary tissue were used as an infection model, confirming preferential infection from M cells and the basolateral compartment (Koestler et al., 2019; Llanos-Chea et al., 2019; Ranganathan et al., 2019). HIE can be co-cultured with PMNs to study the interplay of Shigella with respect to PMN-epithelial cell interaction (Lemme-Dumit et al., 2022). Finally, 3D epithelial organoids derived from primary human tissue could be an interesting model for Shigella infection, especially apical-out organoids with reversed polarity would represent another useful model to investigate invasion on the apical vs. the basolateral side of the epithelium (Co et al., 2021).
As already mentioned, Shigella was traditionally observed to have a scarce ability to invade polarized in vitro cell models from the apical surface (Mounier et al., 1992), so much so that in many experimental models bacteria must be forced on cells by centrifugation, and Shigella mutants that express heterologous proteins to artificially enhance adhesion are used (e.g., AfaE adhesin (Skoudy et al., 2000), AfaI adhesin (Rey et al., 2020)), even in the presence of M cells. Whether the adhesion/invasion mechanism of Shigella in these cultures is faithful to the physiology of infection is a matter of debate.
The state of immunoglobulins in the intestinal lumen remains a fundamental topic to determine the ratio of function/non-functional mAbs. One example is given by the fact that mAbs are not found only as single entities in the luminal tract of the intestine, but also in complex with the secretory component (SC) (Cerutti and Rescigno, 2008). The SC is the soluble part of the polymeric Ig receptor, which is responsible for the transmigration of the IgG from the basolateral to the luminal space of the intestinal tracts (Turula and Wobus, 2018). When the IgG-polymeric Ig receptor complex is exposed in the luminal cavity, the proteases present in the digestive tract cleaves the transmembrane component of this receptor from the SC, which remains attached to the IgGs (Wei and Wang, 2021). The SC-IgG complex favors both the half-life of IgG in the luminal tract of the intestine and the effector functions of the IgG itself (Stadtmueller et al., 2016). Indeed, it was shown that only in the presence of SC an LPS-specific monoclonal antibody against Shigella flexneri exerts its protective role on epithelial cells (Mathias et al., 2013).
Contact haemolysis assay
T3SS-mediated membrane lysis, possibly by translocon-induced pore formation (Chen et al., 2021), requires contact with eukaryotic cell surface. Exploiting this property, Sansonetti and colleagues set up a contact haemolysis assay in which Shigella is incubated with sheep erythrocytes, causing the release of haemoglobin in solution, and thus providing a colorimetric readout for membrane lysis (Clerc et al., 1985; Sansonetti et al., 1986). Haemolysis was demonstrated to be T3SS-dependent and to be 1) temperature-dependent, since the expression of the T3SS is known to occur at 37°C and not at 30°C; 2) plasmid-encoded, it did not occur when non-invasive Shigella spp. were tested; 3) contact-mediated, adhesion between bacteria and erythrocytes must be induced by pelleting of bacteria on red blood cells.
Interestingly, Clerc et al. demonstrated that the amount of contact haemolysis of Shigella spp. was correlated to the ability to penetrate HeLa cells, suggesting that the contact haemolysis assay might represent a time- and cost-effective approximation of the initial steps of the lysis of the phagocytic vacuole, a key step for intracellular multiplication of the bacterium in the colonic epithelium (Clerc et al., 1985).
Since 1986, contact haemolysis assay was reprised in subsequent works to demonstrate membrane disrupting activity of virulence factors and study the role of T3SS translocons IpaBCD. To this date, only Sieroki et al. used contact haemolysis as a functional assay to demonstrate the ability of cross-reactive anti-IpaD (Shigella) -SipD (Salmonella) monoclonal antibodies to inhibit membrane lysis (Sierocki et al., 2021). Interestingly, the tested antibody (mAb IpaD-318) was found to enhance the haemolysis of erythrocytes, but not HeLa cell invasion. The authors hypothesized that mAb IpaD-318 stabilized polymerized IpaD in an intermediate conformation that lost the capacity to control correct IpaB and IpaC translocation (Sierocki et al., 2021). Antibody fragments against IpaD were also reported to significantly reduce erythrocytes haemolysis by wild-type S. flexneri (Barta et al., 2017).
Systems serology approach
No single antibody specificities, qualities, or effector functions investigated so far seem to be absolute predictors of protective immunity in shigellosis; it is thus possible that extra antibody functions and/or the integration of different aspects predict a successful humoral immunity against Shigella. The system serology approach, first developed by the group of Galit Alter in 2015, interrogates clinical serum samples via an array of different serological assays, and by means of machine learning and other high dimensional tools it then integrates the results to define the specific antibody profiles that correlate with favorable patient outcomes in various diseases, including shigellosis (Chung et al., 2015; Arnold and Chung, 2018). The profiling interrogates Fc-effector functions via functional assays and also forsees biophysical measurements (such as binding to Fcγ receptors and binding to specific antigens) (Chung et al., 2015).
Bernshtein and colleagues applied the systems serology approach to profile the sera of patients challenged with S. flexneri 2a in a CHIM (Bernshtein et al., 2022). In their study they employed the following high throughput quantitative antibody assays (Bernshtein et al., 2022): via Luminex they determined the subclass or isotype (IgG1-2-3, IgA1-2, IgM) of antibodies binding to antigens of interest (multiple LPS types, IpaD, IpaB, IpaC, IpaH, VirG). Interestingly, Fcγ receptor binding to immune-complexed antigens was strongly recommended as a possible predictor of antibody effector function, an aspect that is not routinely used in serological labs (Arnold and Chung, 2018; Bernshtein et al., 2022).
Functional assays (Complement deposition, SBA and OPA/OPK) were performed both on opsonized beads and on live Shigella. To measure complement deposition, immune complexes were incubated with baby rabbit complement to determine the ability of antibodies to start the complement cascade. To our best knowledge, this assay is also not routinely used, especially on protein targets since complement deposition on live Shigella is primarily driven by anti-LPS antibodies. However, this assay could help recapitulate useful functional aspects of antibodies with protein specificity, for example revealing that some protein targets could still be drivers of complement deposition when they are more exposed on the bacterial surface. Complement deposition on beads is combined with classical SBA on live Shigella.
At least three main and complementary functional assays were described phagocytosis: antibody-dependent neutrophil phagocytosis (ADNP) in whole blood, antibody-dependent cellular (monocyte) phagocytosis (ADCP), both performed with immune-complexed beads, and classical OPK with live Shigella (Butler et al., 2019; Karsten et al., 2019; Bernshtein et al., 2022).
Discussion
Vaccines targeting human pathogens are fundamental tools for improving global health and combating emerging antimicrobial resistance. In vitro assays evaluating antibody biophysical properties and antibody functionality are key to identifying the features of a protective humoral response, greatly aiding vaccine development. In absence of a clear indication regarding the classes or subclasses of antibodies that are relevant for protection (e.g., IgA vs. IgG) and which is the critical and validated level that correlates with clinical protection for different age groups, it is key to prove ability of antibodies to neutralise, and better to kill, the activity of a pathogen. However, functional assays are pathogen-specific and recapitulate only precise steps of the host pathogen interactions. From the technical point of view a functional assay needs to offer sufficient throughput to allow screening of multiple samples in a cost-effective manner. Therefore, the ideal functional assay balances physiological relevance, suitable throughput, and cost-effectiveness.
