- 1Key Laboratory of Medical Molecular Virology (Ministry of Education (MOE)/National Health Commission (NHC)/Chinese Academy of Medical Sciences (CAMS)), School of Basic Medical Science, Fudan University, Shanghai, China
- 2Shanghai Institute of Infectious Disease and Biosecurity, School of Public Health, Fudan University, Shanghai, China
Pseudomonas aeruginosa is an opportunistic pathogen causing nosocomial infections in severely ill and immunocompromised patients. Ubiquitously disseminated in the environment, especially in hospitals, it has become a major threat to human health due to the constant emergence of drug-resistant strains. Multiple resistance mechanisms are exploited by P. aeruginosa, which usually result in chronic infections difficult to eradicate. Diverse virulence factors responsible for bacterial adhesion and colonization, host immune suppression, and immune escape, play important roles in the pathogenic process of P. aeruginosa. As such, antivirulence treatment that aims at reducing virulence while sparing the bacterium for its eventual elimination by the immune system, or combination therapies, has significant advantages over traditional antibiotic therapy, as the former imposes minimal selective pressure on P. aeruginosa, thus less likely to induce drug resistance. In this review, we will discuss the virulence factors of P. aeruginosa, their pathogenic roles, and recent advances in antivirulence drug discovery for the treatment of P. aeruginosa infections.
1 Introduction
Pseudomonas aeruginosa is a Gram-negative bacterium widely distributed in the environment, usually inhabiting soil, water, plants and humans (Wu and Li, 2015). It is also an environmentally resilient microorganism that can grow in nutrient-deficient conditions and live in a broad temperature range from 4 to 42°C. The tenacious adaptability and survivability of P. aeruginosa allows itself to survive on dry, abiotic surfaces in hospitals for up to 6 months (Diggle and Whiteley, 2020). As one of the main causative agents of opportunistic nosocomial infections, P. aeruginosa primarily infects the gravely ill or immunocompromised patients, especially those with severe burn/surgery wounds, cancer or AIDS. In fact, hospital-acquired P. aeruginosa causes dermatitis, bacteremia, and infections of the respiratory and urinary tracts and of other vital organs, accounting for 10-20% nosocomial cases (Carmeli et al., 1999; Al-Hasan et al., 2008) and 50% fatality (Gómez and Prince, 2007).
Frequent occurrence of drug resistance and persistent colonization on humid surfaces makes P. aeruginosa particularly difficult to treat and eradicate. Multiple intrinsic and acquired resistant mechanisms are exploited by P. aeruginosa, including but not limited to inactivation of antibiotics, modification of drug targets, attenuation of membrane permeability, expression of efflux systems, formation of biofilms and quorum-sensing, which, collectively, contribute to its distinctly low antibiotic susceptibility (Dao et al., 2011; Poole, 2011; Gellatly and Hancock, 2013; Taylor et al., 2014). As a member of the life-threatening “ESKAPE bugs” (standing for Enterococcus faecium, Staphylococcus aureus, Klebsiella pneumoniae, Acinetobacter baumannii, Pseudomonas aeruginosa and Enterobacter species) known for their increasing prevalence of drug resistance and bacterial virulence (Rice, 2008), P. aeruginosa is designated by World Health Organization as a top antibiotic-resistant “priority pathogen” for which new antibiotics are critically required (World Health Organization, 2017).
Therapies targeting bacterial virulence are an effective approach to alleviating growing bacterial resistance to traditional antibiotics that aim to either kill bacteria or inhibit their growth. Antivirulence strategies focus on neutralizing bacterial virulence factors and reducing bacterial infectivity without direct killing of the bacteria. Consequently, there is less selective pressure on the survival of bacteria, thus less likely to induce drug resistance (Maura et al., 2016; Dickey et al., 2017). P. aeruginosa is armed with a variety of virulence factors, such as flagella, pilli and LPS that contribute to bacterial adhesion/colonization to the host, secretion systems that deliver effectors and toxins into the host, proteases and toxins that cause tissue damage, and quorum-sensing and biofilm that confer bacterial communication and drug resistance (Hauser, 2009; Veesenmeyer et al., 2009; Poole, 2011; Gellatly and Hancock, 2013; Newman et al., 2017). A considerable number of antivirulence drug candidates against P. aeruginosa are in preclinical and clinical trials, including antibodies, small molecule inhibitors and alginate oligomers (Cegelski et al., 2008; Rasko and Sperandio, 2010; Fleitas Martínez et al., 2019b). This review summarizes the virulence factors of P. aeruginosa and their pathogenic mechanisms, followed by discussions of various antivirulence strategies to fend off P. aeruginosa infections.
2 The Virulence Factors of P. Aeruginosa
The virulence of pathogens entails their ability to infect the host and to cause clinical symptoms through factors that contribute to bacterial attachment to and colonization and invasion of the host, interruption of host tissue integration, suppression of and escape from host immune response, and depletion of nutrients from the host (Rasko and Sperandio, 2010; Mühlen and Dersch, 2016; Dickey et al., 2017; Diggle and Whiteley, 2020). Successful antivirulence therapy requires a full understanding of bacterial virulence factors and their pathogenic mechanisms so that appropriate drugs can be developed for infection treatment. The virulence factors of P. aeruginosa have been thoroughly studied and are classified differently in various literatures. For clarity and a better readership, we divide them into three main categories, namely bacterial surface structures, secreted factors and bacterial cell-to-cell interaction, which can be further divided into several subclasses as shown in Figure 1 and Table 1.
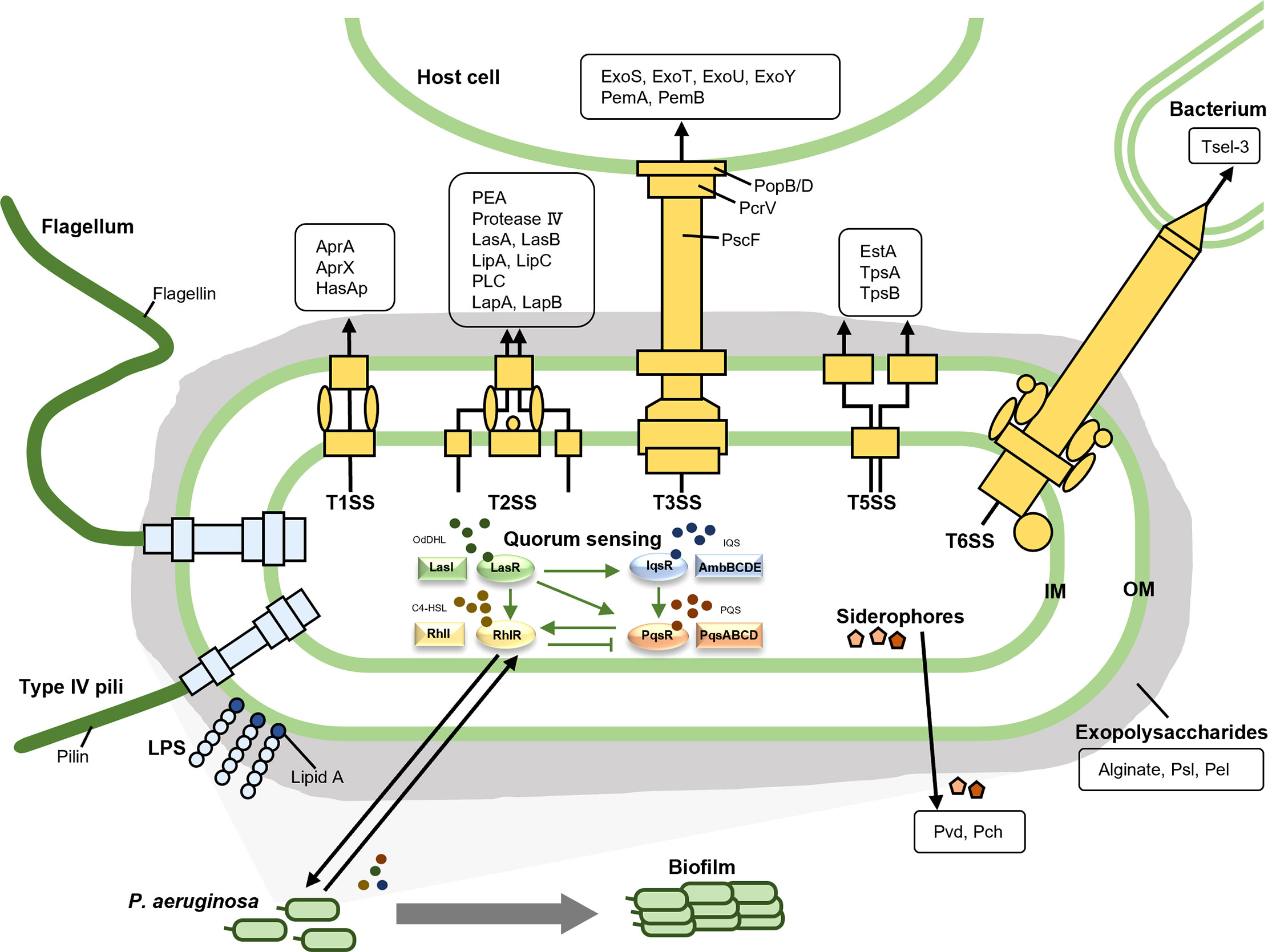
Figure 1 The virulence factors of P. aeruginosa. The virulence factors of P. aeruginosa are divided into three main categories, namely bacterial surface structures, secreted factors, and bacterial cell-to-cell interaction. The bacterial surface structures include surface appendages like type IV pili and flagella, outer membrane components such as lipopolysaccharide, and five secretion systems (T1SS, T2SS, T3SS, T5SS, and T6SS). The secreted factors are illustrated in the black boxes. As for the bacterial cell-to-cell interaction, quorum sensing and biofilm are listed here.
2.1 Bacterial Surface Structures
2.1.1 Surface Appendages
Type IV pili of P. aeruginosa is a motorized fimbriae composed of repeated copies of a 15-kDa protein termed pilin, with three subtypes named Type IVa pili, Type IVb pili and Type IVb-Tad pili (Hahn, 1997; Burrows, 2012a). It is associated with bacterial twitching and swarming motility and adhesion on various surfaces, and plays an important role in biofilm formation, regulation of virulence factors, and bacterial exchange of antibiotic resistance genes (Whitchurch et al., 2004; Overhage et al., 2008; Burrows, 2012b; Persat et al., 2015; Talà et al., 2019). Repeated extension and retraction of type IV pili are driven by cytoplasmic ATPases, facilitating bacterial motility, taxis, and attachment, which in turn contribute to self-organization of microcolonies, formation of biofilms, and uptake of DNAs (Craig et al., 2019). As an important adhesin, type IV pili also enables bacteria to be in intimate contact with surfaces and to influence biofilm formation by regulating cyclic-di-GMP levels (Barken et al., 2010; Webster et al., 2021).
Flagella of P. aeruginosa are hairlike appendages protruding from the bacterial surface, mainly comprising the protein subunits called flagellin. As a motility apparatus that enables bacterial movement and chemotaxis, flagella contribute to bacterial adhesion via flagellin and the flagellar cap protein FliD, and promote biofilm maturation of P. aeruginosa (Dasgupta et al., 2004; Ozer et al., 2021). Flagella can elicit activation of host immune response through Toll-like receptor 5 (TLR5), and are highly immunogenic (Feuillet et al., 2006; Campodónico et al., 2010). Therefore, vaccination with flagellar-antigen preparations and a cocktail vaccine including flagellin B has successfully elicited protective immunity in mouse models (Holder et al., 1982; Faezi et al., 2017; Hassan et al., 2018).
2.1.2 Outer Membrane Components
Lipopolysaccharide (LPS) is a major component of the outer membrane in Gram-negative bacteria, and can be found in all P. aeruginosa strains (Pier, 2007). Composed of lipid A moiety, inner and outer core oligosaccharides, as well as the O-antigen, LPS may be the most extensively studied bacterial molecule due to its high immunogenicity and surface accessibility (Triantafilou and Triantafilou, 2002; Miller et al., 2005). As a microbe-associated molecular pattern (MAMP), LPS can be a potent activator of host immune response via various signal transduction pathways including toll-like receptor 4 (TLR4) and the cystic fibrosis transmembrane conductance regulator (CFTR) (Pier, 2007; Huszczynski et al., 2020). Paradoxically, LPS can stimulate neutrophils to release neutrophil extracellular traps (NETs) to capture invading pathogens, but protect bacteria from phagocytosis (Pier, 2007; Huszczynski et al., 2020). The interaction between LPS and eukaryotic cells contributes to the adherence of P. aeruginosa to the host (Goldberg and Pier, 1996). Of note, even though various LPS-targeted vaccines have been created against P. aeruginosa with encouraging results, most of them failed to show satisfactory efficacy (Hanessian et al. (1971); Cryz et al., 1987; Cryz Jr et al. (1997); Hatano and Pier, 1998; Döring, 2010).
2.1.3 Secretion Systems
Type 1 secretion system (T1SS) is one of the simplest secretion systems in Gram-negative bacteria that plays an active virulent role during the inflammatory phase in P. aeruginosa infection process (Bleves et al., 2010). There exist two types of T1SS in P. aeruginosa, Apr T1SS and HasF T1SS. The Apr T1SS consists of AprD, AprE, and AprF, and is involved in the secretion of alkaline proteases AprA and AprX; the HasF T1SS, composed of HasD, HasE, and HasF, is involved in the secretion of heme acquisition protein HasAp as well as utilization of iron (Duong et al., 2001; Wandersman and Delepelaire, 2004; Bleves et al., 2010).
Type 2 secretion system (T2SS) of P. aeruginosa secretes major extracellular toxins that possess diverse activities associated with the infection of patients with potential respiratory diseases (Proctor et al., 2021). In P. aeruginosa, two T2SS’s have been characterized, Xcp T2SS and Hxc T2SS. While the former secretes numerous exoproteins that participate in the bacterial infection process, including Exotoxin A (PEA), Protease IV, Elastase A and B (LasA and LasB), Lipase A and C (LipA and LipC), and Phospholipase C (PLC), the latter only secretes the low molecular weight alkaline phosphatases, LapA and LapB, that contribute to extracellular alkaline phosphatase activity under phosphate-limiting conditions (Ball et al., 2002; Bleves et al., 2010; Ball et al., 2012).
