- 1Department of Medical Microbiology, School of Laboratory Medicine and Medical Science, University of KwaZulu-Natal, Durban, South Africa
- 2Centre for the AIDS Programme of Research in South Africa (CAPRISA), Durban, South Africa
Cervical cancer disproportionately affects women of reproductive age, with 80% of cases occurring in low- and middle-income countries. Persistent infection with high-risk human papillomavirus (HPV) genotypes has been described as the most common non-systemic biological risk factor for the development of cervical cancer. The mucosal immune system plays a significant role in controlling HPV infection by acting as the first line of host defense at the mucosal surface. However, the virus can evade host immunity using various mechanisms, including inhibition of the antiviral immune response necessary for HPV clearance. Pro-inflammatory cytokines and the vaginal microbiome coordinate cell-mediated immune responses and play a pivotal role in modulating immunity. Recently, diverse vaginal microbiome (associated with bacterial vaginosis) and genital inflammation have emerged as potential drivers of high-risk HPV positivity and disease severity in women. The potential role of these risk factors on HPV recurrence and persistence remains unclear. This article reviews the role of cellular or cytokine response and vaginal microbiome dysbiosis in the clearance, persistence, and recurrence of HPV infection.
Introduction
Human Papillomavirus (HPV), a primary cause of genital warts and cervical cancer, is the most common sexually transmitted infection (Burchell et al., 2006). Despite efforts to implement prophylactic HPV vaccination in young women, cervical cancer is the fourth most common cancer among sexually active women globally (Sung et al., 2021). A majority (~90%) of women clear HPV infections spontaneously within 6–18 months (Myers et al., 2000; Plummer et al., 2007), with very few progressions from precancerous lesion to invasive cervical cancer (Stanley, 2008). Several studies have hypothesized that host defense mechanisms, the genital microbiome, and other factors in the female genital tract play a role in the clearance and persistence of HPV, including the risk of developing cervical cancer (Moscicki et al., 2001; Daud et al., 2011; Łaniewski et al., 2018; Liebenberg et al., 2019; Onywera et al., 2019).
Innate and adaptive immune responses represent the first line of host defense at the mucosal surface against pathogens such as HPV (Lieberman et al., 2008, Barros et al., 2018). Several studies have demonstrated a relationship between genital mucosal cytokine concentrations and the control or elimination of HPV infection in the cervix (Liebenberg et al., 2019, 12–Li et al., 2019). It is hypothesized that cytokine response occurs within days after the establishment of an HPV infection and is subsequently reversed when HPV clearance has been effectively achieved by appropriate effector cells (12, O'Byrne and Dalgleish, 2001; Balkwill and Mantovani, 2001; Hawes and Kiviat, 2002; Amaral et al., 2006). The initial response against HPV infection includes mature antigen presenting cells (APCs) that secret cytokines and contribute in activation and recruitment of other immune cells to the site of infection (Stanley, 2006). Upon interaction with mature APCs, naïve CD4+ and CD8+ T cells differentiate into various T helper effector lineages and cytotoxic T lymphocytes (CTLs), respectively, which needed for the effective clearance of HPV (Steele et al., 2002; Stanley, 2006). However, these cells do not entirely prevent disease progression. HPV can use various immune evasion mechanisms to limit anti-viral activity of immune response, resulting in HPV infection tolerance in the host’s immune system. HPV infection could affect the differentiation of monocytes into mature DCs and distinctively affect the functionality of CD4+/CD8+, and regulatory T cells (Song et al., 2015). Increased secretion of anti-inflammatory cytokine IL-10 in Th2 response has been associated with compromised innate and adaptive immune defense and cervical lesion progression during high-risk HPV infection (Peghini et al., 2012; Scott et al., 2013; Lin et al., 2019). Although studies have demonstrated the anti-viral activities of immune cells, less is known about the cell-mediated mucosal immune response to HPV incidence, persistence, and clearance.
Emerging evidence suggests that women with a low relative abundance of vaginal Lactobacillus species and high proportions of the Gardnerella, Sneathia, and Atopobium genera are less likely to clear HPV infection (Gillet et al., 2011; Gao et al., 2013; Brotman et al., 2014; Mitra et al., 2015; Audirac-Chalifour et al., 2016; Łaniewski et al., 2018; Norenhag et al., 2020). Two recent meta-analyses summarizing findings from several microscopy or molecular studies have reported that women with Lactobacillus-enriched microbiomes were less likely to acquire HPV infection compared to women with overgrowth of bacterial vaginosis (BV)-linked bacteria (Gardnerella, Atopobium, and Prevotella) (Brusselaers et al., 2019; Norenhag et al., 2020). A Lactobacillus gasseri-enriched microbiome was associated with rapid rates of HPV clearance (Brotman et al., 2014; Di Paola et al., 2017), while a L. iners dominant microbiome was commonly reported in women presenting with cervical intraepithelial neoplasia (CIN). Several studies further reported an association between BV-linked microbial communities (Gardnerella, Prevotella, Dialister, Streptococcus, Ureaplasma, Megasphaera, and Mycoplasma) and persistent infection with high-risk HPV that may result in the development of CIN (Norenhag et al., 2020; Wei et al., 2020). Furthermore, specific vaginal microbiota may modulate host immune responses, including critical antiviral and anti-tumor immunity components in the female genital tract (Doerflinger et al., 2014; Anahtar et al., 2015). Diverse microbial communities have been closely associated with altered innate immune responses, host susceptibility to infection (Anahtar et al., 2015; Gosmann et al., 2017; Mtshali et al., 2021), and development of cervical diseases, but proven causality remains unclear.
While there is evidence of a relationship between the vaginal microbiome, host immune responses, and HPV infection, a link between the vaginal microbiome, host responses, and the progression to HPV-associated cervical cancer remains unclear. Therefore, understanding the concept of vaginal microbiome fluctuations and associated host immune responses during HPV infection stages could shed light on possible mechanisms associated with cervical carcinogenesis. This review summarizes the role of vaginal microbiome fluctuations, cytokine, and cellular immune response during HPV infection, persistent and clearance.
HPV Genotypes and Screening
HPV is a member of the non-enveloped double-stranded DNA papillomaviridae family that infects squamous epithelium found beneath the foreskin of the penis, the scrotum, vulva, vagina, cervix, skin, and anus (Doorbar et al., 2015; Harden and Munger, 2017). The papillomavirus structure is icosahedral with approximately 50–60 nm and contains 8000 base pairs (Harden and Munger, 2017; Gupta and Mania-Pramanik, 2019). HPV infection is classified into five major genera, Alphapapillomavirus, Gammapapillomavirus, Betapapillomavirus, Deltapapillomavirus, and Mupapillomavirus, with only Alphapapillomavirus, Gammapapillomavirus and Betapapillomavirus affecting humans (Harden and Munger, 2017). Of the genera affecting humans, Alphapapillomavirus is the most common genus infecting the genital tract (International Agency for Research on Cancer, 2018).
More than 200 genital HPV genotypes have been molecularly characterized, with some categorized into low-risk genotypes (causing genital warts) while others are categorized as high-risk genotypes (causing CIN and cervical cancer) (Juckett and Hartman-Adams, 2010). High-risk HPV types include HPV 16, 18, 31, 33, 35, 39, 45, 51, 52, 56, 68, and 59, with HPV 16 and 18 being the most prevalent (accounting for 70% of cervical cancer cases) genotypes (Clifford et al., 2017). The most common low-risk HPV include HPV 6 and 11, accounting for ~90% of genital warts and rarely developing into cancer (Ebrahim et al., 2016). Cervical cancer precursor lesion screening uses biopsy, colposcopy and acetic acid test, Pap smear, and nucleic acid-based tests (Agorastos et al., 2010). A Pap smear is the main screening tool to identify precancerous cells in the cervix, and any observed abnormalities are further evaluated with colposcopy, biopsy, and molecular-based tests (Agorastos et al., 2010).
HPV Treatment and Prevention Strategies
Although there is no effective treatment for the virus itself, oncogenic HPV infections and subsequent HPV-associated lesions can be prevented by vaccination (WHO, 2022). There are three commercially available prophylactic vaccines targeting different HPV types, including the bivalent (Cervarix®), quadrivalent (Gardasil®), and nonavalent (Gardasil®9) vaccines (Arbyn and Xu, 2018). The bivalent vaccine protects against the two commonly known high-risk oncogenic types, HPV 16 and HPV 18 (Juckett and Hartman-Adams, 2010). The quadrivalent vaccine acts against these and two genital wart-causing low-risk types (HPV 6 and 11) (Stern et al., 2000; Hancock et al., 2018). The nonavalent vaccine is directed against 9 HPV genotypes, the four collectively targeted by the bivalent and quadrivalent vaccines (HPV 16, 18, 6, and 11) and five additional strains associated with cervical cancer (HPV 31, 33, 45, 52, 58) (Lekoane et al., 2019). The adverse effects of HPV vaccination are normal mild local reactions and their safety has resulted in effective HPV vaccination program in majority of developed countries, but the uptake of these vaccinations by native populations is low (Poirier et al., 2021). HPV vaccination is provided to adolescents from 9 to 12 years (Wang et al., 2020) to prevent HPV infections, abnormal cervical cytology, and cervical cancer (Palefsky, 2020). However, these primary prevention strategies are unable to protect against all types of HPVs or eradicate the existing HPV-infected cells, as capsid proteins are established either before viral entry, or in the terminally differentiated epithelium (Castle and Maza, 2016). Women identified with precancerous lesions can be treated using cryotherapy or thermal ablation, and invasive cervical cancer can be treated by surgery, chemotherapy, and radiotherapy (WHO, 2022). Collectively, the partial protection prophylactic vaccines has against certain HPV types that are associated with cervical cancer justifies the need for continual screening and development of additional therapeutic options to resolve cases post-infection.
