- 1Department of Research, Mount Sinai, Medical Center, Miami, FL, United States
- 2Centro de Biofísica y Bioquímica, Instituto Venezolano de Investigaciones Científicas, Caracas, Venezuela
- 3Division of Neonatology, Mount Sinai, Medical Center, Miami, FL, United States
Chagas disease is produced by the parasite Trypanosoma cruzi (T. cruzi), which is the leading cause of death and morbidity in Latin America. We have shown that in patients with Chagas cardiomyopathy, there is a chronic elevation of diastolic Ca2+ concentration ([Ca2+]d), associated with deterioration to further address this issue, we explored the role Na+/Ca2+ exchanger (NCX). Experiments were carried out in noninfected C57BL/6 mice and infected with blood-derived trypomastigotes of the T. cruzi Y strain. Anesthetized mice were sacrificed and the cardiomyocytes were enzymatically dissociated. Diastolic [Ca2+] ([Ca2+]d) was measured using Ca2+ selective microelectrodes in cardiomyocytes from control mice (CONT) and cardiomyocytes from T. cruzi infected mice in the early acute phase (EAP) at 20 dpi, in the acute phase (AP) at 40 dpi, and in the chronic phase (CP) at 120 dpi. [Ca2+]d was 1.5-times higher in EAP, 2.6-times in AP, and 3.4-times in CP compared to CONT. Exploring the reverse mode activity of NCX, we replaced extracellular Na+ in equivalent amounts with N-methyl-D-glucamine. Reduction of [Na+]e to 65 mM caused an increase in [Ca2+]d of 1.7 times in cardiomyocytes from CONT mice, 2 times in EAP infected mice, 2.4 times in AP infected mice and 2.8 in CP infected mice. The Na+ free solution caused a further elevation of [Ca2+]d of 2.5 times in cardiomyocytes from CONT, 2.8 times in EAP infected mice, 3.1 times in AP infected mice, and 3.3 times in CP infected mice. Extracellular Ca2+ withdrawal reduced [Ca2+]d in both CONT and cardiomyocytes from Chagas-infected mice and prevented the increase in [Ca2+]d induced by Na+ depletion. Preincubation with 10µM KB-R7943 or in 1µM YM-244769 reduced [Ca2+]d in cardiomyocytes from infected mice, but not control mice. Furthermore, both NCX blockers prevented the increase in [Ca2+]d associated with exposure to a solution without Na+. These results suggest that Ca2+ entry through the reverse NCX mode plays a significant role in the observed [Ca2+]d dyshomeostasis in Chagas infected cardiomyocytes. Additionally, NCX inhibitors may be a viable therapeutic approach for treating patients with Chagas cardiomyopathy.
Introduction
Chagas disease, caused by the protozoan Trypanosoma cruzi (T. cruzi), affects approximately eight million people in the poorest areas of Central and South America. The prevalent form of transmission of T. cruzi to humans is contact with contaminated feces/urine of the invertebrate vector during a blood meal. However, human infection through blood transfusion, organ transplantation and oral. (Bern et al., 2008) and oral transmission due to ingestion of contaminated fruit juices (Alarcon De Noya et al., 2010) have been reported. Chagas disease is characterized by a varied clinical scenario that evolves from an acute phase, often asymptomatic or oligosymptomatic, to a chronic phase that can manifest as indeterminate, cardiac, or digestive forms (Echavarria et al., 2021). Most infected people develop cardiac conditions characterized by ventricular arrhythmias, heart blocks, heart failure, thromboembolic phenomena, and sudden death (Rassi et al., 2010; Teixeira et al., 2011; Echavarria et al., 2021; http://www.who.int/mediacentre/factsheets/fs340/en/, 2021).
Calcium is a central player in the regulation of cardiac contractility, and its intracellular concentration is maintained through the interaction of Ca2+ transport mechanisms and diastolic leak mediated by the ryanodine receptor (Shannon et al., 2003; Bers and Ginsburg, 2007; Bers, 2014; Freichel et al., 2017; Smith and Eisner, 2019). In healthy cardiomyocytes, the diastolic Ca2+ concentration ([Ca2+]d) is in the range of 100-120 nM (Marban et al., 1980; Lopez et al., 2011; Mijares et al., 2014; Mijares et al., 2020). We have demonstrated dysregulation of [Ca2+]d in cardiomyocytes isolated from Chagas patients, which is correlated with the patient’s clinical condition (Lopez et al., 2011). Furthermore, this diastolic disturbance of [Ca2+] appears to be associated with alterations in the regulation of the intracellular messenger inositol 1,4,5-trisphosphate (Lopez et al., 2011; Mijares et al., 2020).
NCX is a bidirectional high-capacity transporter that represents the primary way of Ca2+ extrusion in excitable cells (Blaustein and Lederer, 1999; Aronsen et al., 2013). It can import 3 Na+ into the cell in exchange for 1 Ca2+ (forward mode) or drive the efflux of 3 Na+ in exchange for 1 Ca2+ (reverse mode) across the plasma membrane (Bers and Ginsburg, 2007). The direction of NCX (forward or reverse) depends on the internal and external concentration of both Na+ and Ca2+ and the membrane potential (Bers, 2000). NCX works almost exclusively in the forward mode (Ca2+ extrusion) in normal cardiomyocytes, driven primarily by the elevated subsarcolemmal [Ca2+]. Elevated diastolic [Na+] ([Na+]d) could change the direction of the fluxes to a more significant influx of Ca2 + and fewer Ca2 + effluxes, resulting in increased intracellular [Ca2+]. However, the dynamic of NCX can be altered in pathophysiological situations such as heart failure, arrhythmia, ischemia-reperfusion injury, and hypertrophy (Bers and Despa, 2006). Recently, Santos-Miranda et al. (Santos-Miranda et al., 2021) reported a cellular arrhythmogenic profile in chronic Chagas cardiomyopathy, which was sensitive to Ni2+ and NCX blocker SEA0400, indicating the involvement of NCX.
In the present study, we evaluated the activity of NCX in cardiomyocytes from control and Chagas infected mice. We showed that NCX activity is enhanced in cardiomyocytes from T. cruzi-infected mice compared to control cardiomyocytes and that the reverse mode of NCX contributes to intracellular Ca2 + dyshomeostasis observed in cardiomyocytes from infected mice.
Material and Methods
Animals
Homozygous male C57BL/10 with a bodyweight of 25 ± 0.45 g. and 12 weeks of age were housed at constant temperature (24 C) and a 12 h light/12 h dark cycle, with free access to water and fed a commercial pelletized diet.
Trypanosoma cruzi Infection
Mice were randomly divided into two groups: Group A (Control) that were not inoculated with T. cruzi and Group B (infected mice) were infected intraperitoneally with 5x10-2 blood-form trypomastigotes of the Y strain of T. cruzi (O’Daly et al., 1984). The Y strain of T. cruzi was originally obtained from patients in Brazil and was kindly provided by Dr. Jose O’Daly, who has maintained the strain over the years through serial passages in homozygous C57B1/6 mice. Parasitemia was monitored by counting the number of parasites/5 μl of blood obtained from the tail vein of infected mice. A positive parasitemia was found in all inoculated animals on days 12-15 post-infection (dpi). Non-infected and infected anesthetized (ketamine/xylazine) mice were sacrificed by cervical dislocation at 20 (early acute phase), 40 (acute phase), and 120-dpi (chronic phase), and the cardiomyocytes were enzymatically dissociated.
Isolation of Cardiomyocytes and Inclusion Criteria
Control and infected mice were anesthetized (ketamine 100 mg/kg/xylazine 5 mg/Kg) and hearts were rapidly removed, attached to a cannula, and mounted on a Langendorff reverse coronary perfusion system for the enzymatic dissociation of ventricular cardiomyocytes (Liao and Jain, 2007). Only Ca2+ tolerant cardiomyocytes with rod-shaped, well-defined striation spacing and a resting sarcomere length of ≥1.75 µm (Wussling et al., 1987) were used and studied within 4-6 h after isolation. The isolation procedure was carried out at 37°C.
