- 1Guangxi Key Laboratory of Aquatic Biotechnology and Modern Ecological Aquaculture, Guangxi Engineering Research Center for Fishery Major Diseases Control and Efficient Healthy Breeding Industrial Technology (GERCFT), Guangxi Academy of Sciences, Nanning, China
- 2China-ASEAN Modern Fishery Industry Technology Transfer Demonstration Center, Beibu Gulf Marine Industrial Research Institute, Guangxi Academy of Marine Sciences, Nanning, China
- 3Guangxi Fisheries Technology Extension Station, Nanning, China
- 4Guangxi Academy of Fishery Science, Nanning, China
Introduction: The bacterium Elizabethkingia miricola is a multispecies pathogen associated with meningitis-like disease that has been isolated from several amphibian species, including the bullfrog, but this is the first isolation in Guangxi. In the present study, the dominant bacteria were isolated from the brains of five bullfrogs with meningitis-like disease on a South China farm in Guangxi.
Methods: The NFEM01 isolate was identified by Gram staining; morphological observations; 16S rRNA, rpoB, and mutT-based phylogenetic tree analysis; and physiochemical characterization and was subjected to drug sensitivity and artificial infection testing.
Results and discussion: As a result of identification, the NFEM01 strain was found to be E. miricola. An artificial infection experiment revealed that NFEM01 infected bullfrogs and could cause symptoms of typical meningitis-like disease. As a result of the bacterial drug sensitivity test, NFEM01 is highly sensitive to mequindox, rifampicin, enrofloxacin, nitrofural, and oxytetracycline and there was strong resistance to gentamicin, florfenicol, neomycin, penicillin, amoxicillin, doxycycline, and sulfamonomethoxine. This study provides a reference to further study the pathogenesis mechanism of E. miricola-induced bullfrog meningitislike disease and its prevention and treatment.
Highlights
• E. miricola was first isolated from bullfrogs in Guangxi.
• E. miricola induced meningitis-like disease in bullfrogs.
• E. miricola was resistant to the majority of antibiotics tested.
Introduction
Aquaculture provides humans with over 1/3 of the high-quality protein consumed (Li et al., 2022). The bullfrog is an important aquatic economy native to eastern North America and has been widely introduced worldwide (Akmentins and Cardozo, 2010). With the progress of socio-economic development and increases in people’s standard of living, the demands for food safety and quality keep increasing. The bullfrog is increasingly consumer friendly as a good quality meat, and recently, demand for bullfrog has been increasing (Schloegel et al., 2010; Zhu et al., 2021). The aquaculture of American bullfrogs for the meat industry has expanded worldwide. China, Taiwan, Brazil, and Ecuador are well-known for their significant production, while the United States, France, Canada, Belgium, Italy, and Spain are well-known for their significant consumption (FAO, 2023). The bullfrog is an economical frog, and the development of artificial aquaculture is rapidly developing to meet the rise in market demand (Zhang et al., 2015). The bullfrog was first introduced to China as a food source from Cuba and Japan (Wu et al., 2004). Since then, the cultivation of the bullfrog has made remarkable developments in China and has been introduced to many provinces (Zhang et al., 2015). Recently, there have been frequent occurrences of bullfrog diseases, especially bacterial pandemics, such as epidemic meningitis-like disease (EMD) and red leg syndrome (RLS), which severely damage the bullfrog aquaculture industry (Pasteris et al., 2006; Trimpert et al., 2021). EMD has been frequently occurring in recent years (Zajmi et al., 2022). Disease in the bullfrog is observed as signs of torticollis, head slanting to one side, swimming in circles, and loss of appetite (Hu et al., 2017), and 60-90% of diseased animals die within several days to weeks after the onset (Hu et al., 2020). It is found that the epidemic of bacterial diseases is the main cause of major loss to economic bullfrog farming (Mauel et al., 2002; Li et al., 2018), so in order for frog aquaculture to healthily and rapidly develop, we should accelerate the study of bacterial diseases in aquaculture and find reasonable measures (Yu et al., 2021).
Elizabethkingia spp. is a pathogen that threatens the lives of humans and animals (Zajmi et al., 2022). Elizabethkingia spp. is a potential zoonotic pathogen (Vancanneyt et al., 1994); it is widely distributed in the natural environment and also exists in the hospital environment (Moore et al., 2016; Chew et al., 2018; Hem et al., 2022). It is a potentially infectious pathogen in the hospital, which can cause newborn meningitis, adult sepsis, and skin and soft tissue infection, and mortality is rather high in infected patients (Furyk et al., 2011). Elizabethkingia spp. infection has been reported worldwide, especially in patients whose immune function is compromised, causing a fatal human infection (Dziuban et al., 2018).
Besides human infection, Elizabethkingia spp. also infects birds (Vancanneyt et al., 1994), dogs (Bordelo et al., 2016), aquaculture animals such as tilapia (Jacobs and Chenia, 2011), catfish (Laith et al., 2017), and many amphibians, including tiger frogs (Xie et al., 2009), spiny frogs (Lei et al., 2019), and northern leopard frogs (Trimpert et al., 2021). According to earlier research, the Enterobacteriaceae (including Proteus vulgaris and Proteus mirabilis), Pseudomonas aeruginosa, Aeromonas, and a number of Staphylococcus epidermidis strains were considered to be the pathogens causing EMD (Cunningham et al., 1996). Another study identified E. miricola as a pathogen of EMD in black-spotted frogs (Hu et al., 2017). A recent study has shown that E. miricola was the pathogen isolated from diseased American bullfrogs (Lithobates catesbeianus) in farms in the Guangdong province (Liu et al., 2022). There are many reports on EMD, which is the most serious disease for many kinds of cultured frogs in recent years (Mauel et al., 2002; Xie et al., 2009; Lei et al., 2019), but there is no consensus on the pathogen. A widespread outbreak of disease occurred in bullfrogs on different farms in Guangxi, resulting in high mortality and severe economic losses. Nevertheless, the underlying cause of the explosion of bullfrog disease is not clear. In the present study, we investigated the pathogen of the bullfrog, characterized the pathogen, and isolated the main bacterial pathogen in meningitis. The results of the study provide a theoretical reference for further studies on bullfrog dermatology and for helping to prevent and treat EMD during bullfrog farming.
Materials and methods
Bacterial isolation
From early May to July 2022, the death rate of cultured bullfrogs was high in Nanning, Guangxi, China. The bullfrogs were raised within a simple fence of 20 square meters. During this time, the water temperature was between 30 and 33 °C. The bullfrogs were fed twice a day with commercial feed (Tongwei Biotechnology Co., Nanning, China). The water in the housing was removed and replaced with fresh water and a continuous flow of fresh water was provided every day.
The outbreak of disease on the farm caused a high number of deaths among bullfrogs. Five bullfrogs with typical symptoms (weight 107.6 ± 3.2 g per bullfrog) and those who were close to death were chosen for the isolation of pathogenic bacteria according to the previous method (Lei et al., 2019). The heads of the bullfrogs were dissected, and the brain tissue was removed. The brain tissue was then put into a sterile homogenizer, and the appropriate amount of sterile water was added and thoroughly homogenized. The 100-μL homogenate was diluted 10 times and placed on an LB plate and Columbia blood agar plates (Huankai Microbial, Guangzhou, China), which were incubated aerobically and anaerobically at 37 °C overnight. The colonies were selected according to their morphological characteristics and labeled on the LB plate. The strains were expanded and identified. The purified bacterial strain was used for Gram staining, morphological observation, physiological and chemical analysis, molecular identification, and subsequent infection.
Morphological observation
Several bacteria from different frogs were examined and all properties seemed equivalent, so a colony was selected randomly, and the purified NFEM01 strain was cultured for 48 h. After gram staining, the NFEM01 strain was observed under an optical microscope. Hemolytic activity was determined on a Columbia blood agar plate. The NFEM01 strain was dehydrated by ethanol (25%, 50%, 75%, 100%) for 30 minutes then dried, gold-plated, and visualized using a Hitachi s-3400N (Hitachi, Tokyo, Japan) scanning electron microscope (Bozzola, 2014).
