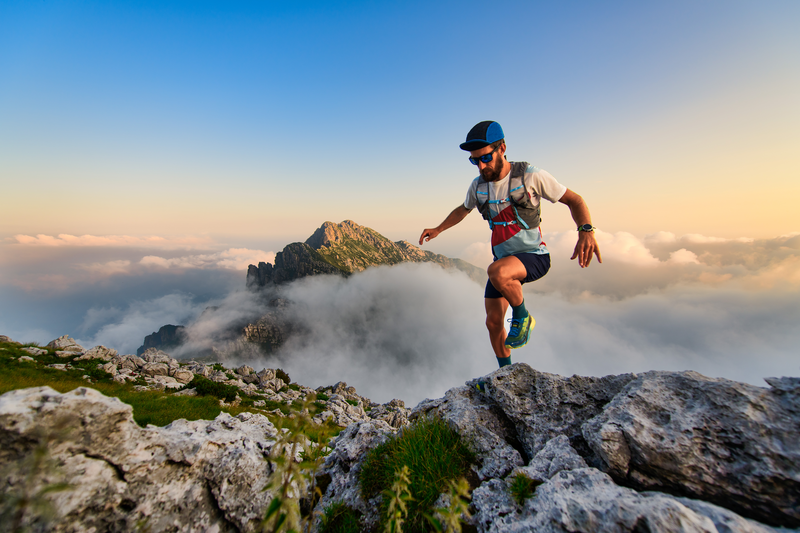
95% of researchers rate our articles as excellent or good
Learn more about the work of our research integrity team to safeguard the quality of each article we publish.
Find out more
MINI REVIEW article
Front. Cell. Infect. Microbiol. , 12 April 2022
Sec. Fungal Pathogenesis
Volume 12 - 2022 | https://doi.org/10.3389/fcimb.2022.860779
This article is part of the Research Topic Proceedings of the Mycology 2021 View all 6 articles
Exposure to fungi is inevitable, yet only a small number of patients with significant clinical risk develop invasive aspergillosis (IA). While timing of exposure in relation to immune status, environmental and occupational factors will influence the probability of developing IA, factors specific to the individual will likely play a role and variation in the host’s genetic code associated with the immunological response to fungi have been linked to increased risk of developing IA. Screening for SNPs in genes significantly associated with IA (e.g. Pentraxin-3, Toll-like receptor 4, Dectin-1, DC-SIGN) could form part of the clinical work-up on admission or post allogeneic stem cell transplantation, to complement fungal biomarker screening. Through the combination of clinical and genetic risk with mycological evidence, we are approaching a time when we should be able to accurately predict the risk of IA in the haematology patient, using predictive modelling to stratifying each individual’s management. Understanding the host and their immune responses to infection through genomics, transcriptomics and metabolomics/proteomics is critical to achieving how we manage the individual’s risk of IA, underpinning personalized medicine. This review will investigate what is known about the genetic risk associated with developing IA, primarily in haematology patients and whether these strategies are ready to be incorporated into routine clinical practice, and if not what are the remaining hurdles to implementation.
The exposure of the human being to fungi, whether environmental or commensal, is inevitable. Yet most fungi only demonstrate a pathogenic capacity when permitted conditions within the host that are preferential to fungal proliferation (e.g. immunosuppression or disruption to anatomical barriers). The number of patients requiring immunosuppressive conditioning increases annually, and despite many patients having similar degrees of clinical risk for developing invasive fungal disease (IFD), only a relatively small proportion develop IFD despite the inevitable exposure to fungi, which itself is very difficult to control or obviate.
Why certain patients develop IFD remains unclear. In simple terms, luck may play a part, where the timing of exposure to a significant fungal burden does not coincide with a period of increased clinical risk (e.g. prolonged, profound neutropenia) of developing IFD. In the unlucky few, enhanced clinical risk corresponds with exposure to high fungal burdens due to local environmental conditions, close proximity to construction or even occupational hazards. The virulence of the infecting organism and subsequent exposure to such a strain may be significant. Certain strains of Aspergilllus may have an increased capacity for causing infection, however, a recent UK and Ireland epidemiological study using next generation sequencing determined that clinical strains were intrinsically linked to those circulating in the environment [Rhodes et al., (Forthcoming)].
Obviously clinical management can minimize the risk of developing IFD, with antifungal prophylactic strategies widely employed in high risk groups (e.g. acute leukaemia or allogeneic stem cell recipients) and can successfully lower the incidence of IFD [e.g. invasive aspergillosis (IA)] (Wang et al., 2020). Nevertheless, even in the absence of antifungal prophylaxis the majority (>85%) of high risk haematology patients will not develop IFD/IA, despite the apparent risk and inevitable fungal exposure (Barnes et al., 2018).
This indicates other factors may play a role in governing the risk of developing IFD in the individual patient already considered clinically at high-risk of IFD. Genetic variants, specifically single nucleotide polymorphisms (SNPs), in the host’s genetic code associated with the innate immunological response to fungi have been linked to increased risk of developing IA (Cunha and Carvalho, 2019). Given an individual’s genetic code is unique, increased risk of IFD due to the presence of genetic polymorphisms provides a reason as to why only certain patients develop IFD. Prior knowledge to the presence of these polymorphisms within the specific patient could be used to stratify risk of IFD on an individual basis (personalized medicine). Screening for SNPs could form part of the clinical work-up on admission or post allogeneic stem cell transplantation, to complement fungal biomarker screening.
Despite our increasing knowledge on the importance of SNPs in regards to developing IFD, in particular IA, approaches to implement such tests in prospective practice remain limited (Tanpaibule et al., 2020). This review will investigate what is known about the genetic risk associated with developing IA and whether these strategies are ready to be incorporated into routine clinical practice, and if not what are the remaining hurdles to implementation.
