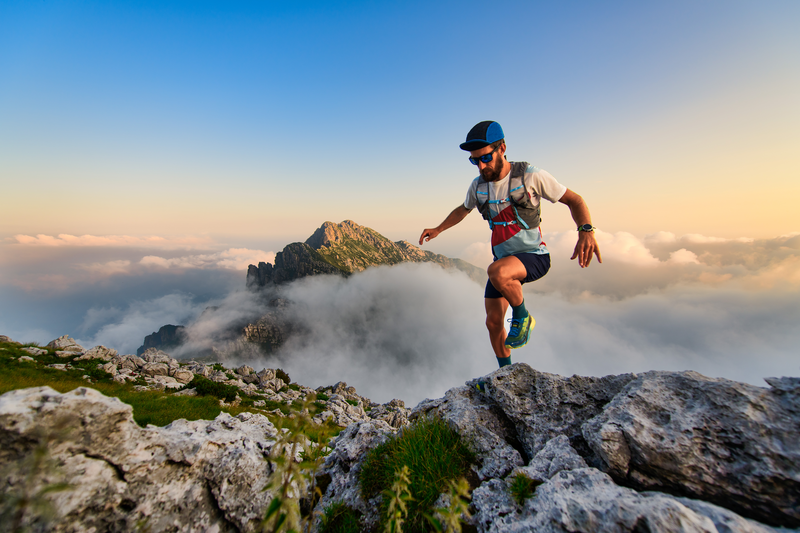
95% of researchers rate our articles as excellent or good
Learn more about the work of our research integrity team to safeguard the quality of each article we publish.
Find out more
REVIEW article
Front. Cell. Infect. Microbiol. , 28 February 2023
Sec. Microbiome in Health and Disease
Volume 13 - 2023 | https://doi.org/10.3389/fcimb.2023.1110787
This article is part of the Research Topic Gut Microbe and Colorectal Cancer View all 7 articles
Recovery from gastrointestinal (GI) surgery is often interrupted by the unpredictable occurrence of postoperative complications, including infections, anastomotic leak, GI dysmotility, malabsorption, cancer development, and cancer recurrence, in which the implication of gut microbiota is beginning to emerge. Gut microbiota can be imbalanced before surgery due to the underlying disease and its treatment. The immediate preparations for GI surgery, including fasting, mechanical bowel cleaning, and antibiotic intervention, disrupt gut microbiota. Surgical removal of GI segments also perturbs gut microbiota due to GI tract reconstruction and epithelial barrier destruction. In return, the altered gut microbiota contributes to the occurrence of postoperative complications. Therefore, understanding how to balance the gut microbiota during the perioperative period is important for surgeons. We aim to overview the current knowledge to investigate the role of gut microbiota in recovery from GI surgery, focusing on the crosstalk between gut microbiota and host in the pathogenesis of postoperative complications. A comprehensive understanding of the postoperative response of the GI tract to the altered gut microbiota provides valuable cues for surgeons to preserve the beneficial functions and suppress the adverse effects of gut microbiota, which will help to enhance recovery from GI surgery.
Gastrointestinal (GI) surgery, including gastrectomy, sleeve gastrectomy, Roux-en-Y gastric bypass (RYGB) surgery, and colorectal resections, has a well-established role in the treatment of different diseases, such as inflammatory bowel disease, metabolic syndrome, obesity, and multiple cancers (Tarazi et al., 2022). With the advances in technique, technology, and clinical care, the prognosis of GI surgery has been improved greatly. However, recovery from GI surgery is often disturbed by unpredictable postoperative complications. After surgical reconstruction, both the GI tract and gut microbiota gradually reach a new steady state. If this new state is not achieved or lacks microbial components essential for GI health, complications such as infections and anastomotic leak (AL) can develop, in which gut microbiota is not just a local bystander but one of the main determinants.
The commensal microbiota coevolves with the host, and the host immune system learns and develops from exposure to the commensal microbiota (Littman and Pamer, 2011). Gut microbiota helps absorption by breaking down ingested carbohydrates, fatty acids, and proteins into nutrients (Kolodziejczyk et al., 2019). Commensal bacteria contribute to colonization resistance and maintain epithelial mucosal barrier function, providing a functional layer against pathogen infection, and perturbation of the gut microbiota increases the risk of infectious complications (Ducarmon et al., 2019). Infectious complication as a tipping point allows pathogenic bacteria to cause major postoperative complications, including AL, disseminated infection, or superinfection.
The perioperative events of GI surgery from preoperative preparation to the recovery phase, exposing gut microbiota to substantial environmental changes and surgical stress, cumulatively influence its composition and function. Preoperative bowel preparations are known to disrupt gut microbiota (Nagata et al., 2019; Nalluri-Butz et al., 2022). GI segment resection exposes the lumen of the bowel to oxygen and vessel ligation causes a transient local blood supply interruption (Hartman et al., 2009). The bowel lumen exposure changes the oxygen partial pressure within the anaerobic intestinal environment, leading to the community shift in obligate anaerobes and facultative anaerobes (Hartman et al., 2009). Local tissue ischemia and reperfusion induce a decrease in the relative abundance of Lactobacillus and an increase in Escherichia coli (E. coli) (Wang et al., 2012; Wang et al., 2013). In addition, anatomical alterations affect GI physiology. Adaptations in GI physiology after GI surgery include changes in gastric emptying, intestinal transit, bile acid metabolism, GI surface area, acidity, and secretions, which alters the habitat of gut microbiota (Steenackers et al., 2021). Gut microbiota can sense changes in the local microenvironment and modifies its population density through quorum sensing, which also contributes to the dynamic shift of microbial phenotype (Coquant et al., 2021). Prolonged changes in circumstances give rise to potentially lethal pathogens, such as Pseudomonas aeruginosa (P. aeruginosa) and Enterococcus faecalis (E. faecalis), or make health-care-associated pathogens predominate, such as Clostridium difficile (Zaborin et al., 2014).
Therefore, postoperative rebuilding of gut microbiota is important. Inadequate or inappropriate rebuilding of gut microbiota can contribute to the development of postoperative complications. A comprehensive understanding of the interaction between host and gut microbiota is needed to enhance recovery from GI surgery. Hence, this review focuses on perioperative gut microbiota alteration and its implication in recovery from GI surgery (Figure 1).
Figure 1 The effect of perioperative events on the gut microbiota and recovery from GI surgery. Except for the basic condition of the host, the whole process of GI surgery, from preoperative preparation to recovery from GI surgery, has a significant and accumulated effect on the gut microbiota. (A) The baseline gut microbiota is dependent on different daily diets, exercise, and pre-existing diseases, such as obesity, type 2 diabetes mellitus, cardio-metabolic diseases, non-alcoholic fatty liver disease, inflammatory bowel disease, and GI cancer. (B) Neoadjuvant chemo- or radiotherapy reduces tumor size and kills metastatic cells to facilitate the subsequent tumor removal, which may disrupt gut microbiota. Preoperative bowel preparation, including fasting, MBP, and oral antibiotics, eliminates bulky GI content and reduces the luminal bacterial load, leaving behind certain mucosal bacteria. Besides, prophylactic intravenous antibiotics have a further effect on the composition of gut microbiota. (C) Surgical procedures, including laparotomy or laparoscopy, GI segment resection, and subsequent GI reconstruction, change GI physiology, exert further stress on the gut microbiota, and shift bacterial phenotypes. (D) The healthy recovery from GI surgery is challenged by postoperative complications, such as infection, anastomotic leak, GI dysmotility, malabsorption, and cancer recurrence, which are substantially influenced by perioperative alteration of gut microbiota. Appropriate rebuilding of commensal bacteria may prevent postoperative complications and enhance postoperative recovery. MBP, mechanical bowel preparation; GI, gastrointestinal.
The baseline status of gut microbiota before surgery depends on daily diet, exercise, and chronic illness. The relationship between gut microbiota, diet, and host health is complex. Diet has an indirect effect by affecting gut microbiota composition and its production of metabolites that, in turn, can maintain health, increase the risk of disease, and be used as a treatment for certain diseases (Perler et al., 2022). Preoperative dietary modulation has been a complementary consideration among surgeons to prevent postoperative complications. A previous study using an animal model demonstrates that a short course of dietary prehabilitation with a low-fat and high-fiber diet can reverse the adverse effect of a high-fat Western-type diet (WD) on anastomotic healing via its effects on the gut microbiota, improving overall diversity and decreasing postoperative collagenolytic Enterococus (Hyoju et al., 2020). Dietary prehabilitation also improves the outcome of surgically operated mice exposed to WD diet, antibiotics, and short-term starvation (Keskey et al., 2022). Moreover, butyrate, a major product of anaerobic bacteria that has known beneficial effects on the immune system, is identified as a potential candidate biomarker to assess microbiota readiness for surgery in the context of dietary prehabilitation (Keskey et al., 2022), which helps to determine whether gut microbiota are recovered enough to support a host during stress period of preoperative treatment and surgery. Exercise as an environmental factor also has effects on gut microbiota composition, which could provide benefits to health and disease prevention. Recent studies suggest that exercise can improve the development of commensal bacteria, enrich microflora diversity, and enhance the number of beneficial microbial species (Monda et al., 2017). Meanwhile, pre-existing diseases substantially alter the composition of preoperative gut microbiota and its postoperative response to GI surgery. Increasing evidence has shown that gut microbiota is changed at the site of malignant tumors and the imbalance of gut microbiota can trigger carcinogenesis (Wong and Yu, 2019). Numerous metabolic diseases (malnutrition, obesity, type 2 diabetes mellitus, cardio-metabolic diseases, and non-alcoholic fatty liver disease), autoimmune diseases (rheumatoid arthritis, spondyloarthritis, and systemic lupus erythematosus), and living environmental and behavioral factors (alcohol and smoking) are linked to the alteration of gut microbiota and affected by it, which has been reviewed in detail elsewhere (Capurso and Lahner, 2017; Jiao et al., 2020; Fan and Pedersen, 2021).
Changes in gut microbiota during preoperative treatment include both long-term chemo- or radiotherapy, not for all patients on GI surgery, and short-term just prior to surgery, such as fasting, bowel preparation, and antibiotic prophylaxis.
