- 1Faculty of Business and Economics, University of Lausanne, Lausanne, Switzerland
- 2Institute of Microbiology, Lausanne University and University Hospital of Lausanne, Lausanne, Switzerland
- 3Division of Pulmonology, Department of Medicine, Lausanne University Hospital, University of Lausanne, Lausanne, Switzerland
- 4Infectious Diseases Service, Lausanne University and University Hospital of Lausanne, Lausanne, Switzerland
Background: The microbial diagnosis of tuberculosis (TB) remains challenging and relies on multiple microbiological tests performed on different clinical specimens. Polymerase chain reactions (PCRs), introduced in the last decades has had a significant impact on the diagnosis of TB. However, questions remain about the use of PCRs in combination with conventional tests for TB, namely microscopy and culture. We aimed to determine the performance of microscopy, culture and PCR for the diagnosis of pulmonary tuberculosis according to the type of clinical specimen in order to improve the diagnostic yield and to avoid unnecessary, time and labor-intensive tests.
Methods: We conducted a retrospective study (2008-2018) on analysis (34’429 specimens, 14’358 patients) performed in our diagnostic laboratory located in the Lausanne University Hospital to compare the performance of microbiological tests on sputum, induced sputum, bronchial aspirate and bronchoalveolar lavage (BAL). We analysed the performance using a classical “per specimen” approach and a “per patient” approach for paired specimens collected from the same patient.
Results: The overall sensitivities of microscopy, PCR and culture were 0.523 (0.489, 0.557), 0.798 (0.755, 0.836) and 0.988 (0.978, 0.994) and the specificity were 0.994 (0.993, 0.995), 1 (0.999, 1) and 1 (1, 1). Microscopy displayed no significant differences in sensitivity according to the type of sample. The sensitivities of PCR for sputum, induced sputum, bronchial aspirate and BAL were, 0.821 (0.762, 0.871), 0.643 (0.480, 0.784), 0.837 (0.748, 0.904) and 0.759 (0.624, 0.865) respectively and the sensitivity of culture were, 0.993 (0.981, 0.998), 0.980 (0.931, 0.998), 0.965 (0.919, 0.988), and 1 (0.961, 1) respectively. Pairwise comparison of specimens collected from the same patient reported a significantly higher sensitivity of PCR on bronchial aspirate over BAL (p < 0.001) and sputum (p < 0.05) and a significantly higher sensitivity of culture on bronchial aspirate over BAL (p < 0.0001).
Conclusions: PCR displayed a higher sensitivity and specificity than microscopy for all respiratory specimens, a rational for a smear-independent PCR-based approach to initiate tuberculosis microbial diagnostic. The diagnosis yield of bronchial aspirate was higher than BAL. Therefore, PCR should be systematically performed also on bronchial aspirates when available.
1 Introduction
With over ten million new cases in 2020 and about 1,5 million deaths, tuberculosis represents a major public health concern (WHO, 2021a). Rapid and reliable diagnosis is important to reduce morbidity and mortality associated with tuberculosis and to control transmission. When tuberculosis is suspected based on clinical symptoms, epidemiological information and radiological findings, microbial confirmation is key to establish the diagnosis. Despite progress during the last decades, the microbiological diagnosis of tuberculosis continues to be a challenge particularly in paucibacillary disease. Historically, the diagnosis of tuberculosis was based on microscopy and culture. Mycobacterial culture represents the reference method due to a low limit of detection (< 10 organisms for liquid cultures) and because it gives access to the strain for phenotypic antibiotic susceptibility test (van Zyl-Smit et al., 2011; WHO, 2021a). However, culture is challenging due to the slow growth of Mycobacterium tuberculosis and because it requires biosafety level three (BSL3) laboratories (Palomino, 2005; Pfyffer and Wittwer, 2012). Microscopy based on the visualization of acid-fast bacilli provides rapid results (<30 minutes) but has a limited sensitivity and specificity (limit of detection between 103 and 104 bacilli per ml) (Opota et al., 2019b). In order to increase their sensitivity, these tests may need to be repeated over several clinical specimen (Boehme et al., 2010; Campelo et al., 2021; WHO, 2021a).
More recently, molecular diagnosis, in particular polymerase chain reaction (PCR) have improved the diagnosis of tuberculosis with a limit of detection between 10 and 103 colony forming units per ml and a turnaround time between two to six hours (Boehme et al., 2010; van Zyl-Smit et al., 2011; Opota et al., 2016; WHO, 2021b). PCR was initially available in laboratories specialized in molecular diagnostics, through methods developed in-house assays (Greub et al., 2016; WHO, 2021c). Commercial all-inclusive systems, such as the GeneXpert system, now allow a greater number of laboratories to perform this analysis independently of a specialized infrastructure (Boehme et al., 2010; Opota et al., 2016; Dorman et al., 2018; WHO, 2021b). The GeneXpert system not only improved the initial diagnostic of tuberculosis but can be used to assess patient’s infectious potential, on the basis of the semi-quantitative results (van Zyl-Smit et al., 2011; Opota et al., 2016; Opota et al., 2019b). In addition, rapid molecular test also shortens airborne isolation for hospitalized patients with presumptive tuberculosis (Lippincott et al., 2014)
More than 70% of the tuberculosis infections are pulmonary tuberculosis, for which sputum is the usual specimen collected in adults and older children who are able to collaborate. Other respiratory specimens can be considered when patients are not able to provide sputum or to increase microbial diagnostic yield (WHO, 2021c). This includes induced sputum, obtained by nebulization of sterile hypertonic saline (3% or 7% saline solution inhaled) followed by coughing and expectoration of airway secretions, bronchial aspirate, and bronchoalveolar lavages (Schaaf and Reuter, 2009; Weiszhar and Horvath, 2013).
In this study, we aimed to assess the performance of the different tests for the microbial diagnostic of tuberculosis according to the type of clinical specimen. Providing robust updated data may enable to choose optimal combination of test and specimen to: i) reach the maximum sensitivity, specificity and negative and predicative value, ii) prioritize the tests and specimens in the situation of limited resources or shortage of material and iii) reduce unnecessary costs.
There is no standard method to address the performance of diagnostic tests, particularly for tuberculosis where several microbiological tests on multiple clinical samples are frequently required. We applied data analytics methods that integrate multiple parameters, including, the type of microbiological test, the type of specimens, the sampling period, and the patients. In this study, we performed both a classical “per specimen” method to determine the performance of each diagnostic test and a “per patient” approach comparing paired specimens collected from the same patient.
This study provides data to establish diagnostic stewardship guidelines and diagnostic protocols. These data will help to establish more effective strategies to diagnose pulmonary tuberculosis in order to increase the rate of documentation, to accelerate the diagnosis and avoid unnecessary testing. In addition, it should provide analytical strategies that may also be suitable to study other infectious diseases while keeping associated medical, social and economic costs to a strict minimum.
2 Material and methods
2.1 Study design and data
Our laboratory is located in the Lausanne University Hospital (CHUV), a 1’500 beds tertiary-care hospital in a low-tuberculosis-prevalence country (Switzerland), with approximately six new cases per year per 100,000 population (Federal Office of Public Health; http://www.bag.admin.ch/). The data included microbiology analyses for patients with suspected mycobacterial infection from 2008 to 2018. They were automatically extracted from the Laboratory Information System (MOLIS, CGM).
For all specimens, information regarding the microbial diagnostic of mycobacteria were extracted. This included information regarding microscopy, PCR, cultures, molecular and phenotypic resistance genes together with the type of specimen and the date of collection. Each specimen was given a unique coded number. Similarly, each patient was given a unique coded number. The database was generated to allow analysis by date of sampling and by patients (see Section 2.4). For the microbial diagnostic of the disease, we generated a composite gold standard including microbiological findings and epidemiological and clinical data (see Section 2.3). The initial database included 34’429 specimens corresponding to 14’358 patients, including 8’587 sputum, 2’257 induced sputum, 8’610 bronchial aspirate and 4’576 bronchoalveolar lavages (BAL). Demographic data that correspond to the distribution of ages at first consultation by gender and TB status are presented in Figure 1.
