- Institute for Biomedical Sciences, Georgia State University, Atlanta, GA, United States
Gonorrhea is a global health concern. Its etiological agent, Neisseria gonorrhoeae, rapidly acquires antimicrobial resistance and does not confer protective immunity as a consequence of infection. Attempts to generate an effective vaccine for gonorrhea have thus far been unsuccessful, as many structures on the bacterial envelope have the propensity to rapidly change, thus complicating recognition by the human immune system. In response to recent efforts from global health authorities to spur the efforts towards development of a vaccine, several new and promising steps have been made towards this goal, aided by advancements in computational epitope identification and prediction methods. Here, we provide a short review of recent progress towards a viable gonococcal vaccine, with a focus on antigen identification and characterization, and discuss a few of the tools that may be important in furthering these efforts.
Introduction
Neisseria gonorrhoeae (Ngo) causes the common sexually-transmitted infection (STI) gonorrhea. Since its first isolation in the late nineteenth century, Ngo has become an increasingly urgent global health threat. Ngo afflicted an estimated 87 million people in 2016 (Rowley et al., 2019), and the organism has to date developed resistance to every class of antimicrobial drug used for treatment (Unemo et al., 2019). Gonorrhea infects both men and women. In men, complications can include prostatitis, urethritis, and epididymitis, and in women pelvic inflammatory disease, infertility, or ectopic pregnancy. Infection by, and subsequent clearance of Ngo does not confer a protective immune response (Liu et al., 2011), so reinfection is common. Progress towards an effective gonococcal vaccine has been slow, as the propensity of Ngo for high-frequency phase and antigenic variation makes identification of suitable immunogens difficult (Criss et al., 2005; Zhu et al., 2011; Jerse et al., 2014). Further complicating matters is the fact that correlates of protection for gonorrhea are unknown (Russell et al., 1999). In response to these difficulties, global health authorities have redoubled efforts towards the development of an effective vaccine for gonorrhea prevention. This limited review will discuss early trials of gonococcal vaccines, recent breakthroughs in characterization of novel vaccine targets, and the emergence of bioinformatic tools which aid in this venture. In addition, it will briefly cover studies that have investigated cross protection against gonorrhea generated by meningococcal vaccines. For a more comprehensive review of gonorrhea vaccine development, including epidemiological and vaccine impact information, host-pathogen interactions, animal models, and considerations for other STI pathogens, the reader is directed towards recent reviews by Broutet et al. (2014), Russell et al. (2019) and Gottlieb et al. (2020).
Previous Vaccine Attempts
Previous attempts to generate an effective gonococcal vaccine have been unsuccessful. As mentioned, the propensity for Ngo to alter the antigenic identity and expression levels of surface structures has made identification of vaccine targets challenging. To date only two candidate gonococcal vaccines have reached human trials. One candidate targeted pilin, the major component of the type IV pilus (Boslego et al., 1991), though no difference in protection was seen between placebo and vaccinated groups. It is likely that antigenic variability of pilin contributed to protection failures in this trial (Tramont and Boslego, 1985). The other trial utilized heat-killed, partially lysed whole gonococcal cells that, while able to generate bactericidal antibodies in a high percentage of recipients, did not show long-term protection; within one year post-vaccination, similar numbers of subjects were infected with Ngo regardless of their vaccination status (Greenberg et al., 1974; Greenberg, 1975). Despite these failures, other immunization efforts have shown that it is at least possible to reduce susceptibility to gonococcal infection in chimpanzees (Arko et al., 1976). This study suggested that natural infection generates some level of acquired immunity in cases of gonococcal urethritis or pharyngitis, as previously colonized chimps required a significantly higher bacterial dose to achieve reinfection. However, protection waned over time, and immunity was not seen when previously “immune” chimps were reinfected years later. Finally, the outer-membrane porin (PorB) has received considerable attention as a vaccine antigen due to its roles in adherence and dissemination. For example, Zhu et al. formulated with PorB as a DNA-based vaccine (Zhu et al., 2004), which expressed porB from plasmid, and as recombinant protein alone or presented in viral replicon particles (Zhu et al., 2005). The DNA-based vaccine was able to induce both a Th1 and Th2 response, depending on method of delivery, and the viral replicon particle-based method showed some protective capability in mice. Prior to this, an animal trial was conducted comparing porin formulated in liposomes, proteosomes, and gonococcal membrane blebs (Wetzler et al., 1992). This study found that porin delivered in liposomes, which mimics the in vivo structure, generated greater numbers of anti-porin antibodies, including those that recognized surface-exposed sections of porin, compared to other delivery methods. However, this study did not proceed to clinical trials. Nevertheless, progress towards a gonococcal vaccine continues, and new, promising targets for vaccination are being identified.
TonB-Dependent Transporters
Ngo produces eight TonB-dependent transporters (TdTs), which are integral outer-membrane β-barrel transporters. Of these, three are aided by an associated lipid-modified protein tethered to the outer membrane. The TdTs play a critical role in allowing Ngo to survive in the nutrient-depleted environment encountered within the human host by allowing the pathogen access to transition metals such as iron and zinc. Upon binding their host ligands, which include iron-sequestering proteins like transferrin and lactoferrin, and zinc-sequestering proteins such as S100 proteins, the TdTs liberate their bound metal ions. The metals are then transported through the TdT barrel into the periplasm - aided by the proton motive force - and are intercepted by a cognate ABC transporter that translocates the metal into the cytoplasm (Cornelissen and Hollander, 2011; Cornelissen, 2018). Critically, the TdTs are well-conserved across Ngo and Neisseria meningitidis (Nme) isolates, and only a few are capable of phase variation, making them promising targets for vaccination efforts. The TdTs are in many cases capable of eliciting an antibody response (Price et al., 2004; Stork et al., 2010), and they play critical roles in Ngo survival and infection (Cornelissen et al., 1998; Hagen and Cornelissen, 2006; Calmettes et al., 2015; Jean et al., 2016). Despite their promise, it is feasible that the binding of host ligands during metal piracy may generate a quasi-mimicry of self-antigens, thus contributing to immune evasion. Interestingly, however, Frandoloso et al. showed that a nonbinding mutant of the surface lipoprotein TbpB in Haemophilus parasuis, which cannot interact with transferrin but still retains the overall structure of the wildtype, can generate an enhanced protective response compared to native TbpB in a porcine challenge model (Frandoloso et al., 2015). While it is unclear whether this phenomenon is transferable to the transporters themselves, Cash et al. found that mutagenesis of the gonococcal TdT TbpA could abrogate human transferrin binding without appreciable disruption of the overall TbpA structure (Cash et al., 2015). As generation of a nonbinding Ngo TbpA appears possible, using it as an immunogen to test protection relative to the wildtype may be a promising way forward. Indeed, as researchers gain more insight into other TdT/host protein pairs (Jean et al., 2016; Maurakis et al., 2019) and further characterize TdT structures and binding propensities (Sikora et al., 2018; Yadav et al., 2019; Kammerman et al., 2020), similar studies with the other TdTs are feasible.