HPV Infection in Young Women
Young women in sub-Saharan Africa continue to bear a disproportionate burden of HPV (Pimple and Mishra, 2022), where the HIV epidemic has reached its greatest scale (UNAIDS, 2017). Adolescent girls and young women are more susceptible to HPV infection than their male counterparts (Bruni et al., 2010; Insinga et al., 2010; Edna Omar et al., 2017). Bruni et al. (2010) showed that HPV infection peaks in younger women around the age of sexual debut and declines during later decades of life (Bruni et al., 2010). The unique vulnerability of women to HPV infection comes from several different behavioral and biological factors. While the risk for infection differs from person to person, increased number of sexual partners, early sexual debut, the use of intravaginal insertion products, uncircumcised male partners, and the number of prior pregnancies is some of the documented behavioral risk factors in women (Sasagawa et al., 2012; Dreyer, 2018). Several biological factors, including vaginal surface, the relatively larger area of cervical epithelium undergoing squamous metaplasia, microabrasion in cervical mucosa (Figure 1A), immunosuppression, co-occurrence of STIs, disturbance of vaginal microenvironment and menstruation, defective immune responses associated with genetic variations, and condomless sex, may predispose women to become infected with HPV (Insinga et al., 2010; Dempsey and Mendez, 2010). Despite evidence for an etiological role for HPV, these risk factors remain largely unexplored. Understanding the role of behavioral and biological risk factors for HPV infection in adolescent girls and young women could be crucial to developing effective ways to prevent HPV infection, and prophylactic vaccines.
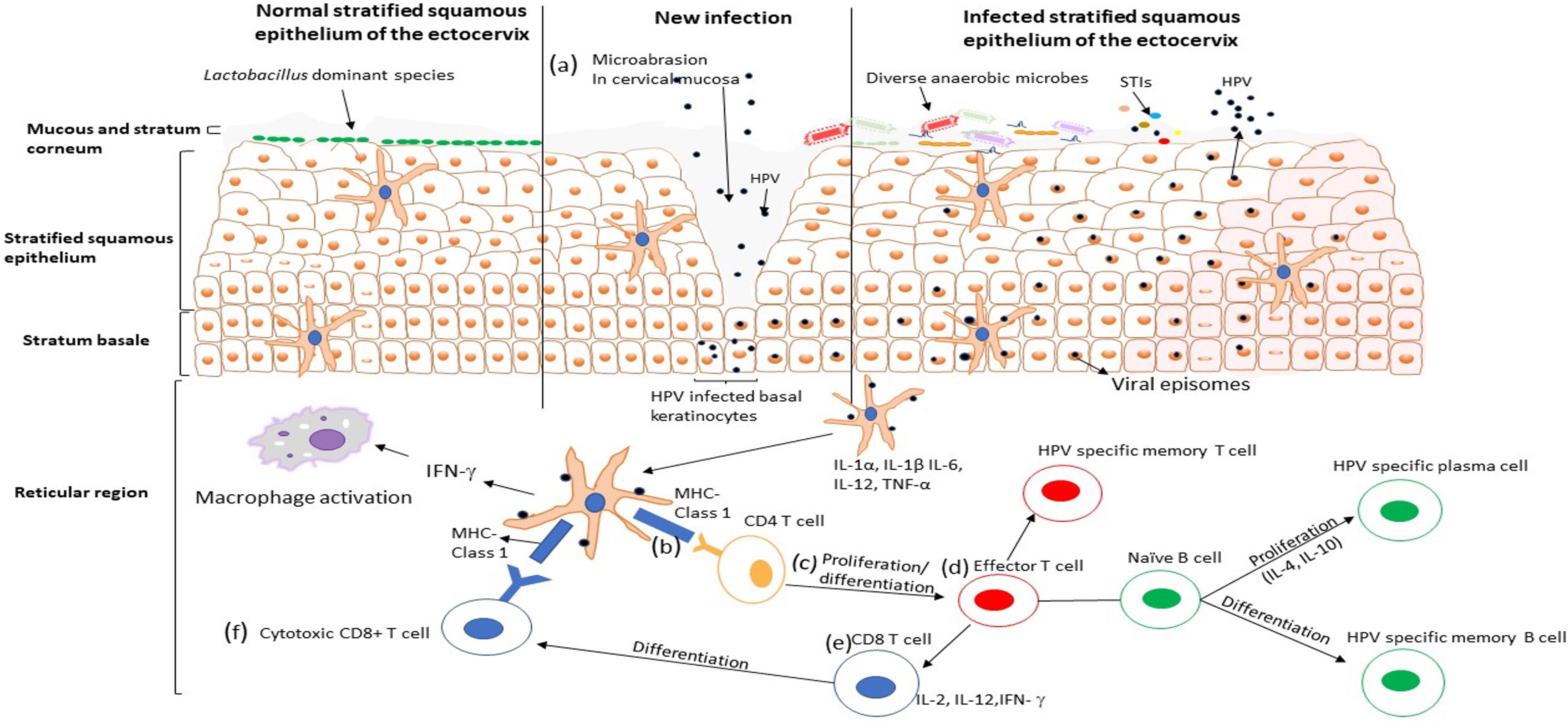
Figure 1 HPV infection cycle in the ectocervix of the female genital tract. (A) The HPV virus particles penetrate the stratified squamous epithelium through microabrasion. The virus particles infect the basal keratinocytes in the basement membrane and establish an infection, viral replication occurs, and the infected cells are transported up the epithelium. (B) Viral particles are taken up by dendritic cells that secrete T cells attracting pro-inflammatory cytokines and chemokines (IL-1 α, IL-1β, IL-6, TNF-α, IL-12), which may facilitate activation and recruitment of CD4+ T cells. CD4+ T cells recognise HPV antigen presented by the dendritic cells and (C) undergoes proliferation and differentiation into the effector T cell. (D) The effort T cells [which proliferate into HPV specific memory T cell and (E, F)cytotoxic CD8+ T cell] binds with the naïve B cells, which differentiate to HPV specific plasma cells and HPV specific memory Bcells. Activation of cellular and humoral immunity is associated with clearance of HPV infection.
Immune Response and Evasion Mechanisms
Women are particularly vulnerable to HPV and are predominantly infected through heterosexual transmission (Bosch et al., 2002; Bruni et al., 2010; Branstetter et al., 2017). HPV infections are localized to keratinocytes. The mucosal epithelium provides the first line of defense against pathogen entry and mediates the initial host immune response against HPV (Stanley, 2006). Pathogens like HPV are detected by an intricate matrix of innate and adaptive immune responses of the lower and upper female genital tract.
Innate Immune Response
Innate immune cells like neutrophils, monocytes, macrophages, eosinophils, mast cells, dendritic cells, and other associated cells identify and elicit protecting responses to invading pathogens through pattern recognition receptors such as toll-like receptors (TLR), nucleotide oligomerization domain (NOD)-like receptors (NLRs), and retinoic acid-inducible gene I (RIG-I)-I-like receptors (RLRs) (Bordignon et al., 2017). Antigen-presenting cells (APCs) such as Langerhans cells (LC), macrophages, and dendritic cells play an essential role in connecting the innate immune response to the adaptive immune system (Woodworth, 2002). LCs are the only APCs that can access the HPV proteins in the epithelial cells of the surface layer (Doorbar et al., 2012). HPV transfection of DCs leads to changes in DC migratory pattern and induces cytokine production, which may suppress immune response to viral antigens (Khaiboullina et al., 2013). Macrophages contribute to the clearance of HPV infection through the production of tumor necrosis factor-alpha and nitric oxide-dependent mechanisms (Routes et al., 2005; Bergot et al., 2011). Natural killer cells have been associated with viral clearance, elimination of HPV infected cells, and cancer prevention in HPV-related carcinogenesis (de Freitas et al., 2017). Although less is known about neutrophils and cervical cancer, recent data demonstrates that high concentrations of neutrophils are strongly associated with poor prognosis and disease progression in women with cervical cancer (Alvarez et al., 2017).