Neoadjuvant chemoradiotherapies for cancer can lead to compositional changes in gut microbiota. Chemotherapy drives a severe gut microbiota dysbiosis with significant decreases in the relative abundances of Firmicutes and Actinobacteria and increases in the relative abundance of Proteobacteria in patients with non-Hodgkin’s lymphoma (Montassier et al., 2015). In animal studies, 5-fluorouracil (5-Fu) reduces gut microbial community richness and diversity, leading to a decreased relative abundance of Firmicutes, Proteobacteria, Tenericutes, Cyanobacteria, and Candidate division TM7, and an increased relative abundance of Verrucomicrobia and Actinobacteria (Li et al., 2017), which is ameliorated by a probiotic mixture (Tang et al., 2017). In addition, radiotherapy reduces gut microbial diversity in patients with rising radiation enteropathy, which is significantly associated with an increased relative abundance of Clostridium IV, Roseburia, and Phascolarctobacterium (Reis Ferreira et al., 2019). In a mouse model, the relative abundances of phylum Proteobacteria, genera Escherichia, Shigella and Eubacterium xylanophilum_group, and species Lactobacillus murinus are correlated with radiation dose, and after 7 days of radiation, the diversity of gut microbiota is significantly decreased, in which the relative abundance of Proteobacteria and Bacteroides is increased, while that of Tenericutes and Roseburia is decreased, which also can be mitigated by administration of compound probiotics (Zhao et al., 2021). Moreover, not only can chemotherapy and radiotherapy alter gut microbiota, but gut microbiota can directly or indirectly modulate cancer response to chemotherapy, radiotherapy, and immunotherapy (Iida et al., 2013; York, 2018; Tonneau et al., 2021).
In addition, bowel preparations for major surgery instantly disrupt gut microbiota and might lead to prolonged detrimental consequences (Perez-Cobas et al., 2013; Zarrinpar et al., 2014; Jalanka et al., 2015). Physiological stress induced by surgical processes, involving skin incision, tissue dissection, organ resection, and anastomosis, can increase susceptibility to infection. Therefore, the current clinical practice of bowel preparation is used to eliminate potential pathogens as much as possible before major surgery to minimize intraoperative contamination. Daily feeding/fasting rhythms lead to daily cyclical compositional fluctuations in the gut microbiota, which contribute to gut microbial diversity and affect host metabolism (Zarrinpar et al., 2014). Unlike these daily gut microbiota fluctuations, altered jejunal microbiota with decreased abundance of Betaproteobacteria and Bacteroidales is observed in dogs subjected to a prolonged period of fasting (Kasiraj et al., 2016). MBP is generally used to eliminate bulky contents and reduce the bacterial load in the GI tract, which leaves behind certain strains of mucosa-associated bacteria and induces shifts in gut microbiota. The lavage leads to an instant and substantial change in the bacterial levels and composition of gut microbiota, specifically decreased Bifidobacterium and Lactobacillus and increased E. coli and Staphylococcus, which can be restored within 14 days, and the recovery rate is dose-dependent (Wu et al., 2012; Jalanka et al., 2015). MBP has a profound effect on the gut metabolome, but it also recovers to baseline within 14 days (Nagata et al., 2019). In contrast, MBP with oral antibiotics shapes gut microbiota composition acutely and longitudinally, which requires at least 30 days to return to a level similar to baseline (Nalluri-Butz et al., 2022). Oral or intravenous antibiotics and the local application of topical antimicrobial solutions are variably used as routine practices to disinfect the intestine and skin (Guenaga et al., 2011; Nelson et al., 2014). Oral antibiotics are often used for bowel preparation and most patients receive intravenous antibiotics at the time of GI surgery, both of which facilitate the clearance of potential pathogens, exert selective pressure on bacterial composition and function, and further perturb commensal microbial communities (Antonopoulos et al., 2009; Ubeda and Pamer, 2012; Ferrer et al., 2014). The effects of these preparations for surgery on gut microbiota preservation and bacterial phenotype shifts remain unknown and need further study.
The surgery itself is the major factor affecting gut microbiota composition and function. After surgical resection of diseased, obstructed, or ischemic intestinal segments, intestinal reconstructions are generally required to reestablish intestinal continuity. These surgical reconstructions of the GI tract affect the intraluminal environment, intestinal permeability, transit time, food digestion, and nutrient absorption, but the GI tract can adapt to the new anatomical structure, which is a natural compensatory process and nearly allows patients to recover to normalcy (Carswell et al., 2014; Tappenden, 2014; Ladebo et al., 2021). It is now believed that much of food digestion and nutrient absorption depends on the action of the gut microbiota, and gut microbes use ingested nutrients for their fundamental biological processes, metabolic outputs of which have significant impacts on host physiology (Gentile and Weir, 2018). Besides, each part of the intestine has a specialized ecosystem that facilitates the breakdown, processing, and absorption of nutrients, and the surgical reconstructions of the GI tract can change downstream gut microbiota, affecting the metabolism and immune functions (Sinclair et al., 2018; Li et al., 2021). The effects of these changes on the clinical outcomes of GI surgery need further investigation.
Sleeve gastrectomy (SG) and Roux−en−Y gastric bypass (RYGB) are the most common surgical approaches to treat morbid obesity. The beneficial effects of bariatric surgery are not only contributed by stomach pouch restriction and malabsorptive configuration induced by the surgical operation itself, but changes in gut microbiota may also be part of the mechanism. Although these surgeries are not for treating specific gastrointestinal diseases in this setting, their effects on gut microbiota are now being studied. Gut microbiota is altered by surgery and the profound alterations persist in the first year of follow-up with an increase in Bacteroides and Proteobacteria and a decrease in Firmicutes in most studies (Luijten et al., 2019). The shift of gut microbiota after a bariatric surgery significantly differs between different surgical procedures. RYGB has a deeper impact on the composition and function of gut microbiota than SG (Farin et al., 2020; Salazar et al., 2022). Laparoscopic RYGB leads to a higher relative abundance of aero-tolerant bacteria (E. coli and Streptococcus), whereas anaerobes (Clostridium) are more abundant after SG (Farin et al., 2020). In addition, enrichment of Akkermansia muciniphila is observed 6 months after both surgeries (Farin et al., 2020). In another short-term study, the relative abundance of Akkermansia, Eubacterium, Haemophilus, and Blautia is higher 3 months after SG, while the relative abundance of Veillonella, Slackia, Granucatiella, and Acidaminococcus is higher after RYGB (Sanchez-Alcoholado et al., 2019). Changes in gut microbiota are an adaptive response to the altered gut environment, and specific gut microbiota signatures mediate the successful rate of bariatric surgery through their interaction with the bile acids milieu (Gutierrez-Repiso et al., 2019). Moreover, these alterations to the gut microbiota after RYGB are conserved among humans, rats, and mice, with a rapid and sustained increase in Bacteroidetes, Proteobacteria (Escherichia), and Verrucomicrobia (Akkermansia) (Liou et al., 2013). Besides, fecal microbiota transplant (FMT) from RYGB-treated mice to germ-free mice not receiving intestinal reconstruction leads to weight loss and decreased fat mass in the recipient mice compared to those that receive FMT from sham surgery mice (Liou et al., 2013), suggesting that gut microbiota after RYGB contributes to the effects of RYGB on the body weight and metabolism. These studies indicate that intestinal surgery-induced altered gut microbiota-host interactions, in turn, affect the prognosis of surgery.
Alterations of gut microbiota after gastrectomy are observed in patients with gastric cancer, showing greater species diversity and richness, higher abundance of oral microbes, aerobes (Streptococcus and Enterococcus), and facultative anaerobes (Escherichia, Enterobacter, and Streptococcus) than control participants (Erawijantari et al., 2020). In addition, gut microbiota plays an important role in the occurrence and development of colorectal cancer (CRC) (Lin et al., 2019). It is worth noting that several CRC-related bacteria (Fusobacterium nucleatum and Atopobium parvulum) and secondary bile acids such as genotoxic deoxycholic acid are significantly enriched in patients undergoing total gastrectomy compared with the control group (Erawijantari et al., 2020). In contrast, surgery greatly reduces the diversity of the gut microbiota in CRC patients (Cong et al., 2018; Deng et al., 2018). The gut microbiota of postoperative patients and CRC patients differ significantly. The relative abundance of Proteobacteria is increased in postoperative CRC patients compared with that in preoperative CRC patients and healthy individuals, and the Klebsiella has a higher proportion in postoperative CRC patients than that in preoperative CRC patients, which is also significantly associated with infectious diseases and lymphatic invasion (Cong et al., 2018). Subdividing postoperative CRC patients according to the presence or absence of newly developed adenoma, the gut microbiota of patients with newly developed adenoma is different from that of clean intestine patients and is similar to the gut microbiota of carcinoma patients (Jin et al., 2019). The difference in the gut microbiota between the two groups can be used as biomarkers to distinguish postoperative patients with or without newly developed adenoma with an AUC value of 0.72 (Jin et al., 2019), suggesting that gut microbiota may be used as non-invasive biomarkers to predict newly developed adenomas and prevent cancer recurrence in postoperative patients. These changes in the gut microbiota are long-lasting and correlate with the clinical course. Especially in CRC patients with postoperative complications, the gut microbiota shows significant changes in postoperative CRC patients, which do not resolve, even 24 months after surgery (Schmitt et al., 2021). The association between long-term gut microbiota alteration and postoperative complications indicates that gut microbiota modulation may help to optimize the outcome of CRC patients after surgery.
Numerous postoperative complications, such as infections, AL, GI dysmotility, malabsorption, and cancer recurrence, continue to hinder recovery from GI surgery with complex reconstructive procedures. A better molecular understanding of the perioperative interaction between the GI tract and gut microbiota will make surgery safer and further prevent complications.
Postoperative infection, surgical site infections (SSIs) in particular, is the most common reason for readmission, which contributes to the increased cost of health care (Merkow et al., 2015). In general, GI surgery is subjected to a greater risk of postoperative infection compared with other surgical procedures because the GI tract is inhabited by a wide cluster of microorganisms. Traditionally, postoperative infection is often caused by inadequate preoperative topical or intestinal disinfection, which can be prevented by antibiotics as prophylaxis the day before surgery, even without mechanical bowel preparation (Espin Basany et al., 2020). However, complete depletion of gut and skin microbiota is not possible and has potential negative effects. Many postoperative infections arise from the patient’s gut microbiota, usually after unintended inhibition of beneficial bacteria and translocation of antibiotic-resistant pathogenic bacteria (Deitch, 2012; Yu et al., 2014). Conversely, a diverse and protective gut microbiota provides an important biological layer against infectious complications, because commensal bacteria contribute to colonization resistance against both endogenous and exogenous pathogens through competitive inhibition, production of antimicrobial peptides, and activation of the host immune system (Ducarmon et al., 2019; Isles et al., 2022).
