- 1State Key Laboratory of Genetic Engineering, Ministry of Education Key Laboratory of Contemporary Anthropology, Human Phenome Institute, Fudan University, Shanghai, China
- 2Ministry of Education Key Laboratory for Biodiversity Science and Ecological Engineering, Department of Microbiology and Microbial Engineering, School of Life Sciences, Fudan University, Shanghai, China
- 3The State Key Laboratory of Reproductive Regulation and Breeding of Grassland Livestock, College of Life Sciences, Inner Mongolia University, Hohhot, China
- 4Central Laboratory, Anhui Provincial Institute of Parasitic Diseases, Anhui, China
Background: Schistosomiasis, the second most neglected tropical disease defined by the WHO, is a significant zoonotic parasitic disease infecting approximately 250 million people globally. This debilitating disease has seriously threatened public health, while only one drug, praziquantel, is used to control it. Because of this, it highlights the significance of identifying more satisfactory target genes for drug development. Protein translocation into the endoplasmic reticulum (ER) is vital to the subsequent localization of secretory and transmembrane proteins. The signal peptidase complex (SPC) is an essential component of the translocation machinery and functions to cleave the signal peptide sequence (SP) of secretory and membrane proteins entering the ER. Inhibiting the expression of SPC can lead to the abolishment or weaker cleavage of the signal peptide, and the accumulation of uncleaved protein in the ER would affect the survival of organisms. Despite the evident importance of SPC, in vivo studies exploring its function have yet to be reported in S. japonicum.
Methods: The S. japonicum SPC consists of four proteins: SPC12, SPC18, SPC22 and SPC25. RNA interference was used to investigate the impact of SPC components on schistosome growth and development in vivo. qPCR and in situ hybridization were applied to localize the SPC25 expression. Mayer’s carmalum and Fast Blue B staining were used to observe morphological changes in the reproductive organs of dsRNA-treated worms. The effect of inhibitor treatment on the worm’s viability and pairing was also examined in vitro.
Results: Our results showed that RNAi-SPC delayed the worm's normal development and was even lethal for schistosomula in vivo. Among them, the expression of SPC25 was significantly higher in the developmental stages of the reproductive organs in schistosomes. Moreover, SPC25 possessed high expression in the worm tegument, testes of male worms and the ovaries and vitellarium of female worms. The SPC25 knockdown led to the degeneration of reproductive organs, such as the ovaries and vitellarium of female worms. The SPC25 exhaustion also reduced egg production while reducing the pathological damage of the eggs to the host. Additionally, the SPC-related inhibitor AEBSF or suppressing the expression of SPC25 also impacted cultured worms’ pairing and viability in vitro.
Conclusions: These data demonstrate that SPC is necessary to maintain the development and reproduction of S. japonicum. This research provides a promising anti-schistosomiasis drug target and discovers a new perspective on preventing worm fecundity and maturation.
Introduction
Schistosomiasis, a neglected tropical disease caused by the parasitic flatworm Schistosoma japonicum, affects more than 230 million people worldwide (Barnett, 2018). This trematode has a complicated life cycle involving asexual reproduction within molluscan intermediate hosts and sexual reproduction within definitive mammalian hosts. As a striking parasite, S. japonicum can last for decades in the host, and every pair of worms can possess a prodigious egg output of 1,000 ~ 2,200 per day (Cheever et al., 1994; Kuntz et al., 2007; Schwartz and Fallon, 2018). The prodigious egg production resulting from the pairing of female worms is swept into the circulation and then deposited or trapped in the host organ, which can cause inflammatory reactions and disturb normal function, such as liver fibrosis and hypertension (Hambrook and Hanington, 2020; Takaki et al., 2020). Thus, worm transmission and disease pathogenesis were both completed by the eggs. Both host pathological disruption and disease transmission will be prevented if the parasites can be killed or deprived of oviposition capacity. However, only one drug (praziquantel) is currently effective in treating schistosomiasis. This medication does not prevent reinfection and has little effect on the schistosomula (Brindley and Sher, 1987; Brindley et al., 1989). Besides, the emergence of drug-resistant parasites also emphasizes the urgent need for novel therapeutics (Kuntz et al., 2007; Le Clec'h et al., 2021). Although considerable research has been employed over the years, the extremely complex life cycle and the technological barriers to genetic manipulation of Schistosoma make it challenging to find an effective gene as the antischistosomal drug target (Kuntz et al., 2007; Han et al., 2009; Cioli et al., 2014; Eyayu et al., 2020).
Detailed dynamic transcriptome studies indicated that a subset of highly expressed mRNA in the development stage of male-female worm pairing has also been characterized as being related to protein biosynthesis (Wang et al., 2017). The comparative analysis of single-sex and double-sex infections of S. japonicum also showed that the differentially expressed proteins of paired females were mainly enriched in the bioinformatics related to protein synthesis (Li et al., 2020). Recent research also revealed that a specialized ribosomal protein expression profile after pairing was upregulated to regulate the protein synthesis process for sexual maturation (Sun et al., 2015). The increasing body of evidence suggests that protein synthesis-related genes involved in parasite development, maturation, and reproduction can serve as an essential group of drug targets to block the spread of schistosomiasis (Avelar et al., 2019; Zeng et al., 2022). Consistent with this notion, the results of a large-scale RNAi screen of S. mansoni revealed that many gene phenotypes affecting neuromuscular function, tissue integrity, stem cell maintenance, and parasite survival are associated with protein synthesis processes (Guidi et al., 2015; Ittiprasert et al., 2019; Wang et al., 2020).
The signal peptidase complex (SPC) was used in the translocation of proteins into the endoplasmic reticulum (ER) by cleaving the signal peptide sequence (SP) of secretory and membrane proteins (Paetzel et al., 2000; Haase Gilbert et al., 2013). In S. cerevisiae, the composition of SPC has four proteins: Sec11, Spc1p, Spc2p and Spc3p. Previously, studies have shown that depletion of SPC3 and Sec11p elicits the accumulation of uncleaved protein in the ER. It also confirmed that SPC3 and Sec11p are responses for the viability of yeast cells, while Spc1p and Spc2p are essential but not necessarily for S. cerevisiae growth. (Mullins et al., 1996; Meyer and Hartmann, 1997). In Drosophila, Spase12 depletion was embryonic lethal and resulted in developmental defects in tissues, indicating that Spase12 could regulate cell viability, development, and differentiation (Haase Gilbert et al., 2013). In mammals, SPC18, SPC21, SPC22/23, SPC12 and SPC25 comprise the SPC, and all five subunits are essential to the proper release of the secreted protein and localization of membrane proteins synthesized with cleaved signal peptides (Shelness et al., 1993). ER-related protein is essential in eukaryotic cells and is particularly critical in fast-growing cells (Kalies and Romisch, 2015). It has been confirmed that the protein synthesis process can serve as a target for potential cancer drugs and bacterial virulence factors (Harbut et al., 2012). In yeast and mammalian cells, the function of signal peptidases has been elucidated. However, there is little information about its role in multicellular organisms, for example, in S. japonicum. Similar to cancer cells, the ovary, vitellarium and testis of S. japonicum have cells with high proliferative activity, requiring more protein synthesis to maintain the cell proliferation and differentiation (Buszczak et al., 2014; do Patrocinio et al., 2020). Given the importance of SPC in other species and its expression pattern in S. japonicum, we hypothesized that SPC might be essential to worm survival and fecundity.
To examine this hypothesis, we contributed to characterizing SPC molecular function in S. japonicum. In this study, we employed dsRNA-mediated RNA interference (RNAi) in vivo to characterize gene function. We observed that SPC played an essential role in the development and survival of schistosomula. Further studies confirmed that SPC25 had higher expression levels in the reproductive organs of worms. In addition, this gene was shown to be essential for maintaining the normal morphology of worm reproductive organs and for the oviposition of the female worm. These findings provided insights into genes that regulated the development and egg production of S. japonicum and revealed a novel class of antischistosomal candidates for therapeutic opportunities.
Materials and methods
Parasites and animals
The S. japonicum (Anhui isolate) strain used in this study was obtained from Oncomelania Anhui snails provided by the pathobiology laboratory of the National Institute of Parasitic Diseases, Chinese Center for Disease Control and Prevention, Shanghai. Six-week-old female Kunming mice purchased from Shanghai Animal Center, Chinese Academy of Sciences (Shanghai, China), were infected with S. japonicum. Schistosome-infected Kunming mice were euthanized at 30 or 42 days post-infection (dpi) by carbon dioxide asphyxiation. And then, parasites were isolated from the infected mice by hepatic-portal perfusion using a saline solution containing heparin sodium (Li et al., 2018).
Amplification and sequencing
Total schistosome RNA was extracted using TRIzol (Takara, Japan) from different developmental stages, sexes and regions of worms. The extracted RNA was quantified using a Nanodrop ND2000C Spectrophotometer (Nanodrop Technologies, Wilmington, DE). The cDNA was synthesized from 1000 ng of RNA using the PrimeScript RT reagent Kit with gDNA Eraser (Takara, Japan) according to the manufacturer’s instructions. Primers to amplify target sequences were designed using the Primer 5 program described in Table 1 (Li et al., 2018). Target fragments were amplified from S. japonicum cDNA and sequenced by Huajin Biotech Co. Ltd (Shanghai, China).
The full-length sequences of SPC12 (Sjc_0006936), SPC18 (Sjc_0009443), SPC22 (Sjc_0006565) and SPC25 (Sjc_0003692) were obtained from the S. japonicum database and NCBI. The primers were designed for fragments for dsRNA synthesis, riboprobe of RNA and RT-qPCR using the Primer 5 program described in Table 1 (Li et al., 2018). Fragments of green-fluorescent protein (GFP, KU312311.1) were used as the negative control in the following RNAi experiments.
Gene expression detection
Different time points
The samples collected at 14, 16, 18, 20, 22, 24, 26 and 28 dpi of both genders of worms were used to profile the levels of the target-gene transcript at different time points among the paring stages of S. japonicum for qRT-PCR analysis (Guidi et al., 2015).
Different organs
In order to detect the expression of target genes in different tissues, the female and male worm was dissected into three parts (anterior, ovary and vitellarium) and two parts (testis and posterior), respectively, according to the previous method (Hahnel et al., 2013). The parasites were collected from mice after 28 dpi (Zhu et al., 2016).
Gene knockdown detection
For detection of the knockdown level of the target gene, the harvested six pairs of worms (males and females were separated) were used to extract total RNA for qRT-PCR analysis. The qRT-PCR analysis was carried out in triplicates technical replicates using strictly designed primers. The gene encoding 26S proteasome non-ATPase regulatory subunit 4 gene (SjPSMD) was used as the endogenous standard for each sample. The 2-ΔCt method was used to calculate the relative expression (Li et al., 2018).
RNA interference
dsRNA synthesis
Special specific primers with the T7 promoter were designed to amplify a PCR product of ~500 bp for the target gene. PCR products were used to generate the dsRNA of the target gene using the MEGAscript T7 transcription kit (Catalogue No. AM1334, Ambion) following the manufacturer’s guidelines. End products were checked with agarose gel electrophoresis (Collins and Collins, 2016; Wang et al., 2017). Moreover, a non-specific green fluorescent protein (GFP) sequence was successfully used to produce dsRNA, serving as a negative control (Li et al., 2018).