Adaptive Immune Response
The adaptive immune response is another protective line of host defense against pathogens. It is differentiated into two pathways, the T-helper (Th)1 cell responses that promote cell-mediated immunity and Th2 cell responses that promote humoral immunity (Stanley, 2006). During HPV infection, the antigen is taken up by DCs through phagocytosis and migrates to site lymphoid tissues to activate adaptive immunity via the expression of inflammatory cytokines such as IL-1α, IL-1β, IL-6, TNF-α, and IL-12 (Sasagawa et al., 2012). T lymphocytes (CD4+) recognized HPV antigen presented by mature APCs (Figure 1B) and undergo proliferation/differentiation (Figure 1C), and digest the antigen into shorter peptides or differentiate into effector T cells that interact with naive B cells and produce cytokines to assist in the maturation of B cell responses (Figure 1D) (Steele et al., 2002). An anti-viral immune response needs a Th1 cell response which secrete cytokines such as IL-2, IL-12, and IFN-γ to activate immune cells, including the naïve CD8+ T-lymphocytes (Figure 1E) that later differentiate into cytotoxic T lymphocytes (CTL) and become effector T-cells that can kill CIN or HPV infected cells (Figure 1F) (Sasagawa et al., 2012). CTLs, CD4+ T cells and other Th1 responses have been associated with effective clearance of HPV 16 and HPV 18 (Torcia, 2019), while the lack of these T lymphocyte lineages has been associated with persistent HPV infection and the development of high-grade disease (Pao et al., 1995). Furthermore, Th2 cells produce cytokines such as IL-4, IL-6, IL-8 and IL-10 that help B cells differentiate into plasma cells that produce HPV-specific antibodies into circulation (Woodworth, 2002). Some naive B and T cells transform into HPV-specific memory B and T cells that migrate to the bone marrow to survive as long-lived memory cells and differentiate into plasma cells or activated T cells upon reinfection (Stern et al., 2000). In contrast, previous studies demonstrated that women with high grade HPV+ cervical lesions had an increased IL-17-associated (Th17) response known to be pro-tumorigenic in HPV-associated cancers (Walch-Ruckheim et al., 2015). Activation and recruitment of Th17 cells is mediated by stromal tumor-associated fibroblasts and tumor-derived chemokines (CXCR3) (Walch-Ruckheim et al., 2015). Taken together, these findings suggest that not all innate and adaptive immune lineages are capable of migrating to sites of infection to kill HPV-infected cells, some actually promote progression of cervical lesions to invasive cervical cancer.
Evasion of Host Immune Responses by HPV
Innate Immune Evasion by HPV
HPV can deploy various mechanisms to evade host immune responses to establish persistent infections. One of the mechanisms involves altering APC function, including pathogen recognition receptors. High-risk HPV has been suggested to inhibit keratinocyte CCL20 expression, which reduces the ability of LCs to induce a cytotoxic immune response by compromising LCs infiltration (Chandra et al., 2016). In addition, HPV infection has been shown to affect the differentiation of monocytes into mature DCs, leading to altered functionality of DCs (Song et al., 2015). Another evasion mechanism of HPV infection is by inhibiting the recruitment of macrophages and other immune cells into the site of infection by the HPV E6 and E7 proteins. (HPV16 only) (Stern et al., 2000). HPV16 E6 and E7 proteins have been shown to prevent translocation of macrophages via downregulation of cytokines (TNF-α and MIP-3α) responsible for activation of these multifunctional cells (Hacke et al., 2010; Bashaw et al., 2017). Hasan et al. (2007) demonstrated that HPV-16 downregulated the expression and function of TLR-9 in human epithelial cells (Hasan et al., 2007). These findings suggest that overexpression of oncoprotein may inhibit the dendritic cell function in the epithelium.
Natural killer cells are another key component of the innate immune response that HPV evades. E5 protein has been shown to inhibit CD1d on the surfaces of cells infected with HPV6 or 16, resulting in decreased cytotoxic activity of NK cells. Furthermore, high-risk-HPV genotype (HPV-18) has been associated with suppression of the cyclic guanosine monophosphate-adenosine monophosphate synthase, which plays a role in activating type I interferon genes and production of inflammatory cytokine response (Lo Cigno et al., 2020). The interferons play a role in inhibiting viral replication in the host cells and activating immune cells that can eradicate the infected cells (Doorbar et al., 2015). Studies have demonstrated that E6, HPV16 and 18 evade innate immune response by downregulating the transcription of type-1 IFNs and IFN Kappa secretion (Koromilas et al., 2001; Woodby et al., 2018). More studies on the interaction between HPV proteins and type I IFN response remain critical.
Evasion of Adaptive Immune Response by HPV
The virus is also able to escape adaptive immune responses. Although the mechanisms by which HPV can evade the host immune system remain unclear, HPV proteins and regulatory cytokines have been suggested as contributors (Westrich et al., 2017). HPV16 E5 and E7 have been reported to reduce expression of interferons and HLA class-1 molecules, resulting in a lack of CTL response against HPV (Stern et al., 2000). In addition, studies have reported high concentrations of an anti-inflammatory cytokine (IL-10) in women with persistent HPV infection compared to those who cleared the HPV infection. Similarly, increased levels of immunosuppressive cells such as transforming growth factor-beta (TGF-β)-producing Tregs have been observed in cervical tissues and vulval intraepithelial neoplasia (VIN) lesions. The increased concentrations of IL-10 and TGF-β in tissues may indirectly undermine T cell function by limiting the ability of APCs to promote CD4+ T cell differentiation and proliferation, thereby modulating the adaptive immune responses (Sasagawa et al., 2012). Another mechanism by which CD4+ and CD8+ T cell function is inhibited involves downregulation of surface MHC I expression and the impairment of APC trafficking and maturation (Hilders et al., 1995; Keating et al., 1995; Bontkes et al., 1998). Furthermore, dysregulated expression of IL-8 receptor by HPV16 E6 protein affected the CD8+ mediated response (Cho et al., 2001). Collectively, these finding suggest that APC and T cell response downregulated by immunosuppressive immune response may result in HPV-immune evasion, and consequently lead to persistent HPV infection and the development of high-grade disease
Association Between Genital Inflammation and HPV
Inflammation is defined as the natural immune response following injury or infection (Ferrero-Miliani et al., 2007; Medzhitov, 2010). It is generally characterised by elevated levels of cellular markers and pro-inflammatory cytokines in the genital tract (Passmore et al., 2016). Host defense mechanisms, including immune mediators in the female genital tract microenvironment, play a role in the clearance and persistence of HPV and the risk of developing cervical cancer (Stanley, 2010; Daud et al., 2011; Łaniewski et al., 2018; Liebenberg et al., 2019; Onywera et al., 2019; Moscicki A-B et al., 2020).
Contradicting data have been reported between HPV and altered cytokine milieu profiles (Łaniewski et al., 2018; Liebenberg et al., 2019, 12). Łaniewski et al. (2018) showed a strong association between invasive cervical carcinoma and elevated concentrations of TNF-α, TNF-β, MIP-1α, GMCSF, and IL-10 (Łaniewski et al., 2018). In addition, HPV persistence was associated with chemokine MIP-1α and growth factor GM-CSF that play a significant role in activation and recruitment granulocytes (Marks et al., 2011). Another study showed that women with elevated concentrations of mucosal cytokine interleukin (IL)−10, IL−12, macrophage inflammatory protein (MIP)−1α and TNF−α were less likely to clear any HPV type while low levels of these cytokines (including IL-8) correlated with HPV clearance (12). A positive correlation between elevated concentrations of several cytokines (IL-36γ, MIP-1β, RANTES, IP-10, IL-2, IL-4, Flt-3L, sCD40L) and invasive cervical cancer carcinoma in women with BV were reported (Łaniewski et al., 2018). The β-chemokines (RANTES and MIP-1β), IL-8, IP-10, known to promote immune cell activation and recruitment and have shown to limit cellular entry of pathogens (Mhatre et al., 2012). Increased mucosal cytokine profiles in the reproductive tract of women infected with HPV was also associated with HPV prevalence, clearance, acquisition, persistence and increased HIV acquisition risk (Liebenberg et al., 2019). The apparent association between HPV infection and genital cytokine responses may likely indicate the role of cellular immunity to control HPV infection. Moscicki et al. (2020) found that 9 of the 13 cytokines (IL-4, IL-5, IL-10, IL-12, IL-13, IFN-γ, IFN-2α, MIP-1α, and TNF-1α) tested were elevated after the clearance of HPV infection compared to prior visits (Moscicki AB et al., 2020). Production of anti-inflammatory cytokine IL-4, IL-5, IL-10, and IL-13 are known to promote macrophage activation and promote the differentiation of B lymphocytes that are crucial for the production and secretion of antibodies (Fernandes et al., 2015). In contrast, two studies did not show the relation between increased concentrations of pro inflammatory cytokines and HPV acquisition or clearance (Kriek et al., 2016; Shannon et al., 2017). Understandably, while there are no structural conformations on host cells during HPV invasion, HPV also maintains the anti-inflammatory state likely by avoiding the host immunity through disruption of the interplay between infected cells and effector cells (98). There is a need to better understand the cellular or other factors associated with the cytokines in the different HPV status categories.
Association Between the Vaginal Microbiome and HPV Infection
The vagina and ectocervix are dominated by lactic-acid producing bacteria and cervicovaginal fluids that act as a lubricant that traps invading pathogens (Lee et al., 2013; Aldunate et al., 2015). A genital environment dominated by Lactobacillus spp. has been associated with optimal pregnancy outcomes, lack of abnormal vaginal symptoms and urogenital disease, and reduced risk for several STIs, including HPV and HIV (Petrova et al., 2013). In contrast, the opposite is observed in the genital tract dominated by non-optimal vaginal microbiota. Several Lactobacillus spp. have been described in the non-BV state, although the most frequent and abundant are L. crispatus, L. gasseri,and L. jensenii (Ravel et al., 2011; Bik et al., 2019),. L. gasseri enriched microbiome was also associated with rapid rates of HPV clearance (Brotman, 2011). A recent study showed that women with L.crispatus enriched microbiome were less likely to have prevalent high-risk HPV infection than women with overgrowth of pathogenic microorganisms such as those linked with BV (Gardnerella, Atopobium, and Prevotella) (105). Furthermore, L.crispatus abundance in the genital tract was also associated with HPV clearance, suggesting the relative association between clearance and L. crispatus (105).