Type 3 secretion system (T3SS), composed of the needle complex, effectors, chaperones and regulation proteins, is one of the most important virulence factors of P. aeruginosa (Cornelis, 2006; Sato and Frank, 2011). The needle complex is formed by a basal body, a needle filament and a translocon, which protrudes from the bacterial surface to act as a “molecular syringe” to inject effector proteins of P. aeruginosa into host cells that come in contact. The basal body of the needle complex consists of ATPase complex, cytoplasmic ring (C ring), export apparatus and the cytoplasmic base, whereas the needle filament is formed by multiple copies of a protein termed PscF; the translocon is composed of two pore-forming proteins (termed PopB and PopD) and the needle-pore bridge PcrV. The effectors are commonly injected into host cells by the T3SS to initiate bacterial invasion and exert cytotoxicity. ExoS, ExoT, ExoU, and ExoY are four well-studied T3SS effectors of P. aeruginosa, while the other two, PemA and PemB, still remain obscure. T3SS chaperones are proteins facilitating secretion or assembly of their cognate partners such as effectors and structural proteins of the T3SS. Proteins that regulate the secretion process are mostly transcriptional modulators, secretion or traffic regulators, and chaperones (Hauser, 2009; Horna and Ruiz, 2021b).
The T3SS enables P. aeruginosa to invade and infect the host and promotes pathogenicity. It is directly related to the clinical manifestations and severity of infections, and acts as a key factor of bacterial virulence. Mutations in T3SS effectors can reduce or abolish bacterial virulence (Zhang et al., 2018); the lethality of P. aeruginosa in patients infected by strains lacking PcrV is greatly reduced, compared with those possessing PcrV (Roy-Burman et al., 2001). The close association of the T3SS with the severity of clinical infections makes it one of the most extensively studied virulence factors of P. aeruginosa, and certainly one of the most promising therapeutic targets for antivirulence drug discovery and development (Horna and Ruiz, 2021b).
Type 5 secretion system (T5SS) is the simplest secretion system of P. aeruginosa, which can be divided into autotransporters (AT) and a two-partner secretion system (Ma et al., 2020). The T5SS secretes a variety of proteins related to bacterial virulence and adhesion, including EstA, TpsA and TpsB (Ma et al., 2020). EstA can increase the expression of rhamnolipid and, subsequently, promote biofilm formation; TpsA and TpsB are two β-barrel outer membrane exoproteins that mainly secrete large virulence proteins (>100 kDa), participate in immune escape, and enhance bacterial adhesion (Jacob-Dubuisson et al. (2001); Henderson et al., 2004). In addition, autotransport proteases, as one of the main secretions of the T5SS, plays a crucial role in nutrient acquisition. AaaA, for example, is such an autotransport protease involved in nitrogen acquisition of peptides in chronic infections (Hood et al., 2010).
Type 6 secretion system (T6SS) is a versatile secretion system where three independent T6SS hemolysin coregulated protein (Hcp) secretion islands (H1-, H2- and H3-T6SS) have been characterized in P. aeruginosa (Mougous et al. (2006); Basler et al., 2013). The bacteriolytic effectors Tse1-3 secreted by H1-T6SS can hydrolyze the peptidoglycan cell wall of competing bacteria, while the effectors PldA and PldB of H2- and H3-T6SS can promote bacterial endocytosis into host cells via the PI3K/Akt pathway (Russell et al., 2011; Jiang et al., 2014; Bleves, et al., 2016).
2.2 Secreted Factors
2.2.1 Exopolysaccharides
Exopolysaccharides are sugar-based extracellular macromolecules secreted by P. aeruginosa to enhance bacterial tolerance to harsh survival environments such as desiccation, oxidizing agents, and host defense (Franklin et al., 2011). As one of the main compositions of extracellular polymeric substances essential for the functional and structural integrity of biofilms, exopolysaccharides are important for P. aeruginosa in biofilm formation, and can also act as adhesins contributing to bacterial persistence in patients (Nivens et al., 2001; Song et al., 2003; Ellis and Kuehn, 2010). At present, alginate, Psl and Pel are three exopolysaccharides discovered in P. aeruginosa. Alginate is generally secreted by the strains isolated from cystic fibrosis patients, while Psl and Pel are mainly produced by the strains obtained from the environment (Franklin et al., 2011). Although the mechanisms of their action remain poorly understood, the antibodies targeting alginate or Psl have been developed for antivirulence therapy (DiGiandomenico et al., 2014; Loos et al., 2019).
2.2.2 Siderophores
Siderophores are iron-chelating compounds secreted by the bacteria to help with iron accumulation. Two siderophores are produced by P. aeruginosa: the fluorescent high-affinity peptidic pyoverdine (Pvd) and pyochelin (Pch) with a relatively lower affinity (Cornelis, 2010b). Pvd and Pch can chelate irons, especially Fe3+, from transferrin and lactoferrin to promote bacterial growth, and are both required for full virulence of P. aeruginosa (Sass et al., 2020). Despite being of low-affinity for irons, pyochelin can be produced by the bacteria in place of pyoverdine, even in the presence of strong iron chelators (Cunrath et al., 2020; Pan et al., 2021), indicative its indispensable role in the iron niche of Pseudomonas aeruginosa.
2.2.3 Proteases
Alkaline protease (AprA) is a virulence factor secreted by type I secretion system and controlled by the quorum-sensing circuit (Bleves et al., 2010). AprA can degrade complement components, as well as IFN-γ and TNF-α, thereby counteracting host immune defense and exacerbating infections in the body (Matsumoto et al., 1998). Elastase A and B (LasA and LasB) are produced by P. aeruginosa to destroy elastin, an important component of the pulmonary tissue and blood vessels, impairing lung function and causing pulmonary hemorrhage (Kessler et al., 1997). Protease IV is significantly associated with corneal virulence of P. aeruginosa (Bradshaw et al., 2018). Not only can protease IV interfere with host immunity by degrading various biologically important molecules, such as complement components, immunoglobulins, and surfactant proteins, but also damage host tissues and enhance bacterial infection through degradation of fibrinogen, lactoferrin, transferrin, and elastin (Engel et al. (1998); Malloy et al., 2005; Cheng, 2016; O'Callaghan et al., 2019). Since protease IV is a serine protease, known serine protease inhibitors that block its enzymatic activity may be developed into therapeutics to prevent tissue damage caused by P. aeruginosa infection.
2.2.4 Toxins
The four effectors secreted by the T3SS, namely ExoS, ExoT, ExoU, and ExoY, are commonly referred to as the toxins of P. aeruginosa. ExoS and ExoT, sharing a 76% sequence similarity, are both bifunctional cytotoxins with GTPase activating protein (GAP) activity and adenosine diphosphate ribosyl transferase (ADPRT) activity. They can disrupt the host actin cytoskeleton to interfere with cell-to-cell adhesion, and induce apoptosis of host cells. ExoU is a potent phospholipase and the most virulent T3SS effector that causes rapid necrotic cell death. Notably, ExoU and ExoS almost never co-exist in one strain. ExoY can be classified as an adenylate or nucleotidyl cyclase that significantly increases the levels of cAMP, cGMP, cUMP and, to a lesser extent, cCMP. Found in over 90% analyzed isolates, ExoT and ExoY are the two most frequent T3SS effectors in P. aeruginosa (Hauser, 2009; Horna and Ruiz, 2021b; Horna and Ruiz, 2021a). While the strains expressing ExoS are closely related to poor prognosis of infected patients, strains expressing ExoU are usually more resistant to antibiotics (Choy et al., 2008; Agnello and Wong-Beringer, 2012; Shen et al., 2015; Agnello et al., 2016; Horna et al., 2019).
Exolysin (ExlA) is a pore-forming toxin identified from the P. aeruginosa clinical isolate CLJ1 that lacks a T3SS locus (Elsen et al., 2014). ExlA is encoded by an exclusive two-gene genetic element——exlA-exlB, and displays cytolysin activity. ExlA induces plasma membrane permeabilization, resulting in necrotic cell death, a process dependent on the type IV pili for bacterial adhesion (Elsen et al., 2014; Pauline et al., 2017).
Extoxin A of P. aeruginosa (PEA) is one of the most toxic exocellular factors secreted by the T2SS under regulation by iron and glycose metabolisms (Lamont et al., 2002; Balasubramanian et al., 2012; Abdelali et al., 2014). PEA inhibits host protein synthesis by catalyzing the ADP ribosylation of cell elongation factor 2 (EF-2) and induces programmed cell death (Michalska and Wolf, 2015).
Lipase A (LipA) is the major extracellular lipase secreted by P. aeruginosa type II secretion system (Verma et al., 2020). It can wreak havoc on tissues by degrading the major lung surfactant lipid dipalmitoylphosphatidylcholine as well as host cell membranes (Zhang and Zhang, 2021). In addition, studies have shown that LipA interacts with alginate in the extracellular biofilm matrix produced by P. aeruginosa itself through electrostatic interactions, contributing to bacterial drug resistance (Viegas et al., 2020; Zhang and Zhang, 2021).
Phospholipase C (PLC) is produced by P. aeruginosa in two forms: hemolytic and non-hemolytic. Several studies have shown that hemolytic PLC can induce host vascular permeability, organ damage and cell death (Vasil et al., 1991). Low concentrations of hemolytic PLC can increase IL-8 expression, and cause excessive neutrophil recruitment involved in pulmonary inflammation and tissue destruction (König et al., 1997). In addition, hemolytic PLC inhibits neutrophil respiratory burst by interfering with a protein kinase C (PKC)-specific, non-p38 kinase-dependent signaling pathway, contributing to P. aeruginosa survival in an immune environment rich in neutrophils and, thus, chronic bacterial infection (Terada et al., 1999; Monturiol-Gross et al., 2021). Published animal studies have shown that inhibition of hemolytic PLC affords a significant protective effect on the function of P. aeruginosa-infected lungs (Wargo et al., 2011).
Lipoxygenase (LoxA), a lipoxygenase secreted by P. aeruginosa, can interfere with host lipid signaling and regulate the bacterial invasion process (Morello et al., 2019b). During P. aeruginosa infection of the lung, LoxA oxidizes a variety of host polyunsaturated fatty acids, and produces lipid peroxidative mediators such as lipoxin A4, resulting in host cell death (Lu et al., 2020). LoxA also inhibits the expression of major chemokines such as MIP and KC and the subsequent recruitment of immune cells (Kalms et al., 2017; Aldrovandi et al., 2018). Inhibiting the activity of LoxA may help alleviate the severity of P. aeruginosa infection (Dar et al., 2022).
Leukocidin is also a virulence factor secreted by P. aeruginosa, which is cytotoxic to leukocytes in the host immune system (Homma et al., 1984; Bouillot et al., 2020). Specifically, leukocidin can cause swelling of leukocytes via increased permeability of the plasma membrane, and attenuate immune function, thereby contributing to the infection of the host by P. aeruginosa (Scharmann, 1976; Hirayama et al., 2013).
Pyocyanin (PCN) is a blue secondary metabolite with redox activity found in sputum from P. aeruginosa infected lungs (Wilson et al., 1988; Lau et al., 2004). The zwitterionic characteristic of PCN facilitates its entry into the cytoplasmic membrane of the host, where the oxidative stress induced by PCN contributes to its cytotoxicity to host cells (Hall et al., 2016).
2.3 Bacterial Cell-To-Cell Interaction
2.3.1 Quorum-Sensing
Quorum sensing (QS) is a bacterial cell-to-cell communication system, in which the bacteria recognize the self-secreted small signal molecules called autoinducers, coordinate expression of multiple genes involved in bacterial community behaviors, virulence, and biofilm formation (Cegelski et al., 2008). Four QS pathways exist in P. aeruginosa, which are las, rhl, iqs, and pqs, utilizing respectively four autoinducers, namely N-(3-oxododecanoyl) -l-HSL (OdDHL), N-butanoyl-L-homoserine lactone (C4-HSL), 2-(2-hydroxyphenyl) -thiazole-4-carbaldehyde (IQS), and 2-heptyl-3-hydroxy-4(1H)-quinolone (PQS) (Dickey et al., 2017). Under certain conditions with adequate bacterial density, the autoinducers will be produced and combine with their receptors to control the transcription of related toxicity genes (Yaeger et al., 2021). QS plays a key role in the virulence of P. aeruginosa, such as regulating the release of multiple virulence factors (including elastase, alkaline protease, exotoxin A, rhamnolipids, pyocyanin and lipase) and promoting the maturation of biofilm as shown by Figure 2 (O'Donnell et al., 2020; Rezzoagli et al., 2020).
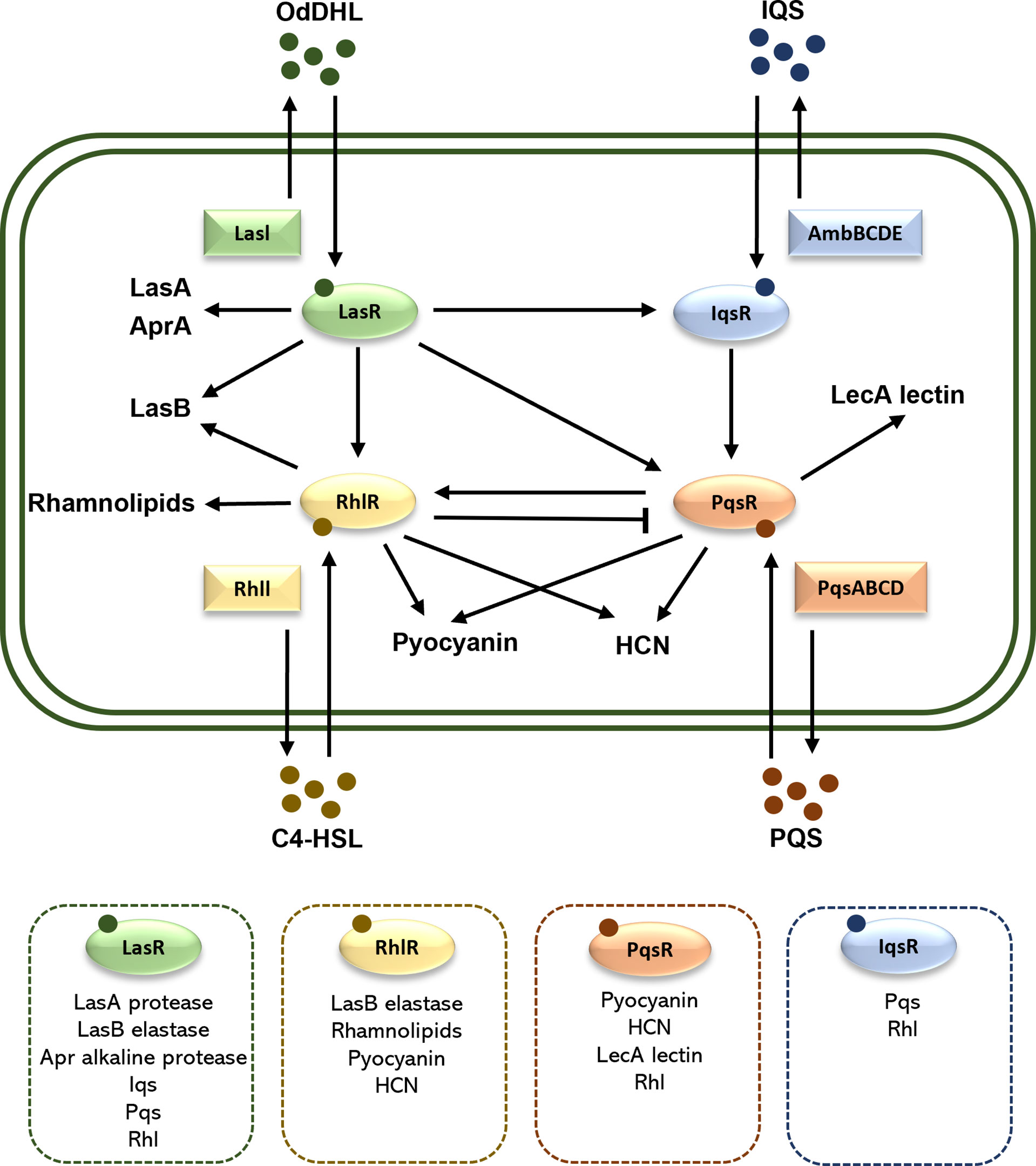
Figure 2 Schematic illustration of the QS system in P. aeruginosa. There are four QS systems in P. aeruginosa, las, rhl, iqs and pqs. They included respectively the receptors LasR, RhlR, IqsR, and PqsR, the autoinducers OdDHL, C4HSL, IQS, and PQS, as well as the transcription factors LasI, RhlI, AmbBCDE, and PqsABCD. The arrows indicate a stimulatory effect, while the perpendicular line indicates an inhibitory effect. In the dashed box are the associated virulence factors and QS systems. HCN, hydrogen cyanide.