The primary functions of any testing are to either exclude or confirm a diagnosis. While in principle a single test has the potential to facilitate both parameters, in practice, the pre-requisite high sensitivity required to exclude infection when the test is negative usually compromises specificity to below levels necessary for confidence in a positive result required to confirm infection. With the incidence of IA generally being low (<10%), the pre-test probability of not having IA is high (>90%) and can be exploited by mycological testing [combined Aspergillus PCR and Galactomannan EIA (GM)] to exclude infection when both tests are consistently negative (Arvanitis et al., 2015). The combined screening strategies are employed to provide sufficient sensitivity and also have the capacity to increase confidence in diagnosis through the availability of sequential/multiple positive biomarker results, but even when a patient is positive on multiple occasions by both PCR and GM, the post-test probability is not always convincing of infection (55%) (Barnes et al., 2013). Confidence in infection can be enhanced by performing a diagnostic work-up (Bronchoscopy/HRCT Chest) of any high-risk patient with multiple positive biomarker results, but these additional investigations are not always performed in a timely manner, and waiting on these results to commence treatment is not recommended given the deleterious impact of delaying necessary antifungal therapy.
As with all clinical conditions “prevention is better than a cure”, to achieve this, action is taken against an anticipated outcome, based on the odds of developing future disease. The odds are influenced by various variables, including clinical conditions, environmental exposure and potentially genetic predisposition to infection. Combining these risk factors into a strategic algorithm, also incorporating existing biomarker screening has the potential to provide a personalized pre-emptive approach, where various thresholds can be set to change patient management (e.g. continue to monitor, antifungal prophylaxis, pre-emptive therapy, targeted therapy) based on the probability of developing IA.
Empirical antifungal therapy (treating a fever refractory to broad spectrum antibiotics) is widely administered in high-risk hematology patients. This approach was incorporated in an era when access to enhanced mycological testing was not available and this strategy bases the risk of developing IA solely on underlying clinical risk, leading to many patients receiving unnecessary antifungal therapy which is far from ideal given the increasing rates of antifungal resistance and the adverse effects/drug-drug interactions associated with antifungal drugs. Biomarkers can be early indicators of infection in hematology patients with IA. Positivity permits the opportunity to pre-empt overt disease, provided biomarker screening does not generate excessive numbers of false positive results, but the current post-test probability of IA associated with multiple positives remains insufficient for purpose (Barnes et al., 2013; Aguado et al., 2015; Cruciani et al., 2019). Combining biomarkers with clinical risk and data on genetic predisposition to IA has the potential to significantly improve how we manage patients at risk of IA.
An extensive number of SNPs have been linked to increased susceptibility of developing IA, where genetic variations in the genes encoding pattern recognition receptors [PRRs, e.g. toll like receptors (TLR) or C-type lectin receptors] alter the signalling pathway of the host’s innate immune response, but a wide array of genetic variations in other genes encoding innate immunity components have been documented, along with genetic variation in components of the host’s adaptive immunity (e.g. Th-17 or IFN-γ, Table 1) (van der Velden et al., 2011; Sainz et al., 2012; Lupiañez et al., 2015; Fisher et al., 2017; Cunha et al., 2018; Cunha and Carvalho, 2019).
Table 1 A summary of selected genetic variants associated with aspergillosis in various patient cohorts.
The significant number of polymorphisms linked to increased susceptibility reflects the complexity of the host’s immune response to Aspergillus and screening for such a large number of polymorphisms is a major challenge when attempting to incorporate genetic testing into routine strategic algorithms. While next generation sequencing surely holds the key for screening hosts or donors for polymorphism associated with aspergillosis (and will undoubtedly highlight additional genetic markers), further work is needed to elucidate the associated phenotypic alterations to protein structure, function and implications to any immunological pathways involving such proteins. Not all genetic variants have been functionally characterized, and it is essential this is addressed to develop an enhanced understanding of the host’s defense to IA. Further knowledge regarding the presence of multiple polymorphism’s within the same, but more so different genes, need also be gained in order to improve our understanding of the cumulative effect of genetic variation on the risk of developing IA. In order for genetic screening of patients to be incorporated into routine clinical practice, automated pipelines will be required to perform genetic analysis, but also to calculate risk of developing IA based on complementary or confounding genetic and clinical risk factors and diagnostic results.
With many polymorphisms identified in single center studies, overcoming sample size, ethnical diversity and other clinical forms of population heterogeneity is critical, together with ensuring the influence of methodological, sample selection and statistical biases are minimized (van der Velden et al., 2011). Confidence in the significance of any association between a polymorphism and risk of IA is enhanced by the availability of multiple studies confirming the link.
Pentraxin-3 (PTX3) forms complexes on the surface of Aspergillus conidia where it acts as an opsonin by improved labelling of the microbe, resulting in enhanced phagocytosis and control of Aspergillus infection. Levels of PTX3 in the lung-alveolar fluid can be relatively low compared to other components capable of opsonizing Aspergillus conidia, but PTX3 genetic variants impair both alveolar expression of the protein and the antifungal effect mechanism of neutrophils (neutrophil extracellular traps), which play a primary role in the innate response during hyphal tissue invasion (Cunha and Carvalho, 2019). PTX3 has significant stimulus on pro-inflammatory cytokines and also interacts with many serum proteins involved the complement cascade but also CLRs, such as Dectin-1, with PTX3 potentially amplifying the Dectin-1 response (Cunha and Carvalho, 2019; Kang et al., 2020).
