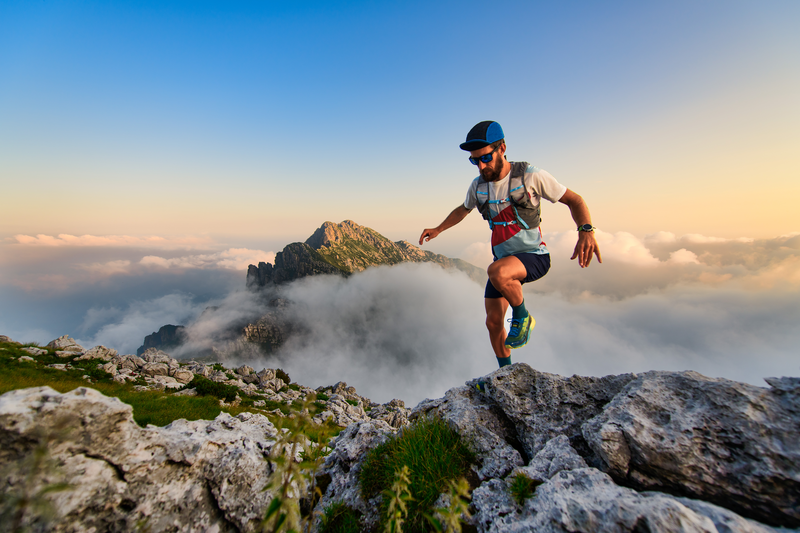
94% of researchers rate our articles as excellent or good
Learn more about the work of our research integrity team to safeguard the quality of each article we publish.
Find out more
ORIGINAL RESEARCH article
Front. Cell. Infect. Microbiol. , 17 February 2022
Sec. Parasite and Host
Volume 12 - 2022 | https://doi.org/10.3389/fcimb.2022.834850
This article is part of the Research Topic Insights in Parasite and Host: 2021 View all 9 articles
During co-evolution Plasmodium parasites and vertebrates went through a process of selection resulting in defined and preferred parasite-host combinations. As such, Plasmodium falciparum (Pf) sporozoites can infect human hepatocytes while seemingly incompatible with host cellular machinery of other species. The compatibility between parasite invasion ligands and their respective human hepatocyte receptors plays a key role in Pf host selectivity. However, it is unclear whether the ability of Pf sporozoites to mature in cross-species infection also plays a role in host tropism. Here we used fresh hepatocytes isolated from porcine livers to study permissiveness to Pf sporozoite invasion and development. We monitored intra-hepatic development via immunofluorescence using anti-HSP70, MSP1, EXP1, and EXP2 antibodies. Our data shows that Pf sporozoites can invade non-human hepatocytes and undergo partial maturation with a significant decrease in schizont numbers between day three and day five. A possible explanation is that Pf sporozoites fail to form a parasitophorous vacuolar membrane (PVM) during invasion. Indeed, the observed aberrant EXP1 and EXP2 staining supports the presence of an atypical PVM. Functions of the PVM include the transport of nutrients, export of waste, and offering a protective barrier against intracellular host effectors. Therefore, an atypical PVM likely results in deficiencies that may detrimentally impact parasite development at multiple levels. In summary, despite successful invasion of porcine hepatocytes, Pf development arrests at mid-stage, possibly due to an inability to mobilize critical nutrients across the PVM. These findings underscore the potential of a porcine liver model for understanding the importance of host factors required for Pf mid-liver stage development.
Plasmodium falciparum (Pf) is the causative agent for most of the mortality and morbidity associated with malaria. The disease begins when a human is bitten by an infectious mosquito and parasites (sporozoites) are injected into the skin and travel to the liver. In the liver, sporozoites traverse or transmigrate through multiple hepatocytes. Eventually, the parasites undergo productive invasion of a final hepatocyte, resulting in the parasite being surrounded by a parasitophorous vacuole made up of the parasitophorous vacuolar membrane (PVM) of host origin (Vaughan and Kappe, 2017). The PVM gets modified by the parasite as it develops and matures over the subsequent period of seven days, presumably to support the acquisition of host nutrients needed for parasite growth and to act as barrier against intracellular host defenses (Nyboer et al., 2018).
A comprehensive understanding of the key processes required for successful Pf invasion and maturation within the hepatocyte is lacking, including the molecular mechanisms that define host specificity (Mota et al., 2001; Mello-Vieira et al., 2020). Although key host receptors such as cluster of differentiation 81 (CD81) (Silvie et al., 2003), class B scavenger receptor type 1 (SR-B1) (Rodrigues et al., 2008), Ephrin A2 (Kaushansky et al., 2015), and highly sulfated heparin sulfate proteoglycans (HSPGs) (Coppi et al., 2007) have been identified as playing a role, their reciprocal interacting partners in the parasite remain largely unknown. Similarly, while there is an abundant number of parasite proteins implicated in sporozoite entry into hepatocytes such as circumsporozoite protein (CSP) [reviewed in (Sinnis and Coppi, 2007)], apical merozoite antigen 1 (AMA1), merozoite apical erythrocyte-binding ligand (MAEBL) (Yang et al., 2017), and the thrombospondin-related anonymous protein (TRAP) (Ejigiri et al., 2012), many of the corresponding host ligands remain to be identified. It is unclear why Pf sporozoites show exclusive human tropism, with natural infection appearing limited to humans (Liu et al., 2016; Sato, 2021), unlike their rodent malaria counterparts P. yoelii (Py) and P. berghei (Pb) (Prudencio et al., 2011). A prevailing theory is the narrow pre-defined window of parasite-host selectivity. For instance, Silvie et al. (2003) showed that Pf sporozoites are unable to achieve invasion in mouse hepatocytes while Py sporozoites can invade both human and mouse hepatocytes (Silvie et al., 2003). While this makes logical sense as mice are very evolutionary distant from humans, it remains unclear whether Pf can infect and mature in non-human hepatocytes from a more closely related species such as pigs, which have been used as general surgical models, as pharmacological models, in xenotransplantation research, in gene modification research, and in toxicology and immunological studies (Wernersson et al., 2005; Walters and Prather, 2013; Meier et al., 2015). Identifying potential hidden host reservoirs for malaria parasites would be critically relevant for elimination of the disease in the future. Additionally, a better understanding of the molecular mechanism behind sporozoite invasion and maturation is key to the identification of novel treatment targets. Here, we investigated whether Pf sporozoites can invade and mature in porcine hepatocytes.
