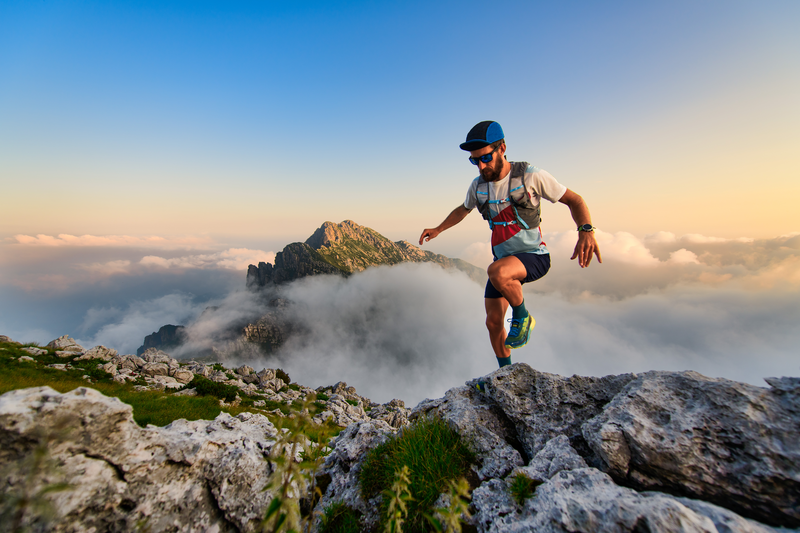
95% of researchers rate our articles as excellent or good
Learn more about the work of our research integrity team to safeguard the quality of each article we publish.
Find out more
ORIGINAL RESEARCH article
Front. Cell. Infect. Microbiol. , 06 May 2022
Sec. Clinical Microbiology
Volume 12 - 2022 | https://doi.org/10.3389/fcimb.2022.882555
This article is part of the Research Topic Chagas Disease Novel Drug Targets and Treatments View all 11 articles
Chagas disease (CD), caused by the hemoflagellate protozoan Trypanosoma cruzi, affects more than six million people worldwide and presents an unsatisfactory therapy, based on two nitroderivatives, introduced in clinical medicine for decades. The synthetic peptide, with CTHRSSVVC sequence (PepA), mimics the CD163 and TNF-α tripeptide “RSS” motif and binds to atheromatous plaques in carotid biopsies of human patients, spleen tissues, and a low-density lipoprotein receptor knockout (LDLr−/−) mouse model of atherosclerosis. CD163 receptor is present on monocytes, macrophages, and neutrophils, acting as a regulator of acute-phase processes and modulating aspects of the inflammatory response and the establishment of infections. Due to the potential theranostic role of PepA, our aim was to investigate its effect upon T. cruzi infection in vitro and in vivo. PepA and two other peptides with shuffled sequences were assayed upon different binomials of host cell/parasite, including professional [as peritoneal mouse macrophages (PMM)] and non-professional phagocytes [primary cultures of cardiac cells (CM)], under different protocols. Also, their impact was further addressed in vivo using a mouse model of acute experimental Chagas disease. Our in-vitro findings demonstrate that PepA and PepB (the peptide with random sequence retaining the “RS” sequence) reduced the intracellular parasitism of the PMM but were inactive during the infection of cardiac cells. Another set of in-vitro and in-vivo studies showed that they do not display a trypanocidal effect on bloodstream trypomastigotes nor exhibit in-vivo efficacy when administered after the parasite inoculation. Our data report the in-vitro activity of PepA and PepB upon the infection of PMM by T. cruzi, possibly triggering the microbicidal arsenal of the host professional phagocytes, capable of controlling parasitic invasion and proliferation.
According to the World Health Organization (WHO), neglected tropical diseases (NTDs) are communicable diseases that lack attention, care, and investments in areas of research and development of vaccines and medicines, affecting very poor communities with impaired public health access to early diagnosis and treatment (WHO, 2022).
Among the list of 20 NTDs, Chagas disease (CD), also known as American trypanosomiasis, affects more than six million people worldwide and is responsible for about 10,000 deaths per year (Sales Junior et al., 2017).
The etiological agent of CD is the protozoan Trypanosoma cruzi that presents different forms in both vertebrate and invertebrate hosts and that can be transmitted by distinct pathways, including via a vector insect and the oral route (Chatelain, 2017; Lidani et al., 2019). CD has two phases, acute and chronic, and since its discovery in 1909 by Carlos Chagas, it poses as a serious public health problem (WHO, 2022). Vaccines are not available and then control and treatment are exclusively related to drug therapy based on two very old pharmacological entities—benznidazole (Bz) and nifurtimox (NF)—introduced in clinical use for more than five decades (Soeiro and de Castro, 2009; Soeiro and de Castro, 2011; Soeiro, 2022). Both nitroderivatives have serious drawbacks including their limited efficacy, especially in the later chronic phase, and severe side effects with discontinuation (about 20%) of treatment (Soeiro and de Castro, 2011; Vieira et al., 2019; de Araújo et al., 2020).