Polymorphisms in the PTX3 gene were first reported in 2014 where the homozygous haplotype (h2/h2) was associated with increased susceptibility to IA in allogeneic SCT patients in both the discovery and confirmatory study (Table 1) (Cunha et al., 2014). The haplotype resulted in defective PTX3 expression and ultimately resulted in impaired phagocytosis and fungal clearance. Since then several additional studies have confirmed the significance of this haplotype with increased risk of aspergillosis in SCT, solid organ transplantation recipients and patients with chronic respiratory conditions (Cunha et al., 2015; Wójtowicz et al., 2015; He et al., 2018). Its significance was also confirmed in the US study of Fisher and colleagues who screened for the presence of 20 SNP’s previously reported to be significantly associated with IA in 2609 SCT patients (483 with proven/probable IA) (Fisher et al., 2017). The number of independent studies corroborating the significance of this polymorphism across various ethnicities and clinical settings imply the presence pf PTX3 polymorphisms to be a robust indication of increased risk of aspergillosis.
A Spanish study screening 27 SNPs primarily in C-type lectin receptors (CLR) demonstrated six significant associations between polymorphisms in genes encoding Dectin-1 and DC-SIGN (Table 1) (Sainz et al., 2012). Dectin-1 recognizes (1-3)-β-D-Glucan, a major cell wall component of most fungi, while the other CLR (e.g. DC-SIGN, Dectin-2, Mannose Binding protein) primarily recognize mannose components of the fungal cell wall (Hardison and Brown, 2012). Ligand binding to these PRRs stimulates innate immune responses including fungal recognition, phagocytosis, induction of antifungal effector mechanisms and production of cytokines/chemokines, but also stimulates and controls the pro-inflammatory (TH1/TH17) adaptive immune response. The significance of two genetic variants in DC-SIGN (rs11465384 and rs7248637) and one in Dectin-1 (rs7309123) were confirmed in a UK study, with the two Dectin-1 SNPs (rs7309123 and rs3901533) also being confirmed in a recent Chinese study (White et al., 2017; Chen et al., 2019). DC-SIGN rs7248637 and Dectin-1 rs7309123 were also found to be associated with IA when defined cases of IA included proven/probable IA or patients that were positive by serum GM testing in the large US validation study (Fisher et al., 2017). A recent systematic review and meta-analysis confirmed the two Dectin-1 SNPs (rs7309123 and rs3901533) to be significantly associated with risk of IA (Zhou et al., 2019).
With their broad role in antimicrobial defense, genetic variations in TLRs have also been associated with increased susceptibility to IFD. While definitive, primary fungal ligands recognized by TLRs are still being identified, recognition of mannosylated cell wall components may play an important role in the innate immune response via the induction of pro-inflammatory cytokines (e.g. TNF-α and IL-6), neutrophil recruitment and fungal clearance (Salazar and Brown, 2018). It is unclear if TLRs play a primary role in human antifungal immunity, and they may have a collaborative role with CLRs or have increased responsibility in hosts with altered immunity. A significant link between the TLR4 haplotype (D299G/T399I) and increased risk of aspergillosis has also been confirmed in various studies including SCT and patients with chronic respiratory conditions (Bochud et al., 2008; Carvalho et al., 2008; de Boer et al., 2011; Koldehoff et al., 2013). While this provides confidence in the association between haplotype and aspergillosis, other studies contradict this finding, highlighting the difficulties in stratifying any approach screening for specific polymorphisms (Carvalho et al., 2009; Fisher et al., 2017).
Conflicting studies where the significance of polymorphisms deemed previously relevant is questioned, immediately focus attention on the few confirmed SNPS, but while unconfirmed SNPs could reflect limitations of the initial study, it could reflect ethnicity/evolutionary/clinical differences in the populations studied, and SNPs with conflicting results should not be immediately discarded without evaluation in large scale trials.
Of the two largest studies to date, one performed an extensive validation study of polymorphisms previously reported to be associated with IA in hematology patients. Two of 20 SNPs (PTX3 rs2305619 and Dectin 1 rs16910526) had a significant association with proven/probable IA confirmed (Fisher et al., 2017). Further polymorphisms in genes encoding C-type lectin receptors (Dectin 1, DC-SIGN), TLR (TLR6), chemokines (CXCL10), cytokines (IFN-γ), immune receptors (TNFR1), Ca2+ binding protein (S100B) and fibrinolytic proteins (plasminogen) were confirmed significant when patients with serum GM positivity were considered cases, in addition to more stringently classified proven/probable IA. The AspBIOmics consortium identified 36 SNPs within 14 immunomodulatory genes that were associated with immune response, had laboratory evidence of a biological function and/or had been previously reported as significantly associated with infectious diseases (Lupiañez et al., 2015). A total of 781 hematology patients (149 with proven/probable IA) were screened for the presence of these SNPs over two phases, the latter used to confirm results. Polymorphisms in IL4R (rs2107356) and IL8 (rs2227307) were significantly associated with increased risk of IA, whereas polymorphisms in IL12B (rs3212227) and IFN-γ (rs2069705) reduced the risk of IA, with the effects IL4R and IFN-γ polymorphisms emphasized in the allogeneic patient.
Given the range of polymorphisms associated with increased risk of IA it is unlikely that a single polymorphism will provide the required clinical utility. Testing multiple high-risk genes for the presence of indicative SNP’s seems inevitable but also astute, where specific patterns of genetic polymorphisms rather than a single genetic variation may meet the desired clinical requirements for predicting IA (de Boer et al., 2011; White et al., 2017). Advancing the field based on current knowledge would indicate that testing for well characterized SNPs in of PTX3, TLR4 and C-type lectin receptors would be a good starting place for strategic screening. However, while a recent prospective study in an Asian hematology population screened for relevant SNP’s in these genes, the only significant association with increased risk of IA was with C genotype in IL10 rs1800896, possibly indicating ethnical influence (Tanpaibule et al., 2020). If there is a requirement to screen for excessive numbers (>20) of SNPs in each patient then performing white cell functionality (human biomarker) studies to assess and individual’s peripheral blood mononuclear cells in response to Aspergillus stimuli might be an alternative approach (Zoran et al., 2022; Griffiths et al., 2021).
