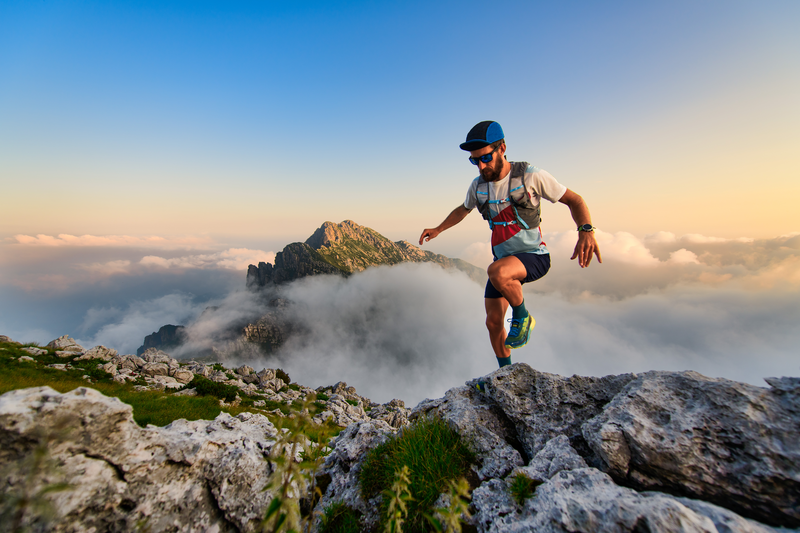
94% of researchers rate our articles as excellent or good
Learn more about the work of our research integrity team to safeguard the quality of each article we publish.
Find out more
ORIGINAL RESEARCH article
Front. Cell. Infect. Microbiol. , 27 July 2022
Sec. Fungal Pathogenesis
Volume 12 - 2022 | https://doi.org/10.3389/fcimb.2022.924764
The emergence of Neisseria gonorrhoeae strains resistant to extended-spectrum cephalosporins (ESCs) is a worldwide concern because this class of antibiotics represents the last empirical treatment option for gonorrhea. The abusive use of antimicrobials may be an essential factor for the emergence of ESC resistance in N. gonorrhoeae. Cephalosporin resistance mechanisms have not been fully clarified. In this study, we mapped mutations in the genome of N. gonorrhoeae isolates after resistance induction with cefixime and explored related metabolic pathways. Six clinical isolates with different antimicrobial susceptibility profiles and genotypes and two gonococcal reference strains (WHO F and WHO Y) were induced with increasing concentrations of cefixime. Antimicrobial susceptibility testing was performed against six antimicrobial agents before and after induction. Clinical isolates were whole-genome sequenced before and after induction, whereas reference strains were sequenced after induction only. Cefixime resistance induction was completed after 138 subcultures. Several metabolic pathways were affected by resistance induction. Five isolates showed SNPs in PBP2. The isolates M111 and M128 (ST1407 with mosaic penA-34.001) acquired one and four novel missense mutations in PBP2, respectively. These isolates exhibited the highest minimum inhibitory concentration (MIC) for cefixime among all clinical isolates. Mutations in genes contributing to ESC resistance and in other genes were also observed. Interestingly, M107 and M110 (ST338) showed no mutations in key determinants of ESC resistance despite having a 127-fold increase in the MIC of cefixime. These findings point to the existence of different mechanisms of acquisition of ESC resistance induced by cefixime exposure. Furthermore, the results reinforce the importance of the gonococcal antimicrobial resistance surveillance program in Brazil, given the changes in treatment protocols made in 2017 and the nationwide prevalence of sequence types that can develop resistance to ESC.
Neisseria gonorrhoeae (gonococcus) is the causative agent of gonorrhea, a sexually transmitted infection with an estimated 87 million new cases per year globally (Rowley et al., 2019). Since the introduction of the first antimicrobial treatment for gonorrhea, gonococcus has developed antimicrobial resistance (AMR) against all classes of antimicrobials. Resistance to extended-spectrum cephalosporins (ESCs) is a current challenge worldwide, as this class of antimicrobials represents the last empirical monotherapy for gonorrhea (Unemo, 2015).
After the first report of ESC-resistant N. gonorrhoeae (Ameyama et al., 2002), new cases were reported in 2011 in France (Unemo et al., 2012) and in 2012 in Spain (Cámara et al., 2012), with both isolates belonging to the ST1407. This clone was responsible for a large proportion of cases of decreased susceptibility and resistance to ESCs in several countries (Unemo and Nicholas, 2012). In N. gonorrhoeae, resistance to cephalosporins is primarily due to mutations in penicillin-binding protein 2 (PBP2), encoded by penA. Some isolates have acquired several mutations in penA by DNA transformation and recombination with penA genes from commensal Neisseria spp. (Ameyama et al., 2002; Ito et al., 2005; Osaka et al., 2008). Besides that, mutations in porin PorB1b and in the efflux pump MtrCDE can contribute to AMR by decreasing the influx and increasing the efflux of ESCs (Hagman and Shafer, 1995; Gill et al., 1998; Olesky et al., 2002; Zhao et al., 2009; Ohneck et al., 2011; Golparian et al., 2014).
Surveillance of gonococcal antimicrobial susceptibility has stimulated changes in the treatment of gonorrhea to slow up the emergence of resistant isolates. Several reports of oral cefixime treatment failures and an increase in resistance levels raised concerns about the use of cefixime, mainly as monotherapy (Chisholm et al., 2010; Unemo and Nicholas, 2012; Allen et al., 2013; Lewis, 2015; Unemo, 2015; Barbee et al., 2018; Unemo et al., 2019). Therefore, several countries have introduced dual antimicrobial therapy, such as ceftriaxone plus azithromycin, with the possibility of replacing ceftriaxone for cefixime when administration of an intramuscular injection of ceftriaxone is contraindicated or refused (Unemo et al., 2020). In Brazil, clinical protocols for the management of sexually transmitted infections were introduced in 1993 (Moherdaui et al., 1998). Until 2017, ciprofloxacin and azithromycin were the first-line treatment for uncomplicated gonococcal urethritis (Brasil, 2015). After that, however, ceftriaxone plus azithromycin was recommended based on the findings of the first national surveillance of antimicrobial susceptibility in N. gonorrhoeae (Bazzo et al., 2018). Cefixime is not commercialized in Brazil, which decreases the selection pressure on N. gonorrhoeae isolates (Brasil, 2020).
This study aimed to evaluate the behavior of the most prevalent sequence types (STs) of N. gonorrhoeae isolates in Brazil upon exposure to the antimicrobial cefixime. Gonococcus isolates with borderline resistance to cefixime were identified in Brazil in 2015–2016 (Bazzo et al., 2018) and, in 2017, the country issued its first nationwide recommendation for the use of an ESC for gonococcal infection. Given that cephalosporin resistance mechanisms are not fully clarified, this study may contribute to further understanding of the molecular mechanisms of ESC resistance in N. gonorrhoeae driven by selective pressure.
Eight gonococcal isolates were selected: a single blood culture isolate from an inpatient at the University Hospital of the Federal University of Santa Catarina; five male urethral discharge isolates selected from a nationwide study, the Brazilian-GASP (Gonococcal Antimicrobial Surveillance Programme) (Bazzo et al., 2018); and two reference strains, WHO F and WHO Y (Unemo et al., 2016).
