- 1College of Plant Protection, Yangzhou University, Yangzhou, Jiangsu, China
- 2The Key Laboratory of Biotechnology for Medicinal Plants of Jiangsu Province, School of Life Science, Jiangsu Normal University, Xuzhou, Jiangsu, China
- 3Hainan Yazhou Bay Seed Laboratory, Sanya Nanfan Research Institute of Hainan University, Sanya, China
B vitamins are essential micro-organic compounds for the development of humans and animals. Vitamin B6 comprises a group of components including pyridoxine, pyridoxal, and pyridoxamine. In addition, vitamin B6 acts as the coenzymes in amino acid biosynthesis, decarboxylation, racemic reactions, and other biological processes. In this study, we found that the expressions of a gene encoding pyridoxine biosynthesis protein (PDX1) were significantly upregulated in the early infectious stages in M. oryzae. Furthermore, deletion of MoPDX1 slowed vegetative growth on different media, especially on MM media, and the growth defect was rescued when MoPdx1-protein was expressed in mutants strains and when commercial VB6 (pyridoxine) was added exogenously. However, VB6 content in different strains cultured in CM media has no significant difference, suggested that MoPdx1 was involved in de novo VB6 biosynthesis not in uptake process, and VB6 regulates the vegetative growth of M. oryzae. The ΔMopdx1 mutants presented abnormal appressorium turgor, slowed invasive growth and reduced virulence on rice seedlings and sheath cells. MoPdx1 was located in the cytoplasm and present in spore and germ tubes at 14 hours post inoculation (hpi) and then transferred into the appressorium at 24 hpi. Addition of VB6 in the conidial suspentions could rescue the defects of appressorium turgor pressure at 14 hpi or 24 hpi, invasive growth and pathogenicity of the MoPDX1 deletion mutants. Indicated that MoPdx1 affected the appressorium turgor pressure, invasive growth and virulence mainly depended on de novo VB6, and VB6 was biosynthesized in conidia, then transported into the appressorium, which play important roles in substances transportation from conidia to appressorium thus to regulate the appressorium turgor pressure. However, deletion of MoPDX1 did not affect the ability that scavenge ROS produced by rice cells, and the mutant strains were unable to activate host defense responses. In addition, co-immunoprecipitation (Co-IP) assays investigating potential MoPdx1-interacting proteins suggested that MoPdx1 might take part in multiple pathways, especially in the ribosome and in biosynthesis of some substances. These results indicate that vitamins are involved in the development and pathogenicity of M. oryzae.
Introduction
As one of the world’s leading food crops, rice (Oryza sativa) has long been affected by rice diseases, that can result in large-scale production reduction. Rice blast is one of the most significant and devastating diseases in rice production, causing losses of 10% to 30% every year (Skamnioti and Gurr, 2009; Sakulkoo et al., 2018). Due to the rapid variation of field strains of the disease, resistant varieties of rice lose their resistance quickly after a few years of planting. Meanwhile, large-scale pesticide use readily causes environmental pollution and residues. Therefore, analyzing the pathogenic mechanism of the rice blast fungus Magnaporthe oryzae could provide candidate targets for developing new fungicides and offer new strategies for preventing and controlling rice blast.
The infection cycle of M. oryzae was composed of four diverse stages. Initially, conidia sense hydrophobic surface signals and adhere to the host surface by secreting spore tip mucilage (Wilson and Talbot, 2009). The second stage is spore germination and development of the germ tube, then forms the appressorium. The third stage is formation of penetration peg. With the maturation of the appressorium, enormous turgor pressure, approximately 8~10 MPa, develops, providing a massive mechanical force to penetrate the host cuticle and enter the host cells (Howard et al., 1991; Foster et al., 2017). Finally, infectious hyphae disrupt the nutrients and water of the host and secret effectors to suppress plant immunity (Giraldo et al., 2013). Further, they spread into the neighboring host cells, and eventually, the host cells die (Jia et al., 2016; Gupta et al., 2021).
B vitamins are essential micro-organic compounds for the development of humans and animals, and consist of 8 kinds of water-soluble complex vitamins, including vitamins B1, B2, B3, B5, B6, B7, B9, and B12 (Kennedy, 2016; Calderón-Ospina and Nava-Mesa, 2020). All of the B vitamins act synergistically to regulate metabolism, enhance functions of the nervous system and immune systems, and promote cell growth and division (Kennedy, 2016; Wendołowicz et al., 2018). Among them, VB6 comprises a group of compounds including pyridoxine, pyridoxal, and pyridoxamine. Pyridoxamine is the aminated product of pyridoxine, and pyridoxal is the formyl derivative of pyridoxine. Pyridoxal, pyridoxine, and pyridoxamine have equal activities in animals. In general, pyridoxal, pyridoxine, and pyridoxamine are converted into the enzymatically active forming pyridoxal-5-phosphate (PLP), pyridoxine-5-phosphate (PNP), and pyridoxamine-5-phosphate (PMP), respectively, which carry out their vitamin activities (Rosenberg, 2012). More than 160 enzymes with different catalytic activities have been identified that require VB6 (particularly PLP) as coenzymes to function (approximately 4% of all catalytic activity) (Percudani and Peracchi, 2009); PLP-dependent enzymes take part in amino acid biosynthesis, decarboxylation, and racemic reactions (Eliot and Kirsch, 2004). PLP regulates the activity of the transcription factor PdxR, and feedback regulates the biosynthesis of VB6 in Streptococcus pneumonia (El Qaidi et al., 2013). Together, these studies suggest that VB6 plays multiple functions in different cellular processes.
VB6 can be synthesized by plants, fungi, bacteria, archaea, and protists. However, animals and some obligate pathogens require it only from foods (Eliot and Kirsch, 2004; Phillips, 2015). In Arabidopsis, deletion of pyridoxine biosynthesis protein (de novo vitamin B6 biosynthesis protein, PDX1) leads to abnormal development of the roots and seedlings, and the mutants were more sensitive to osmotic stress and oxidative stress, suggesting that VB6 might be an antioxidant (Chen and Xiong, 2005). In tomatoes, SlPdx1.2 and SlPdx1.3 regulate the antioxidant capacity of cells, thus cofferring resistantance to Botrytis cinerea infection (Zhang et al., 2014). In rice, pyridoxal phosphate synthase OsPdx1 positively regulated rice immunity to Xanthomonas oryzae pv. oryzicola (Xoc), inducing stomatal closure by promoting the biosynthesis of abscisic acid (ABA), which is targeted and degraded by the effector protein AvrRxo1 of Xoc (Liu et al., 2022). In fungi, the functions of Pdx1 have only been reported for Cercospora nicotianae, one of plant pathogens. Cercosporin, the main pathogenic factor generated by C. nicotianae, could absorb light and interact with oxygen to produce superoxide ions (), hydrogen peroxide (H2O2), highly destructive singlet oxygen (1O2), and other substances to promote host infection. In addition to function in the biosynthesis of VB6, Pdx1 of C. nicotianae is also a 1O2 resistance proteins involved in the resistance process of cercosporin and other photosensitizers, and in protecting itself from reactive oxygen species (ROS) (Ehrenshaft et al., 1999). Furthermore, Pdx1 was found to be located in vesicles of C. nicotianae (Chung et al., 2002). However, the molecular mechanisms of Pdx1 in its antioxidant capacity and vesicle localization are still unknown. In addition, there are no known functions of Pdx1 in the virulence of plant pathogens.
In this work, the expression of PDX1 was significantly upregulated at the early infectious stages compared with the hyphal stage in M. oryzae. Furthermore, the MoPDX1 was deleted, and it was found that the vegetative growth of mutants was significantly slower in MM media (uncomplete media) than in CM and SDC media, and exogenous VB6 (pyridoxine) could rescue the growth defects. We found that MoPdx1 was involved in the pathogenicity of M. oryzae mediating de novo biosynthesis of VB6. Besides that, a Co-IP assay was carried out to identify its potential interacting protein and thereby investigate the functions of Pdx1 in M. oryzae.
Materials and methods
Strains and culture conditions
The strain Guy11 (isolated from Guyana, which has relatively higher virulent) was used as a wild-type in this work. The Guy11, mutants and complementary strains were cultured at 28°C in CM, MM, and SDC media for 7 days, respectively. Fungal mycelia were harvested after growth in liquid CM media with shaking for 48 h at 28°C and were used for DNA and RNA extraction. Fungal protoplasts were prepared and transformed as described previously (Sweigard et al., 1992). Transformants were selected on TB3 media with hygromycin B or zeocin (Yang et al., 2019). For conidiation statistics, mycelial blocks were cultured on SDC media for 7 days in the dark and then moved to constant illumination under fluorescent light for 3 days (Zhang et al., 2009).
Target gene deletion and complementary strains obtained in M. oryzae
The approximately 1.0 kb up- and downstream- sequences of MoPDX1 (MGG_05980) were separately amplified from M. oryzae genomic DNA with the primers shown in Table S1. They were ligated to the pCX62 vectors in two steps to generate the MoPDX1 deletion vectors, which were confirmed by sequencing. Then, the deletion vectors were transformed into the Guy11 protoplasts, which were selected on TB3 media supplemented with hygromycin B. Candidate transformants were verified with inner and outer primers (in Table S1) of MoPDX1 through PCR and further verified by Southern blot analysis (Figure S1). For complementary strains, fragments including the full-length coding region without the termination code and approximately 1.5 kb native promoters were cloned into the pYF11 vectors, which express green fluorescence protein (GFP), and sequenced. Then, the complementary vectors were transformed into the MoPDX1 deletion mutants and selected by zeocin-resistance. Candidate strains were verified by GFP screening and Western blot assays.
Vitamin supplementation assay
To test the function of vitamins in the development of M. oryzae, hyphal blocks of Guy11, two ΔMopdx1 mutants, and complementary strains were cultured on MM media supplemented with 10-4 mg/mL of VB1 (Vitamin B1, Cat#V8020, Solarbio), VB2 (Riboflavin, Cat#V8060, Solarbio), VB3 (Nicotinic acid, Cat#N8060, Solarbio), VB6 (Pyridoxine HCl, Cat#V8030, Solarbio), VH (D-biotin, Lot F801BA0013, Sangon Biotech, Shanghai, China), or PABA(4-aminobenzoic acid, Lot F906BA0033, Sangon Biotech, Shanghai, China) alone or lacking only VB1, VB3, and VB6, at 28°C for 7 days in the dark. The hyphal blocks of the indicated strains cultured on MM media were used as controls. For the invasive growth complementation assay, 10-4 mg/mL of VB6 were added in the conidial suspensions of the MoPDX1 deletion mutants, then injected into the 21-d rice sheath cells, results were observed at 24 and 48 hours post inoculation (hpi), respectively. For the virulence complementation assay, 25 μL of conidial suspensions (5×104 spores/mL) of mutants supplemented with 10-4 mg/mL VB6 were inoculated on the surface of 7-day barely leaves. For the appressorium turgor complementation assay, 10-4 mg/mL of VB6 were added in the conidial suspensions of indicated strains, 25 μL of them were cultured on the hydrophobic slides (Fisherbrand, 12540A, Germany), different concentrations of 1-4 M glycerol were used to treat the appressoria for 3 min to detect the their incipient cytorrhysis. At least 100 appressoria were counted at 14 and 24 hpi for each experiments, respectively.
Intracellular VB6 measurements
The indicated strains were reactivated from PDA media and cultured on CM media at 28°C for 5 d in the dark. Then, the samples were shaken in liquid CM media for 48 h, and the mycelium pellets were collected and quickly ground into powders in liquid nitrogen. The material extraction method was described in the previous study (Liu et al., 2016a). Intracellular VB6 in different samples were detected by HPLC-MS (Agilent 6460, USA) analysis. The steps were as follows: at 0~5 min, the C18 column (2.1× 50 mm, 2.7 μm; Agilent, USA) was rinsed with 100% water (pH 3.0-4.0) containing 5 mM ammonium acetate; at 6~7 min, the C18 column was rinsed with 100% acetonitrile; and at 8~15 min, the C18 column was rinsed again with 100% water (pH 3.0-4.0) containing 5 mM ammonium acetate. The flow rate was 0.2 mL per minute, and the temperature of the C18 column was 35°C. The peak flow of the VB6 standard was at approximately1.25 min. The intracellular VB6 content in different samples was calculated according to a previously described method (Liu et al., 2016a).