Different experimental therapeutic approaches have been pursued aiming to find new drug candidates to face this sad scenario. The search includes several strategies such as i) repositioning studies of drugs already available in the market for other illness conditions, ii) identification of new antiparasitic agents using diverse libraries (composed of natural and synthetic products), iii) design and synthesis of new chemical entities selectively directed to molecular targets as well as iv) combined therapy, and v) molecular hybridization (hybrid compounds) combining two molecules (or parts of them) in a single new chemical entity to act on multiple targets of interest (Scarim et al., 2018; Simões-Silva et al., 2019; Soeiro, 2022).
The knowledge regarding the mechanisms involved in the pathological manifestations of CD has still not been fully gained (Soeiro, 2022). This knowledge could bring relevant contributions to the identification of more selective and complementary therapy for CD. In fact, despite being able to control and reduce the parasitic load in the acute phase, the host immune response and triggered inflammation are not able to fully eliminate the infection, and a progressive unbalanced inflammatory response (triggered by residual parasitism) is associated with the deteriorated conditions and may represent an important target for CD therapy (Benvenuti et al., 2017; Soeiro, 2022).
An interesting study demonstrated the ability of a peptide sequence—CTHRSSVVC (PepA), which mimics the CD163 molecule tripeptide “RSS” motif (Etzerodt et al., 2014) to bind inflammatory foci present in atheroma plaques in carotid biopsies of human patients, spleen tissues, and a low-density lipoprotein receptor knockout (LDLr−/−) mouse model of atherosclerosis (Silva et al., 2016). CD163 is present on professional phagocytes such as monocytes, macrophages, and neutrophils; eliminates hemoglobin and haptoglobin complexes; and acts as an acute-phase controller. Moreover, it plays a role in modulating inflammatory responses and the establishment of infections (Fabriek et al., 2009). In-vitro studies showed that the tracer [18F]AlF-NODAMP-C6-CTHRSSVVC binds CD163 receptors allowing a clear visualization of cancer cells using positron emission tomography (PET), although it was not able to detect subtle differences in CD163 levels of tumors induced by different treatments (Fernandes et al., 2021). No selective binding of the sulfo-Cy5-CTHRSSVVC peptide into macrophages could be noticed in vitro, but this tracer accumulates in a 4T1 tumor-bearing BALB/c mice model (Kovacs et al., 2022). Besides CD163, the RSS motif is likewise present in TNF-α (Etzerodt et al., 2014; Riethmueller et al., 2016; Zunke and Rose-John, 2017), stimulating its analysis in additional theranostic approaches (Silva et al., 2016; Fernandes et al., 2021; Kovacs et al., 2022).
Thus, as these previous reports suggest the potential theranostic role of PepA, our aim was to investigate its effect upon T. cruzi infection in vitro and in vivo. PepA and PepB (the peptide with random sequence retaining the “RS” sequence) reduced the intracellular parasitism of peritoneal mouse macrophages but were inactive during the infection of cardiac cells. Additional in-vitro and in-vivo studies showed that they do not display a trypanocidal effect on bloodstream trypomastigotes nor exhibit in-vivo efficacy when administered in a mouse model of acute T. cruzi infection after the parasite inoculation. The bulk of our data demonstrates the in-vitro activity of PepA and PepB upon the infection of PMM by T. cruzi, possibly triggering the microbicidal arsenal of the host professional phagocytes, capable of controlling parasitic invasion and proliferation.
Peptide sequences (Figure 1) were synthesized by the Chinese Peptides Company (Hangzhou, China) and fully characterized by nuclear magnetic resonance (NMR); carbon, hydrogen, and nitrogen (C, H, N) composition analyses; and MALDI/FAB mass spectrometry as reported (Silva et al., 2016). Stock solutions were prepared at 6 mM in sterile deionized water. The original sequence (CTHRSSVVC) which mimics the “RSS” motif of the CD163 molecule was named peptide A (PepA), the random sequence retaining the “RS” sequence of peptide A was called peptide B (CGRSKAMFC, PepB), and a scrambled sequence of peptide A was named peptide C (CHVSVRTSC, PepC). The reference compound for trypanocidal activity was the nitroderivative benznidazole [N-benzyl-2-(2-nitroimidazol-1-yl) acetamide2-nitroimidazole, Bz] (Laboratório Farmacêutico do Estado de Pernambuco, Brazil) (Figure 1), which was prepared in a stock concentration of 50 mM diluted in dimethylsulfoxide (100% DMSO), with the final in-test concentration never exceeding 0.6% for in-vitro experiments to avoid non-specific toxicity. For in-vivo assays, the peptides were diluted daily and prepared in sterile and deionized water. The reference drug was diluted in a solution composed of sterile and deionized water with 3% of Tween 80 (Guedes-da-Silva et al., 2016).
