- 1Chongqing Key Laboratory of Vector Insects, College of Life Sciences, Chongqing Normal University, Chongqing, China
- 2Guangdong Laboratory for Lingnan Modern Agriculture, Guangzhou, China
- 3Engineering Research Centre of Biological Control, Ministry of Education, South China Agricultural University, Guangzhou, China
Background: Wolbachia is the most abundant bacterial endosymbiont among insects. It can play a prominent role in the development, reproduction and immunity of its given insect host. To date, Wolbachia presence is well studied within aphids, whiteflies and planthoppers, but relatively few studies have investigated its presence in psyllids.
Methods: Here, the infection status of Wolbachia in five species of psyllid, including Asian citrus psyllid Diaphorina citri and longan psyllid Cornegenapsylla sinica was investigated. The phylogenetic relationships of different Wolbachia lines and their infection density and patterns in D. citri and C. sinica from different countries was also examined.
Results: The infection rates of Wolbachia in D. citri and C. sinica were both 100%, and their sequencing types are ST173 and ST532 respectively. Phylogenetic analysis revealed that the Wolbachia lines in D. citri and C. sinica both belong to the Con subgroup of Wolbachia supergroup B. In addition, Wolbachia displayed a scattered localization pattern in the 5th instar nymphs and in the reproductive organs of both D. citri and C. sinica but differed in other tissues; it was highest in the midgut, lowest in the salivary glands and medium in both the testes and ovaries.
Conclusion: Our findings assist in further understanding the coevolution of Wolbachia and its psyllid hosts. Given that Wolbachia could play an important role in insect pest control and pathogen transmission inhibition, our findings may also provide new insights for development of control strategies for D. citri and C. sinica.
Introduction
Symbiotic bacteria are ubiquitous in animal hosts, among which the endosymbiont Wolbachia is the most abundant one in arthropods (Dale et al., 2006; Zug and Hammerstein, 2012). Wolbachia contains several supergroups, all of which are different in their physiological roles and host distribution (Lo et al., 2007; Ros et al., 2009; Bing et al., 2014). In arthropod hosts, Wolbachia has been reported in various tissues but mainly resides in the reproductive organs, where it is associated with the induction of different reproductive alterations such as feminization, parthenogenesis, male killing, and cytoplasmic incompatibility (Stouthamer et al., 1999; Hancock et al., 2011; Lv et al., 2021), in turn, aiding the spread of Wolbachia infection in its host populations (Saridaki and Bourtzis, 2010). Recently, extensive evidence has shown that Wolbachia can benefit a number of insects via a mutualistic relationship (Zug and Hammerstein, 2015). For example, Wolbachia can protect arthropod hosts against a variety of pathogens and abiotic stresses (Teixeira et al., 2008; Brownlie et al., 2009; Iturbe-Ormaetxe et al., 2011). Some Wolbachia strains are also essential for successful egg development, such as in bed bugs, parasitic wasps and collembolan species (Dedeine et al., 2001; Timmermans and Ellers, 2008; Kremer et al., 2009; Hosokawa et al., 2010), while others can enhance the fecundity of its female host insect (Dedeine et al., 2001; Dobson et al., 2004; Fry et al., 2004; Dong et al., 2006).
The infection, distribution of Wolbachia and its ability to manipulate the reproductive properties of arthropod hosts have attracted much interest concerning its role in the host’s biology, ecology, and evolution, as well as in the development of novel, symbiont based and environmentally friendly based methods for pest and disease management (Bourtzis and Miller, 2006; Hedges et al., 2008; Moreira et al., 2009; Laidoudi et al., 2020; Ilinsky et al., 2022; Zong et al., 2022). For instance, recent studies have shown that, the presence of Wolbachia in some insect species may provide antiviral protection, and inhibit the infection and transmission of certain pathogens such as Dengue, Zika, Chikungunya, Yellow fever, Mayaro viruses and rice ragged stunt virus (RRSV) (Moreira et al., 2009; Walker et al., 2011; Van den Hurk et al., 2012; Frentiu et al., 2014; Tan et al., 2017; Ryan et al., 2019).
The Asian citrus psyllid Diaphorina citri Kuwayama and longan psyllid Cornegenapsylla sinica Yang et Li are both phloem feeding insect pests. D. citri is considered one of the most destructive citrus pests due to its capability to transmit the bacterial causal agent of Huanglongbing or citrus greening, Candidatus Liberibacter asiaticus (CLas) (Halbert and Manjunath, 2004), while C. sinica is a devastating pest of Longan that vectors the longan pathogen witches’ broom virus (LgWB) (Chen et al., 1992; Seo et al., 2017; Tran et al., 2019). New environmentally friendly strategies and products are urgently required to manage these pests since few efficient control strategies are available due to the rapid evolution of high levels of insecticide resistance (Cuthbertson and Vanninen, 2015; Chen et al., 2021).
Similar to many other insect species, D. citri and C. sinica are also infected with Wolbachia (designated wDi and wSin). Although the direct influence of Wolbachia on D. citri and C. sinica biology remains to be determined, recent studies indicate that the relative abundance of wDi may be associated with the abundance of CLas within hosts (Fagen et al., 2012), where it may contribute to the regulation of phage lytic cycle genes in CLas (Jain et al., 2017). These findings highlight the importance of wDi in citrus greening disease management.
As previously mentioned, strategies based on the maternally inherited endosymbiont Wolbachia is currently under development for insect borne diseases control by either population replacement or population suppression strategies (Hoffmann et al., 2011; Zheng et al., 2019; Crawford et al., 2020; Neupane et al., 2022). Such disease control approaches are based on the ability of Wolbachia to inhibit the pathogen transmission of insect vectors (Hedges et al., 2008; Moreira et al., 2009; Bian et al., 2013). Thus, to achieve this purpose, the Wolbachia infection and distribution status in these psyllid insects should be determined.
In the current study, the adults of D. citri, C. sinica as well as another three similarly geospatially distributed species of psyllid in South China, Macrohomotoma sinica Yang et Li, Blastopsylla occidentalis Taylor and Pseudophacopteron canarium Yang et Li, were collected. The infection and distribution of the Wolbachia endosymbiont in these five species, and their phylogenetic relationship with each other was investigated. This was expected to provide new insights for the development of alternative and environmentally friendly strategies for insect vector control.
Materials and methods
Insect collecting and rearing
The five populations of psyllid, D. citri, C. sinica, M. sinica, B. occidentalis and P. canarium were collected from citrus, longan, banyan, eucalyptus and olive trees respectively during August 2022. They were continuously reared on their respective seedling plants in separated glasshouses in South China Agricultural University, Guangzhou at 26-28°C, 60-80% relative humidity and 14:10h (L:D) photoperiod. New seedling plants with fresh shoots were provided for the sample cultures every two weeks.
PCR detection of Wolbachia
Total DNA was extracted from each single adult of the five species of psyllid using the TIANamp Genomic DNA Kit (Tiangen, Beijing, China) following the manufacturer’s instructions. The general primers used for Wolbachia detection were wsp 81F and wsp 691R (Zhou et al., 1998), which target a DNA fragment of Wolbachia’s outer surface protein (wsp) gene (Table 1). The PCR reactions were run in a 25μl buffer containing 1μl of the template DNA lysate, 1μl of each primer, 2.5mM MgCl2, 200mM for each dNTP and 1 unit of DNA Taq polymerase (Invitrogen, Guangzhou, China). PCR products were visualized by 1.0% agarose gel electrophoresis, stained with Gold View in 0.5 × TBE buffer (Sangon, Shanghai, China) and photographed under UV light. In total, DNA from 100, 100, 64, 52 and 22 individual adults of D. citri, C. sinica, M. sinica, B. occidentalis and P. canarium was extracted respectively.
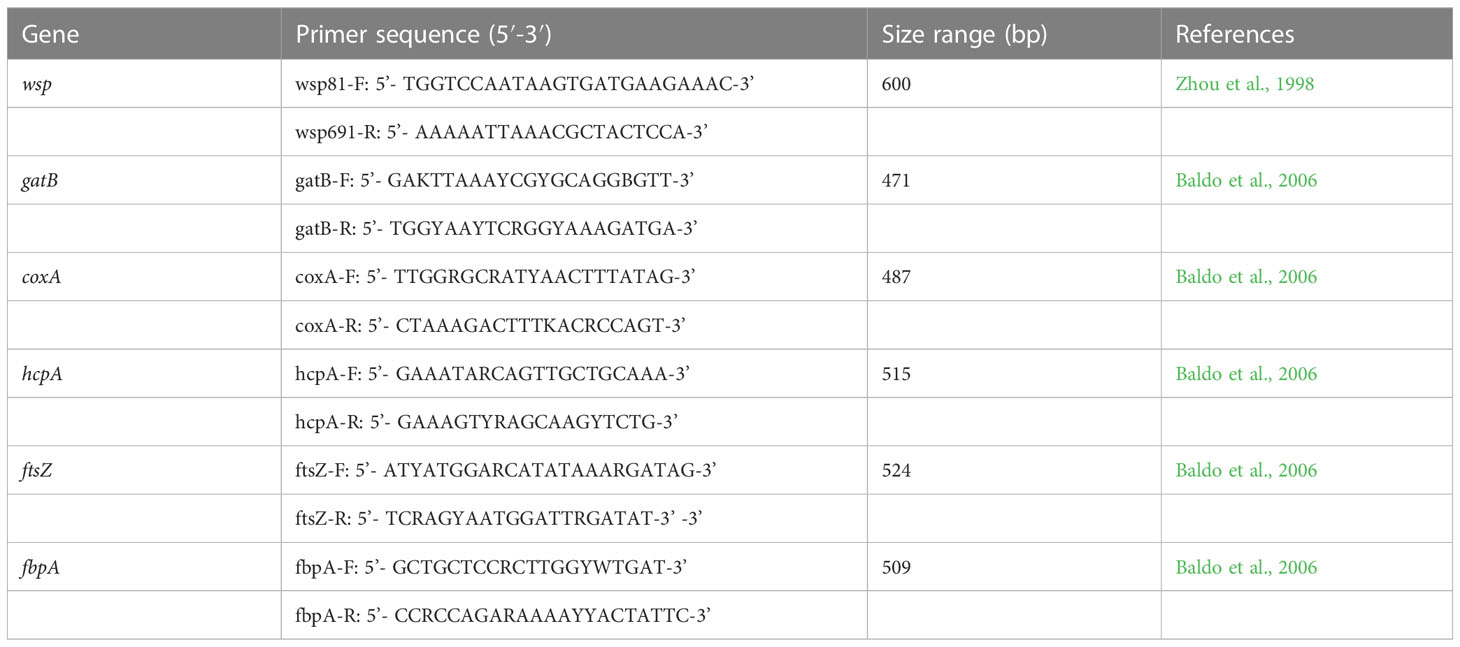
Table 1 The primers used for wsp and MLST gene amplification of Asian citrus psyllid and longan psyllid.