Schistosomula silencing in vivo
After receiving 60 cercariae, 15 Kunming mice were divided into five groups at random: SPC12, SPC18, SPC22, SPC25 and GFP dsRNA treatment group. Subsequently, each mouse was injected with 10 μg (25 pmol) of dsRNA dissolved in 0.2 ml saline buffer (0.7% NaCl) into the tail vein at the same dose on 1, 6, 10, 14, 18, 22, 26 dpi, respectively (Figure 1A). This injected dose ensures sustained suppression of target gene expression in S. japonicum. Worms were collected at 30 dpi, as described in a previous study (Li et al., 2018).
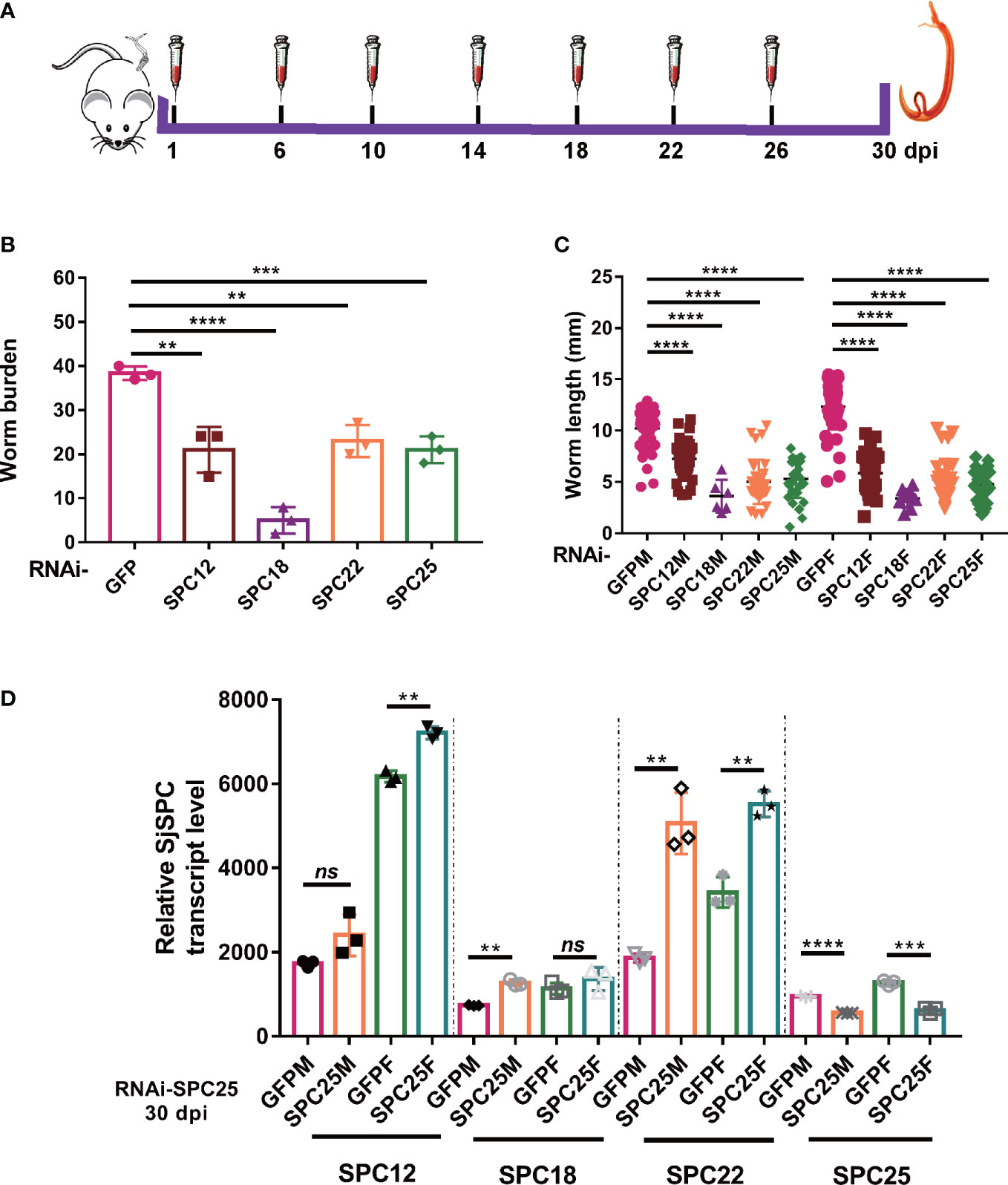
Figure 1 Suppressing the expression of SPC elements dramatical impairing the development and leading to the death of schistosomula. (A) Schematic diagram of the gene interference process of schistosomula in vivo. (B) Assessment of worm burden of dsRNA-mediated RNA effects. (C) Evaluation of worm length recovered from mice at 30 dpi. And worm length data were obtained from all worms recovered in three mice. (D) The expression level of SPC25 and other components of SPC in the RNAi-SPC25 worms. Each group had three biological replicates (three mice), and the data were shown as means ± SEM. **p < 0.01, ***p < 0.001, ****p < 0.0001, ns, not significant, t-test.
Adult worm silencing in vivo
Two groups (RNAi-SPC25 and RNAi-GFP) of 6-week-old female mice infected with 40 ± 2 freshly shed cercariae, with five mice per group. This dose of cercariae infection ensures that no mortality occurs in the experimental mice at 42 dpi. At 26 dpi, 10 μg (25 pmol) of dsRNA was injected into the corresponding groups via the tail vein for the first time, and then additional injections were given every four days (days 26, 30, 34 and 38 post-infection) until 42 dpi (Figure 2A). Harvested parasites were treated similarly, as described above.
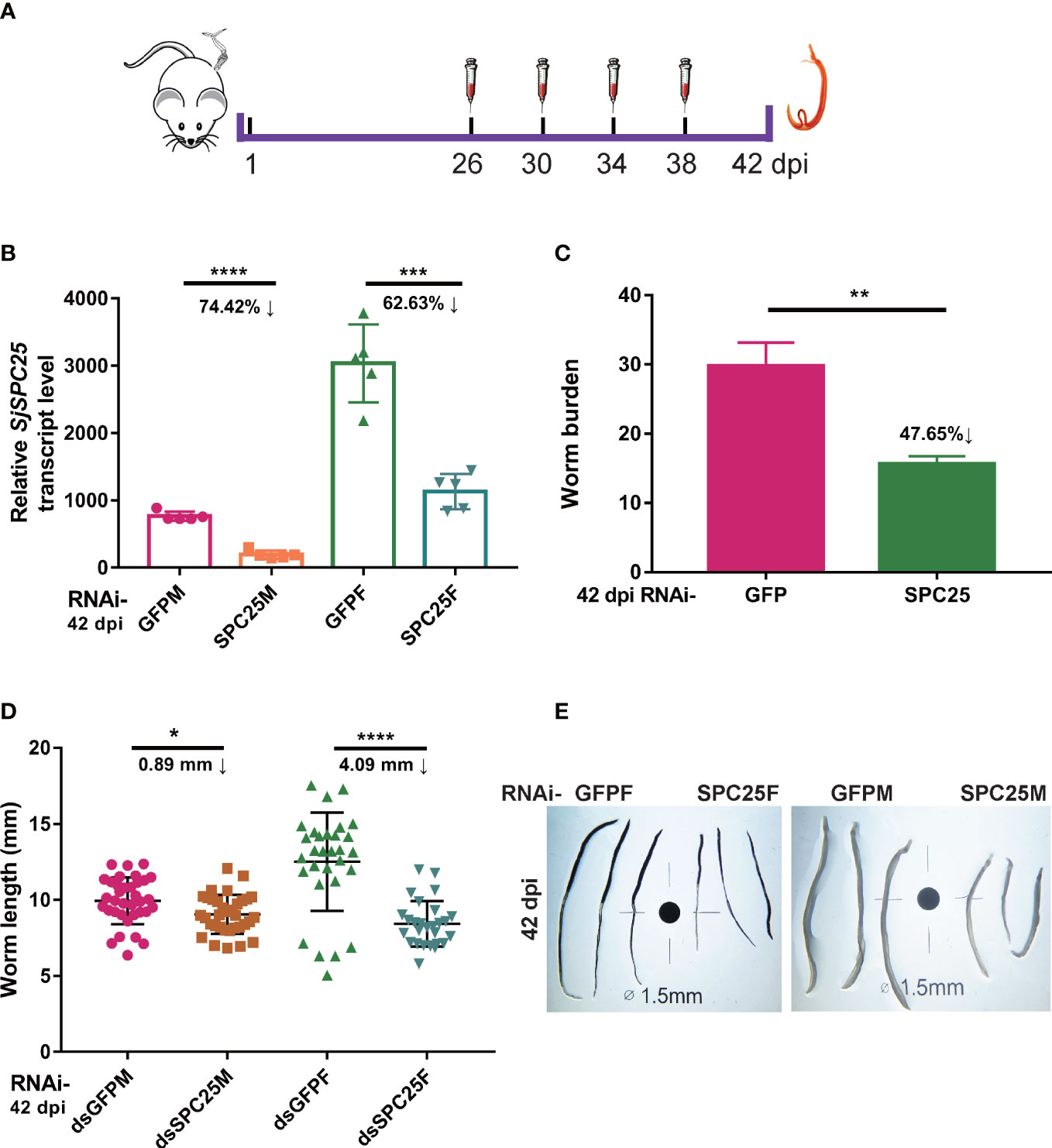
Figure 2 Effection on development and survival of adult S. japonicum. (A) Schematic diagram of the gene interference process of adult worms in vivo. (B) Efficacy assessment of dsRNA-mediated SPC25 knockdown of worm collected at 42 dpi by qRT-PCR. (C) The number of worms collected at 42 dpi from the dsGFP- or dsSPC25-injected mouse. Each group had five biological replicates. (D) Measurement of all worm lengths recovered from five mice in each group at 42 dpi. The data were shown as means ± SEM. (E) Comparison of the length of worms collected at 42 dpi. *p < 0.05, **p < 0.01, ***p < 0.001, ****p < 0.0001, t-test.
RNAi experiments in vitro
At 37°C and 5% CO2, 6 freshly perfused pairs of worms were treated with 10 μg/ml dsRNA in 3 ml medium in a 12-well plate (DMEM, 10% fetal bovine serum, 1% HEPES buffer, 2% penicillin/streptomycin solution). The worms were kept in culture for observation for up to 30 days. The culture was refreshed every other day, and fresh dsRNA was added on days 1, 3, 7, 11, 15, 19, 23 and 27, respectively. During this time, worms were observed daily for motility and viability (Wang et al., 2019; Wang et al., 2020). Samples of treated parasites were collected to access gene knockdown 15 days and 30 days after the dsRNA treatment.
Schistosoma imaging
Worm length
For quantitation of parasite growth, the dsRNA-treated worms were fixed with AFA (95% alcohol, 3% formaldehyde, and 2% glacial acetic acid) and photographed under a Nikon SMZ445 dissecting microscope at 4 X. Then, the captured digital micrographs of worm length and diameter were analyzed using ImageJ software (https://imagej.nih.gov/ij/) and GraphPad Prism 6.0 (Shen et al., 2017).
