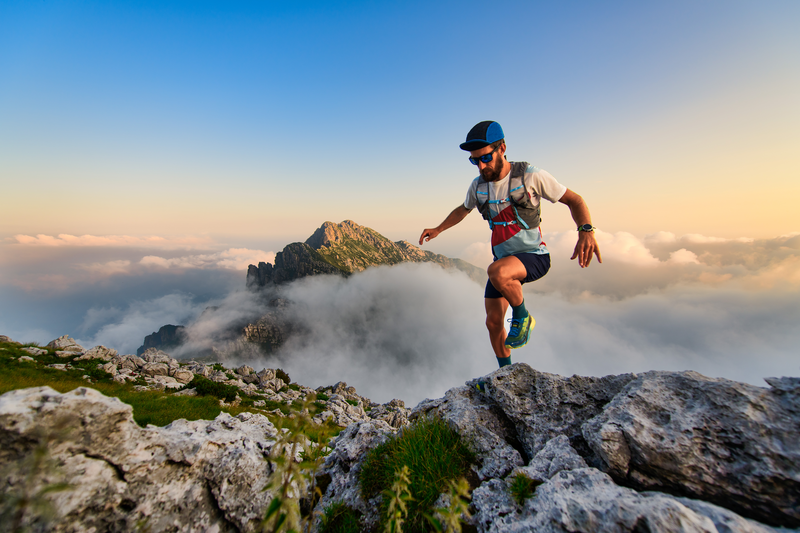
95% of researchers rate our articles as excellent or good
Learn more about the work of our research integrity team to safeguard the quality of each article we publish.
Find out more
ORIGINAL RESEARCH article
Front. Cell. Infect. Microbiol. , 27 May 2022
Sec. Clinical Microbiology
Volume 12 - 2022 | https://doi.org/10.3389/fcimb.2022.807095
This article is part of the Research Topic Antimicrobial Resistance: a One Health Perspective View all 9 articles
Bedaquiline (BDQ), a new antitubercular agent, has been used to treat drug-resistant tuberculosis (TB). Although mutations in atpE, rv0678, and pepQ confer major resistance to BDQ, the mechanisms of resistance to BDQ in vitro and in clinical settings have not been fully elucidated. We selected BDQ-resistant mutants from 7H10 agar plates containing 0.5 mg/L BDQ (the critical concentration) and identified mutations associated with BDQ resistance through whole genome sequencing and Sanger sequencing. A total of 1,025 mutants were resistant to BDQ. We randomly selected 168 mutants for further analysis and discovered that 157/168 BDQ-resistant mutants harbored mutations in rv0678, which encodes a transcriptional regulator that represses the expression of the efflux pump, MmpS5–MmpL5. Moreover, we found two mutations with high frequency in rv0678 at nucleotide positions 286–287 (CG286–287 insertion; accounting for 26.8% [45/168]) and 198–199 (G198, G199 insertion, and G198 deletion; accounting for 14.3% [24/168]). The other mutations were dispersed covering the entire rv0678 gene. Moreover, we found that one new gene, glpK, harbors a G572 insertion; this mutation has a high prevalence (85.7%; 144/168) in the isolated mutants, and the minimum inhibitory concentration (MIC) assay demonstrated that it is closely associated with BDQ resistance. In summary, we characterized 168/1,025 mutants resistant to BDQ and found that mutations in rv0678 confer the primary mechanism of BDQ resistance. Moreover, we identified a new gene (glpK) involved in BDQ resistance. Our study offers new insights and valuable information that will contribute to rapid identification of BDQ-resistant isolates in clinical settings.
Multidrug-resistant tuberculosis (MDR-TB), caused by drug-resistant Mycobacterium tuberculosis (Mtb), results from various factors, including substandard treatment regimens, treatment nonadherence, drug malabsorption, and drug–drug interactions during anti-TB therapy. This hampers the efforts of the World Health Organization (WHO)’s End TB Strategy (Nguyen et al., 2018; World Health Organization, 2019). In 2018, MDR-TB including rifampicin-resistant TB accounted for 3.4% of new TB cases and 18% of previously treated cases (World Health Organization, 2019). Moreover, approximately 6.2% of MDR-TB cases develop extensively drug-resistant TB (World Health Organization, 2019). Therefore, there is an urgent need to discover new and effective anti-TB drugs.
Bedaquiline (BDQ), a new antitubercular agent, has better efficacy against both drug-susceptible and drug-resistant Mtb in vitro (Andries et al., 2005) and improved treatment outcomes and cure rates among patients with drug-resistant TB (Diacon et al., 2014; Schnippel et al., 2018). As a result, WHO recommended the addition of BDQ to treatment regimens for drug-resistant TB (WHO, 2019). However, since the introduction of BDQ to treatment regimens, BDQ resistance has also emerged (Veziris et al., 2017).