Bloodstream trypomastigote forms of the Y strain of T. cruzi were obtained from male Swiss Webster mice at the peak of parasitemia by cardiac puncture (Meirelles et al., 1986; Batista et al., 2010).
Primary cultures of mouse embryonic cardiac cells (CM) were obtained as described (Meirelles et al., 1986). CM were seeded into 24-well plates with 50 × 103 cells per well. Peritoneal mouse macrophages (PMM) were obtained by peritoneal lavage of male Swiss mice (Santos et al., 2020). PMM were plated into 96-well plates (50 × 103 cells per well) and 24-well plates (30 × 104 cells per well). All cultures were maintained at 37°C/5% CO2.
The toxicity of the peptides was investigated upon PMM using the alamarBlue test (Invitrogen, Waltham, MA, USA) according to the manufacturer’s recommendations (Timm et al., 2014; Guedes-da-Silva et al., 2016). PMM were treated for 48 h with increasing concentrations of the tested peptides (up to 500 µM). Following the exposition period, the colorimetric test was performed with alamarBlue® (cell viability detection test) and absorbance was determined by a spectrophotometer (570 and 600 nm). All assays were performed in triplicate in at least two individual assays (Timm et al., 2014; Guedes-da-Silva et al., 2016).
The effect of the peptides on intracellular forms in PMM was performed under the following protocols: i) pretreatment: PMM were rinsed and incubated for 24 h with different concentrations of the tested peptides and with Bz up to a maximum concentration of 50 μM. After incubation, the culture medium was replaced to remove the tested compounds and PMM were infected for 2 h with trypomastigote forms of T. cruzi (Y strain, M.O.I. 10 parasites:1 cell). Then, cultures were rinsed to remove the non-internalized parasites and incubated for 48 h at 37°C with RPMI culture medium. ii) Posttreatment: PMM were infected for 2 h with T. cruzi (Y strain, M.O.I. 10 parasites:1 cell), rinsed, and incubated for 24 h at 37°C. PMM were then incubated for 48 h at 37°C with increasing concentrations (up to 50 μM) of the peptides and Bz.
The effect of the peptides on intracellular forms of T. cruzi present in CM was performed as follows: i) pretreatment: CM cultures were incubated for 24 h with different concentrations of the peptides and Bz (up to 50 μM). Then, the cultures were rinsed to remove the compounds and infected for 24 h with bloodstream trypomastigote forms of T. cruzi (Y strain, M.O.I. 10 parasites:1 cell). After infection, the samples were rinsed to remove non-internalized parasites and incubated for 48 h at 37°C/5% CO2. ii) Posttreatment: CM were infected with bloodstream trypomastigote forms (Y strain, M.O.I. 10:1), and after 24 h, the cultures were washed to remove non-internalized parasites and incubated for 48 h at 37°C/5% CO2 with increasing concentrations of peptides (up to 50 μM).
After the different assays, PMM and CM were rinsed with phosphate-buffered saline (PBS) and fixed with Bouin solution. Then, the samples were stained with Giemsa for quantification (under light microscopy) of the percentage of infected host cells, the number of parasites per cell to calculate the infection index (multiplication between these two factors). Infected cultures not subjected to the treatments were used as negative controls (Guedes da Silva et al., 2016).
All assays were performed in duplicates in at least two individual assays (Timm et al., 2014; Guedes-da-Silva et al., 2016).
Bloodstream trypomastigotes (Y strain) were incubated with the tested peptides and Bz up to a concentration of 100 µM. After 2 and 24 h of incubation at 37°C, the number of live parasites (identified by their characteristic morphology and movement) was determined under a light microscope by quantification in a Neubauer chamber to determine the EC50 values. Controls were performed with parasites kept under the same conditions, in the absence of peptides (de Araújo et al., 2019).
In-vitro studies represent the analysis of two individual assays performed in duplicates.