Gene sequencing of mtCOI and Wolbachia MLST
The total template DNA extraction, PCR amplification reaction and target DNA visualization of psyllid adults was the same as previously described for the PCR detection of Wolbachia. The primers used for the PCR amplification of psyllid mtCOI gene are COI F: 5’ AGGAGGTGGAGACCCAATCT 3’ and COI R: 5’ TCAATTGGGGGAGAGTTTTG 3’ (Boykin et al., 2012). Target PCR products were purified and sent out for complete bidirectional sequencing in Sangon Biotech Co., Ltd. (Shanghai, China).
For the MLST analysis of Wolbachia in D. citri and C. sinica, the wsp gene and five MLST genes (gatB, coxA, hcpA, ftsZ, and fbpA) were amplified via the special PCR primers shown in Table 1. Again, the target PCR products were purified and sent to Sangon Biotech Co., Ltd. for complete bidirectional sequencing.
Phylogeny analysis of the psyllid species
The mtCOI gene sequences from another 14 psyllid species were selected as references based on the study of Percy et al. (2018) (Table 2). The mtCOI sequences were firstly aligned using Lasergene v7.1 (DNASTAR, Inc., Madison, WI), and then the phylogeny of the mtCOI sequences were analyzed independently with Neighbor-Joining Algorithm (NJ) based on the Tamura-Nei model using MEGA 6.0 software. The mtCOI sequence of the bed bug Pariaconus ohiacola (KY294009) was used as the out group (Table 2). A discrete gamma distribution was applied for each analysis with 1,000 bootstrap replicates (NJ BS).
Sequence typing and phylogenetic analysis of Wolbachia
The wsp and five MLST genes of Wolbachia strains in the D. citri (wDi) and C. sinica (wSin) were blasted in the GenBank on the NCBI website. The phylogenetic relationships of Wolbachia in these two vector psyllids was analyzed based on their wsp and the five MLST genes. Firstly, the wsp genes of Wolbachia from 8 subgroups of supergroup A and 7 subgroups of supergroup B were used as references. The wsp gene of Wolbachia from the filarial parasite Brugia malayi (AJ252061) was used as the out group in the phylogeny analysis based on the wsp (Table 3). Secondly, the wsp gene sequences of Wolbachia obtained in this study were separately compared with other sequences from China, Thailand, Singapore, Pakistan, Iran, India, Saudi Arabia, Jamaica, Brazil and USA strains that are deposited in both the NCBI and the Wolbachia database (http://pubmlst.org/wolbachia/wsp/) (Table 4), using the Bayesian methods as described above. The MLST genes of Wolbachia from 5 subgroups of supergroup A and 3 subgroups of supergroup B were used as references; the MLST gene sequences of Cimex lectularius were used as the out group in the phylogeny analysis of Wolbachia based on the MLST genes (Table 5).
The phylogeny of wsp and MLST sequences was analyzed independently via Neighbor-Joining Algorithm (NJ) based on the Tamura-Nei model using MEGA 6.0 software. A discrete gamma distribution was applied for each analysis with 1,000 bootstrap replicates. For Wolbachia genes, unique sequences were searched for in the Wolbachia MLST database (http://www.pubmlst.org/wolbachia/), resulting in their ST numbers being determined (Baldo et al., 2006).
Infection density of Wolbachia in D. citri and C. sinica
The density of Wolbachia in different D. citri and C. sinica tissues was determined using qPCR. The psyllid adults were dissected at 7 days post-emergence after the final molt under a stereomicroscope, which included their salivary glands, midgut, testes, and ovaries. Primers used in Wolbachia quantitative detection were 16S-F: 5’-GAGTGAAGAAGGCCTTTGGG-3’, and 16S-R 5’-CACGGAGTTAGCCAGGACTTC-3’ (Gong et al., 2020), which amplify a fragment of the Wolbachia 16S rRNA gene. The Actin genes of D. citri (forward: 5’-ACTGCCCTGGCTCCCTC T-3’, reverse: 5’-CGGACTCGTCGTATTCTTGTTT-3’) and C. sinica (forward: 5’-ACTGCCCTG GCTCCCTCT-3’, reverse: 5’-CGGACTCGTCGTATTCTTGTTT-3’) were used as the housekeeping genes. Three repeats and 5 adult individuals in each repeat were detected.
FISH visualization of Wolbachia
Wolbachia distribution differs in nymphal and adult tissues. Fluorescence in situ hybridization (FISH) was used according to the description in Li et al. (2020). Briefly, entire 4th instar nymphs, and the ovaries and testes of 5-7d old adults after dissection, were fixed for 30min in fresh 4% paraformaldehyde prepared in 1×PBS with 0.1% Triton X-100. The samples were then washed three times for 5min in 1×PBS, immersed in hybridization solution overnight in a 46˚C water bath in the dark. Following this, the samples were washed once for 5min in each of four solutions in turn (2×SSC with 0.015% (w/v) DTT; 1×SSC with 0.015% (w/v) DTT; 0.5×SSC with 0.015% (w/v) DTT; 1×PBS alone), then stained for 1h with VECTASHIELD® Antifade Mounting Medium with DAPI (Vector Laboratories, CA, USA) at room temperature. They were then washed again in 1×PBS before being mounted with anti-fluorescence quenching mounting medium. The distribution was then be observed under an inverted fluorescence microscope (Nikon Eclipse TieU). The Cy5 5’-end-labeled Wolbachia 16S rRNA probes (W2-Cy3: 5’-CTTCTGTGAGTACCGTCATTATC-3’) were used for the hybridization.
Statistical analysis
The relative titers of Wolbachia in the different treatments were firstly normalized and then calculated using the method of 2-ΔΔct with the accompanying software in a Bio-Rad thermocycler (Bio Rad CFX Manager). All data was analyzed using one-way analysis of variance (ANOVA), and means were compared using the Duncan’s test (SPSS 17.0) at P<0.01. All figures were drawn using Sigmaplot 10.0.
Results
Wolbachia infection rates in different species of psyllids
The PCR detection results revealed that Wolbachia was present in D. citri, C. sinica and M. sinica adults, with its infection rates being 100% in both D. citri (100/100) and C. sinica (100/100), and approximately 6.25% in M. sinica (4/64). However, Wolbachia was absent in B. occidentalis (0/52) and P. canarium (0/22) (Figure 1).
Phylogenetic relationships of D. citri and C. sinica
The mtCOI gene of D. citri and C. sinica were successfully amplified, with the sequences submitted to GenBank (accession number MF614818 for D. citri; MN728680 for C. sinica). The phylogeny trees of D. citri, C. sinica and the 14 other Diaphorina insects showed that the phylogeny trees of D. citri, C. sinica and the 14 other psyllids showed that D. citri and C. sinica were firstly clustered into one branch, and the D. citri and C. sinica clustered with the Cacopsylla psyllids into one peripheral branch (Figure 2). As expected, D. citri and C. sinica have a close phylogenetic relationship to each other.