2.3.2 Biofilm
Biofilm is the specific aggregated forms of bacteria encased in extracellular polymeric substances (EPS), which can help pathogenic bacteria sidestep unfavorable conditions such as temperature fluctuation, nutrient deficiency and antibiotics killing, while increasing bacterial persistency on biotic and abiotic surfaces (Rollet et al., 2009; Thi et al., 2020). The ability of biofilm formation is the most significant hallmark of P. aeruginosa, and the highly structured biofilms can usually be found in chronically infected patients (Mann and Wozniak, 2012; Römling and Balsalobre, 2012). Biofilm development is complicated and multiregulated, in which QS plays an essential role and its related virulence factors (such as LipA and LipC) can increase thickness and robustness of biofilms (Rosenau et al., 2010; Tielen et al., 2010; Tielen et al., 2013).
3 Current Antivirulence Strategies to Combat P. Aeruginosa
Despite the large number of different types of virulence factors exploited by P. aeruginosa, screening for suitable antivirulence therapeutic targets is somehow challenging, which necessitates a clear understanding of the pathogenic mechanisms of this bacterium. The pathogenesis of P. aeruginosa has been extensively studied. In fact, this Gram-negative bacterium is generally referred to as a model pathogen for drug development targeting conserved virulence factors, such as T3SS, quorum sensing and biofilm formation (Dickey et al., 2017). In addition, some virulence factors unique to P. aeruginosa, such as siderophores, specific proteases and exopolysaccharide, are also used as targets for the development of drugs for P. aeruginosa. Further, there are some drugs under development that target the bacterial surface structures such as flagella and LPS. These antivirulence therapeutics aim to interrupt the pathways critical for pathogenesis but not essential for bacterial growth by adopting the following strategies: interfering with the expression of bacterial virulence factors, neutralizing existing toxins, inhibiting quorum sensing and biofilm formation, and preventing bacterial attachment to and invasion of the host.
Current antivirulence drug candidates can be classified into the categories of antibodies, small molecules, polypeptides and others. The antibodies can be fully structured monoclonal antibodies, modified bispecific antibodies, or immunoglobulin preparations; the small molecules are usually screened from libraries of natural products or synthetic chemicals; the polypeptides are mostly antibiotics that tend to kill the bacteria as exemplified by colistin and Murepavadin; chitosan and alginate oligomers encompass other class of potential drugs. We searched the clinical trial database related to P. aeruginosa on ClinicalTrial.gov and preclinical studies on PubMed. On the basis of drug targets, the representative drug candidates for antivirulence therapy listed in Table 2 are classified into 5 types: (1) disrupting T3SS function, (2) inhibiting quorum sensing and biofilm formation, (3) neutralizing secreted virulence factors, (4) targeting other bacterial surface structures, and (5) modulating bacterial metabolisms.
3.1 Disrupting T3SS Functions
Among the five secretion systems discovered in P. aeruginosa, the T3SS is regarded as the most relevant to human pathogenesis (Horna and Ruiz, 2021b). Since the T3SS exists in most gram-negative pathogens such as Pseudomonas, Shigella, Salmonella, Escherichia coli, Vibrio, Yersinia, and etc, antivirulence drugs targeting the T3SS have the potential to be effective against various pathogenic bacteria (Coburn et al., 2007). The current drug candidates targeting the T3SS are primarily modified antibodies and screened/designed small molecules.
MEDI3902 (BiS4αPa) is a bivalent human immunoglobulin IgG1κ monoclonal antibody that bi-specifically targets PcrV and Psl of P. aeruginosa (DiGiandomenico et al., 2014). PcrV is one of the major components of T3SS translocation apparatus, located at the T3SS needle tip, and acts as the bridge between the needle and the pore on the host cell membrane triggered by the T3SS. PcrV facilitates injection of T3SS effectors into the host, and its deprivation causes loss of virulence in P. aeruginosa (Sawa et al., 1999). Psl, as one of the main structural components of P. aeruginosa biofilms, is a mannose- and galactose-rich exopolysaccharide that plays an important role in bacterial attachment to the host. Targeting both PcrV and Psl by MEDI3902 remarkably reduced P. aeruginosa infection in animal models (DiGiandomenico et al., 2014). Although a Phase 1 clinical trial in healthy subjects confirmed the safety and efficacy of MEDI3902 (Ali et al., 2019), results from a Phase 2 study were unsatisfactory (NCT02696902). Another antibody-derived drug candidate KB001-A, a recombinant, PEGylated Fab targeting PcrV, also showed ineffectiveness in phase 1 and 2 clinical studies despite its safety record (Jain et al., 2018).
Aside from antibodies, several small molecule candidates were developed for antivirulence therapy of P. aeruginosa. Fluorothiazinon (FT), a T3SS inhibitor derived from 2,4-disubstituted-4H-[1,3,4]-thiadiazine-5-ones, inhibits secretion of T3SS effectors ExoT and ExoY and protects mice from P. aeruginosa infections (Sheremet et al., 2018), and is currently in a phase 2 clinical trial (NCT03638830). However, the specific target of FT in P. aeruginosa T3SS remains unclear, and its mechanism of action warrants further investigation (Horna and Ruiz, 2021a). INP1855, a hydroxyquinoline, reduces mortality of mice infected by P. aeruginosa by possibly targeting PscN, an ATPase of the T3SS involved in recruitment and secretion of various effectors, and flagella that mediate cytotoxicity (Anantharajah et al., 2016). More detailed mechanistic studies are needed confirm its mode of action.
We recently developed a fluorescence-polarization-based, high-throughput screening strategy for antivirulence drug discovery targeting the T3SS. Dhydrotanshinone (dHTSN) and dihydrotanshinone 1 (dHTSN1), derivatives of a subset of natural herbal compounds termed tanshinones used for the treatment of cardiovascular and cerebrovascular diseases in traditional Chinese medicine, were discovered and validated to be host protective against P. aeruginosa. These tanshinone derivatives reduced T3SS effector secretion, alleviated cytotoxicity to macrophages, and rescued mice from lethal doses of P. aeruginosa infection in the lungs (Feng et al., 2019). The T3SS needle protein PscF is hydrophobic and highly prone to aggregation on its own. To prevent its premature degradation and ensure its proper translocation and needle assemly, the two bacterial chaperone proteins PscE and PscG associate into a dimer to sequester the nonpolar segments of PscF, forming a druggable trimeric structure (Quinaud et al., 2005). It is this PscF-PscE-PscG trimer that we used as a target for the screening of compound libraries, leading to the discovery of dHTSN and dHTSN1 as competitive inhibitors of PscF binding to PscE-PscG complex, or inhibitors of T3SS needle biogenesis (Figure 3) (Feng et al., 2019). Due to a proven safety track record of tanshinones in clinical use, these T3SS needle assembly inhibitors may have great potential in the therapy of P. aeruginosa-associated pulmonary infections, particularly those caused by antibiotic resistant strains.
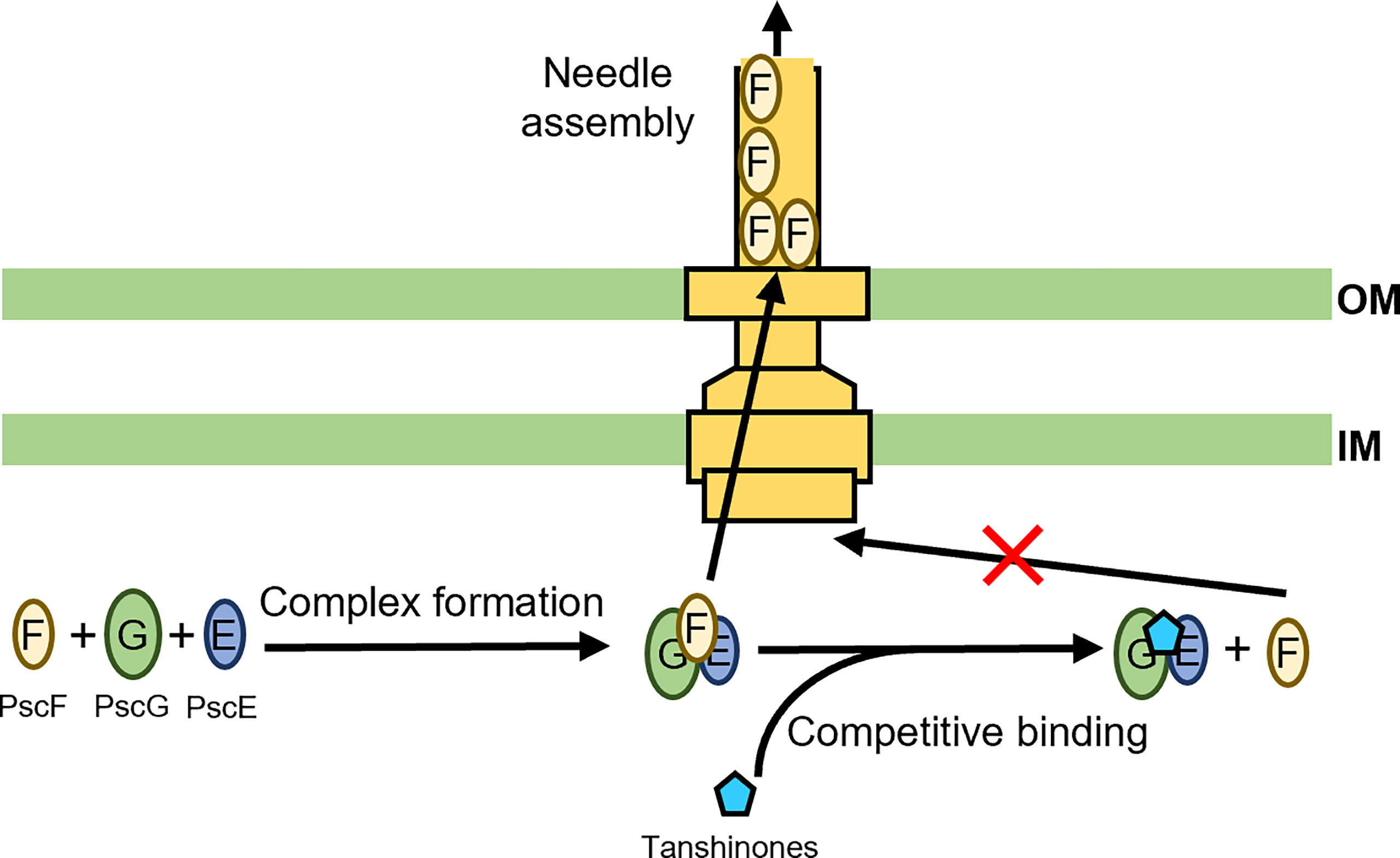
Figure 3 Schematic representation of tanshinones inhibiting T3SS needle elongation. PscF, PscG, and PscE form a complex that facilitates proper translocation of PscF and subsequent needle assembly (Quinaud et al., 2005; Feng et al., 2019). The tanshinones can act as competitive inhibitors to interrupt the complex formation, resulting in disruption of T3SS needle biogenesis (Quinaud et al., 2005; Feng et al., 2019).
3.2 Inhibiting Quorum Sensing and Biofilm Formation
Quorum sensing (QS) and biofilm formation are two complicated processes influenced by a variety of bacterial physiological activities. Biofilm formation contributes to bacterial persistency and resistance and is the consequence of multiple processes including, but not limited to, QS (Rollet et al., 2009; Thi et al., 2020). Inhibition of QS or biofilm formation can significantly attenuate bacterial virulence, which makes them potential targets for antivirulence therapy. Efficacy of current drug candidates targeting QS or biofilm formation of P. aeruginosa, mostly small molecules screened from chemical libraries or FDA-approved compounds, has been verified in animal models or in clinical trials.
Two candidates, M34 and Clofoctol, act as inhibitors of the pqs pathway, and suppress expression of pqs-related virulence traits in P. aeruginosa. The compound M34 was identified in a whole-cell high-throughput screen, where the pqsA-sacB and the pqsA-GFP bacterial strains were constructed for a readout that suppressed pqsA expression, and further optimized through a structure-activity relationship analysis. M34 and its derivatives possibly target the transcription factor PqsR, inhibit PQS-pqsR binding, block the synthesis of pqs-dependent signaling molecules, and protect mice from P. aeruginosa infection (Starkey et al., 2014). Clofoctol, previously used in the treatment of infections by Gram-positive bacteria, is selected from a library of FDA-approved compounds; it inhibits the pqs system and reduces mortality of G. mellonella larvae infected by P. aeruginosa by possibly targeting PqsR as M34 (D'Angelo et al., 2018).