Physiological and chemical characteristics
Physiological and biochemical characteristics of the NFEM01 strain were analyzed using an API® 20E (bioMérieux, Marcy l’Etoile, France) bacterial identification system. The physiological and biochemical characteristics of the NFEM01 isolate were assessed based on previously published methods (Shayegani et al., 1978).
16S rRNA, rpoB, and mutT gene sequences and phylogenetic tree analysis
The genomic DNA of the NFEM01 strain was extracted using a bacterial genomic DNA kit (Qiagen, Hilden, Germany). Primers 27 F/1492 R (Weisburg et al., 1991), Eliz rpoB F/Eliz rpoB R, and mutT F/mutT R (Table 1) were used to amplify the 16S rRNA gene, rpoB gene (Kenna et al., 2018), and mutT gene (Zhang et al., 2020) respectively. Amplified products were detected using 1.5% agarose gel electrophoresis. The positive amplification products were sequenced by Aoke Dingsheng Biotechnology Co., Ltd. (Wuhan, China). Gene sequence analysis using the Basic Local Alignment Search Tool (BLAST) (https://blast.ncbi.nlm.nih.gov/Blast.cgi) and a nucleotide sequence identity of > 98% was used as the criterion for identification. All gene sequences are stored in the NCBI GenBank database with the registration number PRJNA893762.
Antibiotic susceptibility test
Antimicrobial susceptibility was tested using the Kirby Bauer disk diffusion method. Bacterial suspensions were uniformly distributed on a Mueller Hinton agar plate (Hangzhou Tianhe Microbial Reagent Co., Ltd., Hangzhou, China). Antibiotic disks were placed on the surface of the culture plate. The plate was incubated at 28°C for 24 h. The inhibition zone was measured, and the results were interpreted according to the Clinical Laboratory Standards Institute (CLSI) standard (2016) and previous research (Jorgensen and Turnidge, 2015).
Artificial infection test
After 7 days of domestication, 60 healthy bullfrogs (7.07 ± 0.82 g) were divided into six groups. The NFEM01 isolate was cultured in LB liquid at 37°C for 24 h. The bacterial concentration (colony-forming units) CFU·mL-1 was determined by LB plate counts after the strain had been subjected to 10-fold serial dilution. A group of 10 bullfrogs was infected by a 0.2 ml intraperitoneal injection with 5.18 × 104, 5.18 × 105, 5.18 × 106, 5.18 × 107, 5.18 × 108, 5.18 × 109 CFU·mL-1 of NFEM01, respectively. Phosphate buffered saline (PBS) was the negative control. Clinical symptoms and mortality from infection to 14 days post-infection were recorded. The brains of the dead bullfrogs were collected to reisolate NFEM01.
Histopathological observation
The samples of the liver, spleen, kidney, intestine, and brain of the bullfrog from the pathogenicity study were fixed in 10% buffered formalin, trimmed, dehydrated using ethanol, and embedded in paraffin blocks for histopathological examination. These blocks were sectioned and stained with hematoxylin and eosin (H&E).
Statistical analysis
Data were analyzed in the statistical program GraphPad Prism version 5 (GraphPad Software, San Diego, California, USA) using one-way analysis of variance (ANOVA) followed by Tukey’s multiple comparison test. P < 0.05 were considered significant differences.
Results
Bullfrogs with meningitis-like disease
The diseased bullfrogs showed signs of severe neurological disorder (Figure 1A), the liver, spleen, and kidney were enlarged (Figure 1B), and the spine of a frog was curved (Figure 1C). The healthy bullfrog neck (Figure 1D), liver, spleen, kidney (Figure 1E), and spine (Figure 1F) are also shown.
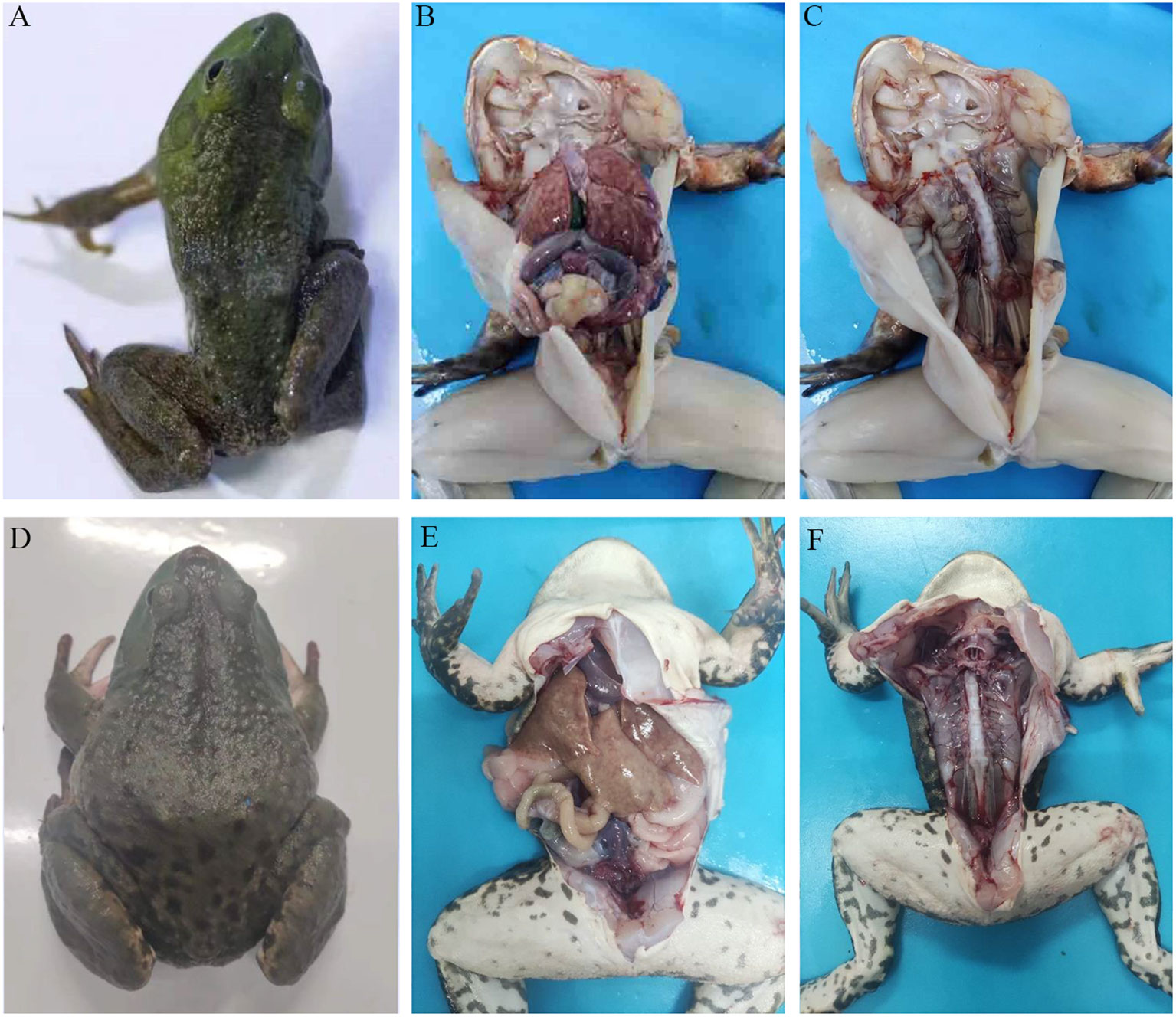
Figure 1 Clinical features of a bullfrog epidemic meningitis-like disease. The diseased bullfrogs showed serious torticollis (A), the liver, spleen, and kidney were enlarged (B), and the spine of one frog was curved (C). The healthy bullfrog neck (D), liver, spleen, kidney (E), and spine (F) are shown.