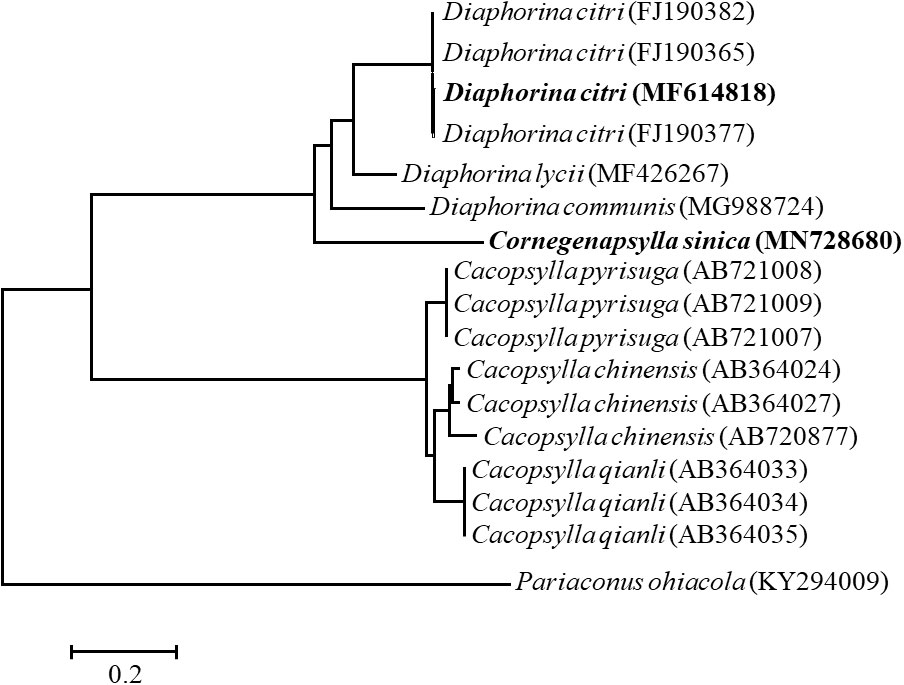
Figure 2 The phylogenetic relationship of Diaphorina citri and Cornegenapsylla sinica based on mtCOI gene. The tree was constructed and analyzed by Neighbor-Joining (NJ) method using 1000 bootstraps replicates. Numbers at the nodes indicate the percentages of reliability of each branch of the tree. Branch length is drawn proportional to the estimated sequence divergence.
Sequence typing and phylogenetic relationships of Wolbachia in D. citri and C. sinica
The results from the sequence typing analysis revealed that the Wolbachia in D. citri and C. sinica were ST173 and ST532 respectively (Table 6). The phylogenetic analysis of Wolbachia based on their wsp genes showed that all the Wolbachia lines were clustered into two main branches, i.e., A branch and B branch; Wolbachia lines from D. citri and C. sinica in the current study were firstly clustered into one branch of Con subgroup with Tribolium confusum, then clustered with Trichogramma deion (Dei subgroup) and Trichogramma kaykai (Kay subgroup), Apoanagyrus diversicornis (Div subgroup), Aedes albopictus and Culex pipiens (Pip subgroup) in turn; all of which belong to the supergroup B (Figure 3).
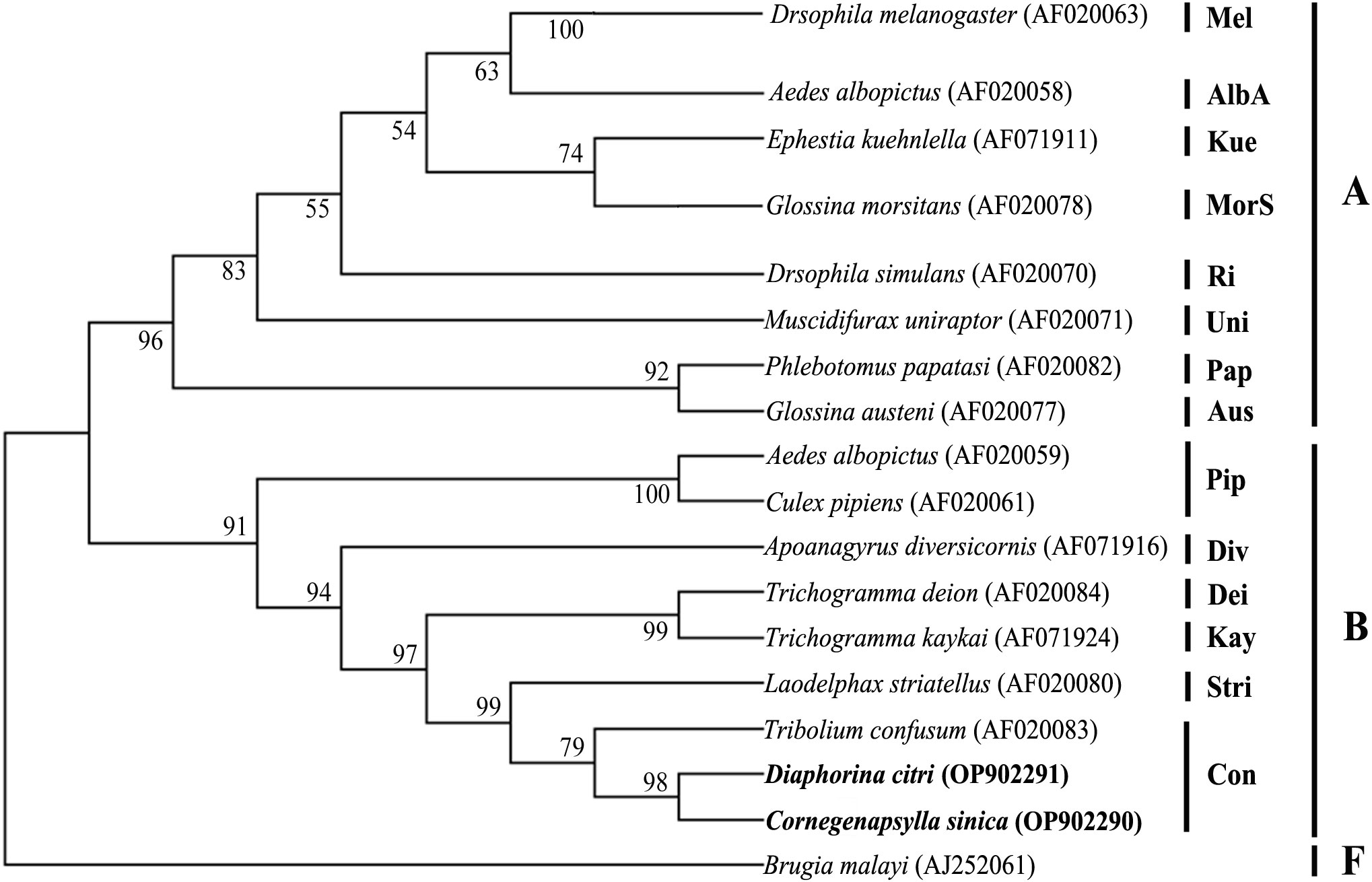
Figure 3 The phylogenetic relationships of Wolbachia from different insect hosts based on the DNA sequence of wsp gene. The tree was constructed and analyzed by Neighbor-Joining (NJ) method using 1000 bootstraps replicates.
Our Bayesian phylogenetic analysis of the Wolbachia indicated that all the Wolbachia strains in the D. citri populations collected from different regions in China were highly homologous; clustering into one branch. There were small variants to the strains of D. citri populations collected from South Asia, West Asia and America in another sister branch. Although Wolbachia wSin in C. sinica also belongs to the Wolbachia supergroup B, it is phylogenetically distant from all the Wolbachia wDi strains (Figure 4).When analyzed, the phylogeny of Wolbachia different lines based on the MLST genes, Wolbachia lines of C. sinica (ST532) and Drosophila simulans (ST16) were firstly clustered into one branch, then into another branch with Wolbachia of D. citri (ST173). Wolbachia lines of B. tabaci (ST378) and T. confusum (ST30) were firstly clustered into one branch together, becoming a sister branch of Wolbachia lines of C. sinica, D. simulans and D. citri in supergroup B (Figure 5). This result is consistent with the phylogeny of Wolbachia based on the wsp, gatB, coxA, hcpA, ftsZ, and fbpA genes (Figures 3, S1).
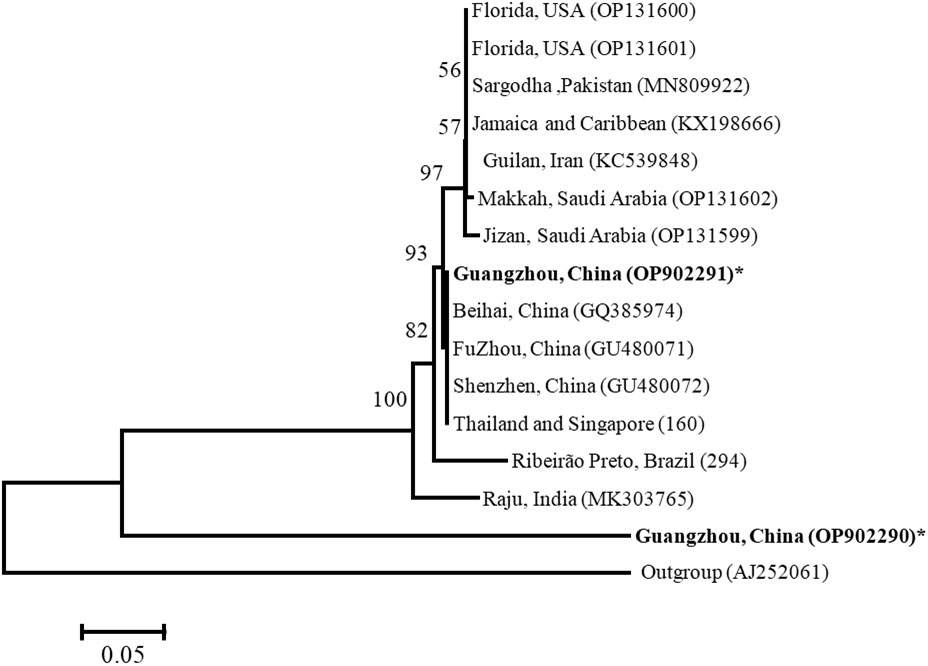
Figure 4 Bayesian analysis of Wolbachia in Diaphorina citri and Cornegenapsylla sinica host Guangzhou (China) populations, compared with those available in GenBank and the Wolbachia database based on wsp gene. The tree was constructed and analyzed by Neighbor-Joining (NJ) method using 1000 bootstraps replicates. Refer to Figure 2 for the meaning of the numbers and branch length.