In situ hybridization
In order to determine the SPC25 mRNA localization, in situ hybridization was performed in S. japonicum. The WISH probe was labeled with digoxin and then purified according to the method provided by the kit (Ambion, Catalogue No. AM1908). Worms were harvested from the infected mice after 30 dpi. Separated female and male worms were fixed with 4% paraformaldehyde dissolved in PBSTX (1X PBS, 0.3% TritonX-100). Subsequently, the fixed parasites were used to present the location of the SPC25 transcript using the WISH method according to what was previously described (Collins et al., 2016; Wang et al., 2019; Wendt et al., 2020).
Mayer’s carmalum
To further evaluate the impact on the worm reproductive organ, the collected worms were stained with Mayer’s carmalum. Briefly, recovered worms were fixed with AFA, and then treated with glacial acetic acid and dehydration in an ethanol gradient. The samples were dyed with 2.5% alumcarmine for 5 minutes, incubated in 5% aluminium potassium sulfate for 15 minutes, and then destained in 70% acidic ethanol. Parasites dehydrated through an ethanol gradient were transferred to methyl salicylate for clarification and mounted with Canada Balsam on glass slides (Shen et al., 2017; Li et al., 2018). Morphometric observations were performed on male and female worms under a light microscope (Nikon 80i) and Laser Scanning Confocal Microscopy (LSCM, Nikon A1-Ni).
Fast Blue B
Fast Blue B staining could be visualized the developmental level of the vitellarium due to the characteristic of strong affinity with the vitelline droplets within the mature vitellarium of female worms. The separated female worms were fixed in 75% alcohol. Then, the samples were washed, stained with 1% Fast Blue B for 1 minute, dehydrated, clarified in ethanol with dimethylbenzene (1:1), and embedded in Canada Balsam. Images of the staining of vitelline cells were collected with a Nikon 80i microscope (Wang et al., 2017).
Liver egg burden, hatchability and tissue histopathology
To provide further evidence of the role of SPC25 in worm fecundity, the worm eggs laid by knockdown parasites were numbered in mouse livers. The liver was recovered from each mouse and then divided into three parts to conduct three investigatory components: one was used for the depositing eggs number calculation, one for egg hatching rate assessment, and another part for granuloma analysis according to the technique previously described (Anderson et al., 2019; Avelar et al., 2019; Cheng et al., 2020).
Egg burden
For egg counting, individuals from the right liver lob were weighed and homogenized into homogenate suspensions, which were then digested with 10 ml 5% NaOH solution overnight at 37°C. The degree of egg burden was determined by microscopic observation based on counting the number of eggs on 7 slides prepared from each liver homogenate, as previously described (Avelar et al., 2019; Cheng et al., 2019).
Egg hatching rate
After verifying the change in liver egg burden, the eggs laid by dsRNA-treated worms were isolated and purified to calculate the hatching rate. To do this, about 200 eggs were divided among 24-well culture plates, and every 30 minutes for up to 4 hours, the number of miracidia that hatched was counted using a light microscope. The miracidia were further fixated with iodine and collected to count as previously described (Wang et al., 2019).
Tissue histopathology
The collected left liver from each mouse was fixed with paraformaldehyde overnight. After dehydration, the tissues were cleared with xylene and embedded in paraffin wax. 4 μm thick sections were prepared and the tissues were stained with hematoxylin and eosin (HE) and Masson staining to evaluate the egg granuloma formation and the deposition of collagen fibers (Luo et al., 2022). At last, the sections were observed under a microscope (Cheng et al., 2020; Hu et al., 2020).
Inhibitor treatment of parasites
6 freshly perfused pair worms were cultured in wells of 12 well plates in 3 ml media. Cultures were supplemented with AEBSF inhibitor (Selleck, China) dissolved in DMSO (Conseil et al., 1999). Different concentrations (10 μM, 5 μM, 2.5 μM, 1 μM) of AEBSF inhibitor were added to the wells and 0.1% DMSO to controls. Cultures and inhibitor were renewed every other day. Three independent biological replicates were used in this experiment.
Statistical analysis
Data are expressed as the mean ± SEM for at least three independent experiments. Different groups of dsRNA treatment were tested for significant differences using Student’s t-test, where P-value ≤ 0.05 was considered statistically significant (Luo et al., 2022). Statistical analyses were performed with GraphPad Prism 6.0.
Results
Silencing of SPC elements led to developmental delay and death in schistosomula
To explore the function of SPC pathway elements in the growth and development of parasites, we used RNAi technology to interfere with target genes. Six injections of dsRNA into mice via the tail vein were used to achieve long-term sustained repression of SPC elements (Figure 1A). After 30 dpi, adult worms were perfused from the hepatic portal system. SPC components knockdown resulted in a significant decrease in the number of adult worms (44.57% for RNAi-SPC12, 86.28% for RNAi-SPC18, 39.99% for RNAi-SPC22, and 45.21% for RNAi-SPC25) recovered from infected mice when compared to unspecific control (Figure 1B). The experimental results confirmed that most of the SPC RNAi-treated worms failed to establish infection in the host. Hence, SPC components are essential for schistosomula to establish infection in hosts.
At the same time, we also found that the growth of worms in the RNAi-SPC component group was significantly delayed. The length of worms showed that schistosomula recovery from the RNAi-SPC25 group in both female and male worms (5.16 ± 0.2781 mm and 4.797 ± 0.2438 mm, respectively) was significantly smaller than that of dsGFP-treated parasites (10.21 ± 0.2083 mm and 12.30 ± 0.2711 mm, respectively). Compared with the control group, worm length was inhibited in other groups, among which the lengths of SPC12 male and female worms were shortened by 29.13% and 52.63%, SPC18 was shortened by 54.57% and 72.40%, SPC22 was shortened by 50.86% and 57.51%, respectively (Figure 1C). Thus, we reasoned that SPC components appear to be essential for schistosome growth and survival in vivo.
Analysis of transcriptome data using different stages of S. japonicum in the laboratory showed that SPC elements have the same expression pattern (Wang et al., 2019). The expression of SPC elements was relatively constant in males and significantly up-regulated in females at 20-26 dpi (Figure S1A). This time is a critical period that the reproductive system develops rapidly in the female worm. In addition, the results of S. japonicum single-cell sequencing showed that the SPC element had the similar cellular localization (unpublished data). The four SPC elements had higher expression in the tegument cell populations and germ cell populations of male and female worms (Figure S1B). Based on the above results, we can infer that SPC elements have similar biological functions.
In order to gain a more profound understanding of the explicit function of SPC elements in S. japonicum, we selected one of the SPC components for further research. Previous studies confirmed that the RNAi-SPC18 group had a worm reduction rate of 86.28%, the number of worms recovered from this experimental group could not be sufficient for the requirement of subsequent experiments. Therefore, we selected SPC25 for further investigation.
Though seven times with dsRNA injection via tail vein, SPC25 mRNA levels, compared to the RNAi-GFP group, were significantly exhausted in both genders, ranging from 41.39% to 51.12% reduction in male and female worms at 30 dpi, respectively (Figure 1D). The results also showed that the expression of other components of SPC was upregulated after the knockdown of SPC25 (Figure 1D). This result shows SPC25-specific dsRNA is effective for SPC25 silencing during the development of worms in vivo.
Expression pattern and localization of the SPC25 transcript in S. japonicum
Gene-expression profiles at different developmental time points
The expression profile of SPC25 was measured at different developmental time points in the male and female worms of S. japonicum using SYBR real-time RT-PCR. The results demonstrated that the SPC25 mRNA had distinctive expression patterns between male and female parasites. We found that SPC25 was highly expressed in the female worm and gradually increased after worm pairing. Moreover, after the pairing of male and female worms, its expression level was relatively stable and slightly decreased in the male (Figure 3A). These results were consistent with the early study about the detailed dynamic transcriptome (Wang et al., 2017).
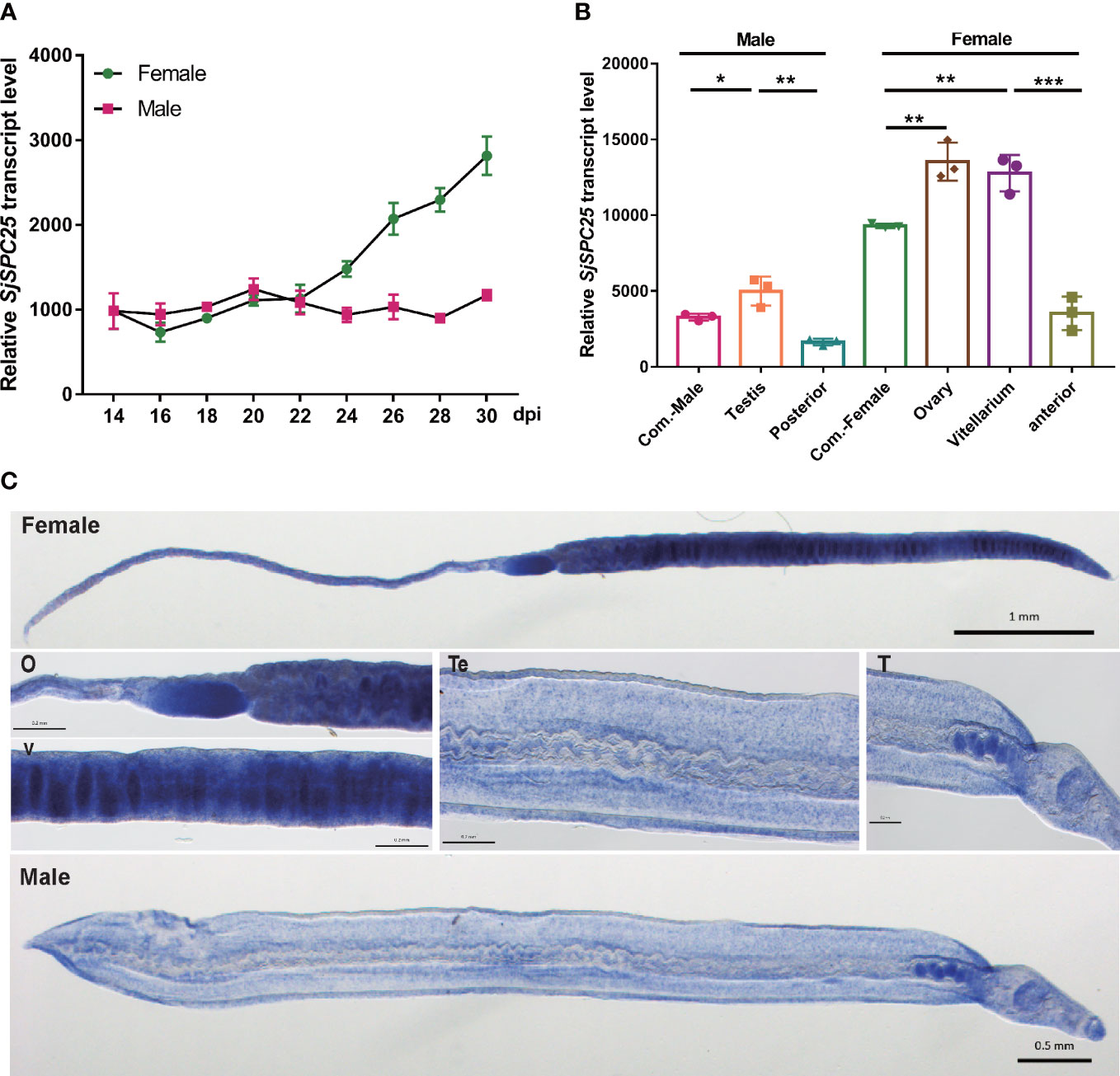
Figure 3 Profiles of the SPC25 transcript level. (A) SPC25 mRNA expression level in male and female worms at different development stages of S.japonicum. (B) The transcript level of SPC25 at isolated reproductive organs. All experiments were performed in triplicate with three biological replicates, and each group’s data were shown as means ± SEM. (C) In situ hybridization analysis of SPC25 in the male and female worms. Te, tegument; T, testis; O, ovary; V, vitellarium. *p < 0.05, **p < 0.01, ***p < 0.001, t-test.