Lifestyle factors, underlying diseases, medications, antibiotics, and surgical procedures can perturb gut microbiota leading to loss of colonization resistance and increased susceptibility to the invasion of pathogenic bacteria. Besides, many in−hospital infections originate from the patient’s gut microbiota when the beneficial bacterial population is suppressed (Wang et al., 2019). Preoperative dysbiosis of gut microbiota is associated with higher rates of postoperative infectious complications, including abdominal/pelvic infections and pulmonary infections, in CRC patients (Liu et al., 2021). The increased relative abundance of Klebsiella in postoperative CRC patients is significantly and positively associated with infectious diseases, such as bacterial invasion of epithelial cells and Staphylococcus aureus infection, revealed by the correlation analysis between differentiated metabolic pathways and genera (Cong et al., 2018). The causative bacteria of surgical site infections are identified to be P. aeruginosa, Staphylococcus aureus, and Enterococcus spp., which are also enriched in the fecal microbiota of postoperative CRC patients (Ohigashi et al., 2013). A gut microbiota-based model base on six genera (Hungatella, Epulopiscium, Fusobacterium, Ruminococcaceae_ucg_009, Actinomyces, and Ralstonia) is used to predict surgical site infections after ileocolonic resection for Crohn’s disease (CD) patients with an AUC of 0.78 (Julien et al., 2022).
The effect of perioperative modulation of gut microbiota by probiotic or synbiotic on postoperative infectious complications has been evaluated in clinical trials (Table 1). A meta-analysis shows that perioperative probiotic or prebiotic supplements may reduce the overall incidence of infectious complications, including wound infections, respiratory infections, and urinary tract infections, in patients undergoing GI surgery, and the most used strains are Lactobacillus and Bifidobacterium (Yang et al., 2017). Their role in postoperative infections may be due to the perioperative stabilization of gut microbiota and alleviation of the systemic inflammatory response (Kotzampassi et al., 2015; Polakowski et al., 2019). Using probiotics or prebiotics as a potential alternative to maintaining the beneficial structure of gut microbiota throughout the whole process of hospitalization may be a promising strategy to reduce the risk of postoperative infections and can be included in enhanced recovery after surgery. However, the results of existing clinical studies are inconsistent, which may be due to disparities in type, dosage, and administration strategies (timing and duration) of probiotics/prebiotics, thus large-scale randomized clinical trials are needed to confirm the efficacy and safety of probiotics/prebiotics in surgical patients.
Table 1 Effect of synbiotics or probiotics on infectious complications, anastomotic leak, and gastrointestinal motility after gastrointestinal surgery.
Anastomotic leak (AL) is one of the most potentially devastating complications that can develop after major surgical bowl reconstruction and has plagued surgeons for decades. Although surgical technique and postoperative care have been improved over the past several decades, AL continues to occur and can lead to peritonitis, sepsis, and even death (Caulfield and Hyman, 2013; Shogan et al., 2013). After anastomosis is performed, the repairing process of the GI tract is immediately initiated and divided into three phases, including the lag phase, the fibroplasia phase, and the maturation phase (Sajid et al., 2012). Ultimately, the healing process results in the repair of the intestinal epithelial barrier with the involvement of complex molecular and cellular interactions of host cells, luminal proliferative components, and gut microbiota (Kurashima and Kiyono, 2017). Among these factors contributing to anastomotic healing, gut microbiota remains largely overlooked and should be focused on. Indeed, germ-free mice have a reduced regenerative response of the epithelia and a hampered healing process of the intestinal barrier compared with conventional mice (Hooper et al., 2001). In addition, conventionalized germ-free rats show a significantly better wound healing of intestinal anastomoses than germ-free animals and rats that are colonized by either Lactobacillus acidophilus or E. coli (Okada et al., 1999), suggesting that the effect of gut microbiota on the healing of intestinal anastomoses depends on differences in the types of bacteria. Therefore, gut microbiota can either assist or hinder intestinal wound healing through cooperation or competition between different microbial species (Koliarakis et al., 2020). Further studies focusing on gut microbiota could be one such avenue for uncovering the elusive pathogenesis of AL.
The gut microbiota participates in the physiological process of intestinal wound healing and epithelial repair through a variety of molecular mechanisms. Members of the gut microbiota can interact with different intestinal epithelial lineages through innate immune receptors, such as toll-like receptor (TLR) 4 and 2, by recognizing gut microbial components, such as lipopolysaccharide and flagellin, thus modulating homeostasis in the gut and playing an important role in the epithelial repair after injury (Burgueno and Abreu, 2020). Metabolites produced by gut microbiota are also involved in the intestinal epithelial repair. Butyrate, one of the SCFAs derived from the bacterial fermentation of dietary fibers, regulates colonocyte proliferation, strengthens the gut barrier, limits pathogen growth, and suppresses inflammatory response (Martin-Gallausiaux et al., 2021). Several animal studies have shown that exogenous butyrate administration improves the healing of colonic anastomoses and enhances colonic anastomotic strength in rats (Rolandelli et al., 1997; Mathew et al., 2010; Bosmans et al., 2017). Besides, perioperative supplementation of inulin and galactooligosaccharides, which modulate the gut microbiota to increase the production of butyrate through enhancement of butyrate-producing bacteria, improve anastomotic healing and reinforce the gut barrier in mice (Hajjar et al., 2021). Moreover, SCFAs produced by gut microbiota may be a mechanism of intestinal resistance to colonization by P. aeruginosa, which has been identified as an AL-related pathogen (Levison, 1973; Williamson and Alverdy, 2021). These data indicate the importance of functional gut microbiota to ensure adequate healing, but the stress of perioperative events and surgery itself may induce a shift of gut microbiota to a pathologic phenotype, which leads to an AL.
Previous studies have investigated the causal relationship between AL and gut microbiota. Anastomotic injury, without preoperative MBP or antibiotic prophylaxis, contributes to remarkable compositional and functional changes in the anastomotic tissue-associated microbiota with a 200-fold and 500-fold increase in the relative abundance of Escherichia-Shigella and Enterococcus, respectively (Shogan et al., 2014). However, these alterations were not observed in the stool microbiota (Shogan et al., 2014), suggesting that certain bacteria having adhesive properties invade the anastomotic site, which may potentially complicate or accelerate anastomotic healing.
Clinically relevant animal models of AL are created to investigate the responsible gut microbiota-related mechanisms involved in the pathogenesis of AL. A novel model of AL, in which rats are exposed to preoperative radiation as in patients with advanced rectal cancer and undergo a distal colon resection followed by intestinal inoculation with P. aeruginosa, a common pathogen in the gut following radiation exposure, is developed (Olivas et al., 2012). Only those rats that are both exposed to radiation and colonized by P. aeruginosa occur AL. Sequencing of retrieved P. aeruginosa strains from the leaking anastomotic tissues demonstrates that the appearance of a single nucleotide polymorphism mutation in the mexT gene leads to a stop codon and subsequent a non-functional truncated protein, which induces P. aeruginosa in vivo transformation to more destructive phenotype with enhanced abilities of collagen degradation, invasion, and cytotoxicity. This study indicates that interactions between pathogen and host stimulate and contribute to the development of bacterial virulence and subsequent AL. More molecular detail on the microbiota-dependent pathogenesis of AL also has been identified. A commensal bacterium, E. faecalis, contributes to the pathogenesis of AL by degrading collagen and activating host matrix metalloproteinase 9 (MMP9) (Shogan et al., 2015). Rat receiving a low colonic reconstruction and segmental devascularization develop a 50% incidence of AL. The leaking anastomotic tissues are colonized by E. faecalis strains with high collagenase activity. In contrast, the healed anastomotic tissues harbor low collagenase E. faecalis strains. Both eliminations of E. faecalis strains by direct topical antibiotics and pharmacological suppression of intestinal MMP9 activation prevent AL. Mechanically, high collagenase-producing strains directly cleave collagen I and indirectly break down collagen IV by converting tissue MMP9 to its active form, which is dependent on the collagenase-encoding genes gelE and sprE. Fusobacterium nucleatum (F. nucleatum) also can induce colon AL by activating epithelial cells to express MMP9 (Shi et al., 2022). Recent studies using FMT demonstrate a causal role of the preoperative and postoperative gut microbiota in AL. FMT using postoperative stool samples from AL patients leads to poor anastomotic healing in rats (Jin et al., 2022). Mice receiving preoperative stool samples from AL patients with CRC also display poor surgical healing, which is correlated with two bacterial strains, Alistipes onderdonki (A. onderdonkii) and Parabacteroides goldsteinii (P. goldsteinii), and A. onderdonkii supplementation leads to a higher rate of leaks in mice (Hajjar et al., 2022). Overall, some individual pathogens, including E. faecalis, P. aeruginosa, F. nucleatum, and A. onderdonkii, have been implicated in the pathogenesis of AL by promoting the breakdown of collagen (Figure 2). A short course of dietary prehabilitation decreases postoperative collagenolytic Enterococcus and reduces AL in mice (Hyoju et al., 2020). Although the effect of probiotics or synbiotics on the AL is not satisfactory in most clinical cases according to limited reports (Table 1), P. goldsteinii plays an anti-inflammatory effect and improves wound healing in a murine AL model (Hajjar et al., 2022), representing a potential probiotic that may enhance postoperative recovery in patients at risk of developing AL.
Figure 2 Role of bacteria in the pathogenesis of anastomotic leak. Surgical resection and reconstruction induce physiological stress and lead to a release of inflammatory signals, which contribute to phenotype transformation or selection of gut microbiota. E. Faecalis and P. aeruginosa sense and respond to the host’s local stress signals resulting in increased adherence capacity and collagenase production. Bacterial collagenase directly breaks down collagen I and indirectly breaks down collagen IV by converting local tissue MMP9 to its active form. SCFAs-producing microbiota prevents P. aeruginosa colonization. Besides, F. nucleatum can stimulate epithelial cells’ MMP9 expression contributing to the loss of submucosa collagen I and III, and A. onderdonkii-induced TNF-α and IL-1β production by monocytes and neutrophils are also involved in MMP9-dependent breakdown of collagen. This process results in a breakdown of anastomotic tissue and is involved in the pathogenesis of anastomotic leak. MMP9, matrix metalloproteinase 9; SCFAs, short chain fatty acids; F. nucleatum, Fusobacterium nucleatum.
