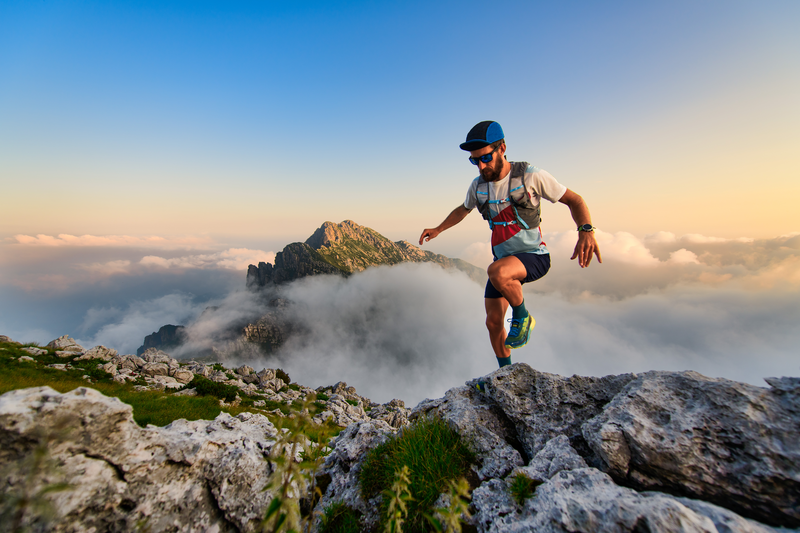
94% of researchers rate our articles as excellent or good
Learn more about the work of our research integrity team to safeguard the quality of each article we publish.
Find out more
ORIGINAL RESEARCH article
Front. Cell. Infect. Microbiol. , 29 April 2022
Sec. Fungal Pathogenesis
Volume 12 - 2022 | https://doi.org/10.3389/fcimb.2022.892893
This article is part of the Research Topic Alternative Approaches to Antifungal Drugs Against Drug-resistant Fungi View all 5 articles
Interactions of isavuconazole and colistin were evaluated against 57 common Candida strains belonging to the species Candida albicans (n = 10), Candida glabrata (n = 10), Candida kefyr (n = 8), Candida krusei (n = 10), Candida parapsilosis (n = 9), and Candida tropicalis (n = 10) by a broth microdilution checkerboard technique based on the European Committee on Antimicrobial Susceptibility Testing (EUCAST) reference methodology for antifungal susceptibility testing. Results were analyzed with the fractional inhibitory concentration index and by the response surface analysis. Interpretation by the fractional inhibitory concentration index showed synergy for 50%, 80%, 90%, and 90% of the C. kefyr, C. krusei, C. glabrata, and C. tropicalis strains, respectively. Combination of isavuconazole with colistin against C. albicans and C. parapsilosis exhibited only indifference for 100% and 90% of the strains, respectively. The results were confirmed by response surface analysis for all species except for C. glabrata, for which an indifferent interaction was found for the majority of strains. Antagonistic interaction was never seen regardless of the interpretation model was used.
Candidemia is a severe and life-threatening infection caused by different Candida species. In Germany, candidemia ranks as the 6th most common bloodstream infection after Escherichia coli, Staphylococcus aureus, coagulase-negative staphylococci, Klebsiella pneumoniae, and enterococci (Schöneweck et al., 2021). From the bloodstream, Candida can disseminate to multiple organs involving most commonly the liver, spleen, kidney, myocardium, or eyes and less frequently the brain (Pilmis et al., 2017). Invasive candidiasis is associated with a high mortality rate of about 40%. While many different Candida species can be responsible for invasive diseases, 95% of the infections are caused by the five species Candida albicans, Candida glabrata, Candida krusei, Candida parapsilosis, and Candida tropicalis (Yapar, 2014). Although C. albicans is still responsible for the majority of infections in Germany (Mohr et al., 2020; Schroeder et al., 2020), non-albicans Candida species can represent up to 60% of the cases of candidemia in other parts of the world like France (Vannini et al., 2022), Italy (Montagna et al., 2013), or the United States (Pfaller et al., 2012). Compiled data from the ARTEMIS DISK registry including data from 147 medical centers in 41 countries all over the world for the years 1997–2007 indicated that despite a decrease in frequency, C. albicans remained the most frequent species worldwide, while the frequency of C. glabrata and C. krusei was stable, and the frequency of C. parapsilosis and C. tropicalis increased (Pfaller et al., 2010). Echinocandins are recommended as first-line therapies for invasive candidiasis, and azoles can be used, as step-down therapies (Cornely et al., 2012), but decreased susceptibility and even resistance to antifungals can occur. Decreased susceptibility or drug resistance in Candida species can be intrinsic, like decreased caspofungin susceptibility in C. parapsilosis (Pfaller et al., 2006) or fluconazole resistance in C. krusei (Orozco et al., 1998), or can be acquired like echinocandin resistance in C. glabrata (Aldejohann et al., 2021). The high mortality rate, the shift towards more difficult to treat Candida species, and the lack of efficacy in monotherapy for some difficult-to-treat infections make alternative approaches necessary (Vitale, 2021). It has been shown in vitro (Schwarz et al., 2007), in vivo (Schwarz et al., 2006b), and in patients (Day et al., 2013) that a combination of two antifungals can increase