Appressorial formation, turgor pressure, and rice infection assays
For appressorial formation, spores of the indicated strains were collected with a double filter cloth, and 20 μL of a 5×104 spores/mL suspensions were dripped onto hydrophobic slides. At least 100 appressoria were counted at 24 hpi. For turgor pressure, 1-4 M glycerol was used to treat the appressoria for 3 min at 14 and 24 hpi, and at least 100 appressoria were counted to observe their collapse rate. For rice spray assays, 5×104 spores/mL conidia suspensions of the indicated strains were sprayed onto the surface of 11-d rice CO-39 (a susceptible variety to rice blast) seedlings. After incubation in a moist chamber at 28°C for 24 h in the dark, the samples were transferred into another moist chamber at 28°C for 6 days under continuous12 h/12 h light and dark conditions. For rice leaf sheath injection assays, 15×104 spores/mL conidial suspensions were injected into 21-d rice leaf sheaths, and the conditions of disease occurrence were consistent with those of the rice spray assay. The experiments were repeated three times with the same results.
Quantitative reverse transcription PCR (qRT–PCR) analysis
For expression analysis assays of MoPDX1 at different stages of M. oryzae, hyphal blocks of Guy11 strains were cultured in liquid CM media for 48 h at 28°C, conidia of Guy11 were collected in SDC media, 5×104 spores/mL conidial suspensions of Guy11 were sprayed onto 11-d rice seedlings. Samples were collected at 8, 24, 48 and 72 hpi, respectively. RNA was extracted from all samples using an RNA extraction kit (Invitrogen, USA). The concentration of total RNA was measured and then reverse transcribed (R223-01, Vazyme). Quantitative RT–PCR (qRT–PCR) was performed on a Bio-Rad CFX96 instrument (Bio-Rad, USA) according to the manufacturer’s instructions. The expression of MoPDX1 at the mycelium stage (MY) was set at 1.0. To evaluate the expression level of PR genes, 5×104 spores/mL conidial suspensions of Guy11 and mutant strains were singly sprayed onto 11-d rice seedlings, and samples were collected at 48 hpi. Sterile water sprays were used as negative controls. The stable-expression rice gene EF1-α (primers in Table S1) was set as an internal control. All experiments were performed for three independent replicates with the same results.
Co-inmunoprecipitation assays
Hyphal blocks of complementary strains were cultured in liquid CM media at 28°C for 48 h, filtered, and ground into powders in liquid nitrogen as soon as possible. Powders were lysed with 1 mL of protein lysis buffer [10 mM Tris/Cl(pH7.5); 150 mM NaCl; 0.5 mM EDTA; 0.5% NP-40] and 100 mM protease inhibitor PMSF (Cat No. ST506, Beyotime), shaken every 10 min, and then centrifuged at 1,2000 rpm at 4°C for 20 min, the supernatant was used as the total protein. Total protein was co-incubated with commercial GFP beads (Lot 91009001A-04, Ychromotek) at 4°C overnight. Then, the beads were centrifuged at 500 rpm at 4°C for 2 min and washed five times with washing buffer [10 mM Tris/Cl(pH7.5); 150 mM NaCl; 0.5 mM EDTA]. The proteins were eluted within the elution buffer (200 mM glycine, pH2.5), which were neutralized with 1M Tris-base (pH10.4). The candidate interacting proteins of MoPdx1 were further analyzed through mass spectrography (the instrument was LTQ Orbitrap Velos) at Shenzhen BGI Co., LTD, China. The single GFP interaction proteins were used as controls.
Protein identification analysis
Identified peptides were blasted in the protein database of M. oryzae (https://fungidb.org/fungidb/showQuestion.do?questionFullName=UniversalQuestions.UnifiedBlast). The proteins were analyzed in the GO (Gene Ontology), KOG (Eukaryotic orthologous groups), and KEGG (Kyoto Encyclopedia of Genes and Genomes) pathway databases, respectively.
Statistical analysis
One-way ANOVA analysis with IPM SPSS Statistics Software was used to conduct variance analysis. LSD test was used to make multiple comparisons, the mean difference is significant at the 0.05 level in the whole text. Student-Newman-Keuls test was used to display the means for groups in homogeneous subsets. Three replicates were set for each sample, and all experiments were performed for three independent replicates with the same results.
Results
Transcript analysis of MoPDX1 at different stages of M. oryzae
We identified a gene MoPDX1 encoded a pyridoxine biosynthesis protein in M. oryzae. The transcript level of the gene was analyzed at different stages, such as the mycelium stage (MY), conidial stage (CO), and infectious stage at 8, 24, 48, and 72 hpi. Results showed that the gene MoPDX1 was significantly upregulated at the early infection stage, approximately 2.4 folds, 3.8 folds, and 1.7 folds at 8, 24, and 48 hpi compared with that of at the mycelium stage, respectively. However, there were no significant differences at the conidial stage and the infectious stage at 72 hpi (Figure 1). Suggesting that MoPdx1 might play important roles in the early stage of infection of M. oryzae.
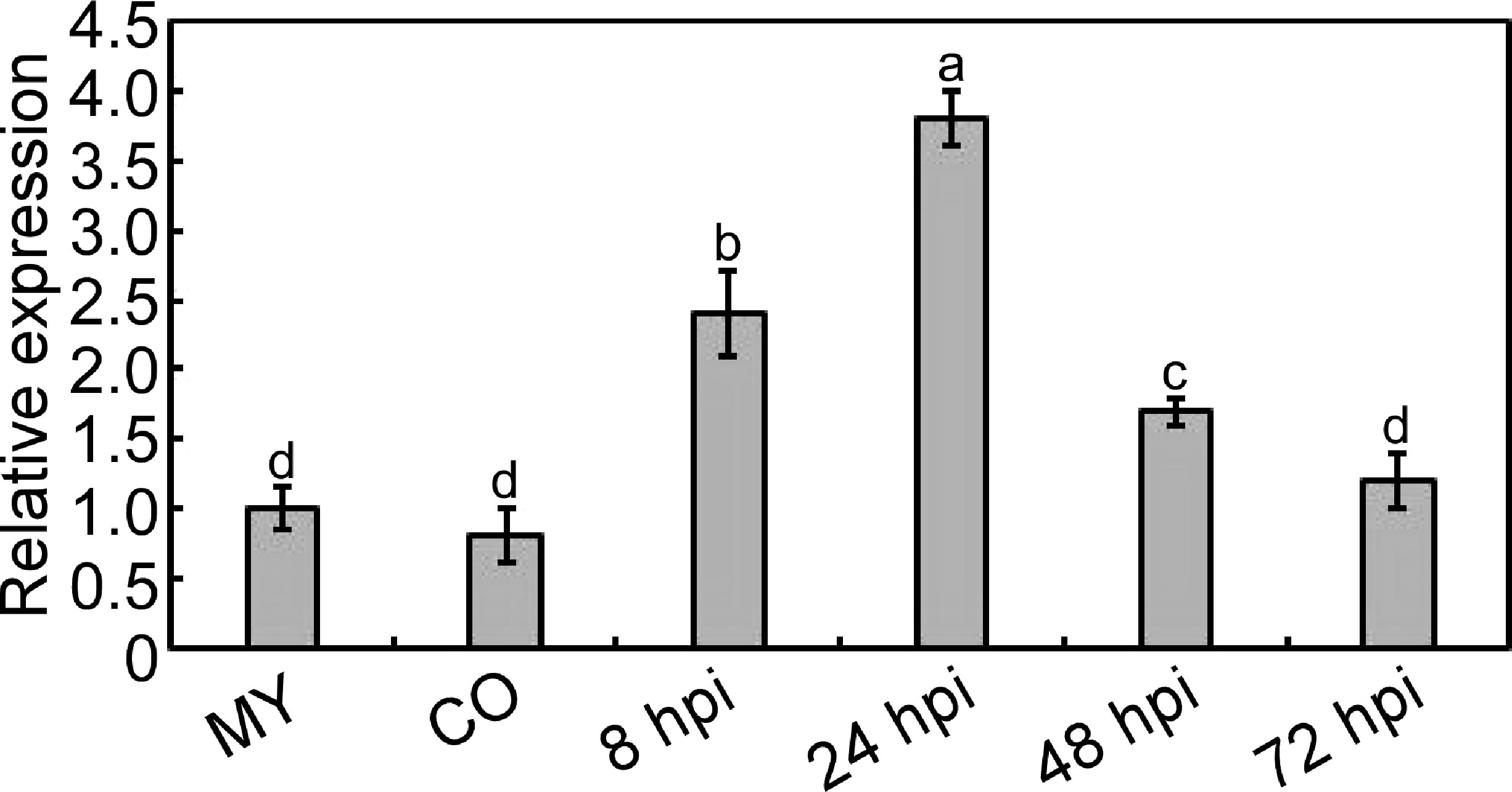
Figure 1 Transcription analysis of MoPDX1 at different stages of M. oryzae. Total RNA was extracted from the mycelium (MY) stages, conidia (CO) stages, and infectious stages at 8, 24, 48, and 72 hours post inoculation (hpi), respectively. The RNA was reverse transcribed and then quantified by q-RT PCR. The transcript level of MoPDX1 at the mycelium stage was set at 1.0. Experiments were repeated three times with similar results. Error bars represent the standard deviation. Lowercase represent significant differences (LSD and Student-Newman-Keuls test, p<0.05).
MoPdx1 regulates vegetative growth via de novo VB6 biosynthesis in M. oryzae
To further explore the function of MoPdx1 in M. oryzae, the MoPDX1 gene was replaced with the hygromycin (HPH) with the one-step method. Southern blotting confirmed that two transforments were successfully knocked out and that only one copy of HPH was inserted into the genome of M. oryzae (Figure S1). Furthermore, complementary strains were acquired. Vegetative growth of wild-type Guy11, two MoPDX1 deletion mutants, and complementary strains on CM, MM, and SDC media were measured. The results showed that the vegetative growth of the MoPDX1 mutants on the three kinds of media all significantly slowed compared to that of Guy11 and complementary strains, especially on MM media, on which the mutants nearly stopped growing (Figures 2A, B). Given that MoPdx1 encoding a protein involved in the biosynthesis of pyridoxine, one of VB6 compounds, and there are only basic trace substances in MM media, we want to determine whether vegetative growth defects of the MoPDX1 mutants could be mitigated by the addition of various vitamin B elements, including VB1, VB2, VB3, VB6, VH, and PABA, which are components of CM media. The results showed that only the exogenous addition of VB6 could entirely rescue the growth defects of two MoPDX1 deletion mutants, while addition of VB1, VB2, VB3, VH, and PABA individually or together could not (Figure 3 and Table 1). Moreover, the VB6 content in different strains in CM media was measured with HPLC-MS and it was found that there was no significant difference between the WT and MoPDX1 deletion mutants (Figure S2 and Table S2). Indicating that MoPdx1 regulates the vegetative growth via de novo VB6 biosynthesis not in uptake process in M. oryzae.
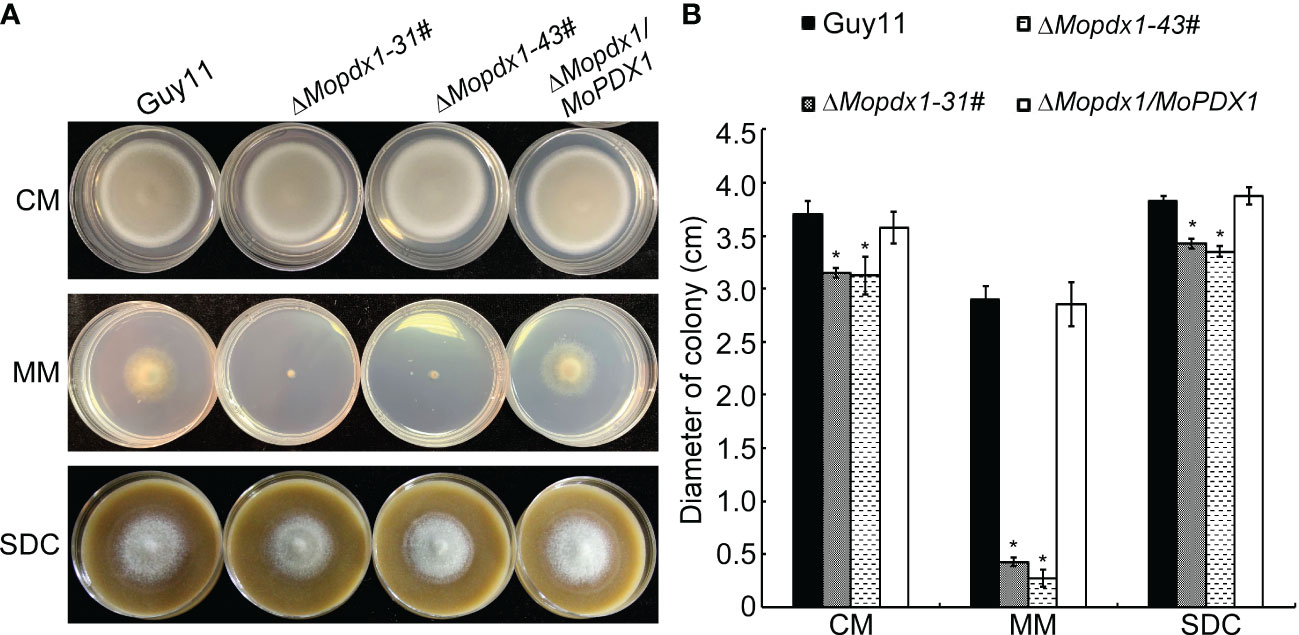
Figure 2 Deletion of MoPDX1 affects the vegetative growth of M. oryzae. (A) Colony morphology of Guy11, two MoPDX1 deletion mutants, and complementary strains after growth at 28°C on CM, MM, and SDC media for 7 days. (B) Colony diameters of indicated strains on three kinds of media after 7 days post inoculation (dpi) at 28°C. Experiments were repeated three times with similar results. Error bars represent the standard deviation. Asterisks represent significant differences (LSD and Student-Newman-Keuls test, p<0.05).