Gene-expression levels in the isolated gonad
To further corroborate the association between SPC25 and worm development, we checked the abundance of the SPC25 gene transcript in different dissected organs of a worm. The results suggested that SPC25 mRNA expression was significantly higher in the ovary and vitellarium of adult female S. japonicum. It was also predominantly expressed in the testes compared with the posterior in the male schistosomes (Figure 3B). We reasoned that SPC25 might be a crucial gene for supporting the further development of reproductive organs.
Whole-mount in situ hybridization
Whole-mount in situ hybridization (WISH) experiments were carried out to clarify the relationship between SPC25 and the reproductive organs of S. japonicum. We observed the transcriptional signals of SPC25 in the ovary and vitellarium of female worms and the tegument and testes of male worms (Figure 3C), indicating that SPC25 may participate in the development of the reproductive system in parasites. These findings agreed with the results obtained through gene-expression detection of worm-dissected organs. Moreover, SPC25 was also observed in tissues other than reproductive organs in S. japonicum. Consistent with the experimental results, the single-cell sequencing data of S.japonicum also showed that the SPC25 gene has a higher expression level in germline stem cells and tegument cells (data unpublished). Based on the specific location of SPC25 transcription in S.japonicum, we next examined the morphology of reproductive organs in the dsRNA-treated parasites.
SPC25 silencing leads to morphological changes of reproductive organ in S. japonicum
In the RNAi-GFP group, the reproductive system exhibited a developmentally mature vitellarium and ovary, and uteruses were filled with eggs in the female worms; the male testicular lobes were loaded with mature spermatocytes. Compared with these, SPC25 silenced parasites had less pigmentation in intestinal tissue, dysplasia in the vitellarium, ovary and testes, and lacked mature germ cells (Figure 4A). The Fast Blue B staining results showed that female worms failed to get mature vitellarium in the RNAi-SPC25 group (Figure 4B).
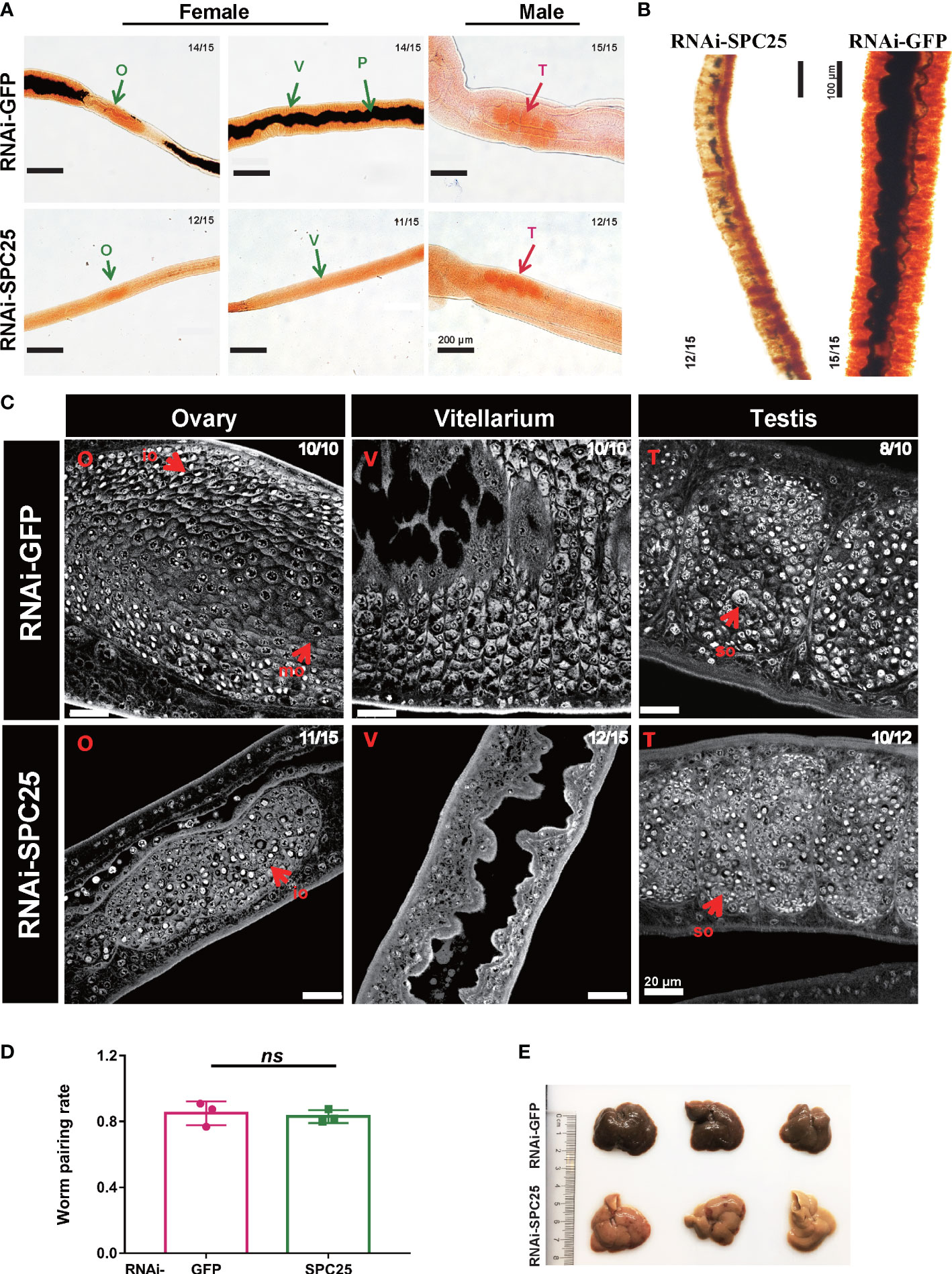
Figure 4 Morphological analysis of the reproductive organ in recovered RNAi-SPC25 worms at 30 dpi. (A) Microscopy images of carmalum staining of S. japonicum. (B) Microscopy images of Fast Blue B staining female worm. Positive Fast Blue B staining indicates mature vitelline cells. (C) Confocal laser scanning micrographs documenting morphological changes in the reproductive organs of RNAi-SPC25 group and control group worms. T, testis; O, ovary; V, vitellarium; P, intestinal pigment deposition; mo, mature oocytes; io, immature oocytes; so, spermatocytes. (D) The pairing rates of RNAi-SPC25 and RNAi-GFP worms. Each group had three biological replicates, and the data were shown as means ± SEM. (E) Liver receiving from the mice injected with dsGFP and dsSPC25. X/Y: number of worms with the same phenotype/number of worms analyzed in one biological replicate. ns, not significant, t-test.
The laser scanning confocal microscopy image provided a qualitative analysis of the changes at the cellular level due to SPC25 knockdown. The size of the testicular lobes displayed a significant reduction, accompanied by rare mature spermatogonia and spermatocytes in the testicular lobes of male worms from the SPC25 dsRNA-treated group (Figure 4C). Moreover, most RNAi-SPC25 female parasites failed to get mature vitellarium and ovary, which has significantly decreased diameters of ovaries or even no ovaries, scantily organized vitelline cells within the vitelline lobes and rare eggs in the uterus (Figure 4C). Moreover, we also found that inhibiting the expression of SPC25 reduced the pathological damage to the host’s liver without affecting the pairing of male and female worms (Figures 4D, E). Mice in the RNAi-SPC25 group had less egg deposition and granuloma in the liver (Figure 4E).
These results about worm morphology provide evidence that SPC25 has a high probability of participating in sexual maturation in schistosome development. Therefore, we next explored the effect of silencing SPC25 on oviposition in mature S.japonicum.
SPC25-knowdown impairs reproductive system of adult parasites in vivo
While defining the effects of SPC25 suppression on the adult worm oviposition, we carried out RNA interference on the parasite from 26 dpi to 42 dpi (Figure 2A). SPC25 was also knockdown in the adult stage of S. japonicum (Figure 2B). Following hepatic portal perfusion, we only recovered about 50% of RNAi-treated worms initially infected (Figure 2C), suggesting RNAi-SPC25 parasites cannot maintain infection in vivo. Measurement of the control and RNAi-SPC25 groups’ worm lengths confirmed that the exhaustion of SPC25 could prevent the further development of adult female worms and had almost no effect on the normal development of adult male worms. In particular, the further growth of adult females was significantly delayed. Compared with the control group, the length of the females in the RNAi-SPC25 group was shortened by 4.09 mm. Inhibiting the expression of SPC25 in mature males had little effect on the development of the worms (Figures 2D, E).
To further analyze the morphological changes of adult worms caused by SPC25 knockdown, the parasites were stained with Fast Blue B staining and Mayer’s carmalum. Compared with controls, we found that adult worms treated with dsSPC25 presented morphologic changes, which mainly reflect the severe degeneration of the female worm’s original mature reproductive organs (vitellarium), accompanied by the size decrease or even loss of vitellarium cells (Figures 5A, B). Consistent with our observations of S. japonicum morphologic changes, the parasite images from confocal microscopy also displayed a severe impairment on the adult female reproductive system, including a high density of immature cells within the ovary and lower density cells within the vitellarium, compared to the RNAi-GFP group (Figure 5C). Whereas, no morphological alterations were observed in the testes of adult survival male worms (Figure 5C). These results showed clear evidence that SPC25 was crucial for maintaining the normal development and morphology of the reproductive organs of female worms.