their potency and decrease resistance. However, against invasive candidiasis, no favorable combination has been found (Johnson et al., 2004). Apart from the combination of two antifungals, combinations of antifungal and other molecules can also lead to favorable interactions (Schwarz et al., 2019). The antibiotic colistin has shown in vitro synergy in combination with amphotericin B against Candida species (Schwarz et al., 2022), but routine use in critically ill patients is limited due to the nephrotoxicity of the molecules. Isavuconazole is a broad-spectrum azole with favorable tolerability (Ledoux et al., 2018), which makes it an interesting partner for combinations of molecules. In vitro combination of isavuconazole with colistin has been shown to be synergistic against Aspergillus nidulans, Aspergillus niger (Schwarz et al., 2020b), and Candida auris (Schwarz et al., 2020a) and could thus be also an interesting combination against Candida species. We therefore explored the combination of isavuconazole with colistin against common Candida species by a checkerboard technique based on the European Committee on Antimicrobial Susceptibility Testing (EUCAST) methodology for antifungal susceptibility testing.
In this study, a total of 57 clinical Candida strains belonging to 6 common Candida species were included. Candida species included 10 C. albicans, 10 C. glabrata (teleomorph in the Nakaseomyces clade), 8 Candida kefyr (teleomorph Kluyveromyces marxianus), 10 C. krusei (teleomorph Pichia kudriavzevii), 9 C. parapsilosis, and 10 C. tropicalis. Candida strains were mainly obtained from the Department of Microbiology of the University Hospital Marburg (n = 53). Additional isolates (n = 4) belonged to the collections of the American Type Culture Collection (ATCC) and the Deutsche Sammlung von Mikroorganismen und Zellkulturen (DSM). The complete Internal Transcribed Spacer (ITS)1-5.8S-ITS2 region of the non-collection strains was sequenced as described elsewhere (Schwarz et al., 2006a) to obtain molecular identification of the strains to the species level. Sequences were deposited at GenBank under the accession numbers OL351325 to OL351356 (Schwarz et al., 2022) and under OM859334 to OM859357. Only C. krusei strain U2106778 was identified by matrix-assisted laser desorption ionization–time of flight (MALDI-TOF) due to ITS region sequence heterogeneity (Zhao et al., 2015). C. krusei ATCC 6258 and C. parapsilosis ATCC 22019 were used as quality controls in each batch of microplates.
Isavuconazole (Pfizer, Berlin, Germany) stock solution was prepared at 3,200 µg/ml in dimethyl sulfoxide (DMSO). A stock solution of colistin (Merck, Darmstadt, Germany) at 12,800 µg/ml was prepared in sterile, distilled water. Stock solutions were kept at −25°C until use.
For this study, Roswell Park Memorial Institute 1640 (RPMI) medium (with L-glutamine, with pH indicator, but without bicarbonate) (Merck) prepared in double strength was used as the test medium. It contained 2% (w/v) of D-glucose and was buffered with 3-(N-morpholino)propanesulfonic acid (Merck) at a final concentration of 0.165 mol/L. The final pH of 7.0 was adjusted with 2 molar NaOH. The medium was sterilized through a 0.22-µm pore size filter by vacuum filtration (Merck).
An antifungal susceptibility testing protocol, modified for broth microdilution checkerboard procedures, based on the EUCAST guidelines was used in this study (Arendrup et al., 2020). Combination experiments were carried out in Nunclon™ delta surface 96-well microtiter plates for adherent cells (Thermo Fisher Scientific, Darmstadt, Germany). The combination of isavuconazole with colistin was studied on a two-dimensional checkerboard (Bidaud et al., 2021). Twofold serial dilutions of each drug were done in the double strength test medium. Final concentrations for isavuconazole ranged from 0.0001 to 0.03 µg/ml for C. albicans, C. kefyr, C. parapsilosis, and C. tropicalis or 0.002–0.5 µg/ml for C. glabrata and C. krusei. Colistin concentrations ranged from 1 to 64 µg/ml for all tested species. Before the addition of the inoculum, each well contained 100 µl of double-strength RPMI medium with 1% (v/v) of DMSO.