BV is a condition characterized by a shift in vaginal microbiota from Lactobacillus dominant towards more diverse bacteria, including strict and facultative anaerobes such as Gardnerella, Prevotella, and Sneathia, often resulting in vaginitis and discharge (105, Cohen et al., 2012). BV has been associated with elevated levels of cytokines (IL-1α, IL-1β, IL-18, IL-7, MIP-1α, MIP-1β, IL-8, MIF, TNF-α, and TNF-β) and cellular (CCR5+CD4+ T cells) biomarkers of inflammation associated with increased HIV acquisition risk (Cohen et al., 2012; Eade et al., 2012). Furthermore, numerous studies have suggested a link between BV and other STIs such as Chlamydia trachomatis, Neisseria gonorrhea, and cervical HPV (Cherpes et al., 2003; Brotman, 2011). The ulcerative and highly inflammatory sequelae caused by STIs and/or BV (inflammation) provide biologically plausible mechanisms supporting a possible increased susceptibility to HPV among co-infected individuals. A high relative abundance of Gardnerella and Atopobium vaginae were associated with CIN (Godoy-Vitorino et al., 2018; Berggrund et al., 2020). Increased abundance of Sneathia, Atopobium, and Gardnerella is associated with incident high-risk HPV infection (Onywera et al., 2021). A study by Lee et al. (2013) showed that HPV-infected women had lower Lactobacillus species and increased Fusobacteria and Sneathia compared to HPV-uninfected women (Lee et al., 2013). In agreement with these findings, longitudinal analysis from 32 sexually active women showed that a low Lactobacillus community with high proportions of the genera Atopobium species were associated with the low rate of HPV clearance (Kyrgiou et al., 2017). While existing evidence suggests the association between HPV infection and genital dysbiosis, justifiable concerns that positive associations merely reflect residual confounding by unmeasured sexual risk behaviors (such as engaging in condomless sex and having sex with uncircumcised partner) still exists (Averbach et al., 2010). Thus, further research investigating this interplay is warranted. Detailed understanding of the genital microbial composition and structure in women with HPV infection may help identify the causal connections between microbiota, HPV infection, and cervical cancer.
Association Between HPV and HIV
HPV Infection and HIV Risk
Epidemiological and meta-analyses data suggest that sexually transmitted infections like herpes, gonorrhoea, chlamydia, and syphilis can increase the risk of HIV acquisition (Fleming and Wasserheit, 1999; Ward and Rönn, 2010). Other biological correlates of HIV risk that have been described to date is mucosal pro-inflammatory cytokines and BV-associated bacteria that, if altered prior to infection, were associated with higher risk of acquiring HIV (Anahtar et al., 2015; Masson et al., 2015; Yegorov et al., 2019). However, less is known about the mechanism by which HPV infection might increase HIV acquisition risk.
In addition to its role as a biological factor in the development of anogenital cancers, HPV may also be an important co-factor in the increased risk of HIV acquisition in women. Overall, HIV infection risk doubled in women with prevalent HPV infection, with either oncogenic or non-oncogenic HPV genotypes (Liu et al., 2018). Another study showed a significant association between high-risk HPV genotypes and HIV acquisition (Looker et al., 2018). Persistent HPV infection has been associated with an increased biomarkers of HIV acquisition, and the causal link is still not well-understood (Chikandiwa et al., 2017). Higher frequency of the CD4+ T cells in the stroma and epithelium are closely associated with HPV lesion regression (Monnier-Benoit et al., 2006) and consequently increasing mucosal HIV target cell frequency and activation (McKinnon et al., 2011). In addition, pro-inflammatory cytokines essential for HPV clearance are also known to increased risk of HIV acquisition (Masson et al., 2015; Liebenberg et al., 2019). Further research is still needed to explore the association between HPV and HIV and validate HPV as a potential risk factor for HIV acquisition, and if found to be true, these may highlight the importance of decreasing HPV burden in settings with high prevalence to curb HIV infections.
HPV Infection in Women Living With HIV
HIV infection has also been associated with the development of CIN2, CIN3, and invasive cervical carcinoma in HPV infected population (Clifford et al., 2017). Although multiple types of HPV have been associated with HIV infection, HPV16 is the commonest cause of cervical carcinoma in HIV infected population (Dreyer, 2018). Studies have reported that women living with HIV (WLWH) are more likely to be infected with high-risk HPV and multiple HPV genotypes, resulting in the development of pre-invasive lesions that, if left untreated, can develop into invasive cervical cancer (98, Liu et al., 2018). WLWH have up to five times more cervical cancer than HIV-uninfected (Liu et al., 2018). In two large prospective studies that assessed the prevalence of HPV genotypes in women living in the United States, HPV 6 or 11 was 3.6 and 5.6 times high in WLWH compared to their HIV-uninfected counterparts, respectively (Bruni et al., 2010; Bogale et al., 2020). Furthermore, patients living with HIV were at increased risk of cervical abnormalities and onward HPV transmission due to high prevalence of high HPV viral load (Looker et al., 2018). Given the growing evidence of an increased risk of cervical cancer in WLWH, regular HPV screening and possibly treatment for cervical cancer is needed to effectively control HPV and its adverse sequelae.
Conclusion
While there is substantial progress in increasing vaccine access and immunization coverage, young women, particularly in sub-Saharan African remain disproportionately infected by HPV. Factors that may render women more vulnerable to HPV infection have not been fully characterized. This review showed that the vaginal microbiome, cellular, and cytokine markers of inflammation are some of the biological markers that are associated with neoplastic disease in cervical carcinogenesis. While significant progress has been made in understanding how HPV evades immunity, mechanistic studies on how risk factors influence host-mucosal microenvironment and viral persistence are warranted. The role of other biological risk factors such as intravaginal insertion practices (for hygenic and sexual enhancement purposes) are less studied and could be important drivers of HPV risk in young women. There remains a need to conduct more preclinical models to understand how biological risk factors might block efficient HPV clearance from the mucosa and pave the way for cervical cancer.
Author Contributions
All authors contributed to the article and approved the submitted version.
Funding
This review was conducted as part of the DST-NRF Centre of Excellence (CoE) in HIV Prevention, supported by the Department of Science and Innovation and the National Research Foundation (grant 96354). LN was funded by DST-NRF CoE in HIV Prevention (grant 96354) and Poliomyelitis Research Foundation Research Bursary (bursary# 21/73). SN was supported by Columbia University-Southern African Fogarty AITRP Programme (grant# D43TW00231), National Research Fund Thuthuka Research Grant (grant# TTK160510164586), and Poliomyelitis Research Foundation Research Grant (grant# 16/17). GM was funded by Poliomyelitis Research Foundation Research Grant (grant# 18/16) and National Research Foundation Grant (grant# SFP180507326699). AM was supported by National Research Foundation Grant (grant# PDG210309589501)
Conflict of Interest
The authors declare that the research was conducted in the absence of any commercial or financial relationships that could be construed as a potential conflict of interest.
Publisher’s Note
All claims expressed in this article are solely those of the authors and do not necessarily represent those of their affiliated organizations, or those of the publisher, the editors and the reviewers. Any product that may be evaluated in this article, or claim that may be made by its manufacturer, is not guaranteed or endorsed by the publisher.