Taken together, these animal data suggest that bacterial phenotypes can be reshaped in vivo by injured tissue, which may have a profound effect on the recovery from GI surgery. Targeting the gut microbiota as a modifiable factor may be a novel strategy for the prevention of AL.
GI dysmotility is common after major GI reconstruction. This dysmotility can range from malabsorption, which is associated with increased motility, to postoperative ileus (POI). Gut microbiota plays a significant role in the regulation of GI physiology, particularly GI motility (Zheng et al., 2022).
Altered gut microbiota after GI surgery substantially contributes to changes in GI motility. POI is one of the most frequent complications after GI surgery. Mice treated with oral antibiotics show a moderate alleviation in small intestinal POI, whereas POI is improved by antibiotic treatment in the colon (Pohl et al., 2017). Besides, there is a big difference in the quantity and composition of the gut microbiota between the small and large intestines (Mowat and Agace, 2014; Martinez-Guryn et al., 2019), indicating the essential role of gut microbiota in the induction of POI. In the clinical setting, CRC patients with ileus have a lower α-diversity and a higher Firmicutes to Bacteroidetes ratio of gut microbiota compared with CRC patients without ileus (Jin et al., 2020). The relative abundance of Proteobacteria is high in CRC patients with ileus, whereas the relative abundances of Bacteroidetes, Firmicutes, and Fusobacterium are high in CRC patients without ileus. At the genus level, Escherichia-Shigella, Ralstonia, and Veillonella are significantly greater in the group with ileus than that without ileus. For POI, CRC patients with a low abundance of Faecalibacterium have a high risk of POI, and Faecalibacterium as a biomarker has an AUC value of 0.74 for the prediction of POI and an AUC value of 0.67 for the prediction of POI that occurs 6 months after discharge from hospital (Jin et al., 2020). Preoperative probiotic treatment improves bowel movement in the guinea pig with POI, probably by restoring the beneficial bacterial species Bifidobacterium bifidum and Bifidobacterium longum and increasing butyrate production (Shin et al., 2021). Moreover, a meta-analysis of 21 randomized controlled trials shows that prophylactic supplements of probiotics or synbiotics can effectively shorten the time of first flatus, first defecation, and first diet and reduce the incidence of POI in patients receiving GI cancer surgery (Tang et al., 2022). In contrast, preoperative stimulation of the efferent loop with probiotics does not affect the appearance of POI in patients undergoing loop ileostomy closure, which may be due to the previous existence of POI after colorectal cancer surgery (Rodriguez-Padilla et al., 2021). Nevertheless, perioperative gut microbiota modulation by supplementation of probiotics or synbiotics may be used to improve the recovery of GI motility after GI surgery (Table 1), but the underlying mechanisms and what changes in gut microbiota resulting from bowel preparation and surgery contribute to GI dysmotility remain to be elucidated.
Complex anatomical GI reconstructions, such as gastrectomy, RYGB surgery, and pancreaticoduodenectomy, can lead to fat malabsorption, dumping syndrome, and vitamin deficiencies. Patients subjected to RYGB are at an increased risk of malabsorption resulting in trace element deficiency and osteopenia (Hammer, 2012; Billeter et al., 2015). Gut microbiota seems to be an important mediator in this process because FMT from RYGB-treated mice to germ-free mice without RYGB results in weight loss and decreased fat mass when compared with mice receiving FMT from sham surgery controls, potentially by regulating the production of SCFAs (Liou et al., 2013). The energy-reabsorbing potential of the gut microbiota, indicated by the Bacteroidetes to Firmicutes ratio, is decreased following laparoscopic sleeve gastrectomy (Damms-Machado et al., 2015), and gut microbiota can also play a significant role in the effects of RYGB on energy homeostasis (Chakravartty et al., 2015). The increased relative abundance of Gammaproteobacteria is closely associated with malabsorption after RYGB (Furet et al., 2010). Although malabsorption can be treated by vitamin supplementation and dietary modifications to adapt to the changed metabolism in many cases (Bland et al., 2016), the effect of the gut microbiota in the digestion and absorption after major surgery with GI reconstruction needs a further understanding, which will provide cues for development of therapies to accelerate postoperative recovery.
The risk of colorectal cancer (CRC) may be increased after gastric bypass over time, whereas the risk of hormone-related cancers, including breast, endometrium, and prostate cancer, is associated with bariatric surgery (Derogar et al., 2013; Mackenzie et al., 2018). Besides, CRC risk is affected by sex and type of surgery. An increased risk of CRC is observed in males compared to females, especially 3 years or more after bariatric surgery, whereas CRC risk is decreased in females after RYGB but not sleeve gastrectomy (Hussan et al., 2022). Postoperative alteration of gut microbiota influences cancer risk and recurrence. Both decreases in absorptive GI mucosa and changes in the gut microbiota after bowel reconstruction contribute to disruption in the processing of bile acid (Flynn et al., 2015; Albaugh et al., 2019), which may expose colonocytes to more secondary bile acid, in particular deoxycholic acid (DCA), activating multiple signaling pathways including EGFR and Wnt in enterocytes that can lead to the development of CRC (Jia et al., 2018). Increased mucosal exposure to bile acid has been proposed to increase the risk of CRC after RYGB (Kant et al., 2014).
Gut microbiota also has been associated with CRC recurrence after surgery. Although patients undergoing surgery are often optimized with adjuvant or neoadjuvant chemoradiotherapy, up to one-third of them will get a postoperative CRC recurrence (Jeffery et al., 2007). In the traditional view, cancer recurrence is due to the postoperative blossom of shed tumor cells either at the surgical site or unknowingly present in the distal organs at the time of surgery. However, an opinion that the gut microbiota influences postoperative cancer recurrence is emerging (Figure 3).
Figure 3 Possible mechanism of bacteria-associated colorectal cancer recurrence after surgical resection. A specific local context is created by surgical resection and reconstruction. Shed cancer cells occur during and after surgery. Context-dependent colonization of collagenase-producing bacteria, such as Enterococcus faecalis, cooperating with local macrophages, impairs anastomotic healing and promotes shed cancer cell proliferation, migration, and invasion, leading to cancer recurrence. MMP9, matrix metalloproteinase 9.
Spatial-specific gut microbiota profiles link to CRC recurrence. The composition of gut microbiota at adjacent-tumor sites of patients with CRC recurrence is different from that in patients without CRC recurrence (Huo et al., 2022). At the phylum level, the relative abundance of Fusobacteria at adjacent tumor sites is much higher in patients with CRC recurrence than that in patients without CRC recurrence (Huo et al., 2022). Gut microbiota can serve as biomarkers to predict the risk of CRC recurrence. Besides, the postoperative gut microbiota can drive shed cancer cells to a migratory and aggressive phenotype, assisting them to escape immune surveillance to promote a postoperative recurrence. Through matrix metalloproteinases or the urokinase-plasminogen system, the collagenase-producing bacteria can bind to and degrade extracellular matrix, breakdown products of which are well known to promote proliferation, migration, and invasion of cancer cells (Shogan et al., 2015; He et al., 2016; Jacobson et al., 2020). E. faecalis, a high collagenase-producing strain, cooperating with macrophages reshapes colonic epithelial cells to a mesenchymal phenotype that is similar to the phenotype seen in mesenchymal transition, which is a fundamental biological process of tumor metastasis (Belogortseva et al., 2017). Indeed, colonization of collagenase-producing E. faecalis or Proteus mirabilis promotes shed cancer cells to form a tumor in mice on a high-fat diet undergoing a colon resection and anastomosis, which can be prevented by administration of a collagenase inhibitor (Gaines et al., 2020). Thus, gut microbiota-targeted strategies may be used to reduce the risk of CRC recurrence by eliminating these bacteria or inhibiting their collagenase activities.
Emerging evidence supports the role of gut microbiota in our understanding of postoperative complications. The causative role of gut microbiota in recovery from GI surgery needs to be further defined through in-depth research of the shift of bacterial phenotype, the interaction between gut microbiota and host, and their effects on the local microenvironment. Comprehensive knowledge of perioperative gut microbiota can provide a possibility for designing novel appropriate strategies for personalized bowel preparation. Fecal culture is limited to detail molecular information. Both before and after surgery, next-generation sequencing and phenotype analysis are necessary to serially track the composition and function of the bacterial community, which will advance the understanding of the gut microbiota and guide clinical care over the entire course of recovery. Resultant therapeutic innovations in the maintenance of gut microbiota and control of postoperative complications include methods to selectively kill pathogenic bacteria using narrow-spectrum antibiotics, antibody-labeled antibiotics, or engineered bacteriophages, and methods to restore gut microbiota using probiotics, prebiotics, bacterial ligands, or FMT. The molecular mechanistic understanding of the role of gut microbiota in postoperative complications will finally help to enhance recovery from GI surgery.
ZZ managed the major literature research and wrote the first draft of the manuscript. YH, JT, WX, and WHZ managed literature research and reviewed the manuscript. WZ critically reviewed the manuscript and provided valuable discussions and criticisms. All authors contributed to the article and approved the submitted version.
This study was supported by National Natural Science Foundation of China (grant number 82274475).
The authors declare that the research was conducted in the absence of any commercial or financial relationships that could be construed as a potential conflict of interest.
The reviewer XC declared a shared parent affiliation with the authors to the handling editor at the time of review.
All claims expressed in this article are solely those of the authors and do not necessarily represent those of their affiliated organizations, or those of the publisher, the editors and the reviewers. Any product that may be evaluated in this article, or claim that may be made by its manufacturer, is not guaranteed or endorsed by the publisher.