In this review we presented the main functional assays used to evaluate the functionality of anti-Shigella antibodies, and we discussed that each assay could approximate different stages of Shigella mechanism of infection (Figure 2). SBA titres have been proven to correlate with increased protection linked to efficient antibody-induced complement mediated killing. However, we could speculate that the valence of SBA could extend beyond bacterial lysis, indirectly providing a general indication on the efficacy of opsonization on live bacteria, which is at the heart of multiple immune defence mechanisms. It is well known that SBA titers speak with OPA/OPK titers (West et al., 2005; Brotcke Zumsteg et al., 2014), since the two mechanisms rely on antibody-dependent complement deposition which can signal to phagocytes via Fcγ receptors and phagocytic complement receptors CR1 and CR3. Complement deposition on bacteria also makes an important intracellularly defence signal that favour autophagy and clearance of intracellular pathogens (Wang and Ravetch, 2019), and SBA titers could also reflect that.
The penetration of Shigella in the colonic epithelium is arguably the most crucial step in the pathogenesis of shigellosis to be inhibited by antibodies. For this reason, functional assays measuring the capacity of antibodies to prevent adhesion and/or invasion of in vitro epithelial models are fundamental tools. Importantly, adhesion/invasion assays are the only assays simulating events occurring at the level of the intestinal lumen, opening interesting options in testing the significance of mucosal subclasses of antibodies. Despite the clear importance, no adhesion/invasion assays were implemented in clinical testing so far.
Among the difficulties in setting up such functional assay, there is identifying a relevant target/s of the antibodies to be tested. In fact, effector functions of antibodies might act in specific niches of the host or in certain steps of the lifecycle of the infection, while the pathogen can modulate the expression of antigens as mechanism to infect and in turn evade the host response. While SBA was shown to be mainly driven by antibodies targeting the LPS, the initial steps of invasion, which are still not completely elucidated, are mediated by the T3SS, possibly in combination with other virulence factors greatly increasing the complexity of the possible targets to be addressed or suggesting a synergistic effect of multiple antibodies and mechanisms during various steps of the infection process.
Expression and structural modifications of relevant surface antigens are probably modulated by environmental factors such as bile salts exposure, pH, temperature, glucose concentration and oxygen, which are thought to signal the bacterium its localization between the small and the large intestine. These factors need to be necessarily considered when standardizing bacterial growth/conditions to perform experiments, with deoxycholate and oxygen density combined with the presence of eukaryotic membranes are probably the most promising.
In conclusions, availability of a panel of multiple functional assays would be fundamental for the dissection of the immune response induced by Shigella infection or vaccination in preclinical and clinical setting, and to unravel the role played by antibodies in inducing protection against shigellosis.
Author contributions
All authors contributed to the conception and writing of the article and approve the final version of the article.
Funding
This work was funded by Wellcome Trust (grant n.219666/Z/19/Z, “Human monoclonal antibodies against Shigella (ShiMabs), for therapy and vaccine acceleration”.
Acknowledgments
We would like to acknowledge Maria Michelina Raso, Assia Duatti, Gianmarco Gasperini, Vanessa Arato, Carlo Giannelli, Miren Iturriza, and Danilo Moriel Gomez for the inspiring conversations and advice on writing this review. We thank Dr. Rino Rappuoli for coordinating the grant.
Conflict of interest
EB, GV, FM, MP, and OM are employees of the GSK group of companies. GSK Vaccines Institute for Global Health S.r.l. is an affiliate of GlaxoSmithKline Biologicals SA. FM, OR and MP report ownership of shares/share options. EB and GV are participating in a Postdoctoral fellowship funded by Wellcome Trust.
Publisher’s note
All claims expressed in this article are solely those of the authors and do not necessarily represent those of their affiliated organizations, or those of the publisher, the editors and the reviewers. Any product that may be evaluated in this article, or claim that may be made by its manufacturer, is not guaranteed or endorsed by the publisher.
Abbreviations
ADCP, Antibody-dependent cellular phagocytosis; ADNP, Antibody-dependent neutrophil phagocytosis; AIA, Adhesion/invasion inhibition assay; AMR, Antimicrobial resistance; ATP, Adenosine triphosphate; BRC, Baby rabbit complement; CFU, Colony forming units; CHIM, Controlled human infection model; CP, Classical pathway; CR, Complement receptor; ELISA, Enzyme linked Immunosorbent assay; FcγR, Fcγ receptors; GMMA, Generalized Modules for Membrane Antigens; HIE, Human intestinal enteroid; Ig, Immunoglobulin; Ipa, Invasion protein antigen; MAC, membrane attack complex; M cells, Microfold cells; LMICs, Low- and middle-income countries; LPS, Lipopolysaccharide; OAg, O-antigen; OPA, opsonophagocytosis assay; OPKA, opsonophagocytic killing assay; PMNs, Polymorphonuclear (cells); rEPA, Recombinant exotoxin A of Pseudomonas aeruginosa; SBA, Serum bactericidal assay; SC, Secretory component; sIgA, Secretory IgA; T3SS, Type 3 secretion system; WHO, World Health Organization.
References
Arnold, K. B., Chung, A. W. (2018). Prospects from systems serology research. Immunology 153 (3), 279–289. doi: 10.1111/imm.12861
Aruta, M. G., Carducci, M., Micoli, F., Necchi, F., Rossi, O. (2021). Increasing the high throughput of a luminescence-based serum bactericidal assay (L-SBA). Biotech. (Basel) 10 (3), 19. doi: 10.3390/biotech10030019
Ashida, H., Suzuki, T., Sasakawa, C. (2021). Shigella infection and host cell death: a double-edged sword for the host and pathogen survival. Curr. Opin. Microbiol. 59, 1–7. doi: 10.1016/j.mib.2020.07.007
Baker, S., The, H. C. (2018). Recent insights into shigella. Curr. Opin. Infect. Dis. 31 (5), 449–454. doi: 10.1097/QCO.0000000000000475
Barta, M. L., Guragain, M., Adam, P., Dickenson, N. E., Patil, M., Geisbrecht, B.V., et al. (2012). Identification of the bile salt binding site on IpaD from shigella flexneri and the influence of ligand binding on IpaD structure. Proteins 80 (3), 935–945. doi: 10.1002/prot.23251