Ca2+ and Na+ Selective Microelectrodes
Double-barreled Ca2+ and Na+ selective microelectrodes were prepared as previously described (Eltit et al., 2013). Each ion-selective microelectrode was individually calibrated before and after the determination of [Ca2+]d and the [Na+]d as previously described (Mijares et al., 2014). Ca2+ selective microelectrodes with a Nernstian response between pCa3 and pCa7 (30.5 mV/pCa units at 37°C) and Na+ selective microelectrodes with Nernstian responses between 100 and 10 mM [Na+]e, and an adequate response (40–45 mV) between 10 and 1 mM [Na+]e were used experimentally (Lopez et al., 1983; Lopez et al., 2000; Eltit et al., 2013). The Ca2+- and Na+-microelectrode response was not affected by any of the drugs used in the present study.
Recording of Diastolic Calcium and Sodium Concentrations
Isolated cardiomyocytes from control mice and T. cruzi infected mice were impaled with double-barrel Ca2+ or Na+ microelectrodes to measure [Ca2+]d and [Na+]d, respectively, and potentials were recorded using a high impedance electrometer (WPI 773 electrometer, FL, USA) as previously described (Lopez et al., 2000; Lopez et al., 2017). The potential of the 3M KCl microelectrode barrel (Vm) was electronically subtracted from the potential recorded by the Ca2+ selective barrel (VCa) to produce a differential Ca2+ specific potential (VCaE) that represents the cardiomyocyte [Ca2+]d. A similar approach was used to produce the specific Na+ potential (VNaE), which represents the cardiomyocyte [Na+]d. The potentials were acquired at a frequency of 1,000 Hz with the AxoGraph software (version 4.6; Axon Instruments, CA, USA) and stored in a computer for further analysis.
Individual cardiomyocyte measurements of [Ca2+]d and [Na+]d from control and infected mice were accepted when there was i) an abrupt drop to a steady level of Vm equal to or more negative than -80 mV in healthy cells and -70 mV in unhealthy cardiomyocytes; ii) a stable recording of Vm and VCa potentials or Vm and VNa potentials for more than 60 seconds and an abrupt return to baseline at the exit of the microelectrode from the cell. Vm was used as a biological indicator of the integrity of cardiomyocyte sarcolemma in real-time; therefore, any cardiomyocytes with a recorded Vm <-70 mV were discarded. These criteria were not met in 18% of total impalements performed in cardiomyocytes from control mice and 41% in infected mouse cardiomyocytes.
Reverse Mode NCX Protocol
The effect of NCX (reverse mode) on [Ca2+]d in cardiomyocytes from control and infected mice was determined by preincubating cardiomyocytes in a solution in which [Na+]e was partially or completely removed (see solutions below) and replaced by N-methyl-D-glucamine hydrochloride. Measurements of [Ca2+]d were carried out before and after incubation in low Na+ solutions.
Equilibrium Potentials
The equilibrium potentials for Ca2+ (ECa) and Na+ (ENa) were calculated using the Nernst equation (Eion= RT/zF x ln [ion]i/[ion]e) and [Ca2+]d and [Na+]d obtained in cardiomyocytes from CONT and infected mice in EAP, AP, and CP. The equilibrium potential of the Na+/Ca2+ exchanger (ENCX) was estimated using the following equation: ENCX = 3ENa - 2ECa (with a stoichiometry of 3:1), where ENa and ECa are the equilibrium potentials of Na+ and Ca2+ obtained from the Nernst equation.
Solutions
All solutions were made with ultrapure water supplied by a Milli-Q system (Millipore, Bedford, MA). The Tyrode solution had the following composition (in mM): 130 NaCl, 5 KCl, 2.5 CaCl2, 1 MgCl2, 20 NaHCO3, 0.33 NaH2PO4, and 10 glucose gassed with 95% O2 and 5% CO2, pH 7.4. A low- or Na+-free solution was prepared by the partial or total withdrawal of [Na+]e, and replacement by an equivalent amount of impermeable cation N-methyl-D-glucamine (NMG) hydrochloride to maintain osmolarity. A Ca2 +-free solution was prepared, omitting CaCl2 and adding 1 mM of EGTA and 2 mM of MgCl2. Solutions 2-(2-(4-(4-nitrobenzyloxy-phenyl-ethyl-isothiourea methanesulfonate (KB-R7943) and N- (3-aminobenzyl) -6- 4- [(3-fluorobenzyl-oxy] phenoxy nicotinamide (YM-244769) were made by adding the desired concentration of the reagent to the Tyrode solution. All experiments were carried out at 37°C.
Statistical Analysis
Experimental results are expressed as means ± SD; nmice represents the number of mice used and ncells the number of successful measurements in cardiomyocytes isolated from CONT and infected mice used for statistical analysis. Data were analyzed using one-way analysis of variance ANOVA for repeated measures, followed by Tukey’s multiple comparison tests to determine significance. A p<0.05 was considered significant. GraphPad Prism 9 (GraphPad Software, CA, USA) was used for statistical analysis.
Results
[Ca2+]d and [Na+]d in Ventricular Cardiomyocytes of Chagasic Rodents
We previously reported a significant elevation in [Ca2+]d in cardiomyocytes obtained from patients with chronic Chagas cardiomyopath, which was related to the extent of their cardiac dysfunction (Lopez et al., 2011; Mijares et al., 2020). Figure 1 shows representative records of simultaneous measurement of Vm and [Ca2+]d in a single cardiomyocyte isolated from (A) CONT mice, (B) EAP-infected mice (20 dpi), (C) AP-infected mice (40 dpi), and (D) CP-infected mice (120 dpi). The cardiomyocytes of Chagas-infected mice showed partial depolarization and increased [Ca2+]d, which aggravated as a function of days after infection. The mean Vm and [Ca2+]d in quiescent cardiomyocytes from CONT mice were 81 ± 1 mV and 122 ± 3 nM. Respectively. In cardiomyocytes from EAP-infected mice, Vm was 78 ± 1.7 mV and [Ca2+]d 194 ± 23 nM (p<0.001 compared to CONT cardiomyocytes); in cardiomyocytes from AP-infected mice, Vm was 76 ± 1.6 mV and [Ca2+]d 320 ± 38 nM (p<0.001 compared to CONT cardiomyocytes) and in cardiomyocytes from CP-infected mice, Vm was 72 ± 1.8 mV and [Ca2+]d 470 ± 43 nM (p<0.001 compared to CONT cardiomyocytes) (Figures 2A, B).