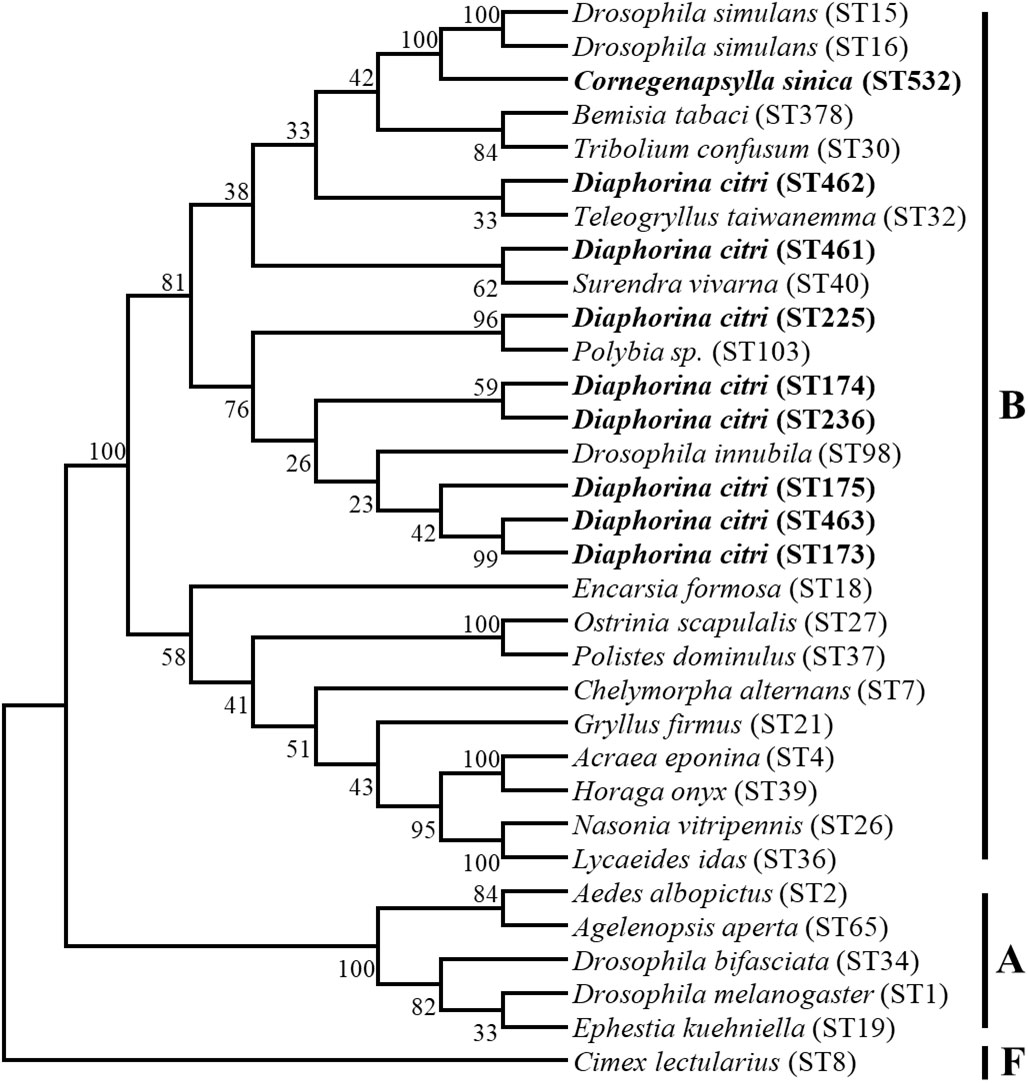
Figure 5 The phylogenetic relationships of Wolbachia from different insect hosts based on the DNA sequence of MLST gene. The tree was constructed and analyzed by Neighbor-Joining (NJ) method using 1000 bootstraps replicates.
Infection of Wolbachia in D. citri and C. sinica
The qRT-PCR results demonstrated that the infection densities of Wolbachia in different tissues of D. citri and C. sinica were consistent. The infection in the midgut was highest, followed by the ovary and testes. Infection in the salivary glands was the lowest when comparing the four tissues (Figure 6).
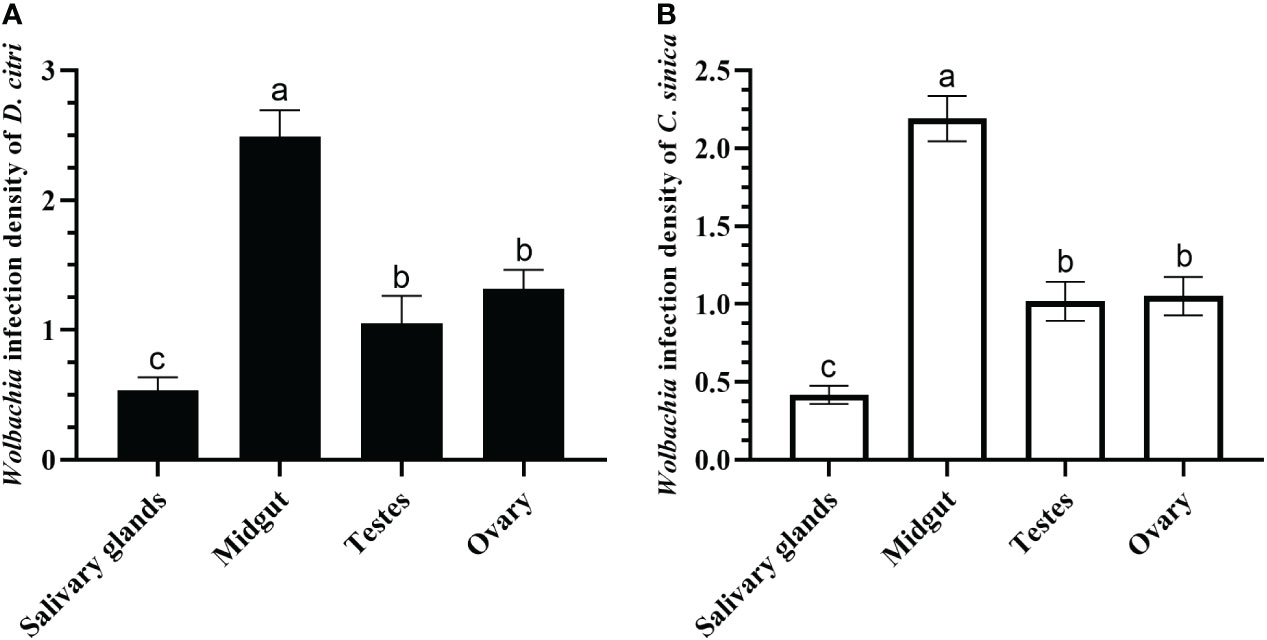
Figure 6 Wolbachia titers in salivary glands, midgut, testes, and ovary of Diaphorina citri (A) and Cornegenapsylla sinica (B). Error bars indicate 95% confidence intervals. Different letters indicate significant differences among different tissues (P <0.05).
Distribution of Wolbachia in D. citri and C. sinica
The localizations of Wolbachia in D. citri and C. sinica hosts were visualized by FISH. Wolbachia was clearly scattered throughout the whole body of the 5th nymphal instar and the reproductive organs of adults (Figure 7).
