- 1Department of Microbiology and Immunology, Louisiana State University (LSU) Health Sciences Center at Shreveport, Shreveport, LA, United States
- 2Department of Pharmacology, Toxicology, and Neuroscience, Louisiana State University Health Sciences Center at Shreveport, Shreveport, LA, United States
Introduction: Bordetella are respiratory pathogens comprised of three classical Bordetella species: B. pertussis, B. parapertussis, and B. bronchiseptica. With recent surges in Bordetella spp. cases and antibiotics becoming less effective to combat infectious diseases, there is an imperative need for novel antimicrobial therapies. Our goal is to investigate the possible targets of host immunomodulatory mechanisms that can be exploited to promote clearance of Bordetella spp. infections. Vasoactive intestinal peptide (VIP) is a neuropeptide that promotes Th2 anti-inflammatory responses through VPAC1 and VPAC2 receptor binding and activation of downstream signaling cascades.
Methods: We used classical growth in vitro assays to evaluate the effects of VIP on Bordetella spp. growth and survival. Using the three classical Bordetella spp. in combination with different mouse strains we were able to evaluate the role of VIP/VPAC2 signaling in the infectious dose 50 and infection dynamics. Finally using the B. bronchiseptica murine model we determine the suitability of VPAC2 antagonists as possible therapy for Bordetella spp. infections.
Results: Under the hypothesis that inhibition of VIP/VPAC2 signaling would promote clearance, we found that VPAC2-/- mice, lacking a functional VIP/VPAC2 axis, hinder the ability of the bacteria to colonize the lungs, resulting in decreased bacterial burden by all three classical Bordetella species. Moreover, treatment with VPAC2 antagonists decrease lung pathology, suggesting its potential use to prevent lung damage and dysfunction caused by infection. Our results indicate that the ability of Bordetella spp. to manipulate VIP/VPAC signaling pathway appears to be mediated by the type 3 secretion system (T3SS), suggesting that this might serve as a therapeutical target for other gram-negative bacteria.
Conclusion: Taken together, our findings uncover a novel mechanism of bacteria-host crosstalk that could provide a target for the future treatment for whooping cough as well as other infectious diseases caused primarily by persistent mucosal infections.
Introduction
“Whooping cough” or pertussis disease is a re-emerging illness responsible for over 160,000 childhood deaths in 2014 (Yeung et al., 2017), with 30% being in neonates and most being infants under 6 weeks of age (Shi et al., 2021). Despite high vaccine coverage, numbers of cases are increasing due to the replacement of a whole-cell pertussis vaccine with an acellular vaccine (Smallridge et al., 2014; Dorji et al., 2018). The classical Bordetella species consist of three highly similar species which cause infection in a wide variety of mammals. Bordetella pertussis (BP) has the most stringent host range restricted only to humans (Mattoo and Cherry, 2005; van Beek et al., 2018; Decker and Edwards, 2021); Bordetella parapertussis (BPP), which is a pathogen of humans and sheep; and Bordetella bronchiseptica (BB), that has a broader host range including humans and other mammals (Kilgore et al., 2016). Interestingly, BB is a natural pathogen of mice, which causes a disease that mimics the human persistent illness, providing a natural model of disease to study in-depth host-pathogen interactions (Rougier et al., 2006; Zhao et al., 2011; Mills and Gerdts, 2014; Linz et al., 2016; Gestal et al., 2019). Importantly, BB is the evolutionary ancestor of BPP and BP, which resulted in the nascent classical strains containing a restricted genome paired with a greater host specificity (Parkhill et al., 2003; Park et al., 2012). However, many Bordetella spp. virulence factors, such as the type 3 secretion system (Linz et al., 2016), still remain conserved among them. Additionally, BB causes disease by robustly and persistently colonizing in the murine respiratory tract with an inoculum as low as 5 CFU (Weyrich et al., 2014). With this, murine models allow us to explore how Bordetella spp. suppress host immune responses at the molecular level. Like other pathogens, Bordetella spp. have evolved mechanisms in order to cause characteristic long-term disease, which allow for the expression of virulence factors (Andreasen and Carbonetti, 2008; Paccani et al., 2008; Fedele et al., 2017; Gestal et al., 2019) to be finely regulated in response to host cues such as blood, serum, iron, CO2, and hormones such as catecholamines (Brickman et al., 2007; Armstrong et al., 2012; Hester et al., 2012; Gestal et al., 2018). Understanding the mechanisms that Bordetella spp. utilize to suppress host-immune responses might provide novel avenues for therapeutic development to treat pertussis disease (Gestal et al., 2019).
Whooping cough has three different stages of disease: the catarrhal stage, which is the most contagious and leads to disease dissemination, the paroxysmal phase that is characterized by the violent and continuous coughing, and the pneumonic stage that can lead to death, especially in infants (Mikelova et al., 2003; Kilgore et al., 2016). Current research focuses on suppressing the nasal colonization to block transmission. However, targeting the pneumonic stage is necessary to prevent deaths or permanent damage to the respiratory system. Macrolides are the most common antibiotic given to patients infected with Bordetella spp. However, in Asia, antibiotic resistance is emerging, posing a risk of worldwide dissemination (Feng et al., 2021; Wu et al., 2022). Current research focuses on immunotherapies that prevent pathology associated with disease. One treatment for Bordetella spp. infection is targeting the sphingosine-1 phosphate receptor, which reduces pathology-associated morbidity but does not affect bacterial numbers (Skerry et al., 2015; Skerry et al., 2017). Other immunotherapies for diseases such as COVID-19 target the vasoactive intestinal peptide or VIP, which modulates the balance between pro- and anti-inflammatory responses (El Karim et al., 2008; Campos-Salinas et al., 2014; Askar et al., 2020) with promising results.
Vasoactive intestinal peptide (VIP) is a 28-amino acid neuropeptide expressed by neurons and leukocytes (Said, 1986). This molecule exerts immunosuppressive functions by interacting with its two G-protein coupled receptors (GPCRs), VPAC1, and VPAC2. In T cells, VIP/VPAC2 signaling promotes selective differentiation of Th2 effectors (Pozo and Delgado, 2004), survival of Th2 T cells (Sharma et al., 2006; Leceta et al., 2021), and alteration of the Th1 ratios in regulatory T- cells (Jimeno et al., 2012), consequently skewing equilibrium towards an anti-inflammatory Th2 response. VIP is one of the most abundant neuropeptides in the lungs (Baraniuk et al., 1990; Pozo and Delgado, 2004), suggesting a critical function for this peptide during lung immune responses. Given the influence this peptide has on the regulatory T-cell response and its abundance in the lungs, we hypothesized that VIP/VPAC2 axis can be utilized as immunotherapy during Bordetella spp. disease to decrease infection factors such as bacterial burden and pathology.
Our results demonstrate that the VIP/VPAC2 axis is critical for the colonization and persistence of all three classical Bordetella spp. in the lower respiratory tract. They also suggest that Bordetella spp. utilize the T3SS, a btrS-mediated mechanism, to manipulate VIP/VPAC2 signaling of the host and promote long-term lung colonization. Our preliminary data indicate that VPAC2 antagonists can be used to decrease lung pathology caused by whooping cough, indicating that the use of this axis for the development of immunotherapies can be applied to attenuate infection caused by Bordetella spp. and possibly other respiratory pathogens and pathologies that in infants can trigger pulmonary hypertension and death (Scanlon et al., 2022).
Materials and methods
Bacterial strains and culture conditions
All Bordetella spp. were cultured on 100 x 15mm Petri dishes containing Difco Bordet-Gengou (BG) agar (BD, cat. 248200) supplemented with 10% sheep defibrinated blood and 20 μg/mL streptomycin, herein referred to as BGS agar (Dewan et al., 2017; Gestal et al., 2018; Gestal et al., 2019). For liquid cultures, Luria-Bertani or Stainer-Scholte media were used (Gestal et al., 2018). The Bordetella strains used include the B. pertussis strain Bp536, B. parapertussis strain Bpp12822, and B. bronchiseptica strain BbRB50 (Gestal et al., 2018). The RB50ΔbtrS knockout (Gestal et al., 2019) and the T3SS knockout (RB50ΔbscN) mutants were generated in a previous study (Yuk et al., 1998). See Table 1 for the bacterial strains used for the following experiments and their associated references.
Bacterial growth in physiologically relevant media supplemented with VIP
To evaluate the antimicrobial activity of VIP (Sigma Aldrich, cat number V6130-250UG) the experiments were performed in a 96-well plate (Corning, Coastar, Cat number 3591). Inoculums of 0.1 OD600 of the 3 classical Bordetella spp. were inoculated in phenol red-free DMEM (Gibco, Cat #3105-028) containing 10% FBS (Gibco, Cat #10-082-147) supplemented with different concentrations of VIP or in artificial sputum matrix media (BioChemazone, Cat #BZ274), to mimic the host environment. The initial concentration was 25nM, considering that this will be a physiologically relevant concentration of VIP (Henning and Sawmiller, 2001). Concentration was doubled up to 200nM, which has been reported to be maximum concentration possible in the brain. To further investigate the ability of VIP to kill bacteria, we then tested up to 1000 nM of VIP in DMEN. Additionally we also evaluated the effects on VIP on synthetic sputum media. The OD600 measurements were performed overnight (≥12 hours) using the Biotek Synergy H1® 96-well plate reader. To calculate the replication time, we followed previously published protocols (Pope et al., 2010) combined with the built-in analytic calculations available in GraphPad Prism v9.5.0.