BDQ exerts its antitubercular activity by inhibiting the activity of the F1F0-adenosine triphosphate (ATP) synthase encoded by the atpE gene (Andries et al., 2005). Therefore, mutations in atpE (A28V, A28P, G61A, A63P, and I66M) are associated with high levels of BDQ resistance (10–128-fold MIC). BDQ-resistant strains are currently being selected in in vitro studies, but they are rarely found in patients with TB (Nieto Ramirez et al., 2020), thus suggesting that mutations in the atpE gene have a fitness cost within these patients in comparison to in vitro cultures. Moreover, there is another ATP-synthase-independent mechanism of BDQ resistance (Nguyen et al., 2018). The drug efflux system functions as a non-target-based mechanism related to resistance against many antimicrobials, including BDQ (Andries et al., 2014). The rv0678 gene encodes the Mtb transcriptional repressor of the MmpS5–MmpL5 efflux system, and high frequency mutations in the rv0678 gene result in low BDQ resistance (2–8-fold MIC) in vitro and in clinical settings (Hartkoorn et al., 2014; Coeck et al., 2015). Mutations in the rv0678 gene result in damaged function and upregulation of the MmpS5–MmpL5 efflux system. Interestingly, the MmpS5–MmpL5 efflux pump-based mechanism also exists in clofazimine resistance (Hartkoorn et al., 2014).
A previous study showed that pepQ gene mutations also lead to low-level BDQ resistance (up to fourfold) in mice (Almeida et al., 2016; Degiacomi et al., 2020) To our knowledge, mutations in atpE, rv0678, and pepQ gene have shown to be the major mechanisms of Mtb resistant to BDQ. However, Mtb isolates from one patient showed an increased MIC to BDQ, but no mutations were found in atpE, Rv0678, and pepQ and their respective upstream regions (Andres et al., 2020), suggesting the existence of other undetermined mechanisms of BDQ resistance. Consistent with this, Peterson et al. found that BDQ-treated Mtb could initiate a regulatory network regulated by Rv0324 and Rv0880 that coordinates multiple resistance mechanisms to push Mtb into a BDQ-tolerant state (Peterson et al., 2016). Therefore, the identification of new BDQ resistance-related genes will contribute to a comprehensive understanding of BDQ-resistant mechanisms that are critical for reducing the emergence of resistance and anti-TB drug discovery.
This study investigated BDQ-resistance-related mutations through Sanger and whole genome sequencing (WGS).
The Mtb H37Rv strain was cultured in Middlebrook 7H9 broth (7H9; Difco, Detroit) liquid medium containing 0.05% Tween 80 and 10% oleic acid, albumin, dextrose, and catalase (OADC) or plated on Middlebrook 7H10 agar medium (Difco, Detroit) containing 10% OADC and 0.5% glycerol.
BDQ-resistant mutants were screened, as previously shown (Zhang et al., 2015; Pi et al., 2019). In brief, log phase Mtb H37Rv cultures were spread on 7H10 agar plates inoculated with 0.5 mg/L BDQ (Abcam, Cambridge, UK) and incubated at 37°C for 4 weeks. The critical concentration, which is approximately eightfold MIC, was chosen based on the MIC values reported in previous studies: clinical BDQ-resistant isolates have MIC values >0.25 mg/L (Veziris et al., 2017), and low-level BDQ resistant strains (with 2–8-fold MIC) are often seen both in vitro and in clinical settings; therefore, 0.5 mg/L was used for mutant selection. All colonies were picked and transferred onto new Middlebrook 7H10 agar plates containing 0.5 mg/L BDQ to demonstrate their resistance.
Genomic DNA extracted from BDQ-resistant strains and Mtb H37Rv using cetyltrimethylammonium bromide (Benjak et al., 2015) was analyzed by WGS using the Illumina MiSeq platform (Illumina Inc., San Diego, CA, USA) (Zhang et al., 2015). For each mutant, approximately 500 Mb to 1.5 Gb (110- to 350-fold genome coverage) sequences were produced, followed by the removal of the barcodes. Single-nucleotide variants (SNVs), insertions, and deletions harboring 1–58 bp were sorted and called with more than four reads using the Mtb H37Rv genome (NC_000962.3) as a reference. The proline–glutamic acid/proline–proline–glutamic acid family genes with mutations were not included in the analysis. Mutations harbored by MtbH37Rv contrasting to the online genome (NC_000962.3) were also removed and not analyzed.
The BDQ-resistance-related genes were subjected to PCR amplification using the primers listed in Supplementary Table S1 and the genomic DNA of BDQ-resistant isolates selected in vitro as a template. The obtained PCR products were sent for sequencing using the Sanger method to determine the mutations harbored in these genes in the isolated BDQ-resistant mutants.