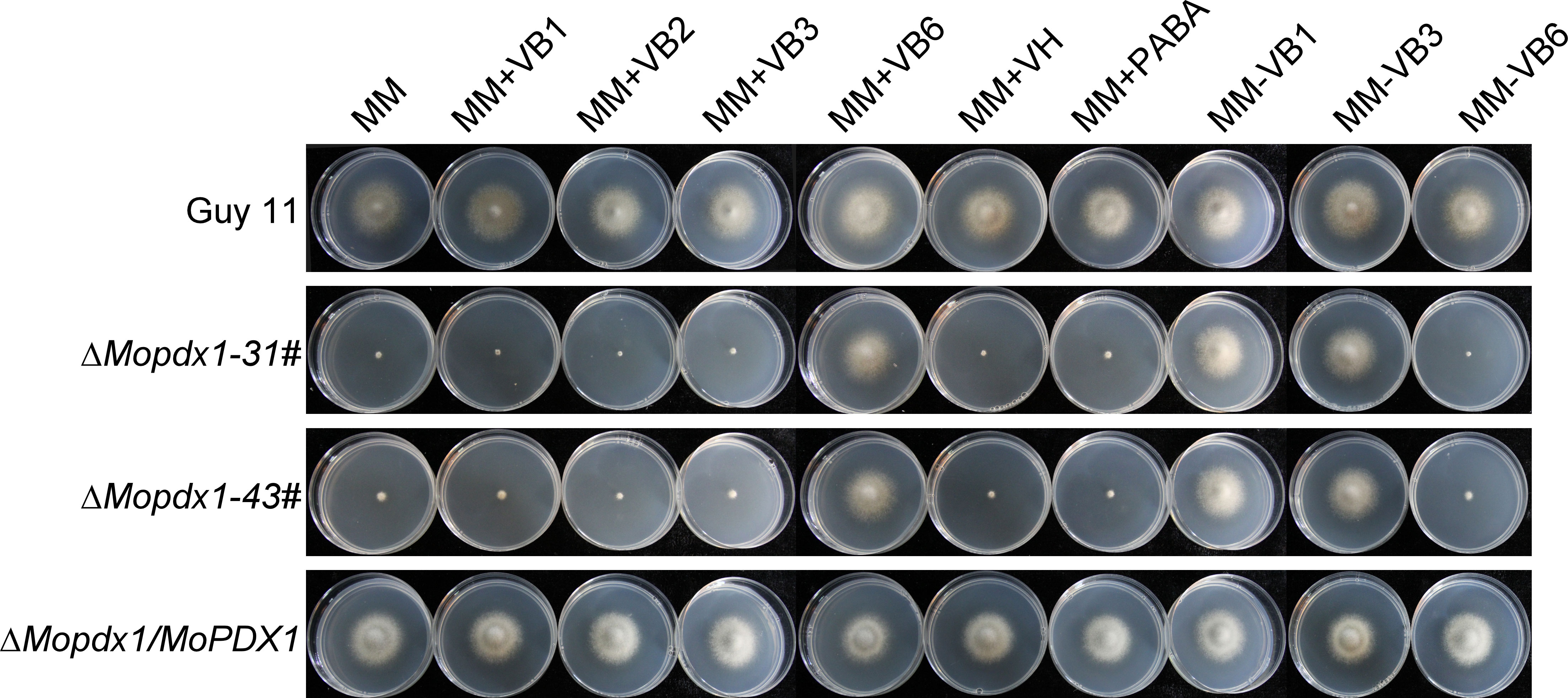
Figure 3 The addition of VB6 but not other kinds of vitamin B rescued the defect in vegetative growth of MoPDX1 deletion mutants. Hyphal blocks of indicated strains were cultured at 28°C for 7 days in the dark on MM media erther individually supplemented with VB1, VB2, VB3, VB6, VH, or PABA, or only lacking VB1, VB3, and VB6. Experiments were repeated three times with similar results.
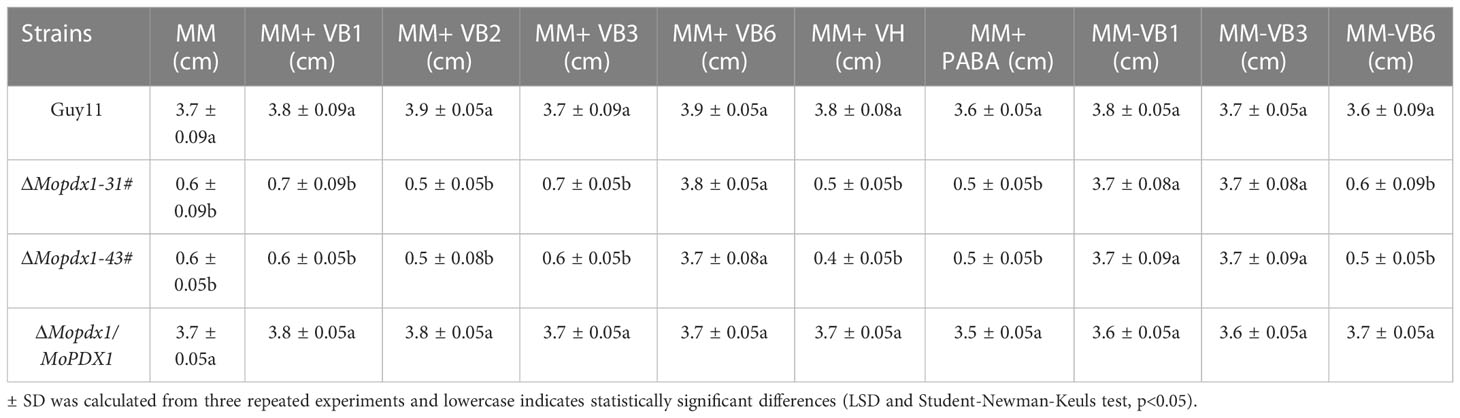
Table 1 Statistical analysis of the wild type, ΔMopdx1 mutants, and the complement strains on MM media with different types of vitamin B.
Deletion of MoPDX1 affects the appressorium turgor pressure of M. oryzae
We further evaluated conidiation, conidial germination, appressorium formation, and turgor pressure in Guy11, two MoPDX1 deletion mutants, and their complementary strains. The results showed that there was no significant difference in conidiation, conidial germination, or appressorium formation between Guy11 and the deletion mutants (Table 2). However, appressorium collapse rate in the two MoPDX1 deletion mutants rose dramatically at 2-4 M glycerol compared with that of in Guy11 and complementary strains, approximately 64.8% under the treatment of 2 M glycerol, 84.2% under the treatment of 3 M glycerol and 91.9% under the treatment of 4 M glycerol; while it was significantly reduced in the deletion mutants at 1 M glycerol (Table 2). The results indicate that the deletion of MoPDX1 affects the appressorium turgor pressure of M. oryzae.
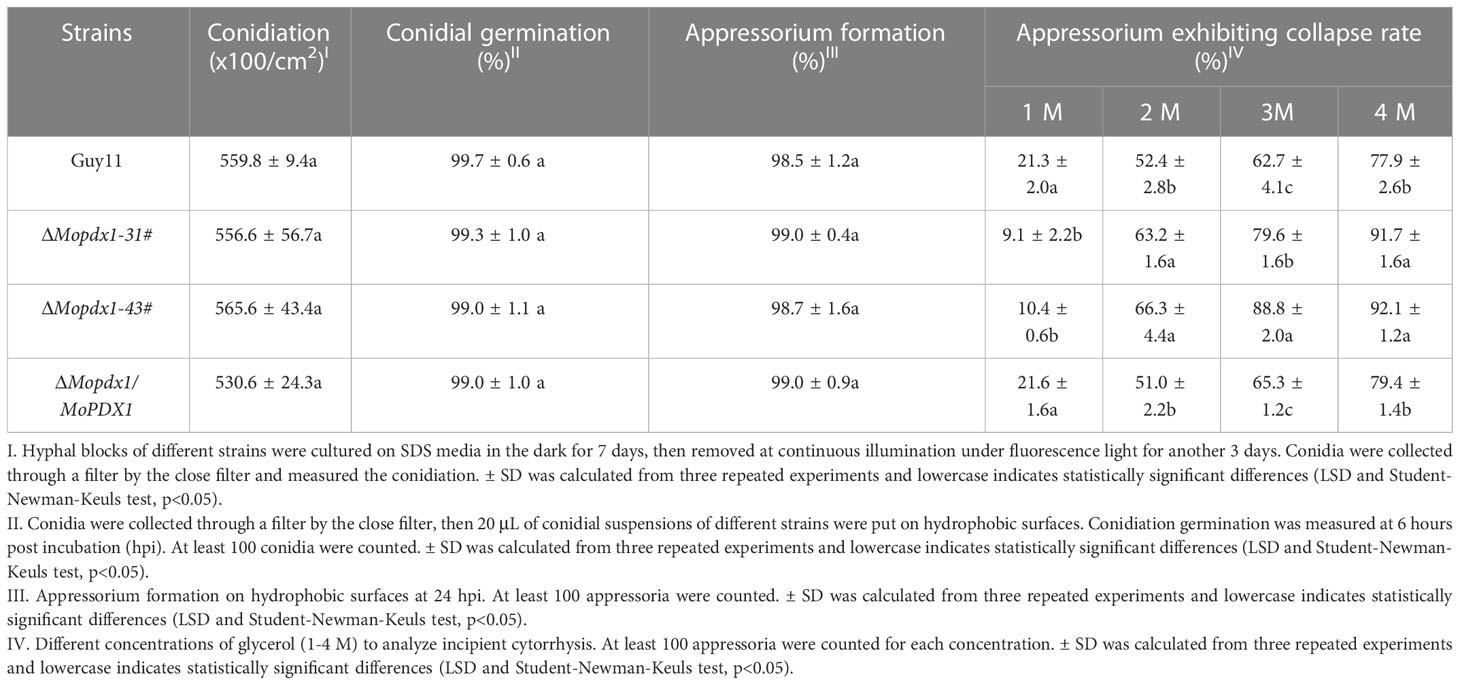
Table 2 Conidiation, conidial germination, appressorium formation, and turgor pressure assays of the wild type, ΔMopdx1 mutant, and the complement strains.
MoPdx1 is involved in the pathogenicity of M. oryzae
The appressorium is an important structure of M. oryzae which produces penetrating pegs that successfully penetrate into the host cell. Deletion of MoPDX1 affected the appressorium turgor of M. oryzae; therefore, virulence assays were performed. The 5×104 spores/mL of conidial suspensions of Guy11, two MoPDX1 deletion mutants and complementary strains were sprayed on 11-d rice CO-39 seedlings, and disease symptoms were observed at 7 days post inoculation (dpi). The two MoPDX1 deletion mutants presented the most restricted lessons and bits of typical lesions of rice blast compared to that caused by Guy11 and complementary strains (Figures 4A, C). In addition, lesion biomass assays of all the diseased leaves suggested that the content of M. oryzae was markedly reduced treated with the two ΔMopdx1 mutants (Figure 4D). Moreover, conidial suspensions (15×104 spores/mL) of the different strains was injected into 21-d rice sheath cells and observed at 5 dpi. Lesion incidence was in keeping with that of rice spraying, with lesions reduced by two-thirds in the two ΔMopdx1 mutants (Figure 4B). Overall, the results suggested that MoPdx1 is involved in the pathogenicity of M. oryzae.