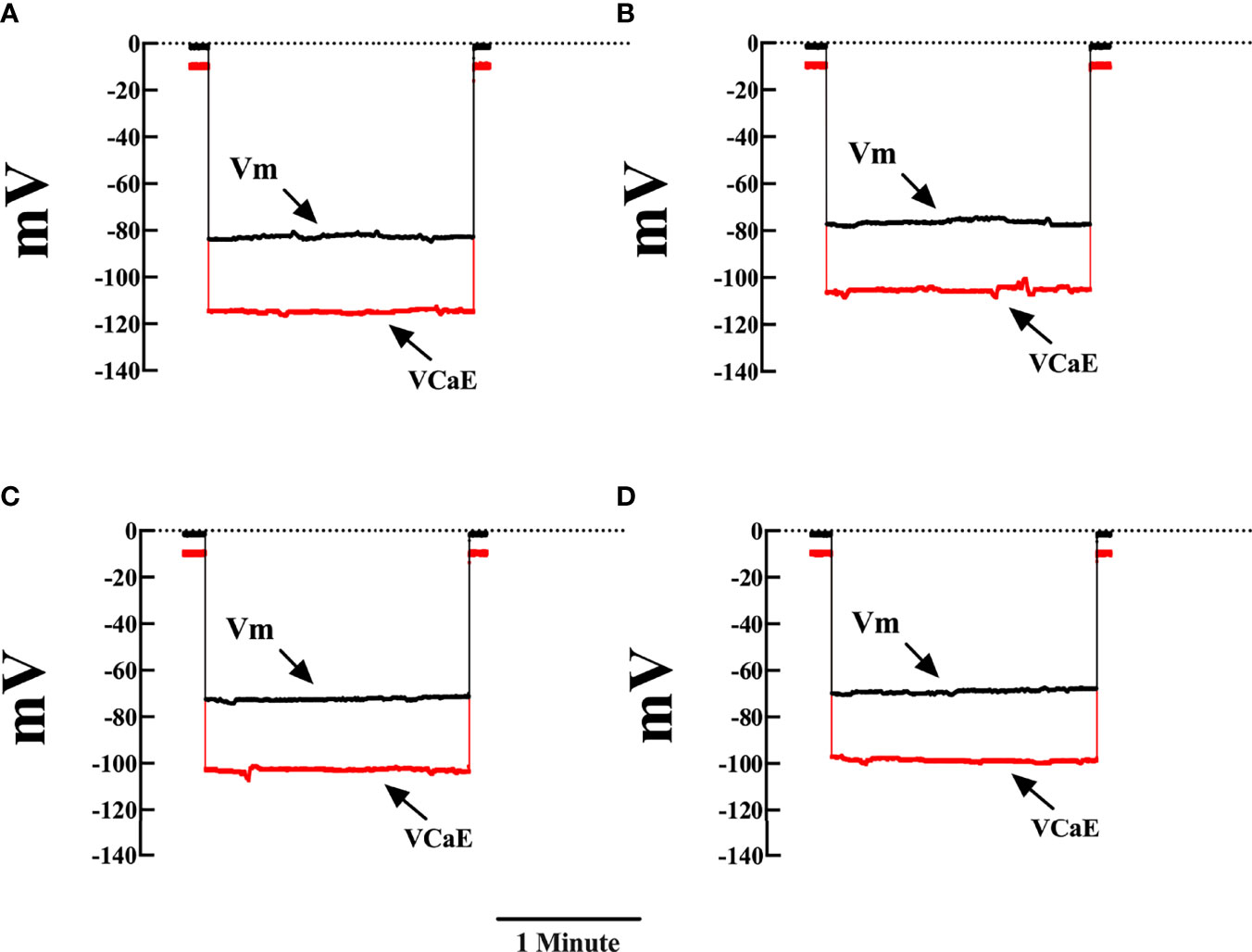
Figure 1 Representative measurements of Vm and [Ca2+]d in cardiomyocytes from control and Chagas infected mice. Simultaneous measurements of membrane potential (Vm) and [Ca2+]d (A) Cardiomyocyte from control mice (Vm was -82 mV and [Ca2+]d 122 nM). (B) Cardiomyocyte from EAP-infected mice (Vm was -76mV and [Ca2+]d 200 nM). (C) Cardiomyocyte from AP-infected mice (Vm was -73 mV and [Ca2+]d 312 nM). (D) Cardiomyocyte from CP-infected mice (Vm was -69 mV and [Ca2+]d 414 nM). Vm, resting membrane potential and VCae, specific calcium potential; CONT, control mice; EAP, infected mice in the early acute phase; AP, infected mice in the acute phase; and CP, infected mice in the chronic phase. Calibration bar = 1 minute.
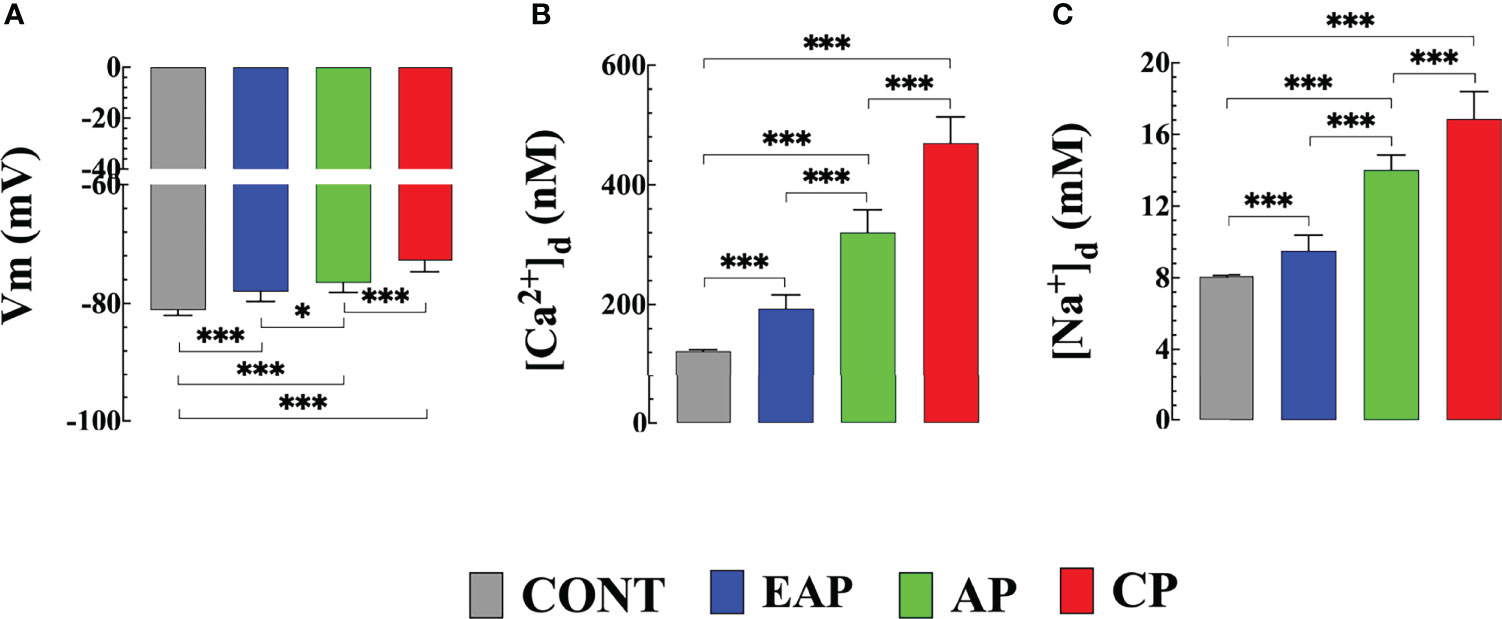
Figure 2 Resting membrane potential, diastolic [Ca2+] and [Na+] in cardiomyocytes from control and Chagas infected mice. (A) The resting membrane potential, (B) [Ca2+]d, and (C) [Na+]d were measured in quiescent cardiomyocytes from CONT mice (ncells=24 and nmice=3), EAP-infected mice (ncells=19 and nmice=3), AP-infected mice (ncells=21 and nmice=3) and CP-infected mice (ncells=18 and nmice=5). Data are expressed as means ± S.D. Statistical analysis was performed using one-way ANOVA, followed by Tukey’s multiple comparison tests, *p < 0.05; ***p < 0.001. CONT, control mice; EAP, early acute phase of infected mice; AP, acute phase of infected mice; and CP, chronic phase of infected mice.
Similar differences in [Na+]d were obtained in the measurements carried out in quiescent cardiomyocytes of Chagas-infected mice at different phases of infection. In cardiomyocytes from CONT mice [Na+]d was 8 ± 0.2 mM; In cardiomyocytes from EAP-infected mice [Na+]d was 9.4 ± 0.7 mM (p<0.001 compared to CONT cardiomyocytes); In cardiomyocytes from AP-infected mice [Na+]d was 13.9 ± 0.8 mM (p<0.001 compared to CONT cardiomyocytes); and in cardiomyocytes from CP-infected mice [Na+]d was 15.9 ± 1 mM (p<0.001 compared to CONT cardiomyocytes) (Figure 2C).
Removal of [Na+]e Increased [Ca2+]d in Cardiomyocytes
In a separate set of experiments, the reverse mode activity of NCX (Ca2+ entry in exchange for Na+ leaving the cell) was studied in quiescent cardiomyocytes isolated from control and infected mice by incubation in a solution in which Na+ was replaced in equivalent amounts by NMG. [Ca2+]d was measured before and after incubation with a low or Na+ free solution. The reduction of [Na+]e to 65 mM caused an increase in [Ca2+]d in CONT cardiomyocytes to 212 ± 27 nM, in cardiomyocytes from EAP-infected mice to 400 ± 24 nM, in cardiomyocytes from AP infected mice to 790 ± 62 nM, and cardiomyocytes from CP-infected mice to 1,320 ± 137 nM (p<0.001 compared to cardiomyocytes from CONT, EAP, AP and CP-infected mice bathed in normal [Na+]e) (Figure 3).