The indicated mutants were picked from 7H10 agar plates in a tube containing 2 ml 7H9 liquid medium containing 10% OADC. After dispersing with a BACspreader (TB Healthcare, Guangdong, China) for 1 min, the bacteria suspension was prepared. BDQ was double diluted in 7H9 liquid medium containing 10% OADC (20–0.078 mg/L). One hundred microliters of each dilution and 100 μl of the suspension were then mixed in a 96-well plate. MICs were shown as the lowest concentration of BDQ at which no visible growth was observed.
The WGS data were submitted to the Sequence Read Archive of the National Center for Biotechnology Information (PRJNA766993).
In order to select mutants resistant to BDQ, approximately 5×109 bacteria were spread onto 7H10 agar plates containing 0.5 mg/L BDQ as numerated by the colony-forming units count in the absence of antibiotics. A total of 1,025 BDQ-resistant isolates were selected, and 168 mutants were randomly selected from the plates and sent for WGS (101 mutants) and PCR (67 mutants) analysis. The mutation frequency of BDQ-resistant mutants was approximately 2×10−7.
To determine the possible mechanisms of resistance to BDQ, the BDQ-resistant mutants and the parent strain H37Rv were performed WGS and genome sequence comparisons. As shown in Table 1, most of the BDQ-resistant mutants (94/101) had rv0678 gene mutations, including SNVs, deletions, insertions, and deletion and insertion. Insertion and deletion mutations in the rv0678 gene cause a frameshift and may result in the loss of its function. No BDQ resistance mutations were identified in the atpE and pepQ genes in these mutants (Table 1). Given that the WGS results suggested that mutations in rv0678 conferred the primary resistance mechanism against BDQ for the mutants identified, PCR and Sanger sequencing of the rv0678 gene were performed on a different set of 67 mutants (Table 2). Similar results were observed, and 63/67 mutants with rv0678 gene mutations (including SNVs, deletion, and insertion) were identified (Table 2). In accordance with its function, which acts as a repressor of the MmpS5–MmpL5 efflux pump, these results reveal that the rv0678 mutation leads to primary resistance to BDQ.
Among all the mutants subjected to WGS and Sanger sequencing analysis, we found two high frequency mutations in the rv0678 gene. One hotspot was found at nucleotide positions 286–287 (CG286–287 insertion) and accounted for 26.8% (45/168) of the mutants (Tables 1, 2). The second hotspot was found at nucleotide positions 198–199 (G198 deletion and insertion and G199 insertion) and accounted for 14.3% (24/168) of the mutants (Tables 1, 2). The other mutations were widespread throughout the entire rv0678 gene with no apparent clustering (Tables 1, 2).
In addition, we identified a few new, previously undetermined genes that may be related to BDQ resistance. Those newly identified genes include glpK, cobQ2, pitA, gid, rv2426c, rv2820c, rv3510c, rv0071, cya, rv2477c, lppB, rv0953c, rv2823c, rv0405, fhaA, rv2722, trcR, rv3785, and rv1723 (Table 3). Moreover, most rv0678 mutants also harbored mutations in the newly identified genes, regardless of the rv0678 mutation. In particular, we found highly prevalent mutations in glpK in the selected isolates, in addition to rv0678 mutations (Table 3). Furthermore, our results showed that most BDQ-resistant mutants (86/101) with mutations in rv0678 also harbored the G572 insertion mutation in glpK (Table 3). Interestingly, a proportion of BDQ-resistant mutants (6/101) with wild-type (WT) rv0678 had a G572 insertion mutation in glpK (Table 3). PCR and Sanger sequencing analysis performed on the remaining 67 BDQ-resistant mutants showed similar results (58/67 mutant harboring rv0678 mutations and the G572 insertion mutation in glpK; 3/67 harboring WT rv0678 and G572 insertion mutation in glpK; Table 2). Therefore, it is likely that insertion mutations in the glpK gene are associated with BDQ resistance. To determine the relationship between the glpK gene mutation and BDQ resistance, an MIC assay was performed. rv0678 (CG286–287 insertion, and A209, G198, and C348 deletions), glpK (G572 insertion), and rv2820c (A342T) mutants had the same MIC as that of mutants with WT rv0678, glpK (G572 insertion), and rv2820c (A342T) gene mutations (Table 4). Moreover, mutants harboring the WT glpK gene, rv0678 (GC285–286 insertion), and rv2820c (A342T) gene mutations also showed the same MIC as mutants with WT rv0678 gene, glpK (G572 insertion), and rv2820c (A342T) gene mutations, while the MIC of Mtb H37Rv was 0.078 µg/ml (Table 4). Together, these results suggest that insertion mutations in glpK may be responsible for resistance to BDQ.