These isolates were selected based on cefixime susceptibility profile and ST. We included cefixime-susceptible isolates with an 8- to 32-fold minimum inhibitory concentration (MIC) lower than 0.125 mg/L and an MIC of 0.125 mg/L (susceptibility threshold breakpoint). ST1407 was included because of previous reports related to ESC resistance spread (Cámara et al., 2012; Unemo et al., 2012). ST338 was included because it was found to be highly prevalent in Florianópolis, Santa Catarina, Southern Brazil (Golfetto, 2018) (Table 1).
Table 1 Antimicrobial susceptibilities and molecular typing of Neisseria gonorrhoeae isolates included in this study.
N. gonorrhoeae isolates were identified using a Vitek2 Compact instrument (BioMérieux S.A., Marcy l’Etoile, France). All isolates were stored at −80°C in casein-peptone soymeal-peptone broth (BD, Difco™, USA) containing 20% glycerol until further analysis.
Gonococcal isolates were recovered from cryopreservation on chocolate agar plates (Laborclin, Parana, Brazil) and subcultured on the same medium. To induce resistance to cefixime, we subcultured a single colony on GC agar (Difco™, New Jersey, USA) supplemented with 1% Vitox (Oxoid™, Hampshire, UK) containing different concentrations of cefixime, starting from 0.002 mg/L. The colonies were subcultured on two GC plates: one containing the same concentration of cefixime and another with a higher concentration (1.5- to 2-fold each test). When the isolate grew on the higher-concentration plate, it was challenged to an even higher concentration of cefixime. All plates were incubated at 35 ± 1°C in 5% CO2 for 18 to 24 h. In vitro induction was considered complete when all isolates achieved stable growth at a cefixime concentration above the resistance breakpoint, according to guidelines of the Brazilian Committee on Antimicrobial Susceptibility Testing (BrCAST) (>0.125 mg/L, http://brcast.org.br/, accessed in March 2021). After resistance induction, all isolates were confirmed as N. gonorrhoeae using a Vitek2 Compact instrument and stored at −80°C in casein-peptone soymeal-peptone broth containing 20% glycerol.
Antimicrobial susceptibility testing was performed for six antimicrobial agents (Table 1) by agar dilution, as recommended by the Clinical and Laboratory Standards Institute. MICs were interpreted according to CLSI (CLSI, 2019) and BrCAST (available in http://brcast.org.br/) standards. N. gonorrhoeae WHO F, G, K, L, and M were used as controls (Unemo et al., 2016).
N. gonorrhoeae multi-antigen sequence typing (NG-MAST) was performed by PCR and sequencing of two N. gonorrhoeae genes (porB and tbpB), following the protocol developed by Martin et al. (2004). STs were identified by comparison with the NG-MAST database (http://www.ng-mast.net, accessed in 2018) (Golfetto, 2018).
All isolates were sequenced, except WHO F and WHO Y before resistance induction. Genomic DNA was extracted using a PureLink™ Genomic DNA Mini Kit (Thermo Fisher Scientific Inc., Waltham, Massachusetts, USA) and quantified using the Qubit dsDNA BR Assay Kit (Thermo Fisher Scientific Inc). After quantification, DNA was diluted to 0.5 ng/µl. Illumina sequencing libraries were generated using an Illumina Nextera XT DNA Library Kit (Illumina Inc., San Diego, CA, USA). Whole-genome sequencing of paired-end libraries (PE, 2 × 250 bp) was performed using the Illumina MiSeq platform (Illumina Inc.).
Raw read quality was checked using FastQC version 0.11.8 (available in https://www.bioinformatics.babraham.ac.uk/projects/fastqc/), and quality-based trimming and filtering were performed using Trimmomatic version 0.39 (Bolger et al., 2014). Only paired-end reads from clinical isolates before cefixime resistance induction were assembled using SPAdes version 3.13.1 (Bankevich et al., 2012). Scaffold sequences were annotated using Prokka version 1.14.0 (Seemann, 2014). For reference strains, the genomes available at NCBI were used (WHO F, GenBank accession no. LT591897.1 and WHO Y, accession no. LT592161.1). In silico sequence typing was performed using MLST version 2.0 (https://cge.cbs.dtu.dk/services/MLST, accessed in January 2021).
Chromosomal and plasmid antibiotic resistance genes from assembled draft genomes of clinical isolates before resistance induction were predicted using the Comprehensive Antimicrobial Resistance Database (CARD) version 3.1.1 (https://card.mcmaster.ca/, accessed in January 2021) (McArthur et al., 2013). Mutations in antibiotic resistance-related genes were curated using Artemis version 18.0.0 (Carver et al., 2005).
For identification of cefixime resistance-induced mutations in the genome of gonococcal isolates, a single-nucleotide polymorphism (SNP)-calling pipeline was constructed by mapping reads from genomes sequenced after resistance induction onto the assembled genomes. This procedure was performed using BWA version 0.7.17-r1188 (Li and Durbin, 2009). SAMTools version 1.7 (Li et al., 2009) was used for manipulation of alignments. SNP calling was performed using BCFtools version 1.7 (Li, 2011), with a minimum Phred quality score of 23. The vcfutils.pl script was used to filter variants with at least 20-fold coverage depth. SNPs, nonsynonymous mutations, frameshift mutations caused by indels, and nonsense mutations resulting in premature stop codons in hypothetical proteins and coding sequences were annotated using SnpEff version 4 (Cingolani et al., 2012). A heatmap of mutations in known coding sequences in N. gonorrhoeae isolates after selection for resistance to cefixime (Figure 2) was constructed using R software version 4.0.2, and hierarchical clustering was applied using the default Ward2 algorithm (Murtagh and Legendre, 2014).
The Institutional Ethics Review Board of the University Hospital of the Federal University of Santa Catarina (HU/UFSC) approved this study under reference number CAAE 13727619.4.0000.0121.
Raw data and genome assemblies of all gonococcal isolates were deposited in the NCBI database under accession numbers SAMN26195797 to SAMN26195810.
Draft genome sequences of isolates before selection for resistance to cefixime were used for genotypic characterization and identification of possible AMR determinants. Relevant genetic traits for antimicrobial resistance in N. gonorrhoeae are described in Table 2.
Table 2 Genetic traits of relevance for antimicrobial resistance in Neisseria gonorrhoeae isolates included in this study.
All isolates included in this study were found susceptible to the antimicrobials currently in use for the treatment of gonococcal infection in Brazil (ceftriaxone plus azithromycin) (Brasil, 2020). Of all isolates, M009, recovered from a blood sample, was the most susceptible to antimicrobials. This isolate showed mutations in PBP1 and PBP2, which are related to an increase in the MIC of penicillin. Furthermore, M009 was the only isolate to carry a TetM plasmid, which confers a high level of resistance to tetracycline.