Barta, M. L., Shearer, J. P., Arizmendi, O., Tremblay, J. M., Mehzabeen, N., Zheng, Q., et al. (2017). Single-domain antibodies pinpoint potential targets within shigella invasion plasmid antigen d of the needle tip complex for inhibition of type III secretion. J. Biol. Chem. 292 (40), 16677–16687. doi: 10.1074/jbc.M117.802231
Beloqui, A., Brayden, D. J., Artursson, P., Preat, V., Des Rieux, A. (2017). A human intestinal m-cell-like model for investigating particle, antigen and microorganism translocation. Nat. Protoc. 12 (7), 1387–1399. doi: 10.1038/nprot.2017.041
Bernard, A. R., Jessop, T. C., Kumar, P., Dickenson, N. E. (2017). Deoxycholate-enhanced shigella virulence is regulated by a rare pi-helix in the type three secretion system tip protein IpaD. Biochemistry 56 (49), 6503–6514. doi: 10.1021/acs.biochem.7b00836
Bernardini, M. L., Mounier, J., D'hauteville, H., Coquis-Rondon, M., Sansonetti, P. J. (1989). Identification of icsA, a plasmid locus of shigella flexneri that governs bacterial intra- and intercellular spread through interaction with f-actin. Proc. Natl. Acad. Sci. U.S.A. 86 (10), 3867–3871. doi: 10.1073/pnas.86.10.3867
Bernshtein, B., Ndungo, E., Cizmeci, D., Xu, P., Kovac, P., Kelly, M., et al. (2022). Systems approach to define humoral correlates of immunity to shigella. Cell Rep. 40 (7), 111216. doi: 10.1016/j.celrep.2022.111216
Borrow, R., Balmer, P., Miller, E. J. V. (2005). Meningococcal surrogates of protection–serum bactericidal antibody activity. Vaccine 23, 2222–2227. doi: 10.1016/j.vaccine.2005.01.051
Boullier, S., Tanguy, M., Kadaoui, K. A., Caubet, C., Sansonetti, P., Corthesy, B., et al. (2009). Secretory IgA-mediated neutralization of shigella flexneri prevents intestinal tissue destruction by down-regulating inflammatory circuits. J. Immunol. 183 (9), 5879–5885. doi: 10.4049/jimmunol.0901838
Boyd, M. A., Tennant, S. M., Saague, V. A., Simon, R., Muhsen, K., Ramachandran, G., et al. (2014). Serum bactericidal assays to evaluate typhoidal and nontyphoidal salmonella vaccines. Clin. Vaccine Immunol. 21 (5), 712–721. doi: 10.1128/CVI.00115-14
Brotcke Zumsteg, A., Goosmann, C., Brinkmann, V., Morona, R., Zychlinsky, A. (2014). IcsA is a shigella flexneri adhesin regulated by the type III secretion system and required for pathogenesis. Cell Host Microbe 15 (4), 435–445. doi: 10.1016/j.chom.2014.03.001
Brunner, K., Samassa, F., Sansonetti, P. J., Phalipon, A. (2019). Shigella-mediated immunosuppression in the human gut: subversion extends from innate to adaptive immune responses. Hum. Vaccin Immunother. 15 (6), 1317–1325. doi: 10.1080/21645515.2019.1594132
Butler, A. L., Fallon, J. K., Alter, G. (2019). A sample-sparing multiplexed ADCP assay. Front. Immunol. 10, 1851. doi: 10.3389/fimmu.2019.01851
Caboni, M., Pedron, T., Rossi, O., Goulding, D., Pickard, D., Citiulo, F., et al. (2015). An O antigen capsule modulates bacterial pathogenesis in shigella sonnei. PloS Pathog. 11 (3), e1004749. doi: 10.1371/journal.ppat.1004749
Carayol, N., Tran Van Nhieu, G. (2013). The inside story of shigella invasion of intestinal epithelial cells. Cold Spring Harb. Perspect. Med. 3 (10), a016717. doi: 10.1101/cshperspect.a016717
Cerutti, A., Rescigno, M. (2008). The biology of intestinal immunoglobulin a responses. Immunity 28 (6), 740–750. doi: 10.1016/j.immuni.2008.05.001
Chanin, R. B., Nickerson, K. P., Llanos-Chea, A., Sistrunk, J. R., Rasko, D. A., Kumar, D. K. V., et al. (2019). Shigella flexneri adherence factor expression in in vivo-like conditions. mSphere 4 (6). doi: 10.1128/mSphere.00751-19
Charles, H., Prochazka, M., Thorley, K., Crewdson, A., Greig, D. R., Jenkins, C., et al. (2022). Outbreak of sexually transmitted, extensively drug-resistant shigella sonnei in the UK, 2021-22: a descriptive epidemiological study. Lancet Infect. Dis. 22 (10), 1503–1510. doi: 10.1016/S1473-3099(22)00370-X
Chen, K., Magri, G., Grasset, E. K., Cerutti, A. (2020). Rethinking mucosal antibody responses: IgM, IgG and IgD join IgA. Nat. Rev. Immunol. 20 (7), 427–441. doi: 10.1038/s41577-019-0261-1
Chen, P., Russo, B. C., Duncan-Lowey, J. K., Bitar, N., Egger, K. T., Goldberg, M. B., et al. (2021). Topology and contribution to the pore channel lining of plasma membrane-embedded shigella flexneri type 3 secretion translocase IpaB. mBio 12 (6), e0302121. doi: 10.1128/mBio.03021-21
Chiang, I. L., Wang, Y., Fujii, S., Muegge, B. D., Lu, Q., Tarr, P. I., et al. (2021). Biofilm formation and virulence of shigella flexneri are modulated by pH of gastrointestinal tract. Infect. Immun. 89 (11), e0038721. doi: 10.1128/IAI.00387-21
Chisenga, C. C., Bosomprah, S., Simuyandi, M., Mwila-Kazimbaya, K., Chilyabanyama, O. N., Laban, N. M., et al. (2021). Shigella-specific antibodies in the first year of life among Zambian infants: a longitudinal cohort study. PloS One 16 (5), e0252222. doi: 10.1371/journal.pone.0252222
Chowers, Y., Kirschner, J., Keller, N., Barshack, I., Bar-Meir, S., Ashkenazi, S., et al. (2007). O-specific [corrected] polysaccharide conjugate vaccine-induced [corrected] antibodies prevent invasion of shigella into caco-2 cells and may be curative. Proc. Natl. Acad. Sci. U.S.A. 104 (7), 2396–2401. doi: 10.1073/pnas.0610833104
Chung, A. W., Kumar, M. P., Arnold, K. B., Yu, W. H., Schoen, M. K., Dunphy, L. J., et al. (2015). Dissecting polyclonal vaccine-induced humoral immunity against HIV using systems serology. Cell 163 (4), 988–998. doi: 10.1016/j.cell.2015.10.027
Citiulo, F., Necchi, F., Mancini, F., Rossi, O., Aruta, M. G., Gasperini, G., et al. (2021). Rationalizing the design of a broad coverage shigella vaccine based on evaluation of immunological cross-reactivity among s. flexneri serotypes. PloS Negl. Trop. Dis. 15 (10), e0009826. doi: 10.1371/journal.pntd.0009826
Clarkson, K. A., Frenck, R.W., Jr., Dickey, M., Suvarnapunya, A. E., Chandrasekaran, L., Weerts, H. P., et al, et al. (2020). Immune response characterization after controlled infection with lyophilized shigella sonnei 53G. mSphere 5 (5), e00988–e00919. doi: 10.1128/mSphere.00988-19
Clarkson, K. A., Talaat, K. R., Alaimo, C., Martin, P., Bourgeois, A. L., Dreyer, A., et al. (2021). Immune response characterization in a human challenge study with a shigella flexneri 2a bioconjugate vaccine. EBioMedicine 66, 103308. doi: 10.1016/j.ebiom.2021.103308
Clerc, P., Baudry, B., Sansonetti, P. J. (1985). Plasmid-mediated contact haemolytic activity in shigella species: correlation with penetration into HeLa cells. Ann. Inst Pasteur Microbiol. 137A (3), 267–278.