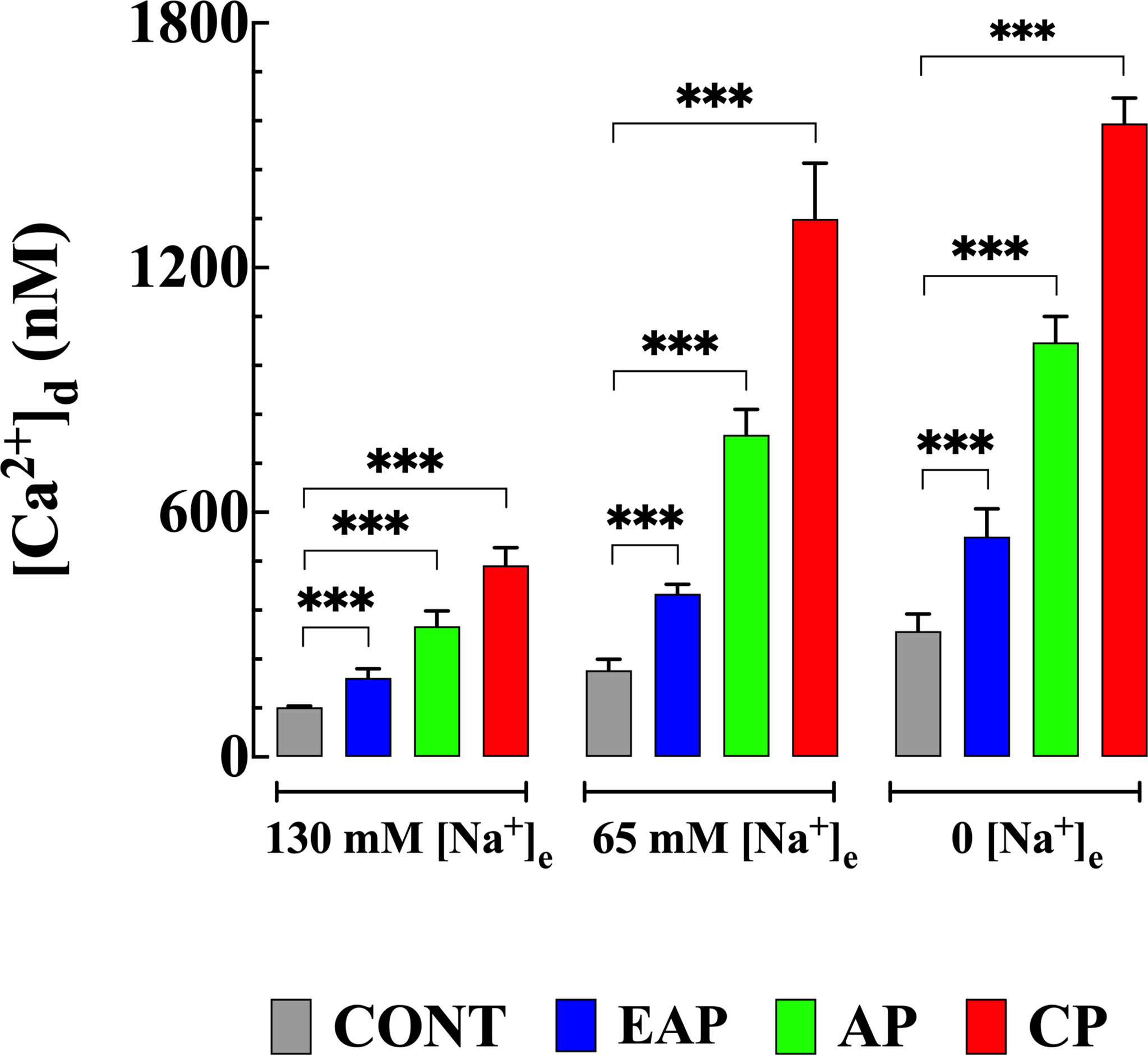
Figure 3 Effects of reduction in [Na+]e on [Ca2+]d in cardiomyocytes from control and Chagas infected mice. [Ca2+]d was measured before and after cardiomyocyte treatments with solutions in which [Na+]e was partially or completely replaced with NMG. [Ca2+]d was determined in quiescent cardiomyocytes from CONT mice (ncells=13-24 and nmice=4), EAP-infected mice (ncells=12-19 and nmice=5), AP-infected mice (ncells=12-21 and nmice=5) and CP-infected mice (ncells=6-18 and nmice=9). Data are expressed as means ± S.D. Statistical analysis was performed as described above, ***p < 0.001. CONT, control mice; EAP, early acute phase of infected mice; AP, acute phase of infected mice; and CP, chronic phase of infected mice.
Incubation in Na+ free solution caused a further increase in [Ca2+]d in control and cardiomyocytes from infected mice. In CONT cardiomyocytes [Ca2+]d increased significantly to 309 ± 42 nM, in cardiomyocytes from EAP-infected mice to 540 ± 69 nM, from AP-infected mice to 1,016 ± 64 nM, and from CP-infected mice to 1,523 ± 62 nM respectively (p<0.001 compared to cardiomyocytes from CONT, and EAP-, AP- and CP- infected mice bathed in normal [Na+]e). (Figure 3). The sustained increase [Ca2+]d was maintained as long as Na+ was absent from the bath solution and reversed when Na+ was reintroduced into the Tyrode solution, except for cardiomyocytes from CP- infected mice where an irreversible elevation [Ca2+]d associated with contracture was observed.
Removal of Extracellular Ca2+ Prevents the Increase in [Ca2+]d Induced by Na+ Depletion
In a different set of experiments, we explored the contribution of the Ca2+ influx to the elevation of [Ca2+]d elicited by the withdrawal of Na+ in quiescent cardiomyocytes from control and infected mice. Cardiomyocytes were incubated for 10 minutes in a Ca2+-free solution prior to the withdrawal of Na+ (see solutions). Incubation in a Ca2+-free solution significantly reduced [Ca2+]d to 98 ± 5 nM in cardiomyocytes from CONT mice (p<0.001 compared to CONT cardiomyocytes in normal [Ca2+]e), to 100 ± 11 nM in cardiomyocytes from EAP-infected mice (p<0.001 compared to EAP cardiomyocytes in normal [Ca2+]e), to 123 ± 14 nM cardiomyocytes from AP-infected mice (p<0.001 compared to AP cardiomyocytes in normal [Ca2+]e), and to 136 ± 11 nM in cardiomyocytes from CP-infected mice (p<0.001 compared to CP cardiomyocytes in normal [Ca2+]e). (Figure 4). Elevation of [Ca2+]d induced by withdrawal of Na+ was abolished in the absence of extracellular Ca2+ in all cardiomyocyte groups (Figure 4).