Primary human hepatocytes were obtained as remnant surgical material from patients undergoing partial hepatectomy at the Radboud University Medical Center (Radboudumc). Human samples were anonymized, and general approval for use of remnant surgical material was granted in accordance to the Dutch ethical legislation, as described in the Medical Research (Human Subjects) Act and confirmed by the Committee on Research involving Human Subjects, in the region of Arnhem-Nijmegen, the Netherlands. Primary porcine hepatocytes were obtained as remnant surgical material from minipigs (Sus scrofa domesticus) undergoing surgical procedures for research and training purposes and were provided by the Animal Research Facility of the Radboudumc.
The viability of porcine hepatocytes was determined by measuring their overall mitochondrial enzymatic activity using 3-(4,5-dimethylthiazol-2-yl)-2,5-diphenyltetrazolium bromide (MTT). MTT was dissolved in phosphate buffered saline (PBS) at a concentration of 5 mg/mL and was added to each well. Hepatocytes were incubated for four hours at 37°C. Subsequently, the MTT solution was removed, and dimethyl sulfoxide (DMSO) was added to the culture for five minutes to dissolve formazan crystals. Next, the amount of converted MTT was determined by measuring the extinction of the well. The analysis was performed with the iMarkTM Microplate Reader (Bio-Rad). Extinction was measured at a wavelength of 570 nm. The reference wavelength was set at 630 nm.
Pf NF135.C10 asexual stages were generated as described previously (Yang et al., 2021). This clone originates from a clinical isolate in Cambodia (Teirlinck et al., 2013). Briefly, asexual and sexual blood stages were cultured in a semi-automated culture system. Sporozoites were produced by feeding Anopheles stephensi (Sind-Kasur Nijmegen strain) using standard membrane feeding of cultured gametocytes. Salivary glands were dissected by hand, collected in William’s B medium [William’s E medium with Glutamax (Thermo Fisher, 32551-087), supplemented with 1× insulin/transferrin/selenium (Thermo Fisher, 41400-045), 1 mM sodium pyruvate (Thermo Fisher, 11360-070), 1× MEM-NEAA (Thermo Fisher, 11140-035), 2.5 µg/ml Fungizone (Thermo Fisher 15290-018), 200 U/ml penicillin/streptomycin (Thermo Fisher 15140-122), and 1.6 µM dexamethasone (Sigma-Aldrich D4902-100MG)], and homogenized in a custom-made glass grinder. Sporozoites were counted in a Bürker-Türk counting chamber using phase-contrast microscopy. All sporozoites were supplemented with heat inactivated human serum at 10% of the total volume prior to hepatocyte infection.
The isolation and infection of fresh hepatocytes has been described elsewhere (Walk et al., 2017; Yang et al., 2021). Briefly, viable hepatocytes were suspended in complete William’s B medium without serum. Hepatocytes were seeded into 96-well flat-bottom plates at a seeding density of 62.500 hepatocytes per well and cultured at 37°C in an atmosphere of 5% CO2 with daily media refreshment.
Two days after plating, sporozoites were added to the wells at a 1:1 ratio. Media was refreshed after three hours to remove non-invaded sporozoites and refreshed daily throughout the time-course. After time-course completion, cells were fixed with 4% paraformaldehyde (Thermo Fisher Scientific: catalogue number 28906) for ten minutes.
After each incubation step, cells were washed thrice with PBS. First, fixed cells were permeabilized with 1% triton x-100 for ten minutes at room temperature. Subsequently, cells were incubated with 0.1 M glycine to avoid nonspecific binding of antibodies to free aldehyde groups. Cells were then incubated with 3% bovine serum albumin for forty-five minutes. Next, cells were incubated with primary antibodies for one hour at room temperature: rabbit anti-Pf HSP70 (Heat Shock Protein, 1:75 dilution), mouse anti-Pf MSP1 (Merozoites Surface Protein, 1:100 dilution), mouse anti-Pf EXP1 (Exported Protein 1, 1:1000 dilution), mouse anti-Pf EXP2 (Exported Protein 2, 1:1000 dilution) or goat anti-Plasmodium berghei (Pb) UIS4 (Upregulated in Infectious Sporozoites 4, 1:300 dilution). Cells were subsequently incubated with secondary antibodies (1:200 dilution) for one hour at room temperature in the dark: goat anti-rabbit Alexa 594 for PfHSP70, donkey anti-mouse Alexa 647 for anti-PfMSP1 staining, goat anti-mouse Alexa 488 for anti-PfEXP1 and anti-PfEXP2 staining, and donkey anti-goat Alexa 594. Subsequently, nuclei were stained with chromatin-specific 4’,6-diamidino-2-phenylindole (DAPI, 1:200 dilution) and incubated for one hour in the dark. Finally, 0.1% sodium azide was added. Cells were stored at 4°C.
Monolayer viability was assessed by brightfield microscopy at 400x magnification. Following immunofluorescent staining, parasite invasion into hepatocytes was visualized with a Leica DMI6000B inverted epifluorescent high content microscope. For each well, a tile size of 9x9 was obtained using a 20x objective. Tiles were manually counted based on PfHSP70 and DAPI positivity in FIJI. Schizonts were characterized using the region of interest (ROI) tool to determine the cross-sectional surface area, circumference, and raw integrated density (RawIntDen) of each manually encircled schizont. The RawIntDen values give the sum of all pixel values in the ROI. The RawIntDen value was corrected for background noise. Background noise was calculated by measuring the total intensity of a whole image divided by the area of the image. The final intensity of the parasite was calculated by subtracting the background value from the measured value. Confocal images were obtained with a Zeiss LSM880 microscope with Airyscan using a 63x (oil) objective and 2x zoom.