Clerc, P. L., Ryter, A., Mounier, J., Sansonetti, P. J. (1987). Plasmid-mediated early killing of eucaryotic cells by shigella flexneri as studied by infection of J774 macrophages. Infect. Immun. 55 (3), 521–527. doi: 10.1128/iai.55.3.521-527.1987
Co, J. Y., Margalef-Catala, M., Monack, D. M., Amieva, M. R. (2021). Controlling the polarity of human gastrointestinal organoids to investigate epithelial biology and infectious diseases. Nat. Protoc. 16 (11), 5171–5192. doi: 10.1038/s41596-021-00607-0
Cohen, D., Ashkenazi, S., Schneerson, R., Farzam, N., Bialik, A., Meron-Sudai, S., et al. (2019). Serum IgG antibodies to shigella lipopolysaccharide antigens - a correlate of protection against shigellosis. Hum. Vaccin Immunother. 15 (6), 1401–1408. doi: 10.1080/21645515.2019.1606971
Cohen, D., Meron-Sudai, S., Bialik, A., Asato, V., Goren, S., Ariel-Cohen, O., et al. (2021). Safety and immunogenicity of a synthetic carbohydrate conjugate vaccine against shigella flexneri 2a in healthy adult volunteers: a phase 1, dose-escalating, single-blind, randomised, placebo-controlled study. Lancet Infect. Dis. 21 (4), 546–558. doi: 10.1016/S1473-3099(20)30488-6
Cohen, D., Atsmon, J., Artaud, C., Meron-Sudai, S., Gougeon, M. L., Bialik, A., et al. (2023). Threshold protective levels of serum IgG to shigella lipopolysaccharide: re-analysis of shigella vaccine trials data. Clin. Microbiol. Infect. 29 (3), 366–371. doi: 10.1016/j.cmi.2022.10.011
Cossart, P., Sansonetti, P. J. (2004). Bacterial invasion: the paradigms of enteroinvasive pathogens. Science 304 (5668), 242–248.
Dickenson, N. E., Zhang, L., Epler, C. R., Adam, P. R., Picking, W. L., Picking, W. D. (2011). Conformational changes in IpaD from shigella flexneri upon binding bile salts provide insight into the second step of type III secretion. Biochemistry 50 (2), 172–180. doi: 10.1021/bi101365f
Diebolder, C. A., Beurskens, F. J., De Jong, R. N., Koning, R. I., Strumane, K., Lindorfer, M. A., et al. (2014). Complement is activated by IgG hexamers assembled at the cell surface. Science 343 (6176), 1260–1263.
Doorduijn, D. J., Bardoel, B. W., Heesterbeek, D. a. C., Ruyken, M., Benn, G., Parsons, E. S., et al. (2020). Bacterial killing by complement requires direct anchoring of membrane attack complex precursor C5b-7. PloS Pathog. 16 (6), e1008606. doi: 10.1371/journal.ppat.1008606
Doorduijn, D. J., Rooijakkers, S. H. M., Heesterbeek, D. A. C. (2019). How the membrane attack complex damages the bacterial cell envelope and kills gram-negative bacteria. Bioessays 41 (10), e1900074. doi: 10.1002/bies.201900074
DuPont, H. L., Levine, M. M., Hornick, R. B., Formal, S. B. (1989). Inoculum size in shigellosis and implications for expected mode of transmission. J. Infect. Dis. 159 (6), 1126–1128. doi: 10.1093/infdis/159.6.1126
Edwards-Jones, B., Langford, P. R., Kroll, J. S., Yu, J. (2004). The role of the shigella flexneri yihE gene in LPS synthesis and virulence. Microbiol. (Reading) 150 (Pt 4), 1079–1084. doi: 10.1099/mic.0.26840-0
Epler, C. R., Dickenson, N. E., Bullitt, E., Picking, W. L. (2012). Ultrastructural analysis of IpaD at the tip of the nascent MxiH type III secretion apparatus of shigella flexneri. J. Mol. Biol. 420 (1-2), 29–39. doi: 10.1016/j.jmb.2012.03.025
Faherty, C. S., Redman, J. C., Rasko, D. A., Barry, E. M., Nataro, J. P. (2012). Shigella flexneri effectors OspE1 and OspE2 mediate induced adherence to the colonic epithelium following bile salts exposure. Mol. Microbiol. 85 (1), 107–121. doi: 10.1111/j.1365-2958.2012.08092.x
Francois, M., Le Cabec, V., Dupont, M. A., Sansonetti, P. J., Maridonneau-Parini, I. (2000). Induction of necrosis in human neutrophils by shigella flexneri requires type III secretion, IpaB and IpaC invasins, and actin polymerization. Infect. Immun. 68 (3), 1289–1296. doi: 10.1128/IAI.68.3.1289-1296.2000
Frenck, R. W., Jr., Conti, V., Ferruzzi, P., Ndiaye, A. G. W., Parker, S., Mcneal, M. M., et al. (2020). Establishment of a controlled human infection model with a lyophilized strain of shigella sonnei 53G. mSphere 5 (5). doi: 10.1128/mSphere.00416-20
Frenck, R. W., Jr., Dickey, M., Suvarnapunya, A. E., Chandrasekaran, L., Kaminski, R. W., Clarkson, K. A., et al. (2021). Efficacy, safety, and immunogenicity of the shigella sonnei 1790GAHB GMMA candidate vaccine: results from a phase 2b randomized, placebo-controlled challenge study in adults. EClinicalMedicine 39, 101076. doi: 10.1016/j.eclinm.2021.101076
Fudała, R., Doroszkiewicz, W., Niedbach, J., Gamian, A., Weintraub, A., Kaca, W. (2003). The factor C3 conversion in human complement by smooth shigella flexneri lipopolysaccharides. Acta Microbiol. Pol. 52 (1), 45–52.
Fukumatsu, M., Ogawa, M., Arakawa, S., Suzuki, M., Nakayama, K., Shimizu, S., et al. (2012). Shigella targets epithelial tricellular junctions and uses a noncanonical clathrin-dependent endocytic pathway to spread between cells. Cell Host Microbe 11 (4), 325–336. doi: 10.1016/j.chom.2012.03.001
Gaboriaud, C., Ling, W. L., Thielens, N. M., Bally, I., Rossi, V. (2014). Deciphering the fine details of c1 assembly and activation mechanisms: "mission impossible"? Front. Immunol. 5, 565.