Male Swiss Webster mice (18–20 g, 4–5 weeks of age) obtained from the animal facilities of the Institute of Science and Biomodels Technology (ICTB) FIOCRUZ were housed at a maximum of five per cage, kept in a specific-pathogen-free room at 20°C to 24°C under a 12-h light and 12-h dark cycle, and provided sterilized water and chow ad libitum. Male mice were used as previous findings showed that they are more vulnerable to experimental infection than females and thus more suitable for therapeutic screenings (Guedes et al., 2015). The animals were acclimated for 7 days before starting the experiments. Infection was performed by intraperitoneal (i.p.) injection of 104 bloodstream trypomastigotes (Y strain). Trypanosoma cruzi-infected mice were treated intraperitoneally with 0.1 ml of the tested peptides for up to 10 consecutive days, starting at 5 dpi, which corresponds to (in this experimental model) the parasitemia onset. Only mice with positive parasitemia were used in the infected groups. Benznidazole at 100 mg/kg/day (as the optimal dose) was run in parallel. Peptides were freshly prepared in sterile and distilled water, dosing according to the body weight of the animals. The experimental animal groups were divided as follows: uninfected (uninfected and untreated), untreated (infected and treated with vehicle only), treated with Bz (infected and treated with daily doses of 0.1 ml at 100 mg/kg, by gavage), and treated with peptides (infected and treated with daily doses of 0.1 ml of each peptide at 10 mg/kg). Parasitemia was performed individually by direct counting of the parasite in the blood (5 µl) under a light microscope. Mice were weighed once a week to monitor possible changes in body weight. Mortality was checked daily up to 30 days after treatment and expressed as the percentage of accumulated mortality (% CM) (Ferreira de Almeida Fiuza et al., 2018). In-vivo studies were performed twice using five animals per group (n = 5).
All procedures were carried out in accordance with the guidelines established by FIOCRUZ Committee of Ethics on Animal Experimentation (CEUA) number L-038/2017.
The statistical analysis was performed by analysis of variance (ANOVA), the data for the different assays were combined, and the significance was set at a p-value of ≤0.05 (Guedes-da-Silva et al., 2016).
Considering the importance of identifying non-toxic agents, the cytotoxic effect of the peptides on PMM was assessed using the alamarBlue test. The findings demonstrated that the tested peptides did not induce loss of cellular viability when PMM were incubated for 48 h (up to 500 µM), while Bz gave LC50 value = 333 ± 165 μM (Table 1).
Table 1 Determination of the cytotoxicity profile (LC50 values) of peptides (PepA, PepB, and PepC) and benznidazole (Bz) on the primary culture of peritoneal mouse macrophages (PMM).
The activity of the peptides and Bz upon the infection of PMM with T. cruzi [Y strain, discrete typing unit (DTU II)] was evaluated by counting the number of intracellular parasites and the percentage of infected host cells to determine the infection index (II) of Giemsa-stained samples (Figure 2). The assays were performed under two different protocols, namely, pre- and posttreatment, by the addition of the tested peptides before and after the establishment of the infection to verify their potential effect directly on the host cells and against the intracellular parasites, respectively (Tables 2, 3). The previous treatment of PMM with PepA and PepB resulted in lower parasite levels as compared with untreated samples, reaching decreases of 62% (p = 0.027) and 49% (p = 0.119), respectively, while PepC and Bz gave smaller (30% and 22%) and non-significant drops (p = 0.684 and p = 0.799), respectively (Tables 2, 3). After the establishment of the infection, PepA and PepB induced a non-significant effect on the parasite load, reaching 36% (p = 0.169) and 48% (p = 0.621) of decreases, respectively, while Bz reached 98% of decline (p = 0.066) (Table 2).
Figure 2 Light microscopy images of peritoneal mouse macrophages pretreated with PepA, PepB, and PepC at 50 µM before infection with Trypanosoma cruzi. (A) Untreated, (B) PepA, (C) PepB, and (D) PepC. Arrows: intracellular parasites.
Table 2 Activity of peptide A (PepA), peptide B (PepB), and benznidazole (Bz) on in-vitro infection of peritoneal mouse macrophages (PMM) by Trypanosoma cruzi (Y strain) submitted to pre- and posttreatment protocols.
Table 3 Activity of peptide C (PepC) and benznidazole (Bz) on in-vitro infection of peritoneal mouse macrophages (PMM) by Trypanosoma cruzi (Y strain) using pretreatment protocol: mean ± SD of the infection index (% of reduction).
The activity of PepA and PepB was additionally investigated using primary cultures of mouse cardiac cells infected with the Y strain. As depicted in Table 4, both peptides only caused modest and non-significant (p > 0.05) reductions in parasitism of CM, reaching maximum values of 35% inhibition of infection rates at the highest concentration (50 μM), while Bz resulted in 96% of decline (Table 4).
Table 4 Activity of peptides A and B and benznidazole (Bz) on in-vitro infection of cardiac cell cultures by Trypanosoma cruzi (Y strain) using pre- and posttreatment with peptides (50 µM) and Bz (5.5 µM) used as a reference drug.