Albaugh, V. L., Banan, B., Antoun, J., Xiong, Y., Guo, Y., Ping, J., et al. (2019). Role of bile acids and GLP-1 in mediating the metabolic improvements of bariatric surgery. Gastroenterology 156 (4), 1041–1051.e4. doi: 10.1053/j.gastro.2018.11.017
Antonopoulos, D. A., Huse, S. M., Morrison, H. G., Schmidt, T. M., Sogin, M. L., Young, V. B. (2009). Reproducible community dynamics of the gastrointestinal microbiota following antibiotic perturbation. Infect. Immun. 77 (6), 2367–2375. doi: 10.1128/IAI.01520-08
Bajramagic, S., Hodzic, E., Mulabdic, A., Holjan, S., Smajlovic, S. V., Rovcanin, A. (2019). Usage of probiotics and its clinical significance at surgically treated patients sufferig from colorectal carcinoma. Med. Arch. 73 (5), 316–320. doi: 10.5455/medarh.2019.73.316-320
Belogortseva, N., Krezalek, M., Guyton, K., Labno, C., Poroyko, V., Zaborina, O., et al. (2017). Media from macrophages co-incubated with enterococcus faecalis induces epithelial cell monolayer reassembly and altered cell morphology. PloS One 12 (8), e0182825. doi: 10.1371/journal.pone.0182825
Billeter, A. T., Probst, P., Fischer, L., Senft, J., Kenngott, H. G., Schulte, T., et al. (2015). Risk of malnutrition, trace metal, and vitamin deficiency post roux-en-Y gastric bypass–a prospective study of 20 patients with BMI < 35 kg/m(2). Obes. Surg. 25 (11), 2125–2134. doi: 10.1007/s11695-015-1676-9
Bland, C. M., Quidley, A. M., Love, B. L., Yeager, C., McMichael, B., Bookstaver, P. B. (2016). Long-term pharmacotherapy considerations in the bariatric surgery patient. Am. J. Health Syst. Pharm. 73 (16), 1230–1242. doi: 10.2146/ajhp151062
Bosmans, J. W., Jongen, A. C., Boonen, B. T., van Rijn, S., Scognamiglio, F., Stucchi, L., et al. (2017). Comparison of three different application routes of butyrate to improve colonic anastomotic strength in rats. Int. J. Colorectal Dis. 32 (3), 305–313. doi: 10.1007/s00384-016-2718-z
Burgueno, J. F., Abreu, M. T. (2020). Epithelial toll-like receptors and their role in gut homeostasis and disease. Nat. Rev. Gastroenterol. Hepatol. 17 (5), 263–278. doi: 10.1038/s41575-019-0261-4
Capurso, G., Lahner, E. (2017). The interaction between smoking, alcohol and the gut microbiome. Best Pract. Res. Clin. Gastroenterol. 31 (5), 579–588. doi: 10.1016/j.bpg.2017.10.006
Carswell, K. A., Vincent, R. P., Belgaumkar, A. P., Sherwood, R. A., Amiel, S. A., Patel, A. G., et al. (2014). The effect of bariatric surgery on intestinal absorption and transit time. Obes. Surg. 24 (5), 796–805. doi: 10.1007/s11695-013-1166-x
Caulfield, H., Hyman, N. H. (2013). Anastomotic leak after low anterior resection: A spectrum of clinical entities. JAMA Surg. 148 (2), 177–182. doi: 10.1001/jamasurgery.2013.413
Chakravartty, S., Tassinari, D., Salerno, A., Giorgakis, E., Rubino, F. (2015). What is the mechanism behind weight loss maintenance with gastric bypass? Curr. Obes. Rep. 4 (2), 262–268. doi: 10.1007/s13679-015-0158-7
Cong, J., Zhu, H., Liu, D., Li, T., Zhang, C., Zhu, J., et al. (2018). A pilot study: Changes of gut microbiota in post-surgery colorectal cancer patients. Front. Microbiol. 9, 2777. doi: 10.3389/fmicb.2018.02777
Consoli, M. L., da Silva, R. S., Nicoli, J. R., Bruna-Romero, O., da Silva, R. G., de Vasconcelos Generoso, S., et al. (2016). Randomized clinical trial: Impact of oral administration of saccharomyces boulardii on gene expression of intestinal cytokines in patients undergoing colon resection. JPEN J. Parenter Enteral Nutr. 40 (8), 1114–1121. doi: 10.1177/0148607115584387
Coquant, G., Aguanno, D., Pham, S., Grellier, N., Thenet, S., Carriere, V., et al. (2021). Gossip in the gut: Quorum sensing, a new player in the host-microbiota interactions. World J. Gastroenterol. 27 (42), 7247–7270. doi: 10.3748/wjg.v27.i42.7247
Damms-Machado, A., Mitra, S., Schollenberger, A. E., Kramer, K. M., Meile, T., Konigsrainer, A., et al. (2015). Effects of surgical and dietary weight loss therapy for obesity on gut microbiota composition and nutrient absorption. BioMed. Res. Int. 2015, 806248. doi: 10.1155/2015/806248
Deitch, E. A. (2012). Gut-origin sepsis: Evolution of a concept. Surgeon 10 (6), 350–356. doi: 10.1016/j.surge.2012.03.003
Deng, X., Li, Z., Li, G., Li, B., Jin, X., Lyu, G. (2018). Comparison of microbiota in patients treated by surgery or chemotherapy by 16S rRNA sequencing reveals potential biomarkers for colorectal cancer therapy. Front. Microbiol. 9, 1607. doi: 10.3389/fmicb.2018.01607
Derogar, M., Hull, M. A., Kant, P., Ostlund, M., Lu, Y., Lagergren, J. (2013). Increased risk of colorectal cancer after obesity surgery. Ann. Surg. 258 (6), 983–988. doi: 10.1097/SLA.0b013e318288463a
Ducarmon, Q. R., Zwittink, R. D., Hornung, B. V. H., van Schaik, W., Young, V. B., Kuijper, E. J. (2019). Gut microbiota and colonization resistance against bacterial enteric infection. Microbiol. Mol. Biol. Rev. 83 (3), e00007–19. doi: 10.1128/MMBR.00007-19
Erawijantari, P. P., Mizutani, S., Shiroma, H., Shiba, S., Nakajima, T., Sakamoto, T., et al. (2020). Influence of gastrectomy for gastric cancer treatment on faecal microbiome and metabolome profiles. Gut 69 (8), 1404–1415. doi: 10.1136/gutjnl-2019-319188
Espin Basany, E., Solis-Pena, A., Pellino, G., Kreisler, E., Fraccalvieri, D., Muinelo-Lorenzo, M., et al. (2020). Preoperative oral antibiotics and surgical-site infections in colon surgery (ORALEV): A multicentre, single-blind, pragmatic, randomised controlled trial. Lancet Gastroenterol. Hepatol. 5 (8), 729–738. doi: 10.1016/S2468-1253(20)30075-3
Fan, Y., Pedersen, O. (2021). Gut microbiota in human metabolic health and disease. Nat. Rev. Microbiol. 19 (1), 55–71. doi: 10.1038/s41579-020-0433-9
Farin, W., Onate, F. P., Plassais, J., Bonny, C., Beglinger, C., Woelnerhanssen, B., et al. (2020). Impact of laparoscopic roux-en-Y gastric bypass and sleeve gastrectomy on gut microbiota: A metagenomic comparative analysis. Surg. Obes. Relat. Dis. 16 (7), 852–862. doi: 10.1016/j.soard.2020.03.014
Ferrer, M., Martins dos Santos, V. A., Ott, S. J., Moya, A. (2014). Gut microbiota disturbance during antibiotic therapy: A multi-omic approach. Gut Microbes 5 (1), 64–70. doi: 10.4161/gmic.27128
Flesch, A. T., Tonial, S. T., Contu, P. C., Damin, D. C. (2017). Perioperative synbiotics administration decreases postoperative infections in patients with colorectal cancer: A randomized, double-blind clinical trial. Rev. Col Bras. Cir 44 (6), 567–573. doi: 10.1590/0100-69912017006004
Flynn, C. R., Albaugh, V. L., Cai, S., Cheung-Flynn, J., Williams, P. E., Brucker, R. M., et al. (2015). Bile diversion to the distal small intestine has comparable metabolic benefits to bariatric surgery. Nat. Commun. 6, 7715. doi: 10.1038/ncomms8715
Folwarski, M., Dobosz, M., Malgorzewicz, S., Skonieczna-Zydecka, K., Kazmierczak-Siedlecka, K. (2021). Effects of lactobacillus rhamnosus GG on early postoperative outcome after pylorus-preserving pancreatoduodenectomy: A randomized trial. Eur. Rev. Med. Pharmacol. Sci. 25 (1), 397–405. doi: 10.26355/eurrev_202101_24407
Furet, J. P., Kong, L. C., Tap, J., Poitou, C., Basdevant, A., Bouillot, J. L., et al. (2010). Differential adaptation of human gut microbiota to bariatric surgery-induced weight loss: links with metabolic and low-grade inflammation markers. Diabetes 59 (12), 3049–3057. doi: 10.2337/db10-0253
Gaines, S., van Praagh, J. B., Williamson, A. J., Jacobson, R. A., Hyoju, S., Zaborin, A., et al. (2020). Western Diet promotes intestinal colonization by collagenolytic microbes and promotes tumor formation after colorectal surgery. Gastroenterology 158 (4), 958–970.e2. doi: 10.1053/j.gastro.2019.10.020
Gentile, C. L., Weir, T. L. (2018). The gut microbiota at the intersection of diet and human health. Science 362 (6416), 776–780. doi: 10.1126/science.aau5812
Guenaga, K. F., Matos, D., Wille-Jorgensen, P. (2011) Mechanical bowel preparation for elective colorectal surgery. Cochrane Database Syst. Rev. 2011 (9), CD001544. doi: 10.1002/14651858.CD001544.pub4
Gutierrez-Repiso, C., Moreno-Indias, I., de Hollanda, A., Martin-Nunez, G. M., Vidal, J., Tinahones, F. J. (2019). Gut microbiota specific signatures are related to the successful rate of bariatric surgery. Am. J. Transl. Res. 11 (2), 942–952.