Grassart, A., Malarde, V., Gobaa, S., Sartori-Rupp, A., Kerns, J., Karalis, K., et al. (2019). Bioengineered human organ-on-Chip reveals intestinal microenvironment and mechanical forces impacting shigella infection. Cell Host Microbe 26 (3), 435–444.e4. doi: 10.1016/j.chom.2019.08.007
Hayani, K. C., Guerrero, M. L., Morrow, A. L., Gomez, H. F., Winsor, D. K., Ruiz-Palacios, G. M., et al. (1992). Concentration of milk secretory immunoglobulin a against shigella virulence plasmid-associated antigens as a predictor of symptom status in shigella-infected breast-fed infants. J. Pediatr. 121 (6), 852–856. doi: 10.1016/S0022-3476(05)80327-0
Heesterbeek, D. A., Bardoel, B. W., Parsons, E. S., Bennett, I., Ruyken, M., Doorduijn, D. J., et al. (2019). Bacterial killing by complement requires membrane attack complex formation via surface-bound C5 convertases. EMBO J. 38 (4). doi: 10.15252/embj.201899852
High, N., Mounier, J., Prevost, M. C., Sansonetti, P. J. (1992). IpaB of shigella flexneri causes entry into epithelial cells and escape from the phagocytic vacuole. EMBO J. 11 (5), 1991–1999. doi: 10.1002/j.1460-2075.1992.tb05253.x
Holst, J., Feiring, B., Fuglesang, J. E., Hoiby, E. A., Nokleby, H., Aaberge, I. S., et al. (2003). Serum bactericidal activity correlates with the vaccine efficacy of outer membrane vesicle vaccines against neisseria meningitidis serogroup b disease. Vaccine 21 (7-8), 734–737. doi: 10.1016/S0264-410X(02)00591-1
Kaminski, R. W., Clarkson, K., Kordis, A. A., Oaks, E. V. (2013). Multiplexed immunoassay to assess shigella-specific antibody responses. J. Immunol. Methods 393 (1-2), 18–29. doi: 10.1016/j.jim.2013.04.002
Kaminski, R. W., Pasetti, M. F., Aguilar, A. O., Clarkson, K. A., Rijpkema, S., Bourgeois, A. L., et al. (2019). Consensus report on shigella controlled human infection model: immunological assays. Clin. Infect. Dis. 69 (Suppl 8), S596–S601. doi: 10.1093/cid/ciz909
Kapulu, M. C., Nakakana, U., Scire, A. S., Sarakinou, E., Conti, V., Rossi, O., et al. (2022). Complement-mediated serum bactericidal activity of antibodies elicited by the shigella sonnei GMMA vaccine in adults from a shigellosis-endemic country: exploratory analysis of a phase 2a randomized study. Front. Immunol. 13, 971866. doi: 10.3389/fimmu.2022.971866
Karsten, C. B., Mehta, N., Shin, S.A., Diefenbach, T. J., Slein, M. D., Karpinski, W., et al. (2019). A versatile high-throughput assay to characterize antibody-mediated neutrophil phagocytosis. J. Immunol. Methods 471, 46–56. doi: 10.1016/j.jim.2019.05.006
Khalil, I. A., Troeger, C., Blacker, B. F., Rao, P. C., Brown, A., Atherly, D. E., et al. (2018). Morbidity and mortality due to shigella and enterotoxigenic escherichia coli diarrhoea: the global burden of disease study 1990-2016. Lancet Infect. Dis. 18 (11), 1229–1240. doi: 10.1016/S1473-3099(18)30475-4
Kim, J. O., Rho, S., Kim, S. H., Kim, H., Song, H. J., Kim, E. J., et al. (2015). Shigella outer membrane protein PSSP-1 is broadly protective against shigella infection. Clin. Vaccine Immunol. 22 (4), 381–388. doi: 10.1128/CVI.00661-14
Koestler, B. J., Ward, C. M., Fisher, C. R., Rajan, A., Maresso, A. W., Payne, S. M. (2019). Human intestinal enteroids as a model system of shigella pathogenesis. Infect. Immun. 87 (4). doi: 10.1128/IAI.00733-18
Kohler, H., Rodrigues, S. P., McCormick, B. A. (2002). Shigella flexneri interactions with the basolateral membrane domain of polarized model intestinal epithelium: role of lipopolysaccharide in cell invasion and in activation of the mitogen-activated protein kinase ERK. Infect. Immun. 70 (3), 1150–1158. doi: 10.1128/IAI.70.3.1150-1158.2002
Kotloff, K. L., Riddle, M. S., Platts-Mills, J. A., Pavlinac, P., Zaidi, A. K. M. (1999). Global burden of shigella infections: implications for vaccine development and implementation of control strategies. Bull. World Health Organ 77 (8), 651–666.
Kotloff, K. L., Winickoff, J. P., Ivanoff, B., Clemens, J. D., Swerdlow, D. L., Sansonetti, P. J., et al. (2018). Shigellosis. Lancet 391 (10122), 801–812. doi: 10.1016/S0140-6736(17)33296-8
Lan, R., Reeves, P. R. (2002). Escherichia coli in disguise: molecular origins of shigella. Microbes Infect. 4 (11), 1125–1132. doi: 10.1016/S1286-4579(02)01637-4
Lederer, I., Much, P., Allerberger, F., Voracek, T., Vielgrader, H. (2005). Outbreak of shigellosis in the Vienna zoo affecting human and non-human primates. Int. J. Infect. Dis. 9 (5), 290–291. doi: 10.1016/j.ijid.2004.11.003
Lefevre, S., Njamkepo, E., Feldman, S., Ruckly, C., Carle, I., Lejay-Collin, M., et al. (2023). Rapid emergence of extensively drug-resistant shigella sonnei in France. Nat. Commun. 14 (1), 462. doi: 10.1038/s41467-023-36222-8
Lemme-Dumit, J. M., Doucet, M., Zachos, N. C., Pasetti, M. F. (2022). Epithelial and neutrophil interactions and coordinated response to shigella in a human intestinal enteroid-neutrophil coculture model. mBio 13 (3), e0094422.
Li, S., Han, X., Upadhyay, I., Zhang, W. (2022). Characterization of functional b-cell epitopes at the amino terminus of shigella invasion plasmid antigen b (IpaB). Appl. Environ. Microbiol. 88 (15), e0038422. doi: 10.1128/aem.00384-22
Libby, T. E., Delawalla, M. L. M., Al-Shimari, F., Maclennan, C. A., Vannice, K. S., Pavlinac, P. B. (2023). Consequences of shigella infection in young children: a systematic review. Int. J. Infect. Dis. 129, 78–95. doi: 10.1016/j.ijid.2023.01.034
Lindberg, A. A., Karnell, A., Weintraub, A. (1991). The lipopolysaccharide of shigella bacteria as a virulence factor. Rev. Infect. Dis. 13 (Suppl 4), S279–S284. doi: 10.1093/clinids/13.Supplement_4.S279
Liu, B., Knirel, Y. A., Feng, L., Perepelov, A.V., Senchenkova, S. N., Wang, Q., et al. (2008). Structure and genetics of shigella O antigens. FEMS Microbiol. Rev. 32 (4), 627–653. doi: 10.1111/j.1574-6976.2008.00114.x
