- 1Laboratório de Imunidade Natural (LIN), Instituto de Patologia Tropical e Saúde Pública, Universidade Federal de Goiás, Goiânia, Brazil
- 2Programa de Pós-graduação em Saúde Pública, Universidade Santo Amaro, São Paulo, Brazil
- 3Instituto René Rachou, Fundação Oswaldo Cruz, Belo Horizonte, Brazil
Interleukin-32 (IL-32) is produced during Leishmania infection, but the components of the parasite that induce its production are unknown. An important multivirulence factor of Leishmania spp. protozoa is the lipophosphoglycan (LPG), which plays a crucial role in the host-parasite interaction. Here, the ability of LPGs from two dermotropic Leishmania species to induce IL-32 production was evaluated in human peripheral blood mononuclear cells (PBMCs). Additionally, the potential receptors involved in this activation were assessed. PBMCs from healthy individuals were stimulated with LPGs from L. amazonensis (La) or L. braziliensis (Lb), live promastigotes of La or Lb and E. coli lipopolysaccharide (LPS, TLR4 agonist) as control. Blockers of TLR4 (Bartonella quintana LPS or monoclonal antibody) and Ponatinib (RIPK2 inhibitor, NOD2 pathway) were used to evaluate the receptors. ELISA was performed for IL-32 expression and cytokine (IL-1β and IL-6) production in cell lysates and in supernatants, respectively. Expression of TLR4 (2 h, 24 h) was assessed by flow cytometry. IL-32γ mRNA transcript was analyzed by qPCR. It was observed that LPG from Leishmania, like whole parasites, induced the production of IL-32, IL-1β and IL-6. Both LPGs induced the expression of IL32γ mRNA. The production of IL-32 was earlier detected (6 h) and positively associated with the production of IL-1β and IL-6. The induction of cytokines (IL-32, IL-1β and IL-6) was dependent on TLR4 and NOD2. The TLR4 was internalized after interaction with LPG. Therefore, our data suggest that LPGs from La and Lb are components of Leishmania able to upregulate IL-32 and other pro-inflammatory cytokines in a TLR4- and NOD2-dependent manner. In addition, LPG-induced IL-32 seems to be necessary for IL-1β and IL-6 production. To identify the parasite factors and host receptors involved in IL-32 induction is crucial to reveal potential targets for novel strategies to control leishmaniasis.
Introduction
Leishmaniases are a group of neglected diseases, endemic especially in subtropical and tropical regions caused by Leishmania protozoan (Alvar et al., 2012). During the blood meal of insect vectors, Leishmania metacyclic promastigote forms are regurgitated on the host skin leading to the development of an innate immune response. Parasites are phagocytosed by neutrophils and mononuclear phagocytes including monocytes, macrophages, and dendritic cells (DCs) (Peters et al., 2008). Inside macrophages, promastigotes differentiate into amastigotes, proliferate, and infect new cells, triggering an inflammatory process (Gontijo and de Carvalho M de, 2013; Rogers et al., 2009).
In lesions of patients with American tegumentary leishmaniasis (ATL), there is a high expression of the pro-inflammatory cytokine interleukin (IL)-32γ (Galdino et al., 2014; Gomes et al., 2017). This cytokine is induced by L. braziliensis or L. amazonensis in human macrophages. It favors the control of the parasites through the production of nitric oxide (NO) and antimicrobial peptides (dos Santos et al., 2017). Recently, the functional consequences of three genetic variants of IL32 modulating the expression of IL-32γ were reported. After exposure to Leishmania, they affected innate and adaptive cytokine production (Santos et al., 2020). In addition, treatment of monocyte-derived macrophages with recombinant IL-32γ and/or with IL-15 reduced L. braziliensis infection in a vitamin D-dependent manner (Silva LL de et al., 2020). Despite our knowledge about the effects of IL-32 on Leishmania infection, the parasite molecules that induce this cytokine have not been identified so far.