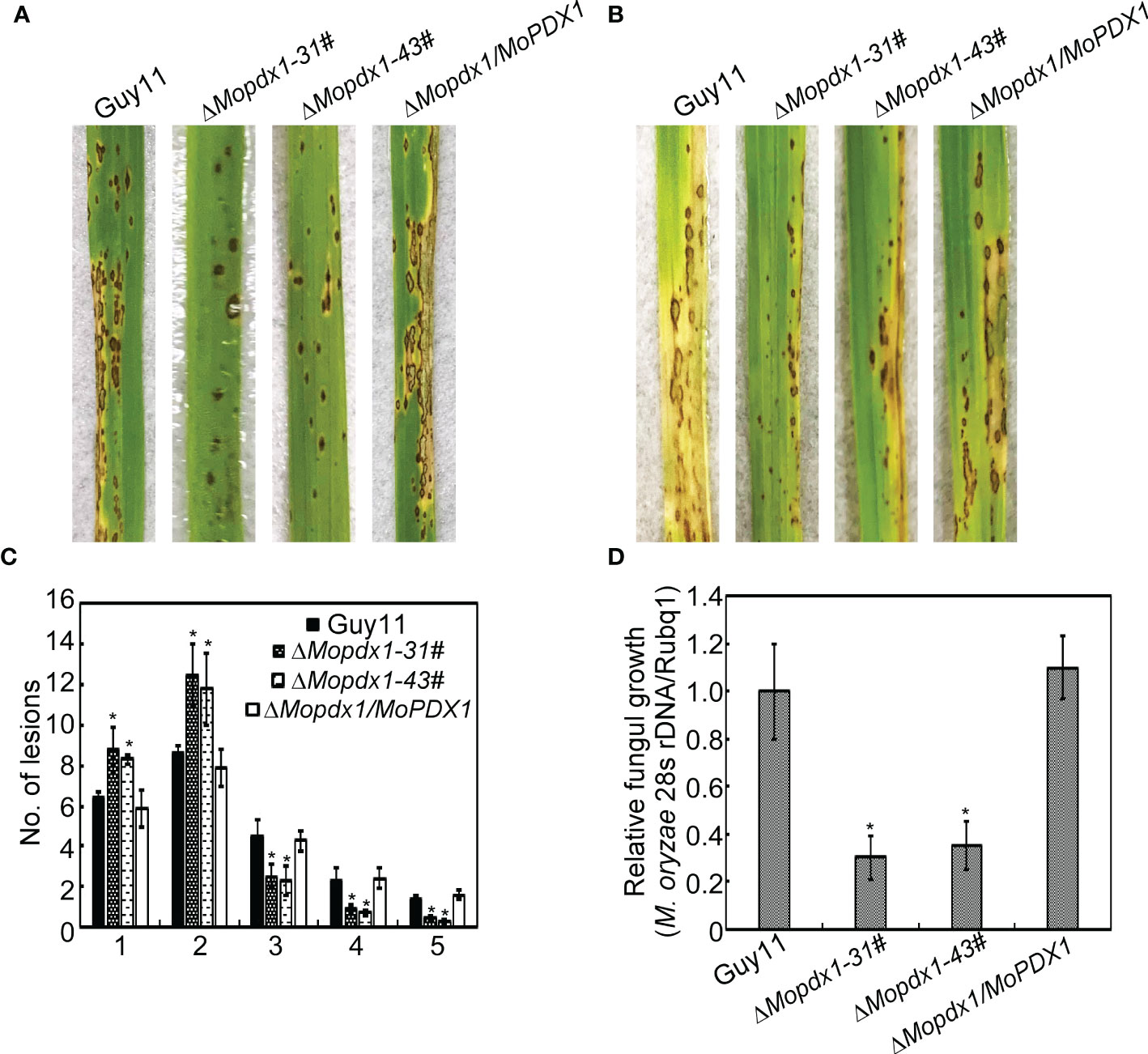
Figure 4 MoPdx1 regulates the pathogenicity of M. oryzae. (A) Five milliliters of conidial suspensions (5×104 spores/mL) of the indicated strains were severally sprayed onto 11-d rice CO-39 seedlings in a moist chamber with 90% relative humidity at 28°C. The diseased leaves were photographed at 7 dpi. (B) Conidial suspensions (15×104 spores/mL) were injected into 21-d rice sheath cells. Photographs were taken at 5 dpi. (C) Lesion-type statistical analysis at 7 dpi. Lesion-type classifications were as referred to Yang et al., 2019. Error bars represent the standard deviation. Asterisks represent significant differences (LSD and Student-Newman-Keuls test, p<0.05). (D) Relative fungal content analysis in leaves of equal area by quantification of M. oryzae 28S rDNA relative to rice Rubq1. Error bars represent the standard deviation. Asterisks represent significant differences (LSD and Student-Newman-Keuls test, p<0.05).
De novo VB6 biosynthesis plays an important role in the invasive growth of M. oryzae
To further explore the causes of the reduced pathogenicity of the two ΔMopdx1 mutants, the growth of infectious hyphae in 21-d rice sheath cells injected with conidial suspensions of Guy11, two MoPDX1 deletion mutants, or the complementary strains were observed at 24 and 48 hpi, respectively. Four types of invasive hyphae are shown in Figure 5A. In Guy11 and complementary strains, type 1 invasive hyphae accounted for 8%, 30% of cells were type 2, 60% of cells were type 3 and 2% of cells were type 4 at 24 hpi. In the two ΔMopdx1 mutants, 18% of cells were type 1, 56% of cells were type 2 and 24% were type 3 (Figure 5B). Most of the cells (approximately 65%) were type 4 and 25% of cells were type 3 in Guy11 and complementary strains at 48 hpi. However, in the two mutant strains, 30% were still type 2, 34% were type 3, and 26% were type 4 (Figure 5B). In addition, conidial suspensions of MoPDX1 deletion mutants were added 10-4 mg/mL of VB6 solutions, and then injected into the rice shealth cells, observed the invasive growth at 24 and 48 hpi. Results found that addition of VB6 could rescue the defects of invasive growth of the mutants whether inoculated 24 or 48 h (Figure 5B). The results suggest that de novo VB6 plays an important role in invasive growth of M. oryzae.
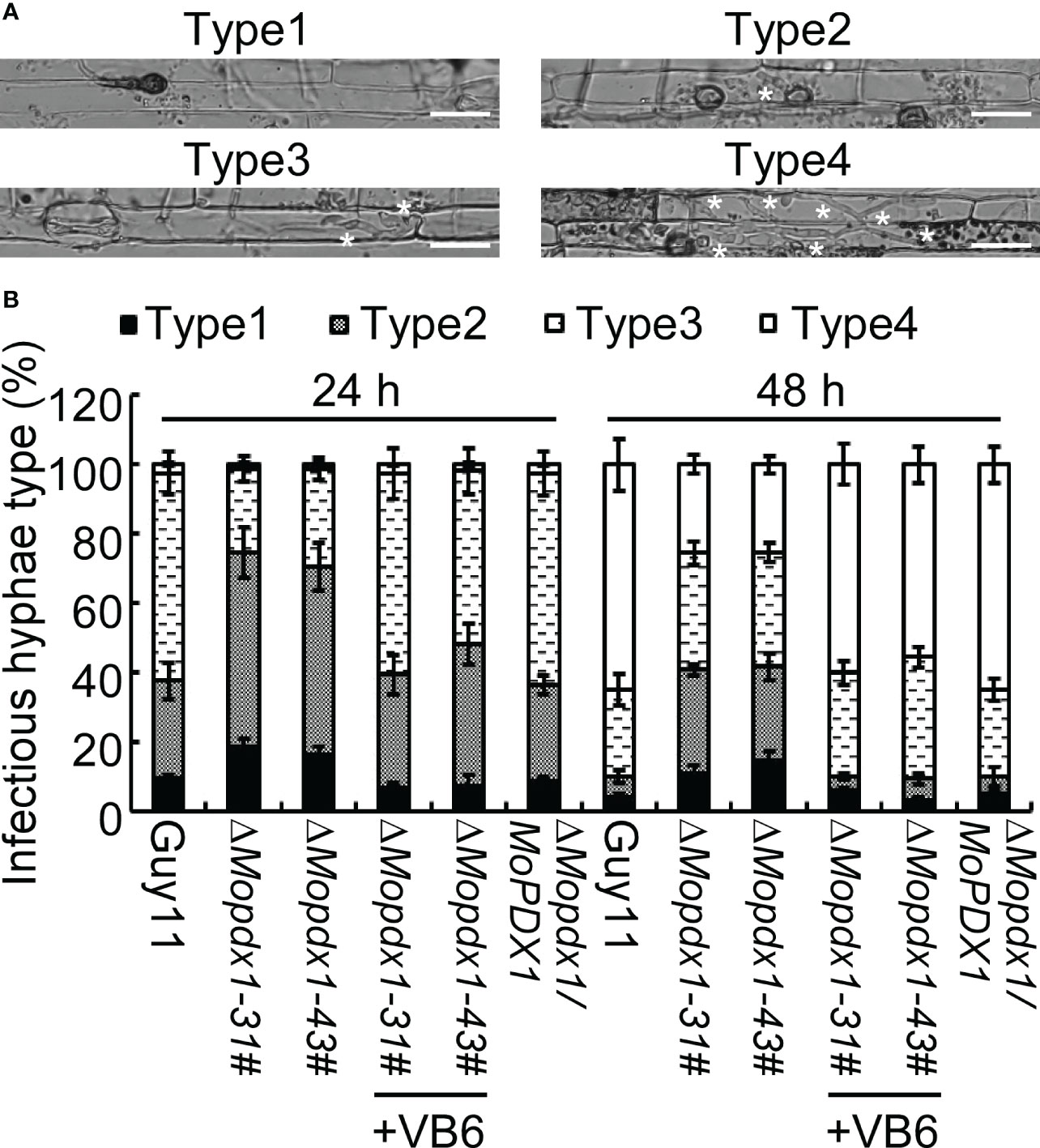
Figure 5 Deletion of MoPDX1 attenuates the growth of infectious hyphae in rice sheath cells. (A) Four types of infectious hyphae (IH) of M. oryzae in rice sheath cells. Type1 has no penetration; type 2 only has penetration peg or a single infectious hypha (IH); type 3 has more than two IH in one rice cell; type 4 has extensive IH into adjacent rice cells. White asterisks indicate IH in rice sheath cells. (B) Statistical analysis of the growth of IH in rice cells at 24 and 48 hpi with or without 10-4 mg/mL of VB6 treating the conidial suspensions of MoPDX1 deletion mutants. One hundred IH were counted in rice cells infected with conidia suspensions of the indicated strains. Error bars represent the standard deviation. The experiments were repeated three times with similar results.
Exogenous addition of VB6 rescue the pathogenicity defect of the ΔMopdx1 mutants
Further, conidial suspensions of MoPDX1 deletion mutants with 10-4 mg/mL VB6 solutions were then inoculated onto the detached 7-d barely leaves, and the lesion incidence was observed at 5 dpi. Results showed that the exogenous addition of commercial VB6 could also rescue the pathogenicity defect of the two ΔMopdx1 mutants on barely leaves (Figure 6). Indicating that VB6 is involved in the virulence of M. oryzae.