Morphological observation
All five diseased bullfrogs were shown to contain one bacterial type for which there was heavy growth on LB plates, with very little growth of other organisms. The predominant bacterial type found on aerobic plates and the colonies were selected. After incubation at 37°C for 24 h, the colonies appeared smooth, raised, round, and white (Figure 2A). The NFEM01 strain was a gram-negative bacterium (Figure 2C). Appearance after growth on Colombia blood agar (Figure 2B) and following scanning electron microscopy (Figure 2D) are shown. The NFEM01 had a clear transparent zone around the colonies on the blood agar plates indicative of beta hemolysis activity. Scanning electron microscopy showed that the bacteria were nearly rod-shaped and approximately 1.4 µm in diameter and 2.6 µm in length.
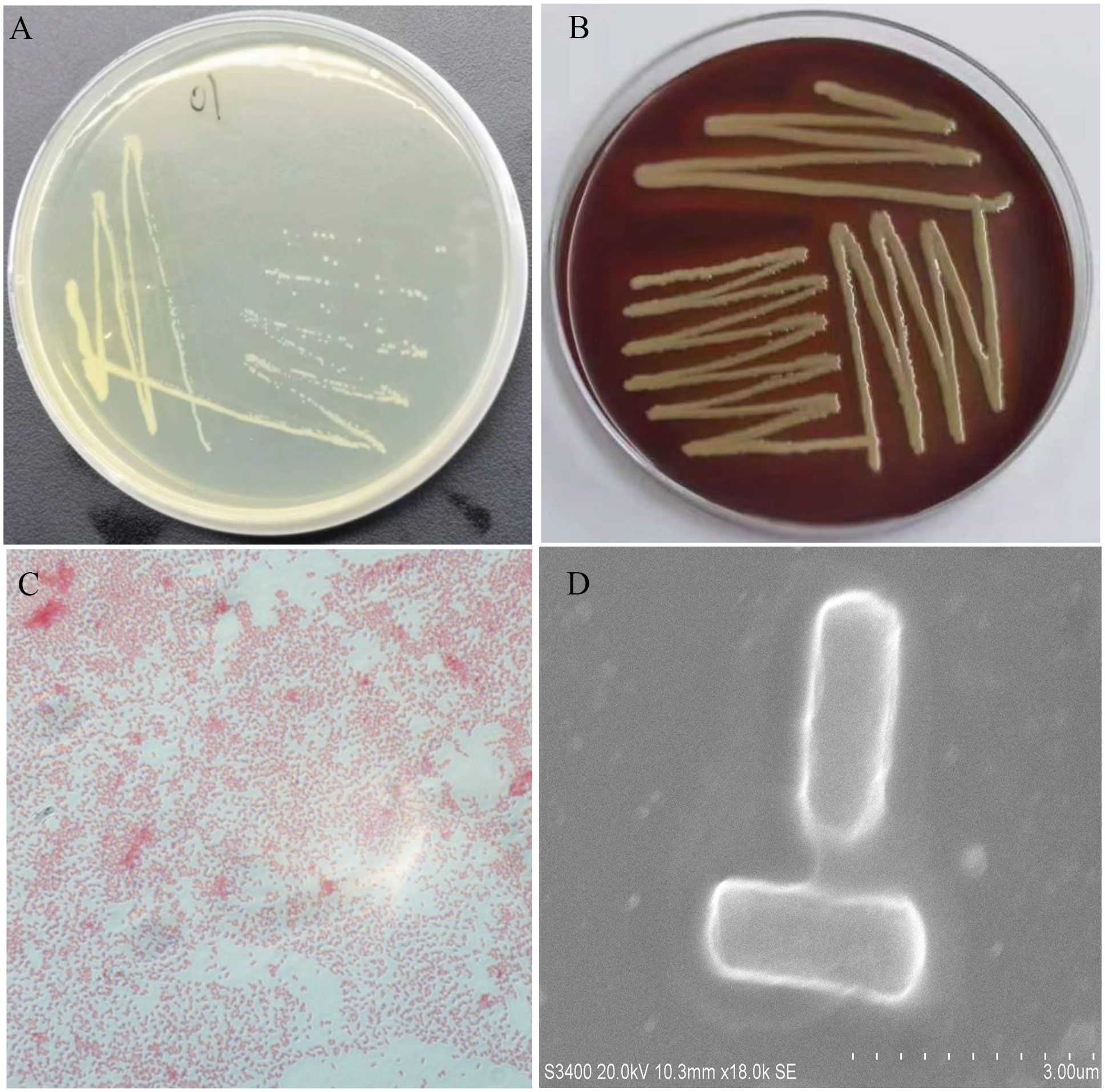
Figure 2 Morphological features of NFEM01 isolated from the occurrence of bullfrog disease cultured in Guangxi. (A) NFEM01 single colonies, (B) the hemolytic activity of the strain was determined according to the hemolytic area around the colony, (C) Gram stained under a light microscope, and (D) individual cells under a scanning electron microscope.
Physiological and chemical characteristics
The results of the physiological and chemical characteristics of NFEM01 are shown in Table 2. The NFEM01 isolate was negative for glucose, lactose, maltose, and mannose utilization but was positive for honey disaccharide, cellulose disaccharide, xylose, arabinose, rhamnose, and sucrose. The hydrolysis of urea was positive, while that of citrate was negative.
Molecular identification
Portions 16S rRNA, rpoB, and mutT gene sequences were amplified from the isolate, sequenced, and the latter submitted to GenBank with the registration number PRJNA893762. Analysis of 16S r RNA, rpoB, and mutT genes sequences by BLAST in NCBI was performed and sequences with high sequence identity were identified. According to phylogenetic characteristics, together with 16S rRNA (Figure 3), rpoB (Figure 4A), and mutT (Figure 4B) gene sequence analysis, the sequences of these genes were clustered with E. miricola and showed 98.86%, 99.85%, and 100% similarity to the FB210601, FL160902, and FL160902 strains, respectively. The NFEM01 was identified as E. miricola.