To check the potential direct effect of the peptides upon the other relevant form of T. cruzi to mammalian infections, the activity of the peptides was assayed upon extracellular bloodstream trypomastigotes (Y strain) through light microscopy. The findings demonstrated that all peptides were inactive against bloodstream forms (EC50 > 100 µM), while Bz achieved likely potency (EC50 value = 12 µM) (Table 5).
Table 5 The biological effect of the peptides and benznidazole (EC50 values at µM) against bloodstream trypomastigotes of Trypanosoma cruzi (Y strain) after 2 and 24 h of incubation at 37°C.
Finally, in-vivo assays were conducted to explore the potential therapeutic effect of the peptides upon a mouse model of acute T. cruzi infection (Swiss mice infected with the Y strain), under administration for 5–10 consecutive days with 10 mg/kg/day, via the intraperitoneal route, starting at the parasitemia onset (5 dpi). Our findings (Figures 3A, B) show that all peptides displayed a maximum of 23% of parasitemia decline (8 dpi), while Bz (optimal dose of 100 mg/kg/day) fully suppressed (>99%) the parasitemia at 8 dpi, corresponding to the parasitemia peak (Figure 3B). Also, the ponderal curve demonstrates that all peptides were unable to protect against weight loss induced by T. cruzi infection, which was clearly reverted during Bz administration (Figure 3C). Indeed, only the reference drug gave 100% of animal survival, while all peptides reached 100% of cumulative death as soon as at 15 dpi, similar to the vehicle-treated mice group (Figure 3D).
Figure 3 Effect of peptides and benznidazole upon a mouse model of acute Trypanosoma cruzi infection. (A) Parasitemia curve, (B) data dispersion of blood parasitism at 8 days postinfection, (C) ponderal curve, and (D) cumulative mortality.
The peptide with CTHRSSVVC sequence (PepA) mimics the CD163 and TNF-α tripeptide “RSS” motif. The CD163 receptor is present on monocytes, macrophages, and neutrophils, acting as a regulator of acute-phase processes and modulating aspects of the inflammatory response and the establishment of infections (Etzerodt et al., 2014; Silva et al., 2016). Hence, due to previous reports regarding the theranostic role of PepA, our aim was to evaluate its effect upon T. cruzi experimental infection in vitro and in vivo. Besides PepA, two other sequences were assessed: one that maintains the “RS” region (PepB) and another that maintains all the amino acids in scrambled order to the original sequence (PepC). As CD163 is present in professional phagocytes, these peptides were screened in PMM by means of distinct protocols exploring the potential cytotoxic profile on mammalian cells as well as their effect on T. cruzi infection before (pretreatment) and after parasite interaction (posttreatment). Our data showed that all peptides showed a non-toxic profile, which is a desirable characteristic for new drug candidates (Gupta et al., 2015).
PepA and PepB reduced the infection indexes of T. cruzi-infected PMM reaching a statistically significant (p = 0.027) maximum decline of 62% when the CTHRSSVVC sequence was added prior to parasite interaction. Accordingly, PepC, the one with scrambled sequence, only reached a non-significant decrease of 30% when added prior to protozoan infection, arguing in favor of the specificity of the RS motif present in PepA and PepB, possibly triggering a microbicidal response induced by the RS sequence region. On the other hand, the lack of activity of the peptides on the trypomastigote forms suggests that the effect of PepA and PepB is on PMM metabolism rather than on the parasite itself due to their inability to lyse bloodstream forms.
No major effect was noticed when cardiac cell cultures were used as host cells, supporting the hypothesis that the sequence region “RS” of PepA and PepB may selectively activate professional phagocytes such as PMM by an immunomodulatory pathway. The lack of therapeutic activity against bloodstream trypomastigotes was also corroborated by in-vivo approaches demonstrating only a mild reduction of the parasitemia in mice treated with the peptides after T. cruzi infection. Although the “RSS” motif is a conserved sequence present only in human and monkey CD163, its soluble form (sCD163) is likewise detectable in mouse serum and is present in TNF-α (Etzerodt et al., 2014; Riethmueller et al., 2016; Zunke and Rose-John, 2017). Macrophages are notoriously heterogeneous. They can adopt a spectrum of phenotypes from the anti-inflammatory M2-type state (CD163) to the pro-inflammatory M1-type state (TNF-α). However, the inflammatory phenotype of the M1 versus M2 macrophage is not constant, which probably reflects the plasticity of monocyte-derived cells in response to microenvironmental changes (Sica et al., 2006; Moghaddam et al., 2018). In this context, their potential biological effect may depend on how the peptide will be presented for specific binding to occur and where they will bind. Since T. cruzi infection stimulates the activation of both M1 and M2 macrophages (Zanluqui et al., 2015), further studies will be necessary to evaluate the mode of action of PepA and PepB on T. cruzi experimental infection. In fact, in addition to CD163, the RSS motif is present in TNF-α and a soluble CD163 exists in mice, and further studies are necessary to reveal the mode of action of the peptides. These studies would include measurements of different inflammatory mediators in vitro besides evaluating the response upon the infection of M1 and M2 macrophage populations as well as the use of distinct in-vivo models and regimens (e.g., pretreatment of mice with peptide before parasite inoculation of murine models of acute and chronic infection). Moreover, other factors must be considered during the experimental assays such as peptide stability in vivo, levels of macrophage subpopulations, and different types and kinetics of inflammation in the acute and chronic models of T. cruzi infection (Silva et al., 2016; Fernandes et al., 2021; Kovacs et al., 2022).