References
Agorastos, T., Sotiriadis, A., Chatzigeorgiou, K. (2010). Can HPV Testing Replace the Pap Smear? Ann. N Y Acad. Sci. 1205, 51–56. doi: 10.1111/j.1749-6632.2010.05661.x
Aldunate, M., Srbinovski, D., Hearps, A. C., Latham, C. F., Ramsland, P. A., Gugasyan, R., et al. (2015). Antimicrobial and Immune Modulatory Effects of Lactic Acid and Short Chain Fatty Acids Produced by Vaginal Microbiota Associated With Eubiosis and Bacterial Vaginosis. Front. Physiol. 6, 164. doi: 10.3389/fphys.2015.00164
Alvarez, K. L. F., Beldi, M., Sarmanho, F., Rossetti, R. A. M., Silveira, C. R. F., Mota, G. R., et al. (2017). Local and Systemic Immunomodulatory Mechanisms Triggered by Human Papillomavirus Transformed Cells: A Potential Role for G-CSF and Neutrophils. Sci. Rep. 7 (1), 9002. doi: 10.1038/s41598-017-09079-3
Amaral, E., Perdigao, A., Souza, M. H., Mauck, C., Waller, D., Zaneveld, L., et al. (2006). Vaginal Safety After Use of a Bioadhesive, Acid-Buffering, Microbicidal Contraceptive Gel (ACIDFORM) and a 2% Nonoxynol-9 Product. Contraception. 73 (5), 542–547. doi: 10.1016/j.contraception.2005.12.006
Anahtar, M. N., Byrne, E. H., Doherty, K. E., Bowman, B. A., Yamamoto, H. S., Soumillon, M., et al. (2015). Cervicovaginal Bacteria are a Major Modulator of Host Inflammatory Responses in the Female Genital Tract. Immunity. 42 (5), 965–976. doi: 10.1016/j.immuni.2015.04.019
Arbyn, M., Xu, L. (2018). Efficacy and Safety of Prophylactic HPV Vaccines. A Cochrane Review of Randomized Trials. Expert Rev. Vaccines 17 (12), 1085–1091. doi: 10.1080/14760584.2018.1548282
Audirac-Chalifour, A., Torres-Poveda, K., Bahena-Román, M., Téllez-Sosa, J., Martínez-Barnetche, J., Cortina-Ceballos, B., et al. (2016). Cervical Microbiome and Cytokine Profile at Various Stages of Cervical Cancer: A Pilot Study. PloS One 11 (4), e0153274. doi: 10.1371/journal.pone.0153274
Averbach, S. H., Gravitt, P. E., Nowak, R. G., Celentano, D. D., Dunbar, M. S., Morrison, C. S., et al. (2010). The Association Between Cervical Human Papillomavirus Infection and HIV Acquisition Among Women in Zimbabwe. AIDS. 24 (7), 1035–1042. doi: 10.1097/QAD.0b013e3283377973
Balkwill, F., Mantovani, A. (2001). Inflammation and Cancer: Back to Virchow? Lancet 357 (9255), 539–545. doi: 10.1016/S0140-6736(00)04046-0
Barros, M. R., de Melo, C. M. L., Barros, MLCMGR, de Cássia Pereira de Lima, R., de Freitas, A. C., Venuti, A. (2018). Activities of Stromal and Immune Cells in HPV-Related Cancers. J. Exp. Clin. Cancer Res. 37 (1), 137. doi: 10.1186/s13046-018-0802-7
Bashaw, A. A., Leggatt, G. R., Chandra, J., Tuong, Z. K., Frazer, I. H. (2017). Modulation of Antigen Presenting Cell Functions During Chronic HPV Infection. Papillomavirus Res. 4, 58–65. doi: 10.1016/j.pvr.2017.08.002
Berggrund, M., Gustavsson, I., Aarnio, R., Lindberg, J. H., Sanner, K., Wikstrom, I., et al. (2020). Temporal Changes in the Vaginal Microbiota in Self-Samples and its Association With Persistent HPV16 Infection and CIN2. Virol. J. 17 (1), 147. doi: 10.1186/s12985-020-01420-z
Bergot, A. S., Kassianos, A., Frazer, I. H., Mittal, D. (2011). New Approaches to Immunotherapy for HPV Associated Cancers. Cancers (Basel). 3 (3), 3461–3495. doi: 10.3390/cancers3033461
Bik, E. M., Bird, S. W., Bustamante, J. P., Leon, L. E., Nieto, P. A., Addae, K., et al. (2019). A Novel Sequencing-Based Vaginal Health Assay Combining Self-Sampling, HPV Detection and Genotyping, STI Detection, and Vaginal Microbiome Analysis. PloS One 14 (5), e0215945. doi: 10.1371/journal.pone.0215945
Bogale, A. L., Belay, N. B., Medhin, G., Ali, J. H. (2020). Molecular Epidemiology of Human Papillomavirus Among HIV Infected Women in Developing Countries: Systematic Review and Meta-Analysis. Virol. J. 17 (1), 179. doi: 10.1186/s12985-020-01448-1
Bontkes, H. J., Walboomers, J., Meijer, C., Helmerhorst, T., Stern, P. L. (1998). Specific HLA Class I Down-Regulation is an Early Event in Cervical Dysplasia Associated With Clinical Progression. Lancet 351 (9097), 187–188. doi: 10.1002/(sici)1097-0215(19981005)78:2<166::aid-ijc8>3.0.co;2-x
Bordignon, V., Di Domenico, E. G., Trento, E., D'Agosto, G., Cavallo, I., Pontone, M., et al. (2017). How Human Papillomavirus Replication and Immune Evasion Strategies Take Advantage of the Host DNA Damage Repair Machinery. Viruses. 9 (12), 1–14. doi: 10.3390/v9120390
Bosch, F. X., Lorincz, A., Muñoz, N., Meijer, C. J., Shah, K. V. (2002). The Causal Relation Between Human Papillomavirus and Cervical Cancer. J. Clin. Pathol. 55 (4), 244–265. doi: 10.1136/jcp.55.4.244
Branstetter, A. J., McRee, A. L., Reiter, P. L. (2017). Correlates of Human Papillomavirus Infection Among a National Sample of Sexual Minority Women. J. Womens Health (Larchmt). 26 (9), 1004–1011. doi: 10.1089/jwh.2016.6177
Brotman, R. M. (2011). Vaginal Microbiome and Sexually Transmitted Infections: An Epidemiologic Perspective. J. Clin. Invest. 121 (12), 4610–4617. doi: 10.1172/JCI57172
Brotman, R. M., Shardell, M. D., Gajer, P., Tracy, J. K., Zenilman, J. M., Ravel, J., et al. (2014). Interplay Between the Temporal Dynamics of the Vaginal Microbiota and Human Papillomavirus Detection. J. Infect. Dis. 210 (11), 1723–1733. doi: 10.1093/infdis/jiu330
Bruni, L., Diaz, M., Castellsague, X., Ferrer, E., Bosch, F. X., de Sanjose, S. (2010). Cervical Human Papillomavirus Prevalence in 5 Continents: Meta-Analysis of 1 Million Women With Normal Cytological Findings. J. Infect. Dis. 202 (12), 1789–1799. doi: 10.1086/657321
Brusselaers, N., Shrestha, S., van de Wijgert, J., Verstraelen, H. (2019). Vaginal Dysbiosis and the Risk of Human Papillomavirus and Cervical Cancer: Systematic Review and Meta-Analysis. Am. J. Obstet Gynecol. 221 (1), 9–18.e8. doi: 10.1016/j.ajog.2018.12.011
Burchell, A. N., Winer, R. L., de Sanjosé, S., Franco, E. L. (2006). Chapter 6: Epidemiology and Transmission Dynamics of Genital HPV Infection. Vaccine. 24Suppl 3, S3/52–S3/61. doi: 10.1016/j.vaccine.2006.05.031
Castle, P. E., Maza, M. (2016). Prophylactic HPV Vaccination: Past, Present, and Future. Epidemiol. Infect. 144 (3), 449–468. doi: 10.1017/S0950268815002198
Chandra, J., Miao, Y., Romoff, N., Frazer, I. H. (2016). Epithelium Expressing the E7 Oncoprotein of HPV16 Attracts Immune-Modulatory Dendritic Cells to the Skin and Suppresses Their Antigen-Processing Capacity. PloS One 11 (3), e0152886. doi: 10.1371/journal.pone.0152886
Cherpes, T. L., Meyn, L. A., Krohn, M. A., Hillier, S. L. (2003). Risk Factors for Infection With Herpes Simplex Virus Type 2: Role of Smoking, Douching, Uncircumcised Males, and Vaginal Flora. Sex Transm Dis. 30 (5), 405–410. doi: 10.1097/00007435-200305000-00006
Chikandiwa, A., Chimoyi, L., Pisa, P. T., Chersich, M. F., Muller, E. E., Michelow, P., et al. (2017). Prevalence of Anogenital HPV Infection, Related Disease and Risk Factors Among HIV-Infected Men in Inner-City Johannesburg, South Africa: Baseline Findings From a Cohort Study. BMC Public Health 17 (Suppl 3), 425. doi: 10.1186/s12889-017-4354-0