Urethral discharge samples (M043, M107, M110, M111, and M128) showed similar resistance determinants according to ST profile. Samples belonging to ST1407/ST1901 (M043, M111, and M128) had a mosaic penA-34.001. The penA mosaic allele, generated by DNA transformation followed by recombination with commensal Neisseria spp., is associated with reduced susceptibility/resistance to ESCs, having a greater impact on the MIC of cefixime than that of ceftriaxone. The MIC of cefixime against these isolates (0.125 mg/L) revealed borderline resistance, according to BrCAST criteria (resistance = MIC > 0.125 mg/L); the MIC of ceftriaxone ranged from 0.03 to 0.06 mg/L. In addition to alterations in the primary ESC resistance mechanism, ST1407 isolates also exhibited alterations in PorB1b porin and MtrCDE efflux pump, influencing the MIC of ESCs; such alterations promote decreased influx and increased efflux of ESCs, respectively.
The MIC of ESCs for samples belonging to ST338/ST1588 (M107 and M110) was lower than that for ST1407. M107 and M110 harbored the penA-19.001 non-mosaic allele, and no alteration was found in the major determinants of ESC resistance. Of the six antimicrobials analyzed, M107 and M110 were only resistant to ciprofloxacin and penicillin. This pattern was associated with mutations in GyrA, ParC, and PBP1 as well as with the presence of β-lactamase-producing plasmid carrying blaTEM-1, which confers high resistance to penicillin but has no impact on the MIC of ESCs.
Isolates belonging to ST1407 and ST338 were resistant to ciprofloxacin because they contained mutations in GyrA and ParC. The 2-fold variation in MIC between STs may be explained by the extra GyrA mutation (D95G) observed in ST1407.
In vitro resistance induction of N. gonorrhoeae isolates to cefixime was completed after 138 subcultures. Figure 1 shows the progress curves during resistance evolution of each isolate. It can be noted that some isolates took longer to grow (M128 and WHO F), whereas others adapted more quickly to cefixime.
Figure 1 Progress curves during resistance evolution to cefixime. Dotted lines indicate minimum inhibitory concentration (MIC) values after induction. (A) M009, (B) M043, (C) M107, (D) M110, (E) M111, (F) M128, (G) WHO F, (H) WHO Y. The MIC for WHO Y is not shown (>32 mg/L).
There was an increase in cefixime MIC, such that all susceptible isolates became resistant according to BrCAST (resistant = MIC > 0.125 mg/L) and CLSI (resistant = MIC > 0.25 mg/L) criteria (Table 1). The MIC for M043 showed a minor variation, with an 8-fold increase compared with the initial MIC, and that for M128 increased 128-fold. The MIC against the unique gonococcus isolate WHO Y, which was initially resistant to cefixime, was greater than 32 mg/L after induction.
The MICs of cefixime, ceftriaxone, and penicillin differed after resistance induction, whereas those of azithromycin, ciprofloxacin, and tetracycline remained stable. We observed a reduction in the MIC of penicillin against M009 and M110; thus, these isolates, which were initially resistant, became sensitive to the antimicrobial. M110, which had a plasmid carrying blaTEM-1, lost the plasmid at the end of subculturing, resulting in a penicillin MIC of 0.5 mg/L.
Subculturing of isolates with cefixime resulted in mutations in genes related or not to ESC resistance. SNPs in the main resistance determinant (penA) were observed in five isolates: WHO F and M009, which do not have the penA mosaic form, and M043, M111, and M128, which belong to ST1407. M111 acquired one (A488V) and M128 acquired four (A318V, R356H, N506H, and G546A) novel mutations in penA. These isolates exhibited the highest cefixime MIC. Table 3 shows the changes in amino acids of isolates as compared with the reference M32091 (GenBank M32091.1).
Table 3 Amino acid sequence alignment of penicillin-binding protein 2 of Neisseria gonorrhoeae isolates before and after selection for resistance to cefixime.
In addition to mutations in penA, we observed nonsynonymous, frameshift, and stop codon mutations in genes contributing to ESC resistance and in other genes. Table S1 shows all mutations in coding genes that emerged in the isolates of this study. Figure 2 shows a heatmap of all mutations in known coding sequences. Several metabolic pathways were affected by cefixime induction. Two of the most affected pathways were cell wall biogenesis and cell division. Pilus formation genes (pil genes), associated with motility, adhesion, and secretion, were also affected at the DNA level. The other mutated genes were related to DNA recombination; energy production; metabolism/transport of amino acids, organic ions, carbohydrates, and coenzymes; post-transcriptional modifications; and translation. One gene was associated with an unknown function.
Figure 2 Heatmap of mutations in known coding sequences in Neisseria gonorrhoeae isolates after selection for resistance to cefixime and description of affected gene functions. Isolates and genes are presented in rows and columns, respectively. The blue color scale represents the amount of acquired mutations. The left sidebar represents the minimum inhibitory concentration (MIC) of cefixime (mg/L). The top bar indicates the function of each gene. _C indicates determinants related to extended-spectrum cephalosporin resistance; asterisk indicates genes that showed a frameshift.
A significant variation in mutation patterns was identified after induction with cefixime. No gene was altered in all isolates. M111 and M128 were grouped for having the highest number of mutations in penA. M107 and M110, which belong to ST338, formed a group. The other group was formed by WHO F isolates, M009 and M043, which exhibited the lowest cefixime MIC values.
WHO Y, which did not show any mutation in penA but exhibited an increase in the MIC of ESCs, suffered mutations in some resistance genes, including porin PorB1b (A26V), MtrC (S23P), and MtrD (M570K). Mutations in PilQ were observed in M128 (G590D) and WHO Y (guanine insertion between nucleotides 1566 and 1567), resulting in a frameshift. Interestingly, M107 and M110 did not acquire mutations in the main determinants of resistance to ESCs. Only M110 acquired an SNP in ponA (PBP1), which resulted in an A615V amino acid change in this protein. Nevertheless, we observed alterations in genes involved in cellular division. M107 acquired a guanine insertion between nucleotides 299 and 300, resulting in a frameshift in zapA, and M110 acquired the A318V mutation in FtsZ.
In this study, we assessed DNA alterations in N. gonorrhoeae isolates after cefixime exposure. WHO F, WHO Y, one N. gonorrhoeae isolate from blood culture, and the two most prevalent STs in Brazil were used (Golparian et al., 2020).
The MIC of cefixime increased above the breakpoint (as determined by CLSI and BrCAST criteria) for all isolates postselection, indicating that isolates acquired resistance to this antimicrobial. A study conducted by Gong et al. (2016) subjected five WHO reference strains of N. gonorrhoeae and one clinical isolate to resistance induction using ceftriaxone. Only the reference strain WHO D acquired resistance after 136 subcultures, increasing the MIC to 1.0 mg/L (initial MIC = 0.016 mg/L). WHO D possessed non-mosaic penA, adenine deletion in the mtrR promoter region, and L421P amino acid change in PBP1. Furthermore, Gong et al. (2016) found that, of all frequently reported genes associated with ceftriaxone resistance in N. gonorrhoeae, only penA was mutated in WHO D (A501V). Although Gong et al. (2016) used liquid cultures to induce resistance, this finding may demonstrate a low ability of ceftriaxone to induce resistance in vitro in N. gonorrhoeae compared with cefixime.