Animal experiments
Wildtype C57BL/6J mice were purchased from Jackson Laboratories, Bar Harbor, ME. VPAC2-/- mice were gifted to us from Dr. Xiao-Hong in the department of Pharmacology, Toxicology, and Neuroscience at LSU Health Shreveport, who originally purchased the mice from Jackson Laboratories (B6.129P2-Vipr2tm1Ajh/J). Breeding colonies were maintained under the care of the employees and the veterinarians at the animal facility at Louisiana State University health science center at Shreveport, LA. All animal experiments were carried out in accordance with all institutional guidelines (AUP:20-038; AUP:22-031) and performed in at least two or more independent experiments, with the total number of mice used for each experiment indicated in the figure legends. Mice were euthanized using 5% CO2 followed by cervical dislocation. Following euthanasia, the nasal cavity, trachea, and lungs were collected in 1 mL of cold PBS in 2 mL tissue homogenization tubes containing a mixture of 0.5 mm and 1.4 mm ceramic beads.
Effect of pre-incubation with VIP on mouse colonization
To evaluate the effects of preincubation with VIP on virulence, bacteria were cultured for 24h in phenol-free DMEM (Gibco) containing 10% FBS (Gibco) supplemented with a 200nM concentration of VIP. These overnight cultures were used to prepare the inoculums as previously described (Gestal et al., 2018). We euthanized mice at day 3 post-infection (Sukumar et al., 2009; Boehm et al., 2019; Wolf et al., 2021) to accurately analyze the effects of VIP on early events of Bordetella spp: colonization, as measuring bacterial burden at later timepoints such as day 7 or day 14 post-infection would be more representative of the infection peak or the initiation of infection clearance, respectively.
Median infectious dose
To evaluate the effects of the VIP/VPAC2 function on Bordetella spp. colonization in the lower respiratory tract, serial dilutions of the bacterial strains indicated in Table 1 (102 - 106 CFU/mL) were prepared then plated to confirm proper dilution factors were used. C57BL/6J and VPAC2-/- mice were then inoculated with the increasing bacterial concentrations, and bacterial colonies from nasal cavity, trachea, and lungs of the mice (n = 4-8 mice per condition) were enumerated at 7 days post-infection. Measuring bacterial burden at day 7 post-infection is defined as the peak of Bordetella spp. infection, and it also supported by previously published methods used to assess the minimal required dose of bacteria for successful infection (Belhart et al., 2019). Thus, from these values, 50% of the bacterial inoculum required to efficiently infect the mice used for these experiments (also referred to as ID50) was calculated.
Time-course of infection
C57BL/6J and VPAC2-/- mice were intranasally challenged with 30-50μL of PBS containing 1x105 CFU/mL of B. pertussis, B. parapertussis, and B. bronchiseptica (bacterial strains listed in Table 1). Bacterial colonies were enumerated from the nasal cavity, trachea, and lungs by plating serial dilutions in BG agar with 20 μg/ml of Streptomycin once every 7 days, starting from day 7 and ending on day 28 post-infection.
VIP antagonist
For the evaluation of the effects of the administration of an antagonist of the VPAC2 receptor, the compound PG 99-465 was purchased from Bachem Americas, Inc. (cat number H-7292.1000BA). C57BL/6J mice were intranasally challenged with the RB50 strain of B. bronchiseptica. The administration of the treatment consisted of daily 50μL doses of an 8.5μM dilution of PG 99-465. This was to ensure proper administration of a 0.1mg/1kg dose in a volume high enough to flood the murine lungs and generate a localized effect. The preparation of the 8.5 μM dilution of PG 99-465 started from the 0.5mg of compound in the commercial format and adding 1x PBS until reaching the desired concentration. The mice were treated until day 14 post-infection, then the bacterial burden was enumerated. Another group of mice were sacrificed at day 7 post-infection for hematoxylin and eosin (H&E) staining to assess inflammation in the lungs.
Statistical analysis
All results were graphed in GraphPrism v9.5.0 and statistical significance was calculated using the Sidaks two-way ANOVA multiple comparison test for all animal experiments. All experiments were performed in at least two or more independent biological replicates. The exact number of mice and technical replicates is indicated in each figure legend. A p-value <0.05 was considered statistically significant. In the figures the asterisks correspond with *p ≤ 0.05, ** p ≤ 0.01, ***p ≤ 0.001, and ****p ≤ 0.0001. Statistical significance is shown only in the relevant figures.
Results
VIP does not affect Bordetella spp growth rate
Previous literature has shown that the Vasoactive Intestinal Peptide (VIP) has an antimicrobial effect on some bacterial species such as Escherichia coli, Pseudomonas aeruginosa and Streptococcus mutans (El Karim et al., 2008; Carion et al., 2015). Therefore, we first evaluated the antimicrobial activity of VIP against all three classical Bordetella species.
To assess the bactericidal or bacteriostatic effect of VIP we performed our studies using a physiologically relevant media, DMEM with 10% FBS or artificial sputum media, supplemented with increasing concentrations of VIP. Our lowest concentration corresponds with the reported concentration of VIP in serum, 25 nM (Ghaferi et al., 2008), and our highest is greater than the reported concentration of VIP in brain, 100nM (Goadsby and Edvinsson, 1994) or previously tested for antimicrobial activity of VIP (Carion et al., 2015). The results show no significant differences between the duplication times of B. pertussis, B. parapertussis or B. bronchiseptica, for any of the concentrations evaluated in our studies (Figure 1). We were not able to identify defect in growth or bacterial killing in DMEM or sputum. Thus, we concluded that VIP does not possess bactericidal nor bacteriostatic activity against the three classical Bordetella spp. at physiologically relevant conditions.
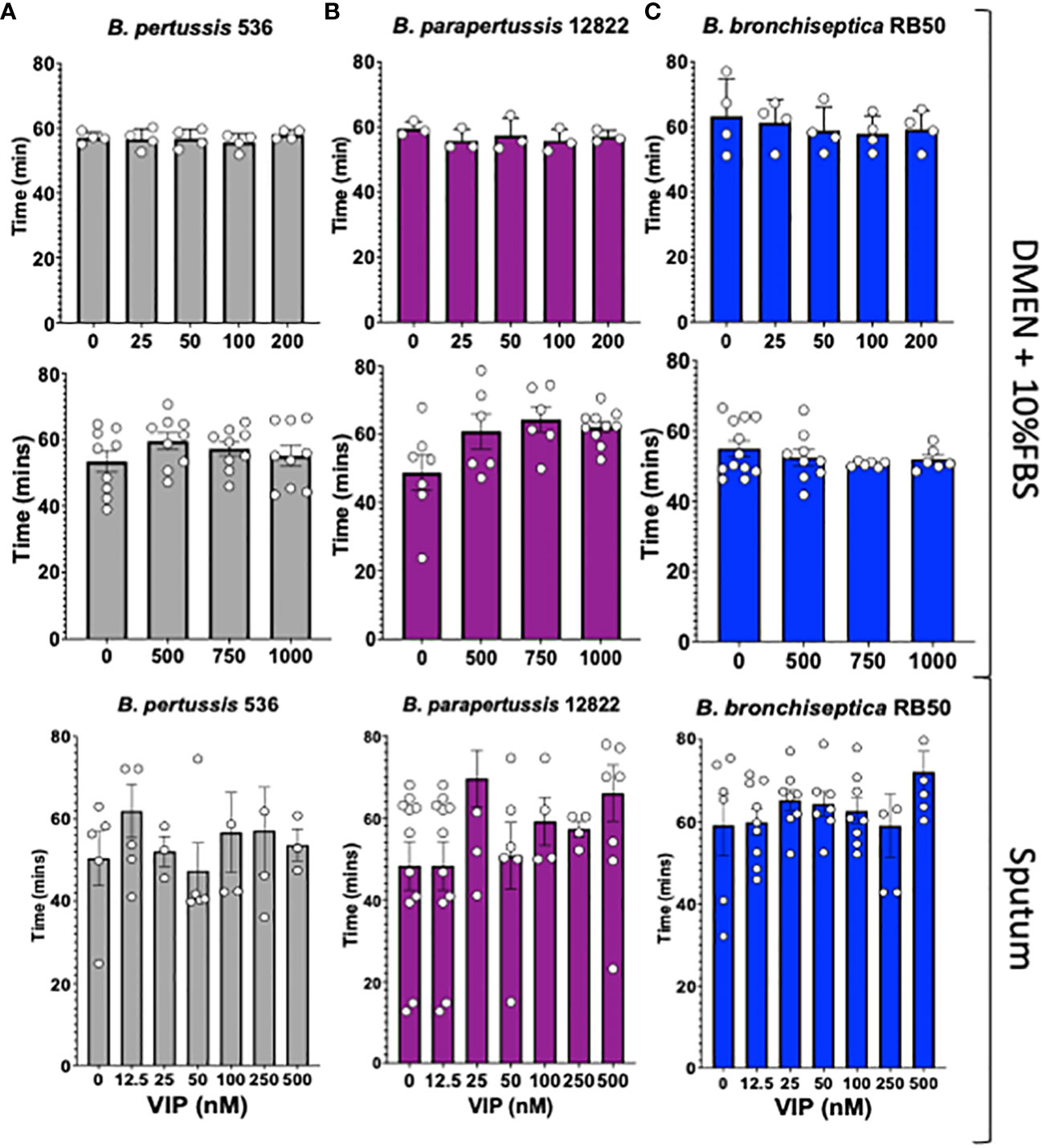
Figure 1 VIP does not kill nor affect the growth of classical Bordetella spp. In vitro cultures of B. pertussis strain 536 in grey (A), B. parapertussis strain 12822 in purple (B), and B. bronchiseptica strain RB50 in blue (C) were inoculated on a 96-well plate using DMEM supplemented with 10% FBS, or synthetic sputum media, containing a series of increasing VIP concentrations (between 0 to 1000nM). The plate was incubated in a Biotek Synergy H1® for 12 hours to monitor bacterial growth by optical density (OD600). Each symbol shows the mean of a single biological replicate containing 3 technical replicates. Duplication time was calculated for each case during the exponential phase. Two-way ANOVA analysis was performed to evaluate statistical differences. N = 3-10, with 3 technical replicates for each assay.