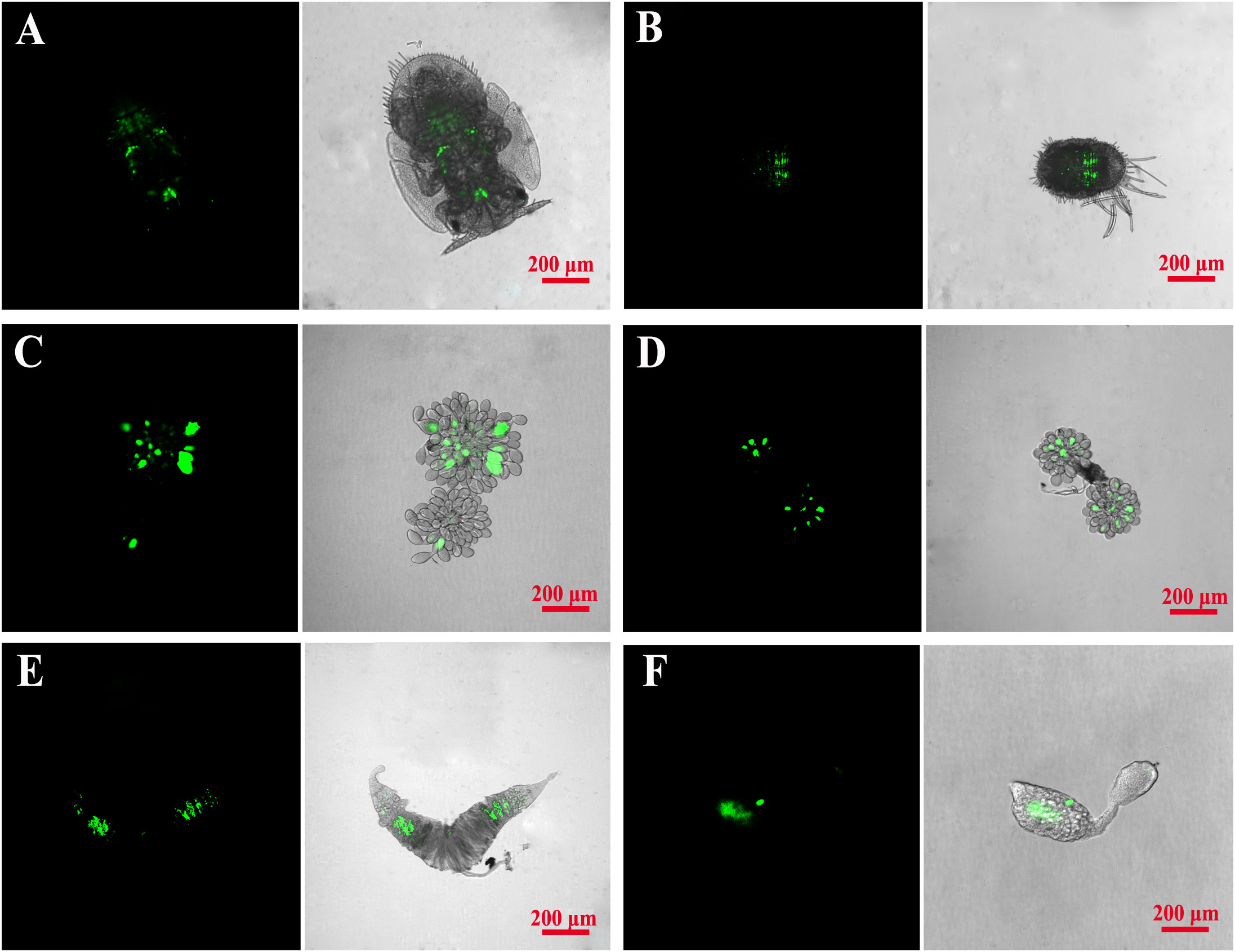
Figure 7 Fluorescence in situ hybridization visualization of Wolbachia in Asian citrus psyllid and longan psyllid. Wolbachia was respectively stained green by specific probes. (A, B): D. citri and C. sinica 4th nymphal stage; (C, D): D. citri and C. sinica ovary; (E, F): D. citri and C. sinica testes.
Discussion
To date, Wolbachia is well studied within aphids, whiteflies and planthoppers, but currently few studies have revealed the presence and physiological roles of Wolbachia in psyllids. This is partly due to the difficulty in getting a Wolbachia negative population via the inactivation method and at the same time not affecting other endosymbionts in the same psyllid host (Liu et al., 2020). In the current study, our results revealed that the infection rates of Wolbachia are 100% in D. citri (CLas vector) and C. sinica (LgWB vector), but much lower or even negative in the psyllids M. sinica, B. occidentalis and P. canarium. Interestingly, based on global samples of Wolbachia, Lashkari et al. (2014) discovered a strong association between the mtCOI gene of D. citri and their Wolbachia strains, while our results of phylogenetic analysis showed that the Wolbachia strains are conserved in the psyllids of Guangzhou (China) populations. Whether the infection of Wolbachia is related to transmit pathogens of these psyllid hosts needs to be further investigated; Understanding this could facilitate our understanding of the interaction relationship between Wolbachia, its vector insect and the pathogen. With regard to the different Wolbachia infection rates in the psyllids, we presumed that the immune-related benefits may be the determining factor. Wolbachia has been confirmed in having the ability to confer protection against pathogen infection in its hosts, leading to reduced pathogen load or decreased host mortality associated with pathogen infection (Hedges et al., 2008). D. citri (CLas vector) and C. sinica (LgWB vector) appear to require larger Wolbachia infection rates (Teixeira et al., 2008; Osborne et al., 2012; Chrostek et al., 2013; Stevanovic et al., 2015), with this hypothesis being evidenced by several previous studies which have revealed that D. citri is naturally infected by the Wolbachia strain wDi at a prevalence of 100% (Meyer and Hoy, 2008; Wang et al., 2010; Guidolin and Consoli, 2013; Chu et al., 2016).
Phylogenetic analysis of the genetic relationship between Wolbachia and their hosts is essential to understanding the evolutionary pathways and transmission processes of Wolbachia in different hosts. The vector mediated interspecific transmission of intracellular bacterial endosymbionts has been confirmed by phylogeny studies that insects sharing the same ecological niche contacts with each other can acquire different Wolbachia strains horizontally, such as sharing the same food sources (Oliver et al., 2010);, host plants (Caspi-Fluger et al., 2012; Li S. J et al., 2017), predators (Jaenike, 2007; Gehrer and Vorburger, 2012) and parasitoids (Duron et al., 2010; Li et al., 2011; Ahmed et al., 2015). Interestingly, Pigeault et al. (2014) reported the strain wCon tended to reduce reproductive investment but maintained or increased immune parameters. Wolbachia strains from D. citri and C. sinica in the current study were clustered into one branch of Con subgroup in the supergroup B, which can assist in predicting the roles of Wolbachia in D. citri and C. sinica.
Wolbachia can also be horizontally transmitted between intraspecies of insect hosts (Ahmed et al., 2013; Chu et al., 2016; Li S. J et al., 2017; Li Y. H et al., 2017; Liu et al., 2023). For example, by comparing the phylogeny of different Bemisia species and their endophytes, Ahmed et al. (2013) revealed the discordance of Wolbachia with their whitefly hosts and testified that Wolbachia can achieve host transfer through horizontal transmission. In the present study, we revealed the Wolbachia lines in D. citri and C. sinica are ST173 and ST532 based on wsp and MLST genes. We therefore predict that horizontal transmission of Wolbachia may not occur between C. sinica and D. citri since they do not share the same host plants.
By using fluorescent in situ hybridization with Wolbachia specific probes, we revealed the spatial distribution of Wolbachia in D. citri and C. sinica. Overall, the distribution patterns of the profiles wDi and wSin also aligned with findings from previous work (Ren et al., 2018), which showed that Wolbachia was clearly scattered throughout the whole body of the 5th nymphal instar and the reproductive organs of adults. The distribution patterns of endosymbionts in their hosts have significant impacts on their transmission and ability to affect their hosts (Li S. J et al., 2017). According to the conclusion of Ahmed et al. (2015), a “scattered” distribution pattern provides more chance for parasitoids to pick up the endosymbiont by their mouthparts when feeding or during a probing check before egg-laying. They then vector the horizontal transmission of the endosymbiont, such as Wolbachia in the current study, between different psyllid individuals.
In addition, the qRT-PCR detection revealed that the infection density of Wolbachia was highest in the midgut, medium in the ovaries and testes, and lowest in the salivary glands of both D. citri and C. sinica. The salivary glands are the key organ for the pathogen transmission of vector insects. Begomoviruses can even self-proliferate in the salivary glands of the whitefly B. tabaci (Wang et al., 2022), and CLas multiplication was also detected in salivary glands of D. citri (Wu et al., 2018). However, Fraser et al. (2020) demonstrated that there is no association between a Wolbachia strain’s ability to inhibit Dengue infection in the mosquito and either its typical density in the midgut or salivary glands, or the degree to which it elevates innate immune response pathways in the mosquito. Concerning the different function of Wolbachia in various hosts’ salivary glands, the interaction relationships between Wolbachia and CLas, Wolbachia and LgWB are worthy of further research.
Significant progress has been achieved in developing Wolbachia based strategies for the control of insect vectors. Guo et al. (2022) demonstrated that the Aedes albopictus HC line infected with a trio of Wolbachia strains exhibited almost complete blockade of Dengue virus (DENV) and Zika virus (ZIKV) in horizontal and vertical transmission. Also, Wolbachia strengthens host immunity, cellular regeneration and causes the expression of microRNAs which could potentially be involved in virus inhibition (Reyes et al., 2021; Yu et al., 2022). Gong et al. (2020) reported the first successful transfer of Wolbachia endosymbiont into a pest planthopper, and that the endosymbiont self-spreads into the host population, causes sufficiently high levels of CI, and inhibits transmission of the rice plant virus RRSV by Nilaparvata lugens. Importantly, it mitigated RRSV associated disease symptoms in rice plants.
When several symbionts are simultaneously present within the same host, interactions between them can take place and affect the dynamics of the microbial population. In these cases, hosts are often seen as shared limited spaces and as resources in which competition for exploitation, termed ‘the tragedy of the commons’ occurs (Vautrin and Vavre, 2009). Bacterial competition for limited resources occurs within infected Wolbachia populations but not in uninfected Wolbachia populations, therefore, implying that bacterial interactions can cause differences in pathogen infection rates among various insect populations (Vautrin and Vavre, 2009). The populations of vector insects carrying Wolbachia are more challenging to infect with pathogens (Krstić et al., 2018). Therefore, the strength of pathogen inhibition is considered to depend on the density of Wolbachia (Teixeira et al., 2008). Further research is also required to determine whether Wolbachia can affect LgWB spread and for its utilization in C. sinica control.