Furanone C-30, a synthetic derivative of furanones that were originally discovered in natural food, interferes with the las pathway via binding to the transcription receptor LasR, inhibits virulence factor expression, and increases bacterial susceptibility of P. aeruginosa biofilms to tobramycin (Hentzer et al., 2003; Wu et al., 2004; Rezzoagli et al., 2020). However, several clinical P. aeruginosa isolates with mexR and nalC mutations are resistant to QS inhibitory activity of furanone C-30 (Maeda et al., 2012). Two screened synthetic compounds, MHY1383 and MHY1387, can inhibit QS virulence by competitive activities interrupting the interaction between OdHSL and LasR, while potently suppressing the biofilm formation of P. aeruginosa via reducing intracellular c-di-GMP levels (Hwang et al., 2021).
Meta-bromo-thiolactone (mBTL) is a novel synthetic molecule designed as an inhibitor of LasR and RhlR, and inhibits both production of the virulence factor pyocyanin and biofilm formation in P. aeruginosa (O’Loughlin et al., 2013). Nitric oxide (NO), a common signaling molecule, can lower the level of c-di-GMP, a key regulator of P. aeruginosa biofilm formation and bacteria dispersal (Barraud et al., 2006; Cutruzzolà et al., 2016); results from a completed phase 2 study of NO have yet to be reported. OligoG CF-5/20 is an alginate oligomer derived from the seaweed Laminaria hyperborean, which inhibits P. aeruginosa biofilm formation via disruption of NDA-Ca2+-DNA bridges and biofilm exopolysaccharide matrix (Powell et al., 2018). Several clinical trials of OligoG CF-5/20 in different stages are either completed without published data or still ongoing. AR-501, the inhaled gallium citrate that acts as iron analog to starve bacteria, inhibits biofilm formation of P. aeruginosa; the Phase 1 and 2 study of this candidate is also ongoing (Woo et al., 2019).
3.3 Neutralizing Secreted Virulence Factors
Many virulence factors function after their secretion by P. aeruginosa. Notable examples include siderophores, protease, exopolysaccharide, and toxins. Much work aiming to neutralize secreted virulence factors focuses on siderophores, protease, and exopolysaccharide. Pyoverdine is an iron-scavenging siderophore of P. aeruginosa that ensures bacterial iron acquisition in the host, contributes to expression of different virulence factors, and is toxic itself. Three small molecule compounds, LK11, LK31, and LK31a, were discovered as pyoverdine inhibitors that protected, as bona fide antivirulence molecules, C. elegans from P. aeruginosa infection (Kirienko et al., 2018). LasB is an extracellular collagenase elastase. As a major virulence factor of P. aeruginosa, it cleaves host tissue components such as elastin or collagen, disrupts cell-to-cell junctions, and causes tissue damages. The mercaptoacetamide-based compounds, phendione and its iron complexes, were proven to be effective LasB inhibitors that prolonged survival of P. aeruginosa-infected G. mellonella larvae (Kany et al., 2018; Galdino et al., 2019; Kaya et al., 2022). Alginate, produced by P. aeruginosa strains isolated from cystic fibrosis (CF) patients with chronic pulmonary infections, plays an important role in the pathology of CF disease by contributing to bacterial adhesion and biofilm formation (May et al., 1991; Boyd and Chakrabarty, 1995; Franklin et al., 2011). Ebselen and its analogues can inhibit production of alginate by P. aeruginosa through covalent modification of Alg44, which interrupts the interaction between Alg44 and c-di-GMP (Kim et al., 2021). Notably, AR-105 is a broadly active human monoclonal antibody against P. aeruginosa alginate, and can protect mice from lethal challenges of P. aeruginosa. However, a Phase 2 clinical trial of AR-105 failed to demonstrate its efficacy compared with a placebo (Loos et al., 2019).
3.4 Targeting Other Bacterial Surface Structures
Aside from the T3SS, other bacterial surface structures such as flagella, pili and lipopolysaccharide (LPS) are also virulence determinants of P. aeruginosa responsible for host infection involving motility, attachment, and induction of inflammation, thus potential targets for antivirulence therapy (Veesenmeyer et al., 2009). KBPA-101 is a human monoclonal antibody against the LPS O-polysaccharide moiety of P. aeruginosa serotype IATS O11, affording full protection of mice infected by P. aeruginosa at low doses (< 5 μg/mouse). While the safety of KBPA-101 in humans has been confirmed in a Phase 1 trial, results from a Phase 2 study have yet to be published (Lazar et al., 2009; Horn et al., 2010; Secher et al., 2011). Of note, KBPA-101 exclusively binds to the O11 serotype of P. aeruginosa isolates only, which may limit its broad clinical use. Anti-Pseudomonas IgY obtained from the yolk of the immunized hens’ egg prevented adhesion of P. aeruginosa to the host by targeting the flagellin (Nilsson et al., 2007). Although a Phase 1 study has validated the feasibility of using IgY as a treatment option (Kollberg et al., 2003), the status of its Phase 2 and 3 trials remains unclear.
3.5 Modulating Bacterial Metabolism
The virulence factors of P. aeruginosa are implicated in bacterial metabolism. For example, quorum sensing has four related transcriptional pathways; siderophores, toxins, and proteases are all regulated by bacterial transcriptional systems that are triggered by different signal molecules. Therefore, targeting pathways involved in bacterial metabolism often produces various comprehensive effects that can be taken advantage of in a potential antivirulence therapy. Cysteamine, a simple aminothiol and an FDA-approved therapeutic for cystinosis, blocked glycine utilization of P. aeruginosa, subsequently impaired chemotaxis, reduced virulence factor production, and alleviated infection of P. aeruginosa in a G. mellonella model (Fraser-Pitt et al., 2021). Polyphosphate kinase (PPK) is critical for P. aeruginosa biofilm formation, quorum-sensing response, and virulence in the host (Rashid et al., 2000). Gallein is a polyhydroxylated small molecule that inhibits both PPK1 and PPK2 enzymes, which in turn suppresses P. aeruginosa biofilm formation and virulence in C. elegans (Neville et al., 2021).
4 Potentials and Challenges
Antivirulence drugs target specific virulence pathways instead of direct killing the bacteria, thus imposing less selective pressure and less likely to induce drug resistance. Since the bacteria treated with antivirulence drugs are still viable, the host commensal flora may remain intact. Although the host immune system is expected to clear the pathogen, it may fail to completely eliminate it, in which case a combination therapy involving antibiotics may still be required for preventing infection recurrence, improving therapeutic efficacy, and achieving bacterial eradication.
Certain inhibitors of quorum sensing and bacterial metabolism may inhibit the production of multiple virulence factors, yet off-target effects may occur when it comes to pathway or metabolism inhibitors, resulting in unexpected side effects. The leading virulence factors may vary in different infection stages of P. aeruginosa: when the infection of a CF patient transits from acute to chronic, virulence factors like the flagellum, T3SS and proteases are down-regulated, and virulence factors as exopolysaccharides are up-regulated (Hogardt and Heesemann, 2013; Fleitas Martínez et al., 2019a). A deep understanding of pathogenic mechanisms of the bacterial virulence factors is essential for antivirulence therapy so that the targets can be properly selected.
Antivirulence therapy can target one specific pathogen or multiple bacteria. Some virulence factors are only present in a specific strain, other conserved virulence factors such as the T3SS are more broadly distributed among different bacteria. For example, the T3SS inhibitor fluorothiazinon has proved to be effective against Chlamydia spp., P. aeruginosa, A. baumannii and Salmonella sp. in vitro and in vivo (Bondareva et al., 2022). The strain specificity may require antivirulence therapy in combination with other classes of therapeutics in the circumstances of multiple bacterial infections or one bacterium with multiple virulence factors.
5 Conclusion
Although the virulence factors of P. aeruginosa are abundant and diverse, much of the current studies on antivirulence therapy focuses on T3SS, quorum sensing and biofilm formation. While other targets include secreted siderophores, proteases and exopolysaccharide, less common are bacterial surface structures such as LPS and flagella, as well as bacterial metabolic pathways. T3SS, quorum sensing and biofilm are ubiquitous in a variety of pathogenic bacteria as relatively conserved virulence factors. Focused efforts on them may lead to effective antivirulence drugs for a variety of pathogenic bacteria. A number of virulence factors of P. aeruginosa have garnered limited attention as potential drug targets, including secretion systems other than T3SS. The exploration of more antivirulence targets for therapy obviously requires in-depth studies of their pathogenic mechanisms by which they operate in P. aeruginosa infection.
Various drug candidates have been evaluated for their antivirulence effects on P. aeruginosa infection. Most of them are small molecules derived from chemical libraries via high-throughput screening, repurposed drugs, and natural compounds, supplemented by several engineered antibodies, then alginate oligomers. Thus far, a majority of the clinical trials documented have failed to achieve desired therapeutic effects, and no drug candidate for antivirulence therapy of P. aeruginosa has been approved or licensed for clinical use. Currently, only three drug candidates are undergoing clinical trials, while many more are in preclinical stages. With the emergence of more and more antibiotic resistant strains, antivirulence therapy will likely become an important alternative, worthy of more extensive and intensive research.
Author Contributions
CL, XH, and WL contributed to initial conceptualization of the review. CL, XH, QW and DY performed initial literature reviews and manuscript drafting. CL and WL contributed to literature review and extensive manuscript editing. All authors contributed to the article and approved the submitted version.
Funding
This work was financially supported by National Natural Science Foundation of China (NSFC 82030062) and China Postdoctoral Science Foundation (2021M690666).
Conflict of Interest
The authors declare that the research was conducted in the absence of any commercial or financial relationships that could be construed as a potential conflict of interest.
Publisher’s Note
All claims expressed in this article are solely those of the authors and do not necessarily represent those of their affiliated organizations, or those of the publisher, the editors and the reviewers. Any product that may be evaluated in this article, or claim that may be made by its manufacturer, is not guaranteed or endorsed by the publisher.
Acknowledgments
We acknowledge National Natural Science Foundation of China and China Postdoctoral Science Foundation for their financial support.
References
Agnello, M., Finkel, S. E., Wong-Beringer, A. (2016). Fitness Cost of Fluoroquinolone Resistance in Clinical Isolates of Pseudomonas Aeruginosa Differs by Type III Secretion Genotype. Front. Microbiol. 7. doi: 10.3389/fmicb.2016.01591
Agnello, M., Wong-Beringer, A. (2012). Differentiation in Quinolone Resistance by Virulence Genotype in Pseudomonas Aeruginosa. PloS One 7 (8), e42973. doi: 10.1371/journal.pone.0042973