The bulk of our findings suggests that the non-toxic profile of PepA and PepB along with their ability to diminish PMM parasitism in vitro justifies additional studies aiming to open new perspectives for alternative therapies for CD.
The raw data supporting the conclusions of this article will be made available by the authors, without undue reservation.
All procedures were carried out in accordance with the guidelines established by FIOCRUZ Committee of Ethics on Animal Experimentation (CEUA) number L-038/2017.
GL contributed to the in-vitro and in-vivo assays and wrote and edited the entire manuscript. DB contributed to the in-vivo assays and analyzed the data. AM contributed to the in-vivo assays. RS provided the peptides and reviewed the manuscript. AL provided the peptides and reviewed the manuscript. MS was responsible for the project and methodology design, analyzed the data, and edited and reviewed the manuscript. All authors contributed to the article and approved the submitted version.
The Carlos Chagas Filho Foundation for Research Support of the State of Rio de Janeiro (FAPERJ), National Council for Scientific and Technological Development (CNPq), Oswaldo Cruz Foundation, PAEF/CNPq/FIOCRUZ, PDTIS, and CAPES funded this study. MS is a research fellow from CNPq and CNE.
The authors declare that the research was conducted in the absence of any commercial or financial relationships that could be construed as a potential conflict of interest.
All claims expressed in this article are solely those of the authors and do not necessarily represent those of their affiliated organizations, or those of the publisher, the editors and the reviewers. Any product that may be evaluated in this article, or claim that may be made by its manufacturer, is not guaranteed or endorsed by the publisher.
The authors would like to thank to Dr. Alexis Murillo Carrasco, Dr. Lucas Freitas de Freitas, and Ludmila F. de A Fiuza (M.Sc.).
Batista, D. G., Batista, M. M., de Oliveira, G. M., do Amaral, P. B., Lannes-Vieira, J., Britto, C. C., et al. (2010). Arylimidamide DB766, A Potential Chemotherapeutic Candidate for Chagas’ Disease Treatment. Antimicrobial Agents Chemother 54 (7), 2940–2952. doi: 10.1128/AAC.01617-09
Benvenuti, L. A., Roggério, A., Cavalcanti, M. M., Nishiya, A. S., Levi, J. (2017). An Autopsy-Based Study of Trypanosoma Cruzi Persistence in Organs of Chronic Chagasic Patients and its Relevance for Transplantation. Transplant. Infect. Dis. 19 (6), e12783. doi: 10.1111/tid.12783
Chatelain, E. (2017). Chagas Disease Research and Development: Is There Light at the End of the Tunnel? Comput. Struct. Biotechnol. J. 15, 98–103. doi: 10.1016/j.csbj.2016.12.002
de Araújo, J. S., García-Rubia, A., Sebastián-Pérez, V., Kalejaiye, T. D., da Silva, P. B., Fonseca-Berzal, C. R., et al. (2019). Imidazole Derivatives as Promising Agents for the Treatment of Chagas Disease. Antimicrobial Agents Chemother 63 (4), e02156–e02118. doi: 10.1128/AAC.02156-18
de Araújo, J. S., da Silva, C. F., Batista, D. G. J., Nefertiti, A., de Almeida Fiuza, L. F., Fonseca-Berzal, C. R., et al. (2020). Efficacy of Novel Pyrazolone Phosphodiesterase Inhibitors in Experimental Mouse Models of Trypanosoma Cruzi. Antimicrobial Agents Chemother 64 (9), e00414–20. doi: 10.1128/AAC.00414-20
Etzerodt, A., Rasmussen, M. R., Svendsen, P., Chalaris, A., Schwarz, J., Galea, I., et al. (2014). Structural Basis for Inflammation-Driven Shedding of CD163 Ectodomain and Tumor Necrosis Factor-α in Macrophages. J. Biol. Chem. 289 (2), 778–788. doi: 10.1074/jbc.M113.520213
Fabriek, B. O., Bruggen, R. V., Deng, D. M., Ligtenberg, A. J. M., Nazmi, K., Schornagel, K., et al. (2009). The Macrophage Scavenger Receptor CD163 Functions as an Innate Immune Sensor for Bacteria. Blood 113 (4), 887–892. doi: 10.1182/blood-2008-07-167064