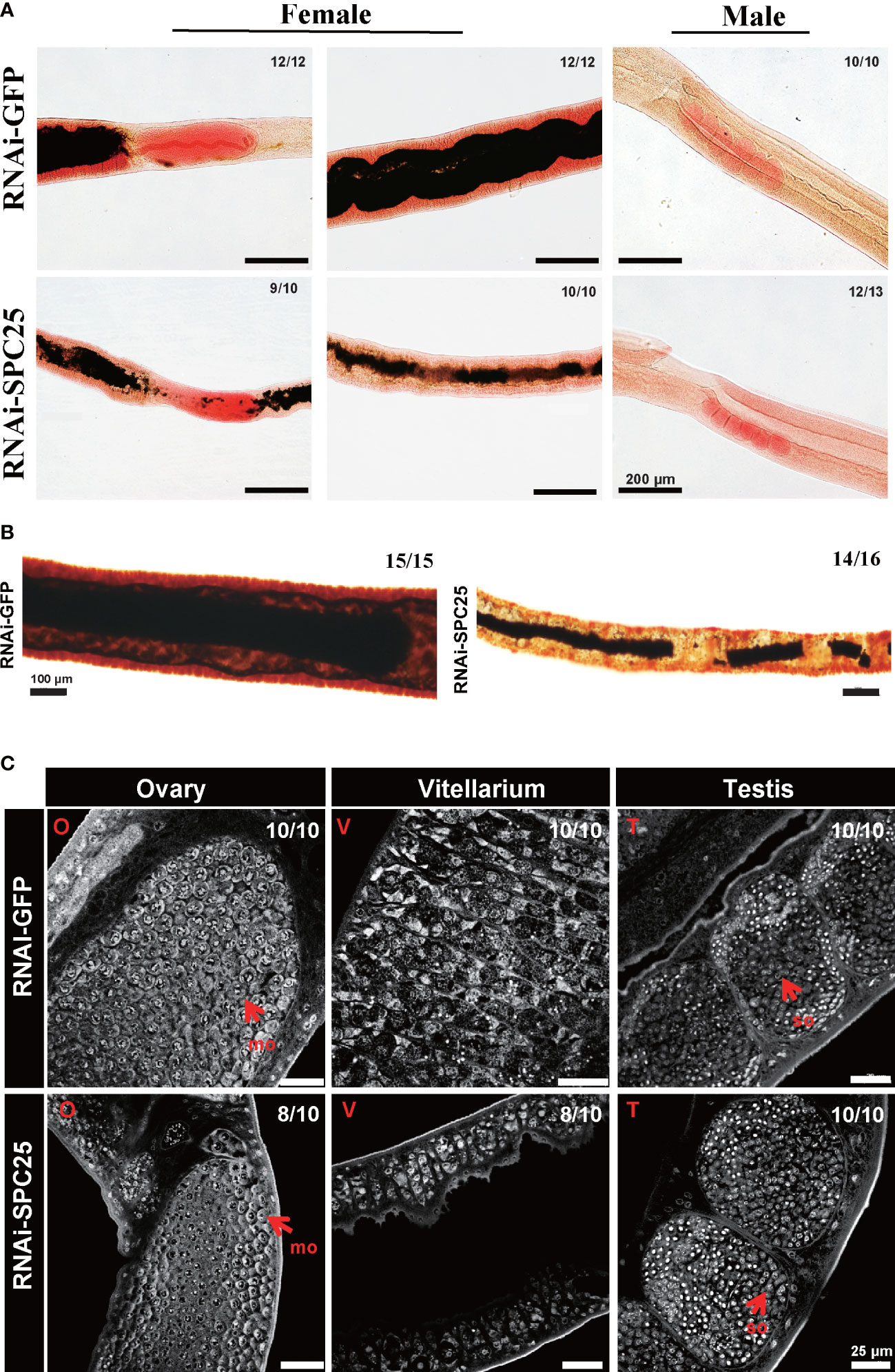
Figure 5 Morphological analysis of the reproductive organ in recovered RNAi-SPC25 worms at 42 dpi. (A) Microscopy images of carmalum staining of S. japonicum. (B) Microscopy images of Fast Blue B staining female worms. Positive Fast Blue B staining indicates mature vitelline cells. (C) Confocal laser scanning micrographs documenting morphological changes in the reproductive organs of RNAi-SPC25 group and control group worms. T, testis; O, ovary; V, vitellarium; P, intestinal pigment deposition; mo, mature oocytes; io, immature oocytes; so, spermatocytes. X/Y: number of worms with the same phenotype/number of worms analyzed in one biological replicate.
The degeneration of female reproductive organs means the reduction of oviposition and weakening pathological damage to the host. Thus, we further explored the reproduction and pathogenicity of RNAi-SPC25 worms.
SPC25-knockdown worms attenuated egg viability and pathogenicity
In the course of studying the effects of SPC25 knockdown on egg production and host pathogenicity, we selected a mouse model infected with SPC25-silenced S.japonicum for 26-42 days (Figure 2A). The experimental results showed that mice injected with dsSPC25 had insignificant hepatosplenomegaly and fewer granulomas on the liver than controls (Figure 6A). The statistical results of the number of oviposition showed that after infection with RNAi-SPC25 worms, the number of eggs in the mouse liver was reduced by 62.02% compared with the control group (Figure 6B). We previously demonstrated that SPC25 expression inhibition results in abnormal ovaries and vitellarium function, leading us to hypothesize that egg motility was impacted. Observing the egg hatching rate showed that nearly 52.80% of eggs laid by RNAi-SPC25 worms were immature, and about 61.20% of eggs from the control group could develop into miracidia (Figure 6C). Results from acridine orange fluorescence staining of eggs were consistent with the hatching rate, which showed a higher proportion of mature eggs in the mouse liver of the RNAi-GFP group than the RNAi-SPC25 group (Figure 6D). As we all know, viable eggs of schistosomes were capable of the formation of granulomas. The weakening of oviposition ability and egg activity means the host’s pathological damage caused by S.japonicum may be alleviated.
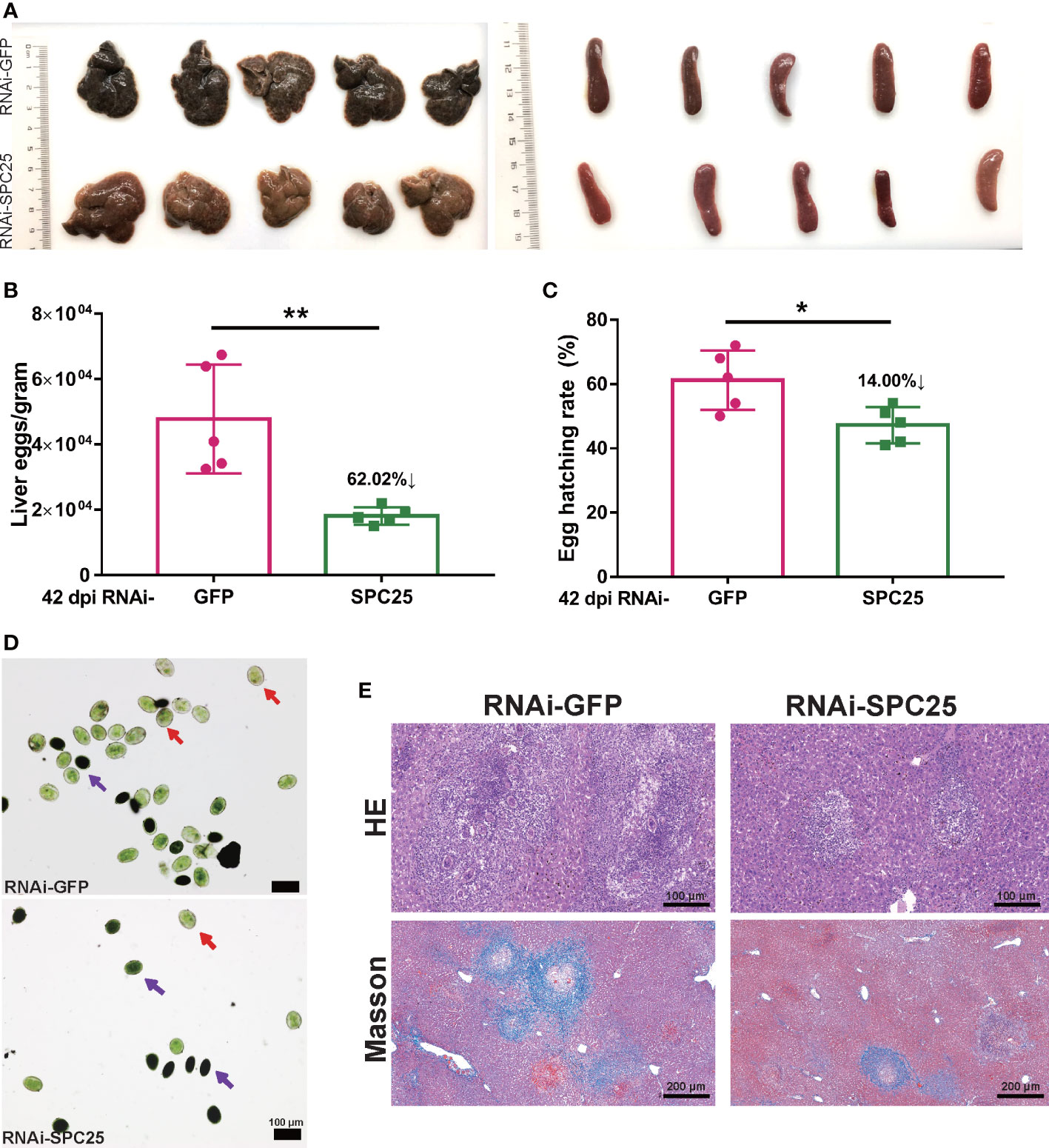
Figure 6 Examination of fecundity and the pathogenicity of RNAi-SPC25 worms. (A) Representative livers and spleens from the five mice per experimental group. (B) Egg number of liver per gram from RNAi-GFP and RNAi-SPC25 group mice on the 42 dpi. (C) Hatching rates of the eggs from the different groups of mice liver. Each group had five biological replicates, and the data were shown as means ± SEM. (D) Acridine orange staining of S. japonicum eggs. Red arrows indicated mature eggs, and purple arrows indicated immature eggs. (E) Histological assessment of mouse liver by HE and Masson staining. Each group had five biological replicates, the images shown were representative experimental results of one mouse. *p < 0.05, **p < 0.01, t-test.
Examination of histological sections from the livers of mice confirmed that eggs of the RNAi-GFP group were capable of generating a larger size of egg-induced granuloma, whereas very small egg-induced granuloma was observed in the RNAi-SPC25 group. According to Masson staining, the RNAi-SPC25 group had significantly less hepatic fibrosis (collagen fibers in blue) than the control group. Moreover, in the RNAi-SPC25 group, the region of hepatic fibrosis created by a single egg was smaller than in the RNAi-GFP group (Figure 6E). Thus, these results also identified our hypothesis that the SPC25 suppression of S. japonicum could reduce the pathological damage to the host.
Inhibition of SPC25 activity results in reduced pairing rate of S. japonicum in vitro
Adult worms were incubated with either the inhibitor or dsRNA to determine whether inhibiting SPC25 activity had an effect on parasite viability in vitro. The efficiency of SPC25 knockdown was detected at 15 days and 30 days using qRT-PCR. We found that SPC25 dsRNA constructs exerted effective transcript level suppression, ranging from 60% to 64% at 15 days and from 65% to 68% compared to controls at 30 days (Figure 7A). After 22 days of treatment, parasites were unable to attach to the surface of cell culture dishes. Partially dsRNA-treated female and male worms became unpaired and had darker bodies after 25 days of culture in vitro (Figure 7B). During this process, we observed that the silencing of SPC25 led to tissue and intestinal edema, muscular hypercontraction. At this time, worms started manifesting muscular and gut vacuolation. After 30 days, some worms showed severe damage and even death, like darker whole bodies (Figure 7B).