Lipophosphoglycan (LPG) is a major multivirulence factor expressed on the Leishmania promastigote surface. This molecule is essential during host-parasite interaction either in the invertebrate or vertebrate hosts (de Assis et al., 2012). Several functions are described for LPG including: binding to the insect’s midgut (Kamhawi et al., 2004), resistance to digestive enzymes (Borovsky and Schlein, 1987), resistance to complement (Brittingham and Mosser, 1996), modulation of phagosome maturation (da Silva Vieira et al., 2019), triggering of TLR2/TLR4 (de Veer et al., 2003; Ibraim et al., 2013), modulation of NO/cytokines and translocation of NF-kB (Paranaíba et al., 2015), induction of neutrophil extracellular traps (NETs) (Guimarães-Costa et al., 2009), induction of protein kinase R (PKR) (Vivarini et al., 2011), and induction of leukotriene B4 (LTB4) and prostaglandin E2 (PGE2) (Tavares et al., 2014; Lima et al., 2017). Several reports have focused on the immunomodulatory properties of LPGs from dermotropic/viscerotropic New World species of Leishmania including L. amazonensis (Nogueira et al., 2016), L. braziliensis (Vieira et al., 2019), L. enriettii (Paranaíba et al., 2015) and L. infantum (Ibraim et al., 2013; Cardoso et al., 2020). In general, they are mediated by TLR4 and in a lesser extent by TLR2. A distinguished feature of the LPGs from dermotropic Leishmania spp. and/or strains is the most pro-inflammatory activity of them compared to those from viscerotropic strains (Nogueira et al., 2016; Vieira et al., 2019; Cardoso et al., 2020). LPG also activates non-canonical NLRP3 inflammasome increasing IL-1β production by murine macrophages (de Carvalho et al., 2019). However, as most of these LPG effects were demonstrated in mice and IL-32 is not expressed in these animals, it is crucial to evaluate whether and through which receptors Leishmania glycoconjugates can modulate IL-32 production in human cells.
Leishmania braziliensis and L. amazonensis are the causative agents of ATL that lead to a spectrum of clinical outcomes ranging from single cutaneous lesions to severe forms like mucocutaneous or anergic diffuse leishmaniasis (Marques et al., 2017). Interspecies polymorphisms occur in the LPGs from those two species and are determinant for the immunopathological events during innate immune responses (Nogueira et al., 2016; Vieira et al., 2019). The LPG of L. braziliensis is devoid of side chains (Soaresa et al., 2005), whereas that of L. amazonensis possess galactoses and glucoses branching off the Gal(β1,4)Man(α1)-PO4 repeat unit motifs (Nogueira et al., 2017). Those biochemical polymorphisms resulted in differential murine macrophage stimulation through TLR2/TLR4 triggering different patterns of cytokine and NF-kB translocation (Ibraim et al., 2013; Nogueira et al., 2016; Vieira et al., 2019). These studies indicated that LPG interspecies polymorphisms could also affect IL-32 induction in human cells.
In fact, we have shown that induction of IL-32γ by L. amazonensis is dependent on NOD2 and TLR4 while by L. braziliensis is dependent on TLR4 but not on NOD2, in human peripheral blood mononuclear cells (PBMCs) (Santos et al., 2020). These results reinforced the need of evaluation of LPG interspecies polymorphisms in the IL-32γ production. As part of a broader study on functional properties of LPGs from dermotropic L. amazonensis or L. braziliensis, here we investigated their ability to induce IL-32 in human cells. The role of TLR4 and NOD2 as receptors for LPG-mediated effects was also investigated.
Methods
Ethics Statement
This study was approved by the Research Ethics Committee of Hospital das Clínicas/UFG, protocol CAAE: 44033514.0.0000.5078. Blood was collected from blood donors from Instituto Goiano de Oncologia e Hematologia (INGOH); individuals were over 18 years old and of both sexes. Before blood collection, patients read and signed the Informed Consent Form.
Parasite’s Culture
Leishmania (Leishmania) amazonensis (IFLA/BR/67/PH8) and Leishmania (Viannia) braziliensis (MHOM/BR/2003/IMG) were cultured as promastigote forms in Grace’s insect medium (Gibco Life Technologies, USA) supplemented with 20% heat-inactivated fetal bovine serum (FBS, Gibco Life Technologies), 100 U/mL of penicillin/streptomycin (Sigma-Aldrich, USA) at 26°C. Stationary-phase parasites were obtained on the 6th day of growth and washed three times with phosphate-buffered saline (PBS; 1,000 g, 10 min, 10°C).
Extraction and Purification of Lipophosphoglycans
The extraction and purification of LPGs were described in Nogueira et al. (2016). Briefly, late log-phase parasites were harvested and washed twice with PBS prior to LPG extraction with solvent E (H2O/ethanol/diethylether/pyridine/NH4OH; 15:15:5:1:0.017). For purification, the solvent and extract were dried under N2 evaporation, resuspended in 2 mL of 0.1 N acetic acid/0.1 M NaCl, and subjected to hydrophobic chromatography using a column of phenyl-Sepharose. The column was sequentially washed with 6 mL of 0.1N acetic acid/0.1 M NaCl, 1 mL of 0.1 N acetic acid and 1 mL of endotoxin free water. The LPG was eluted with 4 mL of solvent and then dried under N2 evaporation. LPG concentration was determined using the phenol-sulfuric method. All solutions were prepared in sterile LPS-free distilled water (Sanobiol, Campinas, Brazil).