A master mix was prepared including 10% heat inactivated human serum, 100 mg/ml FITC dextran, sporozoites (1:1 infection), and William’s B medium, and was added to each well. Controls included master mix containing no sporozoites, and master mix containing cytochalasin D (1:100 dilution). Cells were spun at 3000 revolutions per minute for ten minutes and stored at 37°C for two hours. Subsequently, cells were washed with PBS. Trypsin was added per well and cells were kept at 37°C for five minutes. Finally, 10% fetal bovine serum in PBS was added to each well. Traversal data was obtained by flow cytometry (Beckman Coulter Gallios 10-color), and analysis was performed using FlowJo software (version 10.0.8, Tree Star).
Three biological replicates were used for fresh porcine hepatocytes, and three biological replicates were used for human hepatocytes, of which two replicates were cryopreserved human hepatocytes and one was fresh. Each biological replicate had at least two technical replicates. All statistical tests were performed using Prism 9 (version 9.2.0, GraphPad Software, California USA).
Fresh porcine hepatocytes were isolated based on human protocols as described previously Yang et al. (2021) (Yang et al., 2021). Different seeding densities were compared, and the viability of each density was assessed using brightfield microscopy and mitochondrial enzymatic activity (MTT) from day of invasion up to day five (Figures 1A, B). For all seeding densities, metabolic activity increased post-plating (up to seventy-two hours post-invasion) and remained steady as measured by MTT (Figure 1B). After day seven, the viability of the culture decreased with clumping of cells observed by brightfield microscopy. An optimal seeding density of 62.500 was chosen, as at higher densities the host cells tended to clump instead of forming monolayers (image not shown). This is in line with the seeding density used for primary human hepatocytes (Yang et al., 2021).
Figure 1 (A) Microscopic images of freshly seeded porcine hepatocytes at 62.500, 75.000 and 90.000 hepatocytes per well on days two, three and five post-invasion. (B) Viability of freshly isolated porcine hepatocytes. Porcine hepatocytes were seeded at densities of 62.500, 81.250 and 100.000 hepatocytes per well. MTT levels are depicted of uninfected control hepatocytes on day three, day five, and day seven post-invasion. Mean MTT reading and standard deviation of four technical replicates of two biological replicates. The extinction was measured at a wavelength of 570 nm. The reference wavelength was set at 630 nm. (C, D) Traversal of NF135.C10 sporozoites in fresh human and porcine hepatocytes three hours post-invasion of three biological donors, each with three technical replicates. (C) Fluorescence activated cell sorting gating strategy used to study traversal. Top left panel: selection of hepatocytes. Top right panel: selection of single cells. Bottom left panel: hepatocytes incubated in FITC-Dextran only (negative control). Bottom right panel: hepatocytes incubated with FITC-Dextran and sporozoites. For both bottom panels, the y-axis shows the degree of FITC-positivity in the cells. For all panels, each dot represents a cell/event measured. (D) Mean percentage (± SD) of traversed porcine and human hepatocytes.
Both human (freshly isolated and cryopreserved) and porcine (fresh) hepatocytes were subsequently infected with NF135.C10 sporozoites two days after plating. Traversal assays were performed for three hours post-invasion and a significant presence of FITC dextran-positive cells were detected, indicating NF135.C10 sporozoites were able to traverse porcine hepatocytes, albeit at a lower rate than in human hepatocytes. The percentage of traversed hepatocytes was lower in porcine donors compared to human donors (Figures 1C, D).
To assess whether infection of porcine hepatocytes was possible, liver cells from porcine and human donors were infected with NF135.C10 sporozoites. The number of intracellular schizonts was determined by immunofluorescence assay using DAPI and HSP70 staining on day three, five, and seven post-invasion (Figure 2). NF135.C10 schizonts of comparable size were detected in human and porcine hepatocytes on day three, illustrating the permissiveness of porcine hepatocytes to support Pf development (Figure 2A). The number of intracellular schizonts was significantly lower in porcine compared to human hepatocytes (Figure 2B) (p=0.0005 on day three and p=0.0074 on day five).
Figure 2 (A) Schizont size. Mean sizes (±SD) of NF135.C10 schizonts on day three, day five and day seven post-invasion in primary porcine and human hepatocytes based on immunofluorescence staining with HSP70. Per technical replicate, all or up to seventy-five schizonts were measured for each of the biological replicates (three human and three porcine donors). A two-way RM ANOVA was performed on the median of each replicate. Mean size (±SD) of NF135.C10 schizonts per technical replicate and circumference data is shown in Supplementary Figure 1 and Supplementary Table 1 (B) Schizont number. Mean number (±SD) of NF135.C10 schizonts on day three, day five and day seven post-invasion in primary porcine and human hepatocytes based on immunofluorescence staining with HSP70 and DAPI. All schizonts were counted per technical replicate of each of the biological replicates. A two-way RM ANOVA was performed on the median of each replicate. The mean number (±SD) of NF135.C10 schizonts per technical replicate is shown in Supplementary Figure 2 (C) HSP70 signal. The median HSP70 signal (±IQR) measured per schizont on day three and day five post-invasion is shown, corrected for noise and schizont size. All or up to fifty schizonts were measured per technical replicate of the three biological replicates. A two-way RM ANOVA was performed on the median of each replicate. (D) MSP1 signal. Median MSP1 signal (±IQR) measured per schizont on day three and day five post-invasion, corrected for noise and schizont size. All or up to fifty schizonts were measured per technical replicate of three biological replicates. A two-way RM ANOVA was performed. (E) Expression of typical liver stage protein markers in porcine hepatocytes visualized by confocal microscopy including GAPDH, HSP70, MSP1 and DAPI. For comparative human images, please refer to (Yang et al., 2021). Objective 63×; zoom 2×; scale bar 25 µm. For panels A, C and D, the total number of schizonts analyzed per condition is shown in Supplementary Table 2. RawIntDen: raw integrated density. GAPDH, glyceraldehyde 3-phosphate dehydrogenase; HSP70, heat shock protein 70; IQR, interquartile range; MSP1, merozoite surface protein; NS, not significant; P.I., post-invasion; IQR, interquartile range. *P < 0.05; **P < 0.005; ***P < 0.001; ****P < 0.0001.