Hajjar, R., Gonzalez, E., Fragoso, G., Oliero, M., Alaoui, A. A., Calve, A., et al. (2022). Gut microbiota influence anastomotic healing in colorectal cancer surgery through modulation of mucosal proinflammatory cytokines. Gut. doi: 10.1136/gutjnl-2022-328389
Hajjar, R., Oliero, M., Cuisiniere, T., Fragoso, G., Calve, A., Djediai, S., et al. (2021). Improvement of colonic healing and surgical recovery with perioperative supplementation of inulin and galacto-oligosaccharides. Clin. Nutr. 40 (6), 3842–3851. doi: 10.1016/j.clnu.2021.04.032
Hammer, H. F. (2012). Medical complications of bariatric surgery: Focus on malabsorption and dumping syndrome. Dig Dis. 30 (2), 182–186. doi: 10.1159/000336681
Hartman, A. L., Lough, D. M., Barupal, D. K., Fiehn, O., Fishbein, T., Zasloff, M., et al. (2009). Human gut microbiome adopts an alternative state following small bowel transplantation. Proc. Natl. Acad. Sci. U.S.A. 106 (40), 17187–17192. doi: 10.1073/pnas.0904847106
He, X., Lee, B., Jiang, Y. (2016). Cell-ECM interactions in tumor invasion. Adv. Exp. Med. Biol. 936, 73–91. doi: 10.1007/978-3-319-42023-3_4
Hooper, L. V., Wong, M. H., Thelin, A., Hansson, L., Falk, P. G., Gordon, J. I. (2001). Molecular analysis of commensal host-microbial relationships in the intestine. Science 291 (5505), 881–884. doi: 10.1126/science.291.5505.881
Horvat, M., Krebs, B., Potrc, S., Ivanecz, A., Kompan, L. (2010). Preoperative synbiotic bowel conditioning for elective colorectal surgery. Wien Klin Wochenschr 122 Suppl 2, 26–30. doi: 10.1007/s00508-010-1347-8
Huo, R. X., Wang, Y. J., Hou, S. B., Wang, W., Zhang, C. Z., Wan, X. H. (2022). Gut mucosal microbiota profiles linked to colorectal cancer recurrence. World J. Gastroenterol. 28 (18), 1946–1964. doi: 10.3748/wjg.v28.i18.1946
Hussan, H., Akinyeye, S., Mihaylova, M., McLaughlin, E., Chiang, C., Clinton, S. K., et al. (2022). Colorectal cancer risk is impacted by sex and type of surgery after bariatric surgery. Obes. Surg 32 (9), 2880–2890. doi: 10.1007/s11695-022-06155-0
Hyoju, S. K., Adriaansens, C., Wienholts, K., Sharma, A., Keskey, R., Arnold, W., et al. (2020). Low-fat/high-fibre diet prehabilitation improves anastomotic healing via the microbiome: An experimental model. Br. J. Surg. 107 (6), 743–755. doi: 10.1002/bjs.11388
Iida, N., Dzutsev, A., Stewart, C. A., Smith, L., Bouladoux, N., Weingarten, R. A., et al. (2013). Commensal bacteria control cancer response to therapy by modulating the tumor microenvironment. Science 342 (6161), 967–970. doi: 10.1126/science.1240527
Isles, N. S., Mu, A., Kwong, J. C., Howden, B. P., Stinear, T. P. (2022). Gut microbiome signatures and host colonization with multidrug-resistant bacteria. Trends Microbiol 30 (9), 853–865. doi: 10.1016/j.tim.2022.01.013
Jacobson, R. A., Wienholts, K., Williamson, A. J., Gaines, S., Hyoju, S., van Goor, H., et al. (2020). Enterococcus faecalis exploits the human fibrinolytic system to drive excess collagenolysis: Implications in gut healing and identification of druggable targets. Am. J. Physiol. Gastrointest Liver Physiol. 318 (1), G1–G9. doi: 10.1152/ajpgi.00236.2019
Jalanka, J., Salonen, A., Salojarvi, J., Ritari, J., Immonen, O., Marciani, L., et al. (2015). Effects of bowel cleansing on the intestinal microbiota. Gut 64 (10), 1562–1568. doi: 10.1136/gutjnl-2014-307240
Jeffery, M., Hickey, B. E., Hider, P. N. (2007) Follow-up strategies for patients treated for non-metastatic colorectal cancer. Cochrane Database Syst. Rev. 2007 (1), CD002200. doi: 10.1002/14651858.CD002200.pub2
Jia, W., Xie, G., Jia, W. (2018). Bile acid-microbiota crosstalk in gastrointestinal inflammation and carcinogenesis. Nat. Rev. Gastroenterol. Hepatol. 15 (2), 111–128. doi: 10.1038/nrgastro.2017.119
Jiao, Y., Wu, L., Huntington, N. D., Zhang, X. (2020). Crosstalk between gut microbiota and innate immunity and its implication in autoimmune diseases. Front. Immunol. 11, 282. doi: 10.3389/fimmu.2020.00282
Jin, Y., Geng, R., Liu, Y., Liu, L., Jin, X., Zhao, F., et al. (2020). Prediction of postoperative ileus in patients with colorectal cancer by preoperative gut microbiota. Front. Oncol. 10, 526009. doi: 10.3389/fonc.2020.526009
Jin, X., Liu, Y., Yan, W., Shi, S., Liu, L., Lin, B., et al. (2022). Gut microbiota from nCAL patients promotes colon anastomotic healing by inducing collagen synthesis in epithelial cells. J. Gastroenterol. Hepatol. 37 (9), 1756–1767. doi: 10.1111/jgh.15946
Jin, Y., Liu, Y., Zhao, L., Zhao, F., Feng, J., Li, S., et al. (2019). Gut microbiota in patients after surgical treatment for colorectal cancer. Environ. Microbiol. 21 (2), 772–783. doi: 10.1111/1462-2920.14498
Julien, C., Anakok, E., Treton, X., Nachury, M., Nancey, S., Buisson, A., et al. (2022). Impact of the ileal microbiota on surgical site infections in crohn's disease: A nationwide prospective cohort. J. Crohns Colitis 16 (8), 1211–1221. doi: 10.1093/ecco-jcc/jjac026
Kant, P., Perry, S. L., Dexter, S. P., Race, A. D., Loadman, P. M., Hull, M. A. (2014). Mucosal biomarkers of colorectal cancer risk do not increase at 6 months following sleeve gastrectomy, unlike gastric bypass. Obes. (Silver Spring) 22 (1), 202–210. doi: 10.1002/oby.20493
Kasiraj, A. C., Harmoinen, J., Isaiah, A., Westermarck, E., Steiner, J. M., Spillmann, T., et al. (2016). The effects of feeding and withholding food on the canine small intestinal microbiota. FEMS Microbiol. Ecol. 92 (6), fiw085. doi: 10.1093/femsec/fiw085
Keskey, R., Papazian, E., Lam, A., Toni, T., Hyoju, S., Thewissen, R., et al. (2022). Defining microbiome readiness for surgery: Dietary prehabilitation and stool biomarkers as predictive tools to improve outcome. Ann. Surg. 276 (5), e361–e369. doi: 10.1097/SLA.0000000000004578
Koliarakis, I., Athanasakis, E., Sgantzos, M., Mariolis-Sapsakos, T., Xynos, E., Chrysos, E., et al. (2020). Intestinal microbiota in colorectal cancer surgery. Cancers (Basel) 12 (10), 3011. doi: 10.3390/cancers12103011
Kolodziejczyk, A. A., Zheng, D., Elinav, E. (2019). Diet-microbiota interactions and personalized nutrition. Nat. Rev. Microbiol. 17 (12), 742–753. doi: 10.1038/s41579-019-0256-8
Komatsu, S., Sakamoto, E., Norimizu, S., Shingu, Y., Asahara, T., Nomoto, K., et al. (2016). Efficacy of perioperative synbiotics treatment for the prevention of surgical site infection after laparoscopic colorectal surgery: A randomized controlled trial. Surg. Today 46 (4), 479–490. doi: 10.1007/s00595-015-1178-3
Kotzampassi, K., Stavrou, G., Damoraki, G., Georgitsi, M., Basdanis, G., Tsaousi, G., et al. (2015). A four-probiotics regimen reduces postoperative complications after colorectal surgery: A randomized, double-blind, placebo-controlled study. World J. Surg. 39 (11), 2776–2783. doi: 10.1007/s00268-015-3071-z
Krebs, B., Horvat, M., Golle, A., Krznaric, Z., Papes, D., Augustin, G., et al. (2013). A randomized clinical trial of synbiotic treatment before colorectal cancer surgery. Am. Surg. 79 (12), E340–E342. doi: 10.1177/000313481307901202
Kurashima, Y., Kiyono, H. (2017). Mucosal ecological network of epithelium and immune cells for gut homeostasis and tissue healing. Annu. Rev. Immunol. 35, 119–147. doi: 10.1146/annurev-immunol-051116-052424
Ladebo, L., Pedersen, P. V., Pacyk, G. J., Kroustrup, J. P., Drewes, A. M., Brock, C., et al. (2021). Gastrointestinal pH, motility patterns, and transit times after roux-en-Y gastric bypass. Obes. Surg. 31 (6), 2632–2640. doi: 10.1007/s11695-021-05308-x
Levison, M. E. (1973). Effect of colon flora and short-chain fatty acids on growth in vitro of pseudomonas aeruginsoa and enterobacteriaceae. Infect. Immun. 8 (1), 30–35. doi: 10.1128/iai.8.1.30-35.1973
Li, J. V., Ashrafian, H., Sarafian, M., Homola, D., Rushton, L., Barker, G., et al. (2021). Roux-en-Y gastric bypass-induced bacterial perturbation contributes to altered host-bacterial co-metabolic phenotype. Microbiome 9 (1), 139. doi: 10.1186/s40168-021-01086-x
Li, H. L., Lu, L., Wang, X. S., Qin, L. Y., Wang, P., Qiu, S. P., et al. (2017). Alteration of gut microbiota and inflammatory Cytokine/Chemokine profiles in 5-fluorouracil induced intestinal mucositis. Front. Cell Infect. Microbiol. 7, 455. doi: 10.3389/fcimb.2017.00455
Lin, C., Cai, X., Zhang, J., Wang, W., Sheng, Q., Hua, H., et al. (2019). Role of gut microbiota in the development and treatment of colorectal cancer. Digestion 100 (1), 72–78. doi: 10.1159/000494052
Liou, A. P., Paziuk, M., Luevano, J. M., Jr., Machineni, S., Turnbaugh, P. J., Kaplan, L. M. (2013). Conserved shifts in the gut microbiota due to gastric bypass reduce host weight and adiposity. Sci. Transl. Med. 5 (178), 178ra41. doi: 10.1126/scitranslmed.3005687
Littman, D. R., Pamer, E. G. (2011). Role of the commensal microbiota in normal and pathogenic host immune responses. Cell Host Microbe 10 (4), 311–323. doi: 10.1016/j.chom.2011.10.004