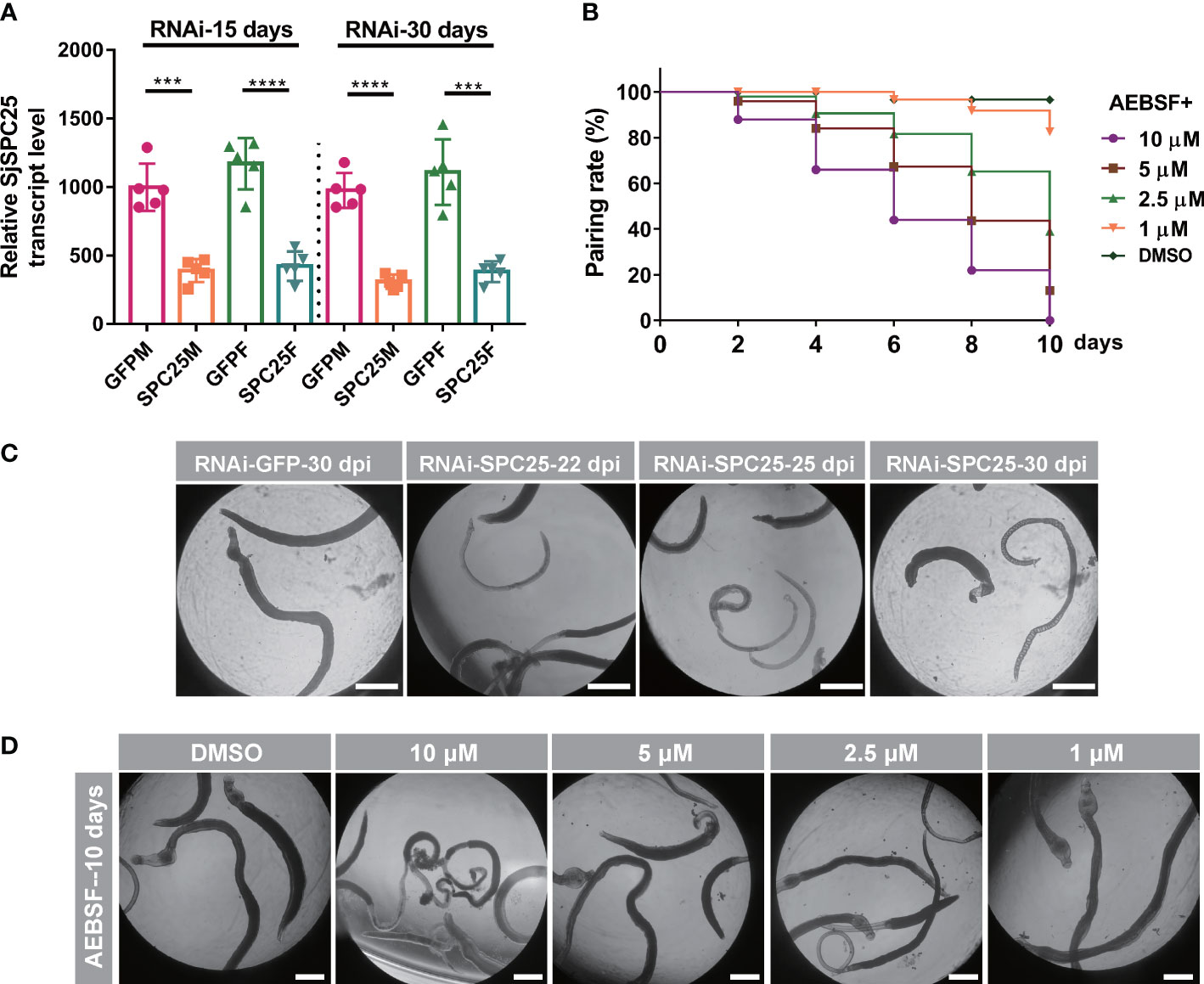
Figure 7 Worm activity analyses of inhibition of SPC25 function in vitro. (A) Efficacy assessment of dsRNA-mediated SPC25 knockdown of worm collected on the 15 dpi and 30 dpi. (B) Effects on worm motility of dsSPC25 treatment. All experiments were performed in triplicate with five biological replicates, and each group’s data were shown as means ± SEM.(C) The pairing rate of the inhibitor ABSEF treated worms in vitro. (D) Detection of visible abnormalities in ABSEF treated worms. Three independent biological replications were performed. And the well had six fresh pairs of worms for each biological experiment. ***p < 0.001, ****p < 0.0001, t-test.
The 4-(2-aminoethyl)-benzenesulfonyl fluoride HCl (AEBSF) is an irreversible serine protease inhibitor that inhibits the protease activity of SPC elements. Moreover, this inhibitor also prevented Toxoplasma gondii invasion of the host cells by specifically affecting parasite targets in a dose-dependent manner (Conseil et al., 1999). Subsequently, adult worms were exposed to four different concentrations (10 µM, 5 µM, 2.5µM and 1 µM). And 2.5 µM was the dose at which more than half of the paired worms were no longer paired, but the viability of male worms was not significantly affected (Figure 7C); 10 µM was the dose at which the worms significantly reduced their viability and were no longer attached to the cell culture dish, and some worms died at least four days after inhibitor exposure (Figure 7D). Consistent with the results of this experiment, when the SPC25 gene interfered in vitro, the worms would weaken the activity and no longer pair on the 22 days, which was consistent with the shape of the worm body after treatment with the inhibitor. The results confirmed that inhibiting the function of SPC25 could prevent the pair and reduce the viability of worms cultured in vitro. However, during experiments of injecting dsRNA or ABSF into mice, it was not found that the pair rate of male and female worms was affected (data not shown).
Discussion
Schistosomiasis, a chronic and potentially lethal tropical disease, is caused by a large number of eggs output from mature female worms deposited in the host liver and intestinal wall, wherein they trigger granuloma formation and other pathological damages (Costain et al., 2018; Hotez et al., 2019; Abou-El-Naga, 2020). If the parasites could be killed or deprived of their ability to egg production in the host, both host pathological disruption and disease transmission will be prevented (deWalick et al., 2012). In the process of analyzing the transcriptome data of S. japonicum, we found a group of exciting genes (signal peptidase complex, SPC) that possess a unique expression pattern that is highly expressed in the developmental stages of the reproductive organ. Meanwhile, recent studies have shown that S. japonicum had some genes silenced that were specifically located in the reproductive organs, which significantly reduced egg production (Beckmann et al., 2010; Wang et al., 2017; Cheng et al., 2019). Moreover, studies of SPC-related genes in other organisms have confirmed that they are crucial to the reproduction and survival of organisms from yeast to mammals. In Drosophila, Spase12 depletion is embryonic lethal and can disrupt the development of all tissues tested, including the eye, wing, leg, and antenna (Haase Gilbert et al., 2013). In addition, SPC18 contributes to progression via TGF-α secretion in gastric cancer, and it is considered an important drug target for cancer treatment (Oue et al., 2013; Oue et al., 2014). Despite the apparent importance of the SPC for multicellular organisms, especially organs or tissues with rapid cell proliferation properties, in vivo studies investigating its function in S. japonicum have yet to be reported. Thus, we attempt to employ the RNA interference (RNAi) platform previously established in our laboratory to define SPC-related gene function (Li et al., 2018; Wang et al., 2022).
In the present study, RNAi-mediated silencing of SPC-related genes in schistosomula showed that SPC knockdown not only caused growth retardation but also reduced the survival rate of the parasites. Interestingly, suppressing the expression of the four SPC components resulted in differences in the worm reduction rate and length shortening. These results may be due to differences in the inhibitory effects of dsRNA on target genes, and of course, it does not rule out differences in the importance of the four elements for SPC (Mullins et al., 1996; Paetzel et al., 2000). After inhibiting SPC18 expression, the worm reduction rate reached 86.28%, and the surviving worms were small. Since the SPC elements had the same expression pattern and location and the expression changes of the remaining elements were consistent after knocking down SPC25, we speculate that the elements have the same biological functions. Meanwhile, the experimental samples obtained from the RNAi-SPC18 group were not conducive to our subsequent gene function research, so we integrated the data of worm length and worm reduction rate and then selected the SPC25 gene for more detailed functional research.
SPC25 expression profiles at different developmental time points and in the reproductive organs of S. japonicum showed that SPC25 might play an important role in parasite reproductive organs. In addition, the WISH results showed that SPC25 has a high expression level in the ovary and vitellarium of female worms, which is consistent with the developmental stage of the ovary and vitellarium in the female worm. These findings implied that inhibiting SPC25 expression may impact the growth of female reproductive organs. As a result, we examined the morphology of the infected worms’ reproductive organs. Moreover, previous studies have shown that the signal peptidase is essential for synthesizing secreted soluble proteins and membrane proteins in bacterial and eukaryotic cells. This enzyme was thought to be a potentially valuable drug target (Paetzel et al., 2000; Harbut et al., 2012; Chen et al., 2018). As a result, effective anti-signal peptidase inhibitors may offer a new class of antiparasitic medications that effectively halt the development of the reproductive system or eradicate the schistosome worm.
In the present study, RNAi-mediated silencing of SPC25 in schistosomula showed that the SPC25 knockdown not only caused growth retardation but also reduced the survival and fecundity of the parasites. Growth delay leading to a lack of adequate resistance of the worm to host clearance may be a significant factor in worm mortality. The SPC25 was significantly overexpressed in the tegument of male worms, and S. japonicum tegument changes are the primary adaptative mechanism for escaping the host’s immune response in several studies (Abath and Werkhauser, 1996; Castro-Borges et al., 2011; Sotillo et al., 2015; Perally et al., 2021). Thus, for the RNAi-treated schistosomula, the inability to settle down might be linked to their immature tegument at 30 dpi (Brouwers et al., 1999). Collectively, these results contributed to our hypothesis that SPC25 is associated with the normal development of schistosomula in vivo. However, it is unclear whether the reason for the attenuated fertility of parasites is the growth retardation or the destruction of reproductive organ function for the RNAi-SPC25 worm. Although the reproductive systems of female parasites showed conspicuous morphological changes, this is not enough to elucidate the association between SPC25 and egg production.
In order to seek the answers to these questions, we conducted RNA interference on the adult parasites. Surprisingly, following hepatic portal perfusion from mice injecting SPC25 dsRNA, we only recovered parasites partly compared with the infected initially. Due to the adult worm possessing complete teguments, the reason for the death of the RNAi-treated parasite might not be similar to that of the schistosomula.
One reason might be that the absence of some excretory/secretory (ES) proteins controlled by SPC25 results in the disability of regulating host immune response, which causes an enhancement of host immune clearance (Duvaux-Miret et al., 1992; Rezende et al., 2004); A possible scenario is that the accumulation of misfolded proteins in the cells caused by the knockdown of SPC25 kills the adult worm (Meyer and Hartmann, 1997; Haase Gilbert et al., 2013; do Patrocinio et al., 2020). Of course, more evidence is required to support this hypothesis. In seeking answers to the mystery of worm death, we found that RNAi-SPC25 leads to a higher death proportion in adult worms than schistosomula and is not fatal for the parasite in vitro culture. Therefore, the death of SPC25-knockdown worm may be partly dependent on the host immunity system.
Another result worth noting is that the reproductive organs of female survival worms appeared to be degenerate, but the testes of male survival worms did not find a distinct alteration. Further evidence about its relationship was presented by staining and confocal images, which showed the exhaustion of SPC25 mRNA-induced disruption in the original, well-developed reproductive organs of the adult female worm. The vitellaria was deprived of orders and regular shapes and presented as a deficiency of reproductive cells or some empty cavity. These deformities caused a significant reduction in the number of eggs laid by the worm. Moreover, the pathological damage to the host was alleviated due to a dramatic decrease in egg deposition in the host’s tissues.
However, why was the morphology of RNAi-SCP25-treated adult males not affected? There may be two reasons for this problem: One cause is that the expression of SPC25 is low in the adult male worm, having a specific resistance to interference, or the knockdown level did not cause abnormal morphology, while the other explains that the existing experimental methods are not enough to detect the phenotypic change in worms. Given the specific phenotype of reproductive organs and survival rates of RNAi-treated worms, we reasoned that SPC25 is essential for maintaining the normal parasitism and fecundity of parasites, at least for the female worm.