In the porcine cells, there was a significant decrease in schizont numbers from day three post-invasion onwards with no remaining schizonts left on day seven, indicating that parasites were unable to survive during this period. While a decrease in the number of intracellular schizonts was also observed in human hepatocytes, intracellular schizonts could still be detected on day seven post-invasion (Figure 2B). However, some degree of parasite nuclear division was observed in schizonts developing in porcine host hepatocytes, as illustrated by DAPI staining (Figure 2E). The integrated HSP70 signal intensity per schizont surface area remained relatively constant between day three and five post-invasion for both human and porcine hepatocytes, suggesting that the health of these parasites remained stable (Figure 2C). As expected, the signal was higher for parasites developing in the human hepatocytes as it is the ideal host for the parasite. Furthermore, a large increase in the MSP1 signal intensity per schizont size, a marker of maturation, was only observed in schizonts present in human hepatocytes (Figure 2D), indicating the ability of these schizonts to mature in the human host but not in the porcine host. Finally, parasites developing in the porcine host displayed typical liver stage markers such as HSP70, GAPDH and MSP1 (Figure 2E).
While schizonts developing in the porcine and human hepatocytes did not differ in size on day three post-invasion, there was a significant difference on day five post-invasion (p=0.0038) (Figure 2A). Schizonts developing in human hepatocytes increased in size from day three to day five (p=0.0008) and from day five to day seven (p=0.0006). In contrast, no significant difference in size was found from day three to day five in surviving schizonts in porcine hepatocytes (p=0.1137), indicating again that maturation is hindered in non-human host cells. Circumference data is shown in supplementary material and showed a similar trend (Figure S1).
As shown by the disappearance of schizonts from day five to day seven post-invasion as well as the steady MSP1 signal, it appears that schizonts cannot mature properly in the porcine hepatocyte system. The integrity of the PVM was next assessed using antibodies against two established PVM proteins, EXP1 and 2 (van Dijk et al., 2005; Labaied et al., 2007; Ploemen et al., 2012; Annoura et al., 2014; Kalanon et al., 2016; Manzoni et al., 2017; Yang et al., 2021). EXP1 and EXP2 have been identified as crucial PVM components for nutrient uptake and protein export in blood stages while a defined role in liver stage development remains elusive (Charnaud et al., 2018; Garten et al., 2018; Mesén-Ramírez et al., 2019; Nessel et al., 2020). In porcine hepatocytes, there was no apparent increase in EXP1 signal (Figure 3A). Although not significant, there was an increasing trend in EXP1 signal intensity observed from day three to day five for schizonts developing in human hepatocytes for some donors (Figure 3B). With regards to the EXP2 signal, there was a decrease in signal in schizonts developing in porcine hepatocytes from day three to day five whereas an increase was observed over time for schizonts in human hepatocytes (Figures 3C, D).
Figure 3 Median EXP signal (±IQR) measured per schizont in porcine hepatocytes on day three and day five post-invasion, corrected for noise and schizont size. All or up to seventy-five schizonts were measured per technical replicate of three biological replicates. A two-way RM ANOVA was performed on the median of each replicate. (A) EXP1 signal in porcine hepatocytes. (B) EXP1 signal in human hepatocytes. (C) EXP2 signal in porcine hepatocytes. (D) EXP2 signal in human hepatocytes. For all panels, the total number of schizonts analyzed per condition is shown in Supplementary Table 2. CHH, cryopreserved human hepatocytes; EXP, exported protein; FHH, fresh human hepatocytes; FPH, fresh porcine hepatocytes; NS, not significant. *P < 0.05; **P < 0.005; ****P < 0.0001.
Two main staining patterns of EXP1 and EXP2 were observed and classified as typical (circle surrounding HSP70 and DAPI staining) and atypical (Figure 4A and Figure S3A). The majority of schizonts developing in porcine hepatocytes showed atypical EXP1 and EXP2 staining, compared to schizonts developing in human hepatocytes that showed mostly a typical EXP1 and EXP2 staining pattern (Figures 4B, C). Schizonts developing in porcine hepatocytes showed an increasing trend of atypical EXP1 and EXP2 staining over time, whereas the proportion of schizonts developing in human hepatocytes with a typical pattern increased over time, although this difference was not significant.
Figure 4 (A) Representative immunofluorescence images of typical liver stage protein markers visualized through confocal microscopy including EXP1 and EXP2, HSP70, and DAPI, classified as a typical (top) or atypical (bottom) staining pattern. Images shown were obtained from infected porcine hepatocytes. Objective 63×; zoom 2×; scale bar 25 µm. (B, C) Mean percentage of human and porcine schizonts with a typical or atypical EXP1(B) or EXP2 (C) staining pattern. Porcine hepatocytes are depicted on the left and human hepatocytes on the right of each bar plot. All or up to seventy-five schizonts were measured per technical replicate of three biological replicates. Results per biological replicate are shown in Supplementary Figure 4. For panels B and C, the total number of schizonts analyzed per condition is shown in Supplementary Table 2. EXP, exported protein; HSP, heat shock protein; PHH, primary human hepatocytes; PPH, primary porcine hepatocytes.
Additionally, fresh porcine hepatocytes were infected with the rodent Pb, showing that UIS4 localized to the PVM and is expressed throughout liver stage development in Pb (Mueller et al., 2005). Unlike porcine hepatocytes infected with Pf, Pb schizonts developing in porcine hepatocytes all showed a typical staining pattern for UIS4 (Figure S3B), suggestive for an intact PVM. Finally, schizonts developing in porcine hepatocytes with atypical EXP1 and EXP2 signal did not show a difference in schizont area (determined by measuring HSP70 signal) compared to schizonts with a typical EXP1 and EXP2 signal (Figure S4), whereas in human hepatocytes schizonts with a typical pattern appeared to be non-significantly larger.