Various predictive risk models of IA have been proposed, incorporating a wide array of clinical parameters (underlying condition/immune status/influence of treatment of underlying condition/co-morbidities/environmental and occupational risk) (Stanzani and Lewis, 2018). The D-index based on the duration and severity of neutropenia has shown to be sensitive (91-100%) by not necessarily specific (58%) for predicting IA (Portugal et al., 2009). Risk scores and indices for IFD have been proposed, but can be restricted to specific patient populations, lack prospective evaluation or have potential study design limitations (Stanzani and Lewis, 2018). A weighted risk score (BOSCORE) model for Hematology patients, initially incorporating four clinical risk factors (Active malignancy, prolonged neutropenia, severe lymphocytopenia and history of IFD) and recalibrated with additional risk factors (high dose steroids, high-risk chemotherapy, CMV reactivation/disease) confirmed the benefit of these strategies for excluding IFD (NPV>96%) and can be used to highlight patients requiring posaconazole prophylaxis, biomarker screening and or investigative bronchoscopy and chest CT (Stanzani and Lewis, 2018).
As with biomarker screening, predictive models based on clinical risk alone appear suitable for identifying patients at low risk of developing or without IA. While these models do highlight the patient with a higher risk of developing IA, significant numbers of high risk patients do not develop IA, limiting confidence in the strategy for confirming or pre-empting a diagnosis. With the number of clinical risk factors associated with risk of IFD increasing [e.g. immunomodulatory therapies, (etanercept)], models will require continual recalibration (Zoran et al., 2019).
The association between polymorphisms in genes encoding proteins involved the host’s immune response and risk of aspergillosis appears undisputed, but judging the weight of risk associated with each polymorphism alone or when combined with other genetic variations or even clinical risk factors is essential but currently limited. While odds or hazard ratios are a statistically sound way of reporting significance, clinical interpretation is dependent on knowing the probability of IA developing in a patient with sufficient genomic and clinical risk, potentially combined with evidence of infection (Figure 1).
Figure 1 A proposed combined strategy incorporating clinical, host and diagnostic risk for managing the risk of IA in the hematology patient.
An understanding of how best to interpret the presence or, indeed, absence of a polymorphism is also important. The Dectin-1 variant rs7309123 has been associated with IA in several studies, yet it can be present in a significant proportion (65%) of hematology patients without IA and likely represents the major allele (White et al., 2017). So while the presence of this particular SNP is significantly associated with increased risk of IA, present in 87% of patients with IA in the referenced study (OR: 3.7 95% CI: 1.5-9.3), the subsequent diagnostic performance parameters [positive predictive value (PPV): 21%; negative predictive value (NPV): 93%] indicate the best way to use the polymorphism is to consider a reduced risk of, or resistance to IA in the absence of the major allele.
Conversely, other well characterized polymorphisms (DC-SIGN rs11465384 and rs7248637) can be much less evident in the patient without IA (11-14%) and while only present in 28-33% of patients with IA, the association between SNPs and IA remains significant (P: 0.001-0.004). Subsequent diagnostic performance parameters place the emphasis on increased risk of IA in the presence of the SNPs (specificity 86-89%), but not sufficiently to accurate predict IA (PPV: 33%) solely on the presence of these polymorphisms (White et al., 2017). While looking for the presence of a combination of SNPs (e.g. Dectin-1 rs7309123, DC-SIGN rs11465384 and rs7248637) previously independently associated with IA can increase the significance of the association (OR: 4.5, 95% CI: 2.2-9.2, P<0.001), the probability of predicting IA is only marginally improved (PPV: 39%). Given the presence of multiple positive biomarkers (Aspergillus PCR and GM) results already provide a probability of IA superior to this (PPV: 55%) shows that polymorphisms alone will not provide sufficient confidence for pre-empting or diagnosing IA (Barnes et al., 2013). Combining genetic screening with clinical risk and diagnostic testing is necessary, and may be further enhanced by monitoring the host’s RNA expression levels (transcriptomics) and immune functionality (proteomics/metabolomics) in response to stimulus by Aspergillus and its antigens (Figure 1).
Through stepwise logistic regression analysis, the AspBIOmics consortium developed a predictive model incorporating significant SNPs (IFN-γ, IL8, IL12p70 and VEGFA) with demographic and clinical risk factors for IA (age, gender, allogeneic SCT, anti-fungal prophylaxis) and compared the discriminatory capacity of this model with a reference model incorporating only demographic/clinical risk factors (Lupiañez et al., 2015). Through received operator characteristic (ROC) analysis the combined SNP/Demographic/Clinical model was shown to provide improved discrimination [Area under curve (AUC): 0.659] over the reference model (AUC: 0.564), highlighting the benefit of incorporating genomics. However, the improved AUC of the combined predictive model can still only be considered of moderate diagnostic capacity and using a threshold to achieve a specificity of 90% would only provide a sensitivity of <30% and vice versa (Lupiañez et al., 2015).