Except for the number decreases, the egg granuloma in the mouse liver has shown a contraction in size. The decrease in egg burden causes a relatively mild immune response, which may contribute to the reduction in granuloma size (Li et al., 2021). Besides, the egg viability might be responsible for introducing smaller granulomata in the SPC25 exhaustion group. So, our attention was switched to the study of egg vitality. Consistent with our hypothesis, we found that most eggs laid by RNAi-SPC25 female worms lost the ability to give rise to miracidia. Moreover, the mature eggs may be produced by female worms before treatment with SPC25 dsRNA. Because the size of liver granulomas caused by dead or immature eggs is usually smaller than mature eggs, these results indirectly confirmed the results related to the egg hatching rate (Shen et al., 2017; Forson et al., 2019; Takaki et al., 2020). Thus, the attenuated pathological damage to the host caused by RNAi-SPC25 may be attributed mainly to two aspects: on the one hand, the reduced number of eggs and/or worms decreases the immune stimulation of the host; on the other hand, the impaired reproductive systems of female worms cause reduced egg activity. Whether one factor or a combination of factors plays a role in lessening the pathological damage of S. japonicum eggs for the host, this result is valuable to prevent the transmission of schistosomiasis.
ABSEF (2.5-10 M), a serine protease inhibitor, dramatically resulted in no longer paired male and female parasites, indicating a decrease in parasite vitality. Compared with adding ABSEF, inhibiting the expression of SPC25 in cultured worms for a short period did not achieve the goal of affecting the viability and pairing of worms in vitro culture. Most likely, the inhibitor affects the activity of other serine proteases rather than only preventing the SPC element from performing its function. We will look for more specific small molecule inhibitors of SPC components for further experimental investigation. In addition, mice injected with the inhibitor ABSEF did not gain protection against schistosomiasis. Further experiments will be performed to explain this result.
Conclusion
In this study, we characterized the SPC biology function, which is proven to play an indispensable role in the growth and survival of Schistosoma in vivo. The exhaustion of SPC25 mRNA can deprive the ability of fecundity, survival, and egg vitality for parasites. Moreover, the inhibitor ABESF can be used as a candidate small molecule drug against schistosomiasis. More importantly, our finding provided a new strategy to research the gene function associated with fecundity in the definitive host and contributed to revealing a novel class of molecular drug target candidates against schistosomiasis, providing new therapeutic opportunities.
Data availability statement
The original contributions presented in the study are included in the article/Supplementary Material. Further inquiries can be directed to the corresponding author.
Ethics statement
The animal study was reviewed and approved by Ethics Committee of the National Institute of Parasitic Diseases, Chinese Center for Disease Control and Prevention in Shanghai, China (IPD2020-10).
Author contributions
WH conceived the project, designed the experiments, and critically revised the manuscript. W-BY, FL and WZ performed the experiments and analyzed the data. W-BY drafted the manuscript. CT, AZ and C-SS helped in the implementation of the experiment. All authors contributed to the article and approved the submitted version.
Funding
This work has been supported by funding from the National Natural Science Foundation of China (grant no. 31725025), Shanghai Municipal Science and Technology Committee of Shanghai outstanding academic leaders plan (grant no. 18XD1400400), Natural Science Foundation of Anhui Province (grant no. 2008085QH430) and Science and Technology Leading Talent Team plan (grant no. 2022SLJRC0023).
Acknowledgments
We are grateful to Jian Li from Department of infectious diseases, Huashan Hospital, State Key Laboratory of Genetic Engineering, Ministry of Education Key Laboratory for Biodiversity Science and Ecological Engineering, Ministry of Education Key Laboratory of Contemporary Anthropology, School of Life Sciences, Fudan University for the platform construction of RNAi screening in vivo.
Conflict of interest
The authors declare that the research was conducted in the absence of any commercial or financial relationships that could be construed as a potential conflict of interest.
Publisher’s note
All claims expressed in this article are solely those of the authors and do not necessarily represent those of their affiliated organizations, or those of the publisher, the editors and the reviewers. Any product that may be evaluated in this article, or claim that may be made by its manufacturer, is not guaranteed or endorsed by the publisher.
Supplementary material
The Supplementary Material for this article can be found online at: https://www.frontiersin.org/articles/10.3389/fcimb.2023.1136056/full#supplementary-material
Supplementary Figure 1 | Expression patterns of SPC elements in S. japonicum. (A) Dynamic expression profiles of SPC12, SPC18, SPC22 and SPC25 in transcriptome sequencing data (mean± SEM, n=3). (B) SPC elements boxplots showing gene expression levels in populations of S.japonicum.
References
Abath, F. G., Werkhauser, R. C. (1996). The tegument of Schistosoma mansoni: functional and immunological features. Parasit. Immunol. 18, 15–20. doi: 10.1046/j.1365-3024.1996.d01-6.x
Abou-El-Naga, I. F. (2020). Heat shock protein 70 (Hsp70) in Schistosoma mansoni and its role in decreased adult worm sensitivity to praziquantel. Parasitology 147, 634–642. doi: 10.1017/S0031182020000347
Anderson, E. C., Frankino, P. A., Higuchi-Sanabria, R., Yang, Q., Bian, Q., Podshivalova, K., et al. (2019). X Chromosome domain architecture regulates caenorhabditis elegans lifespan but not dosage compensation. Dev. Cell 51, 192–207.e196. doi: 10.1016/j.devcel.2019.08.004
Avelar, L., Gava, S. G., Neves, R. H., Silva, M. C. S., Araujo, N., Tavares, N. C., et al. (2019). Smp38 MAP kinase regulation in Schistosoma mansoni: Roles in survival, oviposition, and protection against oxidative stress. Front. Immunol. 10, 21. doi: 10.3389/fimmu.2019.00021
Beckmann, S., Buro, C., Dissous, C., Hirzmann, J., Grevelding, C. G. (2010). The syk kinase SmTK4 of Schistosoma mansoni is involved in the regulation of spermatogenesis and oogenesis. PloS Pathog. 6, e1000769. doi: 10.1371/journal.ppat.1000769
Brindley, P. J., Sher, A. (1987). The chemotherapeutic effect of praziquantel against Schistosoma mansoni is dependent on host antibody response. J. Immunol. 139, 215–220. doi: 10.4049/jimmunol.139.1.215
Brindley, P. J., Strand, M., Norden, A. P., Sher, A. (1989). Role of host antibody in the chemotherapeutic action of praziquantel against Schistosoma mansoni: identification of target antigens. Mol. Biochem. Parasitol. 34, 99–108. doi: 10.1016/0166-6851(89)90001-7
Brouwers, J. F., Skelly, P. J., Van Golde, L. M., Tielens, A. G. (1999). Studies on phospholipid turnover argue against sloughing of tegumental membranes in adult Schistosoma mansoni. Parasitology 119 (Pt 3), 287–294. doi: 10.1017/S0031182099004679
Buszczak, M., Signer, R. A. J., Morrison, S. J. (2014). Cellular differences in protein synthesis regulate tissue homeostasis. Cell 159, 242–251. doi: 10.1016/j.cell.2014.09.016
Castro-Borges, W., Simpson, D. M., Dowle, A., Curwen, R. S., Thomas-Oates, J., Beynon, R. J., et al. (2011). Abundance of tegument surface proteins in the human blood fluke Schistosoma mansoni determined by QconCAT proteomics. J. Proteomics 74, 1519–1533. doi: 10.1016/j.jprot.2011.06.011
Cheever, A. W., Macedonia, J. G., Mosimann, J. E., Cheever, E. A. (1994). Kinetics of egg production and egg excretion by Schistosoma mansoni and S. japonicum in mice infected with a single pair of worms. Am. J. Trop. Med. Hyg. 50, 281–295. doi: 10.4269/ajtmh.1994.50.281
Chen, Y., Murillo-Solano, C., Kirkpatrick, M. G., Antoshchenko, T., Park, H. W., Pizarro, J. C. (2018). Repurposing drugs to target the malaria parasite unfolding protein response. Sci. Rep. 8, 10333. doi: 10.1038/s41598-018-28608-2
Cheng, G., Li, X., Qin, F., Xu, R., Zhang, Y., Liu, J., et al. (2019). Functional analysis of the Frzb2 gene in Schistosoma japonicum. Vet. Res. 50, 108–108. doi: 10.1186/s13567-019-0716-1
Cheng, W. J., Gu, M. J., Ye, F., Zhang, Y. D., Zhong, Q. P., Dong, H. F., et al. (2020). Prohibitin 1 (PHB1) controls growth and development and regulates proliferation and apoptosis in Schistosoma japonicum. FASEB J. 34 (8), 11030–11046. doi: 10.1096/fj.201902787RRR
Cioli, D., Pica-Mattoccia, L., Basso, A., Guidi, A. (2014). Schistosomiasis control: praziquantel forever? Mol. Biochem. Parasitol. 195, 23–29. doi: 10.1016/j.molbiopara.2014.06.002
Collins, J. J., 3rd., Wendt, G. R., Iyer, H., Newmark, P. A. (2016). Stem cell progeny contribute to the schistosome host-parasite interface. Elife 5, e12473. doi: 10.7554/eLife.12473.017
Collins, J. N., Collins, J. J., 3rd (2016). Tissue degeneration following loss of Schistosoma mansoni cbp1 is associated with increased stem cell proliferation and parasite death In vivo. PloS Pathog. 12, e1005963. doi: 10.1371/journal.ppat.1005963
Conseil, V., Soête, M., Dubremetz, J. F. (1999). Serine protease inhibitors block invasion of host cells by Toxoplasma gondii. Antimicrob Agents Chemother. 43, 1358–1361. doi: 10.1128/AAC.43.6.1358
Costain, A. H., Macdonald, A. S., Smits, H. H. (2018). Schistosome egg migration: Mechanisms, pathogenesis and host immune responses. Front. Immunol. 9, 3042. doi: 10.3389/fimmu.2018.03042
deWalick, S., Tielens, A. G., Van Hellemond, J. J. (2012). Schistosoma mansoni: the egg, biosynthesis of the shell and interaction with the host. Exp. Parasitol. 132, 7–13. doi: 10.1016/j.exppara.2011.07.018
do Patrocinio, A. B., Cabral, F. J., Bitencourt, A. L. B., Brigato, O. M., Magalhães, L. G., De Lima Paula, L. A., et al. (2020). Inhibition of 19S proteasome deubiquitinating activity in Schistosoma mansoni affects viability, oviposition, and structural changes. Parasitol. Res. 119 (7), 2159–2176. doi: 10.1007/s00436-020-06686-4
Duvaux-Miret, D., Stefano, G. B., Smith, E. M., Mallozzi, L. A., Capron, A. (1992). Proopiomelanocortin-derived peptides as tools of immune evasion for the human trematode Schistosoma mansoni. Acta Biol. Hung. 43, 281–286.