Livio, S., Strockbine, N.A., Panchalingam, S., Tennant, S. M., Barry, E. M., Marohn, M. E., et al. (2014). Shigella isolates from the global enteric multicenter study inform vaccine development. Clin. Infect. Dis. 59 (7), 933–941. doi: 10.1093/cid/ciu468
Llanos-Chea, A., Citorik, R. J., Nickerson, K. P., Ingano, L., Serena, G., Senger, S., et al. (2019). Bacteriophage therapy testing against shigella flexneri in a novel human intestinal organoid-derived infection model. J. Pediatr. Gastroenterol. Nutr. 68 (4), 509–516. doi: 10.1097/MPG.0000000000002203
MacLennan, C. A., Grow, S., Ma, L. F., Steele, A. D. (2022). The shigella vaccines pipeline. Vaccines (Basel) 10 (9), 1376. doi: 10.3390/vaccines10091376
Mahmoud, R. Y., Stones, D. H., Li, W., Emara, M., El-Domany, R. A., Wang, D., et al. (2016). The multivalent adhesion molecule SSO1327 plays a key role in shigella sonnei pathogenesis. Mol. Microbiol. 99 (4), 658–673. doi: 10.1111/mmi.13255
Mallett, C. P., Vandeverg, L., Collins, H. H., Hale, T. L. (1993). Evaluation of shigella vaccine safety and efficacy in an intranasally challenged mouse model. Vaccine 11 (2), 190–196. doi: 10.1016/0264-410X(93)90016-Q
Mancini, F., Alfini, R., Caradonna, V., Monaci, V., Carducci, M., Gasperini, G., et al. (2023). Exploring the role of GMMA components in the immunogenicity of a 4-valent vaccine against shigella. Int. J. Mol. Sci. 24 (3). doi: 10.3390/ijms24032742
Mancini, F., Micoli, F., Rossi, O. (2022). Setup and characterization of a high-throughput luminescence-based serum bactericidal assay (L-SBA) to determine functionality of human sera against shigella flexneri. Biotech. (Basel) 11 (3). doi: 10.3390/biotech11030029
Marteyn, B., Gazi, A., Sansonetti, P. (2012). Shigella: a model of virulence regulation in vivo. Gut Microbes 3 (2), 104–120. doi: 10.4161/gmic.19325
Martin, P., Alaimo, C. (2022). The ongoing journey of a shigella bioconjugate vaccine. Vaccines (Basel) 10 (2). doi: 10.3390/vaccines10020212
Martinez-Becerra, F. J., Kissmann, J. M., Diaz-Mcnair, J., Choudhari, S. P., Quick, A. M., Mellado-Sanchez, G., et al. (2012). Broadly protective shigella vaccine based on type III secretion apparatus proteins. Infect. Immun. 80 (3), 1222–1231. doi: 10.1128/IAI.06174-11
Martinic, M., Hoare, A., Contreras, I., Alvarez, S. A. (2011). Contribution of the lipopolysaccharide to resistance of shigella flexneri 2a to extreme acidity. PloS One 6 (10), e25557. doi: 10.1371/journal.pone.0025557
Mathan, M. M., Mathan, V. I. (1991). Morphology of rectal mucosa of patients with shigellosis. Rev. Infect. Dis. 13 (Suppl 4), S314–S318. doi: 10.1093/clinids/13.Supplement_4.S314
Mathias, A., Longet, S., Corthésy, B. (2013). Agglutinating secretory IgA preserves intestinal epithelial cell integrity during apical infection by shigella flexneri. Infection Immun. 81 (8), 3027–3034. doi: 10.1128/IAI.00303-13
McArthur, M. A., Maciel, M., Jr., Pasetti, M. F. (2017). Human immune responses against shigella and enterotoxigenic e. coli: current advances and the path forward. Vaccine 35 (49 Pt A), 6803–6806.
McGuire, M. K., Randall, A. Z., Seppo, A. E., Jarvinen, K. M., Meehan, C. L., Gindola, D., et al. (2020). Multipathogen analysis of IgA and IgG antigen specificity for selected pathogens in milk produced by women from diverse geographical regions: the INSPIRE study. Front. Immunol. 11, 614372. doi: 10.3389/fimmu.2020.614372
Medeiros, P., Bolick, D. T., Ledwaba, S. E., Kolling, G. L., Costa, D. V. S., Oria, R. B., et al. (2020). A bivalent vaccine confers immunogenicity and protection against shigella flexneri and enterotoxigenic escherichia coli infections in mice. NPJ Vaccines 5 (1), 30. doi: 10.1038/s41541-020-0180-y
Mel, D. M., Arsic, B. L., Nikolic, B. D., Radovanic, M. L. (1968). Studies on vaccination against bacillary dysentery. 4. oral immunization with live monotypic and combined vaccines. Bull. World Health Organ 39 (3), 375–380.
Micoli, F., Nakakana, U. N., Berlanda Scorza, F. (2021). Antibodies elicited by the shigella sonnei GMMA vaccine in adults trigger complement-mediated serum bactericidal activity: results from a phase 1 dose escalation trial followed by a booster extension. Front. Immunol. 12, 671325. doi: 10.3389/fimmu.2021.671325
Micoli, F., Nakakana, U. N., Berlanda Scorza, F. (2022). Towards a four-component GMMA-based vaccine against shigella. Vaccines (Basel) 10 (2). doi: 10.3390/vaccines10020328
Mills, J. A., Buysse, J. M., Oaks, E. V. (1988). Shigella flexneri invasion plasmid antigens b and c: epitope location and characterization with monoclonal antibodies. Infect. Immun. 56 (11), 2933–2941. doi: 10.1128/iai.56.11.2933-2941.1988
Mitchell, P. S., Roncaioli, J. L., Turcotte, E. A., Goers, L., Chavez, R. A., Lee, A. Y., et al. (2020). NAIP-NLRC4-deficient mice are susceptible to shigellosis. Elife 9. doi: 10.7554/eLife.59022.sa2
Morona, R., Daniels, C., Van Den Bosch, L. (2003). Genetic modulation of shigella flexneri 2a lipopolysaccharide O antigen modal chain length reveals that it has been optimized for virulence. Microbiol. (Reading) 149 (Pt 4), 925–939. doi: 10.1099/mic.0.26141-0
Mortensen, S. A., Sander, B., Jensen, R. K., Pedersen, J. S., Golas, M. M., Jensenius, J. C., et al. (2017). Structure and activation of C1, the complex initiating the classical pathway of the complement cascade. Proc. Natl. Acad. Sci. U.S.A. 114 (5), 986–991. doi: 10.1073/pnas.1616998114
Mounier, J., Vasselon, T., Hellio, R., Lesourd, M., Sansonetti, P. J. (1992). Shigella flexneri enters human colonic caco-2 epithelial cells through the basolateral pole. Infect. Immun. 60 (1), 237–248. doi: 10.1128/iai.60.1.237-248.1992
Muthuramalingam, M., Whittier, S. K., Picking, W. L., Picking, W. D. (2021). The shigella type III secretion system: an overview from top to bottom. Microorganisms 9 (2). doi: 10.3390/microorganisms9020451
Nahm, M. H., Yu, J., Weerts, H. P., Wenzel, H., Tamilselvi, C. S., Chandrasekaran, L., et al. (2018). Development, interlaboratory evaluations, and application of a simple, high-throughput shigella serum bactericidal assay. mSphere 3. doi: 10.1128/mSphere.00146-18