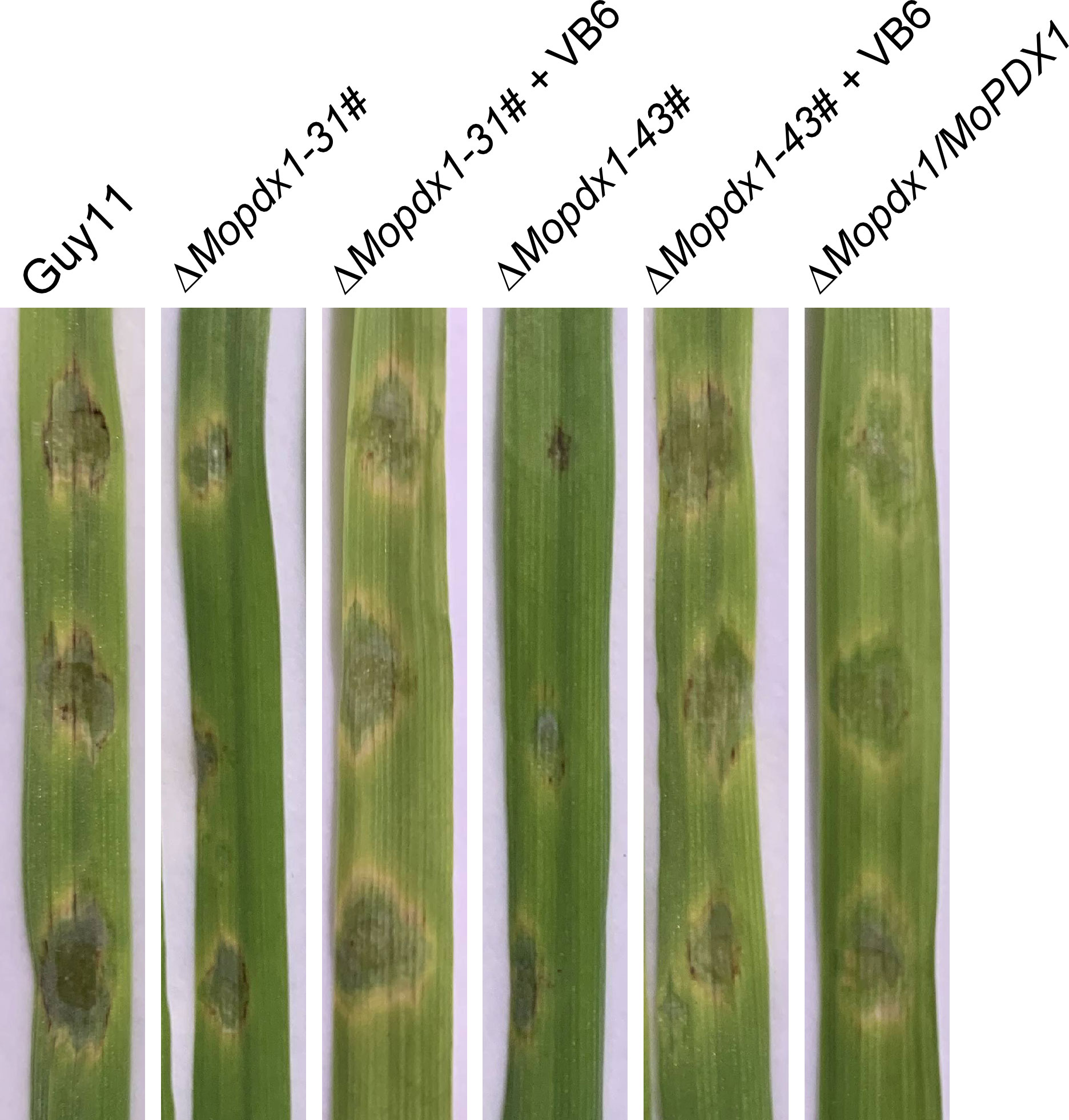
Figure 6 The addition of VB6 rescues the pathogenicity defect of MoPDX1 deletion mutants on barely leaves. Twenty-five microliters of conidial suspensions (5×104 spores/mL) of Guy11, two ΔMopdx1 mutants, and the complementary strains were separately inoculated on the surface of 7-d detached barely leaves, which were then inoculated at 28°C for 24 h in the dark, and for another 4 days under continuous12 h/12 h light/dark conditions. In addition, conidial suspensions of the two mutants were added 10-4 mg/mL VB6 solution. Then, inoculated on the barely leaves under the same environmental conditions as described above. The experiments were repeated three times with similar results.
Deletion of MoPDX1 does not activate the defense response of rice
In general, plants produce a broad-spectrum defense response against the attack of pathogens, including rapid generation of ROS, and changes in the expression levels of pathogenesis-related (PR) proteins (Bradley et al., 1992; Tanaka et al., 2003). We want to determine whether the reduced pathogenicity of the ΔMopdx1 mutants was due to defense responses activated by the host. 3,3’- diaminobenzidine (DAB) was used to stain the ROS in rice sheath cells after inoculation for 48 h. The results showed that the ROS level was not significantly different caused by WT and the two ΔMopdx1 mutants, and approximately 25% of rice sheath cells were stained with DAB (Figure 7A). In addition, the transcript levels of rice defense-related genes PBZ1 and CHT1 (Chen et al., 2014; Liu et al., 2016b) were measured in the rice leaves infected for 48 h by spores of Guy11 and ΔMopdx1 mutants, separately. Water-sprayed rice leaves were used as negative controls. The transcript levels of PBZ1 and CHT1 were also not significantly different between Guy11 and ΔMopdx1 mutants (Figure 7B). Results illustrated that deletion of MoPDX1 does not activate plant defense responses.
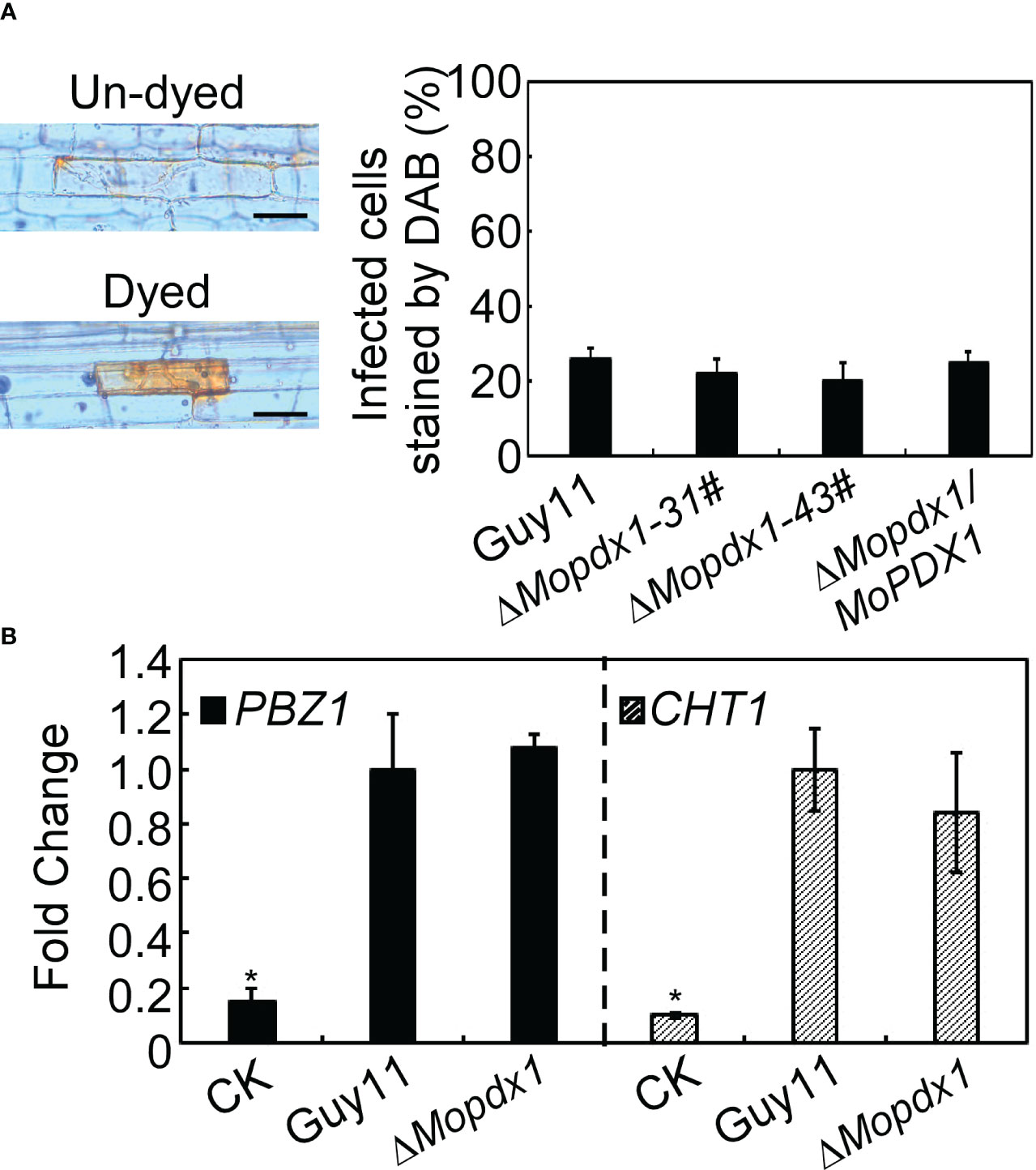
Figure 7 Deletion of MoPDX1 does not activate the defense response of rice. (A) Conidia suspensions (15×104 spores/mL) of indicated strains were injected into 21-d rice sheath cells, DAB (3,3’-diaminobenzidine) was used to dye the ROS produced in rice sheath cells for 10 h at 24 hpi, and then decolorated by alcohol and acetic acid for 4 h. The left panel showed dyed or un-dyed representative photos. The right panel showed the statistical analysis. Error bars represent the standard deviation. There was no significant difference under the different treatments in indicated strains (LSD and Student-Newman-Keuls test, p<0.05). (B) Transcription analysis of the rice defense-related genes PBZ1 and CHT1 by qRT-PCR. Conidial suspensions (5×104 spores/mL) of Guy11 and ΔMopdx1 mutants were separately sprayed onto 11-d rice CO-39 seedlings, rice leaves were collected at 48 hpi and RNA was extracted. Sterile water treatment was used as a control (CK). The stablely expressed rice gene EF1-α was used as an internal control. Error bars represent the standard deviation. Asterisks represent significant differences (LSD and Student-Newman-Keuls test, p<0.05). All experiments were performed for three independent replicates with the same results.
MoPdx1 is located in the cytoplasm of M. oryzae
MoPdx1 co-expressed with a GFP protein to detect its localization at different development stages of M. oryzae, including the mecelium stage, infectious hyphal stages, conidial stage, and appressorium formation stage. The results showed that MoPdx1 was located in the cytoplasm at the mecelium stage, conidial stage, and infectious hyphal stages (Figures 8A, B, E). Furthermore, MoPdx1 was present in spore and germ tubes at 14 hpi and then transferred into the appressorium at 24 hpi (Figures 8C, D). Suggesting that MoPdx1 is located in the cytoplasm of M. oryzae.
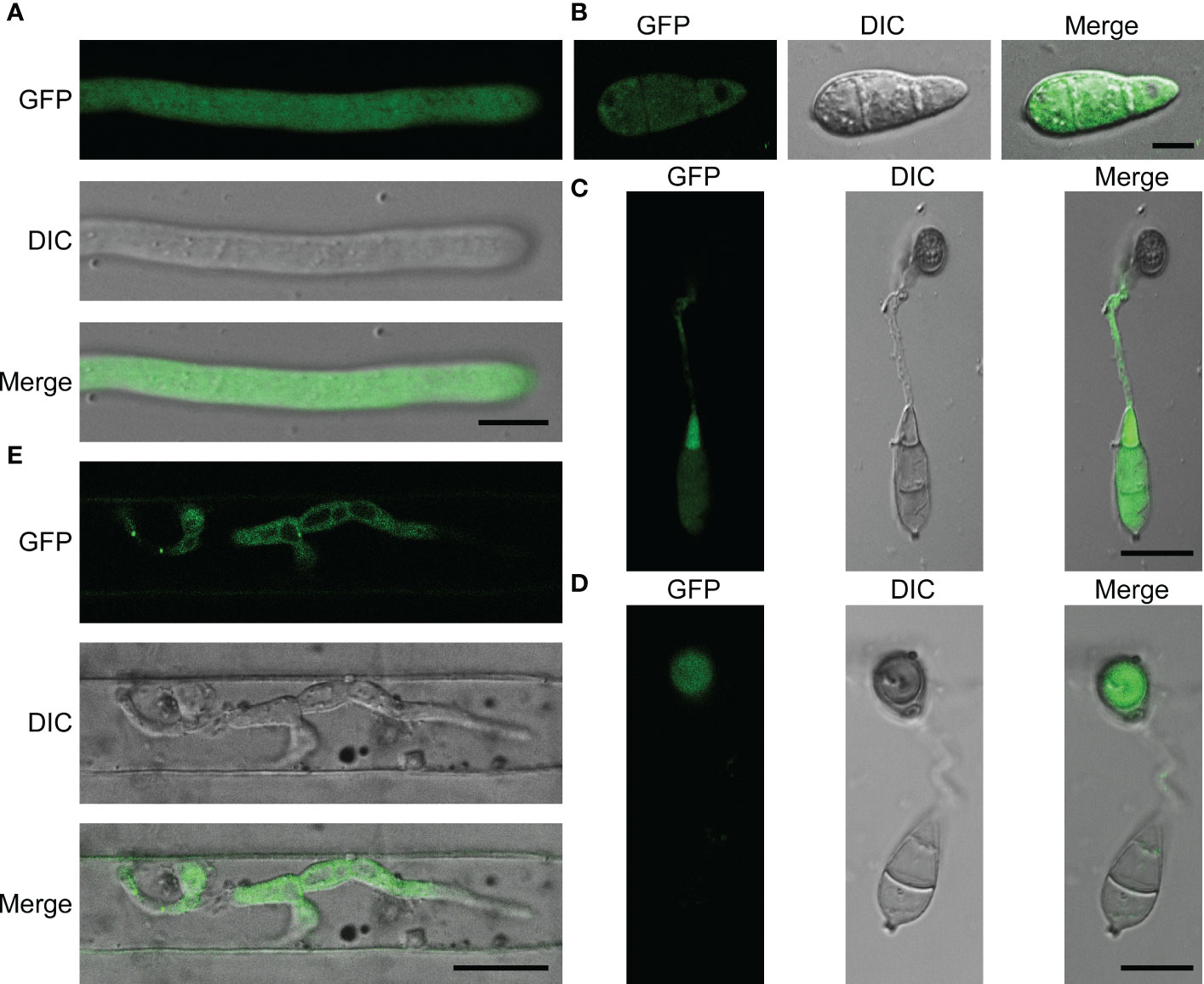
Figure 8 MoPdx1 is located in the cytoplasm of M. oryzae. (A) Hyphal blocks of complementary strains were cultured in liquid CM media at 28°C for 18 h, washed two times with sterile water, and single mycelium were photographed under a confocal fluorescence microscope (Zeiss LSM710, 63x oil). Bar=18 μm. (B) Conidia of complementary strains were observed under a confocal fluorescence microscope (Zeiss LSM710, 63x oil). Bar=18 μm. (C, D) Appressorium formation was observed under a confocal fluorescence microscope (Zeiss LSM710, 63x oil) at 14 and 24 hpi. Bar=18 μm. (E) Conidial suspensions (5×104 spores/mL) of the complementary strains were collected and inoculated into 21-d rice sheath cells, and the green fluorescence of IH were observed at 24 hpi under a confocal fluorescence microscope (Zeiss LSM710, 63x oil). Bar=18 μm.