Despite these advantages, however, large-scale production of integral transmembrane proteins such as the TdTs presents a few technical limitations that may impact commercial vaccine endeavors. 1) Such proteins are produced at relatively low levels in membranes, which necessarily slows production times. 2) Stabilization and solubilization of these proteins requires highly-optimized detergent concentrations. 3) Purification directly from membranes requires specific expertise and equipment that is not necessary for purification from the cytoplasm. To address these issues, Fegan et al. (2019) utilized an innovative approach to generate a TbpA/B hybrid antigen. This novel antigen utilizes the C-lobe of the immunogenic lipoprotein TbpB as a soluble protein “scaffold” onto which putatively immunogenic extracellular loop sections of TbpA are transplanted in place of TbpB loops. Antisera raised against these hybrid antigens recognized surface-exposed TbpA of Nme and was capable of inhibiting transferrin utilization by gonococci. Previously, Price et al. generated a similar hybrid by fusing Ngo TbpA loop 2 to the N-terminal lobe of TbpB, using the A2 domain of cholera toxin as a hosting molecule. These chimeras generated bactericidal and growth-inhibitory antibodies against Ngo (Price et al., 2007). Taken together, these studies suggest hybrid antigens as a promising solution to the technical limitations imposed by the TdTs.
Lipooligosaccharide (LOS)
Gonococcal LOS has garnered attention recently as a feasible vaccine candidate [fully reviewed in (Gulati et al., 2019b)]. While LOS is subject to phase variation (Apicella et al., 1987), it is easily accessible on the gonococcal surface, where it is present in abundance. Furthermore, while many LOS antigens share structures with human glycosphingolipids thus making them ineligible for targeting, two distinct LOS epitopes, recognized by mAb L8 and mAb 2C7 (Gulati et al., 1996; Yamasaki et al., 1999), do not suffer this pitfall. A recent study (Ram et al., 2018) showed that the 2C7 epitope, which is widely conserved amongst gonococci, is immunogenic during natural infection. As such, the 2C7 epitope has attracted attention as a vaccine target. Due to the complications of purifying or directly synthesizing oligosaccharide structures, along with their generally poor immunogenicity, an attractive prospect for utilizing such antigens is to create a peptide mimic – a so-called “mimitope” – with a highly similar structure to the natural oligosaccharide. For 2C7, this was successfully done in 2006 (Ngampasutadol et al., 2006), and continuing work showed that immunization with this mimitope alongside a TH1-stimulating adjuvant resulted in generation of bactericidal IgG, reduced gonococcal colonization, and sped bacterial clearance in experimentally infected mice (Gulati et al., 2019a). As LOS plays a key role in Ngo’s pathogenic lifestyle and a 2C7-based vaccine would ostensibly offer broad cross-reactivity against gonococcal strains, this represents an important step forward in the quest for gonococcal prevention.
Meningococcal Vaccines and OMVs
Vaccines against Nme serogroup B have been suggested to confer protection against gonococcal infections in addition to their intended meningococcal role. Various observational studies noted a decrease in gonococcal infections in regions after administration of meningococcal group B outer-membrane vesicle (OMV) vaccines (Whelan et al., 2016; Longtin et al., 2017; Ochoa-Azze, 2018), perhaps owing to the high level of genetic similarity between Ngo and Nme. In a case-control study of the Nme group B OMV vaccine administered in New Zealand, MeNZB (Arnold et al., 2011), researchers estimated the vaccine to be approximately 31% effective for gonococcal cross-protection, with effectiveness waning over time (Petousis-Harris et al., 2017). Bioinformatic analyses have compared MeNZB, which is no longer on the market, to the currently-available Nme group B vaccine Bexsero® (GSK), which contains the MeNZB OMV antigen alongside Neisseria adhesin A (NadA), factor H binding protein (fHbp), and Neisseria heparin binding antigen (NHBA) (Toneatto et al., 2017). These antigens were also compared to their homologous gonococcal proteins, and assessed for their ability to generate anti-gonococcal antibodies (Semchenko et al., 2019). These analyses found that Ngo strain FA1090 encodes homologues to 20 of 22 core proteins from the Bexsero OMV, of which 16 share >90% identity with the vaccine antigen. While the nadA gene is absent from Ngo and fHbp is not believed to surface exposed in Ngo, NHBA from FA1090 is approximately 69% identical to the Bexsero variant. Furthermore, this study showed that OMV-derived antibodies can recognize gonococcal proteins, and others demonstrated that immunization with Bexsero® accelerates Ngo clearance in a mouse challenge model (Leduc et al., 2020).