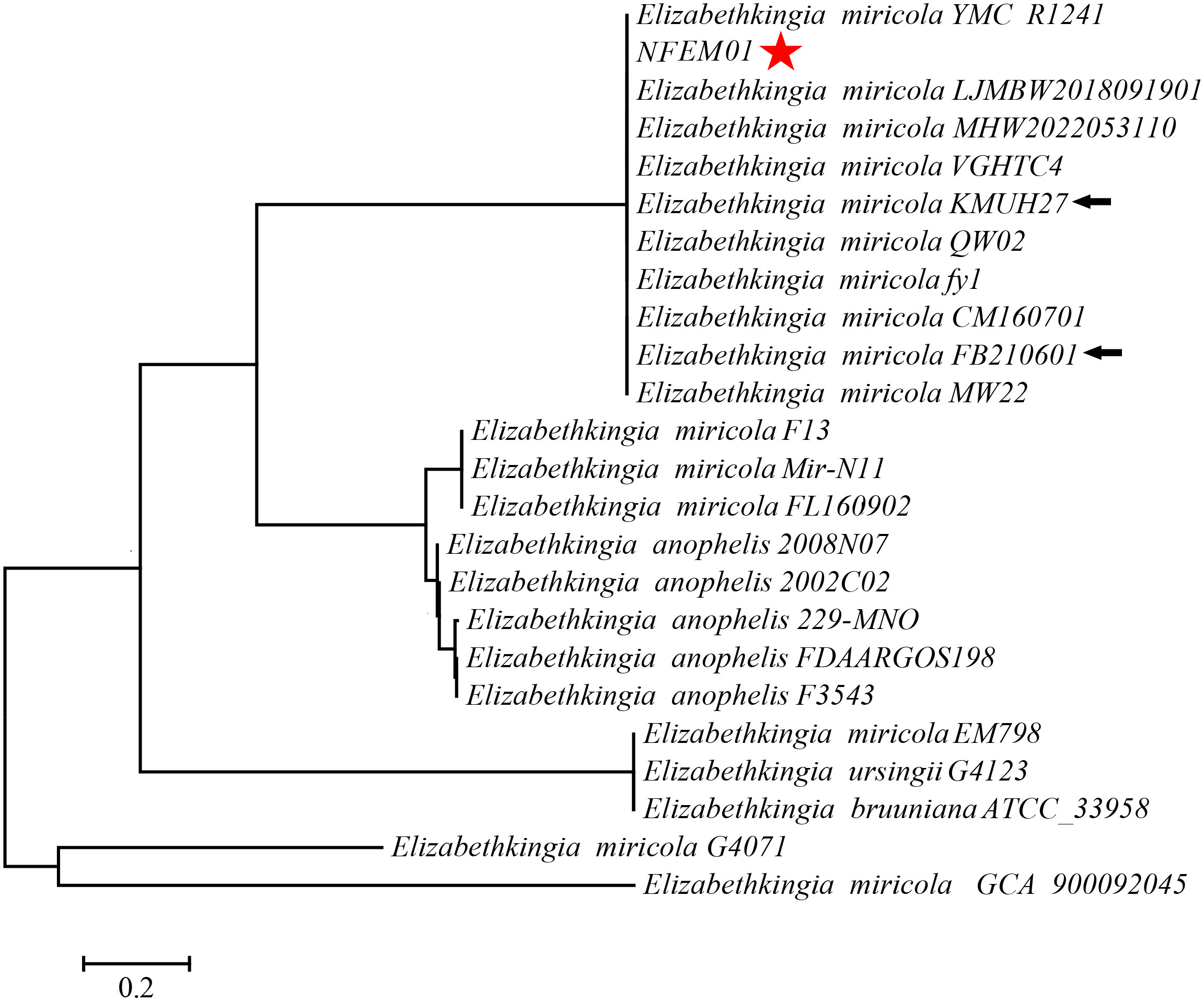
Figure 3 A phylogenetic tree was constructed based on the 16S rRNA gene of NFEM01 by neighbor-joining method and displays the percentage of bootstrap values on each branch point (1000 copies). The scale represents 0.02 nucleotide substitutions each site. Star refers to the strain from this study. The arrow stands for the type strain of the species.
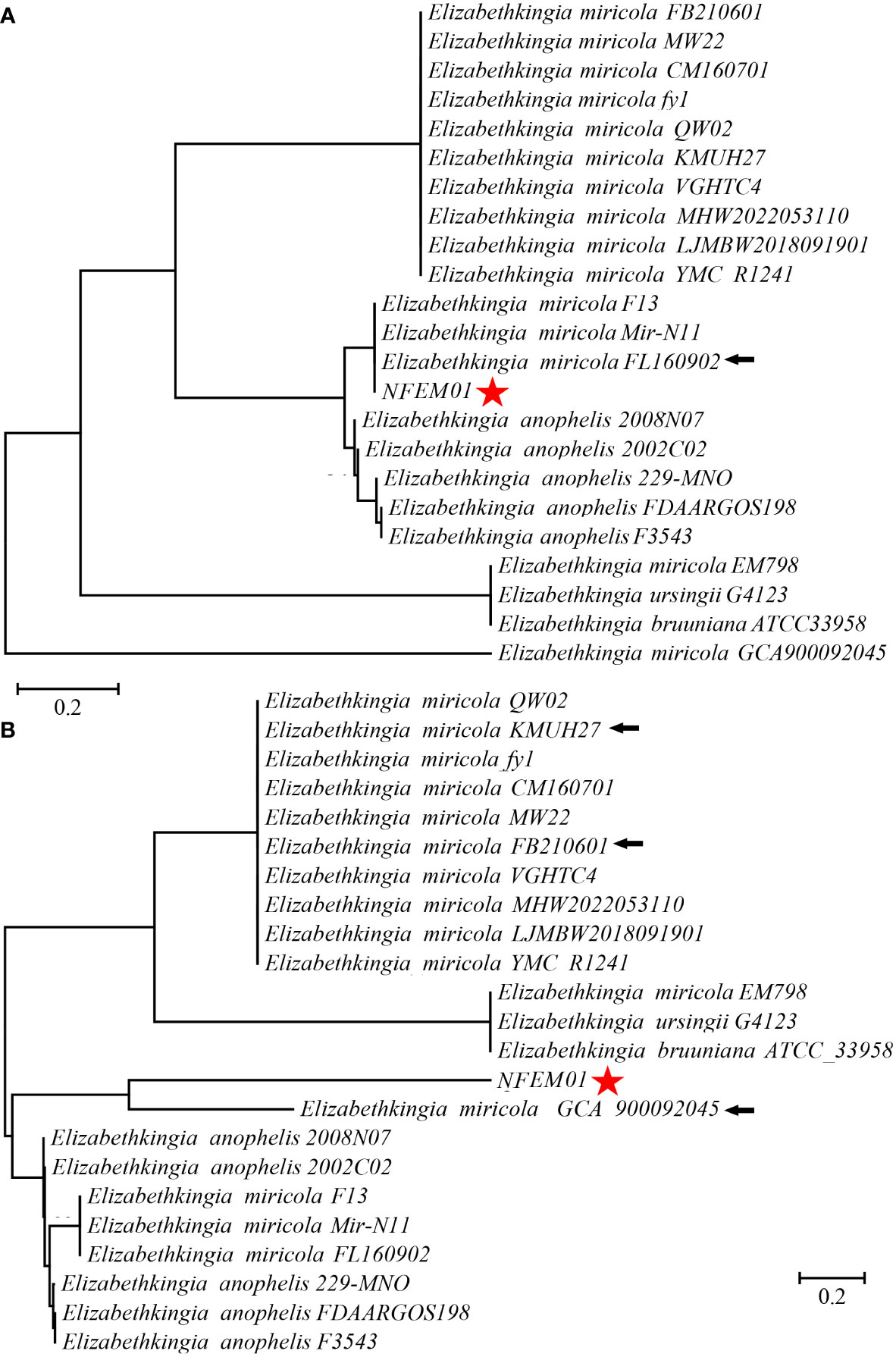
Figure 4 (A) Phylogenetic tree analysis of NFEM01 using the rpoB gene. (B) Phylogenetic tree analysis of NFEM01 using the mutT gene. Star refers to the strain from this study. The arrow stands for the type strain of the species.
Antibiotic susceptibility
The results of the drug sensitivity test for 12 antibiotics showed that the isolated NFEM01 was highly sensitive to mequindox, rifampicin, enrofloxacin, nitrofural, and oxytetracycline but showed strong resistance to gentamicin, florfenicol, neomycin, penicillin, amoxicillin, doxycycline, and sulfamonomethoxine (Table 3).
Artificial infection
The bullfrogs began to die 2 days after the highest dose of artificial infection (5.18 × 109 CFU·mL-1). High mortality occurred 3 days after inoculation of 5.18 × 105, 5.18 × 106, 5.18 × 107, 5.18 × 108, 5.18 × 109 bacteria. The bullfrog mortality rates were 60%, 60%, 100%, 100%, and 100%, respectively (Figure 5). The survival rate of the highest dose group was significantly lower than that of the control group (P < 0.05). Mortality was not observed in the control group. Bullfrog death after artificial infection is similar to natural pathogen-induced death, including congestion and hemorrhage of the skin of the abdomen and hind limb, spleen swelling, ascites, liver swelling, and gastrointestinal congestion. NFEM01 was isolated from all dead infected bullfrogs, while NFEM01 was not isolated from the control bullfrogs.