Obtaining Human Peripheral Blood Mononuclear Cells and Treatments
Blood (20 mL) was obtained by venipuncture, collected in vacuum tubes containing EDTA anticoagulant (BD, Brazil). Blood was diluted v/v in PBS-EDTA 0.01 mM pH 7.3, and layer on 1.077 density gradient (Ficoll, GE Healthcare, Switzerland). After centrifugation (1400 g, 30 minutes, 4°C), PBMCs were harvested and cells were washed twice with PBS-EDTA (600 g, 10 minutes, 4°C); subsequently, cells were resuspended in RPMI 1640 medium (Sigma-Aldrich), supplemented with 2 mM L-glutamine (Sigma-Aldrich), 11 mM sodium bicarbonate, 100 U/ml penicillin, 100 μg/ml streptomycin (Sigma-Aldrich) and 10% FBS (Gibco Life Technologies), named as complete RPMI medium. Cell viability was evaluated with 0.1% trypan blue in PBS, and cells were used only when there was ≥ 90% viability. Cells were quantified in a hematocytometer and distributed as 5 x 105 PBMCs/well/200 µL into 96-well U-bottom plates (Corning-Costar, USA).
PBMCs were cultured in the presence of LPG from L. amazonensis (La) or L. braziliensis (Lb) at 10 μg/mL as previously reported (Nogueira et al., 2016; Vieira et al., 2019), live stationary phase promastigotes (Pro) of La or Lb (10 parasites:1 cell) or recombinant human IL-15 (rhIL-15; 100 ng/mL) (Silva LL de et al., 2020), at 37 °C, 5% CO2, for 24 h, in the presence of polymyxin B (10 μg/mL; Sigma-Aldrich; polymyxin B was used to rule out possible effects of contaminating LPS). Before infections or treatments, in some experiments, PBMCs were pretreated or not, for 1 h with LPS from Bartonella quintana (100 ng/mL) (Popa et al., 2007), neutralizing antibody anti-TLR4 (1 µg/mL; clone HTA125, ABCAM, catalog n. ab30667) and RIPK2 inhibitor (Ponatinib 100 nM; Selleckhem, Houston, USA), at 37°C/5% CO2. LPS from Bartonella and Ponatinib, alone or in combination, do not present cellular toxicity, as assessed by the MTT assay (Kumar et al., 2018) and shown in Figure S1.
Measurement of Cytokines
Cells were lysed with PBS containing 0.1% Triton X-100 for intracellular IL-32. The amount of IL-32 was determined by immunoassay (ELISA) kit, following the manufacturer’s instructions (R&D Systems, USA). The detection limit of IL-32 was 31 pg/mL. The concentrations of IL-1β and IL-6 were determined in cell culture supernatants by ELISA kits, following the manufacturer’s instructions; IL-1β (BD Bioscience, DC, USA) and IL-6 (Biolegend, San Diego, CA, USA). The detection limit was 15.62 pg/mL for both cytokines.
Detection of IL-32γ Isoform
RNA was isolated from PBMCs stored in Trizol as previously described (Heinhuis et al., 2016). cDNA was prepared using the iScript kit (Bio-Rad). Primer pairs for the IL32γ isoform were previously described (Galdino et al., 2014) and were produced by Sigma-aldrich. Diluted cDNA was used for qPCR using the Step One Plus qPCR system (Applied Biosystems, FosterCity, CA, USA) with SYBR Green Mastermix (Applied Biosystems). Fold change and relative expression were calculated with the 2^-ddCT method normalized against the house keeping gene GAPDH.