Previous incubation with VIP has no effect in lung colonization for the three classical Bordetella
We wanted to investigate if prior incubation with VIP would prime Bordetella spp. to better respond to host cues and enhance the bacteria’s ability to colonize the respiratory tract, similar to what has been shown with other host components. To test this hypothesis, overnight cultures of the three classical Bordetella spp. in DMEM supplemented with 10% FBS with or without 200 nM of VIP (as indicated in the figure legend), were used to intranasally inoculate mice. At day 3 post-infection, mice were euthanized to enumerate colonies in the respiratory tract to assess for the ability of the bacteria to colonize the lungs.
The results show no significant differences in bacterial ability to colonize the respiratory tract. It is worth noting that there are limitations to infecting mice with B. pertussis and B. parapertussis. Because the bacteria were inoculated intranasally, it is possible that the inoculums were not fully inhaled due to bubbles or leaks from the nose, leading to inconsistent lung colonization. In addition, colony growth can be unpredictable since both bacteria are not natural pathogens of mice. The number of B. pertussis (Figure 2A), B. parapertussis (Figure 2B), and B. bronchiseptica (Figure 2C) that was isolated from the lungs of mice at day 3 post-infection was not affected by previous incubation with VIP. Similar results were previously observed with pre-incubation with blood and serum where despite increased in vitro cytotoxicity was observed after incubation with serum, no differences in the bacterial ability to colonize mice were detected (Gestal et al., 2018). Thus, we concluded that VIP does not possess any kind of activity that enhances the colonization properties of any of the three classical Bordetella species.
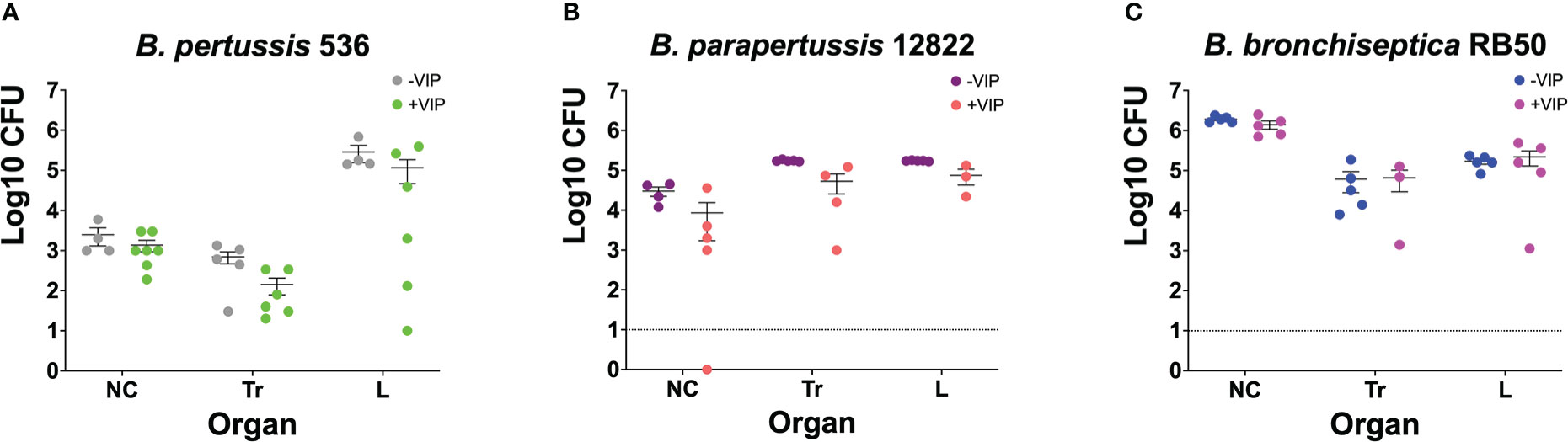
Figure 2 Pre-incubation with VIP does not promote bacterial colonization. Overnight cultures of B. pertussis BP536 (A), B. parapertussis 12822 (B), and B. bronchiseptica RB50 (C), were done in DMEM supplemented with 10% FBS. The overnight cultures were treated with or without 200nM of VIP, as indicated in the legends of each figure. After 24 hours, these cultures were used to intranasally inoculate mice with 30μl of PBS containing 5x105 CFUs of bacteria. At day 3 post-challenge, CFUs were enumerated by counting colonies in BGS agar from the nasal cavity (NC), trachea (Tr), and lungs (L). Two-way ANOVA analysis was performed to evaluate statistical differences. Data was comprised of two independent experiments. The bars represent the mean ± SD, with each data point representing one mouse (n = 4-7 mice per condition).
Functional VIP/VPAC2 axis promotes Bordetella spp. colonization
Previous literature has shown that VPAC2 and VPAC1 vary levels of expression during infection with different pathogens (Ipp et al., 2014; Askar et al., 2020; Yu et al., 2021). Moreover, pathogens such as Salmonella spp. utilize VIP/VPAC axis to promote intracellular survival in macrophages (Askar et al., 2020), suggesting that VIP/VPAC axis might influence infection dynamics and persistence. C57BL/6J and VPAC2-/- mice were intranasally inoculated with increasing bacterial concentrations of B. pertussis, B. parapertussis, and B. bronchiseptica, and euthanized at day 7 post-infection to enumerate colonies from the respiratory tract (Belhart et al., 2019).
Our results show that the ability of B. pertussis to colonize in the lungs of the VPAC2-/- mice was substantially depleted (Figure 3A), as a 10-fold increase of bacterial inoculum was required to establish an efficient lung infection. Similarly, B. parapertussis colonized C57BL/6J (Weyrich et al., 2012) at a lower infectious dose than the VPAC2-/- mice (Figure 3B). Finally, very low doses of B. bronchiseptica RB50 were required to colonize the C57BL/6J lungs efficiently (Weyrich et al., 2012; Belhart et al., 2019; Gestal et al., 2019) (Figure 3C). However, at least 1 x 104 CFUs of RB50 were required to colonize the lungs of VPAC2-/- mice. Overall, this reduced colonization can be the product of a stronger pro-inflammatory response of VPAC2-/- mice, or it can be due to the role of VIP in mucus secretion, which can affect colonization as previously reported (Goetzl et al., 2001; Kim et al., 2006; Samarasinghe et al., 2010; Wu et al., 2011). Nevertheless, our results altogether suggest that the absence of a functional VIP/VPAC2 signaling impairs classical Bordetella spp. colonization.