In the present study, to better characterize the mechanisms of BDQ resistance, we identified 1,025 BDQ-resistant mutants from approximately 5×109 bacteria. The mutation frequency of BDQ-resistant mutants was approximately 2×10-7, which is consistent with the frequency observed in previous studies (Andries et al., 2005; Huitric et al., 2010; Nguyen et al., 2018). Our results indicate that rv0678 mutations may have a low cost in vitro and in clinical settings without causing any loss in fitness (Nguyen et al., 2018), which might account for the high rate of BDQ resistance. We randomly selected and analyzed 168/1,025 BDQ-resistant mutants, and in accordance with previous reports (Villellas et al., 2017), our results suggest that rv0678 mutations are the prominent mechanisms of BDQ resistance. We identified a wide range of mutations within rv0678, leading to BDQ resistance, and this improved our understanding of the mechanisms of resistance to BDQ. In addition, our study provided new perspectives on the mechanisms of resistance to BDQ by confirming that glpK, a previously undetermined gene, is involved in BDQ resistance.
Rv0678, a MarR-like transcriptional repressor, inhibits the expression of the MmpS5–MmpL5 transport system. MmpL5 forms a complex with MmpS5 to facilitate export of cell wall lipid constituents (Tekaia et al., 1999). Recent studies have shown that the MmpS5–MmpL5 transport system also functions as an efflux pump, and its functional impairment is closely associated with drug resistance (Zhang et al., 2015; Villellas et al., 2017). Rv0678 contains six α helices and two β-strands, which form an L-shape (Radhakrishnan et al., 2014). The shorter side, containing α2, α3, α4, β1, and β2, constructs a DNA-binding domain (Radhakrishnan et al., 2014). The longer side forms a dimerization domain consisting of α1, α5, and α6 (Radhakrishnan et al., 2014). Rv0678 has three conserved amino acids (Arg-82, Asp-88, and Arg-90) that localize to the DNA-binding domain and are critical for protein–DNA interactions (Radhakrishnan et al., 2014). Moreover, Arg-30, Glu-104, Arg-107, Glu-113, Tyr-145, and Tyr-157 are responsible for formation of the dimer (Radhakrishnan et al., 2014). In the present study, most rv0678 mutations were found either in the DNA-binding domain or in the dimerization domain. Specifically, R90P, 92 codon shifts, and 157 codon shifts in rv0678, crucial for protein–DNA interactions and dimer formation, were identified in the present study. Moreover, the rv0678 insertion and deletion mutations identified in our study caused a frameshift. Therefore, mutants harboring these mutations in rv0678 may impair the function of either DNA binding or dimerization, leading to loss of function. Our results and those of other groups suggest that mutations in rv0678 confer resistance to BDQ. Consistently, a spectrum of mutations in rv0678 in MDR-TB clinical isolates was identified, although most of them were not involved in BDQ resistance (Villellas et al., 2017). Additionally, the high frequency of mutations in rv0678 was not related to the prior use of BDQ (Villellas et al., 2017). Consistently, our results suggest that rv0678 mutations are frequent in the presence or absence of BDQ, and BDQ-resistant mutants were selected and enriched after BDQ utilization. A proportion of mutations in rv0678 identified in the present study were also found in clinical BDQ-resistant isolates (Villellas et al., 2017; Andres et al., 2020; Degiacomi et al., 2020; Nimmo et al., 2020a; Nimmo et al., 2020b), including G198, CC279–280, and TT279–280 deletions and G198, G199, A275, and G439 insertions and G120T substitution. In particular, 67 codon shifts caused by the G198 deletion and G198 and G199 insertions in rv0678 were the second most common mutations in our BDQ-resistant isolates, indicating that our study contributes to the identification of BDQ-resistant isolates in clinical settings. However, no mutations in atpE gene were found in the isolated mutants. We hypothesize that mutations in atpE result in relatively high-level resistance to BDQ (10–128-fold MIC); however, the BDQ concentration (0.5 µg/ml, eightfold MIC) used in our study is appropriate for the selection of low-level BDQ-resistant mutants. Consistently, mutations in rv0678 with a high frequency have been found in clinical isolates, whereas mutations in atpE have seldom been identified in clinical isolates (World Health Organization, 2019; Andres et al., 2020).
A previous study found that mutations in rv0678 cause cross-resistance to clofazimine both in vitro and in vivo (Hartkoorn et al., 2014; Zhang et al., 2015; Xu et al., 2017). Accordingly, mutants harboring rv0678 G194A, G269C, G304A, T341C, and T365C mutations were also observed in clofazimine-resistant mutants (Zhang et al., 2015), suggesting that these mutants may be cross-resistant to clofazimine, and our results may provide new insights into clofazimine resistance. Whether the mutants identified in the present study were resistant to clofazimine warrants further investigation.