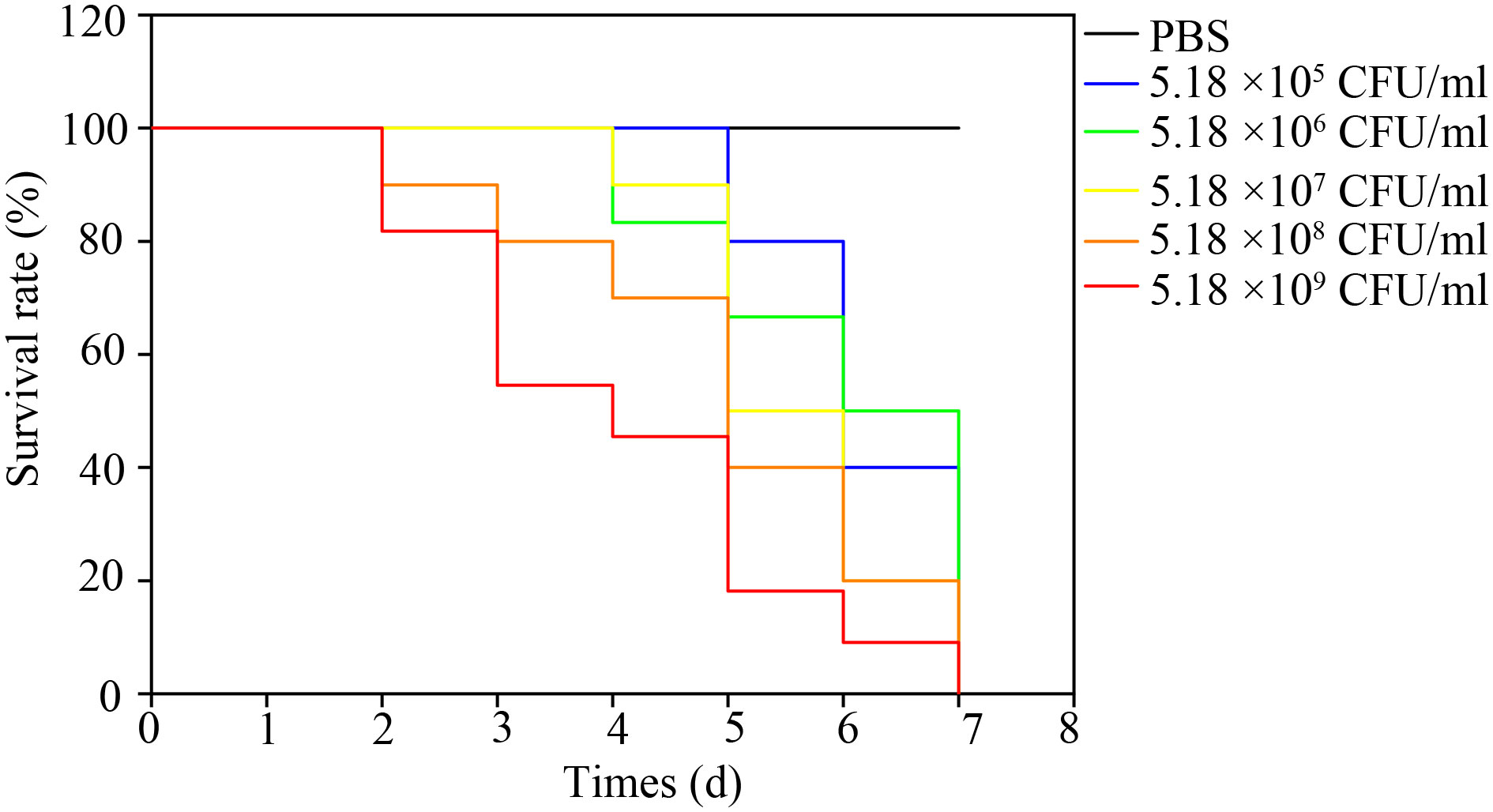
Figure 5 Kaplan-Meier survival curves of bullfrogs infected with different doses of NFEM01. Control group/phosphate-buffered-saline (PBS), the concentrations in the infection group were 5.18 × 104, 5.18 × 105, 5.18 × 106, 5.18 × 107, 5.18 × 108, 5.18 × 109 CFU·mL-1 NFEM01.
Histopathological observation
Histologically, the pathological changes of diseased bullfrogs in the brain (Figure 6A), liver (Figure 6C), spleen (Figure 6E), kidney (Figure 6G), and intestine (Figure 6I) were observed, and the most obvious brain lesions were the thickening of the ventricles, the degeneration of the membrane tissue, and the sharp increase of neuroglia, showing typical pathological changes of encephalitis and meningitis, compared with the healthy bullfrog brain (Figure 6B), liver (Figure 6D), spleen (Figure 6F), kidney (Figure 6H), and intestine (Figure 6J).
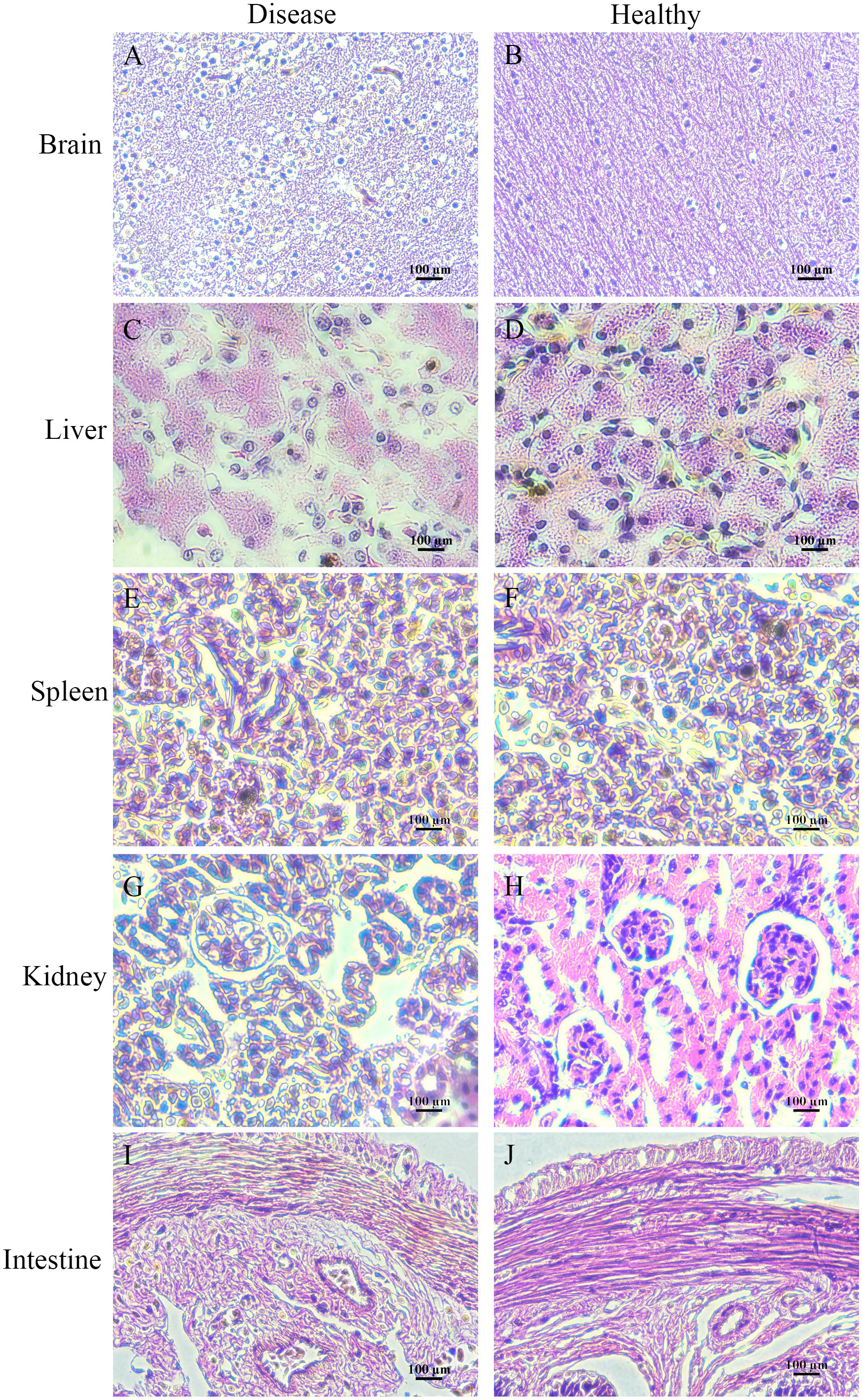
Figure 6 Histologically diseased bullfrog liver (C), spleen (E), kidney (G), intestine (I), and brain (A) were observed in comparison with tissue sections of healthy bullfrog liver (D), spleen (F), kidney (H), intestine (J), and brain (B), where the most obvious signs of brain injury are ventricular thickening, membranous tissue degeneration, and a sharp increase in glia, showing the pathological changes typical of encephalitis and meningitis.