Aldrovandi, M., Banthiya, S., Meckelmann, S., Zhou, Y., Kuhn, H. (2018). Specific Oxygenation of Plasma Membrane Phospholipids by Pseudomonas Aeruginosa Lipoxygenase Induces Structural and Functional Alterations in Mammalian Cells. Biochim. Biophys. Acta 1863 (2), 152–164. doi: 10.1016/j.bbalip.2017.11.005
Al-Hasan, M. N., Wilson, J. W., Lahr, B. D., Eckel-Passow, J. E., Baddour, L. M. (2008). Incidence of Pseudomonas Aeruginosa Bacteremia: A Population-Based Study. Am. J. Med. 121 (8), 702–708. doi: 10.1016/j.amjmed.2008.03.029
Ali, S. O., Yu, X. Q., Robbie, G. J., Wu, Y., Shoemaker, K., Yu, L., et al. (2019). Phase 1 Study of MEDI3902, an Investigational Anti-Pseudomonas Aeruginosa PcrV and Psl Bispecific Human Monoclonal Antibody, in Healthy Adults. Clin. Microbiol. Infect 25 (5), 629.e621–629.e626. doi: 10.1016/j.cmi.2018.08.004
Anantharajah, A., Faure, E., Buyck, J. M., Sundin, C., Lindmark, T., Mecsas, J., et al. (2016). Inhibition of the Injectisome and Flagellar Type III Secretion Systems by INP1855 Impairs Pseudomonas Aeruginosa Pathogenicity and Inflammasome Activation. J. Infect. Dis. 214 (7), 1105–1116. doi: 10.1093/infdis/jiw295
Baer, M., Sawa, T., Flynn, P., Luehrsen, K., Martinez, D., Wiener-Kronish, J. P, et al (2009). An Engineered Human Antibody Fab Fragment Specific for Pseudomonas aeruginosa PcrV Antigen Has Potent Antibacterial Activity. Infection and Immunity 77(3), 1083–90. 10.1128/IAI.00815-08,
Balasubramanian, D., Schneper, L., Kumari, H., Mathee, K. (2012). A Dynamic and Intricate Regulatory Network Determines Pseudomonas Aeruginosa Virulence. Nucleic Acids Res. 41(1), 1–20. doi: 10.1093/nar/gks1039
Ball, G., Durand, É., Lazdunski, A., Filloux, A. (2002). A Novel Type II Secretion System in Pseudomonas Aeruginosa. Mol. Microbiol. 43 (2), 475–485. doi: 10.1046/j.1365-2958.2002.02759.x
Ball, G., Viarre, V., Garvis, S., Voulhoux, R., Filloux, A. (2012). Type II-Dependent Secretion of a Pseudomonas Aeruginosa DING Protein. Res. Microbiol. 163 (6), 457–469. doi: 10.1016/j.resmic.2012.07.007
Barken, K. B., Pamp, S. J., Yang, L., Gjermansen, M., Bertrand, J. J., Klausen, M., et al. (2010). Roles of Type IV Pili, Flagellum-Mediated Motility and Extracellular DNA in the Formation of Mature Multicellular Structures in Pseudomonas Aeruginosa Biofilms. Environ. Microbiol. 10 (9), 2331–43. doi: 10.1111/j.1462-2920.2008.01658.x
Barraud, N., Hassett, D. J., Hwang, S.-H., Rice, S. A., Kjelleberg, S., Webb, J. S. (2006). Involvement of Nitric Oxide in Biofilm Dispersal of Pseudomonas Aeruginosa. J. Bacteriol 188 (21), 7344–7353. doi: 10.1128/JB.00779-06
Basler, M., Ho, B., Mekalanos, J. (2013). Tit-For-Tat: Type VI Secretion System Counterattack During Bacterial Cell-Cell Interactions. Cell 152 (4), 884–894. doi: 10.1016/j.cell.2013.01.042
Basso, P., Ragno, M., Elsen, S., Reboud, E., Golovkine, G., Bouillot, S., et al. (2017). Pseudomonas Aeruginosa Pore-Forming Exolysin and Type IV Pili Cooperate to Induce Host Cell Lysis. MBio 8 (1), e02250–e02216. doi: 10.1128/mBio.02250-16
Baer, M., Sawa, T., Flynn, P., Luehrsen, K., Martinez, D., Wiener-Kronish, J.P., et al(2009). An Engineered Human Antibody Fab Fragment Specific for Pseudomonas aeruginosa PcrV Antigen Has Potent Antibacterial Activity. Infection and Immunity 77(3), 1083-1090. doi: 10.1128/IAI.00815-08.x
Bianchi, S. M., Prince, L. R., McPhillips, K., Allen, L., Marriott, H. M., Taylor, G. W., et al. (2008). Impairment of Apoptotic Cell Engulfment by Pyocyanin, a Toxic Metabolite of Pseudomonas Aeruginosa. Am. J. Respir. Crit. Care Med. 177 (1), 35–43. doi: 10.1164/rccm.200612-1804OC
Bleves, S (2016). Game of Trans-Kingdom Effectors. Trends Microbiol. 24(10), 773–774. doi: 10.1016/j.tim.2016.08.002
Bleves, S., Viarre, V., Salacha, R., Michel, G. P. F., Filloux, A., Voulhoux, R. (2010). Protein Secretion Systems in Pseudomonas Aeruginosa: A Wealth of Pathogenic Weapons. Int. J. Med. Microbiol. 300 (8), 534–543. doi: 10.1016/j.ijmm.2010.08.005
Bondareva, N. E., Soloveva, A. V., Sheremet, A. B., Koroleva, E. A., Kapotina, L. N., Morgunova, E. Y., et al. (2022). Preventative Treatment With Fluorothiazinon Suppressed Acinetobacter Baumannii-Associated Septicemia in Mice. J. Antibiotics 75 (3), 155–163. doi: 10.1038/s41429-022-00504-y
Bonneau, A., Roche, B., Schalk, I. J. (2020). Iron Acquisition in Pseudomonas Aeruginosa by the Siderophore Pyoverdine: An Intricate Interacting Network Including Periplasmic and Membrane Proteins. Sci. Rep. 10 (1), 1–11. doi: 10.1038/s41598-019-56913-x
Bouillot, S., Pont, S., Gallet, B., Moriscot, C., Huber, P. (2020). Inflammasome Activation by Pseudomonas Aeruginosa 'S ExlA Pore-Forming Toxin is Detrimental for the Host. Cell. Microbiol. 22 (11), e13251. doi: 10.1111/cmi.13251
Boyd, A., Chakrabarty, A. M. (1995). Pseudomonas Aeruginosa Biofilms: Role of the Alginate Exopolysaccharide. J. Ind. Microbiol. 15 (3), 162–168. doi: 10.1007/BF01569821
Bradshaw, J. L., Caballero, A. R., Bierdeman, M. A., Adams, K. V., Pipkins, H. R., Tang, A., et al. (2018). Pseudomonas Aeruginosa Protease IV Exacerbates Pneumococcal Pneumonia and Systemic Disease. Msphere 3 (3), e00212–e00218. doi: 10.1128/mSphere.00212-18
Burrows, L. L. (2012a). Pseudomonas Aeruginosa Twitching Motility: Type IV Pili in Action. Annu. Rev. Microbiol. 66 (1), 493–520. doi: 10.1146/annurev-micro-092611-150055
Burrows, L. L. (2012b). Pseudomonas Aeruginosa Twitching Motility: Type IV Pili in Action. Annu. Rev. Microbiol. 66, 493–520. doi: 10.1146/annurev-micro-092611-150055
Campodónico, V. L., Llosa, N. J., Grout, M., Döring, G., Maira-Litrán, T., Pier, G. B. (2010). Evaluation of Flagella and Flagellin of Pseudomonas Aeruginosa as Vaccines. Infect Immun. 78 (2), 746–755. doi: 10.1128/IAI.00806-09
Carmeli, Y., Troillet, N., Eliopoulos, G. M., Samore, M. H. (1999). Emergence of Antibiotic-Resistant Pseudomonas Aeruginosa: Comparison of Risks Associated With Different Antipseudomonal Agents. Antimicro Agents chemother 43 (6), 1379–1382. doi: 10.1128/AAC.43.6.1379
Casilag, F., Lorenz, A., Krueger, J., Klawonn, F., Weiss, S., Häussler, S. (2015). The LasB Elastase of Pseudomonas Aeruginosa Acts in Concert With Alkaline Protease AprA to Prevent Flagellin-Mediated Immune Recognition. Infect Immun. 84 (1), 162. doi: 10.1128/IAI.00939-15
Cegelski, L., Marshall, G. R., Eldridge, G. R., Hultgren, S. J. (2008). The Biology and Future Prospects of Antivirulence Therapies. Nat. Rev. Microbiol. 6 (1), 17–27. doi: 10.1038/nrmicro1818
Cheng, Z. (2016). A Pseudomonas Aeruginosa-Secreted Protease Modulates Host Intrinsic Immune Responses, But How? BioEssays 38 (11), 1084–1092. doi: 10.1002/bies.201600101
Chen, L., Zou, Y., She, P., Wu, Y. (2015). Composition, Function, and Regulation of T6SS in Pseudomonas Aeruginosa. Microb Res. 172, 19–25. doi: 10.1016/j.micres.2015.01.004
Choy, M. H., Stapleton, F., Willcox, M. D. P., Zhu, H. (2008). Comparison of Virulence Factors in Pseudomonas Aeruginosa Strains Isolated From Contact Lens- and non-Contact Lens-Related Keratitis. J. Med. Microbiol. 57 (12), 1539–1546. doi: 10.1099/jmm.0.2008/003723-0
Coburn, B., Sekirov, I., Finlay, B. B. (2007). Type III Secretion Systems and Disease. Clin. Microbiol. Rev. 20 (4), 535–549. doi: 10.1128/CMR.00013-07
Colvin, K. M., Irie, Y., Tart, C. S., Urbano, R., Whitney, J. C., Ryder, C., et al. (2012). The Pel and Psl Polysaccharides Provide Pseudomonas Aeruginosa Structural Redundancy Within the Biofilm Matrix. Environ. Microbiol. 14 (8), 1913–1928. doi: 10.1111/j.1462-2920.2011.02657.x
Cornelis, G. R. (2006). The Type III Secretion Injectisome. Nat. Rev. Microbiol. 4 (11), 811–825. doi: 10.1038/nrmicro1526
Cornelis, G. R. (2010a). The Type III Secretion Injectisome, a Complex Nanomachine for Intracellular ‘Toxin’ Delivery. Biol. Chem. 391 (7), 745–751. doi: 10.1515/BC.2010.079
Cornelis, P. (2010b). Iron Uptake and Metabolism in Pseudomonads. Appl. Microbiol. Biotechnol. 86 (6), 1637–1645. doi: 10.1007/s00253-010-2550-2
Craig, L., Forest, K. T., Maier, B. (2019). Type IV Pili: Dynamics, Biophysics and Functional Consequences. Nat. Rev. Microbiol. 17 (7), 429–440. doi: 10.1038/s41579-019-0195-4
Cryz, S., Fürer, E., Sadoff, J., Germanier, R. (1987). “"A Polyvalent Pseudomonas Aeruginosa O-Polysaccharide-Toxin A Conjugate Vaccine,",” in Basic Research and Clinical Aspects of Pseudomonas Aeruginosa eds.Döring, G., Holder, I. A., Botzenhart, K. (Tübingen:Karger Publishers) 39, 249–255. doi: 10.1159/000414350
Cryz, S., Jr., Lang, A., Rüdeberg, A., Wedgwood, J., Que, J., Fürer, E., et al. (1997). Immunization of Cystic Fibrosis Patients With a Pseudomonas Aeruginosa O-Polysaccharide-Toxin A Conjugate Vaccine. Behring Inst Mitt. 98), 345–349.
Cunrath, O., Graulier, G., Lopez, A. C., Perard, J., Fechter, P. (2020). The Pathogen Pseudomonas Aeruginosa Optimizes the Production of the Siderophore Pyochelin Upon Environmental Challenges. Metallomics 12, 2108–2120. doi: 10.1039/d0mt00029a
Cutruzzolà, F., Frankenberg-Dinkel, N., O'Toole, G. A. (2016). Origin and Impact of Nitric Oxide in Pseudomonas Aeruginosa Biofilms. J. Bacteriol 198 (1), 55–65. doi: 10.1128/JB.00371-15
Daddaoua, A., Molina-Santiago, C., la Torre, J. D., Krell, T., Ramos, J. L(2014). GtrSand GltR Form a Two-Component System: The Central Role of 2-Ketogluconate in the Expression of Exotoxin A and Glucose Catabolic Enzymes in Pseudomonas Aeruginosa. Nucleic Acids Res 42(12), 7654-7663. doi: 10.1093/nar/gku496.x
D'Angelo, F., Baldelli, V., Halliday, N., Pantalone, P., Polticelli, F., Fiscarelli, E., et al. (2018). Identification of FDA-Approved Drugs as Antivirulence Agents Targeting the Pqs Quorum-Sensing System of Pseudomonas Aeruginosa. Antimicrob. Agents Chemother. 62 (11), e01296–01318. doi: 10.1128/AAC.01296-18
Dao, N., Joshi-Datar, A., Lepine, F., Bauerle, E., Olakanmi, O., Beer, K., et al. (2011). Active Starvation Responses Mediate Antibiotic Tolerance in Biofilms and Nutrient-Limited Bacteria. Science 334 (6058), 982–986. doi: 10.1126/science.1211037
Dar, H. H., Epperly, M. W., Tyurin, V. A., Amoscato, A. A., Anthonymuthu, T. S., Souryavong, A. B., et al. (2022). P. Aeruginosa Augments Irradiation Injury via 15-Lipoxygenase Catalyzed Generation of 15-HpETE-PE and Induction of Theft-Ferroptosis. JCI Insight 7 (4), e156013. doi: 10.1172/jci.insight.156013
Dasgupta, N., Arora, S. K., Ramphal, R. (2004). “"The Flagellar System of Pseudomonas Aeruginosa,",” in Pseudomonas: Volume 1 Genomics, Life Style and Molecular Architecture. Ed. Ramos, J.-L. (Boston, MA: Springer US), 675–698. doi: 10.1007/978-1-4419-9086-0_22
Dickey, S. W., Cheung, G. Y. C., Otto, M. (2017). Different Drugs for Bad Bugs: Antivirulence Strategies in the Age of Antibiotic Resistance. Nat. Rev. Drug Discovery 16 (7), 457–471. doi: 10.1038/nrd.2017.23
Diggle, S. P., Whiteley, M. (2020). Microbe Profile: Pseudomonas Aeruginosa: Opportunistic Pathogen and Lab Rat. Microbiol. (Reading England) 166 (1), 30–33. doi: 10.1099/mic.0.000860
DiGiandomenico, A., Keller, A. E., Gao, C., Rainey, G. J., Warrener, P., Camara, M. M., et al. (2014). A Multifunctional Bispecific Antibody Protects Against Pseudomonas Aeruginosa. Sci. Trans. Med. 6 (262), 262ra155–262ra155. doi: 10.1126/scitranslmed.3009655
Döring, G. (2010). Prevention of Pseudomonas Aeruginosa Infection in Cystic Fibrosis Patients. Int. J. Med. Microbiol. 300 (8), 573–577. doi: 10.1016/j.ijmm.2010.08.010
Duong, F., Bonnet, E., Géli, V., Lazdunski, A., Filloux, A. (2001). The AprX Protein of Pseudomonas Aeruginosa: A New Substrate for the Apr Type I Secretion System. Gene 262 (1-2), 147–153. doi: 10.1016/s0378-1119(00)00541-2
Ellis, T. N., Kuehn, M. J. (2010). Virulence and Immunomodulatory Roles of Bacterial Outer Membrane Vesicles. Microbiol. Mol. Biol. Rev. 74 (1), 81–94. doi: 10.1128/MMBR.00031-09