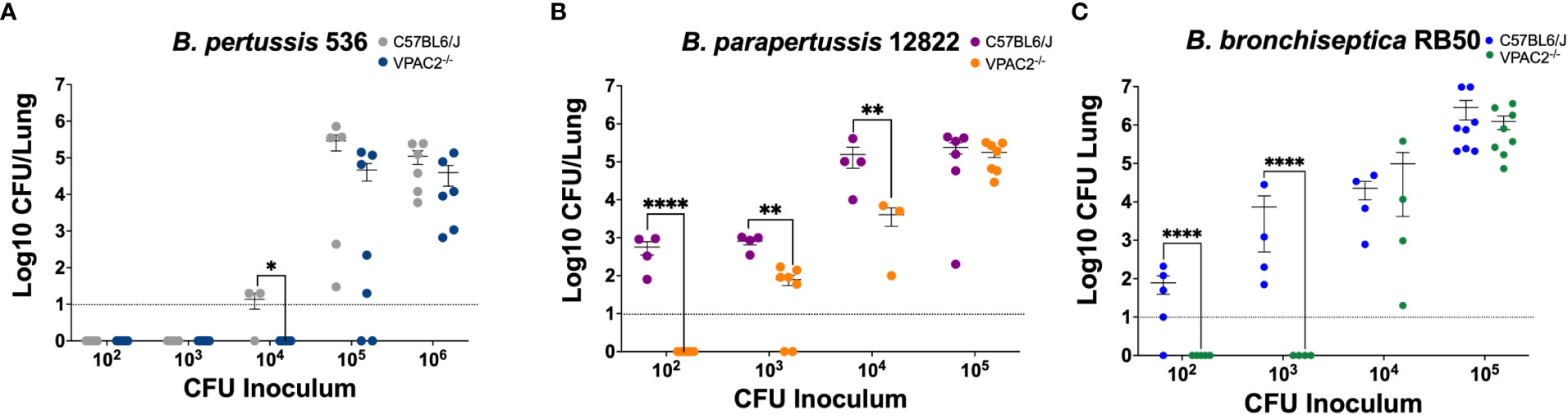
Figure 3 Functional VIP/VPAC2 axis promotes lung colonization by Bordetella spp. C57BL/6J and VPAC2-/- mice were intranasally challenged with increasing bacterial inoculums (x-axis) of B. pertussis BP536 (A), B. parapertussis 12822 (B), and B. bronchiseptica RB50 (C), each mouse strain indicated in the figure legends. At day 7 post-infection, we enumerated colonies from the lungs to evaluate colonization. Inoculations were done in parallel in the two different mice strains, and critical dosages were repeated in at least two independent experiments. Two-way ANOVA analysis was performed to evaluate statistical differences. The bars represent the mean ± SD, with each data point representing one mouse (n = 4-8 mice per condition). *p ≤ 0.05, **p ≤ 0.01, ****p < 0.0001.
Disruption of the VIP/VPAC2 axis mediates clearance of classical Bordetella spp. from the lower respiratory tract
One critical aspect of Bordetella spp. infection is the pneumonic phase that in patients lead to serius illness or even death (Rath et al., 2008; Barger-Kamate et al., 2016; Jiang et al., 2021). Identifying immunotherapies that prevent long term lung damage is of great importance. As VIP is commonly found in lungs, and we saw a defect in VPAC2-/- mice colonization, we wanted to investigate if VIP/VPAC2 signaling plays a role during Bordetella clearance from the lungs. To test this hypothesis, VPAC2-/- or C57BL/6J mice were challenged with B. pertussis, B. parapertussis, or B. bronchiseptica then sacrificed at different time points to evaluate the bacterial burden in the respiratory tract.
Following infection with the B. pertussis strain Bp536, we first analyzed the colonization in the nasal cavity (Figure 4C). Differences were not detectable up to day 28 post-infection. Similarly, the trachea did not reveal any major differences in bacterial load (Figure 4B). In the lungs, however, bacterial enumeration in the VPAC2-/- lungs dropped slightly at day 14 post-infection, with differences in the bacterial burden between VPAC2-/- and C57BL/6J becoming more distinct as early as day 20 (Figure 4A). Thus, our results suggest that disruption of VIP/VPAC2 signaling axis promotes clearance the infection from the lungs.
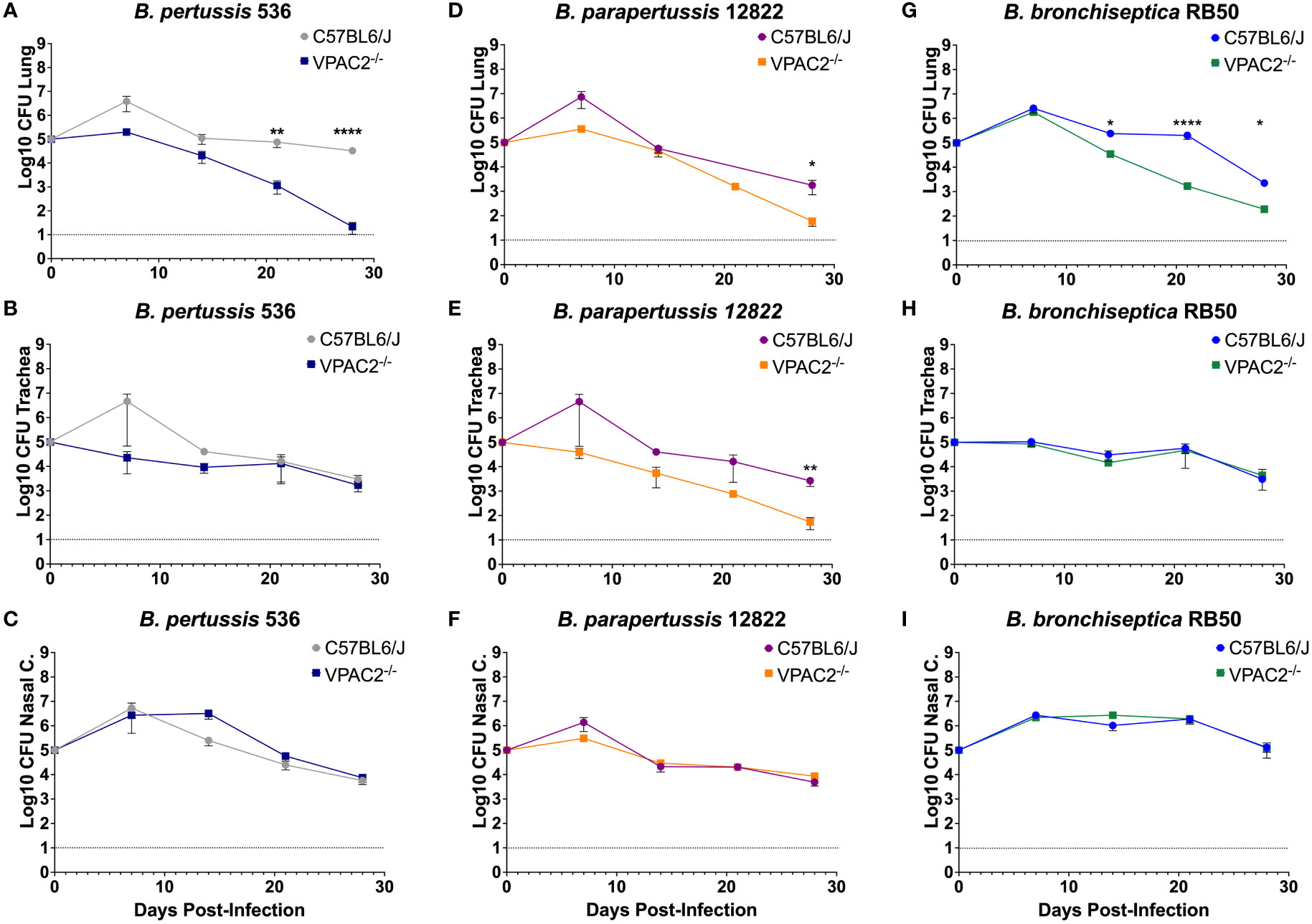
Figure 4 Functional VIP/VPAC2 axis promotes long-term persistent infection in the lungs. C57BL/6J and VPAC2-/- mice, were intranasally challenged with different concentrations of B. pertussis BP536 (A-C), B. parapertussis 12822 (D–F), and B. bronchiseptica RB50 (G–I), with mice strains indicated in each figure legend. At day 7 post-infection, we enumerated colonies from the nasal cavity (A, D, G), trachea (B, E, H), and lungs (C, F, I). Inoculations were done in parallel in the two different mice strains and critical dosages were repeated at least in three independent experiments. Each point represents mean ± SEM (n = 5-20 mice per condition). Two-way ANOVA analysis was performed to evaluate statistical differences. *p ≤ 0.05, **p ≤ 0.01, ****p < 0.0001.
During Bpp infection, no differences were detected in the colonization of the nasal cavity (Figure 4F). However, in the trachea and lungs (Figures 4D–F, respectively) we were able to detect a more rapid decrease in bacterial burden at day 28 post-infection, suggesting that, just like B. pertussis, B. parapertussis infection is more rapidly cleared from the lower respiratory tract of VPAC2-/- mice.
Our results demonstrate that the absence of a functional VIP/VPAC2 axis promotes rapid clearance of B. pertussis and B. parapertussis from the lower respiratory tract. However, these two bacteria are not natural pathogens of mice and cause only an acute disease that is efficiently cleared by the immune system rather than the characteristic long-term and persistent infection of the human pertussis disease (Soumana et al., 2021). To investigate the effects of disrupting VIP/VPAC2 signaling in bacterial clearance in a natural setting of long-term chronic disease, we used the well-established B. bronchiseptica murine model (Gestal et al., 2019) following the same experimental setting as previously indicated.
Our results did not reveal significant differences in the bacterial burden detected in the nasal cavity (Figure 4I) and trachea (Figure 4H) between the C57BL/6J and VPAC2-/- mice. In the lungs, we observed a clear decrease in the bacterial burden by day 14 post-infection (Figure 4G). Overall, these results indicate that the VIP/VPAC2 signaling pathway significantly impacts the clearance of B. pertussis, B. parapertussis, and B. bronchiseptica from the lungs. Because of these observed similarities, the B. bronchiseptica mouse model can be used for a better understanding of host-pathogen interactions at the molecular level, as the differences found became clearer when using a natural pathogen of mice.