Although mutations in atpE, rv0678, and pepQ confer major resistance to BDQ, the mechanism of resistance to BDQ for a portion of isolates identified in vitro and in clinical settings remains unknown, as they had WT atpE, rv0678, and pepQ (Andres et al., 2020). It is likely that there are other unclarified mechanisms of resistance to BDQ. Here, we identified a new gene, glpK, and an insertion mutation in glpK (G572 insertion) that resulted in a frame shift and loss of function, leading to BDQ resistance. GlpK, encoded by rv3696c, is a critical enzyme for the uptake and metabolism of glycerol and phosphorylates glycerol to glycerol-3-phosphate for the synthesis of glycerophospholipids (Larrouy-Maumus et al., 2013; Whitaker et al., 2020) or for glycolysis and gluconeogenesis (Ehrt and Rhee, 2013). A recent report showed that a frameshift mutation in the 7C homopolymeric tract (HT) sequence (CCCCCCC566-572) of glpK, which is equal to the G572 insertion identified in our study, conferred drug tolerance to isoniazid, rifampicin, moxifloxacin, and ethambutol (Safi et al., 2019; Sun et al., 2019). These frameshift mutations in glpK lower Mtb growth under certain culture conditions (glycerol-containing culture conditions) but enhance survival after exposure to different anti-TB agents (Safi et al., 2019; Sun et al., 2019). Moreover, glpK mutants can revert back to WT glpK by introducing additional insertions and deletions in the same glpK HT region, thus reversing the slow growth (Safi et al., 2019). The reversible process between frameshift mutations and the WT in the 7C HT sequence of glpK conferring reversible drug tolerance during anti-TB therapy facilitates the survival of bacteria in vivo, suggesting that similar circumstances may also exist in BDQ resistance. Here, our results showed that mutants harboring the G572 insertion in glpK were associated with BDQ resistance (Table 4). However, mutants with both G572 insertion in glpK and rv0678 did not increase the MIC to BDQ, suggesting that they have no synergetic or superimposed effects on BDQ resistance (Table 4). According to Safi’s study, we hypothesized that the frameshift mutation in glpK conferring resistance to BDQ caused reduced Mtb growth (Safi et al., 2019). We found that a large proportion of the isolated mutants formed small colonies with slow growth on 7H10 agar plates (data not shown).
Interestingly, invertible frameshift mutations in HTs is caused by slipped-strand mispairing errors during proliferation, and there is a high-proportioned HT variants in bacteria with DNA mismatch repair defect, including Mtb (Streisinger and Owen, 1985; Cole et al., 1998; Mizrahi and Andersen, 1998; Canceill et al., 1999; Parkhill et al., 2000; Bayliss et al., 2008; Bayliss, 2009), indicating that the poly-G:C and poly-A:T tracts within the Mtb genome might have invertible insertion and deletion mutations during proliferation, such as the G572 insertion in the 7C HT sequence identified in the present study. Moreover, mutants with frameshift mutations in glpK in an unstable state have been detected in the sputum of patients with TB (Black et al., 2015; Trauner et al., 2017). Worse still, free glycerol is present in human plasma and Mtb-infected mouse lung tissue, and Safi et al. have demonstrated that the in vivo environment offers positive selection for glpK mutations (Safi et al., 2019). Therefore, it should bring to the forefront that frameshift mutation in glpK caused by mismatch repair deficiency confers BDQ resistance in clinical settings. Together, we revealed a previously undetermined role of glpK in low-level BDQ resistance.
In summary, we characterized 168 mutants resistant to BDQ and found that mutations in rv0678 confer the primary mechanism of BDQ resistance. Importantly, we identified a new gene (glpK) involved in BDQ resistance. Further studies are needed to address the role of glpK by constructing glpK knockout and supplement strains. Our study offers new insights and valuable information that will contribute to rapid identification of BDQ-resistant isolates in clinical settings.
The original contributions presented in the study are included in the article/Supplementary Material. Further inquiries can be directed to the corresponding author.
QG and JB performed the experiments. QG, JB, QL, TY, ZhoW, ZhaW, LL, and GZ analyzed the data. QG, JB, and GZ designed the study and wrote the paper. QL, TY, ZhoW, ZhaW, and LL reviewed the paper and supervised the research. All authors contributed to the article and approved the submitted version.
This work was supported by the National Key Research and Development Plan (No. 2020YFA0907200), the National Natural Science Foundation of China (Nos. 82170009, 81873958, and 81802058), the Guangdong Scientific and Technological Foundation (Nos. 2019B1515120041 and 2020B1111170014), the Shenzhen Scientific and Technological Foundation (No. KCXFZ202002011007083 and JCYJ20180228162336873), and China Postdoctoral Science Foundation (No. 2020M670085ZX).
The authors declare that the research was conducted in the absence of any commercial or financial relationships that could be construed as a potential conflict of interest.
All claims expressed in this article are solely those of the authors and do not necessarily represent those of their affiliated organizations, or those of the publisher, the editors and the reviewers. Any product that may be evaluated in this article, or claim that may be made by its manufacturer, is not guaranteed or endorsed by the publisher.