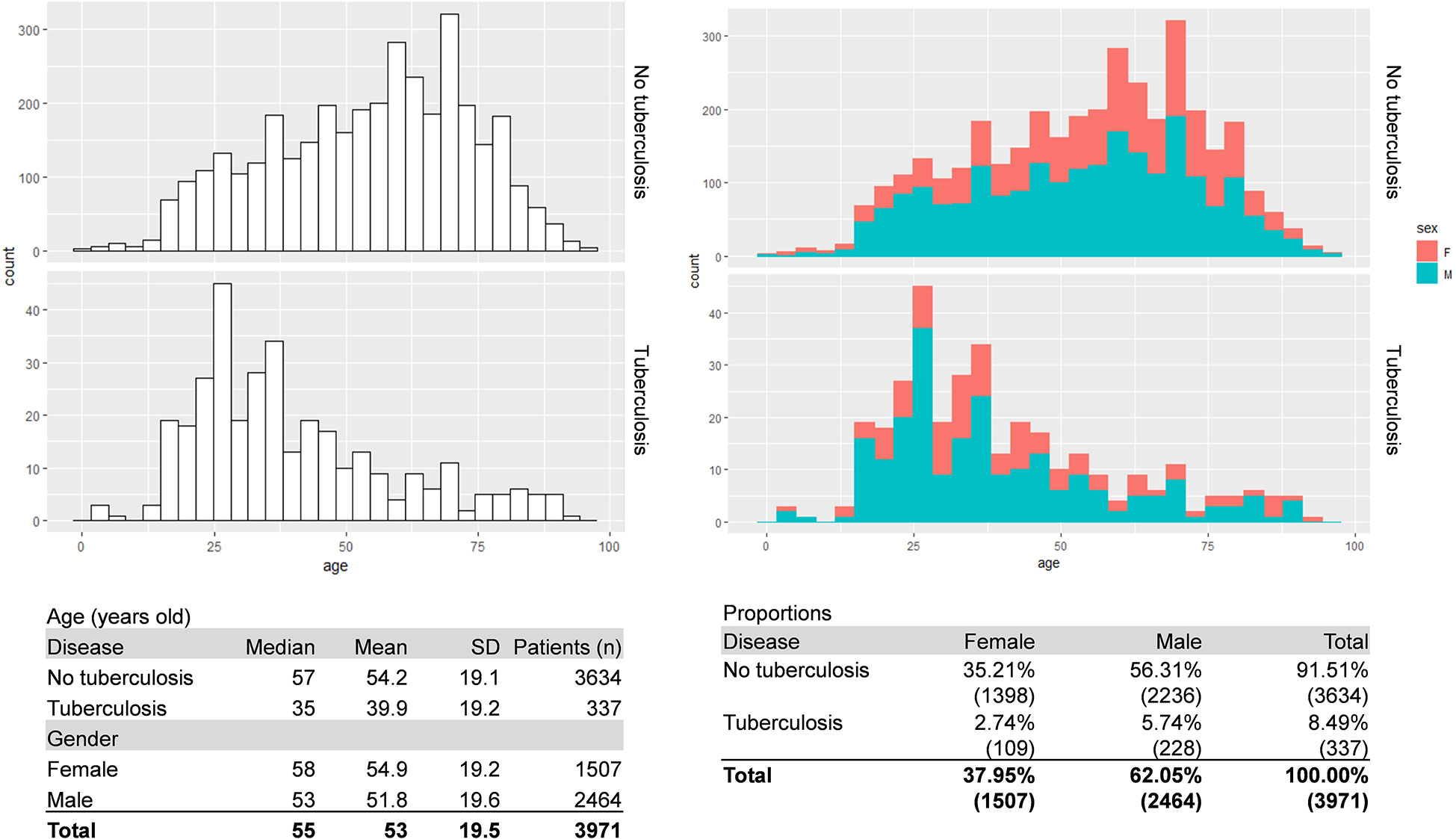
Figure 1 Patient’s demographic. The figure shows the patient’s distribution of ages at first consultation by gender and disease status. A composite gold standard based on microbiological results and clinical data was used for disease status.
2.2 Clinical specimens and mycobacterial diagnostic test
This study focuses on three microbial diagnostic tests commonly used for the diagnostic of mycobacterial infections, namely (i) microscopy (also named smear microscopy) for the direct detection of acid-fast bacilli (AFB) in clinical specimen, (ii) PCR for the detection of DNA of M. tuberculosis complex directly from clinical specimen and (iii) mycobacterial culture. Microscopy consists in acid-fast bacillus staining achieved through a fluorescent auramine-thiazine red staining on a heat-fixed smear as described in (Opota et al., 2016). PCR consisted of either an in-house TaqMan PCR targeting the multicopy M. tuberculosis IS6110 sequence, named PCR “MYTU” (Greub et al., 2016) or using the all-inclusive rapid molecular test Xpert MTB/RIF and Xpert MTB/RIF Ultra (herafter named Xpert) (Cepheid, Ca, USA) (Boehme et al., 2010; Opota et al., 2016; Chakravorty et al., 2017). Mycobacterial culture was achieved using mycobacterial growth indicator tube (MGIT) that consists in culture tubes containing tris 4, 7-diphenyl-1, 10-phenonthroline ruthenium chloride pentahydrate, an oxygen-quenched fluorochrome embedded in silicone at their bottom. Utilization of free oxygen for growing bacteria alleviates the fluorochrome quenching, resulting in fluorescence within the tube that can be visualized under UV light, as explained in a previous publication (Opota et al., 2016). Antibiotic resistance was determined using a combination of molecular and phenotypic testing as previously described (Opota et al., 2016); overall ten strains of 296 positive cultures (3.37%) exhibited rifampicin resistant.
All the microbial analyses were performed on the same sample after splitting it for AFB staining, Xpert analysis and mycobacterial culture (Opota et al., 2016). Sputum, induced sputum, bronchial aspirate and bronchoalveolar lavage were processed as previously described (Opota et al., 2016). In particular, samples with a volume exceeding 3 mL were concentrated by centrifugation (30 minutes, 3000 g). In addition, to increase the homogeneity of the sample before smear preparation, purulent sputum or bronchial aspirates were solubilized with the mucolytic agent N-acetyl-L-cysteine (2% v/v pH 6.8) (Opota et al., 2016).
2.3 Composite gold standard
Mycobacterial culture is the gold standard for the diagnosis of M. tuberculosis because of its lowest limit of detection (LOD < 10 organisms). However, it can be impaired by situation that affect mycobacterial growth such as the introduction of an antibiotic treatment before sampling (van Zyl-Smit et al., 2011). We therefore used a composite gold standard based on microbiological results and clinical data. Discrepant results in the diagnostic of active tuberculosis, especially specimen with positive MTBC PCR and negative culture for M. tuberculosis complex, were manually cured based on clinical and epidemiological data found in medical records. Specimens for which the culture was contaminated by bacteria of the flora were excluded. Specimens with culture positive with nontuberculous mycobacteria qualified as “MOTT” (Mycobacteria other than tuberculosis) were considered negative for M. tuberculosis. This resulted in the “Gold Standard” (GS) reference.
2.4 Performance of the test depending on the clinical specimens and statistical methods
We used two different approaches to determine the performance of microbiological tests depending on the clinical specimens. We first used a common “per specimen” approach to determine the global performance of microscopy, PCR, and culture according to the four types of specimens and independently of the patient using the GS as reference. Then, in order to provide more robust data we performed a “per patient” approach. It consists in pairwise comparison for specimens collected the same day or during a window of 72 hours for the same patient; indeed, samples from the same patient might not be collected the same day. For each patient, the first sample was paired to the following sample if it was of a different type and within a 72-hour window. All the combinations of the four types of specimens were analyzed using this pairwise approach i) to measure the dependence between each pair of types of specimens and ii) to calculate the performance of one sample type to predict a positive of any of the two sample types.
For both approaches, the performance measures include accuracy, sensitivity, specificity, positive and negative predictive values (PPV and NPV). They were computed using the gold-standard (GS) as reference. Their respective 95% confidence intervals were computed using the Clopper–Pearson method. The comparisons of proportions were assessed with a two-sided proportion test with Yates’ continuity correction. The dependence between pairs of types of specimens for the “per patient” approach was measured by the Cohen’s Kappa.