Elsen, S., Huber, P., Bouillot, S., Couté, Y., Fournier, P., Dubois, Y., et al. (2014). A Type III Secretion Negative Clinical Strain of Pseudomonas Aeruginosa Employs a Two-Partner Secreted Exolysin to Induce Hemorrhagic Pneumonia. Cell Host Microbe 15 (2), 164–176. doi: 10.1016/j.chom.2014.01.003
Engel, L. S., Hill, J. M., Caballero, A. R., Green, L. C., O'Callaghan, R. J. (1998). Protease IV, a Unique Extracellular Protease and Virulence Factor From Pseudomonas Aeruginosa. J. Biol. Chem. 273 (27), 16792–16797. doi: 10.1074/jbc.273.27.16792
Faezi, S., Bahrmand, A. R., Mahdavi, M., Siadat, S. D., Sardari, S., Nikokar, I., et al. (2017). Preparation of Pseudomonas Aeruginosa Alginate-Flagellin Immunoconjugate. Biologicals 47, 11–17. doi: 10.1016/j.biologicals.2017.04.001
Feng, C., Huang, Y., He, W., Cheng, X., Liu, H., Huang, Y., et al. (2019). Tanshinones: First-In-Class Inhibitors of the Biogenesis of the Type 3 Secretion System Needle of Pseudomonas Aeruginosa for Antibiotic Therapy. ACS Cent. Sci. 5 (7), 1278–1288. doi: 10.1021/acscentsci.9b00452
Feuillet, V., Medjane, S., Mondor, I., Demaria, O., Pagni, P. P., Galán, J. E., et al. (2006). Involvement of Toll-Like Receptor 5 in the Recognition of Flagellated Bacteria. Proc. Natl. Acad. Sci. United States America 103 (33), 12487–12492. doi: 10.1073/pnas.0605200103
Fleitas Martínez, O., Cardoso, M. H., Ribeiro, S. M., Franco, O. L. (2019a). Recent Advances in Anti-Virulence Therapeutic Strategies With a Focus on Dismantling Bacterial Membrane Microdomains, Toxin Neutralization, Quorum-Sensing Interference and Biofilm Inhibition. Front. Cell. Infect Microbiol. 9. doi: 10.3389/fcimb.2019.00074
Fleitas Martínez, O., Cardoso, M. H., Ribeiro, S. M., Franco, O. L. (2019b). Recent Advances in Anti-Virulence Therapeutic Strategies With a Focus on Dismantling Bacterial Membrane Microdomains, Toxin Neutralization, Quorum-Sensing Interference and Biofilm Inhibition. Front. Cell. infect Microbiol. 9. doi: 10.3389/fcimb.2019.00074
Franklin, M., Nivens, D., Weadge, J., Howell, P. (2011). Biosynthesis of the Pseudomonas Aeruginosa Extracellular Polysaccharides, Alginate, Pel, and Psl. Front. Microbiol. 2. doi: 10.3389/fmicb.2011.00167
Fraser-Pitt, D. J., Dolan, S. K., Toledo-Aparicio, D., Hunt, J. G., Smith, D. W., Lacy-Roberts, N., et al. (2021). Cysteamine Inhibits Glycine Utilisation and Disrupts Virulence in Pseudomonas Aeruginosa. Front. Cell Infect. Microbiol. 11. doi: 10.3389/fcimb.2021.718213
Galdino, A. C. M., Viganor, L., de Castro, A. A., da Cunha, E. F. F., Mello, T. P., Mattos, L. M., et al. (2019). Disarming Pseudomonas Aeruginosa Virulence by the Inhibitory Action of 1,10-Phenanthroline-5,6-Dione-Based Compounds: Elastase B (LasB) as a Chemotherapeutic Target. Front. Microbiol. 10, 1701. doi: 10.3389/fmicb.2019.01701
Gallique, M., Bouteiller, M., Merieau, A. (2017). The Type VI Secretion System: A Dynamic System for Bacterial Communication? Front. Microbiol. 8, 1454. doi: 10.3389/fmicb.2017.01454
Gellatly, S. L., Hancock, R. E. W. (2013). Pseudomonas Aeruginosa: New Insights Into Pathogenesis and Host Defenses. Pathog. Dis. 67 (3), 159–173. doi: 10.1111/2049-632X.12033
Goldberg, J. B., Pier, G. B. (1996). Pseudomonas Aeruginosa Lipopolysaccharides and Pathogenesis. Trends Microbiol. 4 (12), 490–494. doi: 10.1016/S0966-842X(97)82911-3
Gómez, M. I., Prince, A. (2007). Opportunistic Infections in Lung Disease: Pseudomonas Infections in Cystic Fibrosis. Curr. Opin. Pharmacol. 7 (3), 244–251. doi: 10.1016/j.coph.2006.12.005
Hahn, H. P. (1997). The Type-4 Pilus is the Major Virulence-Associated Adhesin of Pseudomonas Aeruginosa – a Review. Gene 192 (1), 99–108. doi: 10.1016/S0378-1119(97)00116-9
Hall, S., McDermott, C., Anoopkumar-Dukie, S., McFarland, A. J., Forbes, A., Perkins, A. V., et al. (2016). Cellular Effects of Pyocyanin, a Secreted Virulence Factor of Pseudomonas Aeruginosa. Toxins 8 (8), 236. doi: 10.3390/toxins8080236
Hanessian, S., Regan, W., Watson, D., Haskell, T. H. (1971). Isolation and Characterization of Antigenic Components of a New Heptavalent Pseudomonas Vaccine. Nat. New Biol. 229 (7), 209–210. doi: 10.1038/newbio229209a0
Hassan, R., El-Naggar, W., Abd El-Aziz, A. M., Shaaban, M., Kenawy, H. I., Ali, Y. M. (2018). Immunization With Outer Membrane Proteins (OprF and OprI) and Flagellin B Protects Mice From Pulmonary Infection With Mucoid and Nonmucoid Pseudomonas Aeruginosa. J. microbiol Immunol. infect 51 (3), 312–320. doi: 10.1016/j.jmii.2016.08.014
Hatano, K., Pier, G. B. (1998). Complex Serology and Immune Response of Mice to Variant High-Molecular-Weight O Polysaccharides Isolated From Pseudomonas Aeruginosa Serogroup O2 Strains. Infect Immun. 66 (8), 3719–3726. doi: 10.1128/IAI.66.8.3719-3726.1998
Hauser, A. R. (2009). The Type III Secretion System of Pseudomonas Aeruginosa: Infection by Injection. Nat. Rev. Microbiol. 7 (9), 654–665. doi: 10.1038/nrmicro2199
Hemmati, F., Salehi, R., Ghotaslou, R., Kafil, H. S., Hasani, A., Gholizadeh, P., et al. (2020). Quorum Quenching: A Potential Target for Antipseudomonal Therapy. Infect Drug Resistance 13, 2989. doi: 10.2147/IDR.S263196
Henderson, I. R., Navarrogarcia, F., Desvaux, M., Fernandez, R. C., Ala'Aldeen, D. (2004). Type V Protein Secretion Pathway: The Autotransporter Story. Microbiol. Mol. Biol. Rev. MMBR 68 (4), 692–744. doi: 10.1128/MMBR.68.4.692-744.2004
Hengzhuang, W., Song, Z., Ciofu, O., Onsøyen, E., Rye, P. D., Høiby, N, et al. (2016). OligoG CF-5/20 Disruption of Mucoid Pseudomonas aeruginosa Biofilm in a Murine Lung Infection Model. Antimicrobial Agents and Chem. 60 (5), 2620–26. doi: 10.1128/AAC.01721-15
Hentzer, M., Wu, H., Andersen, J. B., Riedel, K., Rasmussen, T. B., Bagge, N., et al. (2003). Attenuation of Pseudomonas Aeruginosa Virulence by Quorum Sensing Inhibitors. EMBO J. 22 (15), 3803–3815. doi: 10.1093/emboj/cdg366
Hirayama, T., Kato, I., Matsuda, F., Noda, M. (2013). Crystallization and Some Properties of Leukocidin From Pseudomonas Aeruginosa. Microbiol. Immunol. 27 (7), 575–588. doi: 10.1111/j.1348-0421.1983.tb00619.x
Hogardt, M., Heesemann, J. (2013). “"Microevolution of Pseudomonas Aeruginosa to a Chronic Pathogen of the Cystic Fibrosis Lung,",” in Between Pathogenicity and Commensalism. Eds. Dobrindt, U., Hacker, J. H., Svanborg, C. (Berlin, Heidelberg: Springer Berlin Heidelberg), 91–118. doi: 10.1007/82_2011_199
Holder, I., Wheeler, R., Montie, T. (1982). Flagellar Preparations From Pseudomonas Aeruginosa: Animal Protection Studies. Infect Immun. 35 (1), 276–280. doi: 10.1128/iai.35.1.276-280.1982
Homma, J. Y., Matsuura, M., Shibata, M., Kazuyama, Y., Kato, I. (1984). Production of Leukocidin by Clinical Isolates of Pseudomonas Aeruginosa and Antileukocidin Antibody From Sera of Patients With Diffuse Panbronchiolitis. J. Clin. Microbiol. 20 (5), 855–859. doi: 10.1128/jcm.20.5.855-859.1984
Hood, R. D., Singh, P., Hsu, F., Güvener, T., Carl, M. A., Trinidad, R. R., et al. (2010). A Type VI Secretion System of Pseudomonas Aeruginosa Targets a Toxin to Bacteria. Cell Host Microbe 7 (1), 25–37. doi: 10.1016/j.chom.2009.12.007
Horna, G., Amaro, C., Palacios, A., Guerra, H., Ruiz, J. (2019). High Frequency of the Exou+/Exos+ Genotype Associated With Multidrug-Resistant “High-Risk Clones” of Pseudomonas Aeruginosa Clinical Isolates From Peruvian Hospitals. Sci. Rep. 9 (1), 10874. doi: 10.1038/s41598-019-47303-4
Horna, G., Ruiz, J. (2021a). Type 3 Secretion System as an Anti-Pseudomonal Target. Microbial Pathogen 155, 104907. doi: 10.1016/j.micpath.2021.104907
Horna, G., Ruiz, J. (2021b). Type 3 Secretion System of Pseudomonas Aeruginosa. Microb Res. 246, 126719. doi: 10.1016/j.micres.2021.126719
Horn, M. P., Zuercher, A. W., Imboden, M. A., Rudolf, M. P., Lazar, H., Wu, H., et al. (2010). Preclinical In Vitro and In Vivo Characterization of the Fully Human Monoclonal IgM Antibody KBPA101 Specific for Pseudomonas Aeruginosa Serotype IATS-O11. Antimicro Agents Chemother 54 (6), 2338–2344. doi: 10.1128/AAC.01142-09
Huszczynski, S. M., Lam, J. S., Khursigara, C. M. (2020). The Role of Pseudomonas Aeruginosa Lipopolysaccharide in Bacterial Pathogenesis and Physiology. Pathogens 9 (1), 6. doi: 10.3390/pathogens9010006
Hwang, H.-J., Choi, H., Hong, S., Moon, H. R., Lee, J.-H. (2021). Antipathogenic Compounds That Are Effective at Very Low Concentrations and Have Both Antibiofilm and Antivirulence Effects Against Pseudomonas Aeruginosa. Microbiol. Spectr. 9 (2), e0024921–e0024921. doi: 10.1128/Spectrum.00249-21
Jacob-Dubuisson, F., Locht, C., Antoine, R. (2001). Two-Partner Secretion in Gram-Negative Bacteria: A Thrifty, Specific Pathway for Large Virulence Proteins. Mol. Microbiol. 40 (2), 306–13. doi: 10.1046/j.1365-2958.2001.02278.x
Jain, R., Beckett, V. V., Konstan, M. W., Accurso, F. J., Burns, J. L., Mayer-Hamblett, N., et al. (2018). KB001-A, a Novel Anti-Inflammatory, Found to be Safe and Well-Tolerated in Cystic Fibrosis Patients Infected With Pseudomonas Aeruginosa. J. Cystic Fibrosis 17 (4), 484–491. doi: 10.1016/j.jcf.2017.12.006
Jiang, F., Waterfield, N., Yang, J., Yang, G., Jin, Q. (2014). A Pseudomonas Aeruginosa Type VI Secretion Phospholipase D Effector Targets Both Prokaryotic and Eukaryotic Cells. Cell Host Microbe 15 (5), 600–610. doi: 10.1016/j.chom.2014.04.010
Jyot, J., Balloy, V., Jouvion, G., Verma, A., Touqui, L., Huerre, M., et al. (2011). Type II Secretion System of Pseudomonas Aeruginosa: In Vivo Evidence of a Significant Role in Death Due to Lung Infection. J. Infect. Dis. 203 (10), 1369–1377. doi: 10.1093/infdis/jir045
Kalms, J., Banthiya, S., Yoga, E. G., Hamberg, M., Holzhutter, H. G., Kuhn, H., et al. (2017). The Crystal Structure of Pseudomonas Aeruginosa Lipoxygenase Ala420Gly Mutant Explains the Improved Oxygen Affinity and the Altered Reaction Specificity. BBA - Mol. Cell Biol. Lipids 1862 (5), 463–473. doi: 10.1016/j.bbalip.2017.01.003
Kany, A. M., Sikandar, A., Haupenthal, J., Yahiaoui, S., Maurer, C. K., Proschak, E., et al. (2018). Binding Mode Characterization and Early in Vivo Evaluation of Fragment-Like Thiols as Inhibitors of the Virulence Factor LasB From Pseudomonas Aeruginosa. ACS Infect. Dis. 4 (6), 988–997. doi: 10.1021/acsinfecdis.8b00010
Kaya, C., Walter, I., Yahiaoui, S., Sikandar, A., Alhayek, A., Konstantinović, J., et al. (2022). Substrate-Inspired Fragment Merging and Growing Affords Efficacious LasB Inhibitors. Angew Chem. Int. Ed Engl. 61 (5), e202112295. doi: 10.1002/anie.202112295
Kessler, E., Safrin, M., Abrams, W. R., Rosenbloom, J., Ohman, D. E. (1997). Inhibitors and Specificity of Pseudomonas Aeruginosa LasA. J. Biol. Chem. 272 (15), 9884–9889. doi: 10.1074/jbc.272.15.9884
Kessler, E., Safrin, M., Gustin, J. K., Ohman, D. E. (1998). Elastase and the LasA Protease of Pseudomonas Aeruginosa are Secreted With Their Propeptides. J. Biol. Chem. 273 (46), 30225–30231. doi: 10.1074/jbc.273.46.30225
Kim, S.-K., Ngo, H. X., Dennis, E. K., Thamban Chandrika, N., DeShong, P., Garneau-Tsodikova, S., et al. (2021). Inhibition of Pseudomonas Aeruginosa Alginate Synthesis by Ebselen Oxide and Its Analogues. ACS Infect. Dis. 7 (6), 1713–1726. doi: 10.1021/acsinfecdis.1c00045
Kirienko, D. R., Kang, D., Kirienko, N. V. (2018). Novel Pyoverdine Inhibitors Mitigate Pseudomonas Aeruginosa Pathogenesis. Front. Microbiol. 9. doi: 10.3389/fmicb.2018.03317
Kollberg, H., Carlander, D., Olesen, H., Wejåker, P.-E., Johannesson, M., Larsson, A. (2003). Oral Administration of Specific Yolk Antibodies (IgY) may Prevent Pseudomonas Aeruginosa Infections in Patients With Cystic Fibrosis: A Phase I Feasibility Study. Pediatr. Pulmonol 35 (6), 433–440. doi: 10.1002/ppul.10290
König, B., Vasil, M. L., König, W. (1997). Role of Haemolytic and Non-Haemolytic Phospholipase C From Pseudomonas Aeruginosa in Interleukin-8 Release From Human Monocytes. J. Med. Microbiol. 46 (6), 471–478. doi: 10.1099/00222615-46-6-471