The Supplementary Material for this article can be found online at: https://www.frontiersin.org/articles/10.3389/fcimb.2022.807095/full#supplementary-material
ATP, adenosine triphosphate; BDQ, Bedaquiline; HT, homopolymeric tract; MDR-TB, multidrug-resistant tuberculosis; MIC, minimum inhibitory concentration; Mtb, Mycobacterium tuberculosis; OADC, oleic acid, albumin, dextrose, and catalase; TB, tuberculosis; WGS, whole genome sequencing; WT, wild type.
Almeida, D., Ioerger, T., Tyagi, S., Li, S. Y., Mdluli, K., Andries, K., et al. (2016). Mutations in pepQ Confer Low-Level Resistance to Bedaquiline and Clofazimine in Mycobacterium Tuberculosis. Antimicrob. Agents Chemother. 60 (8), 4590–4599. doi: 10.1128/aac.00753-16
Andres, S., Merker, M., Heyckendorf, J., Kalsdorf, B., Rumetshofer, R., Indra, A., et al. (2020). Bedaquiline-Resistant Tuberculosis: Dark Clouds on the Horizon. Am. J. Respir. Crit. Care Med. 201 (12), 1564–1568. doi: 10.1164/rccm.201909-1819LE
Andries, K., Verhasselt, P., Guillemont, J., Göhlmann, H. W., Neefs, J. M., Winkler, H., et al. (2005). A Diarylquinoline Drug Active on the ATP Synthase of Mycobacterium Tuberculosis. Science 307 (5707), 223–227. doi: 10.1126/science.1106753
Andries, K., Villellas, C., Coeck, N., Thys, K., Gevers, T., Vranckx, L., et al. (2014). Acquired Resistance of Mycobacterium Tuberculosis to Bedaquiline. PloS One 9 (7), e102135. doi: 10.1371/journal.pone.0102135
Bayliss, C. D. (2009). Determinants of Phase Variation Rate and the Fitness Implications of Differing Rates for Bacterial Pathogens and Commensals. FEMS Microbiol. Rev. 33 (3), 504–520. doi: 10.1111/j.1574-6976.2009.00162.x
Bayliss, C. D., Hoe, J. C., Makepeace, K., Martin, P., Hood, D. W., Moxon, E. R. (2008). Neisseria Meningitidis Escape From the Bactericidal Activity of a Monoclonal Antibody is Mediated by Phase Variation of lgtG and Enhanced by a Mutator Phenotype. Infect. Immun. 76 (11), 5038–5048. doi: 10.1128/iai.00395-08
Benjak, A., Sala, C., Hartkoorn, R. C. (2015). “Whole-Genome Sequencing for Comparative Genomics and De Novo Genome Assembly,” in Mycobacteria Protocols (New York: Springer), 1–16.
Black, P. A., de Vos, M., Louw, G. E., van der Merwe, R. G., Dippenaar, A., Streicher, E. M., et al. (2015). Whole Genome Sequencing Reveals Genomic Heterogeneity and Antibiotic Purification in Mycobacterium Tuberculosis Isolates. BMC Genomics 16, 857. doi: 10.1186/s12864-015-2067-2
Canceill, D., Viguera, E., Ehrlich, S. D. (1999). Replication Slippage of Different DNA Polymerases Is Inversely Related to Their Strand Displacement Efficiency. J. Biol. Chem. 274 (39), 27481–27490. doi: 10.1074/jbc.274.39.27481
Coeck, N., Villelas, C., Meehan, C., Lounis, N., Niemann, S., Rigouts, L., et al. (2015). Unexpected High Frequency of Rv0678 Mutations in MDR-TB Patients Without Documented Prior Use of Clofazimine or Bedaquiline. Int. J. Tuberc. Lung Dis. 19, S45. doi: 10.1093/jac/dkw502
Cole, S. T., Brosch, R., Parkhill, J., Garnier, T., Churcher, C., Harris, D., et al. (1998). Deciphering the Biology of Mycobacterium Tuberculosis From the Complete Genome Sequence. Nature 393 (6685), 537–544. doi: 10.1038/31159
Degiacomi, G., Sammartino, J. C., Sinigiani, V., Marra, P., Urbani, A., Pasca, M. R. (2020). In Vitro Study of Bedaquiline Resistance in Mycobacterium Tuberculosis Multi-Drug Resistant Clinical Isolates. Front. Microbiol. 11, 559469. doi: 10.3389/fmicb.2020.559469
Diacon, A. H., Pym, A., Grobusch, M. P., de los Rios, J. M., Gotuzzo, E., Vasilyeva, I., et al. (2014). Multidrug-Resistant Tuberculosis and Culture Conversion With Bedaquiline. N Engl. J. Med. 371 (8), 723–732. doi: 10.1056/NEJMoa1313865
Ehrt, S., Rhee, K. (2013). Mycobacterium Tuberculosis Metabolism and Host Interaction: Mysteries and Paradoxes. Curr. Top. Microbiol. Immunol. 374, 163–188. doi: 10.1007/82_2012_299