Analysis of TLR4 Internalization by Flow Cytometry
PBMCs (5 x 105/well) were cultured in the presence of LPG (10 μg/mL) of L. amazonensis or L. braziliensis for 2 h or 24 h, at 37°C/5% of CO2. Afterwards, in a first set of experiments, cells were fixed with 2% paraformaldehyde for 15 min and incubated with monoclonal antibodies to TLR4 (anti-human CD284, Alexa Fluor 488, Clone HTA 125, eBioscience, San Diego, CA, USA, catalog n. 53-9917-42) or isotype control (IgG2a K mouse Alexa Fluor 488, Clone eBM2a, eBioscience, catalog n. 53-4724-80), for 15 min, to analyze TLR4 expression on the cell membrane. Cells were washed twice with PBS and fixed in 1% paraformaldehyde. For analysis of total expression (cell membrane and intracellular) of TLR4, cells were permeabilized with 0.3% saponin in PBS, and treated with anti-TLR4 as described above. In a second set of experiments, 2h-treated cells were incubated with anti-TLR4 (AlexaFluor 488; for membrane TLR4) for 20 min, washed and fixed with 1% paraformaldehyde. Then, the cells were permeabilized with 0.3% saponin in PBS and incubated with unconjugated anti-TLR4 (Thermo Scientific, anti-human CD284, Clone 76B357.1, catalog n. MA5-16216), for 20 min, washed and incubated for additional 20 min with anti-mouse IgG (H+L)-Texas Red (Sigma-Aldrich, catalog n. SAB3701026; for intracellular TLR4),. After fixation with 1% paraformaldehyde, acquisition (10,000 events in the monocyte gate, FSC x SSC) was performed using an ACCURI C6 cytometer (BD Bioscience). For analysis, the FSC v.4 program (DNS, Los Angeles, CA, USA) was used. In the first set of experiments, in non-permeabilized cells, we consider TLR4 expressed on cell membrane while in permeabilized cells, we consider total TLR4 expression as it includes cell membrane and intracellular TLR4. Intracellular TLR4 expression was obtained by subtracting total expression from membrane expression of TLR4. Data are represented as percent inhibition (%) relative to the untreated control. In the second set of experiments,…the two fluorochromes were analyzed on the same cells.
Statistical Analysis
Data are presented as individual values, quartiles, maximum and minimum values, and medians. The results were evaluated using the Wilcoxon test to compare two paired samples, and the Anova Kruskal-Wallis test followed by Dunn’s test to compare three or more samples. Results expressed as mean and Standard Error of the Mean (SEM) were analyzed by Two-way Anova followed by Bonferroni’s post hoc test. Spearman’s correlation test was also used. GraphPad Prism 5.0 Software Inc. (San Diego, CA, USA) was used for graphs and analyses. The level of significance established was p < 0.05.
Results
Lipophosphoglycans From L. braziliensis and L. amazonensis Induce IL-32 Production in Human Cells
PBMCs were stimulated with LPG from L. amazonensis or L. braziliensis, live promastigotes or rhIL-15 for 24 h. As expected, rhIL-15 induced IL-32; surprisingly, LPGs from La or Lb induced IL-32 production at similar levels of those induced by rhIL-15 (Figures 1A, B). In addition, the LPGs from La (Figure S2A) or Lb (Figure S2B) induced similar amounts of IL-32 as live promastigote forms did (MOI 10:1). No differences were detected between levels of IL-32 induced by LPGs from both species (Figure S2C).
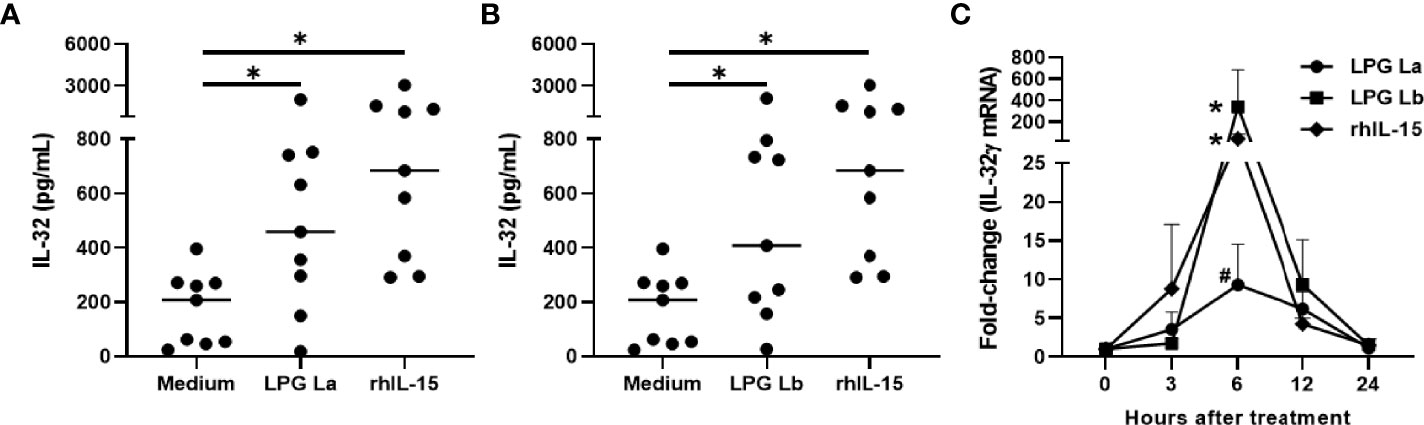
Figure 1 Lipophosphoglycans from Leishmania spp. induces IL-32 production and IL-32γ expression in human mononuclear cells. PBMCs (5 x 105 cells/200 µL) were stimulated with LPG (10 µg/mL) of (A) L. amazonensis (La), (B) L. braziliensis (Lb) or (A, B) rhIL-15 (100 ng/mL). Cell lysates were obtained after 24 h of culture and IL-32 was measured by ELISA. (C) PBMCs were incubated for different periods with LPG (10 µg/mL) from L. amazonensis (La) or from L. braziliensis (Lb) or rhIL-15 (100 ng/mL). The IL-32γ mRNA transcripts were analyzed and quantified by qPCR, using the GAPDH gene as a reference gene. In (A, B), the data represent the median and individual values (n = 9). *p < 0.05, in relation to the control (Anova Kruskal-Wallis followed by Dunn’s post hoc test). In (C) data represent mean and standard error of mean (n = 6). *p < 0.05 (vs. time 0); #p = 0.06 (vs. time 0) (Two-way Anova followed by Bonferroni’s post hoc test).