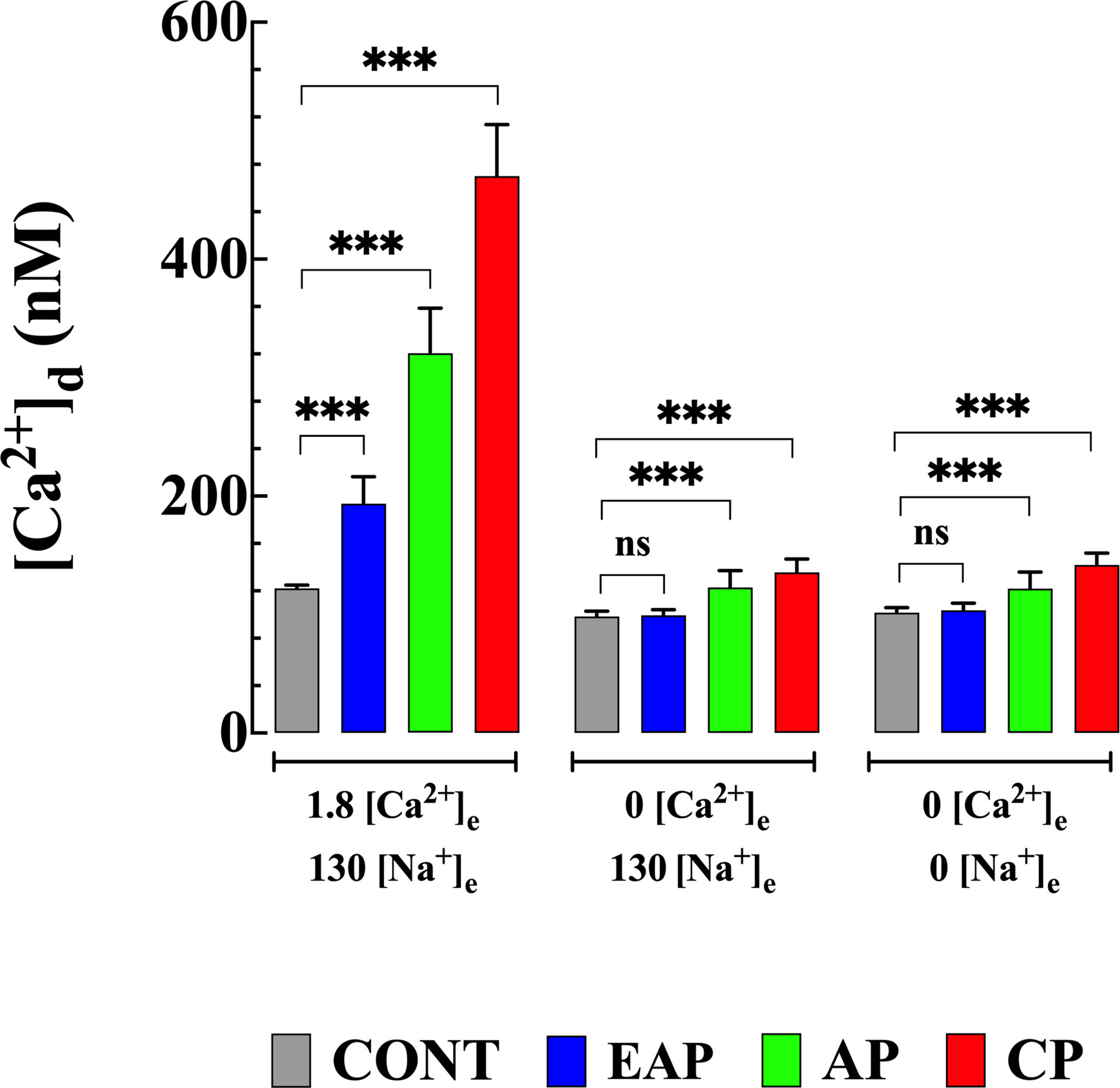
Figure 4 Effect extracellular Ca2+ on Na+ depletion-induced elevation of [Ca2+]d. [Ca2+]d was measured in cardiomyocytes from CONT, EAP-, AP-, and CP-infected mice in normal Tyrode, Ca2+-free Tyrode solution, and Ca2+ and Na+ free Tyrode solution. [Ca2+]d was determined in quiescent cardiomyocytes from CONT (ncells=16-24 and nmice=4), EAP-infect mice (ncells=15-19 and nmice=5), AP-infect mice (ncells=18-21 and nmice=6) and CP-infect mice (ncells=15-18 and nmice=8). Data are expressed as mean ± S.E. Statistical analysis was performed as described above, *** p < 0.001. CONT, control mice; EAP, early acute phase of infected mice; AP, acute phase of infected mice; and CP, chronic phase of infected mice. ns, no significant.
Equilibrium Potentials
Using the Nernst equation and the actual values [Ca2+]d and [Na+]d found in the present study, the estimated ECa in cardiomyocytes from CONT mice was +133 mV, in cardiomyocytes from EAP-infected mice was +127 mV, in cardiomyocytes from AP-infected mice was +119 mV, and in cardiomyocytes from CP-infected mice were +116 mV. While the estimated ENa in cardiomyocytes isolated from CONT mice was +74 mV, EAP-infected mice was +70 mV, AP-infected mice was +59 mV, and CP-infected mice was +56 mV. The equilibrium potential of the Na+/Ca2+ exchanger (ENCX) was -44 mV in cardiomyocytes from CONT, -46 mV from EA-infected mice, -61 mV from AP-infected mice, and –64 mV from CP-infected mice, respectively.
KB-R7943 and YM-244769 Reduced [Ca2+]d and Prevented the Elevation of Ca2+ Induced by the Na+ Free Solution
To determine the mechanisms involved in the elevation of [Ca2+]d caused by exposure to Na+ free medium, quiescent cardiomyocytes from control and infected mice were incubated in KB-R7943, a nonspecific blocker of the NCX reverse mode, which modified Na+ dependent binding (Iwamoto et al., 1996; Akabas, 2004). Cardiomyocyte preincubation with 10 μM KB-R7943 for 10 minutes significantly reduced [Ca2+]d and inhibited the increase in [Ca2+]d induced by the solution without Na+ (Figure 5A). KB-R7943 reduced [Ca2+]d to 152 ± 16 nM in cardiomyocytes from EAP-infected mice, to 186 ± 21 nM from AP-infected mice, and 258 ± 30 nM from CP-infected mice (Figure 5A). No significant effect was observed in cardiomyocytes from CONT mice (116 ± 5 nM). Preincubation with KB-R7943 prevented the elevation of [Ca2+]d caused by the Na+ free solution in cardiomyocytes of control and infected mice (compare Figures 3,5A).
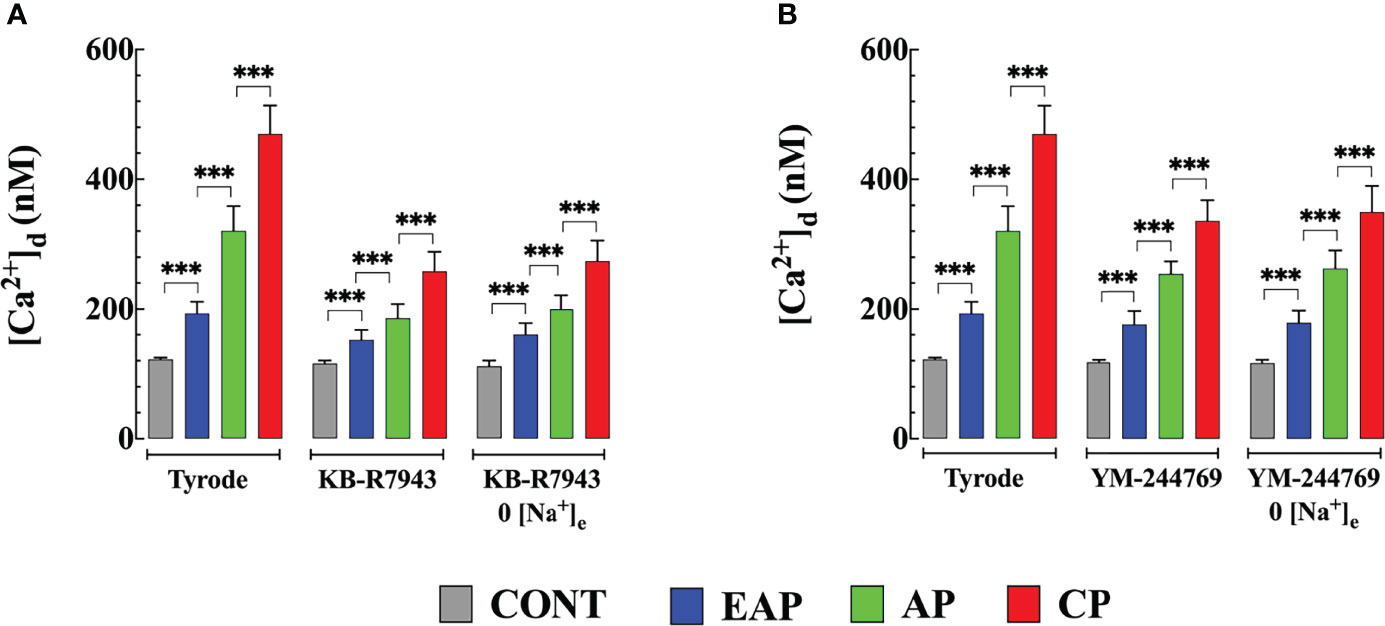
Figure 5 NCX blockers inhibited Na+ withdraw-induced [Ca2+]d increases in cardiomyocytes from control and Chagas infected mice. (A) Effects of KB-R7943 and (B) YM-244769 on [Ca2+]d increases caused by Na+ removal. [Ca2+]d was determined in (i) normal Tyrode solution (ii) after 10 min of incubation with 10 μm KB-R7943) or 1 µM YM-244769 and (iii) after incubation, either KB-R7943 in Na+ free solution or YM-244769 in Na+ free solution. Experiments were carried out in cardiomyocytes from CONT (ncells=15-24 and nmice=6), EAP (ncells=12-19 and nmice=8), AP (ncells=13-21 and nmice=8) and CP cardiomyocytes (ncells=11-18 and nmice=9). Data are expressed as mean ± S.E. Statistical analysis was performed as described above, ***p < 0.001. CONT: control mice; EAP: early acute phase of infected mice; AP: acute phase of infected mice; and CP: chronic phase of infected mice.