Hartkoorn, R. C., Uplekar, S., Cole, S. T. (2014). Cross-Resistance Between Clofazimine and Bedaquiline Through Upregulation of MmpL5 in Mycobacterium Tuberculosis. Antimicrob. Agents Chemother. 58 (5), 2979–2981. doi: 10.1128/aac.00037-14
Huitric, E., Verhasselt, P., Koul, A., Andries, K., Hoffner, S., Andersson, D. I., et al. (2010). Rates and Mechanisms of Resistance Development in Mycobacterium Tuberculosis to a Novel Diarylquinoline ATP Synthase Inhibitor. Antimicrob. Agents Chemother. 54 (3), 1022–1028. doi: 10.1128/AAC.01611-09
Larrouy-Maumus, G., Biswas, T., Hunt, D. M., Kelly, G., Tsodikov, O. V., de Carvalho, L. P. (2013). Discovery of a Glycerol 3-Phosphate Phosphatase Reveals Glycerophospholipid Polar Head Recycling in Mycobacterium Tuberculosis. Proc. Natl. Acad. Sci. U. S. A. 110 (28), 11320–11325. doi: 10.1073/pnas.1221597110
Mizrahi, V., Andersen, S. J. (1998). DNA Repair in Mycobacterium Tuberculosis. What Have We Learnt From the Genome Sequence? Mol. Microbiol. 29 (6), 1331–1339. doi: 10.1046/j.1365-2958.1998.01038.x
Nguyen, T. V. A., Anthony, R. M., Bañuls, A. L., Nguyen, T. V. A., Vu, D. H., Alffenaar, J. C. (2018). Bedaquiline Resistance: Its Emergence, Mechanism, and Prevention. Clin. Infect. Dis. 66 (10), 1625–1630. doi: 10.1093/cid/cix992
Nieto Ramirez, L. M., Quintero Vargas, K., Diaz, G. (2020). Whole Genome Sequencing for the Analysis of Drug Resistant Strains of Mycobacterium Tuberculosis: A Systematic Review for Bedaquiline and Delamanid. Antibiotics (Basel) 9 (3), 133. doi: 10.3390/antibiotics9030133
Nimmo, C., Millard, J., Brien, K., Moodley, S., van Dorp, L., Lutchminarain, K., et al. (2020a). Bedaquiline Resistance in Drug-Resistant Tuberculosis HIV Co-Infected Patients. Eur. Respir. J. 55 (6), 1902383. doi: 10.1183/13993003.02383-2019
Nimmo, C., Millard, J., van Dorp, L., Brien, K., Moodley, S., Wolf, A., et al. (2020b). Population-Level Emergence of Bedaquiline and Clofazimine Resistance-Associated Variants Among Patients With Drug-Resistant Tuberculosis in Southern Africa: A Phenotypic and Phylogenetic Analysis. Lancet Microbe 1 (4), e165–e174. doi: 10.1016/s2666-5247(20)30031-8
Parkhill, J., Wren, B. W., Mungall, K., Ketley, J. M., Churcher, C., Basham, D., et al. (2000). The Genome Sequence of the Food-Borne Pathogen Campylobacter Jejuni Reveals Hypervariable Sequences. Nature 403 (6770), 665–668. doi: 10.1038/35001088
Peterson, E. J. R., Ma, S., Sherman, D. R., Baliga, N. S. (2016). Network Analysis Identifies Rv0324 and Rv0880 as Regulators of Bedaquiline Tolerance in Mycobacterium Tuberculosis. Nat. Microbiol. 1 (8), 16078. doi: 10.1038/nmicrobiol.2016.78
Pi, R., Liu, Q., Jiang, Q., Gao, Q. (2019). Characterization of Linezolid-Resistance-Associated Mutations in Mycobacterium Tuberculosis Through WGS. J. Antimicrob. Chemother. 74 (7), 1795–1798. doi: 10.1093/jac/dkz150
Radhakrishnan, A., Kumar, N., Wright, C. C., Chou, T. H., Tringides, M. L., Bolla, J. R., et al. (2014). Crystal Structure of the Transcriptional Regulator Rv0678 of Mycobacterium Tuberculosis. J. Biol. Chem. 289 (23), 16526–16540. doi: 10.1074/jbc.M113.538959
Safi, H., Gopal, P., Lingaraju, S., Ma, S., Levine, C., Dartois, V., et al. (2019). Phase Variation in Mycobacterium Tuberculosis glpK Produces Transiently Heritable Drug Tolerance. Proc. Natl. Acad. Sci. U. S. A. 116 (39), 19665–19674. doi: 10.1073/pnas.1907631116
Schnippel, K., Ndjeka, N., Maartens, G., Meintjes, G., Master, I., Ismail, N., et al. (2018). Effect of Bedaquiline on Mortality in South African Patients With Drug-Resistant Tuberculosis: A Retrospective Cohort Study. Lancet Respir. Med. 6 (9), 699–706. doi: 10.1016/s2213-2600(18)30235-2
Streisinger, G., Owen, J. (1985). Mechanisms of Spontaneous and Induced Frameshift Mutation in Bacteriophage T4. Genetics 109 (4), 633–659. doi: 10.1093/genetics/109.4.633