2.5 Ethics committee approval
This study was approved by the relevant ethics committee, the Commission Cantonale d’Éthique de la Recherche sur l’Être Humain (CER-2020-00136).
3 Results
3.1 Global performance of microscopy, PCR and culture for the diagnostic of pulmonary tuberculosis
We first determined the global performance of microscopy. The sensitivity, 0.523 (0.489 – 0.557) and the PPV, 0.767 (0.730, 0.800) of microscopy were limited. The specificity, 0.994 (0.993, 0.995), and the NPV 0.982 (0.981, 0.984) were high but must be interpreted according to the low prevalence of microscopy positive specimen 0.036 (0.034, 0.038) (Tables 1; S1).
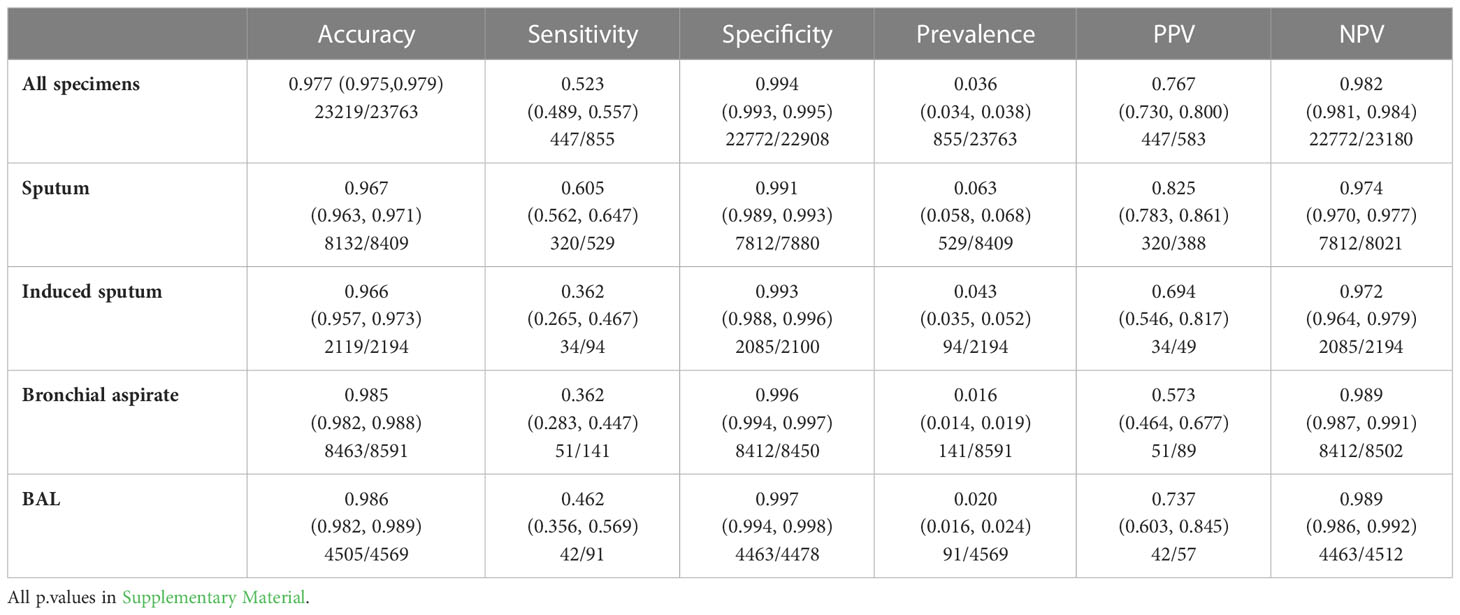
Table 1 Performance of smear microscopy for the diagnostic of pulmonary tuberculosis according of the type of specimen.
Regarding PCR, we first estimated the individual performance of the in-house TaqMan PCR and the rapid molecular test Xpert. The sensitivity of the in-house TaqMan PCR, 0.799 (0.743, 0.848) and Xpert 0.812 (0.760, 0.858) were not significantly different (Tables S2, S3). The specificity for both the in-house TaqMan PCR >0.999 (0.999, 1) and Xpert 0.999 (0.997, 1) were both very high. The NPV were also high but probably increased by the low prevalence of PCR positive specimen (Table S7). Because the two PCR tests displayed similar performance, we considered them as equal for the rest of the study and grouped them as “PCR”. The global performance of PCR were: sensitivity 0.798 (0.755, 0.836), specificity 1 (0.999, 1), PPV 0.997 (0.983, 1) and NPV 0.988 (0.985, 0.990) (Tables 2; S2).
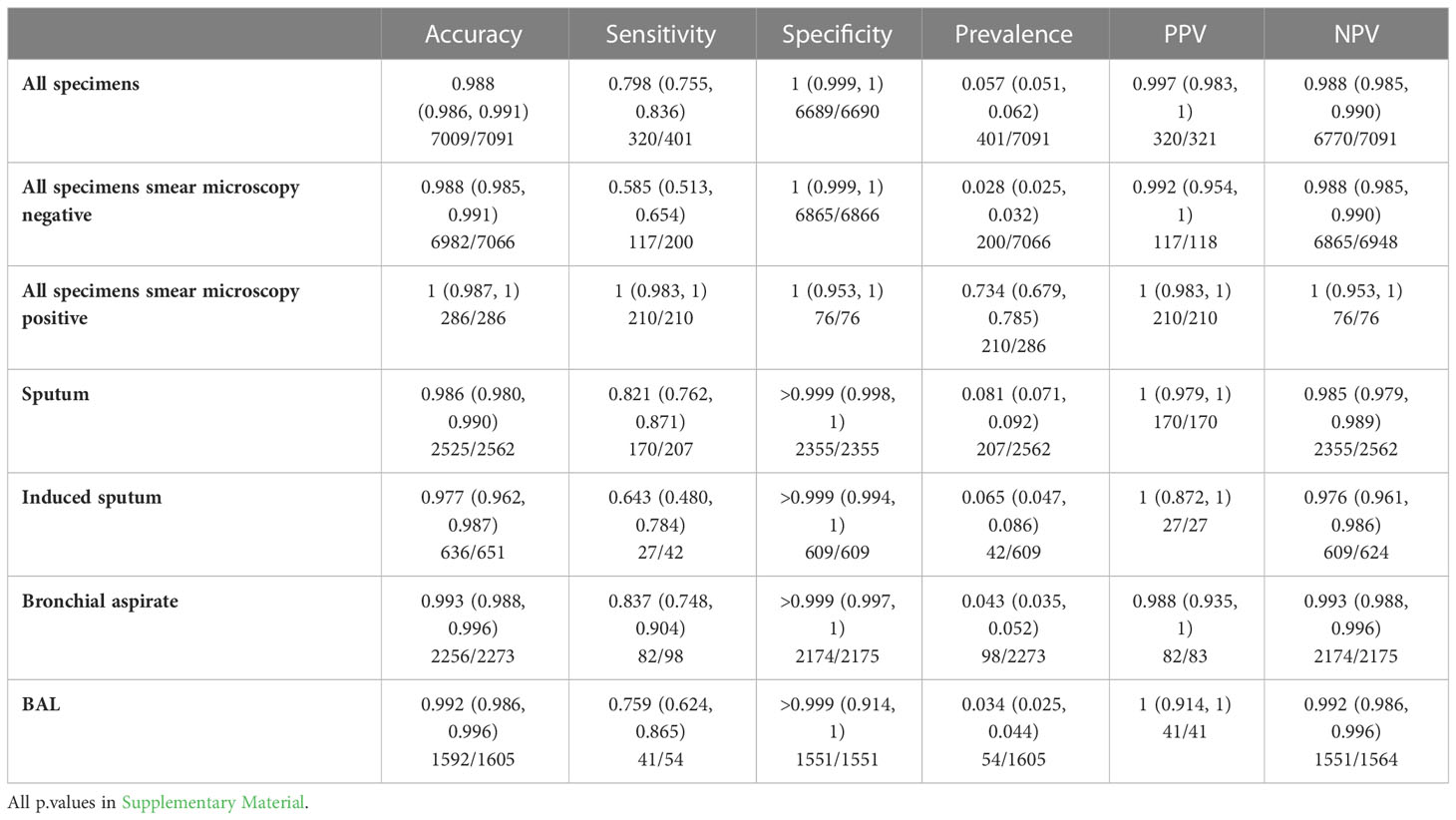
Table 2 Performance of PCR for the diagnostic of pulmonary tuberculosis according to the type of specimen.