IL-32γ is the most expressed isoform in Leishmania infection (Galdino et al., 2014; dos Santos et al., 2017), suggesting that LPG could induce IL-32γ as well in PBMCs. In fact, both LPGs or rhIL-15 were able to induce IL32γ mRNA. The time course of IL-32γ mRNA expression was similar for the three stimuli with peaking at 6 h of incubation (Figure 1C); however L. amazonensis LPG showed lower transcript levels at 6 h compared to L. braziliensis LPG and rhIL-15 (Figure 1C). These data suggest that both LPGs can induce the expression and production of IL-32γ in human cells.
Lipophosphoglycans From L. braziliensis and L. amazonensis Induce IL-32, IL-6 and IL-1β Production in a TLR4-Dependent Manner
L. mexicana, L. braziliensis and L. amazonensis LPGs induce the production of pro-inflammatory cytokines in human and murine macrophages via TLR4 (Rojas-Bernabé et al., 2014; Nogueira et al., 2016; Vieira et al., 2019). We therefore investigated whether the induction of IL-32 by LPGs from L. amazonensis and L. braziliensis occurs in a TLR4-dependent manner in human cells. Indeed, blocking of TLR4 with LPS from Bartonella quintana (BartLPS), a natural TLR4 antagonist (Popa et al., 2007), resulted in a reduction of IL-32 production induced by L. amazonensis LPG (Figure 2A) or L. braziliensis LPG (Figure 2B) as well as by LPS from E. coli (Figure 2C). These results were confirmed by blocking of TLR4 with neutralizing antibodies (Figure S3A). Thus, suggesting that the induction of IL-32 by LPGs from La or Lb is dependent on TLR4.
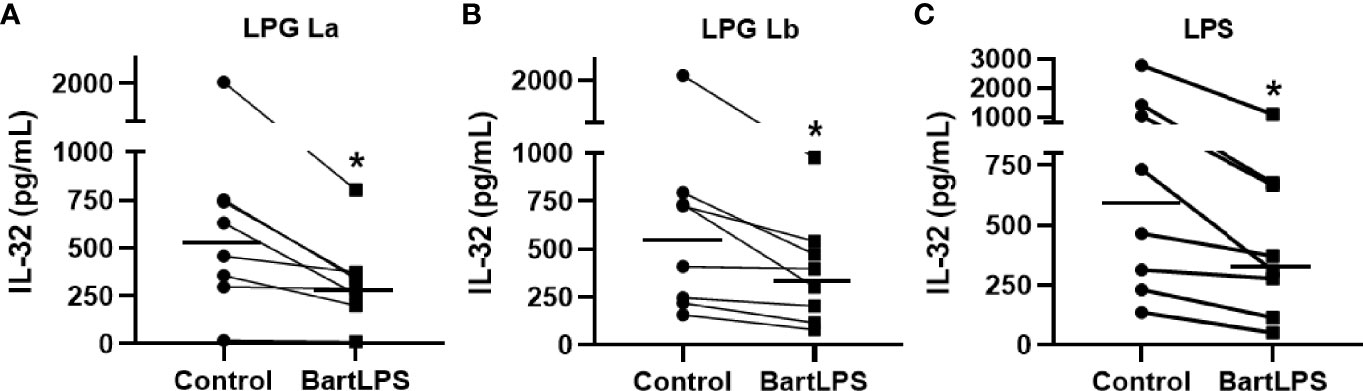
Figure 2 Induction of IL-32 by lipophosphoglycans from Leishmania spp. occurs in a TLR4-dependent manner. Peripheral blood mononuclear cells (5 x 105 cells/200 µL) were treated or not with LPS from Bartonella quintana (BartLPS, 100 ng/ml) for 1 h. After the incubation time, cells were stimulated with LPG (10 µg/ml) of (A) L. amazonensis (La) or from (B) L. braziliensis (Lb) or (C) LPS from E. coli (100 ng/mL), for an additional 24 h. Cell lysates were obtained for IL-32 evaluation by ELISA. IL-32 production was induced by LPG of L. amazonensis (A), L. braziliensis (B) and E. coli LPS (C). Data represent individual measurements and median values (n = 8). *p < 0.05 (vs. control) by Wilcoxon test.