Conclusions
To summarize, characterizing the diversity and ecology of Wolbachia may shed light on the coevolution of Wolbachia and its psyllid hosts, as well as the interactions between psyllid borne pathogens. In addition, to identifying the Wolbachia strains in D. citri and C. sinica psyllid species, findings from this work may benefit the understanding of Wolbachia psyllid relationships. Since Wolbachia induced CI could play an important role in insect pest or pathogen control strategies by reducing insect population size or acting as a drive system for disseminating desirable genes/alleles (Sinkins and Gould, 2006), the next research objectives should be the CI function identification for the ST173 Wolbachia line in D. citri and ST532 Wolbachia line in C. sinica. This would provide new insights for the development of D. citri CLas and C. sinica LgWB control strategies.
Data availability statement
The datasets presented in this study can be found in online repositories. The names of the repository/repositories and accession number(s) can be found below: https://www.ncbi.nlm.nih.gov/, MN728680, https://www.ncbi.nlm.nih.gov/, OP902291, https://www.ncbi.nlm.nih.gov/, OP902290.
Author contributions
DO and B-LQ, conceived and designed the experiments. DO, J-HQ, Z-QS, and LW, performed the experiments. DO, J-HQ, and Z-QS, analyzed the data. B-LQ, contributed to reagents, materials, and analysis tools. DO and B-LQ, wrote the manuscript. All authors contributed to the article and approved the submitted version.
Funding
This study was supported by the Laboratory of Lingnan Modern Agriculture Project (NT2021003), the Open Competition Program of Agricultural Science and Technology Innovation for the 14th Five-year Plan of Guangdong Province (2022SDZG06) and the National High-Level Talent Special Support Plan (2020).
Acknowledgments
We thank Dr. Andrew G. S. Cuthbertson (York, United Kingdom) for his critical comments on an earlier version of the manuscript.
Conflict of interest
The authors declare that the research was conducted in the absence of any commercial or financial relationships that could be construed as a potential conflict of interest.
Publisher’s note
All claims expressed in this article are solely those of the authors and do not necessarily represent those of their affiliated organizations, or those of the publisher, the editors and the reviewers. Any product that may be evaluated in this article, or claim that may be made by its manufacturer, is not guaranteed or endorsed by the publisher.
Supplementary material
The Supplementary Material for this article can be found online at: https://www.frontiersin.org/articles/10.3389/fcimb.2023.1121186/full#supplementary-material
Supplementary Figure 1 | The phylogenetic relationships of Wolbachia from different insect hosts based on the DNA sequence of (A) coxA, (B) fbpA, (C) ftsZ, (D) gatB and (E) hcpA gene. The tree was constructed and analyzed by Neighbor-Joining (NJ) method using 1000 bootstraps replicates.
References
Ahmed, M. Z., De Barro, P. J., Ren, S. X., Greeff, J. M., Qiu, B. L. (2013). Evidence for horizontal transmission of secondary endosymbionts in the Bemisia tabaci cryptic species complex. PloS One 8, e53084. doi: 10.1371/journal.pone.0053084
Ahmed, M. Z., Li, S. J., Xue, X., Yin, X. J., Ren, S. X., Jiggins, F. M., et al. (2015). The intracellular bacterium Wolbachia uses parasitoid wasps as phoretic vectors for efficient horizontal transmission. PloS Pathog. 10, e1004672. doi: 10.1371/journal.ppat.1004672
Baldo, L., Dunning Hotopp, J. C., Jolley, K. A., Bordenstein, S. R., Biber, S. A., Choudhury, R. R., et al. (2006). Multilocus sequence typing system for the endosymbiont Wolbachia pipientis. Appl. Environ. Microb. 72 (11), 7098–7110. doi: 10.1128/AEM.00731-06
Bian, G., Joshi, D., Dong, Y., Lu, P., Zhou, G., Pan, X., et al. (2013). Wolbachia invades Anopheles stephensi populations and induces refractoriness to Plasmodium infection. Science 340 (6133), 748–751. doi: 10.1126/science.1236192
Bing, X. L., Xia, W. Q., Gui, J. D., Yan, G. H., Wang, X. W., Liu, S. S. (2014). Diversity and evolution of the Wolbachia endosymbionts of Bemisia (Hemiptera: Aleyrodidae) whiteflies. Ecol. Evol. 4, 2714–2737. doi: 10.1002/ece3.1126
Boykin, L. M., Armstrong, K. F., Kubatko, L., De Barro, P. (2012). Species delimitation and global biosecurity. Evol. Bioinform. 8, EBO–S8532. doi: 10.4137/EBO.S8532
Brownlie, J. C., Cass, B. N., Riegler, M., Witsenburg, J. J., IturbeOrmaetxe, I., McGraw, E. A. (2009). Evidence for metabolic provisioning by a common invertebrate endosymbiont, Wolbachia pipientis, during periods of nutritional stress. PloS Pathog. 5, e1000368. doi: 10.1371/journal.ppat.1000368
Caspi-Fluger, A., Inbar, M., Mozes-Daube, N., Katzir, N., Portnoy, V., Belausov, E., et al. (2012). Horizontal transmission of the insect symbiont Rickettsia is plant-mediated. Proc. R. Soc B-Biol. Sci. 279, 1791–1796. doi: 10.1098/rspb.2011.2095
Chen, X. D., Neupane, S., Gossett, H., Pelz-Stelinski, K. S., Stelinski, L. L. (2021). Insecticide rotation scheme restores insecticide susceptibility in thiamethoxam-resistant field populations of Asian citrus psyllid, Diaphorina citri kuwayama (Hemiptera: Liviidae), in Florida. Pest Manage. Sci. 77 (1), 464–473. doi: 10.1002/ps.6039
Chen, Y. J., Xu, C. P., Li, K. B., Xia, Y. H. (1992). Insect transmission test of longan pathogen witches’broom virus. Acta Phytopath. Sin. 22 (3), 245–249. doi: 10.13926/j.cnki.apps.1992.03.018
Chrostek, E., Marialva, M. S. P., Esteves, S. S., Weinert, L. A., Martinez, J., Jiggins, F.M., et al. (2013). Wolbachia variants induce differential protection to viruses in Drosophila melanogaster: a phenotypic and phylogenomic analysis. PloS Genet. 9, e1003896. doi: 10.1371/journal.pgen.1003896
Chu, C. C., Gill, T. A., Hoffmann, M., Pelz-Stelinski, K. S. (2016). Inter-population variability of endosymbiont densities in the Asian citrus psyllid (Diaphorina citri kuwayama). Microb. Ecol. 71, 999–1007. doi: 10.1007/s00248-016-0733-9
Crawford, J. E., Clarke, D. W., Criswell, V., Desnoyer, M., Cornel, D., Deegan, B., et al. (2020). Efficient production of male Wolbachia-infected Aedes aegypti mosquitoes enables large-scale suppression of wild populations. Nat. Biotechnol. 38 (4), 482–492. doi: 10.1038/s41587-020-0471-x
Cuthbertson, A. G. S., Vanninen, I. (2015). The importance of maintaining protected zone status against Bemisia tabaci. Insects 6, 432–441. doi: 10.3390/insects6020432
Dale, C., Beeton, M., Harbison, C., Jones, T., Pontes, M. (2006). Isolation, pure culture, and characterization of “Candidatus arsenophonus arthropodicus,” an intracellular secondary endosymbiont from the hippoboscid louse fly Pseudolynchia canariensis. Appl. Environ. Microbiol. 72 (4), 2997–3004. doi: 10.1128/AEM.72.4.2997-3004.2006
Dedeine, F., Vavre, F., Fleury, F., Loppin, B., Hochberg, M. E., Boulétreau, M. (2001). Removing symbiotic Wolbachia bacteria specifically inhibits oogenesis in a parasitic wasp. Proc. Natl. Acad. Sci. U.S.A. 98, 6247–6252. doi: 10.1073/pnas.101304298
Dobson, S. L., Rattanadechakul, W., Marsland, E. J. (2004). Fitness advantage and cytoplasmic incompatibility in Wolbachia single- and superinfected Aedes albopictus. Heredity 93, 135. doi: 10.1038/sj.hdy.6800458
Dong, P., Wang, J. J., Zhao, Z. M. (2006). Infection by Wolbachia bacteria and its influence on the reproduction of the stored-product psocid, Liposcelis tricolor. J. Insect Sci. 6, 24. doi: 10.1673/2006_06_24.1
Duron, O., Wilkes, T. E., Hurst, G. D. D. (2010). Interspecific transmission of a male-killing bacterium on an ecological timescale. Ecol. Lett. 13, 1139–1148. doi: 10.1111/j.1461-0248.2010.01502.x