The culture displayed the highest performance for the diagnostic of tuberculosis using the GS reference with a sensitivity of 0.988 (0.978, 0.994) and a specificity of 1 (1, 1) (Tables 3; S3). In summary when considering all the clinical specimen, microscopy displays limited sensitivity, PCR displays a higher sensitivity than microscopy and an excellent specificity and culture displayed the highest sensitivity and specificity.
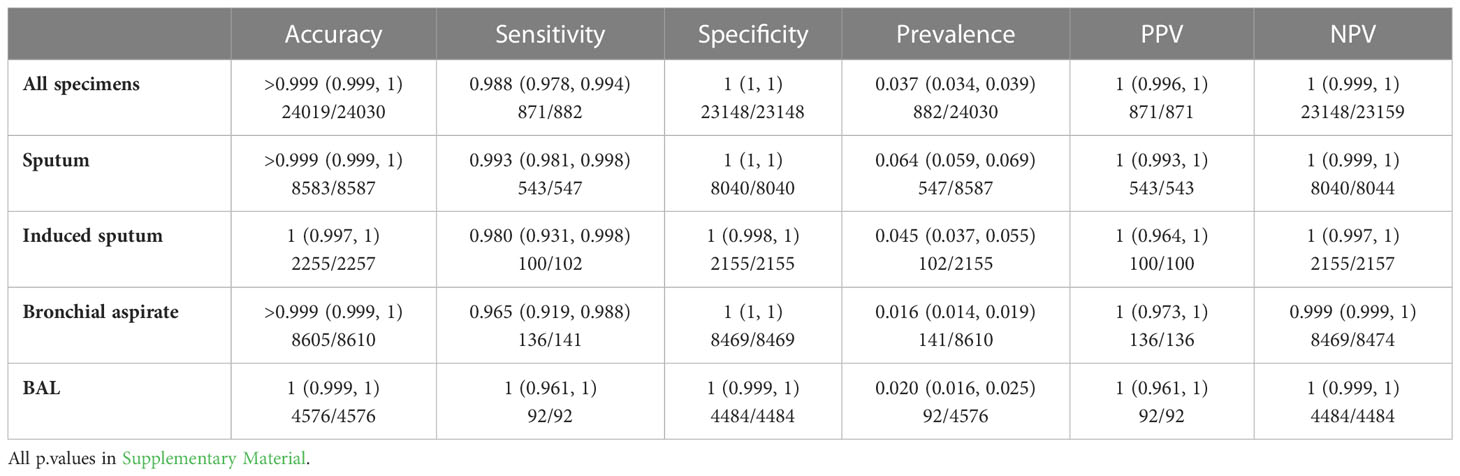
Table 3 Performance of culture for the diagnostic of pulmonary tuberculosis according to the type of specimen.
3.2 Performance of microbiological tests according to the type of specimen using a “per sample” approach or using “per patient” pairwise comparisons.
We next measured the dependence between the specimens using the “per patient” approach and the Cohen’s kappa. Though the Cohen’s kappa is itself difficult to interpret and the confidence intervals shown in the table were not corrected for multiple comparisons, a large value of kappa means that the two sample bring the same information to some extent and thus provides little “complementary” information and are so-called “supllementary”, i.e not really needed. Conversely, a kappa close to zero means that the two sample are “complementary”. The results are shown in Tables S8–S11. The data suggested that for each technique, microscopy, PCR, and culture, most samples are supplementary (i.e. not all needed), except i) for microscopy, induced sputum versus bronchial aspirate and BAL, ii) for PCR, sputum versus induced sputum and iii) for the culture, induced sputum versus BAL. Because of their low robustness, we do not want to over interpret these results by concluding that the so-called “supplementary” tests don’t need to be performed, but this question has to be tackled in additional work since it is however to our knowledge the first study showing such high dependence & redundancy of the various tests.
3.3 Performance of microscopy according to the type of respiratory specimen
Using the classical “per specimen” approachthe sensitivities of microscopy for all specimen, sputum, induced sputum, bronchial aspirate and BAL were 0.523 (0.489, 0.557), 0.605 (0.562, 0.647), 0.362 (0.265, 0.467), 0.362 (0.283, 0.447) and 0.462 (0.356, 0.569) respectively. The sensitivity of sputum was higher than induced sputum (p-value < 0.0001) and bronchial aspirate (p-value < 0.0001) (Tables 4; S4). However, using the “per patient” comparison approach, for paired specimens collected within a window of 72h for the same patient, no significant difference was observed for the various specimens (Tables 4; S4, S9). Altogether, these data suggested a limited benefit of microscopy for tuberculosis microbial diagnosis in a low prevalence setting.
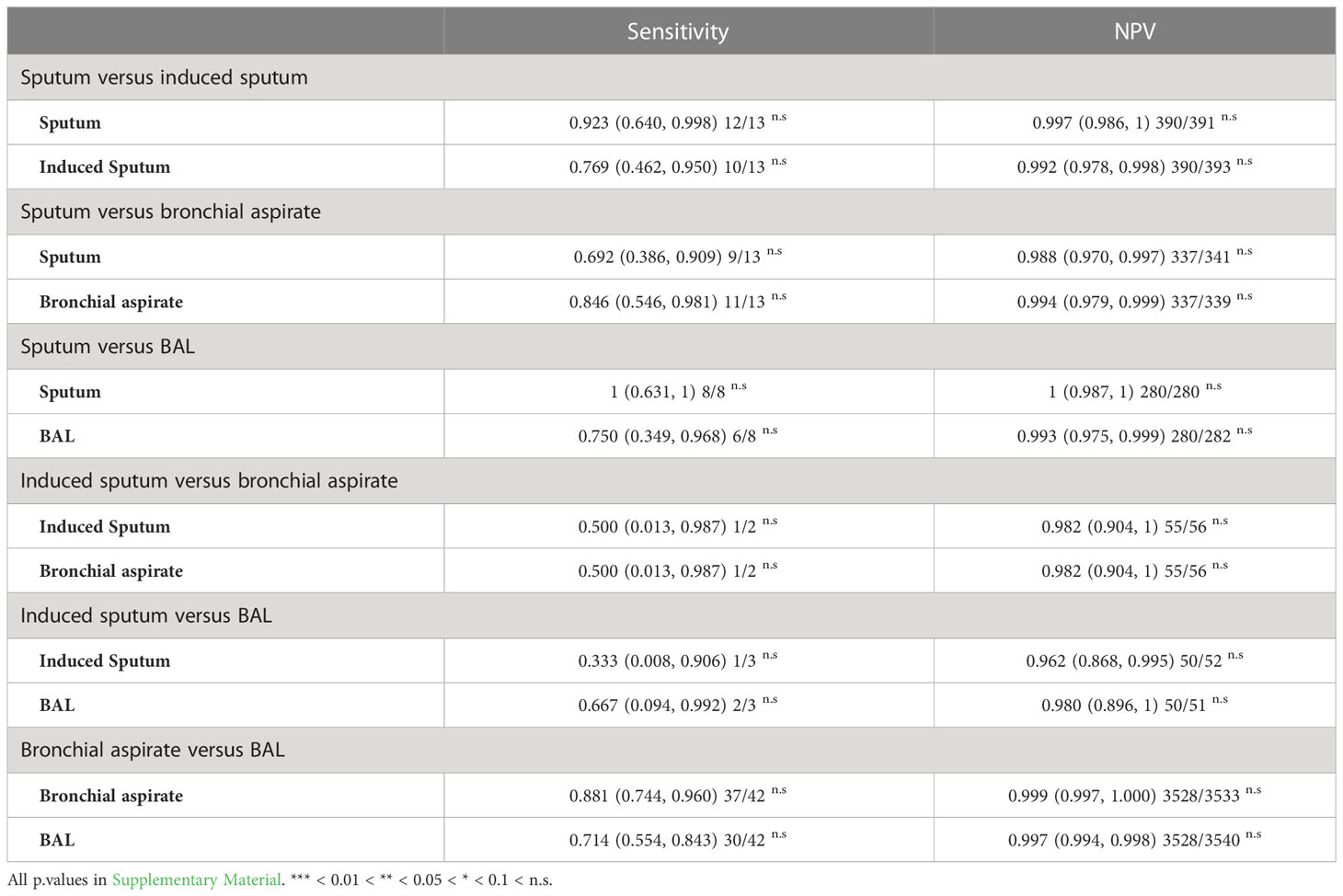
Table 4 Sensitivity of microscopy to predict tuberculosis according to the type of specimen using a 72-hours pairing window in the same patient.