IL-32 induces pro-inflammatory cytokines such as IL-6 and IL-1β in human cells (Netea et al., 2005; Netea et al., 2008). Thus, we decided to investigate whether the LPGs of La and Lb induce the production of proinflammatory cytokines and whether this production is associated with the production of IL-32. We observed that both LPGs induced the production of IL-1β (Figure 3A) and IL-6 (Figure 3B) in a TLR4-dependent manner (Figure S3B, C, and Figure S4). After cell activation with LPGs, positive correlations were observed between the amounts of IL-32 and IL-6 (Figure 3C) or IL-1β (Figure 3D). The strongest associations occurred between L amazonensis LPG-induced IL-32 vs. IL-1β concentrations (r = 0.93) or rhIL-15-induced IL-32 vs. IL-6 (r = 0.97) (Figures 3C, D). Correlations between IL-32 vs. IL-6 concentrations, after stimulation of cells with LPG from the two Leishmania spp. or rhIL-15 were similar (Figure 3C). The data suggested that IL-32 was associated with LPG-induced pro-inflammatory cytokine production. In fact, time course of the production of pro-inflammatory cytokines, IL-6 and IL-1β, showed that these cytokines are produced later after the production of IL-32 by PBMCs stimulated with both LPGs (Figure S5).
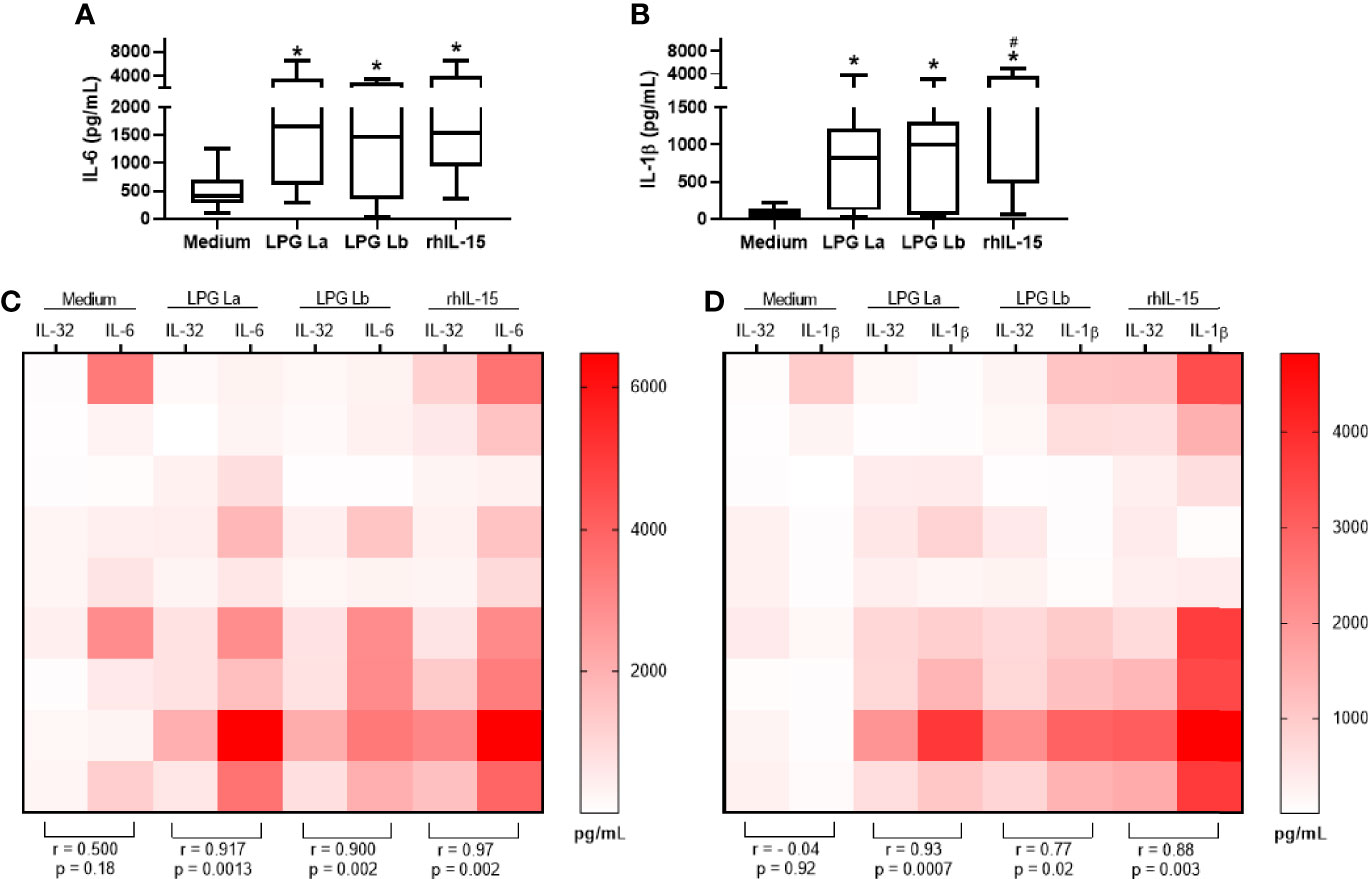
Figure 3 IL-32 production is positively correlated with IL-1β and IL-6 production in human cells stimulated with lipophosphoglycans from Leishmania spp. PBMCs (5 x 105 cells/200 µl) were stimulated with LPG (10 µg/mL) from L. amazonensis (La) or from L. braziliensis (Lb) or with rhIL-15 (100 ng/mL). Supernatants were collected after 24 h of culture for IL-1β (A) and IL-6 (B) cytokine evaluation. In (C, D), heat maps and correlations between IL-32 (measured in cell lysates) in comparison to IL-6 and IL-1β levels, respectively. Data represent medians, quartiles