Before the experiments, Candida strains were cultured on Sabouraud dextrose agar slants supplemented with chloramphenicol and gentamicin (Bio-Rad Laboratories, Feldkirchen, Germany) at 35°C and 95% humidity for 24 h. By using inoculation loops, fungi were transferred from the agar slants to sterile tubes containing pure water. After the cells were counted in a hemocytometer, the suspensions were adjusted to the final inoculum size of 2 × 105 colony forming units (CFU)/ml. One hundred microliters of the inoculum was distributed into each well of the microplates using Eppendorf Xplorer plus (Eppendorf, Hamburg, Germany) electric multichannel pipettes. After incubation at 35°C and 95% humidity for 24 h, optical densities were read spectrophotometrically at a wavelength of 530 nm using a MultiSkan FC spectrometer (Thermo Fisher Scientific). Before the reading, microplates were shaken for 2 min at 1,100 rpm with a PMS-1000 Microplate Shaker (Grant Instruments, Shepreth, UK). Before the analysis of the results, the optical density values of a blank plate, each well inoculated with 100 µl of sterile distilled water and incubated under the same conditions as mentioned above, were subtracted from the values of the microplates inoculated with yeast cells. The final inoculum was further diluted at 1:10, and 50 µl were spread once on Sabouraud dextrose agar plates with a sterile Drigalski spatula. After 24 h of incubation, CFU were counted to ensure inoculum size. Combination experiments were run in duplicate.
Optical density values from the microplates were transformed into a percentage of growth compared to the growth control. Fifty percent of inhibition was chosen as an endpoint for the determination of the minimum inhibitory concentrations (MICs) alone for both drugs and in combination. High off-scale MICs were converted to the next log2 dilution. If the lowest fractional inhibitory concentration index (FICI) on the microplate was ≤0.5 or >0.5 to 4, synergy or indifference was assumed, respectively. For FICIs higher than 4.0, antagonism was concluded (Odds, 2003).
Compared to the FICI, response surface analysis allows the evaluation of drug interactions without using an inhibition endpoint and is therefore independent of MICs. It enables the determination (and visualization) of the interaction for all tested concentrations and not only for the MICs in combination. Based on the growth rates in the wells of the molecules alone (in this study isavuconazole and colistin), dose–response curves for the drugs alone are generated. According to the chosen theoretical model, using the dose–response curves of the two drugs alone, an indifferent dose–response surface is calculated. In this study, the Bliss independence model was chosen, which is based on the hypothesis that drugs act independently from each other. To determine the interaction of the molecules, the (experimentally) observed combination dose–response surface is compared to the predicted (calculated) indifferent dose–response surface. A combination effect is defined as synergistic if the observed effect lies below the predicted indifferent dose–response surface (corresponds to less growth on the microplate and a greater effect of the combination) and antagonistic when the observed effect lies above (corresponds to more growth on the microplate and a weaker effect of the combination). To quantitatively assess the interaction of the drugs, the SUM-SYN-ANT metric is calculated, which is defined as the sum of all effects greater than the predicted indifferent effect (SYN-SUM), minus the sum of all effects weaker than the predicted indifferent effect (ANT-SUM). The intrinsic variability of the broth microdilution technique necessitates the definition of a threshold, for which the interaction of the two drugs is defined as indifferent. This threshold is determined experimentally by combining the active molecules with themselves. Therefore, the combination of isavuconazole with itself was tested on the two-dimensional checkerboard with twofold serial dilutions as described above. The highest concentration of isavuconazole was 0.12 µg/ml in the x- and y-axes. For the determination of the threshold, C. krusei ATCC 6258 was tested in triplicate on these plates. Based on the results of the experimental plates, synergy was assumed when the SUM-SYN-ANT was ≥56.0%, and antagonism was assumed when ≤−56.0%. Between −56.0% and 56.0%, indifference was concluded.
To determine the SUM-SYN-ANT metric of the different tested strains, the results of both runs were combined. All calculations were done by the Combenefit software (http://sourceforge.net/projects/combenefit/) (Di Veroli et al., 2016).
The interactions of isavuconazole with colistin were evaluated by checkerboard and interpretation of the results by FICI or by response surface analysis against strains from six common Candida species as presented in Table 1. A summary of the results is presented in Table 2. Figure 1 shows the synergy distributions for the combination of isavuconazole with colistin against a representative isolate of each tested species.
Table 1 Interaction of isavuconazole with colistin against common Candida species by checkerboard and interpretation by fractional inhibitory concentration index and response surface analysis.