Eyayu, T., Zeleke, A. J., Worku, L. (2020). Current status and future prospects of protein vaccine candidates against Schistosoma mansoni infection. Parasit. Epidemiol. Control 11, e00176. doi: 10.1016/j.parepi.2020.e00176
Forson, P. O., Tetteh-Quarcoo, P. B., Ahenkorah, J., Aryee, R., Okine, E. N., Afutu, E., et al. (2019). Ability of vital and fluorescent staining in the differentiation of Schistosoma haematobium live and dead eggs. Med. Sci. (Basel) 7 (4), 64. doi: 10.3390/medsci7040064
Guidi, A., Mansour, N. R., Paveley, R. A., Carruthers, I. M., Besnard, J., Hopkins, A. L., et al. (2015). Application of RNAi to genomic drug target validation in schistosomes. PloS Negl. Trop. Dis. 9, e0003801. doi: 10.1371/journal.pntd.0003801
Haase Gilbert, E., Kwak, S.-J., Chen, R., Mardon, G. (2013). Drosophila signal peptidase complex member Spase12 is required for development and cell differentiation. PloS One 8, e60908–e60908. doi: 10.1371/journal.pone.0060908
Hahnel, S., Lu, Z., Wilson, R. A., Grevelding, C. G., Quack, T. (2013). Whole-organ isolation approach as a basis for tissue-specific analyses in Schistosoma mansoni. PloS Negl. Trop. Dis. 7, e2336–e2336. doi: 10.1371/annotation/7aad0212-557d-4121-a72f-da2c115ce534
Hambrook, J. R., Hanington, P. C. (2020). Immune evasion strategies of schistosomes. Front. Immunol. 11, 624178. doi: 10.3389/fimmu.2020.624178
Han, Z. G., Brindley, P. J., Wang, S. Y., Chen, Z. (2009). Schistosoma genomics: new perspectives on schistosome biology and host-parasite interaction. Annu. Rev. Genomics Hum. Genet. 10, 211–240. doi: 10.1146/annurev-genom-082908-150036
Harbut, M. B., Patel, B. A., Yeung, B. K., Mcnamara, C. W., Bright, A. T., Ballard, J., et al. (2012). Targeting the ERAD pathway via inhibition of signal peptide peptidase for antiparasitic therapeutic design. Proc. Natl. Acad. Sci. U.S.A. 109, 21486–21491. doi: 10.1073/pnas.1216016110
Hotez, P. J., Engels, D., Gyapong, M., Ducker, C., Malecela, M. N. (2019). Female genital schistosomiasis. N. Engl. J. Med. 381, 2493–2495. doi: 10.1056/NEJMp1914709
Hu, Y., Wang, X., Wei, Y., Liu, H., Zhang, J., Shen, Y., et al. (2020). Functional inhibition of natural killer cells in a BALB/c mouse model of liver fibrosis induced by Schistosoma japonicum infection. Front. Cell Infect. Microbiol. 10, 598987. doi: 10.3389/fcimb.2020.598987
Ittiprasert, W., Mann, V. H., Karinshak, S. E. (2019). Programmed genome editing of the omega-1 ribonuclease of the blood fluke, Schistosoma mansoni. Elife 8, e41337. doi: 10.7554/eLife.41337
Kalies, K. U., Romisch, K. (2015). Inhibitors of protein translocation across the ER membrane. Traffic 16, 1027–1038. doi: 10.1111/tra.12308
Kuntz, A. N., Davioud-Charvet, E., Sayed, A. A., Califf, L. L., Dessolin, J., Arner, E. S., et al. (2007). Thioredoxin glutathione reductase from Schistosoma mansoni: an essential parasite enzyme and a key drug target. PloS Med. 4, e206. doi: 10.1371/journal.pmed.0040206
Le Clec'h, W., Chevalier, F. D., Mattos, A. C. A., Strickland, A., Diaz, R., Mcdew-White, M., et al. (2021). Genetic analysis of praziquantel response in schistosome parasites implicates a transient receptor potential channel. Sci. Transl. Med. 13, eabj9114. doi: 10.1126/scitranslmed.abj9114
Li, J., Xiang, M., Zhang, R., Xu, B., Hu, W. (2018). RNA Interference in vivo in Schistosoma japonicum: Establishing and optimization of RNAi mediated suppression of gene expression by long dsRNA in the intra-mammalian life stages of worms. Biochem. Biophys. Res. Commun. 503, 1004–1010. doi: 10.1016/j.bbrc.2018.06.109
Li, X., Qiao, H., Qin, F., Cheng, G., Liu, J., Li, H., et al. (2020). Comparative analysis of iTRAQ-based proteome profiles of Schistosoma japonicum female worms coming from single-sex infections and bisexual infections. J. Proteomics 213, 103597. doi: 10.1016/j.jprot.2019.103597
Li, Z., Zhang, W., Luo, F., Li, J., Yang, W., Zhu, B., et al. (2021). Allergen-specific treg cells upregulated by lung-stage S. japonicum infection alleviates allergic airway inflammation. Front. Cell Dev. Biol. 9, 678377. doi: 10.3389/fcell.2021.678377
Luo, F., Yang, W., Yin, M., Mo, X., Pang, Y., Sun, C., et al. (2022). A chromosome-level genome of the human blood fluke Schistosoma japonicum identifies the genomic basis of host-switching. Cell Rep. 39, 110638. doi: 10.1016/j.celrep.2022.110638
Meyer, H. A., Hartmann, E. (1997). The yeast SPC22/23 homolog Spc3p is essential for signal peptidase activity. J. Biol. Chem. 272, 13159–13164. doi: 10.1074/jbc.272.20.13159
Mullins, C., Meyer, H. A., Hartmann, E., Green, N., Fang, H. (1996). Structurally related Spc1p and Spc2p of yeast signal peptidase complex are functionally distinct. J. Biol. Chem. 271, 29094–29099. doi: 10.1074/jbc.271.46.29094
Oue, N., Hayashi, T., Ito, R., Nagatsuma, A., Sentani, K., Sakamoto, N., et al. (2013). SEC11A contributes to malignant progression through promotion of EGF and TGF-α secretion in gastric cancer. Cancer Res. 73, 412. doi: 10.1158/1538-7445.am2013-412
Oue, N., Naito, Y., Hayashi, T., Takigahira, M., Kawano-Nagatsuma, A., Sentani, K., et al. (2014). Signal peptidase complex 18, encoded by SEC11A, contributes to progression via TGF-α secretion in gastric cancer. Oncogene 33, 3918–3926. doi: 10.1038/onc.2013.364
Paetzel, M., Dalbey, R. E., Strynadka, N. C. (2000). The structure and mechanism of bacterial type I signal peptidases. a novel antibiotic target. Pharmacol. Ther. 87, 27–49. doi: 10.1016/S0163-7258(00)00064-4
Perally, S., Geyer, K. K., Farani, P. S. G., Chalmers, I. W., Fernandez-Fuentes, N., Maskell, D. R., et al. (2021). Schistosoma mansoni venom allergen-like protein 6 (SmVAL6) maintains tegumental barrier function. Int. J. Parasitol. 51, 251–261. doi: 10.1016/j.ijpara.2020.09.004
Rezende, C. M., Goes, T. S., Goes, V. S., Azevedo, V., Leite, M. F., Goes, A. M. (2004). GM-CSF and TNF-alpha synergize to increase in vitro granuloma size of PBMC from humans induced by Schistosoma mansoni recombinant 28-kDa GST. Immunol. Lett. 95, 221–228. doi: 10.1016/j.imlet.2004.07.015
Schwartz, C., Fallon, P. G. (2018). Schistosoma "Eggs-iting" the host: Granuloma formation and egg excretion. Front. Immunol. 9, 2492. doi: 10.3389/fimmu.2018.02492
Shelness, G. S., Lin, L., Nicchitta, C. V. (1993). Membrane topology and biogenesis of eukaryotic signal peptidase. J. Biol. Chem. 268, 5201–5208. doi: 10.1016/S0021-9258(18)53520-1
Shen, J., Lai, D. H., Wilson, R. A., Chen, Y. F., Wang, L. F., Yu, Z. L., et al. (2017). Nitric oxide blocks the development of the human parasite Schistosoma japonicum. Proc. Natl. Acad. Sci. U.S.A. 114, 10214–10219. doi: 10.1073/pnas.1708578114
Sotillo, J., Pearson, M., Becker, L., Mulvenna, J., Loukas, A. (2015). A quantitative proteomic analysis of the tegumental proteins from Schistosoma mansoni schistosomula reveals novel potential therapeutic targets. Int. J. Parasitol. 45, 505–516. doi: 10.1016/j.ijpara.2015.03.004
Sun, J., Li, C., Wang, S. (2015). The up-regulation of ribosomal proteins further regulates protein expression profile in female Schistosoma japonicum after pairing. PloS One 10, e0129626–e0129626. doi: 10.1371/journal.pone.0129626
Takaki, K. K., Rinaldi, G., Berriman, M., Pagán, A. J., Ramakrishnan, L. (2020). Schistosoma mansoni eggs modulate the timing of granuloma formation to promote transmission. Cell Host Microbe 29 (1), 58–67.e5. doi: 10.1101/2020.04.14.040626
Wang, J., Chen, R., Collins, J. J., 3rd (2019). Systematically improved in vitro culture conditions reveal new insights into the reproductive biology of the human parasite Schistosoma mansoni. PloS Biol. 17, e3000254. doi: 10.1371/journal.pbio.3000254
Wang, J., Yu, Y., Shen, H., Qing, T., Zheng, Y., Li, Q., et al. (2017). Dynamic transcriptomes identify biogenic amines and insect-like hormonal regulation for mediating reproduction in Schistosoma japonicum. Nat. Commun. 8, 14693. doi: 10.1038/ncomms14693
Wang, J., Paz, C., Padalino, G., Coghlan, A., Lu, Z., Gradinaru, I., et al. (2020). Large-Scale RNAi screening uncovers therapeutic targets in the parasite Schistosoma mansoni. Science 369, 1649–1653. doi: 10.1126/science.abb7699
Wang, X., Cheng, S., Chen, X., Zhang, W., Xie, Y., Liu, W., et al. (2022). A metabotropic glutamate receptor affects the growth and development of Schistosoma japonicum. Front. Microbiol. 13, 1045490. doi: 10.3389/fmicb.2022.1045490
Wendt, G., Zhao, L., Chen, R., Liu, C., O'donoghue, A. J., Caffrey, C. R., et al. (2020). A single-cell RNA-seq atlas of schistosoma mansoni identifies a key regulator of blood feeding. Science 369, 1644–1649. doi: 10.1126/science.abb7709
Zeng, F., Yi, C., Zhang, W., Cheng, S., Sun, C., Luo, F., et al. (2022). A new ferritin SjFer0 affecting the growth and development of Schistosoma japonicum. Parasit. Vectors 15, 177. doi: 10.1186/s13071-022-05247-1
Keywords: S. japonicum, SPC, SPC25, RNAi, development, reproduction
Citation: Yang W-B, Luo F, Zhang W, Sun C-S, Tan C, Zhou A and Hu W (2023) Inhibition of signal peptidase complex expression affects the development and survival of Schistosoma japonicum. Front. Cell. Infect. Microbiol. 13:1136056. doi: 10.3389/fcimb.2023.1136056
Received: 02 January 2023; Accepted: 20 February 2023;
Published: 03 March 2023.
Edited by:
Patricia Flavia Quaresma, Federal University of Santa Catarina, BrazilCopyright © 2023 Yang, Luo, Zhang, Sun, Tan, Zhou and Hu. This is an open-access article distributed under the terms of the Creative Commons Attribution License (CC BY). The use, distribution or reproduction in other forums is permitted, provided the original author(s) and the copyright owner(s) are credited and that the original publication in this journal is cited, in accordance with accepted academic practice. No use, distribution or reproduction is permitted which does not comply with these terms.
*Correspondence: Wei Hu, aHV3QGZ1ZGFuLmVkdS5jbg==