Cho, Y. S., Kang, J. W., Cho, M., Cho, C. W., Lee, S., Choe, Y. K., et al. (2001). Down Modulation of IL-18 Expression by Human Papillomavirus Type 16 E6 Oncogene via Binding to IL-18. FEBS Lett. 501 (2-3), 139–145. doi: 10.1016/S0014-5793(01)02652-7
Clifford, G. M., Tully, S., Franceschi, S. (2017). Carcinogenicity of Human Papillomavirus (HPV) Types in HIV-Positive Women: A Meta-Analysis From HPV Infection to Cervical Cancer. Clin. Infect. Dis. 64 (9), 1228–1235. doi: 10.1093/cid/cix135
Cohen, C. R., Lingappa, J. R., Baeten, J. M., Ngayo, M. O., Spiegel, C. A., Hong, T., et al. (2012). Bacterial Vaginosis Associated With Increased Risk of Female-to-Male HIV-1 Transmission: A Prospective Cohort Analysis Among African Couples. PloS Med. 9 (6), e1001251. doi: 10.1371/journal.pmed.1001251
Daud, I. I., Scott, M. E., Ma, Y., Shiboski, S., Farhat, S., Moscicki, A. B. (2011). Association Between Toll-Like Receptor Expression and Human Papillomavirus Type 16 Persistence. Int. J. Cancer J. Int. du cancer. 128 (4), 879–886. doi: 10.1002/ijc.25400
de Freitas, A. C., de Oliveira, T. H. A., Barros, M. R., Jr., Venuti, A. (2017). hrHPV E5 Oncoprotein: Immune Evasion and Related Immunotherapies. J. Exp. Clin. Cancer Res. 36 (1), 71. doi: 10.1186/s13046-017-0541-1
Dempsey, A. F., Mendez, D. (2010). Examining Future Adolescent Human Papillomavirus Vaccine Uptake, With and Without a School Mandate. J. Adolesc. Health 47 (3), 242–2488.e1-8.e6. doi: 10.1016/j.jadohealth.2009.12.009
Di Paolo, M., Sani, C., Clemente, A. M., Iossa, A., Perissi, E., Castronovo, G., et al. (2017). Characterization of Cervico-Vaginal Microbiota in Women Developing Persistent High-Risk Human Papillomavirus Infection. Sci. Rep. 7 (1), 10200. doi: 10.1038/s41598-017-09842-6
Doerflinger, S. Y., Throop, A. L., Herbst-Kralovetz, M. M. (2014). Bacteria in the Vaginal Microbiome Alter the Innate Immune Response and Barrier Properties of the Human Vaginal Epithelia in a Species-Specific Manner. J. Infect. Dis. 209 (12), 1989–1999. doi: 10.1093/infdis/jiu004
Doorbar, J., Egawa, N., Griffin, H., Kranjec, C., Murakami, I. (2015). Human Papillomavirus Molecular Biology and Disease Association. Rev. Med. Virol. 25 Suppl 1, 2–23. doi: 10.1002/rmv.1822
Doorbar, J., Quint, W., Banks, L., Bravo, I. G., Stoler, M., Broker, T. R., et al. (2012). The Biology and Life-Cycle of Human Papillomaviruses. Vaccine. 30Suppl 5, F55–F70. doi: 10.1016/j.vaccine.2012.06.083
Dreyer, G. (2018). Clinical Implications of the Interaction Between HPV and HIV Infections. Best Pract. Res. Clin. Obstet Gynaecol. 47, 95–106. doi: 10.1016/j.bpobgyn.2017.08.011
Eade, C. R., Diaz, C., Wood, M. P., Anastos, K., Patterson, B. K., Gupta, P., et al. (2012). Identification and Characterization of Bacterial Vaginosis-Associated Pathogens Using a Comprehensive Cervical-Vaginal Epithelial Coculture Assay. PloS One 7 (11), e50106. doi: 10.1371/journal.pone.0050106
Ebrahim, S., Mndende, X. K., Kharsany, A. B., Mbulawa, Z. Z., Naranbhai, V., Frohlich, J., et al. (2016). High Burden of Human Papillomavirus (HPV) Infection Among Young Women in KwaZulu-Natal, South Africa. PloS One 11 (1), e0146603. doi: 10.1371/journal.pone.0146603
Edna Omar, V., Orvalho, A., Nália, I., Kaliff, M., Lillsunde-Larsson, G., Ramqvist, T., et al. (2017). Human Papillomavirus Prevalence and Genotype Distribution Among Young Women and Men in Maputo City, Mozambique. BMJ Open 7 (7), e015653. doi: 10.1136/bmjopen-2016-015653
Fernandes, J. V., DE Medeiros Fernandes, T. A., DE Azevedo, J. C., Cobucci, R. N., DE Carvalho, M. G., Andrade, V. S., et al. (2015). Link Between Chronic Inflammation and Human Papillomavirus-Induced Carcinogenesis (Review). Oncol. Lett. 9 (3), 1015–1026. doi: 10.3892/ol.2015.2884
Ferrero-Miliani, L., Nielsen, O. H., Andersen, P. S., Girardin, S. E. (2007). Chronic Inflammation: Importance of NOD2 and NALP3 in Interleukin-1beta Generation. Clin. Exp. Immunol. 147 (2), 227–235. doi: 10.1111/j.1365-2249.2006.03261.x
Fleming, D. T., Wasserheit, J. N. (1999). From Epidemiological Synergy to Public Health Policy and Practice: The Contribution of Other Sexually Transmitted Diseases to Sexual Transmission of HIV Infection. Sex Transm Infect. 75 (1), 3–17. doi: 10.1136/sti.75.1.3
Gao, W., Weng, J., Gao, Y., Chen, X. (2013). Comparison of the Vaginal Microbiota Diversity of Women With and Without Human Papillomavirus Infection: A Cross-Sectional Study. BMC Infect. diseases. 13 (1), 271. doi: 10.1186/1471-2334-13-271
Gillet, E., Meys, J. F. A., Verstraelen, H., Bosire, C., De Sutter, P., Temmerman, M., et al. (2011). Bacterial Vaginosis is Associated With Uterine Cervical Human Papillomavirus Infection: A Meta-Analysis. BMC Infect. diseases. 11 (1), 10. doi: 10.1186/1471-2334-11-10
Godoy-Vitorino, F., Romaguera, J., Zhao, C., Vargas-Robles, D., Ortiz-Morales, G., Vazquez-Sanchez, F., et al. (2018). Cervicovaginal Fungi and Bacteria Associated With Cervical Intraepithelial Neoplasia and High-Risk Human Papillomavirus Infections in a Hispanic Population. Front. Microbiol. 9, 2533. doi: 10.3389/fmicb.2018.02533
Gosmann, C., Anahtar, M. N., Handley, S. A., Farcasanu, M., Abu-Ali, G., Bowman, B. A., et al. (2017). Lactobacillus-Deficient Cervicovaginal Bacterial Communities Are Associated With Increased HIV Acquisition in Young South African Women. Immunity. 46 (1), 29–37. doi: 10.1016/j.immuni.2016.12.013
Gupta, S. M., Mania-Pramanik, J. (2019). Molecular Mechanisms in Progression of HPV-Associated Cervical Carcinogenesis. J. BioMed. Sci. 26 (1), 28. doi: 10.1186/s12929-019-0520-2
Hacke, K., Rincon-Orozco, B., Buchwalter, G., Siehler, S. Y., Wasylyk, B., Wiesmüller, L., et al. (2010). Regulation of MCP-1 Chemokine Transcription by P53. Mol. Cancer. 9 (1), 82. doi: 10.1186/1476-4598-9-82
Hancock, G., Hellner, K., Dorrell, L. (2018). Therapeutic HPV Vaccines. Best Pract. Res. Clin. Obstet Gynaecol. 47, 59–72. doi: 10.1016/j.bpobgyn.2017.09.008
Harden, M. E., Munger, K. (2017). Human Papillomavirus Molecular Biology. Mutat. Res. Rev. Mutat. Res. 772, 3–12. doi: 10.1016/j.mrrev.2016.07.002
Hasan, U. A., Bates, E., Takeshita, F., Biliato, A., Accardi, R., Bouvard, V., et al. (2007). TLR9 Expression and Function Is Abolished by the Cervical Cancer-Associated Human Papillomavirus Type 16. J. Immunol. 178 (5), 3186–3197. doi: 10.4049/jimmunol.178.5.3186
Hawes, S. E., Kiviat, N. B. (2002). Are Genital Infections and Inflammation Cofactors in the Pathogenesis of Invasive Cervical Cancer? JNCI: J. Natl. Cancer Institute 94 (21), 1592–1593. doi: 10.1093/jnci/94.21.1592
Hilders, C. G., Munoz, I. M., Nooyen, Y., Fleuren, G. J. (1995). Altered HLA Expression by Metastatic Cervical Carcinoma Cells as a Factor in Impaired Immune Surveillance. Gynecol Oncol. 57 (3), 366–375. doi: 10.1006/gyno.1995.1156
Insinga, R. P., Perez, G., Wheeler, C. M., Koutsky, L. A., Garland, S. M., Leodolter, S., et al. (2010). Incidence, Duration, and Reappearance of Type-Specific Cervical Human Papillomavirus Infections in Young Women. Cancer Epidemiol. Biomarkers Prev. 19 (6), 1585–1594. doi: 10.1158/1055-9965.EPI-09-1235
Juckett, G., Hartman-Adams, H. (2010). Human Papillomavirus: Clinical Manifestations and Prevention. Am. Fam Physician. 82 (10), 1209–1213.