Due to the lack of specificity of KB-R7943 (Abramochkin and Vornanen, 2014), we also examined the effects of YM-244769, a high-affinity blocker of the NCX reverse mode (Iwamoto and Kita, 2006). Incubation with 1 μM YM-244769 for 10 minutes reduced [Ca2+]d to 176 ± 20 nM in cardiomyocytes from EAP-infected mice, to 254 ± 19 nM from AP-infected mice, and to 336 ± 32 nM from CP-infected mice. YM-244769 did not significantly reduce [Ca2+]d in cardiomyocytes of CONT and EAP-infected mice. YM-244769 fully inhibited the elevation of [Ca2+]d induced by the Na+ free solution in CONT cardiomyocytes and infected mice (compare Figures 3, 5B).
Discussion
Cardiac alterations are a severe and recurrent complication related to Chagas disease and represent the most common causes of heart failure and sudden death in Latin America (Velasco and Morillo, 2020). The present study reinforces our previous finding that progressive deterioration of cardiac function in Chagas cardiomyopathy is associated with defective intracellular Ca2+ regulation. In this report, we demonstrate, for the first time, that ventricular cardiomyocytes from rodents infected with T. cruzi show a partial depolarization associated with aberrant [Ca2+]d and [Na+]d, which aggravated with endpoint after infection (chronic>acute>early acute). Furthermore, we provide evidence that chagasic infection enhances the reverse mode of NCX, causing an elevation of [Ca2+]d, which is not mediated by membrane depolarization.
Calcium is essential in the regulation of cardiac contractility, and defective intracellular Ca2+ homeostasis plays an vital role in the pathogenesis of diverse cardiac diseases (Houser and Margulies, 2003; Lopez et al., 2011; Uryash et al., 2021a; Uryash et al., 2021b). [Ca2+]d is regulated by several mechanisms that controls Ca2+ influx-efflux and intracellular reuptake that allow the maintenance of [Ca2+] within a physiological range (~100 nM) (Lopez et al., 2011; Mijares et al., 2014; Mijares et al., 2020). The [Ca2+]d values obtained from CONT cardiomyocytes are in agreement with previous estimates obtained from humans (Lopez et al., 2011; Mijares et al., 2020) and non-human ventricular myocytes (Mijares et al., 2014; Uryash et al., 2021a; Uryash et al., 2021b) using Ca2+-selective microelectrodes and fluorescent Ca2+ indicator (Piacentino et al., 2003). The abnormal [Ca2+]d in cardiomyocytes from infected mice (chronic>acute>early acute) agree with a similar dysfunction observed in cardiomyocytes obtained from Chagas patients and could potentially promote arrhythmias, similar to those observed in patients with Chagas cardiomyopathy (Benziger et al., 2017; Almeida et al., 2018).
Normal resting [Na+]d in cardiomyocytes is in the range of 7-8 mM (Gray et al., 2001; Mijares et al., 2014), while in cardiomyocytes from infected mice, it was higher (CP>EP>AEP). Intracellular [Na+] is mainly regulated in cardiac myocytes by Na+/K+-ATPase, Na+/Ca2+ exchange, Na+ channels, and amiloride-sensitive Na+/H+ exchanger (Everts et al., 1992; Blaustein and Lederer, 1999; Bers et al., 2003). An elevated [Na+]d, as found in Chagas-infected mice cardiomyocytes, could change the balance of fluxes through NCX to favor a greater inflow of Ca2+ (reverse mode) (Blaustein and Lederer, 1999), contributing to intracellular Ca2+ overload as observed in Chagas-infected mice cardiomyocytes. Furthermore, it is plausible that the partial depolarization observed in cardiomyocytes from infected mice (chronic>acute>early acute) may be related to diastolic Na+ overload. Unfortunately, we did not explore whether the elevation of [Na+]d in cardiomyocytes from infected mice was related to an increased influx or decreased efflux of Na+; therefore, no conclusions can be drawn.
Sodium-calcium exchange is the major Ca2+ efflux mechanism of ventricular cardiomyocytes. NCX mediates an electrogenic exchange of 3 Na+ for 1 Ca2+, which can occur in the forward (Ca2+ extrusion–Na+ entry) or in the reverse (Ca2+ entry– Na+ extrusion) mode (Blaustein and Lederer, 1999; Bers, 2000; Bers, 2002). In this study, we provide evidence for the first time of an enhancement in the reverse mode function of NCX in cardiomyocytes from infected mice (chronic>acute>early acute). The increase in [Ca2+]d mediated by NCX was not associated with membrane depolarization and was dependent on extracellular [Ca2+] and [Na+] in all cardiomyocytes. Therefore, changes in NCX could contribute to intracellular Ca2+ overload, but could also contribute to the arrhythmogenesis observed in Chagas patients (Stein et al. 2018). The elevation of [Ca2+]d was prevented in cardiomyocytes from control and infected mice by removing extracellular Ca2+ and reversed when Na+ was reintroduced into the bath, except for cardiomyocytes from CP-infected mice, where the removal of Na+ (partially or completely) produced irreversible contraction and cell death (40% in 65 mM [Na+]e and 82% in Na+ free solution, respectively).
The mode in which NCX operates is dependent on the Na+ and Ca2+ gradients across the sarcolemma, as well as the membrane potential, following a driving force equal to Em – ENa/Ca (where ENa/Ca= 3ENa –2 ECa with ENa and ECa represent the equilibrium potentials of Na+ and Ca2+, respectively) (Bers and Ginsburg, 2007). If the driving force for 3Na+ is greater than 1Ca2+ in the cell (3:1 Na+/Ca2+ stoichiometry), the NCX will transport Na+ into the cell and take Ca2+ out (forward mode). On the other hand, if under another set of conditions (altered membrane potential, ionic gradients, or post-translational modifications), the driving force for 3Na+ is less than that for 1Ca2+ in the cell, then the electrochemical gradient of Ca2+ will become the dominant inward driving force (reverse mode) (Bers and Ginsburg, 2007). The intracellular ionic changes in cardiomyocytes from infected mice modified the driving force (Em − ENa/Ca) by 5 mV in cardiomyocytes from EAP-infected mice, 22 mV in cardiomyocytes from AP-infected mice, and 29 mV in cardiomyocytes from CP-infected mice.
KB-R7943 significantly reduced [Ca2+]d by 1.3 times in cardiomyocytes from EAP-infected mice, 1.7 times in AP-infected mice, and 1.8 times in CP-infected mice, suggesting that the elevation of [Ca2+]d observed in cardiomyocytes of Chagas infected mice was due in part to an influx of Ca2+ mediated by NCX in its reverse mode. On the contrary, in cardiomyocytes from CONT mice, KB-R7943 did not modify [Ca2+]d, indicating that in healthy quiescent cardiomyocytes, NCX in its reverse mode does not contribute significantly to [Ca2+]d. Furthermore, KB-R7943 prevented the elevation of [Ca2+]d induced by Na+ withdrawal in cardiomyocytes from CONT and cardiomyocytes from infected mice. The involvement of NCX in the pathophysiology of Chagas disease is consistent with the recent publication by Santos-Miranda et al. (Santos-Miranda et al., 2021), which found the contribution of NCX as a cellular arrhythmogenic substrate in isolated cardiomyocytes from infected mice with the Colombian strain of T. cruzi. However, the effect of KB-R7943 on [Ca2+]d must be considered with caution due to the lack of selectivity, since the NCX blocker also affects voltage-gated Na+ and Ca2+ channels, inward rectifying K+ channels in cardiac cells (Watano et al., 1996).