Discussion
Many studies have shown that E. miricola can cause clinical meningitis in addition to pneumonia and meningitis in newborns, children, and the elderly (Dziuban et al., 2018). The immunocompromised are particularly at high risk. In addition to humans and poultry, E. miricola bacteria also infect many aquatic animals (Jacobs and Chenia, 2011; Laith et al., 2017). In recent years, E. miricola bacteria have become an emerging pathogen of frog farming, especially bullfrog farming (Liu et al., 2022). The infected bullfrog shows disease symptoms such as severe torticollis; curvature of the spine; and enlargement of the liver, spleen, and kidneys, which are also reported in several other frog species (Xie et al., 2009; Lei et al., 2019). This phenomenon also occurs in black-spotted frogs (Hu et al., 2017). In the current study, the NFEM01 strain was isolated from the brain of bullfrogs with EMD in Nanning, Guangxi. The surface of a typical NFEM01 colony was smooth and wet and the edge was neat and white, which was consistent with E. miricola isolated from the spiny frog (Lei et al., 2019). NFEM01 has beta-hemolytic activity, which indicates that NFEM01 has strong pathogenic potential.
In the API® 20E test, the NFEM01 strain showed similar characteristics to those reported previously (Huang et al., 2019), but differences between isolates of Elizabethkingia spp. in trypsin response were observed. Some of the characteristics of Elizabethkingia spp. have been summarized. They can all produce catalase, phosphatases, galactosidases, and indole, whereas they cannot hydrolyze starch, use malonate, and ferment galactose, sorbitol, inositol, and salicylic acid. However, some features are variable in the same species and the phenotypic similarities between known species challenge the correct identification of clinical isolates (Nicholson et al., 2018). The genus Elizabethkingia is genetically heterogeneous, and the identification by phenotypic similarity is challenging for the accurate identification of clinical isolates (Bruun and Ursing, 1987).
In previous works, the 16S rRNA gene was used for the clinical reports of most cases (Frank et al., 2013; Chew et al., 2018). However, there are five copies of the 16S rRNA gene in Elizabethkingia spp., and there are some differences between them (Nicholson et al., 2018). Therefore, it is difficult to distinguish Elizabethkingia spp. from the 16S rRNA gene sequence alone, so other methods to support identification are needed (Chew et al., 2018; Lei et al., 2019). The rpoB gene sequencing is superior to other gene targets because it has a higher resolution than 16S rRNA gene sequencing, and is used for delineating new bacterial species (Adékambi et al., 2009; Turton et al., 2010). Nicholson et al. (2018) first proposed using the rpoB gene to identify Elizabethkingia species. Subsequently, Kenna et al. studied the distribution of Elizabethkingia species using rpoB gene sequencing. In their study, 43 isolates from 38 patients formed a cluster with E. miricola and E. bruuniana sp. nov. (Kenna et al., 2018). In a separate study based on 16S rRNA and rpoB gene sequencing, the authors identified six patients infected with E. bruuniana between 2005 and 2017 (Lin et al., 2019). On this basis, we further verified and confirmed the mutT gene of E. miricola by amplification and sequencing. Zhang et al. (2015) established a real-time fluorescent quantitative PCR system based on mutT gene amplification, which could specifically identify E. miricola and had no nonspecific amplification with many bacteria. In our study, a combination of 16S rRNA, rpoB, and mutT genes was used to identify E. miricola isolated from the bullfrog, eliminating a possible error caused by single 16S rRNA-based identification.
Previous antibiotic susceptibility testing revealed E. miricola was resistant to erythromycin and oxytetracycline (Colapietro et al., 2016; Han et al., 2017; Gao et al., 2021). Elizabethkingia spp. is highly resistant to various antibiotics, leading to fewer choices of therapeutic drugs. Because of this, clinically, patients with Elizabethkingia spp. infections have high mortality (Opota et al., 2017). In this study, the NFEM01 was resistant to the majority of antibiotics, including gentamicin, florfenicol, neomycin, penicillin, amoxicillin, doxycycline, and sulfamonomethoxine. Therefore, the choice of antibiotics to treat EMD is limited. The NFEM01 isolate showed high resistance to multiple antibiotics similar to previously reported isolates from the Chinese spiny frog (Lei et al., 2019). The main reason for the multi-drug resistance of Elizabethkingia spp. is that there are many natural resistance genes on its chromosome, which can produce antibiotic-inactivating enzymes and lead to corresponding antibiotic resistance, for example, Metallo-β-lactamases (MBLs) (Opota et al., 2017). The use of Chinese herbal medicines may be a potentially effective approach (Li et al., 2021).
In our study, the mortality of the infected bullfrog was 40% and 100%, respectively when 105 and 107 CFU·mL-1 NFEM01 were injected. These results are similar to those of another study, which found that the mortality rate of infected black-spotted frogs was 50% and 70% when injected with E. miricola FL160902 at 107 and 108 CFU·mL-1, respectively (Hu et al., 2017). Another study indicated that the mortality rates of Chinese spiny frogs after infection at 106, 107, and 108 CFU·mL-1 were 50%, 80%, and 100%, respectively (Lei et al., 2019). These traits suggest that the strains isolated in these studies all show strong pathogenicity to frogs. However, in previous studies, mortality after infection at 108 CFU·mL-1 was 80% and 33.3%, with relatively low lethality in the black-spotted frog (Huang et al., 2019). These studies suggest that different bacterial strains from amphibians may have different pathogenicity or that different amphibian species have differing susceptibility.
Conclusion
In summary, E. miricola was confirmed as the pathogenic bacterium isolated from the brain of bullfrogs with meningitis-like disease. E. miricola was first isolated from the bullfrog in Guangxi and is highly pathogenic to bullfrogs. This provides a reference for further study of the pathogenesis mechanism, propagation, and prevention of the disease.
Data availability statement
The datasets presented in this study can be found in online repositories. The names of the repository/repositories and accession number(s) can be found in the article/supplementary material.
Ethics statement
The animal study was reviewed and approved by the ethics committee of Guangxi Academy of Sciences.
Author contributions
PL, DW, and ZX conceived and designed the research. YC, SX, WL, QY, SYH, SSH, and JS performed the experiments and analyzed the data. DW wrote the manuscript. All authors contributed to the article and approved the submitted version.
Funding
This work was financially supported by grants from the National Natural Science Foundation of China (U20A20102), the Agricultural Science and Technology project of Guangxi (Z2022167), the China Agriculture Research System of MOF and MARA (CARS-46), the Guangxi Innovation Team Project of National Modern Agricultural Industrial Technology System (nycytxgxcxtd-2021-08-02), and the Guangxi mangrove coastal wetland ecological protection and sustainable use of small highland talents project (BGMRC202104).