VIP/VPAC2 axis mediated clearance of Bordetella bronchiseptica is modulated by the type 3 secretion system
Our previous results revealed that using B. bronchiseptica in this study could provide a more mechanistic understanding of the role of VIP/VPAC2 signaling in the infection dynamics. We previously investigated the mechanisms by which Bordetella spp. suppress host immune responses, and we described a Bordetella sigma factor, btrS, a regulator of immunosuppressive pathways in all three of the classical Bordetella species (Gestal et al., 2019). Absence of btrS results in rapid clearance and robust immune responses, with the generation of long-lasting protective immunity (Gestal et al., 2022). We wanted to investigate if the bacterial btrS-signaling pathway is involved in the modulation of VIP/VPAC2 signaling. To determine the effects of btrS on the manipulation of VIP/VPAC2 signaling, we evaluated the differences between murine bacterial infection by using the calculated ID50 values following inoculation with the wildtype BbRB50 strain, a mutant RB50 strain lacking the sigma factor btrS (RB50ΔbtrS). The ID50 values were assessed in these experiments because we predicted that if VIP/VPAC2 axis is modulated by btrS, there would be no observable difference in the ID50 of RB50ΔbtrS in either C57BL6/J or VPAC2-/- mice. However, if btrS is not involved in the modulation of VIP/VPAC2, then a reduction in the capability of colonizing VPAC2-/- mice would be observed, as was previously with the three Bordetella spp. strains. While differences in the ability to colonize VPAC2-/- were observed when investigating the RB50 strain (Figure 5A). Our results did not reveal differences in the ID50 of the RB50ΔbtrS, suggesting that in the absence of btrS there are no alterations in the bacterial ability to colonize neither C57BL6/J nor VPAC2-/- mice (Figure 5B), contrary to the wildtype B. bronchiseptica strain which fails to colonize in VPAC2-/- mice.
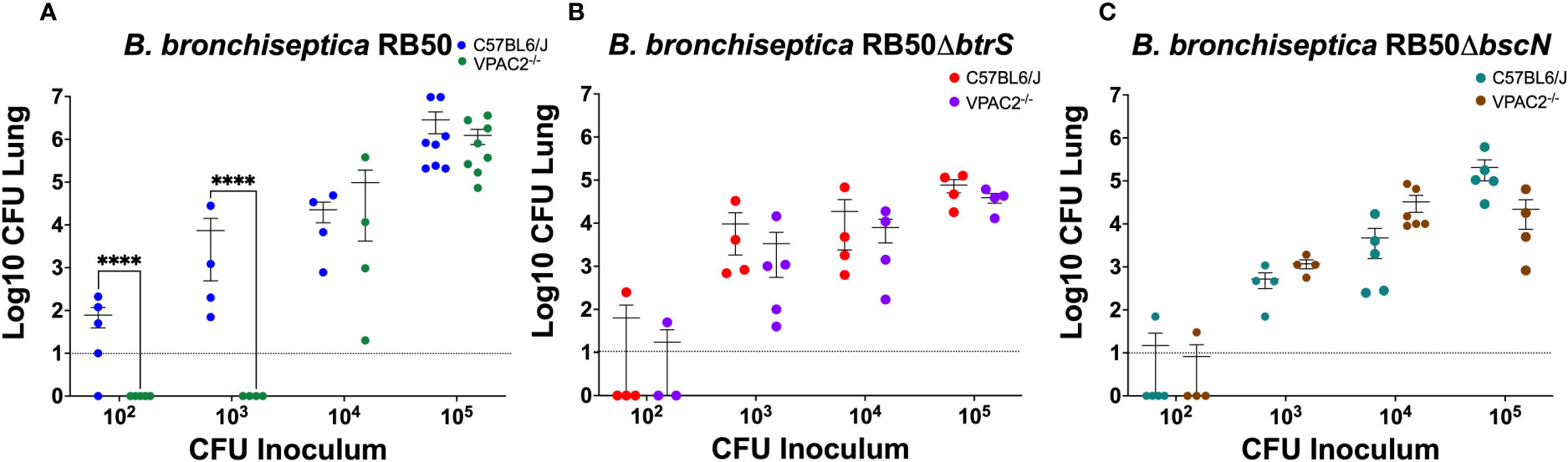
Figure 5 B. bronchiseptica utilize the T3SS to manipulate the host VIP/VAPC2 axis. C57BL/6J and VPAC2-/- mice, were intranasally challenged in parallel with different concentrations of B. bronchiseptica wildtype RB50 (A), the btrS-null mutant RB50ΔbtrS (B), or the T3SS knockout mutant RB50ΔbscN (C), with each mouse strain indicated in each figure legend. At day 7 post-infection, we enumerated colonies from the lungs to evaluate colonization levels. Inoculations were done in parallel with independent experiment performed at least 2 times. Each point represents one mouse, with each bar representing mean ± SD (n = 4-8 mice per condition). Two-way ANOVA analysis was performed to evaluate statistical differences. ****p ≤ 0.0001.
One of the main virulence factors regulated by the btrS-pathway is the type 3 secretion system (T3SS). Using the same experimental setting as previously described, we wanted to evaluate the role of the T3SS in the modulation of VIP/VPAC2 signaling, we again performed an ID50 study. We expected that if manipulation of VIP/VPAC2 signaling is mediated by the T3SS, we will not be able to observe differences in the ID50 between C57BL/6J and VPAC2-/- mice (Figure 5C). Our results did not show difference between the ID50 in C57BL/6J mice and the VPAC2-/- mice with the T3SS mutant of B. bronchiseptica, suggesting that, the modulation of the VIP/VPAC2 axis by Bordetella spp. might be mediated by T3SS. Although VIP/VPAC2 axis is also involved in the modulation between Th1/Th2 responses, the fact that no differences were found in the ID50 of the RB50ΔbscN while differences were found with all the wildtype bacteria, led us to hypothesized that T3SS might mediate Bordetella spp. manipulation of VIP/VPAC2 axis to promote colonization.
The administration of a VPAC2 receptor antagonist mediates clearance of Bordetella bronchispetica in the lungs
Our previous results show that blocking VIP/VPAC2 axis promotes rapid clearance of the lower respiratory tract, suggesting that, the use of an antagonist of the VPAC2 receptor should have a similar effect in clearance to that observed in the VPAC2-/- mutant mice. To evaluate if antagonists of VPAC2 promote clearance, we used the substance PG 99-465 as a treatment for C57BL/6J mice infected with B. bronchiseptica. The mice were treated every 24 hours following inoculation with a 50μL dose of PG 99-465 (8.5μM). At 7 and 14 days post-infection, the mice were euthanized to evaluate lung pathology and colonization respectively.
Although no differences in lung colonization were observed (Figure 6A), this could be the consequence of the short half-life of the antagonist, which is a very small and unstable peptide. However, the pathology results show a clear difference in inflammation of the bronchia and consolidation of the respiratory parenchyma between the untreated and treated mice (Figure 6B). The pathology in mice treated with the antagonist resembled the pathology observed in VPAC2-/- mice, which successfully recover from infection. Overall, this suggest that VPAC2 receptor could be targeted for novel immune therapies against Bordetella spp. infections.
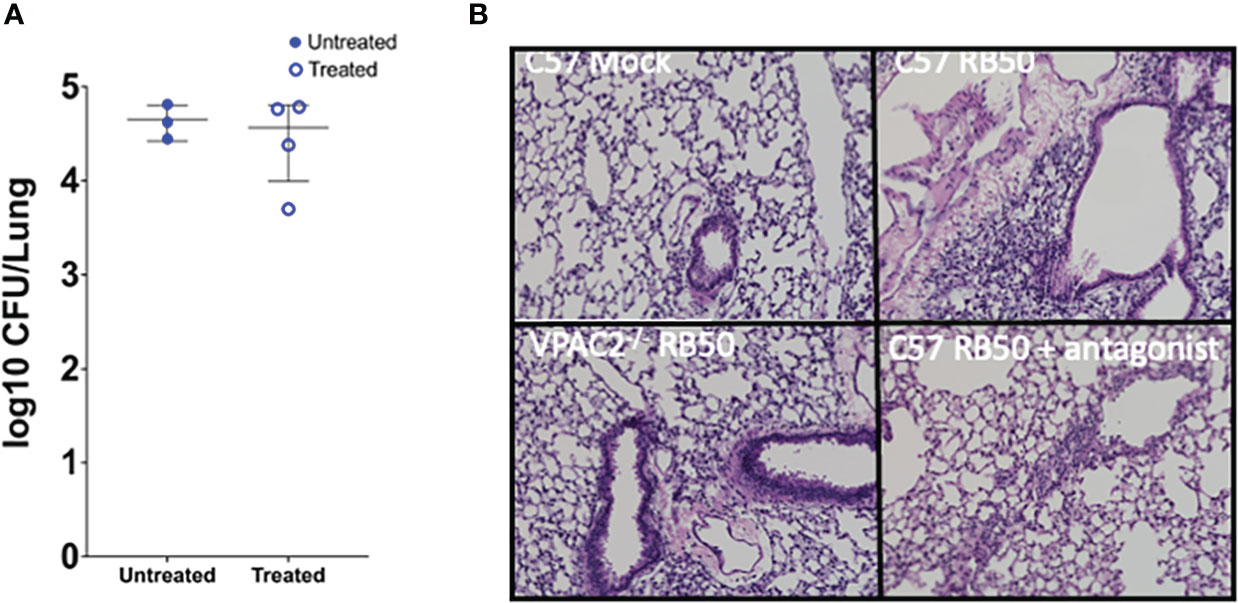
Figure 6 Treatment with VPAC2 antagonist reduce lung pathology. (A) C57BL/6J mice were intranasally challenged with B. bronchiseptica RB50. At day 1 post-infection, we started a treatment with the VPAC2 antagonist daily. Colonies were enumerated from the lungs at day 14, with solid blue points representing untreated mice, with the hollow blue points being the treated animals. Each point represents one mouse, with each bar representing mean ± SD (A has an n=3-4 animals per condition, B has n=4-8 animals per condition). Two-way ANOVA analysis was performed to evaluate statistical differences. (B) H&E pathology staining was done at day 7 post-infection with a minimum of 4 animals per group. The above images are representative of each experimental condition.