Here, we show that Pf is capable of traversing, invading and partially maturing in non-human porcine hepatocytes. Incomplete maturation of schizonts may be due to an aberrant PVM, supported by the increasingly atypical EXP1 and EXP2 expression pattern observed over time. Additionally, the aberrant EXP1 and EXP2 staining suggest that parasite nutrient uptake may be impaired, which could hamper further maturation of the parasites.
Pf shows a remarkably narrow host range compared to other Plasmodium species and appears to uniquely infect primates in vivo and in vitro (Collins et al., 1994; Rathore et al., 2003). This selectivity is partially explained by different invasion requirements between Plasmodium species, as well as incompatibility of invasion proteins between Pf and the non-human host hepatocyte (i.e. a lock and key model), including CSP and CD81 (Rathore et al., 2003; Silvie et al., 2003; Silvie et al., 2006). As Pf is successful in invading porcine hepatocytes, albeit at a lower rate, it suggests that compatibility of parasite invasion ligands with their host counterparts is not the only requirement needed for parasite survival within a hepatocyte.
A key finding is the abrupt mid-liver stage arrest in parasite development coinciding with the increasingly aberrant EXP1 and EXP2 expression, established markers for PVM quality. The PVM forms a barrier with the host environment and is extensively modified by the parasite to provide the appropriate machinery to scavenge sufficient host nutrients and export proteins, whilst simultaneously offering protection from the hepatocytes’ defense mechanisms (Spielmann et al., 2012). Studies with Pb and Py show that complete cytosolic development of schizonts lacking an intact PVM is possible but generally schizonts only develop up to early-liver stage at twenty-four hours post-invasion (van Dijk et al., 2005; Silvie et al., 2006; Ploemen et al., 2012). Sporozoites lacking SLARP/SAP1 (sporozoite Asparagine-rich protein) arrest early (twenty-four hours post-invasion) and fail to express amongst others effector proteins P52, UIS4 and EXP1 (Aly et al., 2008). These proteins are critical for the formation of a PVM and lead to near-complete developmental arrest during early development (Aly et al., 2008). Sporozoites with P52/p36 deletions are incapable of productively invading hepatocytes resulting in near-complete attenuation during early liver stage in Pb and Py, as well as Pf (Ishino et al., 2005; van Dijk et al., 2005; Labaied et al., 2007; VanBuskirk et al., 2009; Ploemen et al., 2012; Manzoni et al., 2017). Finally, the function of B9 remains more controversial in different Plasmodia species: while required in Pb for productive hepatocyte invasion, this is not the case for Pf invasion into human hepatocytes despite structural similarity (Annoura et al., 2014; Fernandes et al., 2021). As schizonts are detected in porcine hepatocytes on day three and (to a lesser amount) on day five, this challenges this prevailing theory that a functional, intact, PVM is required for progression beyond early-stage Pf infection.
An important limitation is that we do not understand the mechanism behind the atypical PVM in porcine hepatocytes. This abnormal event could be due to several factors or a combination thereof, including refractory porcine hepatocytes for productive invasion, the inability of parasites to maintain the PVM, as well as host factors that may compromise the integrity of the PVM. We find that sporozoites do show characteristics of early transformation into normal liver-stage schizonts, as illustrated by the increase in nuclear material and presence of nuclear division. However, the increasingly aberrant expression of EXP1 and EXP2 supports the failure of schizonts to complete maturation up to day seven. The lack of fluorescently tagged EXP1/2 prevented live-imaging and we therefore cannot exactly pinpoint the timing of the loss of EXP1/2 from the PVM over time. This loss may be due to deteriorating parasite health or active degradation by the host. Alternatively, aberrant EXP1 and EXP2 expression of sporozoites in porcine hepatocytes could affect proper parasite development due impaired nutrient scavenging from the host environment.
Mello-Vieira et al. (2020) show that EXP2 is required for liver invasion and maturation and for establishing blood stage infection in a mouse model, which corroborates with our findings of its importance in maturation (Mello-Vieira et al., 2020). Furthermore, the EXP1 C-terminal region exposed to the host cytoplasm interacts with host Apolipoprotein A (ApoH) which is pivotal for successful liver-stage development in Pb, although it is unclear whether it performs the same role in Pf (Sá et al., 2017; Mesén-Ramírez et al., 2019; Wolanin et al., 2019). EXP1 is continuously trafficking to the PVM throughout the first days of the liver stage (Sá et al., 2017). As the parasite matures, its survival becomes increasingly dependent on its ability to scavenge lipids and nutrients from its host cell, for which it requires a functional PVM (Agop-Nersesian et al., 2018). The combined findings in rodent models may be a potential explanation for the inability of Pf to fully mature in porcine hepatocytes.
An aberrant PVM additionally exposes the developing parasites to the hostile host environment and host cell defense mechanisms. Although host responses to the invading parasites may vary between parasite species, targeting intracellular parasites is a conserved defense strategy of infected hepatocytes (Wacker et al., 2017). During parasite infection, diverse host autophagy pathways can be activated in hepatocytes (reviewed in (Agop-Nersesian et al., 2018)). This includes marking the PVM with the autophagy marker LC3, which activates the host cell autophagy machinery to eliminate intracellular parasites. The observed aberrant PVM may be the result of active host destruction of the PVM: the parasite proteins involved in PVM maintenance in human hepatocytes may be incompatible to those in porcine hepatocytes. This could impact the parasites’ ability to secure host resources and protect itself against host degradation.
To our knowledge, Pf infection of porcine hepatocytes has not been reported before. Our findings are confined to an in vitro system and it is not clear whether a similar mechanism of invasion and maturation occurs in vivo. Additionally, the cause of an impaired PVM remains unknown. The aberrant PVM could make the parasite susceptible to or may be a direct response to intracellular host defence mechanisms. Furthermore, these results underline the importance of EXP1 and EXP2 in Pf liver stage development and maturation, although their exact roles are not explored here. Future studies with PfEXP-1 and EXP-2 knockdown parasites, as well as other PVM proteins, may be able to delineate the key processes involved in parasite maturation.