Liu, Y., He, W., Yang, J., He, Y., Wang, Z., Li, K. (2021). The effects of preoperative intestinal dysbacteriosis on postoperative recovery in colorectal cancer surgery: A prospective cohort study. BMC Gastroenterol. 21 (1), 446. doi: 10.1186/s12876-021-02035-6
Liu, Z. H., Huang, M. J., Zhang, X. W., Wang, L., Huang, N. Q., Peng, H., et al. (2013). The effects of perioperative probiotic treatment on serum zonulin concentration and subsequent postoperative infectious complications after colorectal cancer surgery: A double-center and double-blind randomized clinical trial. Am. J. Clin. Nutr. 97 (1), 117–126. doi: 10.3945/ajcn.112.040949
Liu, Z., Qin, H., Yang, Z., Xia, Y., Liu, W., Yang, J., et al. (2011). Randomised clinical trial: the effects of perioperative probiotic treatment on barrier function and post-operative infectious complications in colorectal cancer surgery - a double-blind study. Aliment Pharmacol. Ther. 33 (1), 50–63. doi: 10.1111/j.1365-2036.2010.04492.x
Luijten, J., Vugts, G., Nieuwenhuijzen, G. A. P., Luyer, M. D. P. (2019). The importance of the microbiome in bariatric surgery: A systematic review. Obes. Surg. 29 (7), 2338–2349. doi: 10.1007/s11695-019-03863-y
Mackenzie, H., Markar, S. R., Askari, A., Faiz, O., Hull, M., Purkayastha, S., et al. (2018). Obesity surgery and risk of cancer. Br. J. Surg. 105 (12), 1650–1657. doi: 10.1002/bjs.10914
Mangell, P., Thorlacius, H., Syk, I., Ahrne, S., Molin, G., Olsson, C., et al. (2012). Lactobacillus plantarum 299v does not reduce enteric bacteria or bacterial translocation in patients undergoing colon resection. Dig Dis. Sci. 57 (7), 1915–1924. doi: 10.1007/s10620-012-2102-y
Martinez-Guryn, K., Leone, V., Chang, E. B. (2019). Regional diversity of the gastrointestinal microbiome. Cell Host Microbe 26 (3), 314–324. doi: 10.1016/j.chom.2019.08.011
Martin-Gallausiaux, C., Marinelli, L., Blottiere, H. M., Larraufie, P., Lapaque, N. (2021). SCFA: Mechanisms and functional importance in the gut. Proc. Nutr. Soc. 80 (1), 37–49. doi: 10.1017/S0029665120006916
Mathew, A. J., Wann, V. C., Abraham, D. T., Jacob, P. M., Selvan, B. S., Ramakrishna, B. S., et al. (2010). The effect of butyrate on the healing of colonic anastomoses in rats. J. Invest. Surg. 23 (2), 101–104. doi: 10.3109/08941930903469367
Merkow, R. P., Ju, M. H., Chung, J. W., Hall, B. L., Cohen, M. E., Williams, M. V., et al. (2015). Underlying reasons associated with hospital readmission following surgery in the united states. JAMA 313 (5), 483–495. doi: 10.1001/jama.2014.18614
Mizuta, M., Endo, I., Yamamoto, S., Inokawa, H., Kubo, M., Udaka, T., et al. (2016). Perioperative supplementation with bifidobacteria improves postoperative nutritional recovery, inflammatory response, and fecal microbiota in patients undergoing colorectal surgery: A prospective, randomized clinical trial. Biosci. Microbiota Food Health 35 (2), 77–87. doi: 10.12938/bmfh.2015-017
Monda, V., Villano, I., Messina, A., Valenzano, A., Esposito, T., Moscatelli, F., et al. (2017). Exercise modifies the gut microbiota with positive health effects. Oxid. Med. Cell Longev 2017, 3831972. doi: 10.1155/2017/3831972
Montassier, E., Gastinne, T., Vangay, P., Al-Ghalith, G. A., Bruley des Varannes, S., Massart, S., et al. (2015). Chemotherapy-driven dysbiosis in the intestinal microbiome. Aliment Pharmacol. Ther. 42 (5), 515–528. doi: 10.1111/apt.13302
Mowat, A. M., Agace, W. W. (2014). Regional specialization within the intestinal immune system. Nat. Rev. Immunol. 14 (10), 667–685. doi: 10.1038/nri3738
Nagata, N., Tohya, M., Fukuda, S., Suda, W., Nishijima, S., Takeuchi, F., et al. (2019). Effects of bowel preparation on the human gut microbiome and metabolome. Sci. Rep. 9 (1), 4042. doi: 10.1038/s41598-019-40182-9
Nalluri-Butz, H., Bobel, M. C., Nugent, J., Boatman, S., Emanuelson, R., Melton-Meaux, G., et al. (2022). A pilot study demonstrating the impact of surgical bowel preparation on intestinal microbiota composition following colon and rectal surgery. Sci. Rep. 12 (1), 10559. doi: 10.1038/s41598-022-14819-1
Nelson, R. L., Gladman, E., Barbateskovic, M. (2014) Antimicrobial prophylaxis for colorectal surgery. Cochrane Database Syst. Rev. 2014 (5), CD001181. doi: 10.1002/14651858.CD001181.pub4
Ohigashi, S., Sudo, K., Kobayashi, D., Takahashi, T., Nomoto, K., Onodera, H. (2013). Significant changes in the intestinal environment after surgery in patients with colorectal cancer. J. Gastrointest Surg. 17 (9), 1657–1664. doi: 10.1007/s11605-013-2270-x
Okada, M., Bothin, C., Kanazawa, K., Midtvedt, T. (1999). Experimental study of the influence of intestinal flora on the healing of intestinal anastomoses. Br. J. Surg. 86 (7), 961–965. doi: 10.1046/j.1365-2168.1999.01161.x
Olivas, A. D., Shogan, B. D., Valuckaite, V., Zaborin, A., Belogortseva, N., Musch, M., et al. (2012). Intestinal tissues induce an SNP mutation in pseudomonas aeruginosa that enhances its virulence: Possible role in anastomotic leak. PloS One 7 (8), e44326. doi: 10.1371/journal.pone.0044326
Park, I. J., Lee, J. H., Kye, B. H., Oh, H. K., Cho, Y. B., Kim, Y. T., et al. (2020). Effects of PrObiotics on the symptoms and surgical ouTComes after anterior REsection of colon cancer (POSTCARE): A randomized, double-blind, placebo-controlled trial. J. Clin. Med. 9 (7), 2181. doi: 10.3390/jcm9072181
Perez-Cobas, A. E., Gosalbes, M. J., Friedrichs, A., Knecht, H., Artacho, A., Eismann, K., et al. (2013). Gut microbiota disturbance during antibiotic therapy: A multi-omic approach. Gut 62 (11), 1591–1601. doi: 10.1136/gutjnl-2012-303184
Perler, B. K., Friedman, E. S., Wu, G. D. (2022). The role of the gut microbiota in the relationship between diet and human health. Annu. Rev. Physiol 85, 449–468. doi: 10.1146/annurev-physiol-031522-092054
Pohl, J. M., Gutweiler, S., Thiebes, S., Volke, J. K., Klein-Hitpass, L., Zwanziger, D., et al. (2017). Irf4-dependent CD103(+)CD11b(+) dendritic cells and the intestinal microbiome regulate monocyte and macrophage activation and intestinal peristalsis in postoperative ileus. Gut 66 (12), 2110–2120. doi: 10.1136/gutjnl-2017-313856
Polakowski, C. B., Kato, M., Preti, V. B., Schieferdecker, M. E. M., Ligocki Campos, A. C. (2019). Impact of the preoperative use of synbiotics in colorectal cancer patients: A prospective, randomized, double-blind, placebo-controlled study. Nutrition 58, 40–46. doi: 10.1016/j.nut.2018.06.004
Reddy, B. S., Macfie, J., Gatt, M., Larsen, C. N., Jensen, S. S., Leser, T. D. (2007). Randomized clinical trial of effect of synbiotics, neomycin and mechanical bowel preparation on intestinal barrier function in patients undergoing colectomy. Br. J. Surg. 94 (5), 546–554. doi: 10.1002/bjs.5705
Reis Ferreira, M., Andreyev, H. J. N., Mohammed, K., Truelove, L., Gowan, S. M., Li, J., et al. (2019). Microbiota- and radiotherapy-induced gastrointestinal side-effects (MARS) study: A Large pilot study of the microbiome in acute and late-radiation enteropathy. Clin. Cancer Res. 25 (21), 6487–6500. doi: 10.1158/1078-0432.CCR-19-0960
Rodriguez-Padilla, A., Morales-Martin, G., Perez-Quintero, R., Gomez-Salgado, J., Balongo-Garcia, R., Ruiz-Frutos, C. (2021). Postoperative ileus after stimulation with probiotics before ileostomy closure. Nutrients 13 (2), 626. doi: 10.3390/nu13020626
Rolandelli, R. H., Buckmire, M. A., Bernstein, K. A. (1997). Intravenous butyrate and healing of colonic anastomoses in the rat. Dis. Colon Rectum 40 (1), 67–70. doi: 10.1007/BF02055684
Sadahiro, S., Suzuki, T., Tanaka, A., Okada, K., Kamata, H., Ozaki, T., et al. (2014). Comparison between oral antibiotics and probiotics as bowel preparation for elective colon cancer surgery to prevent infection: Prospective randomized trial. Surgery 155 (3), 493–503. doi: 10.1016/j.surg.2013.06.002
Sajid, M. S., Siddiqui, M. R., Baig, M. K. (2012). Single layer versus double layer suture anastomosis of the gastrointestinal tract. Cochrane Database Syst. Rev. 1, CD005477. doi: 10.1002/14651858.CD005477.pub4
Salazar, N., Ponce-Alonso, M., Garriga, M., Sanchez-Carrillo, S., Hernandez-Barranco, A. M., Redruello, B., et al. (2022). Fecal metabolome and bacterial composition in severe obesity: Impact of diet and bariatric surgery. Gut Microbes 14 (1), 2106102. doi: 10.1080/19490976.2022.2106102
Sanchez-Alcoholado, L., Gutierrez-Repiso, C., Gomez-Perez, A. M., Garcia-Fuentes, E., Tinahones, F. J., Moreno-Indias, I. (2019). Gut microbiota adaptation after weight loss by roux-en-Y gastric bypass or sleeve gastrectomy bariatric surgeries. Surg. Obes. Relat. Dis. 15 (11), 1888–1895. doi: 10.1016/j.soard.2019.08.551
Schmitt, F. C. F., Schneider, M., Mathejczyk, W., Weigand, M. A., Figueiredo, J. C., Li, C. I., et al. (2021). Postoperative complications are associated with long-term changes in the gut microbiota following colorectal cancer surgery. Life (Basel) 11 (3), 246. doi: 10.3390/life11030246