Fagen, J. R., Giongo, A., Brown, C. T., Davis-Richardson, A. G., Gano, K. A., Triplett, E. W. (2012). Characterization of the relative abundance of the citrus pathogen Ca. liberibacter asiaticus in the microbiome of its insect vector, Diaphorina citri, using high throughput 16S rRNA sequencing. Open Microbiol. J. 6, 29. doi: 10.2174/1874285801206010029
Fraser, J. E., O’Donnell, T. B., Duyvestyn, J. M., O’Neill, S. L., Simmons, C. P., Flores, H. A. (2020). Novel phenotype of Wolbachia strain wPip in Aedes aegypti challenges assumptions on mechanisms of Wolbachia-mediated dengue virus inhibition. PloS Pathog. 16 (7), e1008410. doi: 10.1371/journal.ppat.1008410
Frentiu, F. D., Zakir, T., Walker, T., Popovici, J., Pyke, A. T., van den Hurk, A., et al. (2014). Limited dengue virus replication in field-collected Aedes aegypti mosquitoes infected with Wolbachia. PloS Negl. Trop. Dis. 8 (2), e2688. doi: 10.1371/journal.pntd.0002688
Fry, A. J., Palmer, M. R., Rand, D. M. (2004). Variable fitness effects of Wolbachia infection in Drosophila melanogaster. Heredity 93, 379. doi: 10.1038/sj.hdy.6800514
Gehrer, L., Vorburger, C. (2012). Parasitoids as vectors of facultative bacterial endosymbionts in aphids. Biol. Lett. 8, 613–615. doi: 10.1098/rsbl.2012.0144
Gong, J. T., Li, Y., Li, T. P., Liang, Y., Hu, L., Zhang, D., et al. (2020). Stable introduction of plant-virus-inhibiting Wolbachia into planthoppers for rice protection. Curr. Biol. 30 (24), 4837–4845. doi: 10.1016/j.cub.2020.09.033
Guidolin, A. S., Consoli, F. L. (2013). Molecular characterization of Wolbachia strains associated with the invasive Asian citrus psyllid Diaphorina citri in Brazil. Microb. Ecol. 65 (2), 475–486. doi: 10.1007/s00248-012-0150-7
Guo, Y., Guo, J., Li, Y. (2022). Wolbachia wPip blocks zika virus transovarial transmission in Aedes albopictus. Microbiol. Spectr. 10 (5), e02633–e02621. doi: 10.1128/spectrum.02633-21
Halbert, S. E., Manjunath, K. L. (2004). Asian Citrus psyllids (Sternorrhyncha: Psyllidae) and greening disease of citrus: a literature review and assessment of risk in Florida. Fla. Entomol. 87 (3), 330–353. doi: 10.1653/0015-4040(2004)087[0330:ACPSPA]2.0.CO;2
Hancock, P. A., Sinkins, S. P., Godfray, H. C. J. (2011). Strategies for introducing Wolbachia to reduce transmission of mosquito-borne diseases. PloS Negl. Trop. Dis. 5 (4), e1024. doi: 10.1371/journal.pntd.0001024
Hedges, L. M., Brownlie, J. C., O’Neill, S. L., Johnson, K. N. (2008). Wolbachia and virus protection in insects. Science 322, 702. doi: 10.1126/science.1162418
Hoffmann, A. A., Montgomery, B. L., Popovici, J., Iturbe-Ormaetxe, I., Johnson, P. H., Muzzi, F., et al. (2011). Successful establishment of Wolbachia in Aedes populations to suppress dengue transmission. Nature 476 (7361), 454–457. doi: 10.1038/nature10356
Hosokawa, T., Koga, R., Kikuchi, Y., Meng, X. Y., Fukatsu, T. (2010). Wolbachia as a bacteriocyte-associated nutritional mutualist. Proc. Natl. Acad. Sci. U.S.A. 107, 769–774. doi: 10.1073/pnas.0911476107
Ilinsky, Y., Demenkova, M., Bykov, R., Bugrov, A. (2022). Narrow genetic diversity of Wolbachia symbionts in acrididae grasshopper hosts (Insecta, orthoptera). Int. J. Mol. Sci. 23, 853. doi: 10.3390/ijms23020853
Iturbe-Ormaetxe, I., Walker, T., O’ Neill, S. L. (2011). Wolbachia and the biological control of mosquito-borne disease. EMBO Rep. 12, 508–518. doi: 10.1038/embor.2011.84
Jaenike, J. (2007). Spontaneous emergence of a new Wolbachia phenotype. Evolution 61 (9), 2244–2252. doi: 10.1111/j.1558-5646.2007.00180.x
Jain, M., Fleites, L. A., Gabriel, D. W. A. (2017). Small Wolbachia protein directly represses phage lytic cycle genes in “Candidatus liberibacter asiaticus” within psyllids. MSphere 2, e00171. doi: 10.1128/mSphereDirect.00171-17
Kremer, N., Charif, D., Henri, H., Bataille, M., Prevost, G., Kraaijeveld, K. (2009). A new case of Wolbachia dependence in the genus Asobara: evidence for parthenogenesis induction in Asobara japonica. Heredity 103, 248–256. doi: 10.1038/hdy.2009.63
Krstić, O., Cvrković, T., Mitrović, M., Radonjić, S., Hrnčić, S., Toševski, I., et al. (2018). Wolbachia infection in natural populations of Dictyophara europaea, an alternative vector of grapevine flavescence dorée phytoplasma: effects and interactions. Ann. Appl. Biol. 172 (1), 47–64. doi: 10.1111/aab.12400
Laidoudi, Y., Marié, J. L., Tahir, D., Watier-Grillot, S., Mediannikov, O., Davoust, B. (2020). Detection of canine vector-borne filariasis and their Wolbachia endosymbionts in French Guiana. Microorganisms 8 (5), 770. doi: 10.3390/microorganisms8050770
Lashkari, M., Manzari, S., Sahragard, A., Malagnini, V., Boykin, L. M., Hosseini, R. (2014). Global genetic variation in the Asian citrus psyllid, Diaphorina citri (Hemiptera: Liviidae) and the endosymbiont Wolbachia: links between Iran and the USA detected. Pest Manage. Sci. 70 (7), 1033–1040. doi: 10.1002/ps.3643
Li, S. J., Ahmed, M. Z., Lv, N., Shi, P. Q., Wang, X. M., Huang, J. L., et al. (2017). Plant-mediated horizontal transmission of Wolbachia between whiteflies. ISME J. 11, 1019–1028. doi: 10.1038/ismej.2016.164
Li, S. J., Xue, X., Ahmed, M. Z., Ren, S. X., Du, Y. Z., Wu, J. H., et al. (2011). Host plants and natural enemies of Bemisia tabaci (Hemiptera: Aleyrodidae) in China. Insect Sci. 18, 101–120. doi: 10.1111/j.1744-7917.2010.01395.x
Li, Y. H., Ahmed, M. Z., Li, S. J., Lv, N., Shi, P. Q., Chen, X. S., et al. (2017). Plant-mediated horizontal transmission of Rickettsia endosymbiont between different whitefly species. FEMS Microbiol. Ecol. 93, fix138. doi: 10.1093/femsec/fix138
Li, F., Li, P., Hua, H., Hou, M., Wang, F. (2020). Diversity, tissue localization, and infection pattern of bacterial symbionts of the white-backed planthopper, Sogatella furcifera (Hemiptera: Delphacidae). Microb. Ecol. 79, 720–730. doi: 10.1007/s00248-019-01433-4
Liu, Y., Fan, Z. Y., An, X., Shi, P. Q., Ahmed, M. Z., Qiu, B. L. (2020). A single-pair method to screen Rickettsia-infected and uninfected whitefly Bemisia tabaci populations. J. Microbiol. Meth. 168, 105797. doi: 10.1016/j.mimet.2019.105797
Liu, Y., He, Z. Q., Wen, Q., Peng, J., Zhou, Y. T., Mandour, N., et al. (2023). Parasitoid-mediated horizontal transmission of Rickettsia between whiteflies. Front. Cell. Infect. Microbiol. 12. doi: 10.3389/fcimb.2022.1077494
Lo, N., Paraskevopoulos, C., Bourtzis, K., O’Neill, S., Werren, J., Bordenstein, S. (2007). Taxonomic status of the intracellular bacterium Wolbachia pipientis. Int. J. Syst. Evol. Microbiol. 57, 654. doi: 10.1099/ijs.0.64515-0
Lv, N., Peng, J., Chen, X. Y., Guo, C. F., Sang, W., Wang, X. M., et al. (2021). Antagonistic interaction between male-killing and cytoplasmic incompatibility induced by Cardinium and Wolbachia in the whitefly Bemisia tabaci. Insect Sci. 28, 330–346. doi: 10.1111/1744-7917.12793
Meyer, J. M., Hoy, M. A. (2008). Molecular survey of endosymbionts in Florida populations of Diaphorina citri (Hemiptera: Psyllidae) and its parasitoids Tamarixia radiata (Hymenoptera: Eulophidae) and Diaphorencyrtus aligarhensis (Hymenoptera: Encyrtidae). Fla. Entomol. 91 (2), 294–304. doi: 10.1653/0015-4040
Moreira, L. A., Iturbe-Ormaetxe, I., Jeffery, J. A., Lu, G., Pyke, A. T., Hedges, L. M., et al. (2009). A Wolbachia symbiont in Aedes aegypti limits infection with dengue, chikungunya, and plasmodium. Cell 139 (7), 1268–1278. doi: 10.1016/j.cell.2009.11.042
Neupane, S., Bonilla, S. I., Manalo, A. M., Pelz-Stelinski, K. S. (2022). Complete de novo assembly of Wolbachia endosymbiont of Diaphorina citri kuwayama (Hemiptera: Liviidae) using long-read genome sequencing. Sci. Rep. 12 (1), 1–16. doi: 10.1038/s41598-021-03184-0
Oliver, K. M., Degnan, P. H., Burke, G. R., Moran, N. A. (2010). Facultative symbionts in aphids and the horizontal transfer of ecologically important traits. Annu. Rev. Entomol. 55, 247–266. doi: 10.1146/annurev-ento-112408-085305