3.4 Performance of PCR according to the type of respiratory specimen.
Using the classical “per specimen” approachthe sensitivities of PCR for all specimen, sputum, induced sputum, bronchial aspirate and BAL were 0.798 (0.755, 0.836), 0.821 (0.762, 0.871), 0.643 (0.480, 0.784), 0.837 (0.748, 0.904) and 0.759 (0.624, 0.865) respectively. Using the “per patient” pairwise comparison approach, no significant difference in sensitivity was seen between sputum and induced sputum. Using this approach, we found that the sensitivity of bronchial aspirate, was significantly higher than BAL with respectively 0.974 (0.865, 0.999) and 0.564 (0.396, 0.722) (p. value 0.0003). The sensitivity of bronchial aspirate was also higher than sputum with respectively 1 (0.753, 1) versus 0.385 (0.139, 0.684) (p-value = 0.017) (Tables 5; S5, S10).
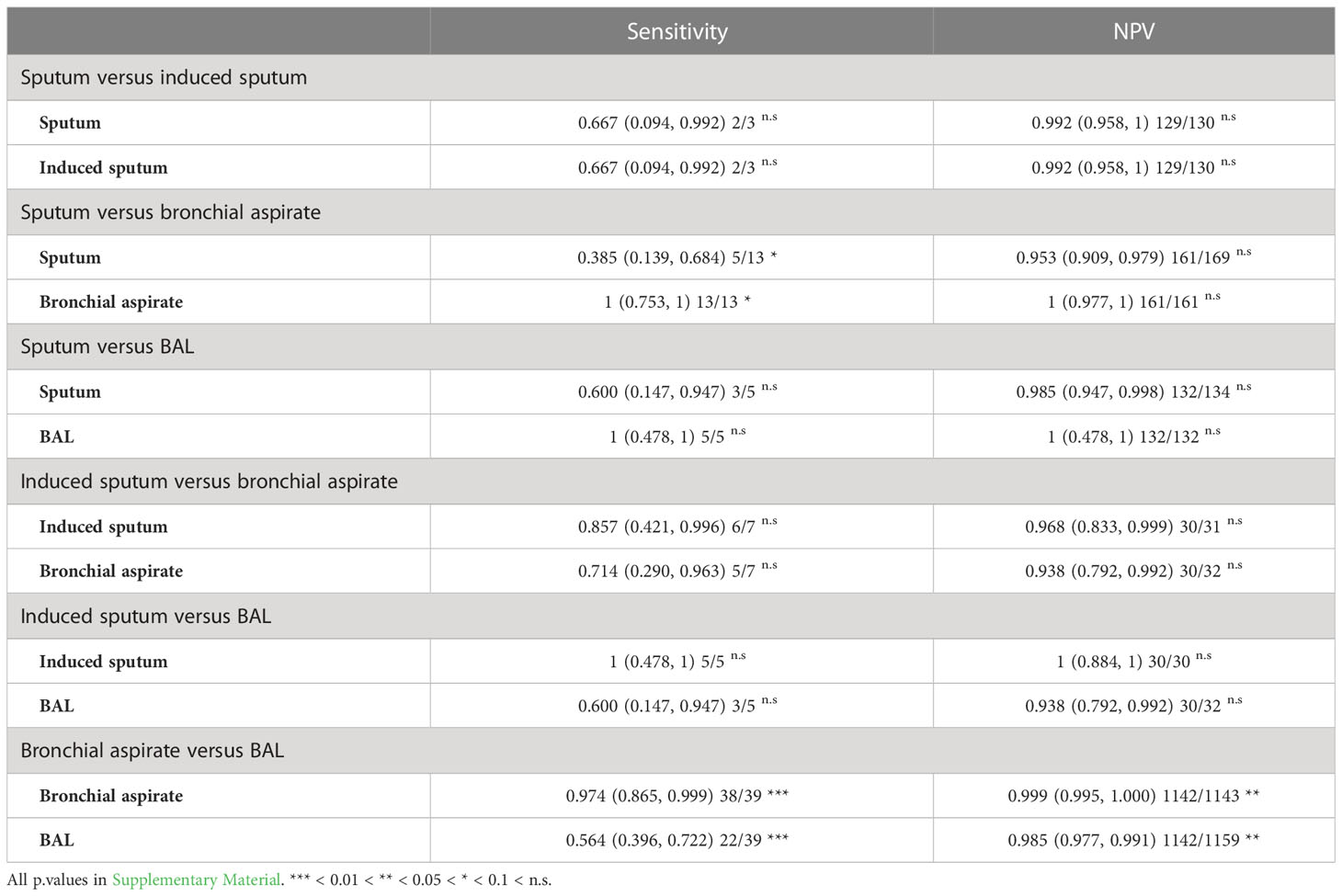
Table 5 Sensitivity of PCR to predict tuberculosis according to the type of specimen using a 72-hours pairing window in the same patient.
These data suggest, no significant difference in the sensitivity of PCR between sputum and induced sputum when the patient can produce spontaneous sputum. In contrast, bronchial aspirate displays higher sensitivity than sputum and BAL. PCR displayed a high specificity for all the respiratory specimens.
3.5 Performance of culture according to the type of respiratory specimen
Using the classical “per specimen” approachthe sensitivity of culture when considering all respiratory specimen was 0.988 (0.978, 0.994). The sensitivities of culture for sputum, induced sputum, bronchial aspirate and BAL were 0.993 (0.981, 0.998), 0.980 (0.931, 0.998), 0.965 (0.919, 0.988), and 1 (0.961, 1).
Using the “per patient” comparison of paired specimens, no significant difference in sensitivity was seen between the different respiratory specimens expect a superiority of bronchial aspirate over BAL, 0.970 (0.914, 0.994) versus 0.636 (0.538, 0.731) (p. value < 0.0001) (Tables 6; S6, S11).