The PBP2 protein is part of class B penicillin-binding proteins, which catalyze the last step of peptidoglycan formation by crosslinking peptides (Ghuysen, 1991). The active site of PBP2, to which β-lactam antimicrobials bind via an acylation reaction, contains three conserved motifs: SxxK, which comprises the amino acids Ser-310 and Lys-313 (α2 helix); SxN, which contains Ser-362 and Asn-364 (between helices α4 and α5); and KTG, comprising amino acids Lys-497, Tre-498, and Gly-499 (β3 sheet) (Powell et al., 2009). The presence of mosaic penA is associated with the emergence of mutations close to these motifs. Such mutations alter protein conformation, impairing the binding of antimicrobials or preventing conformational changes that are necessary for acylation by β-lactams (Osaka et al., 2008; Powell et al., 2009). The first isolate showing a high level of ESC resistance was reported in Japan (H041) (Ohnishi et al., 2011; Unemo and Shafer, 2014). Ceftriaxone and cefixime had MIC values of 2.0 and 4.0 mg/L, respectively, against the isolate. Later studies demonstrated that A311V (observed here in M009 and M128), V316P, and T483S mutations resulted in a significant increase in the MIC values of cefixime and ceftriaxone (Tomberg et al., 2013; Unemo and Shafer, 2014). The A311V alteration is present in most isolates reported with ESC resistance and was found to emerge in M009 and M128 after resistance induction, which probably contributed to the increase in MIC values for these isolates.
Interestingly, the isolates for which MIC values were highest showed amino acid changes not previously described. M128 showed four amino acid changes (A318V, R356H, N506H, and G546A) and M111 exhibited the A488V mutation, also not previously described. These mutations appear close to conserved motifs that are part of the PBP2 active site. Furthermore, the presence of T534A mutations in M111 and A311V mutation in M128, associated with the P551S mutation, whose simultaneous occurrence in isolates with ESC resistance is not common, may be responsible for the high MIC values. It is noteworthy that, in addition to the acquired mutations in penA, these isolates previously exhibited mutations in PorB1b and the mtrR promoter region, which probably helped to increase the MIC. The contribution of PBP2 mutations in M111 and M128 after induction, as well as the ability of the microorganism to face these changes, still needs to be investigated.
The amino acid change T534A, found in this study only in M111, was described in Austria in an isolate belonging to ST1407 with mosaic penA-34.001. The isolate led to treatment failure with the use of cefixime. Transformation experiments confirmed that only this new mutation was responsible for ESC resistance (Unemo et al., 2011). The Austrian isolate also had a deletion in the mtrR promoter region, G120K and A121N mutations in PorB, and L421P mutation in PBP1, as did M111 in the current study. However, the Austrian isolate had lower MICs than M111 (1.0 mg/L for cefixime and 0.5 mg/L for ceftriaxone), suggesting that other mutations might have contributed to the increase in MIC in M111.
P551S was the most frequent PBP2 mutation in isolates postselection. This mutation was observed in WHO F and isolates belonging to ST1407. The P551S mutation is more frequent in isolates lacking the mosaic penA. Kinetic analysis of β-lactam acylation at the active site of PBP2 indicated that two mutations (P551S and F504L) are responsible for reducing the acylation index, which minimizes the affinity for PBP2–antimicrobial complex formation (Powell et al., 2009). These mutations are usually found in non-mosaic type PBP2; however, they emerged in penA-34.001 isolates after antimicrobial selection in the current study.
In addition to the occurrence of penA mutations in most of the isolates postselection, we observed mutations in genes associated or not with ESC resistance. Various metabolic pathways were affected by selection with cefixime. Given that cefixime acts by inhibiting bacterial cell wall formation, most of the mutated genes are involved in cell wall biogenesis and cell division. Thirteen genes exhibited frameshift mutations, which may lead to the formation of defective proteins. Because we did not analyze gene expression, it was not possible to affirm whether these pathways were over- or underexpressed. In addition to a possible association with ESC resistance, the variety of genes found with mutations in this study may represent the acquisition of compensatory mutations. Vincent et al. (2018) demonstrated the acquisition of this type of mutation in acnB gene in an N. gonorrhoeae isolate containing mosaic penA. In fact, compensatory mutations may emerge in N. gonorrhoeae ESC-resistant to improve their fitness, thus being able to maintain and disseminate the lineage.
The mutations associated with decreased permeability of antimicrobials in N. gonorrhoeae are amino acid substitutions at positions 120 and 121 of PorB1b (Olesky et al., 2002; Unemo and Shafer, 2014). The impact of these mutations on cephalosporin resistance in clinical isolates only became apparent with overexpression of the MtrCDE efflux pump (Olesky et al., 2006; Shafer and Folster, 2006; Unemo and Shafer, 2014). Such an effect causes a decrease in antimicrobial input and an increase in output. The PorB1b mutation observed in the current study in WHO Y had not yet been described or related to an increase in the MIC of ESCs. Therefore, complementary experiments are needed to prove whether the mutation influences resistance to ESCs.
WHO Y also exhibited mutations in MtrC and MtrD. MtrD, together with membrane fusion (MtrC) and outer membrane channel (MtrE) proteins, exports antimicrobials out of the bacterial cell (Hagman et al., 1995; Delahay et al., 1997). Mutations in clinical isolates that cause resistance to various antimicrobials through this efflux pump are associated with changes in its repressor (mtrR) (Hagman et al., 1995; Zarantonelli et al., 1999; Unemo and Shafer, 2014). Such changes generally comprise adenine deletion in the mtrR promoter region (found here in ST1407 isolates) and the G45D amino acid change in MtrR (Hagman et al., 1995; Zarantonelli et al., 1999; Unemo and Shafer, 2014). In 2018, it was experimentally found that the presence of a series of mutations in the Mtr efflux pump was associated with azithromycin resistance (Wadsworth et al., 2018). Thus, similar to what was observed for penA, this mosaic-like structure was acquired from other Neisseria spp. (Johnson et al., 2003; McLean et al., 2004). The protein MtrD contains an antimicrobial binding site; in a recent study, it was shown that the amino acid M570 occurs at this site but is conserved in the Escherichia coli efflux system (Lyu et al., 2020). The mutations S23P (MtrC) and M570K (MtrD) founded in WHO Y are not seen in isolates with mtr mosaic (Rouquette-Loughlin et al., 2018; Wadsworth et al., 2018). Point mutations in PorB and MtrC/D, associated with mutations in WHO Y before induction, might have contributed to the increase in MIC.
WHO Y and M128 showed mutations in PilQ. M128 exhibited the G590D mutation, whereas WHO Y exhibited an insertion of guanine between nucleotides 1566 and 1567, which resulted in a frameshift. PilQ belongs to a family of outer membrane proteins known as secretins. PilQ is the only secretin in N. gonorrhoeae; it plays a role in the formation of channels or pores through which the pilus is externalized (Drake and Koomey, 1995; Tønjum et al., 1998). Specific mutations in PilQ (e.g., E666K mutation) can lead to altered permeability of antimicrobials such as penicillin and tetracycline but do not seem to affect the MIC of ESCs (Ropp et al., 2002; Zhao et al., 2005; Zhao et al., 2009; Unemo and Shafer, 2014). A frameshift mutation in pilQ was observed in vitro by Johnson et al. (2014) and found to result in a 10-fold increase in ceftriaxone MIC. Thus, the mutations in PilQ in WHO Y might have contributed to the increase in cefixime and ceftriaxone MICs. Given that the adhesion of gonococcus to epithelial cells is mediated by type IV pili, the expression of this protein is essential for N. gonorrhoeae pathogenesis. Thus, the frameshift in WHO Y might have transformed the isolate into non-competent for infection.