OMVs are bi-layered membrane spheres that are naturally released from Gram-negative bacteria, including Ngo, and have received considerable attention as vaccine antigens for STI pathogens (Chbib et al., 2021). Their surface is decorated with phospholipids, various outer-membrane proteins, and lipopolysaccharide/lipooligosaccharide (Kulp and Kuehn, 2010). Many common OMV proteins in addition to those discussed above share similarity between Ngo and Nme (Marjuki et al., 2019), making OMVs a promising platform for a universal pathogenic Neisseria vaccine. For Ngo, an OMV preparation with microencapsulated IL-12 as an adjuvant was recently shown to accelerate gonococcal clearance and to induce Ngo-specific antibodies when administered intravaginally to BALB/c mice (Liu et al., 2017). Critically, this combination conferred protection against heterologous Ngo strains (Liu et al., 2018), which is necessary for effective vaccination. In another study, an OMV from Nme that was modified to overexpress a mutant fHbp and an attenuated endotoxin was able to elicit human complement-mediated serum bactericidal antibodies against Ngo (Beernink et al., 2019). More recently, detoxified Nme OMVs as immunogens demonstrated a protective effect against gonococcal challenge in mice, which was associated with anti-OMV immunoglobulins that cross-reacted with Ngo (Matthias et al., 2021).
NHBA and Bacterial Ghosts
The previous section briefly discussed NHBA as a component of the Nme Bexsero® vaccine, but this antigen has also independently received attention as a contributor to protection against gonococci. Ngo NHBA is surface exposed and is involved in interactions with host cells (Semchenko et al., 2019). Furthermore, this antigen is conserved across Ngo genomes and has a high sequence identity among isolates. It was recently shown that recombinant NHBA is immunogenic and that anti-NHBA antibodies recognize a wide range of Ngo NHBA variants (Semchenko et al., 2020a). In addition, these antibodies promote C3 deposition and opsonophagocytosis. The biological importance of NHBA should not be overlooked, as it plays a role in formation of gonococcal microcolonies and adherence to epithelial cells (Semchenko et al., 2020b).
An interesting adjunct to vaccination platforms is that of the bacterial ghost. Ghosts are empty shells of Gram-negative bacteria produced from the expression of bacteriophage PhiX174 lysis gene E (Langemann et al., 2010). Expression of gene E in non-host-range bacteria for PhiX174 leads to conversion of Gram-negative bacteria into ghosts, rather than causing lysis. Critically, these ghosts expel their nucleic acids, cytoplasmic proteins, and ribosomes during formation, leaving behind a fused outer and inner membrane with an antigenic profile identical to that of the counterpart bacteria. As a result, these ghosts can be utilized for immunization efforts involving envelope antigens, and can even be adapted as delivery vehicles for molecules such as soluble protein or DNA (Muhammad et al., 2012). Jiao et al. (2018) recently generated a Salmonella enteriditis ghost for the delivery of a PorB-based Ngo DNA vaccine. In this study, the eukaryotic expression plasmid pVAX1 containing a full-length porB gene was loaded into ghosts and used to transfect mouse bone marrow-derived dendritic cells (BMDCs), which were activated in response to the ghost. In addition, mice produced PorB-specific antibodies upon immunization, and mice immunized with the PorB ghost showed elevated CD4+ and CD8+ T-cell counts compared to those immunized with ghost alone, and both groups were higher than the control group. In a later study (Jiao et al., 2020), this S. enteriditis ghost was again utilized for the delivery of an Ngo nspA-based vaccine. The nspA gene was cloned into plasmid and loaded into the ghost, which was used to immunize BALB/c mice either alone, or alongside ghosts containing the Ngo porB gene, as previous reports showed PorB to be an effective adjuvant for this system when delivering S. enteriditis antigens (Jiao et al., 2016). When co-administered, these ghosts elicited specific IgG and stimulated lymphocyte proliferation. Therefore, the bacterial ghost delivery method may be a promising approach for future Ngo vaccine studies.
Epitope Prediction Tools
Recently, high-throughput computational methods have been utilized to speed the process of epitope prediction and characterization for vaccine development. These techniques have even been used to design synthetic particles that themselves may act as vaccine antigens. While examples of these tools are too numerous for the scope of this review, we highlight a few in this section. For example, Jain et al. (2016) utilized these methods to establish a functional pipeline for identifying possible Ngo epitopes. In short, the SignalP server (DTU Health Tech), which utilizes artificial networks and known organism proteomic databases to predict the presence and location of signal sequences and cleavage sites, was used to predict secretory proteins from Ngo. Candidates from this screen were then submitted to HMMTOP (Tusnády and Simon, 2001), a tool for membrane topology prediction, for validation. Finally, candidate proteins were analyzed by VaxiJen 2.0 for antigen prediction (Doytchinova and Flower, 2007) and by HLAPred for identification of T- and B-cell epitopes. This pipeline resulted in identification of 23 potential Ngo vaccine candidates for utilization in further characterizations. Such a pipeline may be applied to other pathogens as well; a simplified schematic of such a workflow is illustrated in Figure 1.