Sun, L., Zhang, L., Wang, T., Jiao, W., Li, Q., Yin, Q., et al. (2019). Mutations of Mycobacterium Tuberculosis Induced by Anti-Tuberculosis Treatment Result in Metabolism Changes and Elevation of Ethambutol Resistance. Infect. Genet. Evol. 72, 151–158. doi: 10.1016/j.meegid.2018.09.027
Tekaia, F., Gordon, S. V., Garnier, T., Brosch, R., Barrell, B. G., Cole, S. T. (1999). Analysis of the Proteome of Mycobacterium Tuberculosis in Silico. Tuber Lung Dis. 79 (6), 329–342. doi: 10.1054/tuld.1999.0220
Trauner, A., Liu, Q., Via, L. E., Liu, X., Ruan, X., Liang, L., et al. (2017). The Within-Host Population Dynamics of Mycobacterium Tuberculosis Vary With Treatment Efficacy. Genome Biol. 18 (1), 71. doi: 10.1186/s13059-017-1196-0
Veziris, N., Bernard, C., Guglielmetti, L., Le Du, D., Marigot-Outtandy, D., Jaspard, M., et al. (2017). Rapid Emergence of Mycobacterium Tuberculosis Bedaquiline Resistance: Lessons to Avoid Repeating Past Errors. Eur. Respir. J. 49 (3), 1601719. doi: 10.1183/13993003.01719-2016
Villellas, C., Coeck, N., Meehan, C. J., Lounis, N., de Jong, B., Rigouts, L., et al. (2017). Unexpected High Prevalence of Resistance-Associated Rv0678 Variants in MDR-TB Patients Without Documented Prior Use of Clofazimine or Bedaquiline. J. Antimicrob. Chemother. 72 (3), 684–690. doi: 10.1093/jac/dkw502
Whitaker, M., Ruecker, N., Hartman, T., Klevorn, T., Andres, J., Kim, J., et al. (2020). Two Interacting ATPases Protect Mycobacterium Tuberculosis From Glycerol and Nitric Oxide Toxicity. J. Bacteriol. 202 (16), e00202–e00220. doi: 10.1128/JB.00202-20
WHO (2019). “WHO Guidelines Approved by the Guidelines Review Committee,” in WHO Consolidated Guidelines on Drug-Resistant Tuberculosis Treatment (Geneva: World Health Organization 2019).
World Health Organization (2019). Global Tuberculosis Report 2019. Geneva: World Health Organization.
Xu, J., Wang, B., Hu, M., Huo, F., Guo, S., Jing, W., et al. (2017). Primary Clofazimine and Bedaquiline Resistance Among Isolates From Patients With Multidrug-Resistant Tuberculosis. Antimicrob. Agents Chemother. 61 (6), e00239–17. doi: 10.1128/aac.00239-17
Keywords: Mycobacterium tuberculosis, bedaquiline, drug resistance, rv0678, glpK
Citation: Guo Q, Bi J, Lin Q, Ye T, Wang Z, Wang Z, Liu L and Zhang G (2022) Whole Genome Sequencing Identifies Novel Mutations Associated With Bedaquiline Resistance in Mycobacterium tuberculosis. Front. Cell. Infect. Microbiol. 12:807095. doi: 10.3389/fcimb.2022.807095
Received: 01 November 2021; Accepted: 26 April 2022;
Published: 27 May 2022.
Edited by:
Rodrigo Cayô, Federal University of São Paulo, BrazilReviewed by:
Jianping Xie, Southwest University, ChinaCopyright © 2022 Guo, Bi, Lin, Ye, Wang, Wang, Liu and Zhang. This is an open-access article distributed under the terms of the Creative Commons Attribution License (CC BY). The use, distribution or reproduction in other forums is permitted, provided the original author(s) and the copyright owner(s) are credited and that the original publication in this journal is cited, in accordance with accepted academic practice. No use, distribution or reproduction is permitted which does not comply with these terms.
*Correspondence: Guoliang Zhang, emhhbmdnbDIwMjBAbWFpbC5zdXN0ZWNoLmVkdS5jbg==
†These authors have contributed equally to this work
Disclaimer: All claims expressed in this article are solely those of the authors and do not necessarily represent those of their affiliated organizations, or those of the publisher, the editors and the reviewers. Any product that may be evaluated in this article or claim that may be made by its manufacturer is not guaranteed or endorsed by the publisher.
Research integrity at Frontiers
Learn more about the work of our research integrity team to safeguard the quality of each article we publish.