Ndungo, E., Randall, A., Hazen, T. H., Kania, D. A., Trappl-Kimmons, K., Liang, X., et al. (2018). A novel shigella proteome microarray discriminates targets of human antibody reactivity following oral vaccination and experimental challenge. mSphere 3 (4). doi: 10.1128/mSphere.00260-18
Ndungo, E., Andronescu, L. R., Buchwald, A. G., Lemme-Dumit, J. M., Mawindo, P., Kapoor, N., et al. (2021). Repertoire of naturally acquired maternal antibodies transferred to infants for protection against shigellosis. Front. Immunol. 12, 725129. doi: 10.3389/fimmu.2021.725129
Ndungo, E., Pasetti, M. F. (2020). Functional antibodies as immunological endpoints to evaluate protective immunity against shigella. Hum. Vaccin Immunother. 16 (1), 197–205. doi: 10.1080/21645515.2019.1640427
Necchi, F., Saul, A., Rondini, S. (2018). Setup of luminescence-based serum bactericidal assay against salmonella paratyphi a. J. Immunol. Methods 461, 117–121. doi: 10.1016/j.jim.2018.06.025
Nickerson, K. P., Chanin, R. B., Sistrunk, J. R., Rasko, D. A., Fink, P. J., Barry, E. M., et al. (2017). Analysis of shigella flexneri resistance, biofilm formation, and transcriptional profile in response to bile salts. Infect. Immun. 85 (6). doi: 10.1128/IAI.01067-16
Oaks, E. V., Hale, T. L., Formal, S. B. (1986). Serum immune response to shigella protein antigens in rhesus monkeys and humans infected with shigella spp. Infect. Immun. 53 (1), 57–63. doi: 10.1128/iai.53.1.57-63.1986
Okamura, N., Nakaya, R. (1977). Rough mutant of shigella flexneri 2a that penetrates tissue culture cells but does not evoke keratoconjunctivitis in guinea pigs. Infect. Immun. 17 (1), 4–8. doi: 10.1128/iai.17.1.4-8.1977
Olive, A. J., Kenjale, R., Espina, M., Moore, D. S., Picking, W. L., Picking, W. D. (2007). Bile salts stimulate recruitment of IpaB to the shigella flexneri surface, where it colocalizes with IpaD at the tip of the type III secretion needle. Infect. Immun. 75 (5), 2626–2629. doi: 10.1128/IAI.01599-06
Pasetti, M. F., Venkatesan, M. M., Barry, E. M. (2020). Oral shigella vaccines, in. in Mucosal Vaccines, 515–536. doi: 10.1016/B978-0-12-811924-2.00030-4
Passwell, J. H., Ashkenazi, S., Banet-Levi, Y., Ramon-Saraf, R., Farzam, N., Lerner-Geva, L., et al. (2010). Age-related efficacy of shigella O-specific polysaccharide conjugates in 1-4-year-old Israeli children. Vaccine 28 (10), 2231–2235. doi: 10.1016/j.vaccine.2009.12.050
Perdomo, J. J., Gounon, P., Sansonetti, P. J. (1994a). Acute inflammation causes epithelial invasion and mucosal destruction in experimental shigellosis. J. Exp. Med. 180 (4), 1307–1319. doi: 10.1084/jem.180.4.1307
Perdomo, J. J., Gounon, P., Sansonetti, P. J. (1994b). Polymorphonuclear leukocyte transmigration promotes invasion of colonic epithelial monolayer by shigella flexneri. J. Clin. Invest. 93 (2), 633–643. doi: 10.1172/JCI117015
PH, Q. S. M., Ledwaba, S. E., Bolick, D. T., Giallourou, N., Yum, L. K., Costa, D.V.S., et al. (2019). A murine model of diarrhea, growth impairment and metabolic disturbances with shigella flexneri infection and the role of zinc deficiency. Gut Microbes 10 (5), 615–630.
Plotkin, S. A. (2020). Updates on immunologic correlates of vaccine-induced protection. Vaccine 38 (9), 2250–2257. doi: 10.1016/j.vaccine.2019.10.046
Plotkin, S. A., Gilbert, P. B. (2012). Nomenclature for immune correlates of protection after vaccination. Clin. Infect. Dis. 54 (11), 1615–1617. doi: 10.1093/cid/cis238
Ranganathan, S., Doucet, M., Grassel, C. L., Delaine-Elias, B., Zachos, N. C., Barry, E. M. (2019). Evaluating shigella flexneri pathogenesis in the human enteroid model. Infect. Immun. 87 (4). doi: 10.1128/IAI.00740-18
Rey, C., Chang, Y. Y., Latour-Lambert, P., Varet, H., Proux, C., Legendre, R., et al. (2020). Transcytosis subversion by m cell-to-enterocyte spread promotes shigella flexneri and listeria monocytogenes intracellular bacterial dissemination. PloS Pathog. 16 (4), e1008446. doi: 10.1371/journal.ppat.1008446
Romero-Steiner, S., Spear, W., Brown, N., Holder, P., Hennessy, T., Gomez De Leon, P., et al. (2004). Measurement of serum bactericidal activity specific for haemophilus influenzae type b by using a chromogenic and fluorescent metabolic indicator. Clin. Diagn. Lab. Immunol. 11 (1), 89–93. doi: 10.1128/CDLI.11.1.89-93.2004
Rossi, O., Molesti, E., Saul, A., Giannelli, C., Micoli, F., Necchi, F. (2020). Intra-laboratory evaluation of luminescence based high-throughput serum bactericidal assay (L-SBA) to determine bactericidal activity of human sera against shigella. High Throughput 9 (2). doi: 10.3390/ht9020014
Sansonetti, P. (2001). Phagocytosis of bacterial pathogens: implications in the host response. Semin. Immunol. 13 (6), 381–390. doi: 10.1006/smim.2001.0335
Sansonetti, P. J., Hale, T. L., Dammin, G. J., Kapfer, C., Collins, H. H., Jr., Formal, S. B. (1983). Alterations in the pathogenicity of escherichia coli K-12 after transfer of plasmid and chromosomal genes from shigella flexneri. Infect. Immun. 39 (3), 1392–1402. doi: 10.1128/iai.39.3.1392-1402.1983
Sansonetti, P. J., Ryter, A., Clerc, P., Maurelli, A. T., Mounier, J. (1986). Multiplication of shigella flexneri within HeLa cells: lysis of the phagocytic vacuole and plasmid-mediated contact hemolysis. Infect. Immun. 51 (2), 461–469. doi: 10.1128/iai.51.2.461-469.1986
Sansonetti, P. J., Arondel, J., Cantey, J. R., Prevost, M. C., Huerre, M. (1996). Infection of rabbit peyer's patches by shigella flexneri: effect of adhesive or invasive bacterial phenotypes on follicle-associated epithelium. Infect. Immun. 64 (7), 2752–2764. doi: 10.1128/iai.64.7.2752-2764.1996
Schroeder, G. N., Hilbi, H. (2008). Molecular pathogenesis of shigella. spp.: controlling Host Cell signaling invasion Death by type III secretion. Clin. Microbiol. Rev. 21 (1), 134–156.
Sereny, B. (1957). Experimental keratoconjunctivitis shigellosa. Acta Microbiol. Acad. Sci. Hung 4 (4), 367–376.