Table 2 Summary of the in vitro interactions of isavuconazole with colistin against common Candida species evaluated by EUCAST broth microdilution checkerboard methodology and interpretation by fractional inhibitory concentration index and response surface analysis.
Figure 1 Synergy distribution for the combination of isavuconazole with colistin against Candida albicans V2105529, Candida glabrata U2106602, Candida kefyr V2108462, Candida krusei N2102435, Candida parapsilosis V2105056, and Candida tropicalis N2102715. The mode of interaction was defined based on the SUM-SYN-ANT metric. IND, indifference; SYN, synergy.
The 57 Candida strains exhibited MICs for isavuconazole ranging from 0.0001 to 0.5 μg/ml (Table 1) with MIC50, MIC90, and geometric mean MIC of 0.008, 0.125, and 0.018 μg/ml, respectively. Isavuconazole MICs ranged from 0.0005 to 0.004, 0.125 to 0.5, 0.03 to 0.25, 0.008 to 0.03, 0.008 to 0.06, and 0.0001 to 0.008 µg/ml for C. albicans, C. glabrata, C. krusei, C. parapsilosis, C. tropicalis, and C. kefyr, respectively. When tested alone, colistin exhibited MICs ranging from 16 to 128 µg/ml for the different species (128 μg/ml being the high-off scale MIC) with MIC50, MIC90, and geometric mean MIC of 64, 128, and 63.23 μg/ml, respectively. The best activity of colistin was seen against C. tropicalis with MICs ranging from 16 to 32 µg/ml. Significant activity was also seen for C. kefyr with a geometric mean MIC of 32 µg/ml. Against C. krusei, colistin MICs were high with a geometric mean MIC of 59.71 µg/ml. Colistin showed almost no activity against C. albicans, C. glabrata, and C. parapsilosis with geometric mean MICs of 119.43, 119.43, and 109.73 µg/ml, respectively. The geometric mean MIC for colistin in combination with the synergistic strains was 9.93 µg/ml. Between experiments, isavuconazole and colistin MICs were within ±1 log2 dilutions in 99.12% of the cases for all Candida species tested (data not shown). The interaction was synergistic for 58% of the strains with FICIs ranging from 0.25 to 0.5 with a geometric mean FICI of 0.4. Synergy was obtained for 0%, 33%, 50%, 80%, 90%, and 90% of C. albicans, C. parapsilosis, C. kefyr, C. krusei, C. glabrata, and C. tropicalis, respectively (Table 2). All other interactions were indifferent. The geometric mean FICI for all strains was 0.48.
Analysis of the checkerboard data of the 57 Candida strains by the response surface approach led to similar results compared to the FICI results. Although synergy was less frequently obtained than by FICI analysis, the tendency if the combination is synergistic, or for a certain species indifferent (at least 50% of the strains for both interpretation models), was correct in 5/6 cases (Table 2). The SUM-SYN-ANT metric for the synergistic strains ranged from 59.37 to 122.67, with a mean of 78.22. For C. glabrata, the mean of the SUM-SYN-ANT metric of all strains was 40.42. Synergy was obtained for 0%, 0%, 10%, 50%, 50%, and 67% of C. albicans, C. parapsilosis, C. glabrata, C. krusei, C. tropicalis, and C. kefyr, respectively. When comparing the results of the FICI with the response surface approach, synergy was obtained for at least 50% of the strains for both interpretation models for C. kefyr, C. krusei, and C. tropicalis. One difference between the interpretation techniques was that interaction against C. glabrata was synergistic (9 of 10 strains) by FICI and indifferent by response surface analysis (9 of 10 strains).
Advantages of drug repurposing are the use of de‐risked compounds, potentially lower development costs, and shorter development timelines (Pushpakom et al., 2019). Repurposed drugs against Candida belong to the antihelmintics (quinacrine), cytotoxic agents (doxorubicin and daunorubicin), or 5α-reductase inhibitors (finasteride) and inhibit filamentation (Miro-Canturri et al., 2019). Colistin is an antibiotic with activity against gram-negative bacteria (Lim et al., 2010), but it has been shown to be able to damage the membrane in C. albicans (Yousfi et al., 2019) and increase its permeability (Bibi et al., 2021), which makes the molecule an interesting partner to test combinations with antifungals.
Compared to other studies, the MICs of isavuconazole for the different Candida species in this study were in the same range as previously reported (Desnos-Ollivier et al., 2019; Jorgensen et al., 2019). MICs for colistin were comparable to those of another study, but apart from C. albicans, only one strain of each species has been tested (Zeidler et al., 2013). Compared to our recently published study on the combination of amphotericin B and colistin, colistin MICs were similar (Schwarz et al., 2022).