Osborne, S. E., Iturbe-Ormaetxe, I., Brownlie, J. C., O’Neill, S. L., Johnson, K. N. (2012). Antiviral protection and the importance of Wolbachia density and tissue tropism in Drosophila simulans. appl. Environ. Microbiol. 78, 6922–6929. doi: 10.1128/AEM.01727-12
Percy, D. M., Crampton-Platt, A., Sveinsson, S., Lemmon, A. R., Lemmon, E. M., Ouvrard, D., et al. (2018). Resolving the psyllid tree of life: phylogenomic analyses of the superfamily psylloidea (Hemiptera). Syst. Entomol. 43 (4), 762–776. doi: 10.1111/syen.12302
Pigeault, R., Braquart-Varnier, C., Marcadé, I., Mappa, G., Mottin, E., Sicard, M. (2014). Modulation of host immunity and reproduction by horizontally acquired Wolbachia. J. Insect Physiol. 70, 125–133. doi: 10.1016/j.jinsphys.2014.07.005
Ren, S. L., Li, Y. H., Ou, D., Guo, Y. J., Qureshi, J. A., Stansly, P. A., et al. (2018). Localization and dynamics of Wolbachia infection in Asian citrus psyllid Diaphorina citri, the insect vector of the causal pathogens of huanglongbing. MicrobiologyOpen 7 (3), e00561. doi: 10.1002/mbo3.561
Reyes, J. I. L., Suzuki, Y., Carvajal, T., Muñoz, M. N. M., Watanabe, K. (2021). Intracellular interactions between arboviruses and Wolbachia in Aedes aegypti. Front. Cell Infect. Microbiol. 11, 690087. doi: 10.3389/fcimb.2021.690087
Ros, V. I. D., Fleming, V. M., Feil, E. J., Breeuwer, J. A. J. (2009). How diverse is the genus Wolbachia? multiple-gene sequencing reveals a putatively new Wolbachia supergroup recovered from spider mites (Acari: Tetranychidae). Appl. Environ. Microbiol. 75, 1036–1043. doi: 10.1128/AEM.01109-08
Ryan, P. A., Turley, A. P., Wilson, G., Hurst, T. P., Retzki, K., Brown-Kenyon, J., et al. (2019). Establishment of wMel Wolbachia in Aedes aegypti mosquitoes and reduction of local dengue transmission in cairns and surrounding locations in northern Queensland, Australia. Gates Open Res. 3, 1547. doi: 10.12688/gatesopenres.13061.1
Saridaki, A., Bourtzis, K. (2010). Wolbachia: More than just a bug in insects genitals. Curr. Opin. Microbiol. 13 (1), 67–72. doi: 10.1016/j.mib.2009.11.005
Seo, J. K., Kim, M. K., Kwak, H. R., Kim, J. S., Choi, H. S. (2017). Complete genome sequence of longan witches’ broom-associated virus, a novel member of the family Potyviridae. Arch. Virol. 162, 2885–2889. doi: 10.1007/s00705-017-3405-2
Sinkins, S. P., Gould, F. (2006). Gene drive systems for insect disease vectors. Nat. Rev. Genet. 7 (6), 427–435. doi: 10.1038/nrg1870
Stevanovic, A. L., Arnold, P. A., Johnson, K. N. (2015). Wolbachia-mediated antiviral protection in Drosophila larvae and adults following oral infection. Appl. Environ. Microbiol. 81, 8215–8223. doi: 10.1128/AEM.02841-15
Stouthamer, R., Breeuwer, J. A., Hurst, G. D. (1999). Wolbachia pipientis: microbial manipulator of arthropod reproduction. Annu. Rev. Microbiol. 53 (1), 71–102. doi: 10.1146/annurev.micro.53.1.71
Tan, C. H., Wong, P. J., Li, M. I., Yang, H., Ng, L. C., O’Neill, S. L. (2017). wMel limits zika and chikungunya virus infection in a Singapore Wolbachia-introgressed Ae. aegypti strain, wMel-sg. PloS Negl. Trop. Dis. 11 (5), e0005496. doi: 10.1371/journal.pntd.0005496
Teixeira, L., Ferreira, A., Ashburner, M., Lurent, K. (2008). The bacterial symbiont Wolbachia induces resistance to RNA viral infections in Drosophila melanogaster. PloS Biol. 6, e2. doi: 10.1371/journal.pbio.1000002
Timmermans, M. J. T. N., Ellers, J. (2008). Wolbachia endosymbiont is essential for egg hatching in a parthenogenetic arthropod. Evol. Ecol. 23, 931. doi: 10.1007/s10682-008-9282-0
Tran, H., Van, H. N., Muniappan, R., Amrine, J., Naidu, R., Gilbertson, R., et al. (2019). Integrated pest management of longan (Sapindales: Sapindaceae) in Vietnam. Int. J. Pest Manage. 10 (1), 18. doi: 10.1093/jipm/pmz016
Van den Hurk, A. F., Hall-Mendelin, S., Pyke, A. T., Frentiu, F. D., McElroy, K., Day, A., et al. (2012). Impact of Wolbachia on infection with chikungunya and yellow fever viruses in the mosquito vector Aedes aegypti. PloS Negl. Trop. Dis. 6 (11), e1892. doi: 10.1371/journal.pntd.0001892
Vautrin, E., Vavre, F. (2009). Interactions between vertically transmitted symbionts: cooperation or conflict? Trends Microbiol. 17 (3), 95–99. doi: 10.1016/j.tim.2008.12.002
Walker, T. J. P. H., Johnson, P. H., Moreira, L. A., Iturbe-Ormaetxe, I., Frentiu, F. D., McMeniman, C. J., et al. (2011). The wMel Wolbachia strain blocks dengue and invades caged Aedes aegypti populations. Nature 476 (7361), 450–453. doi: 10.1038/nature10355
Wang, Y. M., He, Y. Z., Ye, X. T., Guo, T., Pan, L. L., Liu, S. S., et al. (2022). A balance between vector survival and virus transmission is achieved through JAK/STAT signaling inhibition by a plant virus. Proc. Natl. Acad. Sci. U.S.A. 119 (41), e2122099119. doi: 10.2737/FPL-GTR-290
Wang, Z., Tian, S., Xian, J., Chen, S., Liu, T., Yin, Y. (2010). Detection and phylogenetic analysis of Wolbachia in the Asian citrus psyllid (Diaphorina citri) (Homoptera: Psylloidea) populations in partial areas in China. Acta Entomo Sin. 53 (9), 1045–1054. doi: 10.16380/j.kcxb.2010.09.015
Wu, F., Huang, J., Xu, M., Fox, E. G., Beattie, G. A. C., Holford, P., et al. (2018). Host and environmental factors influencing ‘Candidatus liberibacter asiaticus’ acquisition in Diaphorina citri. Pest Manage. Sci. 74 (12), 2738–2746. doi: 10.1002/ps.5060
Yu, J., Li, J. (2022). A delay suppression model with sterile mosquitoes release period equal to wild larvae maturation period. J. Mathematical Biol. 84 (3), 14. doi: 10.1007/s00285-022-01718-2
Zheng, B., Liu, X., Tang, M., Xi, Z., Yu, J. (2019). Use of age-stage structural models to seek optimal Wolbachia-infected male mosquito releases for mosquito-borne disease control. J. Theor. Biol. 472, 95–109. doi: 10.1016/j.jtbi.2019.04.010
Zhou, W., Rousset, F., O'Neill, S. (1998). Phylogeny and PCR-based classification of Wolbachia strains using wsp gene sequences. Proc. R. Soc B-Biol. Sci. 265, 509–515. doi: 10.1098/rspb.1998.0324
Zong, Q., Mao, B., Zhang, H. B., Wang, B., Yu, W. J., Wang, Z. W., et al. (2022). Comparative ubiquitome analysis reveals deubiquitinating effects induced by Wolbachia infection in Drosophila melanogaster. Int. J. Mol. Sci. 23 (16), 9459. doi: 10.3390/ijms23169459
Zug, R., Hammerstein, P. (2012). Still a host of hosts for Wolbachia: analysis of recent data suggests that 40% of terrestrial arthropod species are infected. PloS One 7, e38544. doi: 10.1371/journal.pone.0038544
Keywords: Cornegenapsylla sinica, Diaphorina citri, Wolbachia, pathogen vector, phylogeny, localization pattern
Citation: Ou D, Qiu J-H, Su Z-Q, Wang L and Qiu B-L (2023) The phylogeny and distribution of Wolbachia in two pathogen vector insects, Asian citrus psyllid and Longan psyllid. Front. Cell. Infect. Microbiol. 13:1121186. doi: 10.3389/fcimb.2023.1121186
Received: 11 December 2022; Accepted: 21 February 2023;
Published: 06 March 2023.
Edited by:
Joseph James Gillespie, University of Maryland, United StatesReviewed by:
Olivier Duron, Centre National de la Recherche Scientifique (CNRS), FranceDan-Tong Zhu, The University of Sydney, Australia
Davide Sassera, University of Pavia, Italy
Copyright © 2023 Ou, Qiu, Su, Wang and Qiu. This is an open-access article distributed under the terms of the Creative Commons Attribution License (CC BY). The use, distribution or reproduction in other forums is permitted, provided the original author(s) and the copyright owner(s) are credited and that the original publication in this journal is cited, in accordance with accepted academic practice. No use, distribution or reproduction is permitted which does not comply with these terms.
*Correspondence: Bao-Li Qiu, YmFvbGlxaXVAY3FudS5lZHUuY24=
†These authors have contributed equally to this work