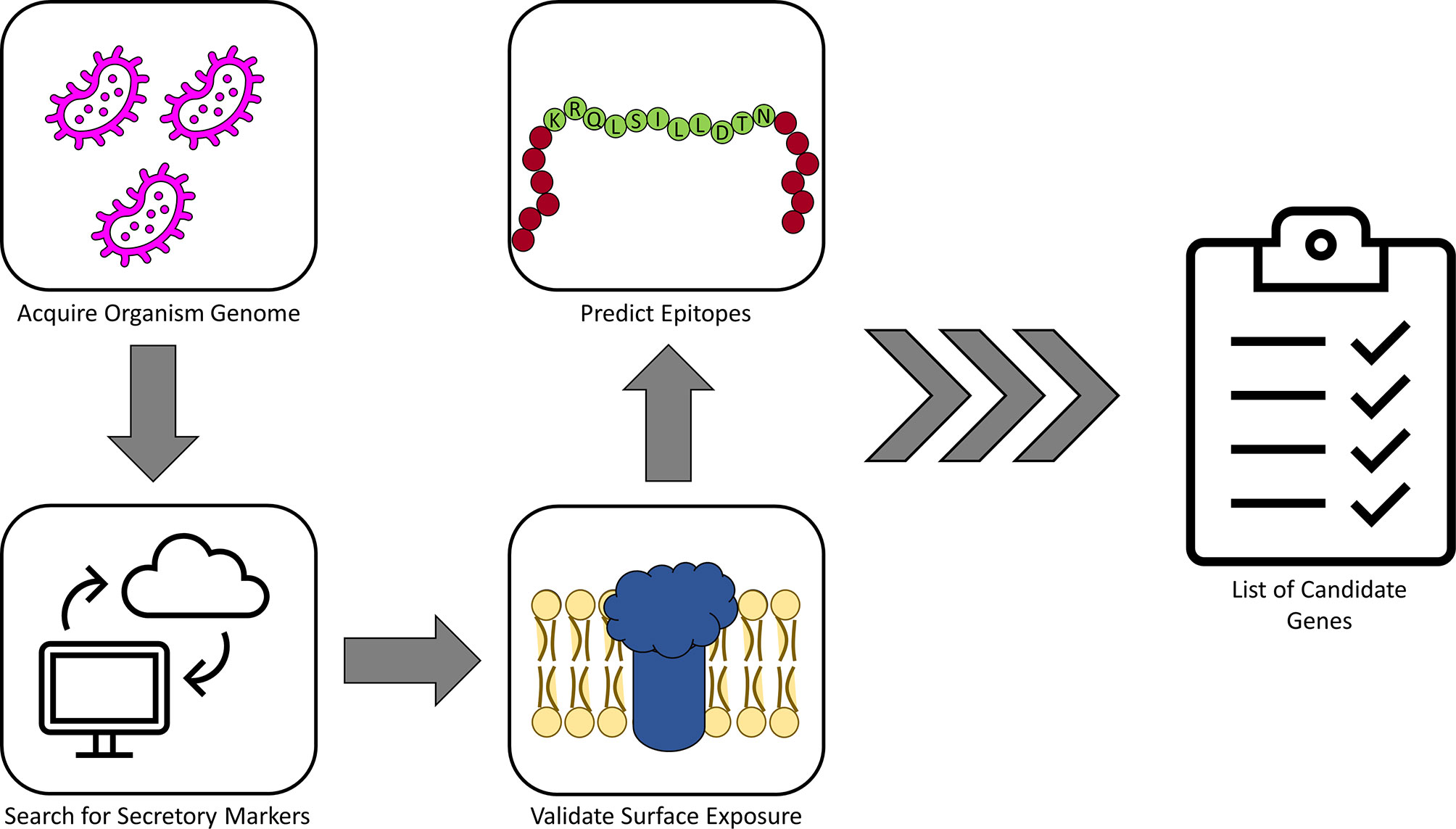
Figure 1 A simplified pipeline for identifying candidate antigens. The cartoon above demonstrates a simplified version of the antigen identification pipeline described in the text. In short, once a genome has been chosen for the organism in question, this genome can be screened for genes likely to produce surface-exposed products, which represent a logical first choice in identifying targets. Once a list of potential surface targets has been identified, these can be further validated by running through topology prediction tools or similar methods to verify the initial screen. From here, the list can be further narrowed down to include only gene products with sufficient epitope characteristics, such as those for B cells and/or T cells, leaving the researcher with a finalized list of candidate targets for evaluation.
PubMLST contains a large database of Ngo genomic data. Baarda et al. (2018) outlined a methodology for “mining” Ngo alleles from PubMLST which may be applied to eventual vaccine design. In this method, one can query a given gonococcal gene and find all alleles of the gene, including their translated protein sequence, within the database. From this point, one can see sequence conservation and distribution within the population, and then proceed to species-specific polymorphism analysis. At this stage, the user can map polymorphisms onto confirmed protein structures via 3D protein analysis tools like PyMOL or UCSF Chimera and identify the conservation of specific antigenic targets, if any are known. In a test example of the workflow, Baarda et al. examined 3,908 Ngo genomes to identify polymorphisms in the MtrE protein. Cumulatively they found that five variants of MtrE represent 98% of MtrE diversity in Ngo, with a large percentage of the protein being conserved across all isolates. Moreover, structural mapping for MtrE revealed the locations of low and high-prevalence polymorphisms within the protein. Such analyses for MtrE and other Ngo proteins are a useful step forward in targeted, rational vaccine design based on exposed, conserved epitopes.
MtrE has been included in other computational vaccine studies as well, such as that performed by Wang et al. (2017), which utilized ferritin nanoparticles as a scaffold for antigenic MtrE loops. The self-assembling ferritin can house relatively large protein domains on its N-terminus (Kanekiyo et al., 2013), so Wang et al. used ferritin from Helicobacter pylori to present MtrE surface-exposed loops as vaccine antigens. These efforts showed that the chimeric molecule could assemble and present loops in a way that should allow antibody access. During the design phase of these chimeras, structural data from the MtrE crystal’s antigenic loops was copied and inserted into a model of the ferritin cage, then the preliminary chimera models were refined for thermodynamic favorability in 3Drefine (Bhattacharya et al., 2016). These chimeras were then purified, and their crystal structures were solved, showing that insertion of MtrE loop peptides did not disrupt the overall structure of the ferritin cage, suggesting that this nanoparticle method could be a suitable platform for delivering Ngo immunogens alone or in an ordered array.
Concluding Remarks
Neisseria gonorrhoeae has presented challenges to researchers for decades. This pathogen causes illness in millions of humans and utilizes an arsenal of antimicrobial resistance strategies and highly-variable surface structures to make treatment and prevention an enormous challenge. Despite these difficulties, the studies described above represent promising steps forward in the effort to combat this urgent threat pathogen. Bioinformatic tools and computational methods now make antigen identification and characterization easier than ever, so it is possible that new targets will emerge as well. This may prove critical to our efforts in combating a pathogen with super bug potential.
Author Contributions
SM performed initial literature review and manuscript drafting, editing. CC performed proofreading and funding acquisition. All authors contributed to the article and approved the submitted version.
Funding
This work was supported by NIH grants R01 AI125421, R01 AI127793, and U19 AI144182 (all to CC).