Combination MICs of colistin ranged from 1 to 32 μg/ml, with a geometric mean MIC for the synergistic strains of 9.93 μg/ml. Peak serum levels of 13 to 32 have been reported in patients with cystic fibrosis (Reed et al., 2001), which would be sufficient against the strains tested in this study, but colistin use in patients is limited due to its nephrotoxicity (Javan et al., 2015). However, in patients with cryptococcosis, it has been shown that synergy can be achieved with lower serum levels than those tested in vitro (Kontoyiannis et al., 2008).
Against common Candida species, in vitro synergy of combinations including colistin has been reported for echinocandins (Zeidler et al., 2013) and for amphotericin B (Schwarz et al., 2022). Combinations of colistin with azoles were evaluated by two studies. One study found indifference for the combinations of colistin with either fluconazole or itraconazole against C. albicans, but both combinations were only tested against one strain (Yousfi et al., 2019). Our study is in accordance with these results. We found indifference for the combination of isavuconazole with colistin by checkerboard and interpretation of the results by FICI and response surface analysis. Another study also evaluated the combination of colistin with fluconazole by checkerboard against one C. albicans strain and found synergy in vitro and in vivo in a Galleria mellonella model of invasive candidiasis (Bibi et al., 2021). This discrepancy compared to our results might be strain specific, as only one strain was tested or might be related to the different azole used.
For the other species tested in this study, we found synergy for the majority, or at least for half of the tested strains, for C. glabrata, C. krusei, C. tropicalis, and C. kefyr. Only C. parapsilosis indifference was found for the majority of the strains. Although synergy was less frequently seen, when the checkboard data were evaluated by response surface analysis, at least 50% of the strains exhibited synergy regardless of the interpretation model used for C. kefyr, C. krusei, and C. tropicalis. For C. parapsilosis, both interpretation models evaluated indifference. Between the two interpretation techniques, one difference was found for C. glabrata. Interpretation by the FICI showed synergy for 90% of the strains, while interpretation by response surface analysis exhibited indifference for 90% of the strains. Nevertheless, despite the formal indifference of the C. glabrata results by response surface analysis, the mean of the SUM-SYN-ANT metric of all strains was quite high (40.42). The discrepancy could indeed be related to the stringent threshold (56.0) used in the present work compared to previous studies (Schwarz et al., 2020a; Schwarz et al., 2022).
In conclusion, we found in vitro synergy of the combination of isavuconazole with colistin against C. glabrata, C. krusei, C. tropicalis, and C. kefyr. Against C. albicans and C. parapsilosis, the combination only exhibited indifference. Antagonism was never seen regardless of interpretation model used. These results warrant further animal experiments.
The datasets presented in this study can be found in online repositories. The names of the repository/repositories and accession number(s) can be found below: https://www.ncbi.nlm.nih.gov/genbank/, OM859334 to OM859357 https://www.ncbi.nlm.nih.gov/genbank/, OL351325 to OL351356.
PS wrote the first draft of the manuscript. PS and IN carried out the experiments. PS, IN, A-LB, and ED performed the analysis of the results. ED, PS, GB, and FS contributed to the revisions. All authors have read and agreed to publish the final version of the manuscript.
Open Access funding provided by the Open Acess Publication Fund of Philipps-Universität Marburg with support of the Deutsche Forschungsgemeinschaft (DFG, German Research Foundation). This study was supported by internal funding.
PS has received research grants from Basilea Pharmaceutica, Gilead, and Pfizer; travel grants from Gilead and Pfizer; and speaking fees from Pfizer. During the past 5 years, ED has received research grants from MSD and Gilead; travel grants from Gilead, MSD, Pfizer, and Astellas; and speaker’s fees from Gilead, MSD, and Astellas.
The remaining authors declare that the research was conducted in the absence of any commercial or financial relationships that could be construed as a potential conflict of interest.
All claims expressed in this article are solely those of the authors and do not necessarily represent those of their affiliated organizations, or those of the publisher, the editors and the reviewers. Any product that may be evaluated in this article, or claim that may be made by its manufacturer, is not guaranteed or endorsed by the publisher.
Aldejohann, A. M., Herz, M., Martin, R., Walther, G., Kurzai, O. (2021). Emergence of Resistant Candida Glabrata in Germany. JAC Antimicrob. Resist. 3 (3), dlab122. doi: 10.1093/jacamr/dlab122
Arendrup, M. C., Meletiadis, J., Mouton, J. W., Lagrou, K., Hamal, P., Guinea, J. (2020). Method for the Determination of Broth Dilution Minimum Inhibitory Concentrations of Antifungal Agents for Yeasts (EUCAST Definitive Document E.Def 7.3.2), Copenhagen, Denmark.