Taking this approach further, one retrospective study combined clinical and genetic risk with mycological evidence of IA in 322 hematology patients, 54 with proven/probable IA (White et al., 2017). Seven genetic markers previously associated with risk of IA were assessed and through stepwise multi-regression analysis a final model incorporating three SNPs (dectin-1 rs7309123, DC-SIGN rs11465384and rs7248637), two clinical risk factors (allogeneic SCT, prior respiratory virus infection) and one mycological test (Aspergillus PCR, GM being excluded as it was used to classify IA) was developed. In the absence of an allogeneic SCT, the risk of IA was low (<5% in 60% of patients without an allogenic SCT), with only 2% of this cohort being at >50% risk when all variables were met (White et al., 2017). The distribution was significantly different in the allogeneic SCT patient, with 96% of patients with risk >5% and 44% of patients with a risk >50%, when a patient was Aspergillus PCR positive with prior respiratory virus or multiple genetic risk markers. In 10% of allogeneic SCT patients who were Aspergillus PCR positive, with prior respiratory virus infection and multiple genetic risk markers the probability of IA was 90%. If the patient lacked any of the significant variables the probability of developing IA was <2%, whereas if four or more variables were present the probability of IA was 79%. An optimal threshold of three variables provided a mean probability of developing IA of 56.7%, compared to 6.3% in patients with <3 variables.
IA in the high risk hematology patient can be confidently excluded though clinical risk modelling but more so combined biomarker screening. Through the combination of clinical and genetic risk with mycological evidence, we are approaching a time when we should be able to accurately predict the risk of IA in the hematology patient. Routine screening for genetic variation that increases risk of IA can help stratify patients, permitting targeted prophylaxis in high risk patients and help to determine the significance of fungal biomarker positivity in patients without overt clinical signs, improving the accuracy of administrating pre-emptive therapy. (Figure 1) It can identify genetic risk in patients that could be rectified by allogeneic SCT, where the donor’s genome lacks any significant polymorphisms associated with IA. Conversely, screening the donor’s genome can identify variants that will further increase the risk of IA post-SCT. However, inconsistencies in the association of specific SNPs with risk of IA need to be resolved through large scale, multi-center trials of promising candidate genes (e.g. PTX3, TLR-4, Dectin-1). Studies combining all parameters are limited and prospective evaluation of such models is required but is complicated by our expanding knowledge of genetic and clinical risks, which will drive the continual development of such modelling. Therefore it is essential that predictive models be automated and easily accessible to the clinician (e.g. BOSCORE - smartphone), which will allow developments in the field to be more easily incorporated but all also provide rapid and convenient results (Stanzani and Lewis, 2018). Understanding the host and their immune responses to infection through genomics, transcriptomics and metabolomics/proteomics is critical to achieving how we manage the individual’s risk of IA, underpinning personalized medicine.
This paper was submitted as part of the supplement on the Proceedings of the Mycology 2021 meeting.
Both authors made a significant contribution to the work reported, whether that is in the conception, study design, execution, acquisition of data, analysis and interpretation, or in all these areas; took part in drafting, revising or critically reviewing the article; gave final approval of the version to be published; have agreed on the journal to which the article has been submitted; and agree to be accountable for all aspects of the work.
PW: performed diagnostic evaluations and received meeting sponsorship from Bruker, Dynamiker, and Launch Diagnostics; received speaker fees, expert advice fees, and meeting sponsorship from Gilead; received speaker and expert advice fees from F2G and speaker fees MSD and Pfizer; and is a founding member of the European Aspergillus PCR Initiative.
The remaining author declares that the research was conducted in the absence of any commercial or financial relationships that could be construed as a potential conflict of interest.
All claims expressed in this article are solely those of the authors and do not necessarily represent those of their affiliated organizations, or those of the publisher, the editors and the reviewers. Any product that may be evaluated in this article, or claim that may be made by its manufacturer, is not guaranteed or endorsed by the publisher.