Conflict of Interest
The authors declare that the research was conducted in the absence of any commercial or financial relationships that could be construed as a potential conflict of interest.
Publisher’s Note
All claims expressed in this article are solely those of the authors and do not necessarily represent those of their affiliated organizations, or those of the publisher, the editors and the reviewers. Any product that may be evaluated in this article, or claim that may be made by its manufacturer, is not guaranteed or endorsed by the publisher.
Acknowledgments
The writing author would like to thank all lab members who contributed to proofreading and review of the text.
References
Apicella, M. A., Shero, M., Jarvis, G. A., Griffiss, J. M., Mandrell, R. E., Schneider, H. (1987). Phenotypic Variation in Epitope Expression of the Neisseria Gonorrhoeae Lipooligosaccharide. Infect. Immun. 55, 1755–1761. doi: 10.1128/iai.55.8.1755-1761.1987
Arko, R. J., Duncan, W. P., Brown, W. J., Peacock, W. L., Tomizawa, T. (1976). Immunity in Infection With Neisseria Gonorrhoeae: Duration and Serological Response in the Chimpanzee. J. Infect. Dis. 133, 441–447. doi: 10.1093/infdis/133.4.441
Arnold, R., Galloway, Y., Mcnicholas, A., O’hallahan, J. (2011). Effectiveness of a Vaccination Programme for an Epidemic of Meningococcal B in New Zealand. Vaccine 29, 7100–7106. doi: 10.1016/j.vaccine.2011.06.120
Baarda, B. I., Zielke, R. A., Nicholas, R. A., Sikora, A. E. (2018). Pubmlst for Antigen Allele Mining to Inform Development of Gonorrhea Protein-Based Vaccines. Front. Microbiol. 9, 2971. doi: 10.3389/fmicb.2018.02971
Beernink, P. T., Ispasanie, E., Lewis, L. A., Ram, S., Moe, G. R., Granoff, D. M. (2019). A Meningococcal Native Outer Membrane Vesicle Vaccine With Attenuated Endotoxin and Overexpressed Factor H Binding Protein Elicits Gonococcal Bactericidal Antibodies. J. Infect. Dis. 219, 1130–1137. doi: 10.1093/infdis/jiy609
Bhattacharya, D., Nowotny, J., Cao, R., Cheng, J. (2016). 3Drefine: An Interactive Web Server for Efficient Protein Structure Refinement. Nucleic Acids Res. 44, W406–W409. doi: 10.1093/nar/gkw336
Boslego, J. W., Tramont, E. C., Chung, R. C., Mcchesney, D. G., Ciak, J., Sadoff, J. C., et al. (1991). Efficacy Trial of a Parenteral Gonococcal Pilus Vaccine in Men. Vaccine 9, 154–162. doi: 10.1016/0264-410X(91)90147-X
Broutet, N., Fruth, U., Deal, C., Gottlieb, S. L., Rees, H. (2014). Vaccines Against Sexually Transmitted Infections: The Way Forward. Vaccine 32, 1630–1637. doi: 10.1016/j.vaccine.2014.01.053
Calmettes, C., Ing, C., Buckwalter, C. M., El Bakkouri, M., Chieh-Lin Lai, C., Pogoutse, A., et al. (2015). The Molecular Mechanism of Zinc Acquisition by the Neisserial Outer-Membrane Transporter Znud. Nat. Commun. 6, 7996. doi: 10.1038/ncomms8996
Cash, D. R., Noinaj, N., Buchanan, S. K., Cornelissen, C. N. (2015). Beyond the Crystal Structure: Insight Into the Function and Vaccine Potential of Tbpa Expressed by Neisseria Gonorrhoeae. Infect. Immun. 83, 4438–4449. doi: 10.1128/IAI.00762-15
Chbib, C., Shah, S. M., Gala, R. P., Uddin, M. N. (2021). Potential Applications of Microparticulate-Based Bacterial Outer Membrane Vesicles (Omvs) Vaccine Platform for Sexually Transmitted Diseases (Stds): Gonorrhea, Chlamydia, and Syphilis. Vaccines (Basel) 9, 1245. doi: 10.3390/vaccines9111245
Cornelissen, C. N. (2018). Subversion of Nutritional Immunity by the Pathogenic Neisseriae. Pathog. Dis. 76, ftx112. doi: 10.1093/femspd/ftx112
Cornelissen, C. N., Hollander, A. (2011). Tonb-Dependent Transporters Expressed by Neisseria Gonorrhoeae. Front. Microbiol. 2, 117. doi: 10.3389/fmicb.2011.00117
Cornelissen, C. N., Kelley, M., Hobbs, M. M., Anderson, J. E., Cannon, J. G., Cohen, M. S., et al. (1998). The Transferrin Receptor Expressed by Gonococcal Strain FA1090 is Required for the Experimental Infection of Human Male Volunteers. Mol. Microbiol. 27, 611–616. doi: 10.1046/j.1365-2958.1998.00710.x
Criss, A. K., Kline, K. A., Seifert, H. S. (2005). The Frequency and Rate of Pilin Antigenic Variation in Neisseria Gonorrhoeae. Mol. Microbiol. 58, 510–519. doi: 10.1111/j.1365-2958.2005.04838.x
Doytchinova, I. A., Flower, D. R. (2007). Vaxijen: A Server for Prediction of Protective Antigens, Tumour Antigens and Subunit Vaccines. BMC Bioinf. 8, 4. doi: 10.1186/1471-2105-8-4
Fegan, J. E., Calmettes, C., Islam, E. A., Ahn, S. K., Chaudhuri, S., Yu, R. H., et al. (2019). Utility of Hybrid Transferrin Binding Protein Antigens for Protection Against Pathogenic Neisseria Species. Front. Immunol. 10, 247. doi: 10.3389/fimmu.2019.00247
Frandoloso, R., Martínez-Martínez, S., Calmettes, C., Fegan, J., Costa, E., Curran, D., et al. (2015). Nonbinding Site-Directed Mutants of Transferrin Binding Protein B Exhibit Enhanced Immunogenicity and Protective Capabilities. Infect. Immun. 83, 1030–1038. doi: 10.1128/IAI.02572-14
Gottlieb, S. L., Ndowa, F., Hook, E. W., 3rd, Deal, C., Bachmann, L., Abu-Raddad, L., et al. (2020). Gonococcal Vaccines: Public Health Value and Preferred Product Characteristics; Report of a WHO Global Stakeholder Consultation, January 2019. Vaccine 38, 4362–4373. doi: 10.1016/j.vaccine.2020.02.073
Greenberg, L., Diena, B. B., Ashton, F. A., Wallace, R., Kenny, C. P., Znamirowski, R., et al. (1974). Gonococcal Vaccine Studies in Inuvik. Can. J. Public Health 65, 29–33.