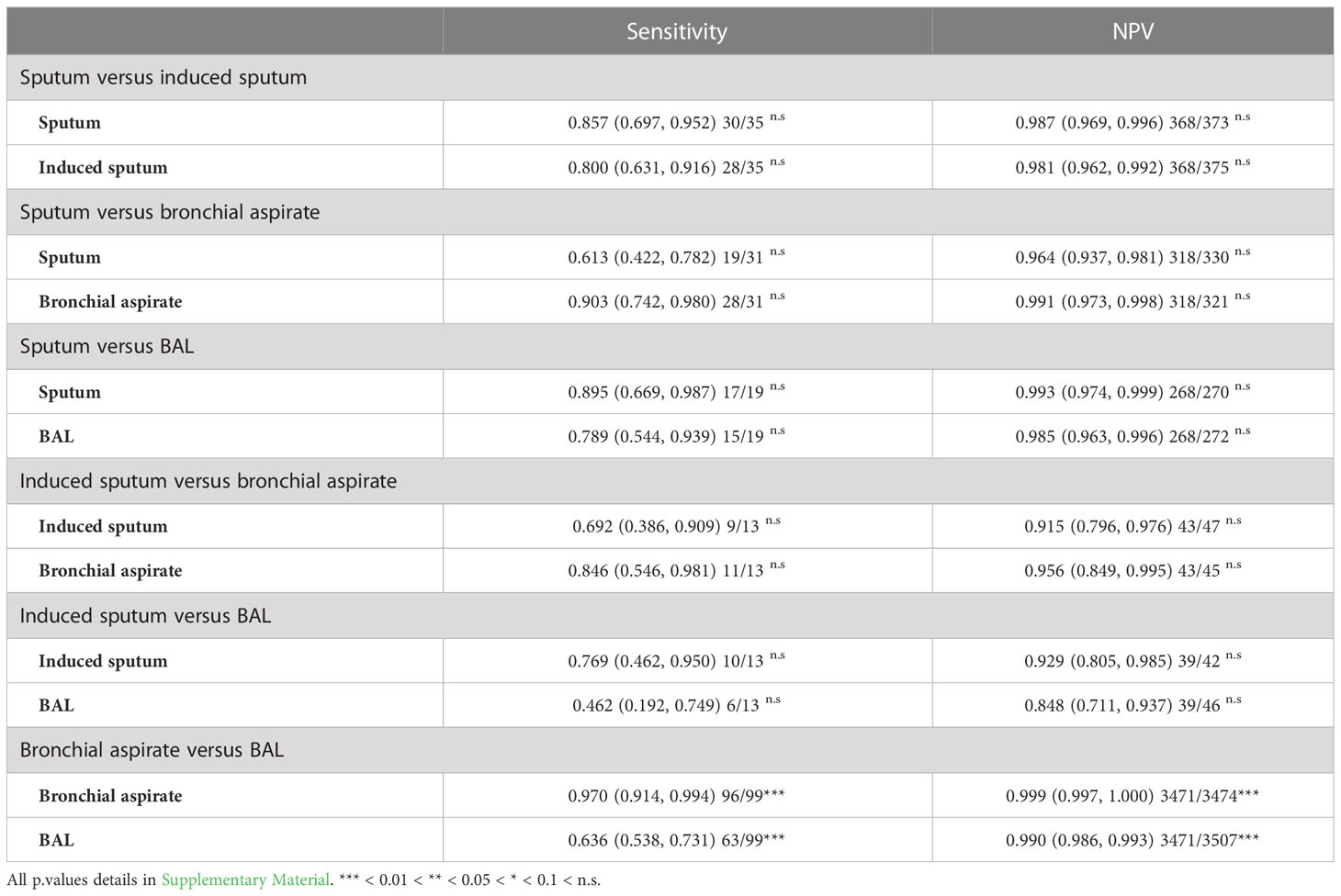
Table 6 Sensitivity of culture to predict tuberculosis according to the type of specimen using a 72-hours pairing window within the same patient.
4 Discussion
The microbial diagnosis of tuberculosis is based on a combination of different microbiological tests that can be performed on different types of clinical samples. We aimed to identify the most efficient tests and specimen in order to guarantee an ideal sensitivity and specificity and to limit the use of unnecessary tests.
4.1 Smear independent diagnostic of tuberculosis
Our results confirm a limited sensitivity of smear microscopy (0.523). The specificity (0.994) remains high, probably because of the extremely low prevalence (0.036) of positive microscopy. Our data suggest a higher sensitivity of microscopy on sputum over the three other samples. This is probably a bias because patients for whom the microbiological diagnosis is not made on spontaneous sputum and who therefore need induced sputum or bronchial aspiration and BAL are patients with paucibacillary infections as previously reported by Cadena et al. (2017). Using a pairwise comparison method to avoid the patient effect, we do not see any significant difference in sensitivity of microscopy between the various clinical specimens. With a time to result lower than 30 minutes, microscopy remains virtually the fastest diagnostic test. However, its sensitivity and specificity is limited and it requires a lot of work by specialized personnel and the performance of this test may vary depending on the experience of the examiner (Opota et al., 2016; Andenmatten et al., 2019). In a region with a low prevalence of tuberculosis, the question arises of the usefulness of this test. In 2016, we introduced in our laboratory a smear-independent algorithm for the diagnostic of tuberculosis (Opota et al., 2016). For all the suspicion of tuberculosis the microbial diagnosis is initiated by PCR; microscopy were not achieved anymore in emergency but grouped once per day. In case of suspected pulmonary tuberculosis, we initiated microbial diagnostic by the rapid molecular test Xpert MTB/RIF further replaced by Xpert MTB/RIF Ultra which is used both to detect M. tuberculosis DNA and to address patient infectiousness based on the semi-quantitative result; microscopic analysis was still performed after treatment start, in particular to guide contact tracing and des-isolation decisions (Opota et al., 2016; Opota et al., 2019b; Opota et al., 2019a). Another study on the diagnosis of nontuberculous mycobacteria (NTM) infection also suggested a limited added-value of microscopy when 16S broad range PCR for the detection of NTM is available (Andenmatten et al., 2019). Our data suggested that microscopy might be useful only for patients with a high pre-test probability of NTM infections, such as immunocompromised patients or patients with clinical and radiological suspicion of having NTM lung disease. In February 2020, we and other diagnostic laboratory experienced an important staff limitation triggered by the SARS-CoV-2 pandemics. Indeed, during the first wave of the SARS-CoV-2 pandemic, the biomedical technicians were reassigned for the management of the SARS-CoV-2 RT-PCR tests. In this context, we had to rapidly identify all the unnecessary analysis among which was smear microscopy. As an immediate response, we therefore push forward, in February 2020, the smear independent algorithm for the diagnostic of mycobacterial infection. Since then, microscopy for the detection of acid-fast bacilli is achieved only on specific request from clinicians or for patients with a confirmed diagnostic of tuberculosis; indeed, microscopy can be useful for treatment follow-up because PCR can remain positive for a long period in patient’s respiratory specimens even when a treatment is well conducted and for contact tracing investigations. Microscopy in addition to pan-mycobacterial PCR can also be requested when there is a high pre-test probability of NTM infection (Andenmatten et al., 2019).
4.2 Towards less cultures?
PCR has improved the diagnostic of tuberculosis with a lower limit of detection than microscopy and an increased specificity for PCR targeting specific M. tuberculosis DNA sequences such as the IS6110. Culture, the oldest microbiological test for tuberculosis, remains the reference method with the lowest limit of detection (Palomino, 2005; van Zyl-Smit et al., 2011). The performance of mycobacterial culture, sensitivity (98.8%) and specificity (100%), was calculated using a composite gold standard including all the microbiological tests as well as clinical data. We reported few patients with positive PCR but negative culture. It will therefore be difficult to do without culture. Further optimization strategies could be implemented by selecting the most performant tests on the most efficient clinical samples regarding pulmonary tuberculosis. Thus, we could consider only a combination of (i) samples for PCR-based diagnosis to have short time to results coupled to (ii) a selection of samples to perform the culture warranted to obtain strains for testing susceptibility towards anti-mycobacterial agents and also to guarantee an optimal sensitivity (with delayed results).
When pulmonary tuberculosis is suspected, sputum is the usual specimen that is collected. Regarding microscopy, as indicated above there is no significant difference between the different types of clinical samples. On the other hand, with regard to PCR and culture which are much more sensitive and reliable tests we observed differences between the clinical specimens. When looking at sputum and induced sputum we do not see a significant difference in terms of sensitivity for culture. Several studies reported an increased sensitivity of induced sputum over sputum (Biswas et al., 2013; Seong et al., 2014). Using the classical approach or the pairwise comparison in the same patient, we did not observe a significant increase in sensitivity with induced sputum compared to spontaneous sputum. However, the pairwise comparisons suggest an increase in the yield of positivity when performing the two specimens. This conclusion should be confirmed by further studies. BAL and bronchial aspirate are generally coupled. The pairwise comparison demonstrates that in the case of tuberculosis the bronchial aspirate (97% of sensitivity) outcompete BAL (63.6% of sensitivity) suggesting a limited added benefit of the BAL for the diagnosis of tuberculosis. Bronchial aspirate and BAL are invasive, which make prospective studies hardly conceivable. Therefore, this retrospective study, giving access to 3’570 pairwise comparison including these specimen provide important data on their performance. Bronchoscopy is not only useful for tuberculosis diagnosis but also to investigate other infectious or non-infectious disease (Sanjeevaiah et al., 2018). For instance, BAL is a very good specimen for the diagnostic of fungal infection such as Pneumocystis jirovecii infections or Aspergillus fumigatus infection (Imbert et al., 2018; Perret et al., 2020; Neofytos et al., 2021). In view of these results, it is important to consider bronchial aspiration for the diagnosis of tuberculosis, confirming previous studies (Maitre et al., 2021). A first step would therefore be to always add a search for mycobacteria on the bronchial aspiration when it is missing in laboratory order. This is what we systematically do in our lab when it is missing. This study provides data for diagnostic stewardship and guidance for physicians and clinical microbiology laboratories. Such data could also help at defining diagnostic strategies in the setting of staff or reagants shortage or to reduce costs. Indeed, even in low prevalence and high-income country, the infrastructure and trained personnel for the diagnostic of tuberculosis is limited (Cannas et al., 2013). The SARS-CoV-2 pandemics negatively impacted tuberculosis control because of the mobilization of trained staff for other activities or because of the disruption of the supply chain of reagents and compounds for tuberculosis diagnostic and treatments (Meneguim et al., 2020; Armstrong et al., 2021). In a context of shortage of reagants or other material for mycobacterial culture like the one we encountered during the COVID-19 pandemic, if a prioritization had to be made, it should be done for the benefit of bronchial aspiration; but this should be decided together with the clinician that can help guiding the decision by providing clinical information for each case.