Interestingly, the MIC of cefixime for M107 and M110 increased 125-fold after induction, whereas that of ceftriaxone increased 31.25-fold. Although this study did not focus on mutations in intergenic regions, a search for changes in the mtrR promoter region was performed, and no mutations were found in M107 or M110. These isolates did not have mutations in the main ESC resistance determinants before induction. After induction, M110 acquired a mutation in ponA, which resulted in an A615V amino acid change in PBP1. In clinical isolates, the contribution of PBP1 to higher ESC MIC values was only observed when associated with other determinants, mtrR and penA, given that ESCs have a higher affinity for PBP2 (Zhao et al., 2009). Therefore, this change alone could not explain the increase in the MIC for M110. Gong et al. (2016) found that a mutation in the subunit of a cell division-related ABC transporter (ftsX) was responsible for an increase in ceftriaxone MIC. In M107 and M110, mutations were observed in zapA and ftsZ, respectively, two genes involved in cell division. ftsZ is part of a group of genes involved in both cell division and synthesis of peptidoglycan, which initiates cell division by forming a polymer called the Z ring. This structure gives rise to new bacterial cells (Ayala et al., 1994). ZapA is a regulator of ftsZ, which helps in the initial formation and posterior stabilization of the polymer (Low et al., 2004). Deletion of zapA in E. coli did not result in a phenotypic change but was shown to be lethal when FtsZ levels were reduced (Johnson et al., 2004). Thus, the frameshift observed in zapA in the M107 isolate, associated with selective environmental pressure of the antimicrobial, which inhibits cell wall formation, may favor bacterial division. As this mechanism has not been observed in clinical isolates circulating in the world, further studies are necessary to elucidate the mechanisms that caused resistance in these isolates. It is suggested that such mechanisms are more involved with resistance to cefixime than to ceftriaxone.
In 2020, a study identified different alleles of RNA polymerase associated with ceftriaxone resistance (Palace et al., 2020). They evidenced that clinical isolates of N. gonorrhoeae from different genetic backgrounds can acquire high-level resistance for ceftriaxone through RNA polymerase mutations. In this study, we observed that mutations in RNA polymerase (rpoB, rpoC, and rpoD) arose in six out of eight isolates postselecion, including isolates mosaic penA-34.001 and nonmosaic.
In the current study, we observed the ability of the antimicrobial cefixime to induce resistance in N. gonorrhoeae isolates in vitro. Although not currently used in Brazil, cefixime can serve as a marker of resistance, given that its MIC is generally higher than that of ceftriaxone. It was interesting to note that different STs acquired mutation resistance to ESCs after exposure to cefixime by different mechanisms. Isolates with penA mosaic were more likely to develop mutations in the penA gene itself. These findings reinforce the importance of the gonococcal antimicrobial resistance surveillance program in Brazil, given the changes in treatment recommendations in 2017, and the nationwide prevalence of STs that can acquire resistance to these antimicrobials.
The datasets presented in this study can be found in online repositories. The names of the repository/repositories and accession number(s) can be found below:
https://www.ncbi.nlm.nih.gov/, SAMN26195797,
https://www.ncbi.nlm.nih.gov/, SAMN26195798
https://www.ncbi.nlm.nih.gov/, SAMN26195799
https://www.ncbi.nlm.nih.gov/, SAMN26195800
https://www.ncbi.nlm.nih.gov/, SAMN26195801
https://www.ncbi.nlm.nih.gov/, SAMN26195802
https://www.ncbi.nlm.nih.gov/, SAMN26195803
https://www.ncbi.nlm.nih.gov/, SAMN26195804
https://www.ncbi.nlm.nih.gov/, SAMN26195805
https://www.ncbi.nlm.nih.gov/, SAMN26195806
https://www.ncbi.nlm.nih.gov/, SAMN26195807
https://www.ncbi.nlm.nih.gov/, SAMN26195808
https://www.ncbi.nlm.nih.gov/, SAMN26195809
https://www.ncbi.nlm.nih.gov/, SAMN26195810.
MAS and MB conceived and designed the study. MCS assisted with sample isolation and identification. MAS, FB, JM, HM, HG, and JP conducted lab experiments. MAS, DM, and JP carried out genome analysis. MRS assisted in bioinformatics analysis. MAS, JP, and MB interpreted the data and wrote the manuscript. MB supervised the study. All authors edited, commented, and approved the final version of the manuscript. All authors contributed to the article and approved the submitted version.
The present study was supported by the Ministry of Health of Brazil, through its Secretariat for Health Surveillance/Department of Diseases of Chronic Condition and Sexually Transmitted Infections.
We thank the Ministry of Health of Brazil and its Diseases of Chronic Condition and Sexually Transmitted Infections for offering support in N. gonorrhoeae isolate identification and MIC determination.
The authors declare that the research was conducted in the absence of any commercial or financial relationships that could be construed as a potential conflict of interest.
All claims expressed in this article are solely those of the authors and do not necessarily represent those of their affiliated organizations, or those of the publisher, the editors and the reviewers. Any product that may be evaluated in this article, or claim that may be made by its manufacturer, is not guaranteed or endorsed by the publisher.
The Supplementary Material for this article can be found online at: https://www.frontiersin.org/articles/10.3389/fcimb.2022.924764/full#supplementary-material
Allen, V. G., Mitterni, L., Seah, C., Rebbapragada, A., Martin, I. E., Lee, C., et al. (2013). Neisseria gonorrhoeae treatment failure and susceptibility to cefixime in Toronto, Canada. Am. Med. Assoc. 309, 163–170. doi: 10.1001/jama.2012.176575
Ameyama, S., Onodera, S., Takahata, M., Minami, S., Maki, N., Endo, K., et al. (2002). Mosaic-like structure of penicillin-binding protein 2 gene (penA) in clinical isolates of neisseria gonorrhoeae with reduced susceptibility to cefixime. Antimicrob Agents Chemother 46, 3744–3749. doi: 10.1128/AAC.46.12.3744-3749.2002
(2020) Brazilian Committee on antimicrobial susceptibility testing v. 8.0. Available at: http://brcast.org.br/.