MoPdx1 is involved in multiple pathways of M. oryzae
To gain further insight into the function of MoPdx1 in M. oryzae, its interacting proteins were enriched by GFP beads using two strains of Guy11 expressing MoPdx1-GFP. A Guy11 strain with only GFP was used as a control. The results showed that 10 proteins had more than two peptides that potentially interacted with the MoPdx1 protein in both two MoPdx1-GFP expressing strains, except the proteins in only GFP expressing strain (Table 3). Furthermore, two proteins previously studied by our laboratory encoded by MGG_11513 (Yang et al., 2018) and MGG_04719 (Yin et al., 2018) were selected to identify the interaction with MoPdx1 through yeast two-hybrid (Y2H), respectively. Both proteins could physically interact with MoPdx1 on -Trp/-Leu/-His medium under 5 mM 3-AT (Figure S3), conforming the reliability of the Co-IP results. In this work, 146 proteins and 275 peptides were identified also in both two MoPdx1-GFP expressing strains, except the proteins in only GFP expressing strain, and further analyzed the function of these IP proteins, which might elucidate the function of MoPdx1 in M. oryzae. Eukaryotic Orthologous Groups (KOG) is a database for identifying orthologous and paralogous proteins. KOG database showed the IP proteins of MoPdx1 mainly focused on translation, ribosomal structure and biogenesis, general function prediction only, posttranslational modification, protein turnover, chaperones, signal transduction mechanisms, cytoskeleton, energy production and conversion, and RNA processing and modification (Figure 9A). The top 10 GO enrichment terms were binding, cellular process, catalytic activity, cell part, cell, metabolic process, organelle, macromolecular complex, organelle part, and structural molecular activity (Figure 9B). The top 10 pathways from KEGG pathway enrichment analysis were metabolic pathways, ribosome, biosynthesis of antibiotics, biosynthesis of secondary metabolites, biosynthesis of amino acids, carbon metabolism, protein processing in the endoplasmic reticulum, spliceosome, pyruvate metabolism, and endocytosis (Table 4). Therefore, MoPdx1 takes part in multiple pathways of M. oryzae, especially in the ribosome and in biosynthesis of various substances.
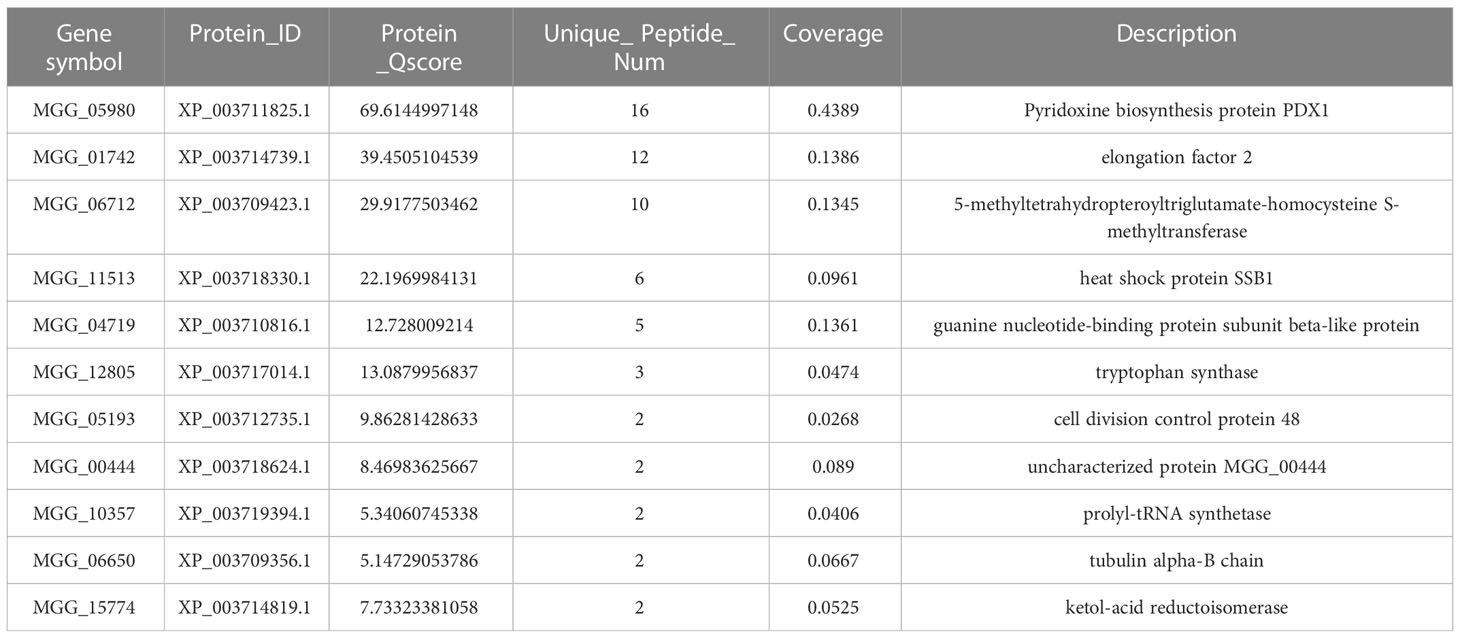
Table 3 Co-IP assays identified more than two peptides that potentially interacted with the MoPdx1 protein in both two MoPdx1-GFP expressing strains, except the proteins in only GFP expressing strains.
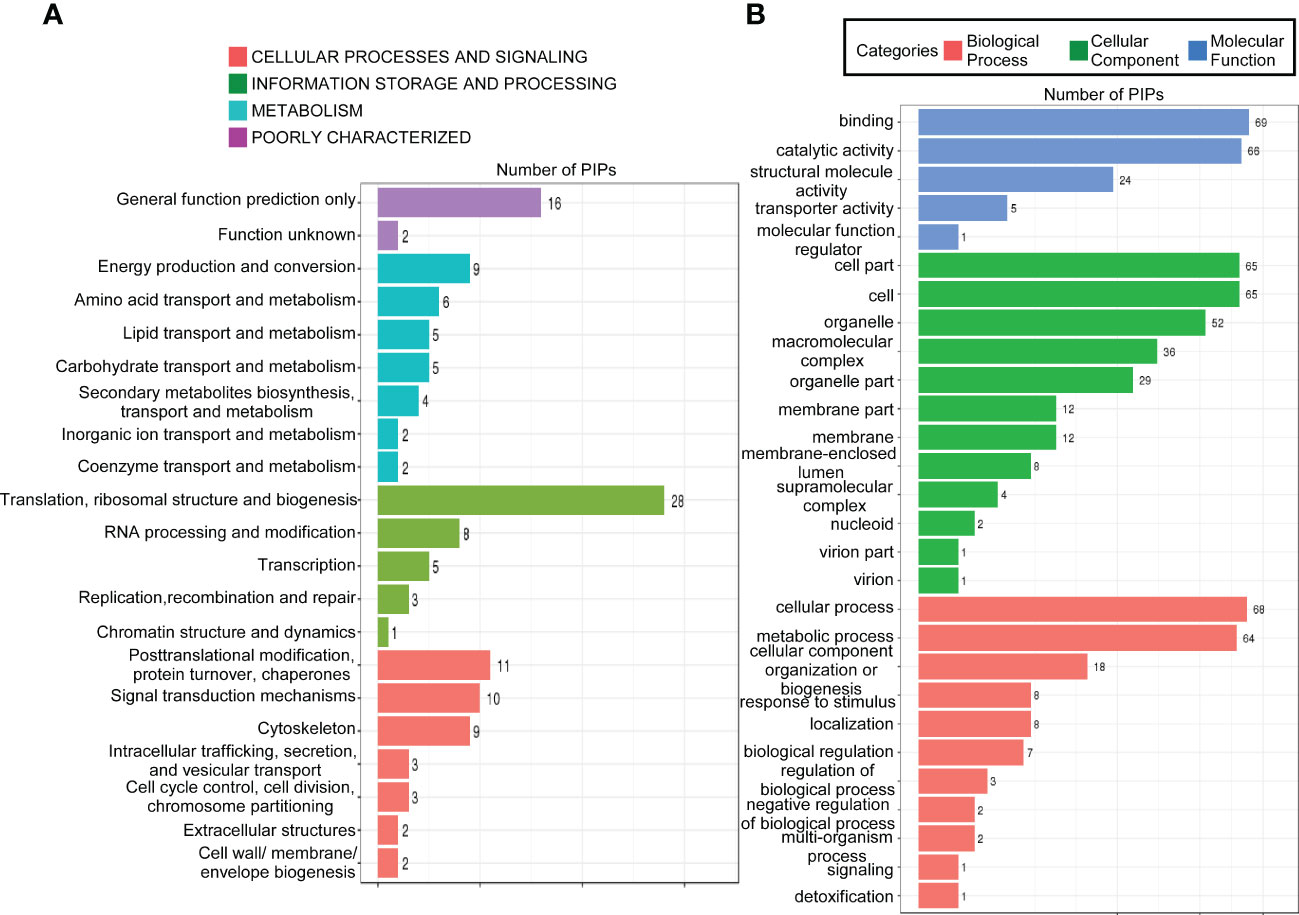
Figure 9 Functional analysis of potential interacting proteins of MoPdx1 in M. oryzae. (A) KOG analysis of potential interaction proteins (PIPs) of MoPdx1. The Y-axis represents the analysis categories, including cellular processes and signaling, information storage and processing, metabolism, and poorly characterized. The X-axis represents the number of PIPs. (B) GO analysis of PIPs of MoPdx1. The Y-axis represents the analysis categories, including biological process, molecular function, and cellular component. The X-axis represents the number of PIPs.
Discussion
In this study, we identified the pyridoxine biosynthesis protein Pdx1 of M. oryzae. Deletion of MoPDX1 made it almost impossible to grow on MM media, exogenous addition of VB6 alone or VB6-containing compounds entirely rescued the growth defect of the mutants on MM media. VB6 content detection showed that there was no significant difference in Guy11, MoPDX1 deletion mutants and complementary strains cultured in liquid CM media. Suggesting that the MoPdx1 protein is involved in de novo VB6 biosynthes not in uptake process, and VB6 plays an important role in the vegetative growth of M. oryzae. However, the vegetative growth of the mutants still has significant difference compared with Guy11 and complementary strains in CM media, indicated that there still had other factors (such as MoPdx1 interacting protein) affect the vegetative growth of the MoPDX1 deletion mutants. The ΔMopdx1 mutants affected appressorium turgor but not affect appressorium formation, indicating that MoPdx1 might take part in the penetrating process of M. oryzae. Besides that, MoPdx1-GFP signals were detected in conidia and germ tube at 14 hpi, further found that the GFP signals were detected in appressoria at 24 hpi in Figures 8C, D. Further, appressorium turgor pressure in the wild type and MoPDX1 deletion mutants were detected at 14 and 24 hpi, found that the appressorium turgor pressure in the mutants were significantly decreased at 2-4 M glycerol compared with that of in wild type at both 14 and 24 hpi. Addition of VB6 in the conidial suspensions at the MoPDX1 deletion mutants could rescue the defects of appressorium turgor pressure at both 14 hpi and 24 hpi (Table 5). The maturation of appressorium of M. oryzae is accompanied by degradation of substances in spores and then transferring to appressorium (Davis et al., 2000; Veneault-Fourrey et al., 2006; Wang et al., 2007; Foster et al., 2017). Therefore, MoPdx1 affected the appressorium function mainly depended on VB6, and VB6 was biosynthesized in conidia and then transported into the appressoria. It is possible that the main function of VB6 was in the transfer of substances from conidia to appressorium at the early stage of appressorium formation (14 hpi), which is crucial for appressorium turgor pressure. Furthermore, deletion of MoPDX1 observably attenuated pathogenicity in rice seedlings and sheaths. The results of the growth of infectious hyphae assay at 24 hpi and 48 hpi, respectively, suggested that attenuated pathogenicity in the ΔMopdx1 mutants was due to the slower growth rate of infectious hyphae. Addition of VB6 in the conidial suspensions of the mutants rescued the invasive growth defects whether inoculated 24 or 48 h. And continuous exogenous addition of VB6 rescued the pathogenicity defect of the mutants on barely leaves. All these results demonstrated that VB6 plays important roles in the development and virulence of M. oryzae regulating vegetative growth, appressorium turgor pressure and invasive growth. In M. oryzae, essential roles in development and pathogenicity were play by amino acid and nutrient metabolism, including methionine metabolism (MoStr3, MoMet6, MoMet13) (Wilson et al., 2012; Yan et al., 2013; Saint-Macary et al., 2015), arginine biosynthesis (MoArg1, MoArg5,6, MoArg7, MoCpa2) (Zhang et al., 2015; Liu et al., 2016a), leucine, isoleucine and valine biosynthesis (MoIlv1, MoIlv2, MoIlv6) (Du et al., 2013; Du et al., 2014), and purine metabolism (MoAde1, MoImd4, MoAde12) (Fernandez et al., 2013; Yang et al., 2019; Zhang et al., 2022). In addition, M. oryzae could not effectively utilize VB6 from rice cells, and must synthesize it in vivo for successful invasion.