4.3 Limits of the study and perspectives
Although this is a retrospective study from a single centre, it does contain a large amount of data over a long period of time. In comparison with the low sensitivity of smear microscopy, PCR detection of M. tuberculosis had higher sensitivity and specificity. In addition, compared to Ziehl-Neelsen and auramine staining, rapid PCR assays such as the Xpert systems are relatively easy to use and require less training and experience. The very short time to results of rapid PCR allows its use as a first-line method for both clinical diagnosis and patient management, as well as for rapid triage of hospitalized patients to avoid nosocomial spread. In addition, the detection of rifampicin resistance is a further advantage of such rapid PCR tests. Nevertheless, smear microscopy is still a mean of rapid diagnostic of tuberculosis in low- and middle-income countries. It is also of great importance for the detection of non-tuberculous mycobacteria, especially in the absence of real-time PCR for the detection of these pathogens. Molecular diagnostic significantly improved the microbial diagnostic of tuberculosis, in particular the initial diagnostic, but is not yet generalized worldwide (Mechai et al., 2020), mainly for economical reasons. To assess the real economic impact of the management of tuberculosis a cost-benefit analysis for the full replacement of microscopy in favor of PCR should be performed since this study demonstrates the effectiveness of PCR over microscopy (Dowdy et al., 2014). Such analysis should incorporate that, in hospital setting, patients might be isolated in specific negative pressure chambers for the duration of the investigation. Such cost-benefit study should also address the risk of nosocomial infection due to delayed diagnostic. Future studies should account for the evolution in practices that may have occurred over the ten years (2008-2018) of the study. It would be worthwhile to relate the data with the evolution of protocols and guidelines that were introduced during the studied period in order not to only add diagnostic tools but also to stop the not useful approaches. This study will also permit to address the dependence between the tests results and many other parameters such as the number of tests, the quality of the clinical specimens and patients characteristics. This will be particularly useful for results interpretation, in particular negative results. Finally, this study is based on a large amount of data over a long period, which was made possible by the fact that all the microbial result are in our LIS since 1995. Although, it may not be the case even in high income country labs, comparison of these results with those obtained at other medical centers should be performed with the view of cross-validating the robustness of the present results.
5 Conclusions
This study demonstrates that many improvements have been made in the microbiological diagnosis of tuberculosis. There is no doubt about the added value of molecular diagnosis compared to microscopy to initiate the diagnosis of tuberculosis. The limit of a generalization of independent algorithms in microscopy lies in the access to molecular diagnosis. New technologies such as the GeneXpert, which are supposed to solve this problem, are not yet generalized. Regarding tuberculosis, the limit in this case is not technological but again, economical. This study provides data for diagnostic stewardship and for editing guidelines and diagnosis protocols with the purpose to reduce the medical, social and economic costs associated with tuberculosis. Indeed, even in low prevalence and high-income country, the infrastructure and trained personnel for the diagnostic of tuberculosis is limited. Therefore, there is a need to identify the most efficient tests and specimens in order to guarantee the sensitivity and specificity and to limit the number of unnecessary tests. In addition, it provides analytical strategies that may also be suitable for the study of other infectious diseases.
Data availability statement
The datasets presented in this article are not readily available because, the data are generated only for the present study. Any re-use would need another ethical authorisation. Requests to access the datasets should be directed to onya.opota@chuv.ch.
Author contributions
OO, KJ, and M-OB contributed to conception and design of the study. RB performed the raw data extraction. RB, M-OB and OO organized the database. JD-L, RN performed part of the statistical analysis under the supervision of M-OB, VC and OO. M-OB and OO performed the statistical analysis. OO and M-OB wrote the first draft of the manuscript. All authors contributed to manuscript revision, read, and approved the submitted version.
Funding
Open access funding provided by University of Lausanne.
Acknowledgments
We are thankful to the Laboratory of Molecular Diagnostic and Mycobacteria of the Institute of Microbiology of the University of Lausanne and Lausanne University Hospital that perform the conventional and molecular analysis for the diagnosis of tuberculosis.
Conflict of interest
The authors declare that the research was conducted in the absence of any commercial or financial relationships that could be construed as a potential conflict of interest.
Publisher’s note
All claims expressed in this article are solely those of the authors and do not necessarily represent those of their affiliated organizations, or those of the publisher, the editors and the reviewers. Any product that may be evaluated in this article, or claim that may be made by its manufacturer, is not guaranteed or endorsed by the publisher.
Supplementary material
The Supplementary Material for this article can be found online at: https://www.frontiersin.org/articles/10.3389/fcimb.2023.1131241/full#supplementary-material
References
Andenmatten, S., Opota, O., Mazza-Stalder, J., Nicod, L., Greub, G., Jaton, K. (2019). Added diagnostic value of 16s rrna gene pan-mycobacterial pcr for nontuberculous mycobacterial infections: A 10-year retrospective study. Eur. J. Clin. Microbiol. Infect. Dis. 38(10):1873–1881. doi: 10.1007/s10096-019-03621-z
Armstrong, D. T., Tacheny, E. A., Olinger, G., Howard, R., Lemmon, M. M., Dasgupta, D., et al. (2021). Sars-cov-2 supply shortages and tuberculosis diagnostics: Current issues requiring immediate solutions. J. Clin. Microbiol. 59, e0077821. doi: 10.1128/JCM.00778-21
Biswas, S., Das, A., Sinha, A., Das, S. K., Bairagya, T. D. (2013). The role of induced sputum in the diagnosis of pulmonary tuberculosis. Lung India: Off. Organ Indian Chest Soc. 30, 199–202. doi: 10.4103/0970-2113.116259
Boehme, C. C., Nabeta, P., Hillemann, D., Nicol, M. P., Shenai, S., Krapp, F., et al. (2010). Rapid molecular detection of tuberculosis and rifampin resistance. N. Engl. J. Med. 363, 1005–1015. doi: 10.1056/NEJMoa0907847
Cadena, A. M., Fortune, S. M., Flynn, J. L. (2017). Heterogeneity in tuberculosis. Nat. Rev. Immunol. 17, 691–702. doi: 10.1038/nri.2017.69
Campelo, T. A., Cardoso de Sousa, P. R., Nogueira, L. L., Frota, C. C., Zuquim Antas, P. R. (2021). Revisiting the methods for detecting mycobacterium tuberculosis: What has the new millennium brought thus far? Access Microbiol. 3, 000245. doi: 10.1099/acmi.0.000245