Keating, P. J., Cromme, F. V., Duggan-Keen, M., Snijders, P. J., Walboomers, J. M., Hunter, R. D., et al. (1995). Frequency of Down-Regulation of Individual HLA-A and -B Alleles in Cervical Carcinomas in Relation to TAP-1 Expression. Br. J. Cancer. 72 (2), 405–411. doi: 10.1038/bjc.1995.346
Khaiboullina, S. F., Morzunov, S. P., Hall, M. R., De Meirleir, K. L., Rizvanov, A. A., Lombardi, V. C. (2013). Human Dendritic Cells Transfected With a Human Papilloma Virus-18 Construct Display Decreased Mobility and Upregulated Cytokine Production. Int. J. Oncol. 43 (5), 1701–1709. doi: 10.3892/ijo.2013.2074
Koromilas, A. E., Li, S., Matlashewski, G. (2001). Control of Interferon Signaling in Human Papillomavirus Infection. Cytokine Growth Factor Rev. 12 (2-3), 157–170. doi: 10.1016/S1359-6101(00)00023-X
Kriek, J.-M., Jaumdally, S. Z., Masson, L., Little, F., Mbulawa, Z., Gumbi, P. P., et al. (2016). Female Genital Tract Inflammation, HIV Co-Infection and Persistent Mucosal Human Papillomavirus (HPV) Infections. Virology. 493, 247–254. doi: 10.1016/j.virol.2016.03.022
Kyrgiou, M., Mitra, A., Moscicki, A. B. (2017). Does the Vaginal Microbiota Play a Role in the Development of Cervical Cancer? Transl. Res. 179, 168–182. doi: 10.1016/j.trsl.2016.07.004
Łaniewski, P., Barnes, D., Goulder, A., Cui, H., Roe, D. J., Chase, D. M., et al. (2018). Linking Cervicovaginal Immune Signatures, HPV and Microbiota Composition in Cervical Carcinogenesis in non-Hispanic and Hispanic Women. Sci. Rep. 8 (1), 7593. doi: 10.1038/s41598-018-25879-7
Lee, J. E., Lee, S., Lee, H., Song, Y. M., Lee, K., Han, M. J., et al. (2013). Association of the Vaginal Microbiota With Human Papillomavirus Infection in a Korean Twin Cohort. PloS One 8 (5), e63514. doi: 10.1371/journal.pone.0063514
Lekoane, K. M. B., Kuupiel, D., Mashamba-Thompson, T. P., Ginindza, T. G. (2019). Evidence on the Prevalence, Incidence, Mortality and Trends of Human Papilloma Virus-Associated Cancers in Sub-Saharan Africa: Systematic Scoping Review. BMC Cancer. 19 (1), 563. doi: 10.1186/s12885-019-5781-3
Liebenberg, L. J. P., McKinnon, L. R., Yende-Zuma, N., Garrett, N., Baxter, C., Kharsany, A. B. M., et al. (2019). HPV Infection and the Genital Cytokine Milieu in Women at High Risk of HIV Acquisition. Nat. Commun. 10 (1), 5227. doi: 10.1038/s41467-019-13089-2
Lieberman, J. A., Moscicki, A. B., Sumerel, J. L., Ma, Y., Scott, M. E. (2008). Determination of Cytokine Protein Levels in Cervical Mucus Samples From Young Women by a Multiplex Immunoassay Method and Assessment of Correlates. Clin. Vaccine Immunol. CVI. 15 (1), 49–54. doi: 10.1128/CVI.00216-07
Lin, W., Niu, Z., Zhang, H., Kong, Y., Wang, Z., Yang, X., et al. (2019). Imbalance of Th1/Th2 and Th17/Treg During the Development of Uterine Cervical Cancer. Int. J. Clin. Exp. Pathol. 12 (9), 3604–3612.
Liu, G., Sharma, M., Tan, N., Barnabas, R. V. (2018). HIV-Positive Women Have Higher Risk of Human Papilloma Virus Infection, Precancerous Lesions, and Cervical Cancer. AIDS. 32 (6), 795–808. doi: 10.1097/QAD.0000000000001765
Li, B., Zhang, L., Zhao, J., Tan, G., Zhang, W., Zhang, N., et al. (2019). The Value of Cytokine Levels in Triage and Risk Prediction for Women With Persistent High-Risk Human Papilloma Virus Infection of the Cervix. Infect. Agent Cancer. 14, 16. doi: 10.1186/s13027-019-0231-z
Lo Cigno, I., Calati, F., Albertini, S., Gariglio, M. (2020). Subversion of Host Innate Immunity by Human Papillomavirus Oncoproteins. Pathogens. 9 (4), 1–20. doi: 10.3390/pathogens9040292
Looker, K. J., Ronn, M. M., Brock, P. M., Brisson, M., Drolet, M., Mayaud, P., et al. (2018). Evidence of Synergistic Relationships Between HIV and Human Papillomavirus (HPV): Systematic Reviews and Meta-Analyses of Longitudinal Studies of HPV Acquisition and Clearance by HIV Status, and of HIV Acquisition by HPV Status. J. Int. AIDS Society. 21 (6), e25110. doi: 10.1002/jia2.25110
Marks, M. A., Viscidi, R. P., Chang, K., Silver, M., Burke, A., Howard, R., et al. (2011). Differences in the Concentration and Correlation of Cervical Immune Markers Among HPV Positive and Negative Perimenopausal Women. Cytokine. 56 (3), 798–803. doi: 10.1016/j.cyto.2011.09.012
Masson, L., Passmore, J.-A. S., Liebenberg, L. J., Werner, L., Baxter, C., Arnold, K. B., et al. (2015). Genital Inflammation and the Risk of HIV Acquisition in Women. Clin. Infect. Dis. 61(2), civ298. doi: 10.1093/cid/civ298
McKinnon, L. R., Nyanga, B., Chege, D., Izulla, P., Kimani, M., Huibner, S., et al. (2011). Characterization of a Human Cervical CD4+ T Cell Subset Coexpressing Multiple Markers of HIV Susceptibility. J. Immunol. 187 (11), 6032–6042. doi: 10.4049/jimmunol.1101836
Medzhitov, R. (2010). Inflammation 2010: New Adventures of an Old Flame. Cell. 140 (6), 771–776. doi: 10.1016/j.cell.2010.03.006
Mhatre, M., McAndrew, T., Carpenter, C., Burk, R. D., Einstein, M. H., Herold, B. C. (2012). Cervical Intraepithelial Neoplasia is Associated With Genital Tract Mucosal Inflammation. Sex Transm Dis. 39 (8), 591–597. doi: 10.1097/OLQ.0b013e318255aeef
Mitra, A., MacIntyre, D. A., Lee, Y. S., Smith, A., Marchesi, J. R., Lehne, B., et al. (2015). Cervical Intraepithelial Neoplasia Disease Progression is Associated With Increased Vaginal Microbiome Diversity. Sci. Rep. 5 (1), 16865. doi: 10.1038/srep16865
Monnier-Benoit, S., Mauny, F., Riethmuller, D., Guerrini, J. S., Căpîlna, M., Félix, S., et al. (2006). Immunohistochemical Analysis of CD4+ and CD8+ T-Cell Subsets in High Risk Human Papillomavirus-Associated Pre-Malignant and Malignant Lesions of the Uterine Cervix. Gynecol Oncol. 102 (1), 22–31. doi: 10.1016/j.ygyno.2005.11.039
Moscicki, A. B., Hills, N., Shiboski, S., Powell, K., Jay, N., Hanson, E., et al. (2001). Risks for Incident Human Papillomavirus Infection and Low-Grade Squamous Intraepithelial Lesion Development in Young Females. JAMA J. Am. Med. Assoc. 285, 2995–3002. doi: 10.1001/jama.285.23.2995
Moscicki, A.-B., Shi, B., Huang, H., Barnard, E., Li, H. (2020). Cervical-Vaginal Microbiome and Associated Cytokine Profiles in a Prospective Study of HPV 16 Acquisition, Persistence, and Clearance. Front. Cell. Infect. Microbiol. 10 (528). doi: 10.3389/fcimb.2020.569022
Moscicki, A. B., Shi, B., Huang, H., Barnard, E., Li, H. (2020). Cervical-Vaginal Microbiome and Associated Cytokine Profiles in a Prospective Study of HPV 16 Acquisition, Persistence, and Clearance. Front. Cell Infect. Microbiol. 10, 569022. doi: 10.3389/fcimb.2020.569022
Mtshali, A., San, J. E., Osman, F., Garrett, N., Balle, C., Giandhari, J., et al. (2021). Temporal Changes in Vaginal Microbiota and Genital Tract Cytokines Among South African Women Treated for Bacterial Vaginosis. Front. Immunol. 12, 730986. doi: 10.3389/fimmu.2021.730986
Myers, E. R., McCrory, D. C., Nanda, K., Bastian, L., Matchar, D. B. (2000). Mathematical Model for the Natural History of Human Papillomavirus Infection and Cervical Carcinogenesis. Am. J. Epidemiol. 151 (12), 1158–1171. doi: 10.1093/oxfordjournals.aje.a010166
Norenhag, J., Du, J., Olovsson, M., Verstraelen, H., Engstrand, L., Brusselaers, N. (2020). The Vaginal Microbiota, Human Papillomavirus and Cervical Dysplasia: A Systematic Review and Network Meta-Analysis. BJOG an Int. J. obstetrics gynaecol. 127 (2), 171–180. doi: 10.1111/1471-0528.15854
O'Byrne, K. J., Dalgleish, A. G. (2001). Chronic Immune Activation and Inflammation as the Cause of Malignancy. Br. J. Cancer. 85 (4), 473–483. doi: 10.1054/bjoc.2001.1943
Onywera, H., Anejo-Okopi, J., Mwapagha, L. M., Okendo, J., Williamson, A. L. (2021). Predictive Functional Analysis Reveals Inferred Features Unique to Cervicovaginal Microbiota of African Women With Bacterial Vaginosis and High-Risk Human Papillomavirus Infection. PloS One 16 (6), e0253218. doi: 10.1371/journal.pone.0253218
Onywera, H., Williamson, A. L., Mbulawa, Z. Z. A., Coetzee, D., Meiring, T. L. (2019). The Cervical Microbiota in Reproductive-Age South African Women With and Without Human Papillomavirus Infection. Papillomavirus Res. 7, 154–163. doi: 10.1016/j.pvr.2019.04.006
Palefsky, J. (2020) Human Papillomavirus Infections: Epidemiology and Disease Associations. Available at: https://www.uptodate.com/contents/human-papillomavirus-infections-epidemiology-and-disease-associations.