Ayala, J. A., Garrido, T., de Pedro, M. A., Vicente, M. (1994). Molecular biology of bacterial septation. In Ghuysen, J. M., Hakenbeck, R. (ed.) New Comprehensive Biochemistry Elsevier 27, 73–101. doi: 10.1016/S0167-7306(08)60408-1
Bankevich, A., Nurk, S., Antipov, D., Gurevich, A. A., Dvorkin, M., Kulikov, A. S., et al. (2012). SPAdes: A new genome assembly algorithm and its applications to single-cell sequencing. J. Comput. Biol. 19, 455–477. doi: 10.1089/cmb.2012.0021
Barbee, L. A., Nayak, S. U., Blumer, J. L., O’Riordan, M. A., Gray, W., Zenilman, J. M., et al. (2018). A phase 1 pharmacokinetic and safety study of extended-duration, high-dose cefixime for cephalosporin-resistant neisseria gonorrhoeae in the pharynx. Sexually Transmitted Dis. 45, 677–683. doi: 10.1097/OLQ.0000000000000844
Bazzo, M. L., Golfetto, L., Gaspar, P. C., Pires, A. F., Ramos, M. C., Franchini, M., et al. (2018). First nationwide antimicrobial susceptibility surveillance for neisseria gonorrhoeae in Brazil, 2015-16. J. Antimicrob Chemother 73, 1854–1861. doi: 10.1093/jac/dky090
Bolger, A. M., Lohse, M., Usadel, B. (2014). Trimmomatic: A flexible trimmer for illumina sequence data. Bioinformatics 30, 2114–2120. doi: 10.1093/bioinformatics/btu170
Brasil (2015). Protocolo clínico e diretrizes terapêuticas (Brasília: Brasil: Atenção integral às pessoas com Infecções Sexualmente Transmissíveis), 119p. Available at: https://bvsms.saude.gov.br/bvs/publicacoes/protocolo_clinico_diretrizes_terapeutica_atencao_integral_pessoas_infeccoes_sexualmente_transmissiveis.pdf.
Brasil (2020). Protocolo clínico e diretrizes terapêuticas para atenção integral às pessoas com infecções sexualmente transmissíveis (Brasília: Brasil), 250p. Available at: http://www.aids.gov.br/pt-br/pub/2015/protocolo-clinico-e-diretrizes-terapeuticas-para-atencao-integral-pessoas-com-infeccoes.
Cámara, J., Serra, J., Ayats, J., Bastida, T., Carnicer-Pont, D., Andreu, A., et al. (2012). Molecular characterization of two high-level ceftriaxone-resistant neisseria gonorrhoeae isolates detected in Catalonia, Spain. J. Antimicrob Chemother 67, 1858–1860. doi: 10.1093/jac/dks162
Carver, T. J., Rutherford, K. M., Berriman, M., Rajandream, M. A., Barrell, B. G., Parkhill, J. (2005). ACT: The Artemis comparison tool. Bioinformatics 21, 3422–3423. doi: 10.1093/bioinformatics/bti553
Chisholm, S. A., Mouton, J. W., Lewis, D. A., Nichols, T., Ison, C. A., Livermore, D. M. (2010). Cephalosporin MIC creep among gonococci: Time for a pharmacodynamic rethink? J. Antimicrob Chemother 65, 2141–2148. doi: 10.1093/jac/dkq289
Cingolani, P., Platts, A., Wang, L. L., Coon, M., Nguyen, T., Wang, L., et al. (2012). A program for annotating and predicting the effects of single nucleotide polymorphisms, SnpEff: SNPs in the genome of drosophila melanogaster strain w1118; iso-2; iso-3. Fly (Austin) 6, 80–92. doi: 10.4161/fly.19695
CLSI (2019). M100 performance standards for antimicrobial susceptibility testing. J. Serv. Marketing 8, 1–320.
Delahay, R. M., Robertson, B. D., Balthazar, J. T., Shafer, W. M., Isonl, C. A. (1997). Involvement of the gonococcal MtrE protein in the resistance of neisseria gonorrhoeae to toxic hydrophobic agents. Microbiology 143, 2127–2133. doi: 10.1099/00221287-143-7-2127
Drake, S. L., Koomey, M. (1995). The product of the pilQ gene is essential for the biogenesis of type IV pill in neisseria gonorrhoeae. Molecular Microbiol. 18, 975–986. doi: 10.1111/j.1365-2958.1995.18050975.x
Ghuysen, J.-M. (1991). SERINE b-LACTAMASES AND PENICILLIN-BINDING PROTEINS. Annu. Rev. Microbiol. 45, 37–67. doi: 10.1146/annurev.mi.45.100191.000345
Gill, M. J., Simjee, S., Al-Hattawi, K., Robertson, B. D., Easmon, C. S. F., Ison, C. A. (1998). Gonococcal resistance to-lactams and tetracycline involves mutation in loop 3 of the porin encoded at the penB locus. Antimicrob. Agents Chemother. 42, 2799–2803. doi: 10.1128/AAC.42.11.2799
Golfetto, L. (2018). Caracterização molecular e determinação do perfil de resistência de isolados clínicos de neisseria gonorrhoeae circulantes na grande florianópolis: série histórica 2008-2016 ([Florianópolis (SC)]: Universidade Federal de Santa Catarina).
Golparian, D., Bazzo, M. L., Golfetto, L., Gaspar, P. C., Schörner, M. A., Benzaken, A. S., et al. (2020). Genomic epidemiology of neisseria gonorrhoeae elucidating the gonococcal antimicrobial resistance and lineages/sublineages across Brazil 2015–16. J. Antimicrob Chemother 75, 3163–3172. doi: 10.1093/jac/dkaa318
Golparian, D., Shafer, W. M., Ohnishi, M., Unemo, M. (2014). Importance of multidrug efflux pumps in the antimicrobial resistance property of clinical multidrug-resistant isolates of neisseria gonorrhoeae. Antimicrob Agents Chemother 58, 3556–3559. doi: 10.1128/AAC.00038-14
Gong, Z., Lai, W., Liu, M., Hua, Z., Sun, Y., Xu, Q., et al. (2016). Novel genes related to ceftriaxone resistance found among ceftriaxone-resistant neisseria gonorrhoeae strains selected in vitro. Antimicrob Agents Chemother 60, 2043–2051. doi: 10.1128/AAC.00149-15
Hagman, K. E., Pan, W., Spratt, B. G., Balthazar, J. T., Judd, R. C., Shafer, W. M. (1995). Resistance of neisseria gonorrhoeae to antimicrobial hydrophobic agents is modulated by the mtrRCDE efflux system. Microbiology 141, 611–622. doi: 10.1099/13500872-141-3-611
Hagman, K. E., Shafer, W. M. (1995). Transcriptional control of the mtr efflux system of neisseria gonorrhoeae. J. Bacteriol. 177, 4162–4165. doi: 10.1128/jb.177.14.4162-4165.1995
Ito, M., Deguchi, T., Mizutani, K. S., Yasuda, M., Yokoi, S., Ito, S. I., et al. (2005). Emergence and spread of neisseria gonorrhoeae clinical isolates harboring mosaic-like structure of penicillin-binding protein 2 in central Japan. Antimicrob Agents Chemother 49, 137–143. doi: 10.1128/AAC.49.1.137-143.2005
Johnson, S. R., Grad, Y., Ganakammal, S. R., Burroughs, M., Frace, M., Lipsitch, M., et al. (2014). In vitro selection of neisseria gonorrhoeae mutants with elevated MIC values and increased resistance to cephalosporins. Antimicrob Agents Chemother 58, 6986–6989. doi: 10.1128/AAC.03082-14