and minimum values and maximum (n = 9 donors). In (A, B), *p < 0.05 (vs. Medium); #p < 0.05 (comparison between LPG of La and Lb) by Anova Kruskal-Wallis followed by Dunn’s post hoc test. In (C, D), r (Spearman’s correlation test) and p values (n = 9 donors) are shown.
Lipophosphoglycans From L. braziliensis and L. amazonensis Induce TLR4 Internalization in Human Cells
We have previously shown that after interaction between L. braziliensis amastigotes and TLR4 there is internalization of this receptor (Galdino et al., 2016). Here, TLR4 expression was analyzed in the monocyte population, separated according to size (FSC-H) and cell complexity (SSC-H) (Figure 4A). In the first set of experiments, we observed that the expression of total TLR4 (membrane + intracellular) was not altered by incubating the cells with both LPGs for 2 h or 24 h (Figure 4B). However, TLR4 expression on monocyte membrane significantly decreased after 2 h of incubation with both LPGs (Figure 4C) in parallel with an increase in intracellular TLR4 (Figure 4D). After 24 h of incubation with LPGs, membrane and intracellular TLR4 expression levels were similar to the initial values (Figures 4B, D). In a second set of experiments, when we evaluated the expression of TLR4 by using antibodies with different fluorochromes, for membrane and intracellular (Figure S6A), we confirmed the internalization of TLR4 after treatment of cells with LPG from both Leishmania spp. (Figure S6B). These data suggest that both LPGs interact and are internalized with TLR4.
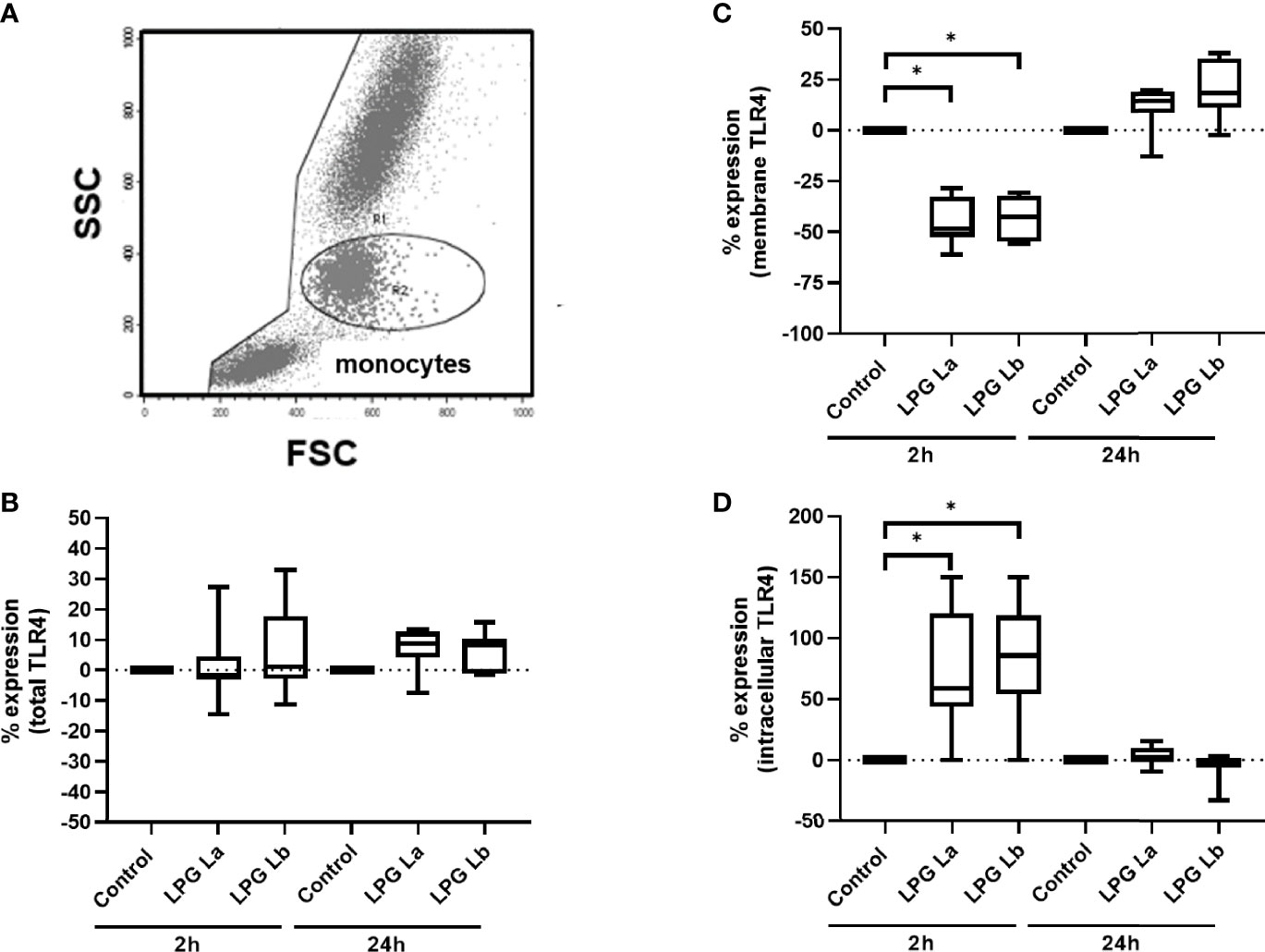