Lamont, I. L., Beare, P. A., Ochsner, U. (2002). Siderophore-Mediated Signaling Regulates Virulence Factor Production in Pseudomonas Aeruginosa. Proc. Natl. Acad. Sci. United States America 99 (10), 7072–7077. doi: 10.1073/pnas.092016999
Lau, G. W., Hassett, D. J., Ran, H., Kong, F. (2004). The Role of Pyocyanin in Pseudomonas Aeruginosa Infection. Trends Mol. Med. 10 (12), 599–606. doi: 10.1016/j.molmed.2004.10.002
Lazar, H., Horn, M. P., Zuercher, A. W., Imboden, M. A., Durrer, P., Seiberling, M., et al. (2009). Pharmacokinetics and Safety Profile of the Human Anti-Pseudomonas Aeruginosa Serotype O11 Immunoglobulin M Monoclonal Antibody KBPA-101 in Healthy Volunteers. Antimicro Agents Chemother 53 (8), 3442–3446. doi: 10.1128/AAC.01699-08
Lewis, K. (2001). Riddle of Biofilm Resistance. Antimicro Agents chemother 45 (4), 999–1007. doi: 10.1128/AAC.45.4.999-1007.2001
Li, S., Chen, S., Fan, J., Cao, Z., Ouyang, W., Tong, N., et al. (2018). Anti-Biofilm Effect of Novel Thiazole Acid Analogs Against Pseudomonas Aeruginosa Through IQS Pathways. Eur. J. Medic Chem. 145, 64–73. doi: 10.1016/j.ejmech.2017.12.076
Loos, A., Weich, N., Woo, J., Lalonde, G., Yee, L., Dummer, W., et al. (2019). “"674. Pre-Clinical and Phase I Safety Data for Anti-Pseudomonas Aeruginosa Human Monoclonal Antibody AR-105",” in Open Forum Infectious Diseases. doi: 10.1093/ofid/ofz360.742
Lu, J., Zhang, C., Hui, Y. L., Show, P. L., Lu, Z. (2020). Overproduction of Lipoxygenase From Pseudomonas Aeruginosa in Escherichia Coli by Auto-Induction Expression and its Application in Triphenylmethane Dyes Degradation. J. Biosci Bioeng 129 (3), 327–332. doi: 10.1016/j.jbiosc.2019.09.006
Maeda, T., García-Contreras, R., Pu, M., Sheng, L., Garcia, L. R., Tomás, M., et al. (2012). Quorum Quenching Quandary: Resistance to Antivirulence Compounds. ISME J. 6 (3), 493–501. doi: 10.1038/ismej.2011.122
Malloy, J. L., Veldhuizen, R. A., Thibodeaux, B. A., O'Callaghan, R. J., Wright, J. R. (2005). Pseudomonas Aeruginosa Protease IV Degrades Surfactant Proteins and Inhibits Surfactant Host Defense and Biophysical Functions. Am. J. Physiol. Lung Cell Mol. Physiol. 288 (2), L409. doi: 10.1152/ajplung.00322.2004
Ma, L., Lu, H., Sprinkle, A., Parsek, M. R., Wozniak, D. J. (2007). Pseudomonas Aeruginosa Psl is a Galactose- and Mannose-Rich Exopolysaccharide. J. bacteriol 189 (22), 8353–8356. doi: 10.1128/JB.00620-07
Mann, E. E., Wozniak, D. J. (2012). Pseudomonas Biofilm Matrix Composition and Niche Biology. FEMS Microbiol. Rev. 36 (4), 893–916. doi: 10.1111/j.1574-6976.2011.00322.x
Mariencheck, W. I., Alcorn, J. F., Palmer, S. M., Wright, J. R. (2003). Pseudomonas Aeruginosa Elastase Degrades Surfactant Proteins A and D. Am. J. Respir. Cell Mol. Biol. 28 (4), 528–537. doi: 10.1165/rcmb.2002-0141OC
Matsumoto, T., Tateda, K., Furuya, N., Miyazaki, S., Ohno, A., Ishii, Y., et al. (1998). Efficacies of Alkaline Protease, Elastase and Exotoxin A Toxoid Vaccines Against Gut-Derived Pseudomonas Aeruginosa Sepsis in Mice. J. Med. Microbiol. 47 (4), 303–308. doi: 10.1099/00222615-47-4-303
Maura, D., Ballok, A. E., Rahme, L. G. (2016). Considerations and Caveats in Anti-Virulence Drug Development. Curr. Opin. Microbiol. 33, 41–46. doi: 10.1016/j.mib.2016.06.001
Ma, Y. U., Wang, C. U., Li, Y. U., Li, J., Niu, L. A. (2020). Considerations and Caveats in Combating ESKAPE Pathogens Against Nosocomial Infections. Advanced Sci. 7 (1), 1901872. doi: 10.1002/advs.201901872
May, T. B., Shinabarger, D., Maharaj, R., Kato, J., Chu, L., DeVault, J. D., et al. (1991). Alginate Synthesis by Pseudomonas Aeruginosa: A Key Pathogenic Factor in Chronic Pulmonary Infections of Cystic Fibrosis Patients. Clin. Microbiol. Rev. 4 (2), 191–206. doi: 10.1128/CMR.4.2.191
Michalska, M., Wolf, P. (2015). Pseudomonas Exotoxin A: Optimized by Evolution for Effective Killing. Front. Microbiol. 6. doi: 10.3389/fmicb.2015.00963
Miller, S. I., Ernst, R. K., Bader, M. W. (2005). LPS, TLR4 and Infectious Disease Diversity. Nat. Rev. Microbiol. 3 (1), 36–46. doi: 10.1038/nrmicro1068
Monturiol-Gross, L., Villalta-Romero, F., Flores-Díaz, M., Alape-Girón, A. (2021). Bacterial Phospholipases C With Dual Activity: Phosphatidylcholinesterase and Sphingomyelinase. FEBS Open Bio 11 (12), 3262–3275. doi: 10.1002/2211-5463.13320
Morello, E., Pérez-Berezo, T., Boisseau, C., Baranek, T., Guillon, A., Bréa, D., et al. (2019a). Pseudomonas Aeruginosa Lipoxygenase LoxA Contributes to Lung Infection by Altering the Host Immune Lipid Signaling. Front. Microbiol. 10. doi: 10.3389/fmicb.2019.01826
Morello, E., Pérez-Berezo, T., Boisseau, C., Baranek, T., Si-Tahar, M. (2019b). Pseudomonas Aeruginosa Lipoxygenase LoxA Contributes to Lung Infection by Altering the Host Immune Lipid Signaling. Front. Microbiol. 10. doi: 10.3389/fmicb.2019.01826
Mougous, J. D., Cuff, M.E., Raunser, S., Shen, A., Zhou, M., Gifford, C.A, et al. (2006). A Virulence Locus of Pseudomonas Aeruginosa Encodes a Protein Secretion Apparatus. Science (New York, N.Y.) 312 (5779), 1526–1530. doi: 10.1126/science.1128393
Mühlen, S., Dersch, P. (2016). “"Anti-Virulence Strategies to Target Bacterial Infections,",” in How to Overcome the Antibiotic Crisis : Facts, Challenges, Technologies and Future Perspectives. Eds. Stadler, M., Dersch, P. (Cham: Springer International Publishing), 147–183. doi: 10.1007/82_2015_490
Neville, N., Roberge, N., Ji, X., Stephen, P., Lu, J. L., Jia, Z. (2021). A Dual-Specificity Inhibitor Targets Polyphosphate Kinase 1 and 2 Enzymes To Attenuate Virulence of Pseudomonas Aeruginosa. mBio 12 (3), e0059221. doi: 10.1128/mBio.00592-21
Newman, J. W., Floyd, R. V., Fothergill, J. L. (2017). The Contribution of Pseudomonas Aeruginosa Virulence Factors and Host Factors in the Establishment of Urinary Tract Infections. FEMS Microbiol. Lett. 364 (15), 1–11. doi: 10.1093/femsle/fnx124
Nilsson, E., Amini, A., Wretlind, B., Larsson, A. (2007). Pseudomonas Aeruginosa Infections are Prevented in Cystic Fibrosis Patients by Avian Antibodies Binding Pseudomonas Aeruginosa Flagellin. J. Chromatogr. B 856 (1), 75–80. doi: 10.1016/j.jchromb.2007.05.029
Nivens, D. E., Ohman, D. E., Williams, J., Franklin, M. J. (2001). Role of Alginate and its O Acetylation in Formation of Pseudomonas Aeruginosa Microcolonies and Biofilms. J. bacteriol 183 (3), 1047–1057. doi: 10.1128/JB.183.3.1047-1057.2001
Notti, R. Q., Stebbins, C. E. (2016). The Structure and Function of Type III Secretion Systems. Microbiol. Spectr. 4 (1), 4.1. 09. doi: 10.1128/microbiolspec.VMBF-0004-2015
O'Callaghan, R., Caballero, A., Tang, A., Bierdeman, M. (2019). Pseudomonas Aeruginosa Keratitis: Protease IV and PASP as Corneal Virulence Mediators. Microorganisms 7 (9), 281. doi: 10.3390/microorganisms7090281
O'Donnell, J. N., Bidell, M. R., Lodise, T. P. (2020). Approach to the Treatment of Patients With Serious Multidrug-Resistant Pseudomonas Aeruginosa Infections. Pharmacother: J. Hum. Pharmacol. Drug Ther. 40 (9), 952–969. doi: 10.1002/phar.2449
O’Loughlin, C. T., Miller, L. C., Siryaporn, A., Drescher, K., Semmelhack, M. F., Bassler, B. L. (2013). A Quorum-Sensing Inhibitor Blocks Pseudomonas Aeruginosa Virulence and Biofilm Formation. Proc. Natl. Acad. Sci. 110 (44), 17981–17986. doi: 10.1073/pnas.1316981110
Overhage, J., Bains, M., Brazas, M., Hancock, R. (2008). Swarming of Pseudomonas Aeruginosa Is a Complex Adaptation Leading to Increased Production of Virulence Factors and Antibiotic Resistance. J. Bacteriol 190 (8), 2671–2679. doi: 10.1128/JB.01659-07
Ozer, E., Yaniv, K., Chetrit, E., Boyarski, A., Meijler, M. M., Berkovich, R., et al. (2021). An Inside Look at a Biofilm: Pseudomonas Aeruginosa Flagella Biotracking. Sci. Adv. 7 (24), eabg8581. doi: 10.1126/sciadv.abg8581
Pan, S. Y., Shih, Y. L., Huang, H. H., Li, L. H., Yang, T. C. (2021). The Involvement of PacIRA System of Stenotrophomonas Maltophilia in the Uptake of Pseudomonas Aeruginosa Pyochelin and Intraspecies Competition for Iron Acquisition. J. microbiol immunol infect 55 (2), 273–281. doi: 10.1016/j.jmii.2021.03.001
Basso, P, Ragno, M., Elsen, S., Reboud, E., Golovkine, G., Bouillot, S, et al. (2017). Pseudomonas Aeruginosa Pore-Forming Exolysin and Type IV Pili Cooperate To Induce Host Cell Lysis. MBio 8 (1), e02250–e02216. doi: 10.1128/mBio.02250-16
Pérez-Pérez, M., Jorge, P., Pérez Rodríguez, G., Pereira, M. O., Lourenço, A. (2017). Quorum Sensing Inhibition in Pseudomonas Aeruginosa Biofilms: New Insights Through Network Mining. Biofouling 33 (2), 128–142. doi: 10.1080/08927014.2016.1272104
Persat, A., Inclan, Y. F., Engel, J. N., Stone, H. A., Gitai, Z. (2015). Type IV Pili Mechanochemically Regulate Virulence Factors in Pseudomonas Aeruginosa. Proc. Natl. Acad. Sci. 112 (24), 7563–7568. doi: 10.1073/pnas.1502025112
Pier, G. B. (2007). Pseudomonas Aeruginosa Lipopolysaccharide: A Major Virulence Factor, Initiator of Inflammation and Target for Effective Immunity. Int. J. Med. Microbiol. 297 (5), 277–295. doi: 10.1016/j.ijmm.2007.03.012
Poole, K. (2011). Pseudomonas Aeruginosa: Resistance to the Max. Front. Microbiol. 2. doi: 10.3389/fmicb.2011.00065
Poole, K., McKay, G. A. (2003). Iron Acquisition and its Control in Pseudomonas Aeruginosa: Many Roads Lead to Rome. Front. Biosci. 8 (1), 661–686. doi: 10.2741/1051
Powell, L. C., Pritchard, M. F., Ferguson, E. L., Powell, K. A., Patel, S. U., Rye, P. D., et al. (2018). Targeted Disruption of the Extracellular Polymeric Network of Pseudomonas Aeruginosa Biofilms by Alginate Oligosaccharides. NPJ biofilms microbiomes 4, 13–13. doi: 10.1038/s41522-018-0056-3
Proctor, L. L., Ward, W. L., Roggy, C. S., Koontz, A. G., Clark, K. M., Quinn, A. P., et al. (2021). Potential Therapeutic Targets for Combination Antibody Therapy Against Pseudomonas Aeruginosa Infections. Antibiotics 10 (12), 1530. doi: 10.3390/antibiotics10121530
Quinaud, M., Chabert, J., Faudry, E., Neumann, E., Lemaire, D., Pastor, A., et al. (2005). The PscE-PscF-PscG Complex Controls Type III Secretion Needle Biogenesis in Pseudomonas Aeruginosa. J. Biol. Chem. 280 (43), 36293–36300. doi: 10.1074/jbc.M508089200
Rashid, M. H., Rumbaugh, K., Passador, L., Davies, D. G., Hamood, A. N., Iglewski, B. H., et al. (2000). Polyphosphate Kinase is Essential for Biofilm Development, Quorum Sensing, and Virulence of Pseudomonas Aeruginosa. Proc. Natl. Acad. Sci. 97 (17), 9636–9641. doi: 10.1073/pnas.170283397
Rasko, D. A., Sperandio, V. (2010). Anti-Virulence Strategies to Combat Bacteria-Mediated Disease. Nat. Rev. Drug Discovery 9 (2), 117–128. doi: 10.1038/nrd3013
Ravel, J., Cornelis, P. (2003). Genomics of Pyoverdine-Mediated Iron Uptake in Pseudomonads. Trends Microbiol. 11 (5), 195–200. doi: 10.1016/s0966-842x(03)00076-3
Rezzoagli, C., Archetti, M., Mignot, I., Baumgartner, M., Kümmerli, R. (2020). Combining Antibiotics With Antivirulence Compounds can Have Synergistic Effects and Reverse Selection for Antibiotic Resistance in Pseudomonas Aeruginosa. PloS Biol. 18 (8), e3000805. doi: 10.1371/journal.pbio.3000805
Rice, L. B. (2008). Federal Funding for the Study of Antimicrobial Resistance in Nosocomial Pathogens: No ESKAPE. J. Infect. Dis. 197 (8), 1079–1081. doi: 10.1086/533452
Roche, B., Mislin, G. L., Schalk, I. J. (2021). Identification of the Fatty Acid Coenzyme-A Ligase FadD1 as an Interacting Partner of FptX in the Pseudomonas Aeruginosa Pyochelin Pathway. FEBS Lett. 595 (3), 370–378. doi: 10.1002/1873-3468.14012
Rollet, C., Gal, L., Guzzo, J. (2009). Biofilm-Detached Cells, a Transition From a Sessile to a Planktonic Phenotype: A Comparative Study of Adhesion and Physiological Characteristics in Pseudomonas Aeruginosa. FEMS Microbiol. Lett. 290 (2), 135–142. doi: 10.1111/j.1574-6968.2008.01415.x
Römling, U., Balsalobre, C. (2012). Biofilm Infections, Their Resilience to Therapy and Innovative Treatment Strategies. J. Internal Med. 272 (6), 541–561. doi: 10.1111/joim.12004