Shen, D. K., Saurya, S., Wagner, C., Nishioka, H., Blocker, A. J. (2010). Domains of the shigella flexneri type III secretion system IpaB protein involved in secretion regulation. Infect. Immun. 78 (12), 4999–5010. doi: 10.1128/IAI.00470-10
Shim, D. H., Suzuki, T., Chang, S. Y., Park, S. M., Sansonetti, P. J., Sasakawa, C., et al. (2007). New animal model of shigellosis in the Guinea pig: its usefulness for protective efficacy studies. J. Immunol. 178 (4), 2476–2482. doi: 10.4049/jimmunol.178.4.2476
Shimanovich, A. A., Buskirk, A. D., Heine, S. J., Blackwelder, W. C., Wahid, R., Kotloff, K. L., et al. (2017). Functional and antigen-specific serum antibody levels as correlates of protection against shigellosis in a controlled human challenge study. Clin. Vaccine Immunol. 24 (2). doi: 10.1128/CVI.00412-16
Sierocki, R., Jneid, B., Orsini Delgado, M. L., Plaisance, M., Maillere, B., Nozach, H., et al. (2021). An antibody targeting type III secretion system induces broad protection against salmonella and shigella infections. PloS Negl. Trop. Dis. 15 (3), e0009231. doi: 10.1371/journal.pntd.0009231
Sina, C., Kemper, C., Derer, S. (2018). The intestinal complement system in inflammatory bowel disease: shaping intestinal barrier function. Semin. Immunol. 37, 66–73. doi: 10.1016/j.smim.2018.02.008
Sistrunk, J. R., Nickerson, K. P., Chanin, R. B., Rasko, D. A., Faherty, C. S. (2016). Survival of the fittest: how bacterial pathogens utilize bile to enhance infection. Clin. Microbiol. Rev. 29 (4), 819–836. doi: 10.1128/CMR.00031-16
Skoudy, A., Mounier, J., Aruffo, A., Ohayon, H., Gounon, P., Sansonetti, P., et al. (2000). CD44 binds to the shigella IpaB protein and participates in bacterial invasion of epithelial cells. Cell Microbiol. 2 (1), 19–33. doi: 10.1046/j.1462-5822.2000.00028.x
Stadtmueller, B. M., Huey-Tubman, K. E., López, C. J., Yang, Z., Hubbell, W. L., Bjorkman, P. J. (2016). The structure and dynamics of secretory component and its interactions with polymeric immunoglobulins. eLife 5, e10640. doi: 10.7554/eLife.10640
Stensrud, K. F., Adam, P. R., La Mar, C. D., Olive, A. J., Lushington, G. H., Sudharsan, R., et al. (2008). Deoxycholate interacts with IpaD of shigella flexneri in inducing the recruitment of IpaB to the type III secretion apparatus needle tip. J. Biol. Chem. 283 (27), 18646–18654. doi: 10.1074/jbc.M802799200
Strasser, J., De Jong, R. N., Beurskens, F. J., Wang, G., Heck, A. J. R., Schuurman, J., et al. (2019). Unraveling the macromolecular pathways of IgG oligomerization and complement activation on antigenic surfaces. Nano Lett. 19 (7), 4787–4796. doi: 10.1021/acs.nanolett.9b02220
Talaat, K. R., Alaimo, C., Martin, P., Bourgeois, A. L., Dreyer, A. M., Kaminski, R. W., et al. (2021). Human challenge study with a shigella bioconjugate vaccine: analyses of clinical efficacy and correlate of protection. EBioMedicine 66, 103310. doi: 10.1016/j.ebiom.2021.103310
Taylor, D. N., Trofa, A. C., Sadoff, J., Chu, C., Bryla, D., Shiloach, J., et al. (1993). Synthesis, characterization, and clinical evaluation of conjugate vaccines composed of the O-specific polysaccharides of shigella dysenteriae type 1, shigella flexneri type 2a, and shigella sonnei (Plesiomonas shigelloides) bound to bacterial toxoids. Infect. Immun. 61 (9), 3678–3687. doi: 10.1128/iai.61.9.3678-3687.1993
Thompson, C. N., Le, T. P., Anders, K. L., Nguyen, T. H., Lu, L. V., Nguyen, V. V., et al. (2016). The transfer and decay of maternal antibody against shigella sonnei in a longitudinal cohort of Vietnamese infants. Vaccine 34 (6), 783–790. doi: 10.1016/j.vaccine.2015.12.047
Tinevez, J.-Y., Arena, E. T., Anderson, M., Nigro, G., Injarabian, L., Andre, A., et al. (2019). Shigella-mediated oxygen depletion is essential for intestinal mucosa colonization. Nat. Microbiol. 4 (11), 2001–2009. doi: 10.1038/s41564-019-0525-3
Turbyfill, K. R., Clarkson, K. A., Oaks, E. V., Kaminski, R. W. (2022). From concept to clinical product: a brief history of the novel shigella invaplex vaccine's refinement and evolution. Vaccines (Basel) 10 (4). doi: 10.3390/vaccines10040548
Turula, H., Wobus, C. E. (2018). The role of the polymeric immunoglobulin receptor and secretory immunoglobulins during mucosal infection and immunity. Viruses 10 (5), 237. doi: 10.3390/v10050237
Wang, T. T., Ravetch, J. V. (2019). Functional diversification of IgGs through fc glycosylation. J. Clin. Invest. 129 (9), 3492–3498. doi: 10.1172/JCI130029
Wassef, J. S., Keren, D. F., Mailloux, J. L. (1989). Role of m cells in initial antigen uptake and in ulcer formation in the rabbit intestinal loop model of shigellosis. Infect. Immun. 57 (3), 858–863. doi: 10.1128/iai.57.3.858-863.1989
Wei, H., Wang, J.-Y. (2021). Role of polymeric immunoglobulin receptor in IgA and IgM transcytosis. Int. J. Mol. Sci. 22 (5), 2284. doi: 10.3390/ijms22052284
West, N. P., Sansonetti, P., Mounier, J., Exley, R. M., Parsot, C., Guadagnini, S., et al. (2005). Optimization of virulence functions through glucosylation of shigella LPS. Science 307 (5713), 1313–1317.
World Health Organization. (2005). Guidelines for the control of shigellosis, including epidemics due to Shigella dysenteriae type 1.
World Health Organization. (2017). WHO global priority pathogens list of antibiotic-resistant bacteria. [Online]. Available: https://www.who.int/news/item/27-02-2017-who-publishes-list-of-bacteria-for-which-new-antibiotics-are-urgently-needed. [Accessed 23/06/2022 2022].
Xu, D., Liao, C., Zhang, B., Tolbert, W. D., He, W., Dai, Z., et al. (2018). Human enteric alpha-defensin 5 promotes shigella infection by enhancing bacterial adhesion and invasion. Immunity 48 (6), 1233–1244.e6. doi: 10.1016/j.immuni.2018.04.014
Yang, F., Yang, J., Zhang, X., Chen, L., Jiang, Y., Yan, Y., et al. (2005). Genome dynamics and diversity of shigella species, the etiologic agents of bacillary dysentery. Nucleic Acids Res. 33 (19), 6445–6458. doi: 10.1093/nar/gki954
Yang, J. Y., Lee, S. N., Chang, S. Y., Ko, H. J., Ryu, S., Kweon, M. N. (2014). A mouse model of shigellosis by intraperitoneal infection. J. Infect. Dis. 209 (2), 203–215. doi: 10.1093/infdis/jit399
Keywords: antibodies, functional assays, Shigella, serum bactericidal assay (SBA), opsonophagocytic killing assay (OPKA), invasion
Citation: Boero E, Vezzani G, Micoli F, Pizza M and Rossi O (2023) Functional assays to evaluate antibody-mediated responses against Shigella: a review. Front. Cell. Infect. Microbiol. 13:1171213. doi: 10.3389/fcimb.2023.1171213
Received: 21 February 2023; Accepted: 27 April 2023;
Published: 16 May 2023.
Edited by:
Andrea L Kwa, Singapore General Hospital, SingaporeReviewed by:
Andrea Puhar, Umeå University, SwedenBen Koestler, Western Michigan University, United States
Copyright © 2023 Boero, Vezzani, Micoli, Pizza and Rossi. This is an open-access article distributed under the terms of the Creative Commons Attribution License (CC BY). The use, distribution or reproduction in other forums is permitted, provided the original author(s) and the copyright owner(s) are credited and that the original publication in this journal is cited, in accordance with accepted academic practice. No use, distribution or reproduction is permitted which does not comply with these terms.
*Correspondence: Omar Rossi, b21hci54LnJvc3NpQGdzay5jb20=