Altogether, our findings highlight the importance of understanding species-specific intracellular host factors involved in Pf liver-stage development. The partial development of Pf sporozoites in porcine hepatocytes underscores its potential as a platform to study early to mid-stage Pf liver-stage development, and to identify essential Pf and host proteins required for the formation and maintenance of the PVM. Additionally, the model may provide a novel platform to study host factors and nutrients required for full Pf liver-stage development.
The raw data supporting the conclusions of this article will be made available by the authors when possible and upon request
The study was conceptualized and designed by SB, AY, and RS. SB, AY, YW, GJG, and AH performed the experiments. JW coordinated the collection of fresh human liver segments. SB, AY, RS, TB, and MM were involved in conceptualization of the data. SC and AY analyzed the data and made figures for the article. SB and AY wrote the first manuscript. All authors contributed and reviewed the manuscript and approved the submitted version.
This study was funded by the Department of Medical Microbiology, Radboud University Medical Center. AY is funded by the Dutch Research Council (NWO) talent scheme veni (VI.Veni.192.171).
Author RS was employed by company TropIQ Health Sciences.
The remaining authors declare that the research was conducted in the absence of any commercial or financial relationships that could be construed as a potential conflict of interest.
All claims expressed in this article are solely those of the authors and do not necessarily represent those of their affiliated organizations, or those of the publisher, the editors and the reviewers. Any product that may be evaluated in this article, or claim that may be made by its manufacturer, is not guaranteed or endorsed by the publisher.
We are grateful for M. van de Vegte-Bolmer, R. Stoter, R. Heutink, J. Klaasen, A. Pouwelsen, L. Pelser-Posthumus and J. Kuhnen of the Malaria Unit at the Radboud University Medical Center for their contribution in parasite, mosquito and sporozoite production. We would also like to thank the Microscopic Imaging Center (MIC) of the Radboud University for access to their facilities. Additionally, we would like to thank R. Woestenink of the Radboudumc Technology Center of Flow cytometry for his help in single cell analysis. We thank T. Kooij, N. Proellochs, A. van der Starre, and T. Roos for providing critical feedback on the project.
The Supplementary Material for this article can be found online at: https://www.frontiersin.org/articles/10.3389/fcimb.2022.834850/full#supplementary-material
Agop-Nersesian, C., Niklaus, L., Wacker, R., Theo Heussler, V. (2018). Host Cell Cytosolic Immune Response During Plasmodium Liver Stage Development. FEMS Microbiol. Rev. 42, 3. doi: 10.1093/femsre/fuy007
Aly, A. S., Mikolajczak, S. A., Rivera, H. S., Camargo, N., Jacobs-Lorena, V., Labaied, M., et al. (2008). Targeted Deletion of SAP1 Abolishes the Expression of Infectivity Factors Necessary for Successful Malaria Parasite Liver Infection. Mol. Microbiol. 69, 1. doi: 10.1111/j.1365-2958.2008.06271.x
Annoura, T., van Schaijk, B. C., Ploemen, I. H., Sajid, M., Lin, J. W., Vos, M. W., et al. (2014). Two Plasmodium 6-Cys Family-Related Proteins Have Distinct and Critical Roles in Liver-Stage Development. FASEB J. 28, 5. doi: 10.1096/fj.13-241570
Charnaud, S. C., Jonsdottir, T. K., Ploemen, P. R., Sajid, H. E., Lin, B. K., Vos, B., et al. (2018). Spatial Organization of Protein Export in Malaria Parasite Blood Stages. Traffic 19, 8. doi: 10.1111/tra.12577
Collins, W. E., Galland, G. G., Sullivan, J. S., Morris, C. L. (1994). Selection of Different Strains of Plasmodium Falciparum for Testing Blood-Stage Vaccines in Aotus Nancymai Monkeys. Am. J. Trop. Med. Hyg 51, 2. doi: 10.4269/ajtmh.1994.51.224
Coppi, A., Tewari, R., Bishop, J. R., Bennett, B. L., Lawrence, R., Esko, J. D., et al. (2007). Heparan Sulfate Proteoglycans Provide a Signal to Plasmodium Sporozoites to Stop Migrating and Productively Invade Host Cells. Cell. Host. Microbe 2, 5. doi: 10.1016/j.chom.2007.10.002
Ejigiri, I., Ragheb, D. R., Pino, P., Coppi, A., Bennett, B. L., Soldati-Favre, D., et al. (2012). Shedding of TRAP by a Rhomboid Protease From the Malaria Sporozoite Surface is Essential for Gliding Motility and Sporozoite Infectivity. PloS Path. 8, 7. doi: 10.1371/journal.ppat.1002725
Fernandes, P., Loubens, M., Marinach, C., Coppée, R., Grand, M., Andre, T. -P., et al. (2021). Plasmodium Sporozoites Require the Protein B9 to Invade Hepatocytes. bioRxiv. doi: 10.1101/2021.10.25.465731
Garten, M., Nasamu, A. S., Niles, J. C., Zimmerberg, J., Goldberg, D. E., Beck, J. (2018). EXP2 Is a Nutrient-Permeable Channel in the Vacuolar Membrane of Plasmodium and is Essential for Protein Export via PTEX. Nat. Microbiol. 3, 10. doi: 10.1038/s41564-018-0222-7