Bibi, M., Murphy, S., Benhamou, R. I., Rosenberg, A., Ulman, A., Bicanic, T., et al. (2021). Combining Colistin and Fluconazole Synergistically Increases Fungal Membrane Permeability and Antifungal Cidality. ACS Infect. Dis. 7 (2), 377–389. doi: 10.1021/acsinfecdis.0c00721
Bidaud, A. L., Schwarz, P., Herbreteau, G., Dannaoui, E. (2021). Techniques for the Assessment of In Vitro and In Vivo Antifungal Combinations. J. Fungi (Basel) 7 (2), 113. doi: 10.3390/jof7020113
Cornely, O. A., Bassetti, M., Calandra, T., Garbino, J., Kullberg, B. J., Lortholary, O., et al. (2012). ESCMID Guideline for the Diagnosis and Management of Candida Diseases 2012: non-Neutropenic Adult Patients. Clin. Microbiol. Infect. 18 Suppl 7, 19–37. doi: 10.1111/1469-0691.12039
Day, J. N., Chau, T. T. H., Wolbers, M., Mai, P. P., Dung, N. T., Mai, N. H., et al. (2013). Combination Antifungal Therapy for Cryptococcal Meningitis. N Engl. J. Med. 368 (14), 1291–1302. doi: 10.1056/NEJMoa1110404
Desnos-Ollivier, M., Bretagne, S., Boullie, A., Gautier, C., Dromer, F., Lortholary, O., et al. (2019). Isavuconazole MIC Distribution of 29 Yeast Species Responsible for Invasive Infections, (2015-2017). Clin. Microbiol. Infect. 25 (5), 634.e631–634.e634. doi: 10.1016/j.cmi.2019.02.007
Di Veroli, G. Y., Fornari, C., Wang, D., Mollard, S., Bramhall, J. L., Richards, F. M., et al. (2016). Combenefit: An Interactive Platform for the Analysis and Visualization of Drug Combinations. Bioinformatics 32 (18), 2866–2868. doi: 10.1093/bioinformatics/btw230
Javan, A. O., Shokouhi, S., Sahraei, Z. (2015). A Review on Colistin Nephrotoxicity. Eur. J. Clin. Pharmacol. 71 (7), 801–810. doi: 10.1007/s00228-015-1865-4
Johnson, M. D., MacDougall, C., Ostrosky-Zeichner, L., Perfect, J. R., Rex, J. H. (2004). Combination Antifungal Therapy. Antimicrob. Agents Chemother. 48 (3), 693–715. doi: 10.1128/aac.48.3.693-715.2004
Jorgensen, K. M., Astvad, K. M. T., Hare, R. K., Arendrup, M. C. (2019). EUCAST Susceptibility Testing of Isavuconazole: MIC Data for Contemporary Clinical Mold and Yeast Isolates. Antimicrob. Agents Chemother. 63 (6):e00073-19. doi: 10.1128/AAC.00073-19
Kontoyiannis, D. P., Lewis, R. E., Alexander, B. D., Lortholary, O., Dromer, F., Gupta, K. L., et al. (2008). Calcineurin Inhibitor Agents Interact Synergistically With Antifungal Agents In Vitro Against Cryptococcus Neoformans Isolates: Correlation With Outcome in Solid Organ Transplant Recipients With Cryptococcosis. Antimicrob. Agents Chemother. 52 (2), 735–738. doi: 10.1128/AAC.00990-07
Ledoux, M. P., Denis, J., Nivoix, Y., Herbrecht, R. (2018). Isavuconazole: A New Broad-Spectrum Azole. Part 2: Pharmacokinetics and Clinical Activity. J. Mycol. Med. 28 (1), 15–22. doi: 10.1016/j.mycmed.2018.02.002
Lim, L. M., Ly, N., Anderson, D., Yang, J. C., Macander, L., Jarkowski, A., 3rd, et al. (2010). Resurgence of Colistin: A Review of Resistance, Toxicity, Pharmacodynamics, and Dosing. Pharmacotherapy 30 (12), 1279–1291. doi: 10.1592/phco.30.12.1279
Miro-Canturri, A., Ayerbe-Algaba, R., Smani, Y. (2019). Drug Repurposing for the Treatment of Bacterial and Fungal Infections. Front. Microbiol. 10. doi: 10.3389/fmicb.2019.00041
Mohr, A., Simon, M., Joha, T., Hanses, F., Salzberger, B., Hitzenbichler, F. (2020). Epidemiology of Candidemia and Impact of Infectious Disease Consultation on Survival and Care. Infection 48 (2), 275–284. doi: 10.1007/s15010-020-01393-9
Montagna, M. T., Caggiano, G., Lovero, G., De Giglio, O., Coretti, C., Cuna, T., et al. (2013). Epidemiology of Invasive Fungal Infections in the Intensive Care Unit: Results of a Multicenter Italian Survey (AURORA Project). Infection 41 (3), 645–653. doi: 10.1007/s15010-013-0432-0