Conflict of interest
The authors declare that the research was conducted in the absence of any commercial or financial relationships that could be construed as a potential conflict of interest.
Publisher’s note
All claims expressed in this article are solely those of the authors and do not necessarily represent those of their affiliated organizations, or those of the publisher, the editors and the reviewers. Any product that may be evaluated in this article, or claim that may be made by its manufacturer, is not guaranteed or endorsed by the publisher.
References
Adékambi, T., Drancourt, M., Raoult, D. (2009). The rpoB gene as a tool for clinical microbiologists. Trends Microbiol. 17 (1), 37–45. doi: 10.1016/j.tim.2008.09.008
Akmentins, M. S., Cardozo, D. E. (2010). American Bullfrog Lithobates catesbeianus (Shaw 1802) invasion in Argentina. Biol. Invasions 12 (4), 735–737. doi: 10.1007/s10530-009-9515-3
Bordelo, J., Viegas, C., Coelho, C., Poeta, P. (2016). First report of bacteremia caused by Elizabethkingia meningoseptica in a dog. Can. Vet. J. 57 (9), 994. Available at: https://europepmc.org/article/PMC/4982576
Bozzola, J. J. (2014). “Conventional specimen preparation techniques for scanning electron microscopy of biological specimens,” in Electron microscopy: Methods and protocols. Ed. Kuo, J. (Totowa, NJ: Humana Press), 133–150.
Bruun, B., Ursing, J. (1987). Phenotypic characterization of Flavobacterium meningosepticum strains identified by DNA-DNA hybridization. Acta Pathol. Microbiol. Immunol. Scand. B 95 (1), 41–47. doi: 10.1111/j.1699-0463.1987.tb03085.x
Chew, K. L., Cheng, B., Lin, R. T. P., Teo, J. W. P. (2018). Elizabethkingia anophelis is the dominant Elizabethkingia species found in blood cultures in Singapore. J. Clin. Microbiol. 56 (3), e01445-17. doi: 10.1128/jcm.01445-17
Colapietro, M., Endimiani, A., Sabatini, A., Marcoccia, F., Celenza, G., Segatore, B., et al. (2016). BlaB-15, a new BlaB metallo-β-lactamase variant found in an Elizabethkingia miricola clinical isolate. Diagn. Micr Infect. Dis. 85 (2), 195–197. doi: 10.1016/j.diagmicrobio.2015.11.016
Cunningham, A., Langton, T., Bennett, P., Lewin, J., Drury, S., Gough, R., et al. (1996). Pathological and microbiological findings from incidents of unusual mortality of the common frog (Rana temporaria). Philos. Trans. R Soc. Lond B Biol. Sci. 351, 1539–1557. doi: 10.1098/rstb.1996.0140
Dziuban, E. J., Franks, J. L., So, M., Peacock, G., Blaney, D. D. (2018). Elizabethkingia in children: A comprehensive review of symptomatic cases reported from 1944 to 2017. Clin. Infect. Dis. 67 (1), 144–149. doi: 10.1093/cid/cix1052
FAO (2023). Cultured aquatic species information programme. rana catesbeiana (Rome: Fisheries and aquaculture department). Available at: https://www.fao.org/fishery/en/culturedspecies/rana_catesbeiana/en.
Frank, T., Gody, J. C., Nguyen, L. B., Berthet, N., Le Fleche-Mateos, A., Bata, P., et al. (2013). First case of Elizabethkingia anophelis meningitis in the central African republic. Lancet 381 (9880), 1876. doi: 10.1016/s0140-6736(13)60318-9
Furyk, J. S., Swann, O., Molyneux, E. (2011). Systematic review: neonatal meningitis in the developing world. Trop. Med. Int. Health 16 (6), 672–679. doi: 10.1111/j.1365-3156.2011.02750.x
Gao, H., Li, T., Feng, L., Zhang, S. (2021). Elizabethkingia miricola causes intracranial infection: a case study. Front. Med-Lausanne 8. doi: 10.3389/fmed.2021.761924
Han, M. S., Kim, H., Lee, Y., Kim, M., Ku, N. S., Choi, J. Y., et al. (2017). Relative prevalence and antimicrobial susceptibility of clinical isolates of Elizabethkingia species based on 16S rRNA gene sequencing. J. Clin. Microbiol. 55 (1), 274–280. doi: 10.1128/jcm.01637-16
Hem, S., Jarocki, V. M., Baker, D. J., Charles, I. G., Drigo, B., Aucote, S., et al. (2022). Genomic analysis of Elizabethkingia species from aquatic environments: Evidence for potential clinical transmission. Curr. Res. Microb. Sci. 3, 100083. doi: 10.1016/j.crmicr.2021.100083
Hu, R., Yuan, J., Meng, Y., Wang, Z., Gu, Z. (2017). Pathogenic Elizabethkingia miricola infection in cultured black-spotted frogs, China 2016. Emerg. Infect. Dis. 23 (12), 2055–2059. doi: 10.3201/eid2312.170942
Hu, R., Zhang, Q., Gu, Z. (2020). Whole-genome analysis of the potentially zoonotic Elizabethkingia miricola FL160902 with two new chromosomal MBL gene variants. J. Antimicrob. Chemother. 75 (3), 526–530. doi: 10.1093/jac/dkz480
Huang, X., Feng, Y., Tang, H., Xiong, G., Li, L., Yang, Y., et al. (2019). Candidate animal disease model of elizabethkingia spp. infection in humans, based on the systematic pathology and oxidative damage caused by E. miricola in pelophylax nigromaculatus. Oxid. Med. Cell Longev 2019, 6407524. doi: 10.1155/2019/6407524
Jacobs, A., Chenia, H. Y. (2011). Biofilm formation and adherence characteristics of an Elizabethkingia meningoseptica isolate from oreochromis mossambicus. Ann. Clin. Microbiol. Antimicrob. 10, 16. doi: 10.1186/1476-0711-10-16
Jorgensen, J. H., Turnidge, J. D. (2015). Susceptibility test methods: dilution and disk diffusion methods. In manual of clinical microbiology. In manual of clinical microbiology (eds Jorgensen, J.H., Carroll, K.C., Funke, G., Pfaller, M.A., Landry, M.L., Richter., S.S., et al Am. Soc. Microbiol., pp1253–1273. doi: 10.1128/9781555817381.ch71
Kenna, D. T. D., Fuller, A., Martin, K., Perry, C., Pike, R., Burns, P. J., et al. (2018). rpoB gene sequencing highlights the prevalence of an e. miricola cluster over other Elizabethkingia species among UK cystic fibrosis patients. Diagn. Microbiol. Infect. Dis. 90 (2), 109–114. doi: 10.1016/j.diagmicrobio.2017.10.014
Laith, A. A., Mazlan, A. G., Ambak, M. A., Jabar, A., Najiah, M. (2017). Isolation and indentification of Elizabethkingia meningoseptica from diseased African catfish Clarias gariepinus. J. Microb. Biotec Food 6, 1070–1076. doi: 10.15414/jmbfs.2017.6.4.1070-1076
Lei, X. P., Yi, G., Wang, K. Y., OuYang, P., Chen, F., Huang, X. L., et al. (2019). Elizabethkingia miricola infection in Chinese spiny frog (Quasipaa spinosa). Transbound Emerg. Dis. 66 (2), 1049–1053. doi: 10.1111/tbed.13101
Li, P., Liu, M., Xiao, H., Yu, Q., Xu, Y. (2021). Application of medicinal plants in prevention and control of aquatic animal pathogens. J. South Agric. 52 (7), 2015–2024. doi: 10.3969/j.issn.2095-1191.2021.07.032
Li, X., Liu, Y., Yang, X., Yang, Y., Ai, X. (2022). Analysis on pathogens of frogs with crooked head, broken head or white eye. Acta Agric. Univ Zhejiangensis 34 (8), 1617–1625. doi: 10.3969/j.issn.1004-1524.2022.08.06