Shi, S., Liu, Y., Wang, Z., Jin, X., Yan, W., Guo, X., et al. (2022). Fusobacterium nucleatum induces colon anastomosis leak by activating epithelial cells to express MMP9. Front. Microbiol. 13, 1031882. doi: 10.3389/fmicb.2022.1031882
Shin, S. Y., Hussain, Z., Lee, Y. J., Park, H. (2021). An altered composition of fecal microbiota, organic acids, and the effect of probiotics in the guinea pig model of postoperative ileus. Neurogastroenterol Motil. 33 (1), e13966. doi: 10.1111/nmo.13966
Shogan, B. D., Belogortseva, N., Luong, P. M., Zaborin, A., Lax, S., Bethel, C., et al. (2015). Collagen degradation and MMP9 activation by enterococcus faecalis contribute to intestinal anastomotic leak. Sci. Transl. Med. 7 (286), 286ra68. doi: 10.1126/scitranslmed.3010658
Shogan, B. D., Carlisle, E. M., Alverdy, J. C., Umanskiy, K. (2013). Do we really know why colorectal anastomoses leak? J. Gastrointest Surg. 17 (9), 1698–1707. doi: 10.1007/s11605-013-2227-0
Shogan, B. D., Smith, D. P., Christley, S., Gilbert, J. A., Zaborina, O., Alverdy, J. C. (2014). Intestinal anastomotic injury alters spatially defined microbiome composition and function. Microbiome 2, 35. doi: 10.1186/2049-2618-2-35
Sinclair, P., Brennan, D. J., le Roux, C. W. (2018). Gut adaptation after metabolic surgery and its influences on the brain, liver and cancer. Nat. Rev. Gastroenterol. Hepatol. 15 (10), 606–624. doi: 10.1038/s41575-018-0057-y
Steenackers, N., Vanuytsel, T., Augustijns, P., Tack, J., Mertens, A., Lannoo, M., et al. (2021). Adaptations in gastrointestinal physiology after sleeve gastrectomy and roux-en-Y gastric bypass. Lancet Gastroenterol. Hepatol. 6 (3), 225–237. doi: 10.1016/S2468-1253(20)30302-2
Tan, C. K., Said, S., Rajandram, R., Wang, Z., Roslani, A. C., Chin, K. F. (2016). Pre-surgical administration of microbial cell preparation in colorectal cancer patients: A randomized controlled trial. World J. Surg. 40 (8), 1985–1992. doi: 10.1007/s00268-016-3499-9
Tang, G., Huang, W., Tao, J., Wei, Z. (2022). Prophylactic effects of probiotics or synbiotics on postoperative ileus after gastrointestinal cancer surgery: A meta-analysis of randomized controlled trials. PloS One 17 (3), e0264759. doi: 10.1371/journal.pone.0264759
Tang, Y., Wu, Y., Huang, Z., Dong, W., Deng, Y., Wang, F., et al. (2017). Administration of probiotic mixture DM1 ameliorated 5-fluorouracil-induced intestinal mucositis and dysbiosis in rats. Nutrition 33, 96–104. doi: 10.1016/j.nut.2016.05.003
Tappenden, K. A. (2014). Intestinal adaptation following resection. JPEN J. Parenter Enteral Nutr. 38 (1 Suppl), 23S–31S. doi: 10.1177/0148607114525210
Tarazi, M., Jamel, S., Mullish, B. H., Markar, S. R., Hanna, G. B. (2022). Impact of gastrointestinal surgery upon the gut microbiome: A systematic review. Surgery 171 (5), 1331–1340. doi: 10.1016/j.surg.2021.10.014
Tonneau, M., Elkrief, A., Pasquier, D., Paz Del Socorro, T., Chamaillard, M., Bahig, H., et al. (2021). The role of the gut microbiome on radiation therapy efficacy and gastrointestinal complications: A systematic review. Radiother Oncol. 156, 1–9. doi: 10.1016/j.radonc.2020.10.033
Ubeda, C., Pamer, E. G. (2012). Antibiotics, microbiota, and immune defense. Trends Immunol. 33 (9), 459–466. doi: 10.1016/j.it.2012.05.003
Wang, F., Li, Q., He, Q., Geng, Y., Tang, C., Wang, C., et al. (2013). Temporal variations of the ileal microbiota in intestinal ischemia and reperfusion. Shock 39 (1), 96–103. doi: 10.1097/SHK.0b013e318279265f
Wang, C., Li, Q., Ren, J. (2019). Microbiota-immune interaction in the pathogenesis of gut-derived infection. Front. Immunol. 10, 1873. doi: 10.3389/fimmu.2019.01873
Wang, F., Li, Q., Wang, C., Tang, C., Li, J. (2012). Dynamic alteration of the colonic microbiota in intestinal ischemia-reperfusion injury. PloS One 7 (7), e42027. doi: 10.1371/journal.pone.0042027
Williamson, A. J., Alverdy, J. C. (2021). Influence of the microbiome on anastomotic leak. Clin. Colon Rectal Surg. 34 (6), 439–446. doi: 10.1055/s-0041-1735276
Wong, S. H., Yu, J. (2019). Gut microbiota in colorectal cancer: Mechanisms of action and clinical applications. Nat. Rev. Gastroenterol. Hepatol. 16 (11), 690–704. doi: 10.1038/s41575-019-0209-8
Wu, Y. J., Wu, C. T., Zhang, X. B., Ou, W. T., Huang, P. (2012). [Clinical study of different bowel preparations on changes of gut flora in patients undergoing colorectal resection]. Zhonghua Wei Chang Wai Ke Za Zhi 15 (6), 574–577.
Xie, H., Lu, Q., Wang, H., Zhu, X., Guan, Z. (2018). Effects of probiotics combined with enteral nutrition on immune function and inflammatory response in postoperative patients with gastric cancer. J. BUON 23 (3), 678–683.
Xu, Q., Xu, P., Cen, Y., Li, W. (2019). Effects of preoperative oral administration of glucose solution combined with postoperative probiotics on inflammation and intestinal barrier function in patients after colorectal cancer surgery. Oncol. Lett. 18 (1), 694–698. doi: 10.3892/ol.2019.10336
Yang, Z., Wu, Q., Liu, Y., Fan, D. (2017). Effect of perioperative probiotics and synbiotics on postoperative infections after gastrointestinal surgery: A systematic review with meta-analysis. JPEN J. Parenter Enteral Nutr. 41 (6), 1051–1062. doi: 10.1177/0148607116629670
Yang, Y., Xia, Y., Chen, H., Hong, L., Feng, J., Yang, J., et al. (2016). The effect of perioperative probiotics treatment for colorectal cancer: Short-term outcomes of a randomized controlled trial. Oncotarget 7 (7), 8432–8440. doi: 10.18632/oncotarget.7045
York, A. (2018). Microbiome: Gut microbiota sways response to cancer immunotherapy. Nat. Rev. Microbiol. 16 (3), 121. doi: 10.1038/nrmicro.2018.12
Yu, L. C., Shih, Y. A., Wu, L. L., Lin, Y. D., Kuo, W. T., Peng, W. H., et al. (2014). Enteric dysbiosis promotes antibiotic-resistant bacterial infection: Systemic dissemination of resistant and commensal bacteria through epithelial transcytosis. Am. J. Physiol. Gastrointest Liver Physiol. 307 (8), G824–G835. doi: 10.1152/ajpgi.00070.2014
Zaborin, A., Smith, D., Garfield, K., Quensen, J., Shakhsheer, B., Kade, M., et al. (2014). Membership and behavior of ultra-low-diversity pathogen communities present in the gut of humans during prolonged critical illness. mBio 5 (5), e01361–e01314. doi: 10.1128/mBio.01361-14
Zarrinpar, A., Chaix, A., Yooseph, S., Panda, S. (2014). Diet and feeding pattern affect the diurnal dynamics of the gut microbiome. Cell Metab. 20 (6), 1006–1017. doi: 10.1016/j.cmet.2014.11.008
Zhang, J. W., Du, P., Gao, J., Yang, B. R., Fang, W. J., Ying, C. M. (2012). Preoperative probiotics decrease postoperative infectious complications of colorectal cancer. Am. J. Med. Sci. 343 (3), 199–205. doi: 10.1097/MAJ.0b013e31823aace6
Zhao, R., Wang, Y., Huang, Y., Cui, Y., Xia, L., Rao, Z., et al. (2017). Effects of fiber and probiotics on diarrhea associated with enteral nutrition in gastric cancer patients: A prospective randomized and controlled trial. Med. (Baltimore) 96 (43), e8418. doi: 10.1097/MD.0000000000008418
Zhao, T. S., Xie, L. W., Cai, S., Xu, J. Y., Zhou, H., Tang, L. F., et al. (2021). Dysbiosis of gut microbiota is associated with the progression of radiation-induced intestinal injury and is alleviated by oral compound probiotics in mouse model. Front. Cell Infect. Microbiol. 11, 717636. doi: 10.3389/fcimb.2021.717636
Keywords: gut microbiota, colorectal cancer, recovery, gastrointestinal surgery, postoperative complications
Citation: Zheng Z, Hu Y, Tang J, Xu W, Zhu W and Zhang W (2023) The implication of gut microbiota in recovery from gastrointestinal surgery. Front. Cell. Infect. Microbiol. 13:1110787. doi: 10.3389/fcimb.2023.1110787
Received: 29 November 2022; Accepted: 15 February 2023;
Published: 28 February 2023.
Edited by:
Yugen Chen, Nanjing University of Chinese Medicine, ChinaReviewed by:
Olga Y. Zaborina, The University of Chicago, United StatesCopyright © 2023 Zheng, Hu, Tang, Xu, Zhu and Zhang. This is an open-access article distributed under the terms of the Creative Commons Attribution License (CC BY). The use, distribution or reproduction in other forums is permitted, provided the original author(s) and the copyright owner(s) are credited and that the original publication in this journal is cited, in accordance with accepted academic practice. No use, distribution or reproduction is permitted which does not comply with these terms.
*Correspondence: Wei Zhang, emhhbmd3ZWlsczE5NjhAMTYzLmNvbQ==
Disclaimer: All claims expressed in this article are solely those of the authors and do not necessarily represent those of their affiliated organizations, or those of the publisher, the editors and the reviewers. Any product that may be evaluated in this article or claim that may be made by its manufacturer is not guaranteed or endorsed by the publisher.
Research integrity at Frontiers
Learn more about the work of our research integrity team to safeguard the quality of each article we publish.