YM-244769 is a potent and highly selective NCX blocker that up to 1 µM (dose used in the present study) preferentially inhibits the reverse mode of NCX, with no effect on the forward mode (Iwamoto and Kita, 2006). YM-244769 significantly reduced [Ca2+]d by 1.3 times in cardiomyocytes from AP- and 1.4 times from CP-infected mice. YM-244769 did not significantly reduce [Ca2+]d in cardiomyocytes from CONT and EAP-infected mice, suggesting that the contribution of NCX to [Ca2+]d is negligible. The effect of YM-244769 on [Ca2+]d cardiomyocytes was small compared to KB-R7943, which is consistent with the fact that YM-244769 is a more specific NCX blocker and that KB-R7943 can act on other Ca2+ entry pathways. Furthermore, YM-244769 blocked the observed elevation of [Ca2+]d caused by the Na+ free solution in all cardiomyocytes. Together, these data strongly support the hypothesis that Ca2+ entry mediated by the reverse NCX mode plays a significant role in [Ca2+]d dyshomeostasis observed in cardiomyocytes isolated from Chagas infected mice during acute and chronic stages of infection. The fact that KB-R7943 or YM-244769 did not normalize [Ca2+]d in cardiomyocytes from infected mice suggests the existence of additional Ca2+ pathways that appear to be altered due to Chagas infection.
Conclusions
These results provide, for the first time, evidence that NCX plays an important role in aberrant diastolic [Ca2+] observed in cardiomyocytes from infected mice (chronic>acute>early acute). These novel findings may open a new therapeutic approach to improve cardiac function in patients suffering from chronic Chagas cardiomyopathy because there is still no effective treatment. Unfortunately, Chagas cardiomyopathy remains largely ignored despite its medical and social relevance.
Study Limitations
Despite the novelty of this study, some limitations should be pointed out. We did not determine whether the elevation of [Na+]d in cardiomyocytes from infected mice could be related to an increased influx or decreased efflux of Na+. Furthermore, the expression of NCX and other proteins involved in intracellular Ca2+ regulation were not studied.
Data Availability Statement
The original contributions presented in the study are included in the article/supplementary material. Further inquiries can be directed to the corresponding author.
Ethics Statement
The animal study was reviewed and approved by Care and Use Handbook of Laboratory Animals published by the US National Institute of Health (NIH publication No. 85-23, revised 1996) and approved by the Institutional Animal Care (IACUC) and Use Committees.
Author Contributions
JRL: Designed research, performed the experiments, and analyzed the data; NL: Performed the experiments; JAA: Designed research; AM: Designed research, and analyzed the data. All authors contributed to manuscript revision, read, and approved the submitted version.
Funding
This work was supported by the Florida Heart Research Foundation.
Conflict of Interest
The authors declare that the research was conducted in the absence of any commercial or financial relationships that could be construed as a potential conflict of interest.
Publisher’s Note
All claims expressed in this article are solely those of the authors and do not necessarily represent those of their affiliated organizations, or those of the publisher, the editors and the reviewers. Any product that may be evaluated in this article, or claim that may be made by its manufacturer, is not guaranteed or endorsed by the publisher.
Acknowledgments
The author thanks Dr. Jose O’Daly for kindly giving us the T cruzi-infected mice.
References
Abramochkin, D. V., Vornanen, M. (2014). Inhibition of the Cardiac ATP-Dependent Potassium Current by KB-R7943. Comp. Biochem. Physiol. A Mol. Integr. Physiol. 175, 38–45. doi: 10.1016/j.cbpa.2014.05.005
Akabas, M. H. (2004). Na+/Ca2+ Exchange Inhibitors: Potential Drugs to Mitigate the Severity of Ischemic Injury. Mol. Pharmacol. 66, 8–10. doi: 10.1124/mol.104.000232.
Alarcon De Noya, B., Diaz-Bello, Z., Colmenares, C., Ruiz-Guevara, R., Mauriello, L., Zavala-Jaspe, R., et al. (2010). Large Urban Outbreak of Orally Acquired Acute Chagas Disease at a School in Caracas, Venezuela. J. Infect. Dis. 201, 1308–1315. doi: 10.1086/651608
Almeida, B. C. S., Carmo, A., Barbosa, M. P. T., Silva, J., Ribeiro, A. L. P. (2018). Association Between Microvolt T-Wave Alternans and Malignant Ventricular Arrhythmias in Chagas Disease. Arq. Bras. Cardiol. 110, 412–417. doi: 10.5935/abc.20180056
Aronsen, J. M., Swift, F., Sejersted, O. M. (2013). Cardiac Sodium Transport and Excitation-Contraction Coupling. J. Mol. Cell Cardiol. 61, 11–19. doi: 10.1016/j.yjmcc.2013.06.003
Benziger, C. P., Do Carmo, G. A. L., Ribeiro, A. L. P. (2017). Chagas Cardiomyopathy: Clinical Presentation and Management in the Americas. Cardiol. Clin. 35, 31–47. doi: 10.1016/j.ccl.2016.08.013
Bern, C., Montgomery, S. P., Katz, L., Caglioti, S., Stramer, S. L. (2008). Chagas Disease and the US Blood Supply. Curr. Opin. Infect. Dis. 21, 476–482. doi: 10.1097/QCO.0b013e32830ef5b6
Bers, D. M. (2000). Calcium Fluxes Involved in Control of Cardiac Myocyte Contraction. Circ. Res. 87, 275–281. doi: 10.1161/01.RES.87.4.275
Bers, D. M. (2002). Cardiac Excitation-Contraction Coupling. Nature 415, 198–205. doi: 10.1038/415198a
Bers, D. M. (2014). Cardiac Sarcoplasmic Reticulum Calcium Leak: Basis and Roles in Cardiac Dysfunction. Annu. Rev. Physiol. 76, 107–127. doi: 10.1146/annurev-physiol-020911-153308
Bers, D. M., Barry, W. H., Despa, S. (2003). Intracellular Na+ Regulation in Cardiac Myocytes. Cardiovasc. Res. 57, 897–912. doi: 10.1016/S0008-6363(02)00656-9
Bers, D. M., Despa, S. (2006). Cardiac Myocytes Ca2+ and Na+ Regulation in Normal and Failing Hearts. J. Pharmacol. Sci. 100, 315–322. doi: 10.1254/jphs.CPJ06001X
Bers, D. M., Ginsburg, K. S. (2007). Na : Ca Stoichiometry and Cytosolic Ca-Dependent Activation of NCX in Intact Cardiomyocytes. Ann. N. Y. Acad. Sci. 1099, 326–338. doi: 10.1196/annals.1387.060
Blaustein, M. P., Lederer, W. J. (1999). Sodium/calcium Exchange: Its Physiological Implications. Physiol. Rev. 79, 763–854. doi: 10.1152/physrev.1999.79.3.763
Echavarria, N. G., Echeverria, L. E., Stewart, M., Gallego, C., Saldarriaga, C. (2021). Chagas Disease: Chronic Chagas Cardiomyopathy. Curr. Probl. Cardiol. 46, 100507. doi: 10.1016/j.cpcardiol.2019.100507
Eltit, J. M., Ding, X., Pessah, I. N., Allen, P. D., Lopez, J. R. (2013). Nonspecific Sarcolemmal Cation Channels are Critical for the Pathogenesis of Malignant Hyperthermia. FASEB J. 27, 991–1000. doi: 10.1096/fj.12-218354
Everts, M. E., Ording, H., Hansen, O., Nielsen, P. A. (1992). Ca(2+)-ATPase and Na(+)-K(+)-ATPase Content in Skeletal Muscle From Malignant Hyperthermia Patients. Muscle Nerve 15, 162–167. doi: 10.1002/mus.880150206
Freichel, M., Berlin, M., Schurger, A., Mathar, I., Bacmeister, L., Medert, R., et al. (2017). “TRP Channels in the Heart,” in Neurobiology of TRP Channels. Ed. Emir, T. L. R.(Boca Raton (FL: CRC Press/Taylor & Francis), 149–185.
Gray, R. P., Mcintyre, H., Sheridan, D. S., Fry, C. H. (2001). Intracellular Sodium and Contractile Function in Hypertrophied Human and Guinea-Pig Myocardium. Pflugers Arch. 442, 117–123. doi: 10.1007/s004240000512
Houser, S. R., Margulies, K. B. (2003). Is Depressed Myocyte Contractility Centrally Involved in Heart Failure? Circ. Res. 92, 350–358.