Johnson, J. E., Lackner, L. L., Hale, C. A., de Boer, P. A. J. (2004). ZipA is required for targeting of DMinC/DicB, but not DMinC/MinD, complexes to septal ring assemblies in escherichia coli. J. Bacteriol 186, 2418–2429. doi: 10.1128/JB.186.8.2418-2429.2004
Johnson, S. R., Sandul, A. L., Parekh, M., Wang, S. A., Knapp, J. S., Trees, D. L. (2003). Mutations causing in vitro resistance to azithromycin in neisseria gonorrhoeae. Int. J. Antimicrob Agents 21, 414–419. doi: 10.1016/S0924-8579(03)00039-6
Lewis, D. A. (2015). Will targeting oropharyngeal gonorrhoea delay the further emergence of drug-resistant neisseria gonorrhoeae strains? Sexually Transmitted Infect 91, 238–240. doi: 10.1136/sextrans-2014-051730
Li, H. (2011). A statistical framework for SNP calling, mutation discovery, association mapping and population genetical parameter estimation from sequencing data. Bioinformatics 27, 2987–2993. doi: 10.1093/bioinformatics/btr509
Li, H., Durbin, R. (2009). Fast and accurate short read alignment with burrows-wheeler transform. Bioinformatics 25, 1754–1760. doi: 10.1093/bioinformatics/btp324
Li, H., Handsaker, B., Wysoker, A., Fennell, T., Ruan, J., Homer, N., et al. (2009). The sequence Alignment/Map format and SAMtools. Bioinformatics 25, 2078–2079. doi: 10.1093/bioinformatics/btp352
Low, H. H., Moncrieffe, M. C., Löwe, J. (2004). The crystal structure of ZapA and its modulation of FtsZ polymerisation. J. Mol. Biol. 341, 839–852. doi: 10.1016/j.jmb.2004.05.031
Lyu, M., Moseng, M. A., Reimche, J. L., Holley, C. L., Dhulipala, V., Su, C.-C., et al. (2020). Cryo-EM structures of a gonococcal multidrug efflux pump illuminate a mechanism of drug recognition and resistance. mBio 11, 1–15. doi: 10.1128/mBio
Martin, I. M. C., Ison, C. A., Aanensen, D. M., Fenton, K. A., Spratt, B. G. (2004). Rapid sequence-based identification of gonococcal transmission clusters in a Large metropolitan area. J. Infect. Dis. 189, 1497–1505. doi: 10.1086/383047
McArthur, A. G., Waglechner, N., Nizam, F., Yan, A., Azad, M. A., Baylay, A. J., et al. (2013). The comprehensive antibiotic resistance database. Antimicrob Agents Chemother 57, 3348–3357. doi: 10.1128/AAC.00419-13
McLean, C. A., Wang, S. A., Hoff, G. L., Dennis, L. Y., Trees, D. L., Knapp, J. S., et al. (2004). The emergence of neisseria gonorrhoeae with decreased susceptibility to azithromycin in Kansas city, Missouri 1999 to 2000. Sexually Transmitted Dis. 31, 73–78. doi: 10.1097/01.OLQ.0000109514.91508.FC
Moherdaui, F., Vuylsteke, B., Siqueira, L. F. G., dos Santos, M. Q., Jr., Jardim, M. L., de Brito, A. M., et al. (1998). Validation of national algorithms for the diagnosis of sexually transmitted diseases in Brazil: results from a multicentre study. Sex Transm Inf 74, 38–43.
Murtagh, F., Legendre, P. (2014). Ward’s hierarchical agglomerative clustering method: Which algorithms implement ward’s criterion? J. Classification 31, 274–295. doi: 10.1007/s00357-014-9161-z
Ohneck, E. A., Zalucki, Y. M., Johnson, P. J. T., Dhulipala, V., Golparian, D., Unemo, M., et al. (2011). A novel mechanism of high-level, broad-spectrum antibiotic resistance caused by a single base pair change in neisseria gonorrhoeae. mBio 2, 1–8. doi: 10.1128/mBio.00187-11
Ohnishi, M., Saika, T., Hoshina, S., Iwasaku, K., Nakayama, S. I., Watanabe, H., et al. (2011). Ceftriaxone-resistant neisseria gonorrhoeae, Japan. Emerging Infect. Dis. 17, 148–149. doi: 10.3201/eid1701.100397
Olesky, M., Hobbs, M., Nicholas, R. A. (2002). Identification and analysis of amino acid mutations in porin IB that mediate intermediate-level resistance to penicillin and tetracycline in neisseria gonorrhoeae. Antimicrob Agents Chemother 46, 2811–2820. doi: 10.1128/AAC.46.9.2811-2820.2002
Olesky, M., Zhao, S., Rosenberg, R. L., Nicholas, R. A. (2006). Porin-mediated antibiotic resistance in neisseria gonorrhoeae: Ion, solute, and antibiotic permeation through PIB proteins with penB mutations. J. Bacteriol 188, 2300–2308. doi: 10.1128/JB.188.7.2300-2308.2006
Osaka, K., Takakura, T., Narukawa, K., Takahata, M., Endo, K., Kiyota, H., et al. (2008). Analysis of amino acid sequences of penicillin-binding protein 2 in clinical isolates of neisseria gonorrhoeae with reduced susceptibility to cefixime and ceftriaxone. J. Infect Chemother 14, 195–203. doi: 10.1007/s10156-008-0610-7
Palace, S. G., Wang, Y., Rubin, D. H. F., Welsh, M. A., Mortimer, T. D., et al. (2020). RNA Polymerase mutations cause cephalosporin resistance in clinical neisseria gonorrhoeae isolates. eLIFE 9, 1–22. doi: 10.7554/eLife.51407
Powell, A. J., Tomberg, J., Deacon, A. M., Nicholas, R. A., Davies, C. (2009). Crystal structures of penicillin-binding protein 2 from penicillin-susceptible and -resistant strains of neisseria gonorrhoeae reveal an unexpectedly subtle mechanism for antibiotic resistance. J. Biol. Chem. 284, 1202–1212. doi: 10.1074/jbc.M805761200
Ropp, P. A., Hu, M., Olesky, M., Nicholas, R. A. (2002). Mutations in ponA, the gene encoding penicillin-binding protein 1, and a novel locus, penC, are required for high-level chromosomally mediated penicillin resistance in neisseria gonorrhoeae. Antimicrob Agents Chemother 46, 769–777. doi: 10.1128/AAC.46.3.769-777.2002
Rouquette-Loughlin, C. E., Reimche, J. L., Balthazar, J. T., Dhulipala, V., Gernert, K. M., et al. (2018). Mechanistic basis for decreased antimicrobial susceptibility in a clinical isolate of neisseria gonorrhoeae possessing a mosaic-like mtr efflux pump locus. Mol. Biol. Physiol. 9, 1–15. doi: 10.1128/mBio.02281-18
Rowley, J., Vander Hoorn, S., Korenromp, E., Low, N., Unemo, M., Abu-Raddad, L. J., et al. (2019). Chlamydia, gonorrhoea, trichomoniasis and syphilis: Global prevalence and incidence estimate. Bull. World Health Organ 97, 548–562. doi: 10.2471/BLT.18.228486
Seemann, T. (2014). Prokka: Rapid prokaryotic genome annotation. Bioinformatics 30, 2068–2069. doi: 10.1093/bioinformatics/btu153