Gulati, S., Mcquillen, D. P., Mandrell, R. E., Jani, D. B., Rice, P. A. (1996). Immunogenicity of Neisseria Gonorrhoeae Lipooligosaccharide Epitope 2C7, Widely Expressed In Vivo With No Immunochemical Similarity to Human Glycosphingolipids. J. Infect. Dis. 174, 1223–1237. doi: 10.1093/infdis/174.6.1223
Gulati, S., Pennington, M. W., Czerwinski, A., Carter, D., Zheng, B., Nowak, N. A., et al. (2019a). Preclinical Efficacy of a Lipooligosaccharide Peptide Mimic Candidate Gonococcal Vaccine. mBio 10, e02552–e02519. doi: 10.1128/mBio.02552-19
Gulati, S., Shaughnessy, J., Ram, S., Rice, P. A. (2019b). Targeting Lipooligosaccharide (LOS) for a Gonococcal Vaccine. Front. Immunol. 10, 321. doi: 10.3389/fimmu.2019.00321
Hagen, T. A., Cornelissen, C. N. (2006). Neisseria Gonorrhoeae Requires Expression of Tonb and the Putative Transporter Tdff to Replicate Within Cervical Epithelial Cells. Mol. Microbiol. 62, 1144–1157. doi: 10.1111/j.1365-2958.2006.05429.x
Jain, R., Sonkar, S. C., Chaudhry, U., Bala, M., Saluja, D. (2016). In-Silico Hierarchical Approach for the Identification of Potential Universal Vaccine Candidates (Puvcs) From Neisseria Gonorrhoeae. J. Theor. Biol. 410, 36–43. doi: 10.1016/j.jtbi.2016.09.004
Jean, S., Juneau, R. A., Criss, A. K., Cornelissen, C. N. (2016). Neisseria Gonorrhoeae Evades Calprotectin-Mediated Nutritional Immunity and Survives Neutrophil Extracellular Traps by Production of Tdfh. Infect. Immun. 84, 2982–2994. doi: 10.1128/IAI.00319-16
Jerse, A. E., Bash, M. C., Russell, M. W. (2014). Vaccines Against Gonorrhea: Current Status and Future Challenges. Vaccine 32, 1579–1587. doi: 10.1016/j.vaccine.2013.08.067
Jiao, H., Yang, H., Zhao, D., Chen, J., Zhang, Q., Liang, J., et al. (2018). Design and Immune Characterization of a Novel Neisseria Gonorrhoeae DNA Vaccine Using Bacterial Ghosts as Vector and Adjuvant. Vaccine 36, 4532–4539. doi: 10.1016/j.vaccine.2018.06.006
Jiao, H., Yang, H., Zhao, D., He, L., Chen, J., Li, G. (2016). The Enhanced Immune Responses Induced by Salmonella Enteritidis Ghosts Loaded With Neisseria Gonorrhoeae Porb Against Salmonella in Mice. FEMS Microbiol. Lett. 363, fnw239. doi: 10.1093/femsle/fnw239
Jiao, H., Yang, H., Zheng, W., Zhang, Q., Zhao, D., Li, G. (2020). Enhancement of Immune Responses by Co-Administration of Bacterial Ghosts-Mediated Neisseria Gonorrhoeae DNA Vaccines. J. Appl. Microbiol. 130, 1770–1777. doi: 10.1111/jam.14815
Kammerman, M. T., Bera, A., Wu, R., Harrison, S. A., Maxwell, C. N., Lundquist, K., et al. (2020). Molecular Insight Into Tdfh-Mediated Zinc Piracy From Human Calprotectin by Neisseria Gonorrhoeae. mBio 11, e00949–e00920. doi: 10.1128/mBio.00949-20
Kanekiyo, M., Wei, C. J., Yassine, H. M., Mctamney, P. M., Boyington, J. C., Whittle, J. R., et al. (2013). Self-Assembling Influenza Nanoparticle Vaccines Elicit Broadly Neutralizing H1N1 Antibodies. Nature 499, 102–106. doi: 10.1038/nature12202
Kulp, A., Kuehn, M. J. (2010). Biological Functions and Biogenesis of Secreted Bacterial Outer Membrane Vesicles. Annu. Rev. Microbiol. 64, 163–184. doi: 10.1146/annurev.micro.091208.073413
Langemann, T., Koller, V. J., Muhammad, A., Kudela, P., Mayr, U. B., Lubitz, W. (2010). The Bacterial Ghost Platform System: Production and Applications. Bioeng Bugs 1, 326–336. doi: 10.4161/bbug.1.5.12540
Leduc, I., Connolly, K. L., Begum, A., Underwood, K., Darnell, S., Shafer, W. M., et al. (2020). The Serogroup B Meningococcal Outer Membrane Vesicle-Based Vaccine 4cmenb Induces Cross-Species Protection Against Neisseria Gonorrhoeae. PloS Pathog. 16, e1008602. doi: 10.1371/journal.ppat.1008602
Liu, Y., Feinen, B., Russell, M. W. (2011). New Concepts in Immunity to Neisseria Gonorrhoeae: Innate Responses and Suppression of Adaptive Immunity Favor the Pathogen, Not the Host. Front. Microbiol. 2, 52. doi: 10.3389/fmicb.2011.00052
Liu, Y., Hammer, L. A., Liu, W., Hobbs, M. M., Zielke, R. A., Sikora, A. E., et al. (2017). Experimental Vaccine Induces Th1-Driven Immune Responses and Resistance to Neisseria Gonorrhoeae Infection in a Murine Model. Mucosal Immunol. 10, 1594–1608. doi: 10.1038/mi.2017.11
Liu, Y., Perez, J., Hammer, L. A., Gallagher, H. C., De Jesus, M., Egilmez, N. K., et al. (2018). Intravaginal Administration of Interleukin 12 During Genital Gonococcal Infection in Mice Induces Immunity to Heterologous Strains of Neisseria Gonorrhoeae. mSphere 3, e00421–e00417. doi: 10.1128/mSphere.00421-17