Aguado, J. M., Vázquez, L., Fernández-Ruiz, M., Villaescusa, T., Ruiz-Camps, I., Barba, P., et al. (2015). PCRAGA Study Group; Spanish Stem Cell Transplantation Group; Study Group of Medical Mycology of the Spanish Society of Clinical Microbiology and Infectious Diseases; Spanish Network for Research in Infectious Diseases. Serum Galactomannan Versus a Combination of Galactomannan and Polymerase Chain Reaction-Based Aspergillus DNA Detection for Early Therapy of Invasive Aspergillosis in High-Risk Hematological Patients: A Randomized Controlled Trial. Clin. Infect. Dis. 60 (3), 405–414. doi: 10.1093/cid/ciu833
Arvanitis, M., Anagnostou, T., Mylonakis, E. (2015). Galactomannan and Polymerase Chain Reaction-Based Screening for Invasive Aspergillosis Among High-Risk Hematology Patients: A Diagnostic Meta-Analysis. Clin. Infect. Dis. 61 (8), 1263–1272. doi: 10.1093/cid/civ555
Barnes, R. A., Stocking, K., Bowden, S., Poynton, M. H., White, P. L. (2013). Prevention and Diagnosis of Invasive Fungal Disease in High-Risk Patients Within an Integrative Care Pathway. J. Infect. 67 (3), 206–214. doi: 10.1016/j.jinf.2013.04.020
Barnes, R. A., White, P. L., Morton, C. O., Rogers, T. R., Cruciani, M., Loeffler, J., et al. (2018). Diagnosis of Aspergillosis by PCR: Clinical Considerations and Technical Tips. Med. Mycol. 56 (suppl_1), 60–72. doi: 10.1093/mmy/myx091
Bochud, P. Y., Chien, J. W., Marr, K. A., Leisenring, W. M., Upton, A., Janer, M., et al. (2008). Toll-Like Receptor 4 Polymorphisms and Aspergillosis in Stem-Cell Transplantation. N. Engl. J. Med. 359 (17), 1766–1777. doi: 10.1056/NEJMoa0802629
Carvalho, A., Cunha, C., Carotti, A., Aloisi, T., Guarrera, O., Di Ianni, M., et al. (2009). Polymorphisms in Toll-Like Receptor Genes and Susceptibility to Infections in Allogeneic Stem Cell Transplantation. Exp. Hematol. 37 (9), 1022–1029. doi: 10.1016/j.exphem.2009.06.004
Carvalho, A., De Luca, A., Bozza, S., Cunha, C., D'Angelo, C., Moretti, S., et al. (2012). TLR3 Essentially Promotes Protective Class I-Restricted Memory CD8+ T-Cell Responses to Aspergillus Fumigatus Inhematopoietic Transplanted Patients. Blood 119, 967–977. doi: 10.1182/blood-2011-06-362582
Carvalho, A., Pasqualotto, A. C., Pitzurra, L., Romani, L., Denning, D. W., Rodrigues, F. (2008). Polymorphisms in Toll-Like Receptor Genes and Susceptibility to Pulmonary Aspergillosis. J. Infect. Dis. 197 (4), 618–621. doi: 10.1086/526500
Chen, M. J., Hu, R., Jiang, X. Y., Wu, Y., He, Z. P., Chen, J. Y., et al. (2019). Dectin-1 Rs3901533 and Rs7309123 Polymorphisms Increase Susceptibility to Pulmonary Invasive Fungal Disease in Patients With Acute Myeloid Leukemia From a Chinese Han Population. Curr. Med. Sci. 39 (6), 906–912. doi: 10.1007/s11596-019-2122-3
Cruciani, M., Mengoli, C., Barnes, R., Donnelly, J. P., Loeffler, J., Jones, B. L., et al. (2019). Polymerase Chain Reaction Blood Tests for the Diagnosis of Invasive Aspergillosis in Immunocompromised People. Cochrane Database Syst. Rev. 9 (9), CD009551. doi: 10.1002/14651858.CD009551.pub4
Cunha, C., Aversa, F., Lacerda, J. F., Busca, A., Kurzai, O., Grube, M., et al. (2014). Genetic PTX3 Deficiency and Aspergillosis in Stem-Cell Transplantation. N. Engl. J. Med. 370 (5), 421–432. doi: 10.1056/NEJMoa1211161
Cunha, C., Carvalho, A. (2019). Genetic Defects in Fungal Recognition and Susceptibility to Invasive Pulmonary Aspergillosis. Med. Mycol. 57 (Supplement_2), S211–S218. doi: 10.1093/mmy/myy057
Cunha, C., Di Ianni, M., Bozza, S., Giovannini, G., Zagarella, S., Zelante, T., et al. (2010). Dectin-1 Y238X Polymorphism Associates With Susceptibility to Invasive Aspergillosis in Hematopoietic Transplantation Through Impairment of Both Recipient- and Donor-Dependent Mechanisms of Antifungal Immunity. Blood 116 (24), 5394–5402. doi: 10.1182/blood-2010-04-279307
Cunha, C., Giovannini, G., Pierini, A., Bell, A. S., Sorci, G., Riuzzi, F., et al. (2011). Genetically-Determined Hyperfunction of the S100B/RAGE Axis Is a Risk Factor for Aspergillosis in Stem Cell Transplant Recipients. PloS One 6 (11), e27962. doi: 10.1371/journal.pone.0027962
Cunha, C., Gonçalves, S. M., Duarte-Oliveira, C., Leite, L., Lagrou, K., Marques, A., et al. (2017). IL-10 Overexpression Predisposes to Invasive Aspergillosis by Suppressing Antifungal Immunity. J. Allergy Clin. Immunol. 140 (3), 867–870.e9. doi: 10.1016/j.jaci.2017.02.034
Cunha, D. O., Leão-Cordeiro, J. A. B., Paula, H. D. S. C., Ataides, F. S., Saddi, V. A., Vilanova-Costa, C. A. S. T., et al. (2018). Association Between Polymorphisms in the Genes Encoding Toll-Like Receptors and Dectin-1 and Susceptibility to Invasive Aspergillosis: A Systematic Review. Rev. Soc. Bras. Med. Trop. 51 (6), 725–730. doi: 10.1590/0037-8682-0314-2018
Cunha, C., Monteiro, A. A., Oliveira-Coelho, A., Kühne, J., Rodrigues, F., Sasaki, S. D., et al. (2015). PTX3-Based Genetic Testing for Risk of Aspergillosis After Lung Transplant. Clin. Infect. Dis. 61 (12), 1893–1894. doi: 10.1093/cid/civ679
Danion, F., Aimanianda, V., Bayry, J., Duréault, A., Wong, S. S. W., Bougnoux, M. E., et al. (2020). Aspergillus Fumigatus Infection in Humans With STAT3-Deficiency Is Associated With Defective Interferon-Gamma and Th17 Responses. Front. Immunol. 11. doi: 10.3389/fimmu.2020.00038