Fernandes, B.. (2021). Development of PET Tracers for M2-Macrophages. Univ. Groningen, 1–174. doi: 10.33612/diss.194780714
Ferreira de Almeida Fiuza, L., Peres, R. B., Simões-Silva, M. R., da Silva, P. B., Batista, D. G. J., da Silva, C. F., et al. (2018). Identification of Pyrazolo[3,4-E][1,4]Thiazepin Based CYP51 Inhibitors as Potential Chagas Disease Therapeutic Alternative: In Vitro and In Vivo Evaluation, Binding Mode Prediction and SAR Exploration. Eur. J. Med Chem. 149, 257–268. doi: 10.1016/j.ejmech.2018.02.020
Guedes-da-Silva, F. H., Batista, D. G. J., Meuser, M. B., Demarque, K. C., Fulco, T., de Araújo, J. S., et al. (2016). In Vitro and In Vivo Trypanosomicidal Action of Novel Arylimidamides Against Trypanosoma Cruzi. Antimicrobial Agents Chemother 60, 2425–2434. doi: 10.1128/AAC.01667-15
Guedes, F. H., Batista, D. G. J., da Silva, C. F., Meuser, M. B., Simões-Silva, M. R., de Araújo, J. S., et al. (2015). Antimicrob Agents Chemother. American Society for Microbiology 59, 12, 7564–7570. doi: 10.1128/AAC.01294-15
Gupta, S., Kapoor, P., Chaudhary, K., Gautam, A., Kumar, R., Raghava, G. O. S., et al. (2015). ‘Antimicrob Agents Chemother’, in Computational Peptidology, vol. pp . Eds. Zhou, P., Huang, J. (New York, NY: Springer New York (Methods in Molecular Biology), 143–157. doi: 10.1007/978-1-4939-2285-7_7
Kovacs, L., Davis, R. A., Ganguly, T., Chammas, R., Sutcliffe, J. L. (2022). Repurposing an Atherosclerosis Targeting Peptide for Tumor Imaging. BioMed. Pharmacother. 145, 112469. doi: 10.1016/j.biopha.2021.112469
Lidani, K. C. F., Andrade, F. A., Bavia, L., Damasceno, F. S., Beltrame, M. H., Messias-Razão, I. J., et al. (2019). Chagas Disease: From Discovery to a Worldwide Health Problem. Front. Public Health 7. doi: 10.3389/fpubh.2019.00166
Meirelles, M. N., de Araújo-Jorge, T. C., Miranda, C. F., de Souza, W., Barbosa, H. S. (1986). Interaction of Trypanosoma Cruzi With Heart Muscle Cells: Ultrastructural and Cytochemical Analysis of Endocytic Vacuole Formation and Effect Upon Myogenesis In Vitro. Eur. J. Cell Biol. 41 (2), 198–206.
Moghaddam, A. S., Mohammadian, S., Vazini, H., Taghadosi, M., Esmaeili, A. S., Mardani, F., et al. (2018). Macrophage Plasticity, Polarization and Function in Health and Disease. J. Cell Physiol 233, 6425–6440. doi: 10.1002/jcp.26429
Riethmueller, S., Ehlers, J. C., Lokau, J., Dusterhöft, S., Knittler, K., Dombrowsky, G., et al. (2016). Cleavage Site Localization Differentially Controls Interleukin-6 Receptor Proteolysis by ADAM10 and ADAM17. Sci. Rep 6, 25550. doi: 10.1038/srep25550
Sales Junior, P. A., Molina, I., Murta, S. M. F., Sánchez-Montalvá, A., Salvador, F., Corrêa-Oliveira, R., et al. (2017). Experimental and Clinical Treatment of Chagas Disease: A Review. Am. J. Trop. Med. Hygiene 97 (5), 1289–1303. doi: 10.4269/ajtmh.16-0761
Santos, C. C., Zhang, H., Batista, M. M., de Oliveira, G. M., Demarque, K. C., Silva Gomes, N., et al. (2020). In Vitro and In Vivo Evaluation of an Adamantyl-Based Phenyl Sulfonyl Acetamide Against Cutaneous Leishmaniasis Models of Leishmania Amazonensis. Antimicrobial Agents Chemother 64 (12), e01188–e01120. doi: 10.1128/AAC.01188-20
Scarim, C. B., Jornada, D. H., Chelucci, R. C., de Almeida, L., Santos, J. L., Chung, H. C., et al. (2018). Current Advances in Drug Discovery for Chagas Disease. Eur. J. Med Chem. 155, 824–838. doi: 10.1016/j.ejmech.2018.06.040
Sica, A., Schioppa, T., Mantovani, A., Allavena, P. (2006). Tumour-Associated Macrophages Are a Distinct M2 Polarised Population Promoting Tumour Progression: Potential Targets of Anti-Cancer Therapy. Eur. J. Cancer 42 (6), 717–727. doi: 10.1016/j.ejca.2006.01.003