Li, P., Yu, Q., Qin, X., Li, F., Chen, X., Dong, D., et al. (2018). Current situation and research prospects of disease control technology system of mariculture in beibu gulf, guangxi. Guangxi Sci. 25 (1), 15–25. doi: 10.13656/j.cnki.gxkx.20180125.001
Lin, J. N., Lai, C. H., Yang, C. H., Huang, Y. H. (2019). Elizabethkingia infections in humans: from genomics to clinics. Microorganisms 7 (9), 295. doi: 10.3390/microorganisms7090295
Liu, C., Ma, J., Sun, J., Su, Y., Jiang, B., Li, W., et al. (2022). Isolation,identification and characterization of Elizabethkingia miricola from Lithobates catesbeiana. J. Zhongkai Univ Agric. Eng. 35 (02), 8–14. doi: 10.3969/i.issn.1674-5663.2022.02.002
Mauel, M. J., Miller, D. L., Frazier, K. S., Hines, M. E. (2002). Bacterial pathogens isolated from cultured bullfrogs (Rana castesbeiana). J. Vet. Diagn. Invest. 14, 431–433. doi: 10.1177/104063870201400515
Moore, L. S., Owens, D. S., Jepson, A., Turton, J. F., Ashworth, S., Donaldson, H., et al. (2016). Waterborne Elizabethkingia meningoseptica in adult critical care. Emerg. Infect. Dis. 22 (1), 9–17. doi: 10.3201/eid2201.150139
Nicholson, A. C., Gulvik, C. A., Whitney, A. M., Humrighouse, B. W., Graziano, J., Emery, B., et al. (2018). Revisiting the taxonomy of the genus elizabethkingia using whole-genome sequencing, optical mapping, and MALDI-TOF, along with proposal of three novel Elizabethkingia species: Elizabethkingia bruuniana sp. nov., Elizabethkingia ursingii sp. nov., and Elizabethkingia occulta sp. nov. Anton Leeuw Int. J. G 111 (1), 55–72. doi: 10.1007/s10482-017-0926-3
Opota, O., Diene, S. M., Bertelli, C., Prod'hom, G., Eckert, P., Greub, G. (2017). Genome of the carbapenemase-producing clinical isolate Elizabethkingia miricola EM_CHUV and comparative genomics with Elizabethkingia meningoseptica and Elizabethkingia anophelis: evidence for intrinsic multidrug resistance trait of emerging pathogens. Int. J. Antimicrob. Ag 49 (1), 93–97. doi: 10.1016/j.ijantimicag.2016.09.031
Pasteris, S. E., Buhler, M. I., Nader-Macias, M. E. (2006). Microbiological and histological studies of farmed-bullfrog (Rana catesbeiana) tissues displaying red-leg syndrome. Aquaculture (Amsterdam Netherlands) 251 (1), 11–18. doi: 10.1016/j.aquaculture.2005.05.007
Schloegel, L., Mosterio, C., James, T., Hipolito, M., Longcore, J., Hyatt, A., et al. (2010). The north American bullfrog as a reservoir for the spread of batrachochytrium dendrobatidis in Brazil. Anim. Conserv. 13, 53–61. doi: 10.1111/j.1469-1795.2009.00307.x
Shayegani, M., Maupin, P. S., McGlynn, D. M. (1978). Evaluation of the API 20E system for identification of nonfermentative gram-negative bacteria. J. Clin. Microbiol. 7 (6), 539–545. doi: 10.1128/jcm.7.6.539-545.1978
Trimpert, J., Eichhorn, I., Vladimirova, D., Haake, A., Schink, A.-K., Klopfleisch, R., et al. (2021). Elizabethkingia miricola infection in multiple anuran species. Transbound Emerg. Dis. 68 (2), 931–940. doi: 10.1111/tbed.13761
Turton, J. F., Shah, J., Ozongwu, C., Pike, R. (2010). Incidence of acinetobacter species other than a. baumannii among clinical isolates of acinetobacter: evidence for emerging species. J. Clin. Microbiol. 48 (4), 1445–1449. doi: 10.1128/jcm.02467-09
Vancanneyt, M., Segers, P., Hauben, L., Hommez, J., Devriese, L. A., Hoste, B., et al. (1994). Flavobacterium meningosepticum, a pathogen in birds. J. Clin. Microbiol. 32 (10), 2398–2403. doi: 10.1128/jcm.32.10.2398-2403.1994
Weisburg, W. G., Barns, S. M., Pelletier, D. A., Lane, D. J. (1991). 16S ribosomal DNA amplification for phylogenetic study. J. Bacteriol 173 (2), 697–703. doi: 10.1128/jb.173.2.697-703.1991
Wu, Z. J., Wang, Y. P., Li, Y. M. (2004). Natural populations of bullfrog (Rana catesbeiana) and their potential threat in the east of zhejiang province. Biodiv Sci. 12 (4), 441–446. doi: 10.17520/biods.2004054
Xie, Z. Y., Zhou, Y. C., Wang, S. F., Mei, B., Xu, X. D., Wen, W. Y., et al. (2009). First isolation and identification of Elizabethkingia meningoseptica from cultured tiger frog, rana tigerina rugulosa. Vet. Microbiol. 138, 140–144. doi: 10.1016/j.vetmic.2009.02.011
Yu, Q., Liu, M., Wei, S., Qin, X., Qin, Q., Li, P. (2021). Research progress and prospects for the use of aptamers in aquaculture biosecurity. Aquaculture 534, 736257. doi: 10.1016/j.aquaculture.2020.736257
Zajmi, A., Teo, J., Yeo, C. C. (2022). Epidemiology and characteristics of elizabethkingia spp. infections in southeast Asia. Microorganisms 10 (5), 882. doi: 10.3390/microorganisms10050882
Zhang, Q., Hu, R., Gu, Z. (2020). A real-time PCR assay for detection of emerging infectious Elizabethkingia miricola. . Mol. Cell Probes 52, 101571. doi: 10.1016/j.mcp.2020.101571
Zhang, C. X., Huang, K. K., Wang, L., Song, K., Zhang, L., Li, P. (2015). Apparent digestibility coefficients and amino acid availability of common protein ingredients in the diets of bullfrog, Rana (Lithobates) catesbeiana. Aquaculture 437, 38–45. doi: 10.1016/j.aquaculture.2014.11.015
Keywords: bullfrog (Rana catesbeiana), meningitis-like disease, Elizabethkingia miricola, isolation and identification, antimicrobial resistance
Citation: Wei D, Cheng Y, Xiao S, Liao W, Yu Q, Han S, Huang S, Shi J, Xie Z and Li P (2023) Natural occurrences and characterization of Elizabethkingia miricola infection in cultured bullfrogs (Rana catesbeiana). Front. Cell. Infect. Microbiol. 13:1094050. doi: 10.3389/fcimb.2023.1094050
Received: 09 November 2022; Accepted: 20 February 2023;
Published: 14 March 2023.
Edited by:
Bo Peng, Sun Yat-sen University, ChinaReviewed by:
Mehdi Fatahi-Bafghi, Shahid Sadoughi University of Medical Sciences and Health Services, IranLeon Cantas, Hammerfest, Norway
Copyright © 2023 Wei, Cheng, Xiao, Liao, Yu, Han, Huang, Shi, Xie and Li. This is an open-access article distributed under the terms of the Creative Commons Attribution License (CC BY). The use, distribution or reproduction in other forums is permitted, provided the original author(s) and the copyright owner(s) are credited and that the original publication in this journal is cited, in accordance with accepted academic practice. No use, distribution or reproduction is permitted which does not comply with these terms.
*Correspondence: Zongsheng Xie, am9uc29ueGllQDEyNi5jb20=; Pengfei Li, cGZsaTIwMTZAZ3hhcy5jbg==
†These authors have contributed equally to this work