Longtin, J., Dion, R., Simard, M., Betala Belinga, J. F., Longtin, Y., Lefebvre, B., et al. (2017). Possible Impact of Wide-Scale Vaccination Against Serogroup B Neisseria Meningitidis on Gonorrhea Incidence Rates in One Region of Quebec, Canada. Open Forum Infect. Dis. 4, 734–735. doi: 10.1093/ofid/ofx180.002
Marjuki, H., Topaz, N., Joseph, S. J., Gernert, K. M., Kersh, E. N., Wang, X. (2019). Genetic Similarity of Gonococcal Homologs to Meningococcal Outer Membrane Proteins of Serogroup B Vaccine. mBio 10, e01668–e01619. doi: 10.1128/mBio.01668-19
Matthias, K. A., Connolly, K. L., Begum, A. A., Jerse, A. E., Macintyre, A. N., Sempowski, G. D., et al. (2021). Meningococcal Detoxified Outer Membrane Vesicle Vaccines Enhance Gonococcal Clearance in a Murine Infection Model. J. Infect. Dis. 225, 650–660. doi: 10.1093/infdis/jiab450
Maurakis, S., Keller, K., Maxwell, C. N., Pereira, K., Chazin, W. J., Criss, A. K., et al. (2019). The Novel Interaction Between Neisseria Gonorrhoeae Tdfj and Human S100A7 Allows Gonococci to Subvert Host Zinc Restriction. PloS Pathog. 15, e1007937. doi: 10.1371/journal.ppat.1007937
Muhammad, A., Champeimont, J., Mayr, U. B., Lubitz, W., Kudela, P. (2012). Bacterial Ghosts as Carriers of Protein Subunit and DNA-Encoded Antigens for Vaccine Applications. Expert Rev. Vaccines 11, 97–116. doi: 10.1586/erv.11.149
Ngampasutadol, J., Rice, P. A., Walsh, M. T., Gulati, S. (2006). Characterization of a Peptide Vaccine Candidate Mimicking an Oligosaccharide Epitope of Neisseria Gonorrhoeae and Resultant Immune Responses and Function. Vaccine 24, 157–170. doi: 10.1016/j.vaccine.2005.07.065
Ochoa-Azze, R. F. (2018). Cross-Protection Induced by VA-MENGOC-BC® Vaccine. Hum. Vaccin Immunother. 14, 1064–1068. doi: 10.1080/21645515.2018.1438028
Petousis-Harris, H., Paynter, J., Morgan, J., Saxton, P., Mcardle, B., Goodyear-Smith, F., et al. (2017). Effectiveness of a Group B Outer Membrane Vesicle Meningococcal Vaccine Against Gonorrhoea in New Zealand: A Retrospective Case-Control Study. Lancet 390, 1603–1610. doi: 10.1016/S0140-6736(17)31449-6
Price, G. A., Hobbs, M. M., Cornelissen, C. N. (2004). Immunogenicity of Gonococcal Transferrin Binding Proteins During Natural Infections. Infect. Immun. 72, 277–283. doi: 10.1128/IAI.72.1.277-283.2004
Price, G. A., Masri, H. P., Hollander, A. M., Russell, M. W., Cornelissen, C. N. (2007). Gonococcal Transferrin Binding Protein Chimeras Induce Bactericidal and Growth Inhibitory Antibodies in Mice. Vaccine 25, 7247–7260. doi: 10.1016/j.vaccine.2007.07.038
Ram, S., Gulati, S., Lewis, L. A., Chakraborti, S., Zheng, B., Deoliveira, R. B., et al. (2018). A Novel Sialylation Site on Neisseria Gonorrhoeae Lipooligosaccharide Links Heptose II Lactose Expression With Pathogenicity. Infect. Immun. 86, e00285–e00218. doi: 10.1128/IAI.00285-18
Rowley, J., Vander Hoorn, S., Korenromp, E., Low, N., Unemo, M., Abu-Raddad, L. J., et al. (2019). Chlamydia, Gonorrhoea, Trichomoniasis and Syphilis: Global Prevalence and Incidence Estimate. Bull. World Health Organ 97, 548–562. doi: 10.2471/BLT.18.228486
Russell, M. W., Hedges, S. R., Wu, H. Y., Hook, E. W. (1999). Mucosal Immunity in the Genital Tract: Prospects for Vaccines Against Sexually Transmitted Diseases–a Review. Am. J. Reprod. Immunol. 42, 58–63. doi: 10.1111/j.1600-0897.1999.tb00466.x
Russell, M. W., Jerse, A. E., Gray-Owen, S. D. (2019). Progress Toward a Gonococcal Vaccine: The Way Forward. Front. Immunol. 10, 2417. doi: 10.3389/fimmu.2019.02417
Semchenko, E. A., Day, C. J., Seib, K. L. (2020a). The Neisseria Gonorrhoeae Vaccine Candidate NHBA Elicits Antibodies That are Bactericidal, Opsonophagocytic and That Reduce Gonococcal Adherence to Epithelial Cells. Vaccines (Basel) 8, 219. doi: 10.3390/vaccines8020219
Semchenko, E. A., Mubaiwa, T. D., Day, C. J., Seib, K. L. (2020b). Role of the Gonococcal Neisserial Heparin Binding Antigen in Microcolony Formation, and Serum Resistance and Adherence to Epithelial Cells. J. Infect. Dis. 221, 1612–1622. doi: 10.1093/infdis/jiz628
Semchenko, E. A., Tan, A., Borrow, R., Seib, K. L. (2019). The Serogroup B Meningococcal Vaccine Bexsero Elicits Antibodies to Neisseria Gonorrhoeae. Clin. Infect. Dis. 69, 1101–1111. doi: 10.1093/cid/ciy1061