Cannas, A., Paglia, M. G., Sakhoo, D. C., Vairo, F., Doulla, B., Nguhuni, B., et al. (2013). Strengthening tuberculosis diagnosis in a low-resource setting: Experience learned in dodoma, tanzania. J. Infect. Dev. Ctries 7, 676–679. doi: 10.3855/jidc.3366
Chakravorty, S., Simmons, A. M., Rowneki, M., Parmar, H., Cao, Y., Ryan, J., et al. (2017). The new xpert mtb/rif ultra: Improving detection of mycobacterium tuberculosis and resistance to rifampin in an assay suitable for point-of-care testing. MBio 8 (4), e00812–17. doi: 10.1128/mBio.00812-17
Dorman, S. E., Schumacher, S. G., Alland, D., Nabeta, P., Armstrong, D. T., King, B., et al. (2018). Xpert mtb/rif ultra for detection of mycobacterium tuberculosis and rifampicin resistance: A prospective multicentre diagnostic accuracy study. Lancet Infect. Dis. 18, 76–84. doi: 10.1016/S1473-3099(17)30691-6
Dowdy, D. W., Houben, R., Cohen, T., Pai, M., Cobelens, F., Vassall, A., et al. (2014). Impact and cost-effectiveness of current and future tuberculosis diagnostics: The contribution of modelling. Int. J. Tuberculosis Lung Dis. 18, 1012–1018. doi: 10.5588/ijtld.13.0851
Greub, G., Sahli, R., Brouillet, R., Jaton, K. (2016). Ten years of r&d and full automation in molecular diagnosis. Future Microbiol. 11, 403–425. doi: 10.2217/fmb.15.152
Imbert, S., Meyer, I., Palous, M., Brossas, J.-Y., Uzunov, M., Touafek, F., et al. (2018). Aspergillus pcr in bronchoalveolar lavage fluid for the diagnosis and prognosis of aspergillosis in patients with hematological and non-hematological conditions. Front. Microbiol. 9, 1877. doi: 10.3389/fmicb.2018.01877
Lippincott, C. K., Miller, M. B., Popowitch, E. B., Hanrahan, C. F., Van Rie, A. (2014). Xpert mtb/rif assay shortens airborne isolation for hospitalized patients with presumptive tuberculosis in the united states. Clin. Infect. Dis. 59, 186–192. doi: 10.1093/cid/ciu212
Maitre, T., Ok, V., Morel, F., Bonnet, I., Sougakoff, W., Robert, J., et al. (2021). Sampling strategy for bacteriological diagnosis of intrathoracic tuberculosis. Respir. Med. Res. 79, 100825. doi: 10.1016/j.resmer.2021.100825
Mechai, F., Cordel, H., Guglielmetti, L., Aubry, A., Jankovic, M., Viveiros, M., et al. (2020). Management of tuberculosis: Are the practices homogeneous in high-income countries? Front. Public Health 8, 443. doi: 10.3389/fpubh.2020.00443
Meneguim, A. C., Rebello, L., Das, M., Ravi, S., Mathur, T., Mankar, S., et al. (2020). Adapting tb services during the covid-19 pandemic in mumbai, india. Int. J. Tuberc. Lung Dis. 24, 1119–1121. doi: 10.5588/ijtld.20.0537
Neofytos, D., Garcia-Vidal, C., Lamoth, F., Lichtenstern, C., Perrella, A., Vehreschild, J. J. (2021). Invasive aspergillosis in solid organ transplant patients: Diagnosis, prophylaxis, treatment, and assessment of response. BMC Infect. Dis. 21, 296. doi: 10.1186/s12879-021-05958-3
Opota, O., Mazza-Stalder, J., Greub, G., Jaton, K. (2019a). The rapid molecular test xpert mtb/rif ultra: Towards improved tuberculosis diagnosis and rifampicin resistance detection. Clin. Microbiol. Infect. 25, 1370–1376. doi: 10.1016/j.cmi.2019.03.021
Opota, O., Senn, L., Prod’hom, G., Mazza-Stalder, J., Tissot, F., Greub, G., et al. (2016). Added value of molecular assay xpert mtb/rif compared to sputum smear microscopy to assess the risk of tuberculosis transmission in a low-prevalence country. Clin. Microbiol. Infect. 22, 613–619. doi: 10.1016/j.cmi.2016.04.010
Opota, O., Zakham, F., Mazza-Stalder, J., Nicod, L., Greub, G., Jaton, K. (2019b). Added value of xpert mtb/rif ultra for diagnosis of pulmonary tuberculosis in a low-prevalence setting. J. Clin. Microbiol. 57. doi: 10.1128/JCM.01717-18
Palomino, J. C. (2005). Nonconventional and new methods in the diagnosis of tuberculosis: Feasibility and applicability in the field. Eur. Respir. J. 26, 339. doi: 10.1183/09031936.05.00050305
Perret, T., Kritikos, A., Hauser, P. M., Guiver, M., Coste, A. T., Jaton, K., et al. (2020). Ability of quantitative pcr to discriminate pneumocystis jirovecii pneumonia from colonization. J. Med. Microbiol. 69, 705–711. doi: 10.1099/jmm.0.001190
Pfyffer, G. E., Wittwer, F. (2012). Incubation time of mycobacterial cultures: How long is long enough to issue a final negative report to the clinician? J. Clin. Microbiol. 50, 4188–4189. doi: 10.1128/JCM.02283-12
Sanjeevaiah, S., Haranal, M. Y., Buggi, S. (2018). Role of flexible bronchoscopy in patients with sputum-negative pulmonary tuberculosis. Indian J. Thorac. Cardiovasc. Surg. 34, 365–369. doi: 10.1007/s12055-018-0645-z
Schaaf, H. S., Reuter, H. (2009). “Chapter 22 - practical approaches to ordering diagnostic tests,” in Tuberculosis. Eds. Schaaf, H. S., Zumla, A. I., Grange, J. M., et al (Edinburgh: W.B. Saunders) 216–226.
Seong, G. M., Lee, J., Lee, J. H., Kim, J. H., Kim, M. (2014). Usefulness of sputum induction with hypertonic saline in a real clinical practice for bacteriological yields of active pulmonary tuberculosis. Tuberculosis Respir. Dis. 76, 163–168. doi: 10.4046/trd.2014.76.4.163
van Zyl-Smit, R. N., Binder, A., Meldau, R., Mishra, H., Semple, P. L., Theron, G., et al. (2011). Comparison of quantitative techniques including xpert mtb/rif to evaluate mycobacterial burden. PLoS One 6, e28815. doi: 10.1371/journal.pone.0028815
Weiszhar, Z., Horvath, I. (2013). Induced sputum analysis: Step by step. Breathe 9, 300. doi: 10.1183/20734735.042912
WHO (2021a). Global tuberculosis report 2021. Geneva: World Health Organization; 2021. Licence: CC BY-NC-SA 3.0 IGO.
WHO (2021b). WHO consolidated guidelines on tuberculosis. Module 2: screening – systematic screening for tuberculosis disease. Geneva: World Health Organization; 2021. Licence: CC BY-NC-SA 3.0 IGO.
Keywords: tuberculosis, PCR, mycobacterial culture, bronchoalveolar lavage (BAL), bronchial aspirate, sputum, induced-sputum, acid-fast bacilli (AFB)
Citation: Boldi M-O, Denis-Lessard J, Neziri R, Brouillet R, von-Garnier C, Chavez V, Mazza-Stalder J, Jaton K, Greub G and Opota O (2023) Performance of microbiological tests for tuberculosis diagnostic according to the type of respiratory specimen: A 10-year retrospective study. Front. Cell. Infect. Microbiol. 13:1131241. doi: 10.3389/fcimb.2023.1131241
Received: 24 December 2022; Accepted: 03 February 2023;
Published: 02 March 2023.
Edited by:
Carina Almeida, Instituto Nacional Investigaciao Agraria e Veterinaria (INIAV), PortugalReviewed by:
Liang-jun Chen, Wuhan University, ChinaAbhishek Mishra, Houston Methodist Research Institute, United States
Copyright © 2023 Boldi, Denis-Lessard, Neziri, Brouillet, von-Garnier, Chavez, Mazza-Stalder, Jaton, Greub and Opota. This is an open-access article distributed under the terms of the Creative Commons Attribution License (CC BY). The use, distribution or reproduction in other forums is permitted, provided the original author(s) and the copyright owner(s) are credited and that the original publication in this journal is cited, in accordance with accepted academic practice. No use, distribution or reproduction is permitted which does not comply with these terms.
*Correspondence: Onya Opota, onya.opota@chuv.ch