Ishino, T., Chinzei, Y., Yuda, M. (2005). Two Proteins With 6-Cys Motifs are Required for Malarial Parasites to Commit to Infection of the Hepatocyte. Mol. Microbiol. 58, 5, 1264–1275. doi: 10.1111/j.1365-2958.2005.04801.x
Kalanon, M., Bargieri, D., Sturm, A., Matthews, K., Ghosh, S., Goodman, C. D., et al. (2016). The Plasmodium Translocon of Exported Proteins Component EXP2 is Critical for Establishing a Patent Malaria Infection in Mice. Cell. Microbiol. 18 (3), 399–412. doi: 10.1111/cmi.12520
Kaushansky, A., Douglass, A. N., Arang, N., Vigdorovich, V., Dambrauskas, N., Kain, H. S., et al. (2015). Malaria Parasites Target the Hepatocyte Receptor Epha2 for Successful Host Infection. Science 350 (6264), 1089–1092. doi: 10.1126/science.aad3318
Labaied, M., Harupa, A., Dumpit, R. F., Coppens, I., Mikolajczak, S. A., Kappe, S. H., et al. (2007). Plasmodium Yoelii Sporozoites With Simultaneous Deletion of P52 and P36 are Completely Attenuated and Confer Sterile Immunity Against Infection. Infect. Immun. 75 (8), 3758–3768. doi: 10.1128/iai.00225-07
Liu, W., Sundararaman, S. A., Loy, D. E., Learn, G. H., Li, Y., Plenderleith, L. J., et al. (2016). Multigenomic Delineation of Plasmodium Species of the Laverania Subgenus Infecting Wild-Living Chimpanzees and Gorillas. Genome Biol. Evol. 8 (6), 1929–1939. doi: 10.1093/gbe/evw128
Manzoni, G., Marinach, C., Topçu, S., Briquet, S., Grand, M., Tolle, M., et al. (2017). Plasmodium P36 Determines Host Cell Receptor Usage During Sporozoite Invasion. eLife 6, e25903. doi: 10.7554/eLife.25903
Meier, R. P. H., Navarro-Alvarez, N., Morel, P., Schuurman, H. J., Strom, S., Bühler, L. H., et al. (2015). Current Status of Hepatocyte Xenotransplantation. Int. J. Surg. 23 (Pt B), 273–279. doi: 10.1016/j.ijsu.2015.08.077
Mello-Vieira, J., Enguita, F. J., de Koning-Ward, T. F., Zuzarte-Luís, V., Mota, M. M., et al. (2020). Plasmodium Translocon Component EXP2 Facilitates Hepatocyte Invasion. Nat. Commun. 11, 1. doi: 10.1038/s41467-020-19492-4
Mesén-Ramírez, P., Bergmann, B., Tran, T. T., Garten, M., Stäcker, J., Naranjo-Prado, I., et al. (2019). EXP1 is Critical for Nutrient Uptake Across the Parasitophorous Vacuole Membrane of Malaria Parasites. PloS Biol. 17, 9. doi: 10.1371/journal.pbio.3000473
Mota, M. M., Pradel, G., Vanderberg, J. P., Hafalla, J. C., Frevert, U., Nussenzweig, R. S., et al. (2001). Migration of Plasmodium Sporozoites Through Cells Before Infection. Science 291 (5501), 141–144 . doi: 10.1126/science.291.5501.141
Mueller, A. K., Camargo, N., Kaiser, K., Andorfer, C., Frevert, U., Matuschewski, K., et al. (2005). Plasmodium Liver Stage Developmental Arrest by Depletion of a Protein at the Parasite-Host Interface. Proc. Natl. Acad. Sci. U. S. A. 102, 8. doi: 10.1073/pnas.0408442102
Nessel, T., Beck, J. M., Rayatpisheh, S., Jami-Alahmadi, Y., Wohlschlegel, J. A., Goldberg, D. E., et al. (2020). EXP1 is Required for Organisation of EXP2 in the Intraerythrocytic Malaria Parasite Vacuole. Cell Microbiol. 22, 5. doi: 10.1111/cmi.13168
Nyboer, B., Heiss, K., Mueller, A. K., Ingmundson, A. (2018). The Plasmodium Liver-Stage Parasitophorous Vacuole: A Front-Line of Communication Between Parasite and Host. Int. J. Med. Microbiol. 308, 1. doi: 10.1016/j.ijmm.2017.09.008
Ploemen, I. H., Croes, H. J., van Gemert, G. J., Wijers-Rouw, M., Hermsen, C. C., Sauerwein, R. W., et al. (2012). Plasmodium Berghei Δp52&P36 Parasites Develop Independent of a Parasitophorous Vacuole Membrane in Huh-7 Liver Cells. PloS One 7, 12. doi: 10.1371/journal.pone.0050772
Prudencio, M., Mota, M. M., Mendes, A. M. (2011). A Toolbox to Study Liver Stage Malaria. Trends Parasitol. 27, 12. doi: 10.1016/j.pt.2011.09.004
Rathore, D., Hrstka, S. C., Sacci, J. B., Jr, De la Vega, P., Linhardt, R. J., Kumar, S., et al. (2003). Molecular Mechanism of Host Specificity in Plasmodium Falciparum Infection: Role of Circumsporozoite Protein. J. Biol. Chem. 278, 42. doi: 10.1074/jbc.M306250200
Rodrigues, C. D., Hannus, M., Prudêncio, M., Martin, C., Gonçalves, L. A., Portugal, S., et al. (2008). Host Scavenger Receptor SR-BI Plays a Dual Role in the Establishment of Malaria Parasite Liver Infection. Cell. Host. Microbe 4, 3. doi: 10.1016/j.chom.2008.07.012
Sá, E., Cunha, C., Nyboer, B., Heiss, K., Sanches-Vaz, M., Fontinha, D., et al. (2017). Plasmodium Berghei EXP-1 Interacts With Host Apolipoprotein H During Plasmodium Liver-Stage Development. Proc. Natl. Acad. Sci. U. S. A. 114, 7. doi: 10.1073/pnas.1606419114
Sato, S. (2021). Plasmodium—A Brief Introduction to the Parasites Causing Human Malaria and Their Basic Biology. J. Physiol. Anthropol. 40, 1. doi: 10.1186/s40101-020-00251-9
Silvie, O., Greco, C., Franetich, J. F., Dubart-Kupperschmitt, A., Hannoun, L., van Gemert, G. J., et al. (2003). Hepatocyte CD81 is Required for Plasmodium Falciparum and Plasmodium Yoelii Sporozoite Infectivity. Nat. Med. 9, 1. doi: 10.1038/nm808