Discussion
Bacteria-host crosstalk is an evolutionary pressure for bacterial pathogens that rely on their ability to detect and respond to the stresses of the host environment, and it is one of the driving forces that dictate disease outcome (El Karim et al., 2008; Gestal et al., 2019). It has been shown that some host molecules including VIP have bactericidal effects (El Karim et al., 2008). However, our results did not reveal any bactericidal effect for VIP against Bordetella spp. when testing at higher concentrations than surpass the concentrations that are physiologically relevant (Carion et al., 2015). Our results indicated that incubation with VIP had no effects in bacterial growth within the host or bacterial infectivity.
Neuropeptides are known to profoundly impact host biology as both enhancers of the host immune response and as antimicrobial agents themselves (Wei et al., 2020). It has been shown that other pathogens such as Clostridium botulinum, can manipulate GABA receptors to also promote colonization and persistence (Höltje et al., 2009). One important function of VIP/VPAC2 signaling is maintaining homeostasis between Th1 and Th2 responses and regulate mucus production which can be critical during the infectious process (Goetzl et al., 2001; Samarasinghe et al., 2010). Thus, it would make sense that Bordetella spp. and other bacterial pathogens have evolved means to manipulate this axis to promote their survival at mucosal sites. In the respiratory tract, VIP triggers bronchial dilation of lung airways which can provide a target for bacteria to facilitate colonization (Groneberg et al., 2001; Lindén et al., 2003). In this work, we wanted to investigate if blocking this signaling cascade would promote clearance of Bordetella spp. First, we observed that in the absence of a functional VIP/VPAC2 axis, the classical Bordetellae fail to colonize the lungs with low infection dosages and infection is more rapidly clear from the lower respiratory tract. Moreover, our results revealed that in the absence of a functional VPAC2-/- receptor classical Bordetella spp. failed to efficiently colonize the lungs and clear more rapidly the infection from the lungs, suggesting that Bordetella harbor mechanisms to manipulate VIP/VPAC2 signaling. We have not evaluated the role of VIP/VPAC2 in the generation of protective immunity or its impact in the Th1/Th17 and Th2 responses, though it is clear that it has an impact on the length of infection, which should be further investigated.
Moreover, we identified one of the possible bacterial mechanisms involved in the manipulation of this host signaling pathway. The sigma factor btrS, which regulates an immunosuppressive pathway, is involved in the manipulation of the host VIP/VPAC2 axis. In fact, we identify that the T3SS plays a role in the bacterial ability to manipulate VIP/VAPC2 in order to promote bacterial colonization in the lungs. It is known that the T3SS is involved in persistence and immunosuppression in Bordetella spp. as well as in other gram-negative bacteria. However, these findings provide a novel function for the T3SS, where bacteria manipulate host VIP/VAPC2 to promote colonization using the T3SS. Importantly, this also suggest that other gram-negative bacteria that harbor a functional T3SS might also have the capability to manipulate the VIP/VPAC2 signaling axis to promote colonization and persistence.
Overall, our results demonstrate that Bordetella spp., and based on the literature possibly other mucosal bacterial pathogens (Askar et al., 2020), may exploit the VIP/VPAC2 axis to promote colonization and long-term persistence.
Data availability statement
The original contributions presented in the study are included in the article/supplementary material. Further inquiries can be directed to the corresponding author.
Ethics statement
The protocols were reviewed and approved by "the Institutional Animal Care and Use Committee at Louisiana State University Health Science at Shreveport. Shreveport, LA, USA". The protocols P20-038 and P22-031 were filed by Dr. Gestal, Assistant Professor. Department of Microbiology and Immunology. Gratis Associate Professor. Department of Pediatrics. LSU Health science center at Shreveport. Animals were care for according to the National Institute of Health guidelines for the care and use of animals.
Author contributions
NF: Perform experiments (he took part in all of them), data analysis, conceptual design, and writing and editing; JP-L: Perform experiments, data analysis, and writing and editing; MRFS-S: Perform experiments, data analysis, and writing and editing; KMP performed experiments, data analysis, and significant re-writing and editing; X-HL: Provide tools, animals, and editing; MG: Perform experiments, design experiments, data analysis, original idea, conceptual design of the story, writing and editing, and funding. All authors contributed to the article and approved the submitted version.
Funding
This research was funded by NIH grant number P20-GM134974 for the COBRE entitled “Center for Applied Immunology and Pathological Processes”. The Center of Excellence for Arthritis and Rheumatology intramural award, CEAR Award. Louisiana Board of Regents LEQSF(2022-25)-RD-A-33. Intramural Research Council Seed package support from LSU Health, Shreveport and the Start-up package from LSU Health, Shreveport. The funding bodies had no role in the design, analysis, or interpretation of the data interest.
Acknowledgments
The authors would like to acknowledge that JP-L and MRFS-S are fellows supported by the Summer Undergraduate Research Fellowship Program of the Fundación Barrie de la Maza and LSU Health science center at Shreveport. We would like to acknowledge the Immunophenotyping core of the COBRE grant P20-GM134974-0181 at Louisiana State University health science center at Shreveport for their help with staining and brain storming. We would also like to acknowledge the histology core at Louisiana State University health science center at Shreveport, CCDS for their help with embedding and sectioning the tissues. The authors would also like to acknowledge the High-Throughput Screening – INLTE core at Louisiana State University health science center at Shreveport, for providing us access to their technologies, such as the Synergy, that allowed us to perform the antimicrobial studies.
Conflict of interest
The authors declare that the research was conducted in the absence of any commercial or financial relationships that could be construed as a potential conflict of interest.
Publisher’s note
All claims expressed in this article are solely those of the authors and do not necessarily represent those of their affiliated organizations, or those of the publisher, the editors and the reviewers. Any product that may be evaluated in this article, or claim that may be made by its manufacturer, is not guaranteed or endorsed by the publisher.