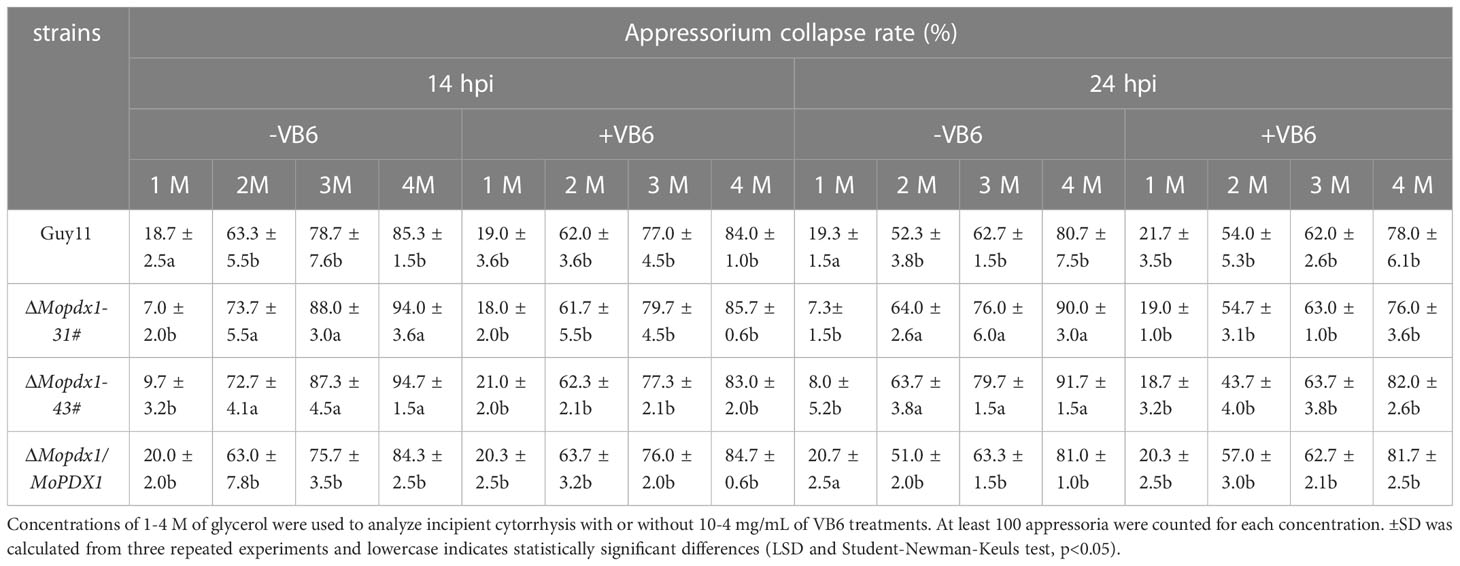
Table 5 Appressorium turgor pressure assays of the wild type, ΔMopdx1 mutant, and the complement strains with or without 10-4 mg/mL of VB6 at 14 or 24 hpi, respectively.
In general, conserved pathogen-associated molecular patterns (PAMPs) triggering plant immunity are the first layer of defense against pathogens with the production of ROS. In the long course of evolution, ROS scavenging system produced by pathogens has helped them successfully invade the host and bypass plant immunity (Nathues et al., 2004; Chi et al., 2009). In this study, DAB staining assays were used to evaluate the ROS content in rice sheath cells, and no significant difference was found in the ROS accumulation in rice cells treated with conidial suspensions of either wild-type or MoPDX1 deletion mutants. Furthermore, the transcript levels of defense-related genes (PBZ1 and CHT1) showed no significant difference, suggested that deletion of MoPDX1 didn’t activate host defense responses. Similar results were previously found for ΔMoade1, ΔMocpa2, and ΔMoimd4 mutants (Fernandez et al., 2013; Liu et al., 2016b; Yang et al., 2019).
In this work, we also explored potential interacting proteins of MoPdx1 by Co-IP assays in vivo to further determine its function in M. oryzae. Ten proteins presented more than two peptides in both MoPdx1-GFP expressing strains except the proteins in only GFP expressing strains. Two proteins of them (encoded by MGG_04719 and MGG_11513) were confirmed to interact with MoPdx1 through the Y2H system, suggesting the reliability of the Co-IP results. MoMip11 protein encoded by MGG_04719 has been reported that linked with MoRgs7 and G-proteins to regulate cAMP signaling, stress responses and pathogenicity of M. oryzae (Yin et al., 2018). The heat-shock protein MoSsb1 (encoded by MGG_11513), which forms a complex with MoSsz1 and MoZuo1, regulated the growth, conidiation, and pathogenicity of M. oryzae mediating cell wall integrity signaling pathway by modulating MoMkk1 biosynthesis (Yang et al., 2018). MoMet6 (encoded by MGG_06712) was a cobalamine-independent methionine synthase, and deletion of MoMET6 lead to nonpathogenic effects on both barely and rice leaves, defective appressorium-mediated penetration, infectious hyphal growth, abnormal vegetative growth, and metabolic perturbations of M. oryzae (Saint-Macary et al., 2015). Mutations of the homologous genes of MGG_10357 and MGG_15774-encoded proteins were inviable in Saccharomyces, showing that these proteins might play essential roles in the development of organisms. In addition, the elongation factor has been reported as a virulence pathogenic factor of pathogens (Hwang et al., 2013; Nouripour-Sisakht et al., 2017; Cui et al., 2018). Tubulin is an important component of the cytoskeleton of organisms. The importance of these potentially interacting proteins at the development and pathogenicity stages suggests that MoPdx1 has an important regulatory effect on the development and virulence of M. oryzae. In addition, the bioinformatic analysis showed that MoPdx1 might participate in multiple pathways, especially in the ribosome and in the biosynthesis of various substances.
However, there were still some inconsistencies between this work and other studies. We found that MoPdx1 was located in the cytoplasm at the hyphal stage, infectious hyphal stages, and conidial stage of M. oryzae. However, previous reports found that Pdx1 in C. nicotianae was located in vesicles (Chung et al., 2002). In addition, MoPdx1 was not found in this study to be involved in the oxidative (mainly H2O2) stress response of M. oryzae (Figure S4). A previous study indicated that Pdx1 in Arabidopsis, tomatoes, and C. nicotianae might be an antioxidant (Ehrenshaft et al., 1999; Chen and Xiong, 2005; Zhang et al., 2014). The reason for these differences might be the difference in species.
Data availability statement
The original contributions presented in the study are included in the article/Supplementary Material. Further inquiries can be directed to the corresponding author.
Author contributions
LY, LL, WF, and ZJ designed and supervised experiments and wrote the manuscript. XL and JW performed the experiments and analyzed the data. All authors contributed to the article and approved the submitted version.
Funding
This work was supported by the Natural Science Foundation of Jiangsu Province of China (BK20200929), China Agriculture Research System of MOF and MARA (Grant No. CARS-30-3-02), Yangzhou University Research Foundation for Advanced Talents (Grant No. 5018/137011834), Hainan Provincial Natural Science Foundation of China (322QN237) and Suzhou Local Rural Talents Training Funding Project.
Conflict of interest
The authors declare that the research was conducted in the absence of any commercial or financial relationships that could be construed as a potential conflict of interest.
Publisher’s note
All claims expressed in this article are solely those of the authors and do not necessarily represent those of their affiliated organizations, or those of the publisher, the editors and the reviewers. Any product that may be evaluated in this article, or claim that may be made by its manufacturer, is not guaranteed or endorsed by the publisher.
Supplementary material
The Supplementary Material for this article can be found online at: https://www.frontiersin.org/articles/10.3389/fcimb.2023.1099967/full#supplementary-material
Supplementary Figure 1 | Southern blot analysis of the MoPDX1 deletion mutants. (A) The strategy of MoPDX1 disruption. The coding region of MoPDX1 was replaced with a hygromycin phosphotransferase (HPH) fragment. (B) Southern blot analysis of two MoPDX1 deletion mutants with a specific inner probe (probe 1) and HPH probe (probe 2).
Supplementary Figure 2 | Measurement of the intracellular VB6 content of different strains on CM media by HPLC-MS analysis. (A-D) HPLC-MS spectra for detecting the intracellular VB6 content of the indicated strains. The peak flow of the VB6 standard was at ~1.25 min.
Supplementary Figure 3 | Interaction of MoPdx1 with proteins encoded by MGG_11513 and MGG_04719 in a yeast two hybrid assay, respectively. MoPDX1 was inserted into vector pGADT7, and MGG_11513 or MGG_04719 were individually inserted into vector pGBKT7. The latter were co-transferred separately with the MoPDX1 vector into yeast AH109 cells and incubated on SD-Leu-Trp for 3 days, then selected on SD-Leu-Trp-His with 1 mM X-α-gal and 5 mM 3-AT (3-amino-1,2,4-triazole) for another 3 days.
Supplementary Figure 4 | MoPdx1 is not involved in the oxidative stress response of M. oryzae. Hyphal blocks of the indicated strains were cultured on CM media with or without 2.5 or 5.0 mM H2O2 at 28°C in the dark. The colony diameters were measured at 7 dpi. Error bars represent the standard deviation. There was no significant difference under the different treatments in indicated strains (LSD and Student-Newman-Keuls test, p<0.05). All experiments were performed for three independent replicates with the same results.
Supplementary Table 1 | Primers used in this study.
Supplementary Table 2 | Measurement of the content of VB6 in different strains on CM media by HPLC-MS.
References
Bradley, D. J., Kjellbom, P., Lamb, C. J. (1992). Elicitor- and wound-induced oxidative cross-linking of a proline-rich plant cell wall protein: a novel, rapid defense response. Cell 70, 21–30. doi: 10.1016/0092-8674(92)90530-P
Calderón-Ospina, C. A., Nava-Mesa, M. O. (2020). B vitamins in the nervous system: current knowledge of the biochemical modes of action and synergies of thiamine, pyridoxine, and cobalamin. CNS Neurosci. Ther. 26, 5–13. doi: 10.1111/cns.13207
Chen, H., Xiong, L. (2005). Pyridoxine is required for post-embryonic root development and tolerance to osmotic and oxidative stresses. Plant J. 44, 396–408. doi: 10.1111/j.1365-313X.2005.02538.x
Chen, Y., Zhai, S., Zhang, H., Zuo, R., Wang, J., Guo, M., et al. (2014). Shared and distinct functions of two Gti1/Pac2 family proteins in growth, morphogenesis and pathogenicity of Magnaporthe oryzae. Environ. Microbiol. 16 (3), 788–801. doi: 10.1111/1462-2920.12204
Chi, M. H., Park, S. Y., Kim, S., Lee, Y. H. (2009). A novel pathogenicity gene is required in the rice blast fungus to suppress the basal defenses of the host. PloS Pathog. 5, e1000401. doi: 10.1371/journal.ppat.1000401
Chung, K. R., Ehrenshaft, M., Daub, M. E. (2002). Functional expression and cellular localization of cercosporin-resistance proteins fused with the GFP in Cercospora nicotianae. Curr. Genet. 41, 159–167. doi: 10.1007/s00294-002-0289-8
Cui, G., Wang, J., Qi, X., Su, J. (2018). Transcription elongation factor GreA plays a key role in cellular invasion and virulence of Francisella tularensis subsp. novicida. Sci. Rep. 8, 6895. doi: 10.1038/s41598-018-25271-5
Davis, D. J., Burlak, C., Money, N. P. (2000). Biochemical and biomechanical aspects of appressorial development on Magnarporthe grisea. Dev. Plant Pathol. 15, 248–256.