de Boer, M. G., Jolink, H., Halkes, C. J., van der Heiden, P. L., Kremer, D., Falkenburg, J. H., et al. (2011). Influence of Polymorphisms in Innate Immunity Genes on Susceptibility to Invasive Aspergillosis After Stem Cell Transplantation. PloS One 6 (4), e18403. doi: 10.1371/journal.pone.0018403
Fischer, M., Spies-Weisshart, B., Schrenk, K., Gruhn, B., Wittig, S., Glaser, A., et al. (2016). Polymorphisms of Dectin-1 and TLR2 Predispose to Invasive Fungal Disease in Patients With Acute Myeloid Leukemia. PloS One 11 (3), e0150632. doi: 10.1371/journal.pone.0150632
Fisher, C. E., Hohl, T. M., Fan, W., Storer, B. E., Levine, D. M., Zhao, L. P., et al. (2017). Validation of Single Nucleotide Polymorphisms in Invasive Aspergillosis Following Hematopoietic Cell Transplantation. Blood 129 (19), 2693–2701. doi: 10.1182/blood-2016-10-743294
Gresnigt, M. S., Cunha, C., Jaeger, M., Gonçalves, S. M., Malireddi, R. K. S., Ammerdorffer, A., et al. (2018). Genetic Deficiency of NOD2 Confers Resistance to Invasive Aspergillosis. Nat. Commun. 9 (1), 2636. doi: 10.1038/s41467-018-04912-3
Griffiths, J. S., White, P. L., Thompson, A., da Fonseca, D. M., Pickering, R. J., Ingram, W., et al. (2021). A Novel Strategy to Identify Haematology Patients at High Risk of Developing Aspergillosis. Front. Immunol. 12. doi: 10.3389/fimmu.2021.780160
Grube, M., Loeffler, J., Mezger, M. (2013). TLR5 Stop Codon Polymorphism Is Associated With Invasive Aspergillosis After Allogeneic Stem Cell Transplantation. Med. Mycol. 51, 818–825. doi: 10.3109/13693786.2013.809630
Hardison, S. E., Brown, G. D. (2012). C-Type Lectin Receptors Orchestrate Antifungal Immunity. Nat. Immunol. 13 (9), 817–822. doi: 10.1038/ni.2369
He, Q., Li, H., Rui, Y., Liu, L., He, B., Shi, Y., et al. (2018). Pentraxin 3 Gene Polymorphisms and Pulmonary Aspergillosis in Chronic Obstructive Pulmonary Disease Patients. Clin. Infect. Dis. 66 (2), 261–267. doi: 10.1093/cid/cix749
Kang, Y., Yu, Y., Lu, L. (2020). The Role of Pentraxin 3 in Aspergillosis: Reality and Prospects. Mycobiology 48 (1), 1–8. doi: 10.1080/12298093.2020.1722576
Kesh, S., Mensah, N. Y., Peterlongo, P., Jaffe, D., Hsu, K., VAN DEN Brink, M., et al. (2005). TLR1 and TLR6 Polymorphisms Are Associated With Susceptibility to Invasive Aspergillosis After Allogeneic Stem Cell Transplantation. Ann. N. Y. Acad. Sci. 1062, 95–103. doi: 10.1196/annals.1358.012
Koldehoff, M., Beelen, D. W., Elmaagacli, A. H. (2013). Increased Susceptibility for Aspergillosis and Post-Transplant Immune Deficiency in Patients With Gene Variants of TLR4 After Stem Cell Transplantation. Transpl. Infect. Dis. 15 (5), 533–539. doi: 10.1111/tid.12115
Lambourne, J., Agranoff, D., Herbrecht, R., Troke, P. F., Buchbinder, A., Willis, F., et al. (2009). Association of Mannose-Binding Lectin Deficiency With Acute Invasive Aspergillosis in Immunocompromised Patients. Clin. Infect. Dis. 49 (10), 1486–1491. doi: 10.1086/644619
Lupiañez, C. B., Canet, L. M., Carvalho, A., Alcazar-Fuoli, L., Springer, J., Lackner, M., et al. (2015). Polymorphisms in Host Immunity-Modulating Genes and Risk of Invasive Aspergillosis: Results From the AspBIOmics Consortium. Infect. Immun. 84 (3), 643–657. doi: 10.1128/IAI.01359-15
Mezger, M., Steffens, M., Beyer, M., Manger, C., Eberle, J., Toliat, M. R., et al. (2008). Polymorphisms in the Chemokine (C-X-C Motif) Ligand 10 Are Associated With Invasive Aspergillosis After Allogeneic Stem-Cell Transplantation and Influence CXCL10 Expression in Monocyte-Derived Dendritic Cells. Blood 111 (2), 534–536. doi: 10.1182/blood-2007-05-090928
Portugal, R. D., Garnica, M., Nucci, M. (2009). Index to Predict Invasive Mold Infection in High-Risk Neutropenic Patients Based on the Area Over the Neutrophil Curve. J. Clin. Oncol. 27 (23), 3849–3854. doi: 10.1200/JCO.2008.21.0856
Rhodes, J., Abdolrasouli, A., Dunne, K., Sewell, T. R., Zhang, Y., Ballard, E., et al. Tracing Patterns of Evolution and Acquisition of Drug Resistant Aspergillus Fumigatus Infection From the Environment Using Population Genomics. Nat. Microbiol. (Forthcoming). doi: 2021.04.07.438821
Rieber, N., Gazendam, R. P., Freeman, A. F., Hsu, A. P., Collar, A. L., Sugui, J. A., et al. (2016). Extrapulmonary Aspergillus Infection in Patients With CARD9 Deficiency. JCI Insight 1 (17), e89890. doi: 10.1172/jci.insight.89890
Sainz, J., Lupiáñez, C. B., Segura-Catena, J., Vazquez, L., Ríos, R., Oyonarte, S., et al. (2012). Dectin-1 and DC-SIGN Polymorphisms Associated With Invasive Pulmonary Aspergillosis Infection. PloS One 7 (2), e32273. doi: 10.1371/journal.pone.0032273
Sainz, J., Salas-Alvarado, I., López-Fernández, E., Olmedo, C., Comino, A., García, F., et al. (2010). TNFR1 mRNA Expression Level and TNFR1 Gene Polymorphisms Are Predictive Markers for Susceptibility to Develop Invasive Pulmonary Aspergillosis. Int. J. Immunopathol. Pharmacol. 23 (2), 423–436. doi: 10.1177/039463201002300205
Salazar, F., Brown, G. D. (2018). Antifungal Innate Immunity: A Perspective From the Last 10 Years. J. Innate Immun. 10 (5-6), 373–397. doi: 10.1159/000488539