Silvie, O., Rubinstein, E., Franetich, J. F., Prenant, M., Belnoue, E., Rénia, L., et al. (2006). Expression of Human CD81 Differently Affects Host Cell Susceptibility to Malaria Sporozoites Depending on the Plasmodium Species. Cell. Microbiol. 8, 7. doi: 10.1111/j.1462-5822.2006.00697.x
Sinnis, P., Coppi, A. (2007). A Long and Winding Road: The Plasmodium Sporozoite’s Journey in the Mammalian Host. Parasitol. Int. 56, 3. doi: 10.1016/j.parint.2007.04.002
Spielmann, T., Montagna, G. N., Hecht, L., Matuschewski, K. (2012). Molecular Make-Up of the Plasmodium Parasitophorous Vacuolar Membrane. Int. J. Med. Microbiol. 302, 4–5. doi: 10.1016/j.ijmm.2012.07.011
Teirlinck, A. C., Roestenberg, M., van de Vegte-Bolmer, M., Scholzen, A., Heinrichs, M. J., Siebelink-Stoter, R., et al. (2013). NF135.C10: A New Plasmodium Falciparum Clone for Controlled Human Malaria Infections. J. Infect. Dis. 207 (4), 656–660. doi: 10.1093/infdis/jis725
VanBuskirk, K. M., O'Neill, M. T., De La Vega, P., Maier, A. G., Krzych, U., Williams, J., et al. (2009). Preerythrocytic, Live-Attenuated Plasmodium Falciparum Vaccine Candidates by Design. Proc. Natl. Acad. Sci. U. S. A. 106, 31. doi: 10.1073/pnas.0906387106
van Dijk, M. R., Douradinha, B., Franke-Fayard, B., Heussler, V., van Dooren, M.W., van Schaijk, B., et al. (2005). Genetically Attenuated, P36p-Deficient Malarial Sporozoites Induce Protective Immunity and Apoptosis of Infected Liver Cells. Proc. Natl. Acad. Sci. U. S. A. 102, 34. doi: 10.1073/pnas.0500925102
Vaughan, A. M., Kappe, S. H. I. (2017). Malaria Parasite Liver Infection and Exoerythrocytic Biology. Cold Spring Harb. Perspect. Med. 7, 6. doi: 10.1101/cshperspect.a025486
Wacker, R., Eickel, N., Schmuckli-Maurer, J., Annoura, T., Niklaus, L., Khan, S. M., et al. (2017). LC3-Association With the Parasitophorous Vacuole Membrane of Plasmodium Berghei Liver Stages Follows a Noncanonical Autophagy Pathway. Cell. Microbiol. 19, 10. doi: 10.1111/cmi.12754
Walk, J., Reuling, I. J., Behet, M. C., Meerstein-Kessel, L., Graumans, W., van Gemert, G. J., et al. (2017). Modest Heterologous Protection After Plasmodium Falciparum Sporozoite Immunization: A Double-Blind Randomized Controlled Clinical Trial. BMC Med. 15 (1), 168. doi: 10.1186/s12916-017-0923-4
Walters, E. M., Prather, R. S. (2013). Advancing Swine Models for Human Health and Diseases. Mo. Med. 110 (3), 212–215.
Wernersson, R., Schierup, M. H., Jørgensen, F. G., Gorodkin, J., Panitz, F., Staerfeldt, H. H., et al. (2005). Pigs in Sequence Space: A 0.66X Coverage Pig Genome Survey Based on Shotgun Sequencing. BMC Genomics 6, 70. doi: 10.1186/1471-2164-6-70
Wolanin, K., Fontinha, D., Sanches-Vaz, M., Nyboer, M., Heiss, B., Mueller, K., et al. (2019). A Crucial Role for the C-Terminal Domain of Exported Protein 1 During the Mosquito and Hepatic Stages of the Plasmodium Berghei Life Cycle. Cell Microbiol. 21 (10), e13088. doi: 10.1111/cmi.13088
Yang, A. S. P., Lopaticki, S., O'Neill, M. T., Erickson, S. M., Douglas, D. N., Kneteman, N. M., et al. (2017). AMA1 and MAEBL are Important for Plasmodium Falciparum Sporozoite Infection of the Liver. Cell. Microbiol. 19, (9). doi: 10.1111/cmi.12745
Keywords: malaria, hepatocyte, Plasmodium falciparum, schizont, porcine (pig) model
Citation: van der Boor SC, van Gemert G-J, Hanssen AEJ, van Waardenburg YM, McCall MBB, Bousema T, de Wilt JHW, Sauerwein RW and Yang ASP (2022) Mid-Liver Stage Arrest of Plasmodium falciparum Schizonts in Primary Porcine Hepatocytes. Front. Cell. Infect. Microbiol. 12:834850. doi: 10.3389/fcimb.2022.834850
Received: 13 December 2021; Accepted: 24 January 2022;
Published: 17 February 2022.
Edited by:
Tania F. De Koning-Ward, Deakin University, AustraliaReviewed by:
Olivier Silvie, U1135 Centre d’Immunologie et de Maladies Infectieuses (INSERM), FranceCopyright © 2022 van der Boor, van Gemert, Hanssen, van Waardenburg, McCall, Bousema, de Wilt, Sauerwein and Yang. This is an open-access article distributed under the terms of the Creative Commons Attribution License (CC BY). The use, distribution or reproduction in other forums is permitted, provided the original author(s) and the copyright owner(s) are credited and that the original publication in this journal is cited, in accordance with accepted academic practice. No use, distribution or reproduction is permitted which does not comply with these terms.
*Correspondence: Saskia C. van der Boor, U2Fza2lhLnZhbmRlcmJvb3JAcmFkYm91ZHVtYy5ubA==; Annie S. P. Yang, QW5uaWUueWFuZ0ByYWRib3VkdW1jLm5s
Disclaimer: All claims expressed in this article are solely those of the authors and do not necessarily represent those of their affiliated organizations, or those of the publisher, the editors and the reviewers. Any product that may be evaluated in this article or claim that may be made by its manufacturer is not guaranteed or endorsed by the publisher.
Research integrity at Frontiers
Learn more about the work of our research integrity team to safeguard the quality of each article we publish.