Shafer, W. M., Folster, J. P. (2006). Towards an understanding of chromosomally mediated penicillin resistance in neisseria gonorrhoeae: Evidence for a porin-efflux pump collaboration. J. Bacteriol 188, 2297–2299. doi: 10.1128/JB.188.7.2297-2299.2006
Tønjum, T., Caugant, D. A., Dunham, S. A., Koomey, M. (1998). Structure and function of repetitive sequence elements associated with a highly polymorphic domain of the neisseria meningitidis PilQ protein. Mol. Microbiol. 29, 111–124. doi: 10.1046/j.1365-2958.1998.00910.x
Tomberg, J., Unemo, M., Ohnishi, M., Davies, C., Nicholas, R. A. (2013). Identification of amino acids conferring high-level resistance to expanded-spectrum cephalosporins in the penA gene from neisseria gonorrhoeae strain H041. Antimicrob Agents Chemother 57, 3029–3036. doi: 10.1128/AAC.00093-13
Unemo, M. (2015). Current and future antimicrobial treatment of gonorrhoea - the rapidly evolving neisseria gonorrhoeae continues to challenge. BMC Infect. Dis. 15, 1–15. doi: 10.1186/s12879-015-1029-2
Unemo, M., Golparian, D., Nicholas, R., Ohnishi, M., Gallay, A., Sednaouie, P. (2012). High-level cefixime- and ceftriaxone-resistant neisseria gonorrhoeae in France: Novel penA mosaic allele in a successful international clone causes treatment failure. Antimicrob Agents Chemother 56, 1273–1280. doi: 10.1128/AAC.05760-11
Unemo, M., Golparian, D., Sánchez-Busó, L., Grad, Y., Jacobsson, S., Ohnishi, M., et al. (2016). The novel 2016 WHO neisseria gonorrhoeae reference strains for global quality assurance of laboratory investigations: Phenotypic, genetic and reference genome characterization. J. Antimicrob Chemother 71, 3096–3108. doi: 10.1093/jac/dkw288
Unemo, M., Golparian, D., Stary, A., Eigentler, A. (2011). First neisseria gonorrhoeae strain with resistance to cefixime causing gonorrhoea treatment failure in Austria 2011. Euro Surveillance 16, 1–3. doi: 10.2807/ese.16.43.19998-en
Unemo, M., Lahra, M. M., Cole, M., Galarza, P., Ndowa, F., Martin, I., et al. (2019). World health organization global gonococcal antimicrobial surveillance program (WHO GASP): Review of new data and evidence to inform international collaborative actions and research efforts. Sexual Health 16, 412–425. doi: 10.1071/SH19023
Unemo, M., Nicholas, R. A. (2012). Emergence of multidrug-resistant, extensively drug-resistant and untreatable gonorrhea. Future Microbiol. 7, 1401–1422. doi: 10.2217/fmb.12.117
Unemo, M., Ross, J. D. C., Serwin, A. B., Gomberg, M., Cusini, M., Jensen, J. S. (2020). 2020 European guideline for the diagnosis and treatment of gonorrhoea in adults. Int. J. STD AIDS. 0, 1–7. doi: 10.1177/0956462420949126
Unemo, M., Shafer, W. M. (2014). Antimicrobial resistance in neisseria gonorrhoeae in the 21st century: Past, evolution, and future. Clin. Microbiol. Rev. 27, 587–613. doi: 10.1128/CMR.00010-14
Vincent, L. R., Kerr, S. R., Tan, Y., Tomberg, J., Raterman, E. L., et al. (2018). In vivo-selected compensatory mutations restore the fitness cost of mosaic penA alleles that confer ceftriaxone resistance in neisseria gonorrhoeae. mBio 9, 1–18. doi: 10.1128/mBio.01905-17
Wadsworth, C. B., Arnold, B. J., Sater, M. R. A., Grad, Y. H. (2018). Azithromycin resistance through interspecific acquisition of an epistasis-dependent efflux pump component and transcriptional regulator in neisseria gonorrhoeae. mBio 9, 1–15. doi: 10.1128/mBio.01419-18
Zarantonelli, L., Borthagaray, G., Lee, E.-H., Shafer, W. M. (1999). Decreased azithromycin susceptibility of neisseria gonorrhoeae due to mtrR mutations. Antimicrob Agents Chemother 43, 2468–2472. doi: 10.1128/AAC.43.10.2468
Zhao, S., Duncan, M., Tomberg, J., Davies, C., Unemo, M., Nicholas, R. A. (2009). Genetics of chromosomally mediated intermediate resistance to ceftriaxone and cefixime in neisseria gonorrhoeae. Antimicrob Agents Chemother 53, 3744–3751. doi: 10.1128/AAC.00304-09
Zhao, S., Tobiason, D. M., Hu, M., Seifert, H. S., Nicholas, R. A. (2005). The penC mutation conferring antibiotic resistance in neisseria gonorrhoeae arises from a mutation in the PilQ secretin that interferes with multimer stability. Mol. Microbiol. 57, 1238–1251. doi: 10.1111/j.1365-2958.2005.04752.x
Keywords: SNP, whole-genome sequencing, PBP2, penA, resistance induction, mutations
Citation: Schörner MA, Mesa D, Barazzetti FH, Martins JM, Machado HdM, Grisard HBdS, Wachter JK, Starick MR, Scheffer MC, Palmeiro JK and Bazzo ML (2022) In vitro selection of Neisseria gonorrhoeae unveils novel mutations associated with extended-spectrum cephalosporin resistance. Front. Cell. Infect. Microbiol. 12:924764. doi: 10.3389/fcimb.2022.924764
Received: 20 April 2022; Accepted: 30 June 2022;
Published: 27 July 2022.
Edited by:
Lucia Martins Teixeira, Federal University of Rio de Janeiro, BrazilReviewed by:
Robert A Nicholas, University of North Carolina at Chapel Hill, United StatesCopyright © 2022 Schörner, Mesa, Barazzetti, Martins, Machado, Grisard, Wachter, Starick, Scheffer, Palmeiro and Bazzo. This is an open-access article distributed under the terms of the Creative Commons Attribution License (CC BY). The use, distribution or reproduction in other forums is permitted, provided the original author(s) and the copyright owner(s) are credited and that the original publication in this journal is cited, in accordance with accepted academic practice. No use, distribution or reproduction is permitted which does not comply with these terms.
*Correspondence: Marcos André Schörner, bWFyY29zLmFuZHJlLnNjaG9ybmVyQHBvc2dyYWQudWZzYy5icg==
Disclaimer: All claims expressed in this article are solely those of the authors and do not necessarily represent those of their affiliated organizations, or those of the publisher, the editors and the reviewers. Any product that may be evaluated in this article or claim that may be made by its manufacturer is not guaranteed or endorsed by the publisher.
Research integrity at Frontiers
Learn more about the work of our research integrity team to safeguard the quality of each article we publish.