Sikora, A. E., Wierzbicki, I. H., Zielke, R. A., Ryner, R. F., Korotkov, K. V., Buchanan, S. K., et al. (2018). Structural and Functional Insights Into the Role of Bamd and Bame Within the β-Barrel Assembly Machinery in Neisseria Gonorrhoeae. J. Biol. Chem. 293, 1106–1119. doi: 10.1074/jbc.RA117.000437
Stork, M., Bos, M. P., Jongerius, I., De Kok, N., Schilders, I., Weynants, V. E., et al. (2010). An Outer Membrane Receptor of Neisseria Meningitidis Involved in Zinc Acquisition With Vaccine Potential. PloS Pathog. 6, e1000969. doi: 10.1371/journal.ppat.1000969
Toneatto, D., Pizza, M., Masignani, V., Rappuoli, R. (2017). Emerging Experience With Meningococcal Serogroup B Protein Vaccines. Expert Rev. Vaccines 16, 433–451. doi: 10.1080/14760584.2017.1308828
Tramont, E. C., Boslego, J. W. (1985). Pilus Vaccines. Vaccine 3, 3–10. doi: 10.1016/0264-410X(85)90003-9
Tusnády, G. E., Simon, I. (2001). The HMMTOP Transmembrane Topology Prediction Server. Bioinformatics 17, 849–850. doi: 10.1093/bioinformatics/17.9.849
Unemo, M., Lahra, M. M., Cole, M., Galarza, P., Ndowa, F., Martin, I., et al. (2019). World Health Organization Global Gonococcal Antimicrobial Surveillance Program (WHO GASP): Review of New Data and Evidence to Inform International Collaborative Actions and Research Efforts. Sex Health 16, 412–425. doi: 10.1071/SH19023
Wang, L., Xing, D., Le Van, A., Jerse, A. E., Wang, S. (2017). Structure-Based Design of Ferritin Nanoparticle Immunogens Displaying Antigenic Loops of Neisseria Gonorrhoeae. FEBS Open Bio 7, 1196–1207. doi: 10.1002/2211-5463.12267
Wetzler, L. M., Blake, M. S., Barry, K., Gotschlich, E. C. (1992). Gonococcal Porin Vaccine Evaluation: Comparison of Por Proteosomes, Liposomes, and Blebs Isolated From Rmp Deletion Mutants. J. Infect. Dis. 166, 551–555. doi: 10.1093/infdis/166.3.551
Whelan, J., Kløvstad, H., Haugen, I. L., Holle, M. R., Storsaeter, J. (2016). Ecologic Study of Meningococcal B Vaccine and Neisseria Gonorrhoeae Infection, Norway. Emerg. Infect. Dis. 22, 1137–1139. doi: 10.3201/eid2206.151093
Yadav, R., Noinaj, N., Ostan, N., Moraes, T., Stoudenmire, J., Maurakis, S., et al. (2019). Structural Basis for Evasion of Nutritional Immunity by the Pathogenic. Front. Microbiol. 10, 2981. doi: 10.3389/fmicb.2019.02981
Yamasaki, R., Koshino, H., Kurono, S., Nishinaka, Y., Mcquillen, D. P., Kume, A., et al. (1999). Structural and Immunochemical Characterization of a Neisseria Gonorrhoeae Epitope Defined by a Monoclonal Antibody 2C7; the Antibody Recognizes a Conserved Epitope on Specific Lipo-Oligosaccharides in Spite of the Presence of Human Carbohydrate Epitopes. J. Biol. Chem. 274, 36550–36558. doi: 10.1074/jbc.274.51.36550
Zhu, W., Chen, C. J., Thomas, C. E., Anderson, J. E., Jerse, A. E., Sparling, P. F. (2011). Vaccines for Gonorrhea: Can We Rise to the Challenge? Front. Microbiol. 2, 124. doi: 10.3389/fmicb.2011.00124
Zhu, W., Thomas, C. E., Chen, C. J., Van Dam, C. N., Johnston, R. E., Davis, N. L., et al. (2005). Comparison of Immune Responses to Gonococcal Porb Delivered as Outer Membrane Vesicles, Recombinant Protein, or Venezuelan Equine Encephalitis Virus Replicon Particles. Infect. Immun. 73, 7558–7568. doi: 10.1128/IAI.73.11.7558-7568.2005
Keywords: Neisseria gonorrhoeae, gonorrhea, vaccine, sexually-transmitted infections, immunity, epitope, computation, modeling
Citation: Maurakis SA and Cornelissen CN (2022) Recent Progress Towards a Gonococcal Vaccine. Front. Cell. Infect. Microbiol. 12:881392. doi: 10.3389/fcimb.2022.881392
Received: 22 February 2022; Accepted: 22 March 2022;
Published: 11 April 2022.
Edited by:
Jesús A. Arenas, University of Zaragoza, SpainReviewed by:
Michael W. Russell, University at Buffalo, United StatesCopyright © 2022 Maurakis and Cornelissen. This is an open-access article distributed under the terms of the Creative Commons Attribution License (CC BY). The use, distribution or reproduction in other forums is permitted, provided the original author(s) and the copyright owner(s) are credited and that the original publication in this journal is cited, in accordance with accepted academic practice. No use, distribution or reproduction is permitted which does not comply with these terms.
*Correspondence: Cynthia Nau Cornelissen, Y2Nvcm5lbGlzc2VuQGdzdS5lZHU=