Iwamoto, T., Kita, S. (2006). YM-244769, a Novel Na+/Ca2+ Exchange Inhibitor That Preferentially Inhibits NCX3, Efficiently Protects Against Hypoxia/Reoxygenation-Induced SH-SY5Y Neuronal Cell Damage. Mol. Pharmacol. 70, 2075–2083. doi: 10.1124/mol.106.028464
Iwamoto, T., Watano, T., Shigekawa, M. (1996). A Novel Isothiourea Derivative Selectively Inhibits the Reverse Mode of Na+/Ca2+ Exchange in Cells Expressing NCX1. J. Biol. Chem. 271, 22391–22397. doi: 10.1074/jbc.271.37.22391
Liao, R., Jain, M. (2007). Isolation, Culture, and Functional Analysis of Adult Mouse Cardiomyocytes. Methods Mol. Med. 139, 251–262. doi: 10.1007/978-1-59745-571-8_16
Lopez, J. R., Alamo, L., Caputo, C., Dipolo, R., Vergara, S. (1983). Determination of Ionic Calcium in Frog Skeletal Muscle Fibers. Biophys. J. 43, 1–4. doi: 10.1016/S0006-3495(83)84316-1
Lopez, J. R., Contreras, J., Linares, N., Allen, P. D. (2000). Hypersensitivity of Malignant Hyperthermia-Susceptible Swine Skeletal Muscle to Caffeine is Mediated by High Resting Myoplasmic [Ca2+]. Anesthesiology 92, 1799–1806. doi: 10.1097/00000542-200006000-00040
Lopez, J. R., Espinosa, R., Landazuru, P., Linares, N., Allen, P., Mijares, A. (2011). [Dysfunction of Diastolic [Ca(2)(+)] in Cardiomyocytes Isolated From Chagasic Patients]. Rev. Esp. Cardiol. 64, 456–462.
Lopez, J. R., Kolster, J., Zhang, R., Adams, J. (2017). Increased Constitutive Nitric Oxide Production by Whole Body Periodic Acceleration Ameliorates Alterations in Cardiomyocytes Associated With Utrophin/Dystrophin Deficiency. J. Mol. Cell Cardiol. 108, 149–157. doi: 10.1016/j.yjmcc.2017.06.004
Marban, E., Rink, T. J., Tsien, R. W., Tsien, R. Y. (1980). Free Calcium in Heart Muscle at Rest and During Contraction Measured With Ca2+ -Sensitive Microelectrodes. Nature 286, 845–850. doi: 10.1038/286845a0
Mijares, A., Altamirano, F., Kolster, J., Adams, J. A., Lopez, J. R. (2014). Age-Dependent Changes in Diastolic Ca(2+) and Na(+) Concentrations in Dystrophic Cardiomyopathy: Role of Ca(2+) Entry and IP3. Biochem. Biophys. Res. Commun. 452, 1054–1059. doi: 10.1016/j.bbrc.2014.09.045
Mijares, A., Espinosa, R., Adams, J., Lopez, J. R. (2020). Increases in [IP3]i Aggravates Diastolic [Ca2+] and Contractile Dysfunction in Chagas’ Human Cardiomyocytes. PLoS Negl. Trop. Dis. 14, e0008162. doi: 10.1371/journal.pntd.0008162
O’Daly, J. A., Simonis, S., de Rolo, N., Caballero, H. (1984). Suppression of Humoral Immunity and Lymphocyte Responsiveness During Experimental Trypanosoma Cruzi Infections. Rev. Inst. Med. Trop. 26(2):67–77. doi: 10.1590/s0036-46651984000200001
Piacentino, V., 3rd, Weber, C. R., Chen, X., Weisser-Thomas, J., Margulies, K. B., Bers, D. M., et al. (2003). Cellular Basis of Abnormal Calcium Transients of Failing Human Ventricular Myocytes. Circ. Res. 92, 651–658. doi: 10.1161/01.RES.0000062469.83985.9B
Rassi, A., Jr., Rassi, A., Marin-Neto, J. A. (2010). Chagas Disease. Lancet 375, 1388–1402. doi: 10.1016/S0140-6736(10)60061-X
Santos-Miranda, A., Joviano-Santos, J. V., Sarmento, J. O., Costa, A. D., Soares, A. T. C., Machado, F. S., et al. (2021). A Novel Substrate for Arrhythmias in Chagas Disease. PloS Negl. Trop. Dis. 15, e0009421. doi: 10.1371/journal.pntd.0009421
Shannon, T. R., Pogwizd, S. M., Bers, D. M. (2003). Elevated Sarcoplasmic Reticulum Ca2+ Leak in Intact Ventricular Myocytes From Rabbits in Heart Failure. Circ. Res. 93, 592–594. doi: 10.1161/01.RES.0000093399.11734.B3
Smith, G. L., Eisner, D. A. (2019). Calcium Buffering in the Heart in Health and Disease. Circulation 139, 2358–2371. doi: 10.1161/CIRCULATIONAHA.118.039329
Stein, C., Migliavaca, C. B., Colpani, V., Rosa, P. R., Sganzerla, D., Giordani, N. E., et al. (2018). Amiodarone for arrhythmia in patients with Chagas disease: A systematic review and individual patient data meta-analysis. PLoS Negl Trop Dis 12 (8), e0006742. doi: 10.1371/journal.pntd.0006742
Teixeira, A. R., Hecht, M. M., Guimaro, M. C., Sousa, A. O., Nitz, N. (2011). Pathogenesis of Chagas’ Disease: Parasite Persistence and Autoimmunity. Clin. Microbiol. Rev. 24, 592–630. doi: 10.1128/CMR.00063-10
Uryash, A., Mijares, A., Esteve, E., Adams, J. A., Lopez, J. R. (2021a). Cardioprotective Effect of Whole Body Periodic Acceleration in Dystrophic Phenotype Mdx Rodent. Front. Physiol. 12, 658042. doi: 10.3389/fphys.2021.658042
Uryash, A., Mijares, A., Flores, V., Adams, J. A., Lopez, J. R. (2021b). Effects of Naringin on Cardiomyocytes From a Rodent Model of Type 2 Diabetes. Front. Pharmacol. 12, 719268. doi: 10.3389/fphar.2021.719268
Velasco, A., Morillo, C. A. (2020). Chagas Heart Disease: A Contemporary Review. J. Nucl. Cardiol. 27, 445–451. doi: 10.1007/s12350-018-1361-1
Watano, T., Kimura, J., Morita, T., Nakanishi, H. (1996). A Novel Antagonist, No. 7943, of the Na+/Ca2+ Exchange Current in Guinea-Pig Cardiac Ventricular Cells. Br. J. Pharmacol. 119, 555–563. doi: 10.1111/j.1476-5381.1996.tb15708.x
Keywords: chagas disease, Na/Ca exchanger, cardiomyopathy, Trypanosoma cruzi (T cruzi), calcium
Citation: Lopez JR, Linares N, Adams JA and Mijares A (2022) The Role of the Na+/Ca2+ Exchanger in Aberrant Intracellular Ca2+ in Cardiomyocytes of Chagas-Infected Rodents. Front. Cell. Infect. Microbiol. 12:890709. doi: 10.3389/fcimb.2022.890709
Received: 06 March 2022; Accepted: 03 June 2022;
Published: 07 July 2022.
Edited by:
Vilma G. Duschak, Consejo Nacional de Investigaciones Científicas y Técnicas (CONICET), ArgentinaReviewed by:
Veronica Jimenez, California State University, Fullerton, United StatesSergio Schenkman, Federal University of São Paulo, Brazil
Copyright © 2022 Lopez, Linares, Adams and Mijares. This is an open-access article distributed under the terms of the Creative Commons Attribution License (CC BY). The use, distribution or reproduction in other forums is permitted, provided the original author(s) and the copyright owner(s) are credited and that the original publication in this journal is cited, in accordance with accepted academic practice. No use, distribution or reproduction is permitted which does not comply with these terms.
*Correspondence: Alfredo Mijares, bWlqYXJlc2FAZ21haWwuY29t