References
Andreasen, C., Carbonetti, N. H. (2008). Pertussis toxin inhibits early chemokine production to delay neutrophil recruitment in response to bordetella pertussis respiratory tract infection in mice. Infect. Immun. 76, 5139–5148. doi: 10.1128/IAI.00895-08
Armstrong, S. K., Brickman, T. J., Suhadolc, R. J. (2012). Involvement of multiple distinct bordetella receptor proteins in the utilization of iron liberated from transferrin by host catecholamine stress hormones. Mol. Microbiol. 84, 446–462. doi: 10.1111/j.1365-2958.2012.08032.x
Askar, B., Higgins, J., Barrow, P., Foster, N. (2020). Immunomodulation by vasoactive intestinal peptide is associated with increased survival and growth of salmonella typhimurium in mice. Cytokine 125, 154787. doi: 10.1016/j.cyto.2019.154787
Baraniuk, J. N., Lundgren, J. D., Okayama, M., Mullol, J., Merida, M., Shelhamer, J. H., et al. (1990). Vasoactive intestinal peptide in human nasal mucosa. J. Clin. Invest. 86, 825–831. doi: 10.1172/JCI114780
Barger-Kamate, B., Deloria Knoll, M., Wangeci Kagucia, E., Prosperi, C., Baggett, H. C., Abdullah Brooks, W. (2016). Pertussis-associated pneumonia in infants and children from low- and middle-income countries participating in the PERCH study. Clin. Infect. Dis. 63, S187–S196. doi: 10.1093/cid/ciw546
Belhart, K., de la Paz Gutierrez, M., Zacca, F., Ambrosis, N., Gestal, M. C., Taylor, D., et al. (2019). Bordetella bronchiseptica diguanylate cyclase BdcA regulates motility and is important for the establishment of respiratory infection in mice. J. Bacteriol. 201. doi: 10.1128/JB.00011-19
Boehm, D. T., Allison Wolf, M., Hall, J. M., Wong, T. Y., Sen-Kilic, E., Basinger, H. D., et al. (2019). Intranasal acellular pertussis vaccine provides mucosal immunity and protects mice from. NPJ Vaccines 4, 40. doi: 10.1038/s41541-019-0136-2
Brickman, T. J., Anderson, M. T., Armstrong, S. K. (2007). Bordetella iron transport and virulence. Biometals 20, 303–322. doi: 10.1007/s10534-006-9031-1
Campos-Salinas, J., Cavazzuti, A., O'Valle, F., Forte-Lago, I., Caro, M., Beverley, S. M., et al. (2014). Therapeutic efficacy of stable analogues of vasoactive intestinal peptide against pathogens. J. Biol. Chem. 289, 14583–14599. doi: 10.1074/jbc.M114.560573
Carion, T. W., McWhirter, C. R., Grewal, D. K., Berger, E. A. (2015). Efficacy of VIP as treatment for bacteria-induced keratitis against multiple pseudomonas aeruginosa strains. Invest. Ophthalmol. Vis. Sci. 56, 6932–6940. doi: 10.1167/iovs.15-17315
Decker, M. D., Edwards, K. M. (2021). Pertussis (Whooping cough). J. Infect. Dis. 224, S310–S320. doi: 10.1093/infdis/jiaa469
Dewan, K. K., Taylor-Mulneix, D. L., Hilburger, L. J., Rivera, I., Preston, A., Eric Harvill, T E.T. (2017). An extracellular polysaccharide locus required for transmission of bordetella bronchiseptica. J. Infect. Dis. 216, 899–906. doi: 10.1093/infdis/jix251
Dorji, D., Mooi, F., Yantorno, O., Deora, R., Graham, R. M., Mukku, T. K., et al. (2018). Bordetella pertussis virulence factors in the continuing evolution of whooping cough vaccines for improved performance. Med. Microbiol. Immunol. 207, 3–26. doi: 10.1007/s00430-017-0524-z
El Karim, I. A., Linden, G. J., Orr, D. F., Lundy, F. T. (2008). Antimicrobial activity of neuropeptides against a range of micro-organisms from skin, oral, respiratory and gastrointestinal tract sites. J. Neuroimmunol. 200, 11–16. doi: 10.1016/j.jneuroim.2008.05.014
Fedele, G., Schiavoni, I., Adkins, I., Klimova, N., Sebo, P. (2017). Invasion of dendritic cells, macrophages and neutrophils by the bordetella adenylate cyclase toxin: A subversive move to fool host immunity. Toxins (Basel) 9. doi: 10.3390/toxins9100293
Feng, Y., Cheng-Chiu, H., Heininger, U., Flavia Hozbor, D., Quanbee Tan, T., Wirsing von König, C.-H. (2021). Emerging macrolide resistance in. Lancet Reg. Health West Pac 8, 100098. doi: 10.1016/j.lanwpc.2021.100098
Gestal, M. C., Rivera, I., Howard, L. K., Dewan, K. K., Hamidou Soumana, I., Dedloff, M., et al. (2018). Blood or serum exposure induce global transcriptional changes, altered antigenic profile, and increased cytotoxicity by classical bordetellae. Front. Microbiol. 9, 1969. doi: 10.3389/fmicb.2018.01969
Gestal, M. C., Howard, L. K., Dewan, K., Johnson, H. M., Barbier, M., Bryant, C., et al. (2019). Enhancement of immune response against bordetella spp. by disrupting immunomodulation. Sci. Rep. 9, 20261. doi: 10.1038/s41598-019-56652-z
Gestal, M. C., Howard, L. K., Dewan, K. K., Harvill, E. T. (2022). Bbvac: A live vaccine candidate that provides long-lasting anamnestic and Th17-mediated immunity against the three classical. mSphere 7, e0089221. doi: 10.1128/msphere.00892-21
Gestal, M. C., Whitesides, L. T., Harvill, E. T. (2019). Integrated signaling pathways mediate bordetella immunomodulation, persistence, and transmission. Trends Microbiol. 27, 118–130. doi: 10.1016/j.tim.2018.09.010
Ghaferi, A. A., Chojnacki, K. A., Long, W. D., Cameron, J. L., Yeo, C. J. (2008). Pancreatic VIPomas: Subject review and one institutional experience. J. Gastrointest Surg. 12, 382–393. doi: 10.1007/s11605-007-0177-0
Goadsby, P. J., Edvinsson, L. (1994). Human in vivo evidence for trigeminovascular activation in cluster headache. Neuropeptide changes and effects of acute attacks therapies. Brain 117 (Pt 3), 427–434. doi: 10.1093/brain/117.3.427
Goetzl, E. J., Voice, J. K., Shen, S., Harmar, A. J. (2001). Enhanced delayed-type hypersensitivity and diminished immediate-type hypersensitivity in mice lacking the inducible VPAC(2) receptor for vasoactive intestinal peptide. Proc. Natl. Acad. Sci. U.S.A. 98, 13854–13859. doi: 10.1073/pnas.241503798
Groneberg, D. A., Springer, J., Fischer, A. (2001). Vasoactive intestinal polypeptide as mediator of asthma. Pulm. Pharmacol. Ther. 14, 391–401. doi: 10.1006/pupt.2001.0306
Henning, R. J., Sawmiller, D. R. (2001). Vasoactive intestinal peptide: Cardiovascular effects. Cardiovasc. Res. 49, 27–37. doi: 10.1016/S0008-6363(00)00229-7
Hester, S. E., Lui, M., Nicholson, T., Nowacki, D., Harvill, E. T. (2012). Identification of a CO2 responsive regulon in bordetella. PloS One 7, e47635. doi: 10.1371/journal.pone.0047635
Höltje, M., Djalali, S., Hofmann, F., Münster-Wandowski, A., Hendrix, S., Boato, F., et al. (2009). A 29-amino acid fragment of clostridium botulinum C3 protein enhances neuronal outgrowth, connectivity, and reinnervation. FASEB J. 23, 1115–1126. doi: 10.1096/fj.08-116855
Ipp, H., Nkambule, B. B., Reid, T. D., de Swardt, D., Bekker, L.-G., Glashoff, R. H. (2014). CD4+ T cells in HIV infection show increased levels of expression of a receptor for vasoactive intestinal peptide, VPAC2. Immunol. Res. 60, 11–15. doi: 10.1007/s12026-014-8487-2
Jiang, W., Wu, M., Chen, S., Li, A., Wang, K., Wang, Y., et al. (2021). Virus coinfection is a predictor of radiologically confirmed pneumonia in children with bordetella pertussis infection. Infect. Dis. Ther. 10, 335–346. doi: 10.1007/s40121-020-00376-5
Jimeno, R., Leceta, J., Martínez, C., Gutiérrez-Cañas, I., Pérez-García, S., Carrión, M., et al. (2012). Effect of VIP on the balance between cytokines and master regulators of activated helper T cells. Immunol. Cell Biol. 90, 178–186. doi: 10.1038/icb.2011.23
Kilgore, P. E., Salim, A. M., Zervos, M. J., Schmitt, H. J. (2016). Pertussis: Microbiology, disease, treatment, and prevention. Clin. Microbiol. Rev. 29, 449–486. doi: 10.1128/CMR.00083-15
Kim, J. S., Okamoto, K., Arima, S., Rubin, B. K. (2006). Vasoactive intestinal peptide stimulates mucus secretion, but nitric oxide has no effect on mucus secretion in the ferret trachea. J. Appl. Physiol. (1985) 101, 486–491. doi: 10.1152/japplphysiol.01264.2005
Leceta, J., Garin, M. I., Conde, C. (2021). Mechanism of immunoregulatory properties of vasoactive intestinal peptide in the K/BxN mice model of autoimmune arthritis. Front. Immunol. 12, 701862. doi: 10.3389/fimmu.2021.701862
Lindén, A., Hansson, L., Andersson, A., Palmqvist, M., Arvidsson, P., Löfdahl, C.-G., et al. (2003). Bronchodilation by an inhaled VPAC(2) receptor agonist in patients with stable asthma. Thorax 58, 217–221. doi: 10.1136/thorax.58.3.217
Linz, B., Ivanov, Y. V., Preston, A., Brinkac, L., Parkhill, J., Kim, M., et al. (2016). Acquisition and loss of virulence-associated factors during genome evolution and speciation in three clades of bordetella species. BMC Genomics 17, 767. doi: 10.1186/s12864-016-3112-5
Mattoo, S., Cherry, J. D. (2005). Molecular pathogenesis, epidemiology, and clinical manifestations of respiratory infections due to bordetella pertussis and other bordetella subspecies. Clin. Microbiol. Rev. 18, 326–382. doi: 10.1128/CMR.18.2.326-382.2005