Pao, C. C., Lin, C. Y., Yao, D. S., Tseng, C. J. (1995). Differential Expression of Cytokine Genes in Cervical Cancer Tissues. Biochem. Biophys. Res. Commun. 214 (3), 1146–1151. doi: 10.1006/bbrc.1995.2405
Passmore, J. A., Jaspan, H. B., Masson, L. (2016). Genital Inflammation, Immune Activation and Risk of Sexual HIV Acquisition. Curr. Opin. HIV AIDS. 11 (2), 156–162. doi: 10.1097/COH.0000000000000232
Peghini, B. C., Abdalla, D. R., Barcelos, A. C., Teodoro, L., Murta, E. F., Michelin, M. A. (2012). Local Cytokine Profiles of Patients With Cervical Intraepithelial and Invasive Neoplasia. Hum. Immunol. 73 (9), 920–926. doi: 10.1016/j.humimm.2012.06.003
Petrova, M. I., van den Broek, M., Balzarini, J., Vanderleyden, J., Lebeer, S. (2013). Vaginal Microbiota and its Role in HIV Transmission and Infection. FEMS Microbiol. Rev. 37 (5), 762–792. doi: 10.1111/1574-6976.12029
Pimple, S., Mishra, G. (2022). Cancer Cervix: Epidemiology and Disease Burden. Cytojournal. 19, 21. doi: 10.25259/CMAS_03_02_2021
Plummer, M., Schiffman, M., Castle, P. E., Maucort-Boulch, D., Wheeler, C. M. (2007). A 2-Year Prospective Study of Human Papillomavirus Persistence Among Women With a Cytological Diagnosis of Atypical Squamous Cells of Undetermined Significance or Low-Grade Squamous Intraepithelial Lesion. J. Infect. Dis. 195 (11), 1582–1589. doi: 10.1086/516784
Poirier, B., Sethi, S., Garvey, G., Hedges, J., Canfell, K., Smith, M., et al. (2021). HPV Vaccine: Uptake and Understanding Among Global Indigenous Communities – a Qualitative Systematic Review. BMC Public Health 21 (1), 2062. doi: 10.1186/s12889-021-12147-z
Ravel, J., Gajer, P., Abdo, Z., Schneider, G. M., Koenig, S. S., McCulle, S. L., et al. (2011). Vaginal Microbiome of Reproductive-Age Women. Proc. Natl. Acad. Sci. United States America. 108 Suppl 1, 4680–4687. doi: 10.1038/srep16865
Routes, J. M., Morris, K., Ellison, M. C., Ryan, S. (2005). Macrophages Kill Human Papillomavirus Type 16 E6-Expressing Tumor Cells by Tumor Necrosis Factor Alpha- and Nitric Oxide-Dependent Mechanisms. J. virol. 79 (1), 116–123. doi: 10.1128/JVI.79.1.116-123.2005
Sasagawa, T., Takagi, H., Makinoda, S. (2012). Immune Responses Against Human Papillomavirus (HPV) Infection and Evasion of Host Defense in Cervical Cancer. J. Infect. Chemother. 18 (6), 807–815. doi: 10.1007/s10156-012-0485-5
Scott, M. E., Shvetsov, Y. B., Thompson, P. J., Hernandez, B. Y., Zhu, X., Wilkens, L. R., et al. (2013). Cervical Cytokines and Clearance of Incident Human Papillomavirus Infection: Hawaii HPV Cohort Study. Int. J. cancer. 133 (5), 1187–1196. doi: 10.1002/ijc.28119
Shannon, B., Yi, T. J., Perusini, S., Gajer, P., Ma, B., Humphrys, M. S., et al. (2017). Association of HPV Infection and Clearance With Cervicovaginal Immunology and the Vaginal Microbiota. Mucosal Immunol. 10 (5), 1310–1319. doi: 10.1038/mi.2016.129
Song, D., Li, H., Li, H., Dai, J. (2015). Effect of Human Papillomavirus Infection on the Immune System and its Role in the Course of Cervical Cancer. Oncol. Lett. 10 (2), 600–606. doi: 10.3892/ol.2015.3295
Stanley, M. (2006). Immune Responses to Human Papillomavirus. Vaccine. 24(Suppl 1), S16–S22. doi: 10.1016/j.vaccine.2005.09.002
Stanley, M. (2008). Immunobiology of HPV and HPV Vaccines. Gynecol Oncol. 109 (2 Suppl), S15–S21. doi: 10.1016/j.ygyno.2008.02.003
Stanley, M. (2010). HPV - Immune Response to Infection and Vaccination. Infect. Agents Cancer. 5 (1), 19. doi: 10.1186/1750-9378-5-19
Steele, J. C., Roberts, S., Rookes, S. M., Gallimore, P. H. (2002). Detection of CD4(+)- and CD8(+)-T-Cell Responses to Human Papillomavirus Type 1 Antigens Expressed at Various Stages of the Virus Life Cycle by Using an Enzyme-Linked Immunospot Assay of Gamma Interferon Release. J. virol. 76 (12), 6027–6036. doi: 10.1128/JVI.76.12.6027-6036.2002
Stern, P. L., Brown, M., Stacey, S. N., Kitchener, H. C., Hampson, I., Abdel-Hady, E. S., et al. (2000). Natural HPV Immunity and Vaccination Strategies. J. Clin. Virol. 19 (1-2), 57–66. doi: 10.1016/S1386-6532(00)00128-1
Sung, H., Ferlay, J., Siegel, R. L., Laversanne, M., Soerjomataram, I., Jemal, A., et al. (2021). Global Cancer Statistics 2020: GLOBOCAN Estimates of Incidence and Mortality Worldwide for 36 Cancers in 185 Countries. CA Cancer J. Clin. 71 (3), 209–249. doi: 10.3322/caac.21660
Torcia, M. G. (2019). Interplay Among Vaginal Microbiome, Immune Response and Sexually Transmitted Viral Infections. Int. J. Mol. Sci. 20 (2), 1–13. doi: 10.3390/ijms20020266
UNAIDS (2017). Joint United Nations Programme on HIV/AIDS, Geneva, Switzerland. https://www.unaids.org/sites/default/files/media_asset/Global_AIDS_update_2017_en.pdf.
Walch-Ruckheim, B., Mavrova, R., Henning, M., Vicinus, B., Kim, Y. J., Bohle, R. M., et al. (2015). Stromal Fibroblasts Induce CCL20 Through IL6/C/EBPbeta to Support the Recruitment of Th17 Cells During Cervical Cancer Progression. Cancer Res. 75 (24), 5248–5259. doi: 10.1158/0008-5472.CAN-15-0732
Wang, R., Pan, W., Jin, L., Huang, W., Li, Y., Wu, D., et al. (2020). Human Papillomavirus Vaccine Against Cervical Cancer: Opportunity and Challenge. Cancer Lett. 471, 88–102. doi: 10.1016/j.canlet.2019.11.039
Ward, H., Rönn, M. (2010). Contribution of Sexually Transmitted Infections to the Sexual Transmission of HIV. Curr. Opin. HIV AIDS. 5 (4), 305–310. doi: 10.1097/COH.0b013e32833a8844
Wei, Z. T., Chen, H. L., Wang, C. F., Yang, G. L., Han, S. M., Zhang, S. L. (2020). Depiction of Vaginal Microbiota in Women With High-Risk Human Papillomavirus Infection. Front. Public Health 8, 587298. doi: 10.3389/fpubh.2020.587298
Westrich, J. A., Warren, C. J., Pyeon, D. (2017). Evasion of Host Immune Defenses by Human Papillomavirus. Virus Res. 231, 21–33. doi: 10.1016/j.virusres.2016.11.023
WHO (2022). Cervical Cancer. Available at: https://www.who.int/news-room/fact-sheets/detail/cervical-cancer
Woodby, B. L., Songock, W. K., Scott, M. L., Raikhy, G., Bodily, J. M. (2018). Induction of Interferon Kappa in Human Papillomavirus 16 Infection by Transforming Growth Factor Beta-Induced Promoter Demethylation. J. virol. 92 (8), 1–17. doi: 10.1128/JVI.01714-17
Keywords: human papillomavirus, cellular, cytokines, inflammation, vaginal microbiota
Citation: Ntuli L, Mtshali A, Mzobe G, Liebenberg LJP and Ngcapu S (2022) Role of Immunity and Vaginal Microbiome in Clearance and Persistence of Human Papillomavirus Infection. Front. Cell. Infect. Microbiol. 12:927131. doi: 10.3389/fcimb.2022.927131
Received: 09 May 2022; Accepted: 14 June 2022;
Published: 07 July 2022.
Edited by:
Dohun Pyeon, Michigan State University, United StatesReviewed by:
Joseph Westrich, Colorado State University, United StatesJose Eleuterio Junior, Federal University of Ceara, Brazil
Copyright © 2022 Ntuli, Mtshali, Mzobe, Liebenberg and Ngcapu. This is an open-access article distributed under the terms of the Creative Commons Attribution License (CC BY). The use, distribution or reproduction in other forums is permitted, provided the original author(s) and the copyright owner(s) are credited and that the original publication in this journal is cited, in accordance with accepted academic practice. No use, distribution or reproduction is permitted which does not comply with these terms.
*Correspondence: Sinaye Ngcapu, sinaye.ngcapu@caprisa.org