Rosenau, F., Isenhardt, S., Gdynia, A., Tielker, D., Schmidt, E., Tielen, P., et al. (2010). Lipase LipC Affects Motility, Biofilm Formation and Rhamnolipid Production in Pseudomonas Aeruginosa. FEMS Microbiol. Lett. 309 (1), 25–34. doi: 10.1111/j.1574-6968.2010.02017.x
Roy-Burman, A., Savel, R. H., Racine, S., Swanson, B. L., Revadigar, N. S., Fujimoto, J., et al. (2001). Type III Protein Secretion Is Associated With Death in Lower Respiratory and Systemic Pseudomonas Aeruginosa Infections. J. Infect. Dis. 183 (12), 1767–1774. doi: 10.1086/320737
Russell, A. B, Hood, R. D., Bui, N. K, LeRoux, M., Vollmer, W., Mougous, J. D. (2011). Type VI Secretion Delivers Bacteriolytic Effectors to Target Cells. Nature 475(7356), 343–7. doi: 10.1038/nature10244
Rutherford, S. T., Bassler, B. L. (2012). Bacterial Quorum Sensing: Its Role in Virulence and Possibilities for its Control. Cold Spring Harbor Perspect. Med. 2 (11), a012427. doi: 10.1101/cshperspect.a012427
Salacha, R., Kovačić, F., Brochier-Armanet, C., Wilhelm, S., Tommassen, J., Filloux, A., et al. (2010). The Pseudomonas Aeruginosa Patatin-Like Protein PlpD is the Archetype of a Novel Type V Secretion System. Environ. Microbiol. 12 (6), 1498–1512. doi: 10.1111/j.1462-2920.2010.02174.x
Sampedro, I., Parales, R. E., Krell, T., Hill, J. E. (2015). Pseudomonas Chemotaxis. FEMS Microbiol. Rev. 39 (1), 17–46. doi: 10.1111/1574-6976.12081
Sass, G., Conrad, L., Nguyen, T., Stevens, D. A. (2020). The Pseudomonas Aeruginosa Product Pyochelin Interferes With Trypanosoma Cruzi Infection and Multiplication In Vitro. Trans. R. Soc. Trop. Med. Hygiene 114 (7), 492–498. doi: 10.1093/trstmh/trz136
Sato, H., Frank, D. (2011). Multi-Functional Characteristics of the Pseudomonas Aeruginosa Type III Needle-Tip Protein, PcrV; Comparison to Orthologs in Other Gram-Negative Bacteria. Front. Microbiol. 2. doi: 10.3389/fmicb.2011.00142
Sato, H., Frank, D. W., Hillard, C. J., Feix, J. B., Pankhaniya, R. R., Moriyama, K., et al. (2003). The Mechanism of Action of the Pseudomonas Aeruginosa-Encoded Type III Cytotoxin, ExoU. EMBO J. 22 (12), 2959–2969. doi: 10.1093/emboj/cdg290
Sawa, T., Yahr, T. L., Ohara, M., Kurahashi, K., Gropper, M. A., Wiener-Kronish, J. P., et al. (1999). Active and Passive Immunization With the Pseudomonas V Antigen Protects Against Type III Intoxication and Lung Injury. Nat. Med. 5 (4), 392–398. doi: 10.1038/7391
Scharmann, W. (1976). Cytotoxic Effects of Leukocidin From Pseudomonas Aeruginosa on Polymorphonuclear Leukocytes From Cattle. Infect Immun. 13 (3), 836–843. doi: 10.1128/iai.13.3.836-843.1976
Secher, T., Fauconnier, L., Szade, A., Rutschi, O., Fas, S. C., Ryffel, B., et al. (2011). Anti-Pseudomonas Aeruginosa Serotype O11 LPS Immunoglobulin M Monoclonal Antibody Panobacumab (KBPA101) Confers Protection in a Murine Model of Acute Lung Infection. J. Antimicro Chemother 66 (5), 1100–1109. doi: 10.1093/jac/dkr038
Shen, E. P., Hsieh, Y.-T., Chu, H.-S., Chang, S.-C., Hu, F.-R. (2015). Correlation of Pseudomonas Aeruginosa Genotype With Antibiotic Susceptibility and Clinical Features of Induced Central Keratitis. Invest. Ophthalmol. Visual Sci. 56 (1), 365–371. doi: 10.1167/iovs.14-15241
Sheremet, A. B., Zigangirova, N. A., Zayakin, E. S., Luyksaar, S. I., Kapotina, L. N., Nesterenko, L. N., et al. (2018). Small Molecule Inhibitor of Type Three Secretion System Belonging to a Class 2,4-Disubstituted-4h-[1,3,4]-Thiadiazine-5-Ones Improves Survival and Decreases Bacterial Loads in an Airway Pseudomonas Aeruginosa Infection in Mice. BioMed. Res. Int. 2018, 5810767. doi: 10.1155/2018/5810767
Song, Z., Wu, H., Ciofu, O., Kong, K.-F., Høiby, N., Rygaard, J., et al. (2003). Pseudomonas Aeruginosa Alginate is Refractory to Th1 Immune Response and Impedes Host Immune Clearance in a Mouse Model of Acute Lung Infection. J. Med. Microbiol. 52 (9), 731–740. doi: 10.1099/jmm.0.05122-0
Starkey, M., Lepine, F., Maura, D., Bandyopadhaya, A., Lesic, B., He, J., et al. (2014). Identification of Anti-Virulence Compounds That Disrupt Quorum-Sensing Regulated Acute and Persistent Pathogenicity. PloS Pathog. 10 (8), e1004321. doi: 10.1371/journal.ppat.1004321
Sundin, C., Henriksson, M. L., Hallberg, B., Forsberg, Å., Frithz-Lindsten, E. (2001). Exoenzyme T of Pseudomonas Aeruginosa Elicits Cytotoxicity Without Interfering With Ras Signal Transduction. Cell. Microbiol. 3 (4), 237–246. doi: 10.1046/j.1462-5822.2001.00108.x
Sun, Z., Kang, Y., Norris, M. H., Troyer, R. M., Son, M. S., Schweizer, H. P., et al. (2014). Blocking Phosphatidylcholine Utilization in Pseudomonas Aeruginosa, via Mutagenesis of Fatty Acid, Glycerol and Choline Degradation Pathways, Confirms the Importance of This Nutrient Source In Vivo. PloS One 9 (7), e103778. doi: 10.1371/journal.pone.0103778
Talà, L., Fineberg, A., Kukura, P., Persat, A. (2019). Pseudomonas Aeruginosa Orchestrates Twitching Motility by Sequential Control of Type IV Pili Movements. Nat. Microbiol. 4 (5), 774–780. doi: 10.1038/s41564-019-0378-9
Taylor, P. K., Yeung, A. T. Y., Hancock, R. E. W. (2014). Antibiotic Resistance in Pseudomonas Aeruginosa Biofilms: Towards the Development of Novel Anti-Biofilm Therapies. J. Biotechnol. 191, 121–130. doi: 10.1016/j.jbiotec.2014.09.003
Terada, L. S., Johansen, K. A., Nowbar, S., Vasil, A. I., Vasil, M. L. (1999). Pseudomonas Aeruginosa Hemolytic Phospholipase C Suppresses Neutrophil Respiratory Burst Activity. Infect Immun. 67 (5), 2371–2376. doi: 10.1128/IAI.67.5.2371-2376.1999
Thi, M. T. T., Wibowo, D., Rehm, B. H. (2020). Pseudomonas Aeruginosa Biofilms. Int. J. Mol. Sci. 21 (22), 8671. doi: 10.3390/ijms21228671
Tielen, P., Kuhn, H., Rosenau, F., Jaeger, K.-E., Flemming, H.-C., Wingender, J. (2013). Interaction Between Extracellular Lipase LipA and the Polysaccharide Alginate of Pseudomonas Aeruginosa. BMC Microbiol. 13 (1), 1–12. doi: 10.1186/1471-2180-13-159
Tielen, P., Rosenau, F., Wilhelm, S., Jaeger, K.-E., Flemming, H.-C., Wingender, J. (2010). Extracellular Enzymes Affect Biofilm Formation of Mucoid Pseudomonas Aeruginosa. Microbiology 156 (7), 2239–2252. doi: 10.1099/mic.0.037036-0
Triantafilou, M., Triantafilou, K. (2002). Lipopolysaccharide Recognition: CD14, TLRs and the LPS-Activation Cluster. Trends Immunol. 23 (6), 301–304. doi: 10.1016/S1471-4906(02)02233-0
Vasil, M. L., Graham, L. M., Ostroff, R. M., Shortridge, V. D., Vasil, A. I. (1991). Phospholipase C: Molecular Biology and Contribution to the Pathogenesis of Pseudomonas Aeruginosa. Antibiot Chemother. 44, 34–47. doi: 10.1159/000420295
Veesenmeyer, J. L., Hauser, A. R., Lisboa, T., Rello, J. (2009). Pseudomonas Aeruginosa Virulence and Therapy: Evolving Translational Strategies. Crit. Care Med. 37 (5), 1777–1786. doi: 10.1097/CCM.0b013e31819ff137
Verma, N., Dollinger, P., Kovacic, F., Jaeger, K.-E., Gohlke, H. (2020). The Membrane-Integrated Steric Chaperone Lif Facilitates Active Site Opening of Pseudomonas Aeruginosa Lipase A. J. Comput. Chem. 41 (6), 500–512. doi: 10.1002/jcc.26085
Viegas, A., Dollinger, P., Verma, N., Kubiak, J., Viennet, T., Seidel, C. A., et al. (2020). Structural and Dynamic Insights Revealing How Lipase Binding Domain MD1 of Pseudomonas Aeruginosa Foldase Affects Lipase Activation. Sci. Rep. 10 (1), 1–15. doi: 10.1038/s41598-020-60093-4
Wargo, M. J., Gross, M. J., Rajamani, S., Allard, J. L., Lundblad, L., Allen, G. B., et al. (2011). Hemolytic Phospholipase C Inhibition Protects Lung Function During Pseudomonas Aeruginosa Infection. Am. J. Respir. Crit. Care Med. 184 (3), 345. doi: 10.1164/rccm.201103-0374OC
Webster, S. S., Lee, C. K., Schmidt, W. C., Wong, G., O'Toole, G. A. (2021). Interaction Between the Type 4 Pili Machinery and a Diguanylate Cyclase Fine-Tune C-Di-GMP Levels During Early Biofilm Formation. Proc. Natl. Acad. Sci. United States America 118 (26), e2105566118. doi: 10.1073/pnas.2105566118
Whitchurch, C. B., Leech, A. J., Young, M. D., Kennedy, D., Sargent, J. L., Bertrand, J. J., et al. (2004). Characterization of a Complex Chemosensory Signal Transduction System Which Controls Twitching Motility in Pseudomonas Aeruginosa. Mol. Microbiol. 52 (3), 873–893. doi: 10.1111/j.1365-2958.2004.04026.x
Wilson, R., Sykes, D. A., Watson, D., Rutman, A., Taylor, G. W., Cole, P. J. (1988). Measurement of Pseudomonas Aeruginosa Phenazine Pigments in Sputum and Assessment of Their Contribution to Sputum Sol Toxicity for Respiratory Epithelium. Infect Immun. 56 (9), 2515–2517. doi: 10.1128/iai.56.9.2515-2517.1988
Woo, J., Hearne, K., Kelson, A., Yee, L., Espadas, C., Truong, V. L (2019). “"716. In Vitro and In Vivo Nonclinical Efficacy of AR-501 (Gallium Citrate)",” in Open Forum Infectious Diseases. (Washington, D. C.:Oxford Academic) 6(Supplement_2), S322. doi: 10.1093/ofid/ofz360.784
World Health Organization (2017) WHO Publishes List of Bacteria for Which New Antibiotics are Urgently Needed. Available at: https://www.who.int/news/item/27-02-2017-who-publishes-list-of-bacteria-for-which-new-antibiotics-are-urgently-needed (Accessed May 7 2022).
Wu, M., Li, X. (2015). “"Chapter 87 - Klebsiella Pneumoniae and Pseudomonas Aeruginosa,",” in Molecular Medical Microbiology (Second Edition). Eds. Tang, Y.-W., Sussman, M., Liu, D., Poxton, I., Schwartzman, J. (Boston: Academic Press), 1547–1564. doi: 10.1016/B978-0-12-397169-2.00087-1
Wu, H., Song, Z., Hentzer, M., Andersen, J. B., Molin, S., Givskov, M., et al. (2004). Synthetic Furanones Inhibit Quorum-Sensing and Enhance Bacterial Clearance in Pseudomonas Aeruginosa Lung Infection in Mice. J. Antimicro Chemother 53 (6), 1054–1061. doi: 10.1093/jac/dkh223
Yaeger, L. N., Coles, V. E., Chan, D. C., Burrows, L. L. (2021). How to Kill Pseudomonas—emerging Therapies for a Challenging Pathogen. Ann. New York Acad. Sci. 1496 (1), 59–81. doi: 10.1111/nyas.14596
Yahr, T. L., Goranson, J., Frank, D. W. (1996). Exoenzyme S of Pseudomonas Aeruginosa is Secreted by a Type III Pathway. Mol. Microbiol. 22 (5), 991–1003. doi: 10.1046/j.1365-2958.1996.01554.x
Yahr, T. L., Vallis, A. J., Hancock, M. K., Barbieri, J. T., Frank, D. W. (1998). ExoY, an Adenylate Cyclase Secreted by the Pseudomonas Aeruginosa Type III System. Proc. Natl. Acad. Sci. 95 (23), 13899–13904. doi: 10.1073/pnas.95.23.13899
Zhang, A., Veesenmeyer, J. L., Hauser, A. R., Payne, S. M. (2018). Phosphatidylinositol 4,5-Bisphosphate-Dependent Oligomerization of the Pseudomonas Aeruginosa Cytotoxin ExoU. Infect Immun. 86 (1), e00402–e00417. doi: 10.1128/IAI.00402-17
Keywords: antivirulence strategies, Pseudomonas aeruginosa, virulence factors, antibiotic resistance, infection
Citation: Liao C, Huang X, Wang Q, Yao D and Lu W (2022) Virulence Factors of Pseudomonas Aeruginosa and Antivirulence Strategies to Combat Its Drug Resistance. Front. Cell. Infect. Microbiol. 12:926758. doi: 10.3389/fcimb.2022.926758
Received: 23 April 2022; Accepted: 09 June 2022;
Published: 06 July 2022.
Edited by:
Raja Veerapandian, Texas Tech University Health Sciences Center El Paso, United StatesReviewed by:
Zheng Pang, Shandong University of Traditional Chinese Medicine, ChinaPaulo Juarez, SMALTIS, France
Copyright © 2022 Liao, Huang, Wang, Yao and Lu. This is an open-access article distributed under the terms of the Creative Commons Attribution License (CC BY). The use, distribution or reproduction in other forums is permitted, provided the original author(s) and the copyright owner(s) are credited and that the original publication in this journal is cited, in accordance with accepted academic practice. No use, distribution or reproduction is permitted which does not comply with these terms.
*Correspondence: Chongbing Liao, Y2xpYW9AZnVkYW4uZWR1LmNu; Wuyuan Lu, bHV3dXl1YW5AZnVkYW4uZWR1LmNu
†These authors have contributed equally to this work