Mikelova, L. K., Halperin, S. A., Scheifele, D., Smith, B., Ford-Jones, E., Vaudry, W., et al. (2003). Predictors of death in infants hospitalized with pertussis: A case-control study of 16 pertussis deaths in Canada. J. Pediatr. 143, 576–581. doi: 10.1067/S0022-3476(03)00365-2
Mills, K. H., Gerdts, V. (2014). Mouse and pig models for studies of natural and vaccine-induced immunity to bordetella pertussis. J. Infect. Dis. 209 Suppl 1, S16–S19. doi: 10.1093/infdis/jit488
Nicholson, T. L., Brockmeier, S. L., Loving, C. L., Register, K. B., Kehrli, M. E., Jr, Shore, S. M. (2014). The bordetella bronchiseptica type III secretion system is required for persistence and disease severity but not transmission in swine. Infect. Immun. 82, 1092–1103. doi: 10.1128/IAI.01115-13
Paccani, S. R., Dal Molin, F., Benagiano, M., Ladant, D., D'Elios, M. M., Montecucco, C., et al. (2008). Suppression of T-lymphocyte activation and chemotaxis by the adenylate cyclase toxin of bordetella pertussis. Infect. Immun. 76, 2822–2832. doi: 10.1128/IAI.00200-08
Park, J., Zhang, Y., Buboltz, A. M., Zhang, X., Schuster, S. C., Ahuja, U., et al. (2012). Comparative genomics of the classical bordetella subspecies: The evolution and exchange of virulence-associated diversity amongst closely related pathogens. BMC Genomics 13, 545. doi: 10.1186/1471-2164-13-545
Parkhill, J., Sebaihia, M., Preston, A., Murphy, L. D., Thomson, N., Harris, D. E., et al. (2003). Comparative analysis of the genome sequences of bordetella pertussis, bordetella parapertussis and bordetella bronchiseptica. Nat. Genet. 35, 32–40. doi: 10.1038/ng1227
Pope, C. F., McHugh, T. D., Gillespie, S. H. (2010). Methods to determine fitness in bacteria. Methods Mol. Biol. 642, 113–121. doi: 10.1007/978-1-60327-279-7_9
Pozo, D., Delgado, M. (2004). The many faces of VIP in neuroimmunology: A cytokine rather a neuropeptide? FASEB J. 18, 1325–1334. doi: 10.1096/fj.03-1440hyp
Rath, B. A., Register, K. B., Wall, J., Sokol, D. M., Van Dyke, R. B. (2008). Persistent bordetella bronchiseptica pneumonia in an immunocompetent infant and genetic comparison of clinical isolates with kennel cough vaccine strains. Clin. Infect. Dis. 46, 905–908. doi: 10.1086/528858
Rougier, S., Galland, D., Boucher, S., Boussarie, D., Vallé, M. (2006). Epidemiology and susceptibility of pathogenic bacteria responsible for upper respiratory tract infections in pet rabbits. Vet. Microbiol. 115, 192–198. doi: 10.1016/j.vetmic.2006.02.003
Said, S. I. (1986). Vasoactive intestinal peptide. J. Endocrinol. Invest. 9, 191–200. doi: 10.1007/BF03348097
Samarasinghe, A. E., Hoselton, S. A., Schuh, J. M. (2010). The absence of the VPAC(2) receptor does not protect mice from aspergillus induced allergic asthma. Peptides 31, 1068–1075. doi: 10.1016/j.peptides.2010.03.001
Scanlon, K. M., Chen, L., Carbonetti, N. H. (2022). Pertussis toxin promotes pulmonary hypertension in an infant mouse model of bordetella pertussis infection. J. Infect. Dis. 225, 172–176. doi: 10.1093/infdis/jiab325
Sharma, V., Delgado, M., Ganea, D. (2006). VIP Protects Th2 cells by downregulating granzyme b expression. Ann. N. Y. Acad. Sci. 1070, 540–544. doi: 10.1196/annals.1317.077
Shi, T., Wang, L., Du, S., Fan, H., Yu, M., Ding, T., et al. (2021). Mortality risk factors among hospitalized children with severe pertussis. BMC Infect. Dis. 21, 1057. doi: 10.1186/s12879-021-06732-1
Skerry, C., Scanlon, K., Ardanuy, J., Roberts, D., Zhang, L., Rosen, H., et al. (2017). Reduction of pertussis inflammatory pathology by therapeutic treatment with sphingosine-1-Phosphate receptor ligands by a pertussis toxin-insensitive mechanism. J. Infect. Dis. 215, 278–286. doi: 10.1093/infdis/jiw536
Skerry, C., Scanlon, K., Rosen, H., Carbonetti, N. H. (2015). Sphingosine-1-phosphate receptor agonism reduces bordetella pertussis-mediated lung pathology. J. Infect. Dis. 211, 1883–1886. doi: 10.1093/infdis/jiu823
Smallridge, W. E., Rolin, O. Y., Jacobs, N. T., Harvill, E. T. (2014). Different effects of whole-cell and acellular vaccines on bordetella transmission. J. Infect. Dis. 209, 1981–1988. doi: 10.1093/infdis/jiu030
Soumana, I. H., Linz, B., Dewan, K. K., Sarr, D., Gestal, M. C., Howard, L. K., et al. (2021). Modeling immune evasion and vaccine limitations by targeted nasopharyngeal bordetella pertussis inoculation in mice. Emerg. Infect. Dis. 27, 2107–2116. doi: 10.3201/eid2708.203566
Sukumar, N., Love, C. F., Conover, M. S., Kock, N. D., Dubey, P., Deora, R., et al. (2009). Active and passive immunizations with bordetella colonization factor a protect mice against respiratory challenge with bordetella bronchiseptica. Infect. Immun. 77, 885–895. doi: 10.1128/IAI.01076-08
van Beek, L. F., de Gouw, D., Eleveld, M. J., Bootsma, H. J., de Jonge, M. I., Mooi, F. R., et al. (2018). Adaptation of bordetella pertussis to the respiratory tract. J. Infect. Dis. 217, 1987–1996. doi: 10.1093/infdis/jiy125
Wei, P., Keller, C., Li, L. (2020). Neuropeptides in gut-brain axis and their influence on host immunity and stress. Comput. Struct. Biotechnol. J. 18, 843–851. doi: 10.1016/j.csbj.2020.02.018
Weyrich, L. S., Rolin, O. Y., Muse, S. J., Park, J., Spidale, N., Kennett, M. J., et al. (2012). A type VI secretion system encoding locus is required for bordetella bronchiseptica immunomodulation and persistence in vivo. PloS One 7, e45892. doi: 10.1371/journal.pone.0045892
Weyrich, L. S., Feaga, H. A., Park, J., Muse, S. J., Safi, C. Y., et al. (2014). Resident microbiota affect bordetella pertussis infectious dose and host specificity. J. Infect. Dis. 209, 913–921. doi: 10.1093/infdis/jit597
Wolf, M. A., Boehm, D. T., DeJong, M. A., Wong, T. Y., Sen-Kilic, E., Hall, J. M., et al. (2021). Intranasal immunization with acellular pertussis vaccines results in long-term immunity to bordetella pertussis in mice. Infect. Immun. 89. doi: 10.1128/IAI.00607-20
Wu, X., Du, Q., Li, D., Yuan, L., Meng, Q., Fu, Z., et al. (2022). A cross-sectional study revealing the emergence of erythromycin-resistant. Front. Microbiol. 13, 901617. doi: 10.3389/fmicb.2022.901617
Wu, D., Lee, D., Sung, Y. K. (2011). Prospect of vasoactive intestinal peptide therapy for COPD/PAH and asthma: A review. Respir. Res. 12, 45. doi: 10.1186/1465-9921-12-45
Yeung, K. H. T., Duclos, P., Nelson, E. A. S., Hutubessy, R. C. W. (2017). An update of the global burden of pertussis in children younger than 5 years: A modelling study. Lancet Infect. Dis. 17, 974–980. doi: 10.1016/S1473-3099(17)30390-0
Yu, H. B., Yang, H., Allaire, J. M., Ma, C., Graef, F. A., Mortha, A., et al. (2021). Vasoactive intestinal peptide promotes host defense against enteric pathogens by modulating the recruitment of group 3 innate lymphoid cells. Proc. Natl. Acad. Sci. U.S.A. 118. doi: 10.1073/pnas.2106634118
Yuk, M. H., Harvill, E. T., Miller, J. F. (1998). The BvgAS virulence control system regulates type III secretion in bordetella bronchiseptica. Mol. Microbiol. 28, 945–959. doi: 10.1046/j.1365-2958.1998.00850.x
Keywords: Bordetella bronchiseptica, Bordetella pertussis, Bordetella parapertussis, Bordetella, VIP (vasoactive intestinal peptide), type 3 secretion system, VPAC2 receptor, immunotherapy
Citation: First NJ, Pedreira-Lopez J, San-Silvestre MRF, Parrish KM, Lu X-H and Gestal MC (2023) Bordetella spp. utilize the type 3 secretion system to manipulate the VIP/VPAC2 signaling and promote colonization and persistence of the three classical Bordetella in the lower respiratory tract. Front. Cell. Infect. Microbiol. 13:1111502. doi: 10.3389/fcimb.2023.1111502
Received: 29 November 2022; Accepted: 01 February 2023;
Published: 29 March 2023.
Edited by:
Thuy Do, University of Leeds, United KingdomReviewed by:
Roger Derek Pechous, University of Arkansas for Medical Sciences, United StatesLonghuan Ma, University of Florida, United States
Copyright © 2023 First, Pedreira-Lopez, San-Silvestre, Parrish, Lu and Gestal. This is an open-access article distributed under the terms of the Creative Commons Attribution License (CC BY). The use, distribution or reproduction in other forums is permitted, provided the original author(s) and the copyright owner(s) are credited and that the original publication in this journal is cited, in accordance with accepted academic practice. No use, distribution or reproduction is permitted which does not comply with these terms.
*Correspondence: Monica C. Gestal, bW9uaWNhLmNhcnRlbGxlZ2VzdGFsQGxzdWhzLmVkdQ==; bWNhcmdlc0BnbWFpbC5jb20=