Du, Y., Hong, L., Tang, W., Li, L., Wang, X., Ma, H., et al. (2014). Threonine deaminase MoIlv1 is important for conidiogenesis and pathogenesis in the rice blast fungus Magnaporthe oryzae. Fungal Genet. Biol. 73, 53–60. doi: 10.1016/j.fgb.2014.10.001
Du, Y., Zhang, H., Hong, L., Wang, J., Zheng, X., Zhang, Z. (2013). Acetolactate synthases MoIlv2 and MoIlv6 are required for infection-related morphogenesis in Magnaporthe oryzae. Mol. Plant Pathol. 14, 870–884. doi: 10.1111/mpp.12053
Ehrenshaft, M., Bilski, P., Li, M. Y., Chignell, C. F., Daub, M. E. (1999). A highly conserved sequence is a novel gene involved in de novo vitamin B6 biosynthesis. Proc. Natl. Acad. Sci. U.S.A. 96, 9374–9378. doi: 10.1073/pnas.96.16.9374
Eliot, A. C., Kirsch, J. F. (2004). Pyridoxal phosphate enzymes: mechanistic, structural, and evolutionary considerations. Annu. Rev. Biochem. 73, 383–415. doi: 10.1146/annurev.biochem.73.011303.074021
El Qaidi, S., Yang, J., Zhang, J. R., Metzger, D. W., Bai, G. (2013). The vitamin B6 biosynthesis pathway in Streptococcus pneumoniae is controlled by pyridoxal 5'-phosphate and the transcription factor PdxR and has an impact on ear infection. J. Bacteriol 195, 2187–2196. doi: 10.1128/JB.00041-13
Fernandez, J., Yang, K. T., Cornwell, K. M., Wright, J. D., Wilson, R. A. (2013). Growth in rice cells requires de novo purine biosynthesis by the blast fungus Magnaporthe oryzae. Sci. Rep. 3, 2398. doi: 10.1038/srep02398
Foster, A. J., Ryder, L. S., Kershaw, M. J., Talbot, N. J. (2017). The role of glycerol in the pathogenic lifestyle of the rice blast fungus Magnaporthe oryzae. Environ. Microbiol. 19, 1008–1016. doi: 10.1111/1462-2920.13688
Giraldo, M. C., Dagdas, Y. F., Gupta, Y. K., Mentlak, T. A., Yi, M., Martinez-Rocha, A. L., et al. (2013). Two distinct secretion systems facilitate tissue invasion by the rice blast fungus Magnaporthe oryzae. Nat. Commun. 4, 1996. doi: 10.1038/ncomms2996
Gupta, L., Vermani, M., Kaur Ahluwalia, S., Vijayaraghavan, P. (2021). Molecular virulence determinants of Magnaporthe oryzae: disease pathogenesis and recent interventions for disease management in rice plant. Mycology 12, 174–187. doi: 10.1080/21501203.2020.1868594
Howard, R. J., Ferrari, M. A., Roach, D. H., Money, N. P. (1991). Penetration of hard substrates by a fungus employing enormous turgor pressures. Proc. Natl. Acad. Sci. U.S.A. 88, 11281–11284. doi: 10.1073/pnas.88.24.11281
Hwang, J., Oh, C. S., Kang, B. C. (2013). Translation elongation factor 1B (eEF1B) is an essential host factor for tobacco mosaic virus infection in plants. Virology 439, 105–114. doi: 10.1016/j.virol.2013.02.004
Jia, Y., Zhou, E., Lee, S., Bianco, T. (2016). Coevolutionary dynamics of rice blast resistance gene pi-ta and Magnaporthe oryzae avirulence gene AVR-pita 1. Phytopathology 106, 676–683. doi: 10.1094/PHYTO-02-16-0057-RVW
Kennedy, D. O. (2016). B vitamins and the brain: mechanisms, dose and efficacy–a review. Nutrients 8, 68. doi: 10.3390/nu8020068
Liu, X., Cai, Y., Zhang, X., Zhang, H., Zheng, X., Zhang, Z. (2016b). Carbamoyl phosphate synthetase subunit MoCpa2 affects development and pathogenicity by modulating arginine biosynthesis in Magnaporthe oryzae. Front. Microbiol. 7, 2023 doi: 10.3389/fmicb.2016.02023
Liu, H., Lu, C., Li, Y., Wu, T., Zhang, B., Liu, B., et al. (2022). The bacterial effector AvrRxo1 inhibits vitamin B6 biosynthesis to promote infection in rice. Plant Commun. 3, 100324. doi: 10.1016/j.xplc.2022.100324
Liu, X., Qian, B., Gao, C., Huang, S., Cai, Y., Zhang, H., et al. (2016a). The putative protein phosphatase MoYvh1 functions upstream of MoPdeH to regulate the development and pathogenicity in Magnaporthe oryzae. Mol. Plant Microbe Interact. 29, 496–507. doi: 10.1094/MPMI-11-15-0259-R
Nathues, E., Joshi, S., Tenberge, K. B., von den Driesch, M., Oeser, B., Bäumer, N., et al. (2004). CPTF1, a CREB-like transcription factor, is involved in the oxidative stress response in the phytopathogen Claviceps purpurea and modulates ROS level in its host Secale cereale. Mol. Plant Microbe Interact. 17, 383–393. doi: 10.1094/MPMI.2004.17.4.383
Nouripour-Sisakht, S., Ahmadi, B., Makimura, K., Hoog, S., Umeda, Y., Alshahni, M. M., et al. (2017). Characterization of the translation elongation factor 1-α gene in a wide range of pathogenic aspergillus species. J. Med. Microbiol. 66, 419–429. doi: 10.1099/jmm.0.000450
Percudani, R., Peracchi, A. (2009). The B6 database: a tool for the description and classification of vitamin B6-dependent enzymatic activities and of the corresponding protein families. BMC Bioinf. 10, 273. doi: 10.1186/1471-2105-10-273
Phillips, R. S. (2015). Chemistry and diversity of pyridoxal-5'-phosphate dependent enzymes. Biochim. Biophys. Acta 1854, 1167–1174. doi: 10.1016/j.bbapap.2014.12.028
Rosenberg, I. H. (2012). A history of the isolation and identification of vitamin B(6). Ann. Nutr. Metab. 61, 236–238. doi: 10.1159/000343113
Saint-Macary, M. E., Barbisan, C., Gagey, M. J., Frelin, O., Beffa, R., Lebrun, M. H., et al. (2015). Methionine biosynthesis is essential for infection in the rice blast fungus Magnaporthe oryzae. PloS One 10, e0111108. doi: 10.1371/journal.pone.0111108
Sakulkoo, W., Osés-Ruiz, M., Oliveira Garcia, E., Soanes, D. M., Littlejohn, G. R., Hacker, C., Talbot, N. J., et al. (2018). A single fungal MAP kinase controls plant cell-to-cell invasion by the rice blast fungus. Science 359, 1399–1403. doi: 10.1126/science.aaq0892
Skamnioti, P., Gurr, S. J. (2009). Against the grain: safeguarding rice from rice blast disease. Trends Biotechnol. 27, 141–150. doi: 10.1016/j.tibtech.2008.12.002
Sweigard, J. A., Chumley, F. G., Valent, B. (1992). Disruption of a Magnaporthe grisea cutinase gene. Mol. Gen. Genet. 232, 183–190. doi: 10.1007/BF00279995
Tanaka, N., Che, F. S., Watanabe, N., Fujiwara, S., Takayama, S., Isogai, A. (2003). Flagellin from an incompatible strain of acidovorax avenae mediates H2O2 generation accompanying hypersensitive cell death and expression of PAL, cht-1, and PBZ1, but not of lox in rice. Mol. Plant Microbe Interact. 16, 422–428. doi: 10.1094/MPMI.2003.16.5.422
Veneault-Fourrey, C., Baroach, M., Egan, M., Wakley, G., Talbot, N. J. (2006). Autophagic fungal cell death is necessary for infection by the rice blast fungus. Science 312, 580–583. doi: 10.1126/science.1124550
Wang, Z. Y., Soanes, D. M., Kershaw, M. J., Talbot, N. J. (2007). Functional analysis of lipid metabolism in Magnarporthe grisea reveals a requirement for peroxisomal fatty acid beta-oxidation during appressorium-mediated plant infection. Mol. Plant Microbe Interact. 20, 475–491. doi: 10.1094/MPMI-20-5-0475
Wendołowicz, A., Stefańska, E., Ostrowska, L. (2018). Influence of selected dietary components on the functioning of the human nervous system. Rocz Panstw Zakl Hig. 69, 15–21.
Wilson, R. A., Fernandez, J., Quispe, C. F., Gradnigo, J., Seng, A., Moriyama, E., et al. (2012). Towards defining nutrient conditions encountered by the rice blast fungus during host infection. PloS One 7, e47392. doi: 10.1371/journal.pone.0047392
Wilson, R. A., Talbot, N. J. (2009). Under pressure: investigating the biology of plant infection by Magnaporthe oryzae. Nat. Rev. Microbiol. 7, 185–195. doi: 10.1038/nrmicro2032
Yan, X., Que, Y., Wang, H., Wang, C., Li, Y., Yue, X., et al. (2013). The MET13 methylenetetrahydrofolate reductase gene is essential for infection-related morphogenesis in the rice blast fungus Magnaporthe oryzae. PloS One 8, e76914. doi: 10.1371/journal.pone.0076914
Yang, J., Liu, M., Liu, X., Yin, Z., Sun, Y., Zhang, H., et al. (2018). Heat-shock proteins MoSsb1, MoSsz1, and MoZuo1 attenuate MoMkk1-mediated cell-wall integrity signaling and are important for growth and pathogenicity of Magnaporthe oryzae. Mol. Plant Microbe Interact. 31, 1211–1221. doi: 10.1094/MPMI-02-18-0052-R
Yang, L., Ru, Y., Cai, X., Yin, Z., Liu, X., Xiao, Y., et al. (2019). MoImd4 mediates crosstalk between MoPdeH-cAMP signalling and purine metabolism to govern growth and pathogenicity in Magnaporthe oryzae. Mol. Plant Pathol. 20, 500–518. doi: 10.1111/mpp.12770
Yin, Z., Zhang, X., Wang, J., Yang, L., Feng, W., Chen, C., et al. (2018). MoMip11, a MoRgs7-interacting protein, functions as a scaffolding protein to regulate cAMP signaling and pathogenicity in the rice blast fungus Magnaporthe oryzae. Environ. Microbiol. 20, 3168–3185. doi: 10.1111/1462-2920.14102
Zhang, Z., Hao, Z., Chai, R., Qiu, H., Wang, Y., Wang, J., et al. (2022). Adenylsuccinate synthetase MoADE12 plays important roles in the development and pathogenicity of the rice blast fungus. J. Fungi (Basel) 8(8), 780. doi: 10.3390/jof8080780
Zhang, Y., Liu, B., Li, X., Ouyang, Z., Huang, L., Hong, Y., et al. (2014). The de novo biosynthesis of vitamin B6 is required for disease resistance against Botrytis cinerea in tomato. Mol. Plant Microbe Interact. 27, 688–699. doi: 10.1094/MPMI-01-14-0020-R
Zhang, Y., Shi, H., Liang, S., Ning, G., Xu, N., Lu, J., et al. (2015). MoARG1, MoARG5,6 and MoARG7 involved in arginine biosynthesis are essential for growth, conidiogenesis, sexual reproduction, and pathogenicity in Magnaporthe oryzae. Microbiol. Res. 180, 11–22. doi: 10.1016/j.micres.2015.07.002
Keywords: Magnaporthe oryzae, MoPdx1, vitamin B6, development, pathogenicity
Citation: Yang L, Liu X, Wang J, Li L, Feng W and Ji Z (2023) Pyridoxine biosynthesis protein MoPdx1 affects the development and pathogenicity of Magnaporthe oryzae. Front. Cell. Infect. Microbiol. 13:1099967. doi: 10.3389/fcimb.2023.1099967
Received: 16 November 2022; Accepted: 30 January 2023;
Published: 07 February 2023.
Edited by:
Chaoyang Xue, Rutgers University, Newark, United StatesReviewed by:
Cong Jiang, Northwest A&F University, ChinaSudhir Navathe, Agharkar Research Institute, India
Wende Liu, Institute of Plant Protection (CAAS), China
Copyright © 2023 Yang, Liu, Wang, Li, Feng and Ji. This is an open-access article distributed under the terms of the Creative Commons Attribution License (CC BY). The use, distribution or reproduction in other forums is permitted, provided the original author(s) and the copyright owner(s) are credited and that the original publication in this journal is cited, in accordance with accepted academic practice. No use, distribution or reproduction is permitted which does not comply with these terms.
*Correspondence: Zhaolin Ji, zhlji@yzu.edu.cn