Silva, R. A., Giordano, R. J., Gutierrez, P. S., Rocha, V. Z., Rudnicki, M., Kee, P., et al. (2016). CTHRSSVVC Peptide as a Possible Early Molecular Imaging Target for Atherosclerosis. Int. J. Mol. Sci. 17 (9), 1383. doi: 10.3390/ijms17091383
Simões-Silva, M. R., De Araújo, J. S., Peres, R. B., da Silva, P. B., Batista, M. M., De Azevedo, L. D., et al. (2019). Repurposing Strategies for Chagas Disease Therapy: The Effect of Imatinib and Derivatives Against Trypanosoma Cruzi. Parasitology 146 (8), 1006–1012. doi: 10.1017/S0031182019000234
Soeiro, M. N. C. (2022). Perspectives for a New Drug Candidate for Chagas Disease Therapy. Memórias Do Instituto Oswaldo Cruz 117, e220004. doi: 10.1590/0074-02760220004
Soeiro, M. N. C., de Castro, S. L. (2009). Trypanosoma Cruzi Targets for New Chemotherapeutic Approaches. Expert Opin. Ther. Targets 13 (1), 105–121. doi: 10.1517/14728220802623881
Soeiro, M. N. C., de Castro, S. L. (2011). Screening of Potential Anti-Trypanosoma Cruzi Candidates: In Vitro and In Vivo Studies. Open Med Chem. J. 5, 21–30. doi: 10.2174/1874104501105010021
Timm, B. L., da Silva, P. B., Batista, M. M., Guedes da Silva, F. H., da Silva, C. F., Tidwell, R. R., et al. (2014). In Vitro and In Vivo Biological Effects of Novel Arylimidamide Derivatives Against Trypanosoma Cruzi. Antimicrobial Agents Chemother 58 (7), 3720–3726. doi: 10.1128/AAC.02353-14
Vieira, J. L., Távora, F. R. F., Sobral, M. G. V., Vasconcelos, G. G., Almeida, G. P. L., Fernandes, J. R., et al. (2019). Chagas Cardiomyopathy in Latin America Review. Curr. Cardiol. Rep. 21 (2), 8. doi: 10.1007/s11886-019-1095
WHO (2022) World Health Organization. Available at: https://www.who.int/news-room/fact-sheets/detail/chagas-disease-(american-trypanosomiasis) (Accessed 9 February 2022).
Zanluqui, N. G., Wowk, P. F., Pinge-Filho, P. (2015). Macrophage Polarization in Chagas Disease. J Clin Cell Immunol 1–6. doi: 10.4172/2155-9899.1000317
Keywords: Chagas disease, Trypanosoma cruzi, experimental chemotherapy, CTHRSSVVC peptide, immunomodulation
Citation: Leite GR, Batista DdGJ, Mazzeti AL, Silva RA, Lugão AB and Soeiro MdNC (2022) The Impact of the CTHRSSVVC Peptide Upon Experimental Models of Trypanosoma cruzi Infection. Front. Cell. Infect. Microbiol. 12:882555. doi: 10.3389/fcimb.2022.882555
Received: 23 February 2022; Accepted: 07 April 2022;
Published: 06 May 2022.
Edited by:
Vilma G. Duschak, Consejo Nacional de Investigaciones Científicas y Técnicas (CONICET), ArgentinaReviewed by:
María Paula Faral-Tello, Institut Pasteur de Montevideo, UruguayCopyright © 2022 Leite, Batista, Mazzeti, Silva, Lugão and Soeiro. This is an open-access article distributed under the terms of the Creative Commons Attribution License (CC BY). The use, distribution or reproduction in other forums is permitted, provided the original author(s) and the copyright owner(s) are credited and that the original publication in this journal is cited, in accordance with accepted academic practice. No use, distribution or reproduction is permitted which does not comply with these terms.
*Correspondence: Maria de Nazaré Correia Soeiro, c29laXJvQGlvYy5maW9jcnV6LmJy
Disclaimer: All claims expressed in this article are solely those of the authors and do not necessarily represent those of their affiliated organizations, or those of the publisher, the editors and the reviewers. Any product that may be evaluated in this article or claim that may be made by its manufacturer is not guaranteed or endorsed by the publisher.
Research integrity at Frontiers
Learn more about the work of our research integrity team to safeguard the quality of each article we publish.