Sánchez-Maldonado, J. M., Moñiz-Díez, A., Ter Horst, R., Campa, D., Cabrera-Serrano, A. J., Martínez-Bueno, M., et al. (2020). Polymorphisms Within the TNFSF4 and MAPKAPK2 Loci Influence the Risk of Developing Invasive Aspergillosis: A Two-Stage Case Control Study in the Context of the Aspbiomics Consortium. J. Fungi (Basel) 7 (1), 4. doi: 10.3390/jof7010004
Seo, K. W., Kim, D. H., Sohn, S. K., Lee, N. Y., Chang, H. H., Kim, S. W., et al. (2005). Protective Role of Interleukin-10 Promoter Gene Polymorphism in the Pathogenesis of Invasive Pulmonary Aspergillosis After Allogeneic Stem Cell Transplantation. Bone Marrow Transplant. 36, 1089–1095. doi: 10.1038/sj.bmt.1705181
Stanzani, M., Lewis, R. E. (2018). Development and Applications of Prognostic Risk Models in the Management of Invasive Mold Disease. J. Fungi (Basel) 4 (4), 141. doi: 10.3390/jof4040141
Stappers, M. H. T., Clark, A. E., Aimanianda, V., Bidula, S., Reid, D. M., Asamaphan, P., et al. (2018). Recognition of DHN-Melanin by a C-Type Lectin Receptor Is Required for Immunity to Aspergillus. Nature 555, 382–386. doi: 10.1038/nature25974
Tanpaibule, T., Jinawath, N., Taweewongsounton, A., Niparuck, P., Rotjanapan, P. (2020). Genetic Risk Surveillance for Invasive Aspergillosis in Hematology Patients: A Prospective Observational Study. Infect. Dis. Ther. 9 (4), 807–821. doi: 10.1007/s40121-020-00331-4
van der Velden, W. J., Blijlevens, N. M., Donnelly, J. P. (2011). Genetic Variants and the Risk for Invasive Mould Disease in Immunocompromised Hematology Patients. Curr. Opin. Infect. Dis. 24 (6), 554–563. doi: 10.1097/QCO.0b013e32834ab1f4
Wang, J., Zhou, M., Xu, J. Y., Zhou, R. F., Chen, B., Wan, Y. (2020). Comparison of Antifungal Prophylaxis Drugs in Patients With Hematological Disease or Undergoing Hematopoietic Stem Cell Transplantation: A Systematic Review and Network Meta-Analysis. JAMA Netw. Open 3 (10), e2017652. doi: 10.1001/jamanetworkopen.2020.17652
White, P. L., Parr, C., Barnes, R. A. (2017). Predicting Invasive Aspergillosis in Hematology Patients by Combining Clinical and Genetic Risk Factors With Early Diagnostic Biomarkers. J. Clin. Microbiol. 56 (1), e01122-17. doi: 10.1128/JCM.01122-17
Wójtowicz, A., Lecompte, T. D., Bibert, S., Manuel, O., Rüeger, S., Berger, C., et al. (2015). PTX3 Polymorphisms and Invasive Mold Infections After Solid Organ Transplant. Clin. Infect. Dis. 61 (4), 619–622. doi: 10.1093/cid/civ386
Zaas, A. K., Liao, G., Chien, J. W., Weinberg, C., Shore, D., Giles, S. S., et al. (2008). Plasminogen Alleles Influence Susceptibility to Invasive Aspergillosis. PloS Genet. 4 (6), e1000101. doi: 10.1371/journal.pgen.1000101
Zhou, P., Xie, Y., Yan, Z., Liu, X., Hua, H. (2019). Association Between Dectin-1 Gene Single Nucleotide Polymorphisms and Fungal Infection: A Systemic Review and Meta-Analysis. Biosci. Rep. 39 (11), BSR20191519. doi: 10.1042/BSR20191519
Zoran, T., Seelbinder, B., White, P. L., Price, J. S., Kraus, S., Kurzai, O., et al. (2022). Molecular Profiling Reveals Characteristic and Decisive Signatures in Patients After Allogeneic Stem Cell Transplantation Suffering From Invasive Pulmonary Aspergillosis. J. Fungi. 8 (2), 171. doi: 10.3390/jof8020171
Zoran, T., Weber, M., Springer, J., White, P. L., Bauer, J., Schober, A., et al. (2019). Treatment With Etanercept and Low Monocyte Concentration Contribute to the Risk of Invasive Aspergillosis in Patients Post Allogeneic Stem Cell Transplantation. Sci. Rep. 9 (1), 17231. doi: 10.1038/s41598-019-53504-8
Keywords: invasive aspergillosis, predictive models, clinical risk, genetic risk, SNPs
Citation: White PL and Price JS (2022) Incorporating the Detection of Single Nucleotide Polymorphisms Associated With Invasive Aspergillosis Into the Clinic. Front. Cell. Infect. Microbiol. 12:860779. doi: 10.3389/fcimb.2022.860779
Received: 23 January 2022; Accepted: 04 March 2022;
Published: 12 April 2022.
Edited by:
Brian Wickes, The University of Texas Health Science Center at San Antonio, United StatesReviewed by:
Agostinho Carvalho, University of Minho, PortugalCopyright © 2022 White and Price. This is an open-access article distributed under the terms of the Creative Commons Attribution License (CC BY). The use, distribution or reproduction in other forums is permitted, provided the original author(s) and the copyright owner(s) are credited and that the original publication in this journal is cited, in accordance with accepted academic practice. No use, distribution or reproduction is permitted which does not comply with these terms.
*Correspondence: P. Lewis White, bGV3aXMud2hpdGVAd2FsZXMubmhzLnVr
Disclaimer: All claims expressed in this article are solely those of the authors and do not necessarily represent those of their affiliated organizations, or those of the publisher, the editors and the reviewers. Any product that may be evaluated in this article or claim that may be made by its manufacturer is not guaranteed or endorsed by the publisher.
Research integrity at Frontiers
Learn more about the work of our research integrity team to safeguard the quality of each article we publish.