Odds, F. C. (2003). Synergy, Antagonism, and What the Chequerboard Puts Between Them. J. Antimicrob. Chemother. 52 (1), 1. doi: 10.1093/jac/dkg301
Orozco, A. S., Higginbotham, L. M., Hitchcock, C. A., Parkinson, T., Falconer, D., Ibrahim, A. S., et al. (1998). Mechanism of Fluconazole Resistance in Candida Krusei. Antimicrob. Agents Chemother. 42 (10), 2645–2649. doi: 10.1128/AAC.42.10.2645
Pfaller, M. A., Boyken, L., Hollis, R. J., Messer, S. A., Tendolkar, S., Diekema, D. J. (2006). In Vitro Susceptibilities of Candida spp. To Caspofungin: Four Years of Global Surveillance. J. Clin. Microbiol. 44 (3), 760–763. doi: 10.1128/JCM.44.3.760-763.2006
Pfaller, M. A., Diekema, D. J., Gibbs, D. L., Newell, V. A., Ellis, D., Tullio, V., et al. (2010). Results From the ARTEMIS DISK Global Antifungal Surveillance Study 1997 to 2007: A 10.5-Year Analysis of Susceptibilities of Candida Species to Fluconazole and Voriconazole as Determined by CLSI Standardized Disk Diffusion. J. Clin. Microbiol. 48 (4), 1366–1377. doi: 10.1128/JCM.02117-09
Pfaller, M., Neofytos, D., Diekema, D., Azie, N., Meier-Kriesche, H. U., Quan, S. P., et al. (2012). Epidemiology and Outcomes of Candidemia in 3648 Patients: Data From the Prospective Antifungal Therapy (PATH Alliance(R)) Registry 2004-2008. Diagn. Microbiol. Infect. Dis. 74 (4), 323–331. doi: 10.1016/j.diagmicrobio.2012.10.003
Pilmis, B., Yang, Z., Lanternier, F., Lortholary, O. (2017). “Systemic Candidiasis,” in Infectious Diseases. Eds. Cohen, J., Powderly, W. G., Opal, S. M. (Amsterdam: Elsevier), 439–445.
Pushpakom, S., Iorio, F., Eyers, P. A., Escott, K. J., Hopper, S., Wells, A., et al. (2019). Drug Repurposing: Progress, Challenges and Recommendations. Nat. Rev. Drug Discovery 18 (1), 41–58. doi: 10.1038/nrd.2018.168
Reed, M. D., Stern, R. C., O'Riordan, M. A., Blumer, J. L. (2001). The Pharmacokinetics of Colistin in Patients With Cystic Fibrosis. J. Clin. Pharmacol. 41 (6), 645–654. doi: 10.1177/00912700122010537
Schöneweck, F., Schmitz, R. P. H., Rissner, F., Scherag, A., Loffler, B., Pletz, M. W., et al. (2021). The Epidemiology of Bloodstream Infections and Antimicrobial Susceptibility Patterns in Thuringia, Germany: A Five-Year Prospective, State-Wide Surveillance Study (AlertsNet). Antimicrob. Resist. Infect. Control 10 (1), 132. doi: 10.1186/s13756-021-00997-6
Schroeder, M., Weber, T., Denker, T., Winterland, S., Wichmann, D., Rohde, H., et al. (2020). Epidemiology, Clinical Characteristics, and Outcome of Candidemia in Critically Ill Patients in Germany: A Single-Center Retrospective 10-Year Analysis. Ann. Intensive Care 10 (1), 142. doi: 10.1186/s13613-020-00755-8
Schwarz, P., Bidaud, A. L., Dannaoui, E. (2020a). In Vitro Synergy of Isavuconazole in Combination With Colistin Against Candida Auris. Sci. Rep. 10 (1), 21448. doi: 10.1038/s41598-020-78588-5
Schwarz, P., Bretagne, S., Gantier, J. C., Garcia-Hermoso, D., Lortholary, O., Dromer, F., et al. (2006a). Molecular Identification of Zygomycetes From Culture and Experimentally Infected Tissues. J. Clin. Microbiol. 44 (2), 340–349. doi: 10.1128/JCM.44.2.340-349.2006
Schwarz, P., Cornely, O. A., Dannaoui, E. (2019). Antifungal Combinations in Mucorales: A Microbiological Perspective. Mycoses. 62 (9), 746–60. doi: 10.1111/myc.12909
Schwarz, P., Djenontin, E., Dannaoui, E. (2020b). Colistin and Isavuconazole Interact Synergistically In Vitro Against Aspergillus Nidulans and Aspergillus Niger. Microorganisms 8 (9), 1447. doi: 10.3390/microorganisms8091447
Schwarz, P., Dromer, F., Lortholary, O., Dannaoui, E. (2006b). Efficacy of Amphotericin B in Combination With Flucytosine Against Flucytosine-Susceptible or Flucytosine-Resistant Isolates of Cryptococcus Neoformans During Disseminated Murine Cryptococcosis. Antimicrob. Agents Chemother. 50 (1), 113–120. doi: 10.1128/AAC.50.1.113-120.2006
Schwarz, P., Janbon, G., Dromer, F., Lortholary, O., Dannaoui, E. (2007). Combination of Amphotericin B With Flucytosine is Active In Vitro Against Flucytosine-Resistant Isolates of Cryptococcus Neoformans. Antimicrob. Agents Chemother. 51 (1), 383–385. doi: 10.1128/AAC.00446-06
Schwarz, P., Nikolskiy, I., Bidaud, A. L., Sommer, F., Bange, G., Dannaoui, E. (2022). In Vitro Activity of Amphotericin B in Combination With Colistin Against Fungi Responsible for Invasive Infections. J. Fungi (Basel) 8 (2), 115. doi: 10.3390/jof8020115
Vannini, M., Emery, S., Lieutier-Colas, F., Legueult, K., Mondain, V., Retur, N., et al. (2022). Epidemiology of Candidemia in NICE Area, France: A Five-Year Study of Antifungal Susceptibility and Mortality. J. Mycol. Med. 32 (1), 101210. doi: 10.1016/j.mycmed.2021.101210
Vitale, R. G. (2021). Role of Antifungal Combinations in Difficult to Treat Candida Infections. J. Fungi (Basel) 7 (9), 731. doi: 10.3390/jof7090731
Yapar, N. (2014). Epidemiology and Risk Factors for Invasive Candidiasis. Ther. Clin. Risk Manag. 10, 95–105. doi: 10.2147/TCRM.S40160
Yousfi, H., Ranque, S., Rolain, J. M., Bittar, F. (2019). In Vitro Polymyxin Activity Against Clinical Multidrug-Resistant Fungi. Antimicrob. Resist. Infect. Control 8, 66. doi: 10.1186/s13756-019-0521-7
Zeidler, U., Bougnoux, M. E., Lupan, A., Helynck, O., Doyen, A., Garcia, Z., et al. (2013). Synergy of the Antibiotic Colistin With Echinocandin Antifungals in Candida Species. J. Antimicrob. Chemother. 68 (6), 1285–1296. doi: 10.1093/jac/dks538
Keywords: antifungal combination, Candida, colistin, EUCAST, in vitro, isavuconazole
Citation: Schwarz P, Nikolskiy I, Bidaud A-L, Sommer F, Bange G and Dannaoui E (2022) In Vitro Synergy of Isavuconazole Combined With Colistin Against Common Candida Species. Front. Cell. Infect. Microbiol. 12:892893. doi: 10.3389/fcimb.2022.892893
Received: 09 March 2022; Accepted: 31 March 2022;
Published: 29 April 2022.
Edited by:
Shahram Mahmoudi, Iran University of Medical Sciences, IranReviewed by:
Andy Mark Borman, Public Health England, United KingdomCopyright © 2022 Schwarz, Nikolskiy, Bidaud, Sommer, Bange and Dannaoui. This is an open-access article distributed under the terms of the Creative Commons Attribution License (CC BY). The use, distribution or reproduction in other forums is permitted, provided the original author(s) and the copyright owner(s) are credited and that the original publication in this journal is cited, in accordance with accepted academic practice. No use, distribution or reproduction is permitted which does not comply with these terms.
*Correspondence: Patrick Schwarz, cGF0cmljay5zY2h3YXJ6QG1lZC51bmktbWFyYnVyZy5kZQ==
†ORCID: Patrick Schwarz, orcid.org/0000-0003-0383-1865
Anne-Laure Bidaud, orcid.org/0000-0001-7020-6686
Gert Bange, orcid.org/0000-0002-7826-0932
Eric Dannaoui, orcid.org/0000-0002-2817-3830
Disclaimer: All claims expressed in this article are solely those of the authors and do not necessarily represent those of their affiliated organizations, or those of the publisher, the editors and the reviewers. Any product that may be evaluated in this article or claim that may be made by its manufacturer is not guaranteed or endorsed by the publisher.
Research integrity at Frontiers
Learn more about the work of our research integrity team to safeguard the quality of each article we publish.