Figure 4 Lipophosphoglycans from Leishmania spp. induces TLR4 internalization. PBMCs (5 x 105 cells/200 μL) were incubated in the presence of LPG (10 μg/mL) from L. amazonensis (La) or L. braziliensis (Lb) for 2 h or 24 h of incubation. The cells were fixed and TLR4 expression was assessed by flow cytometry, defining the monocyte population by FSC-H x SSC-H. Results are shown as percent of TLR4 immunofluorescence intensity (MFI) in LPG-treated cells relative to MFI of control cells incubated in the absence of LPG. (A) Gate for the population of interest. (B) Total TLR4 expression (membrane + intracellular) after 2 h or 24 h (C) TLR4 membrane expression after 2 h or 24 h incubation. (D) Intracellular TLR4 expression (total minus membrane) after 2 h or 24 h of incubation. Data represent medians, interquartiles, and minimum and maximum values (n = 4 donors, evaluated in two independent experiments). *p < 0.05 (vs. control), by Anova Kruskal-Wallis followed by Dunn’s post hoc test.
Lipophosphoglycans From L. braziliensis and L. amazonensis Induce NOD2-Dependent Production of IL-32 and Pro-Inflammatory Cytokines in Human Mononuclear Cells
Dos Santos et al. (2017) (Dos Santos et al., 2017) demonstrated that the NOD2 receptor in human monocytes/macrophages is crucial for immune responses against Leishmania and intracellular control of the parasites. Next, we evaluated whether the NOD2 signaling pathway would also be important for the production of IL-32 and other pro-inflammatory cytokines using Ponatinib. This compound is a potent inhibitor of RIPK2 phosphorylation, an essential kinase for the NOD2 signaling cascade and production of inflammatory cytokines (Dos Santos et al., 2017). Blocking the RIPK2 phosphorylation pathway caused a reduction in IL-32 production induced by La LPG (Figure 5A) and Lb LPG (Figure 5B). Inhibition of NOD2 pathway by Ponatinib also reduced the production of IL-6 (Figure 5C) and IL-1β (Figure 5D) by LPG-stimulated human cells. These data suggest that both LPGs induce the production of IL-32, IL-6 and IL-1β via NOD2 pathway.
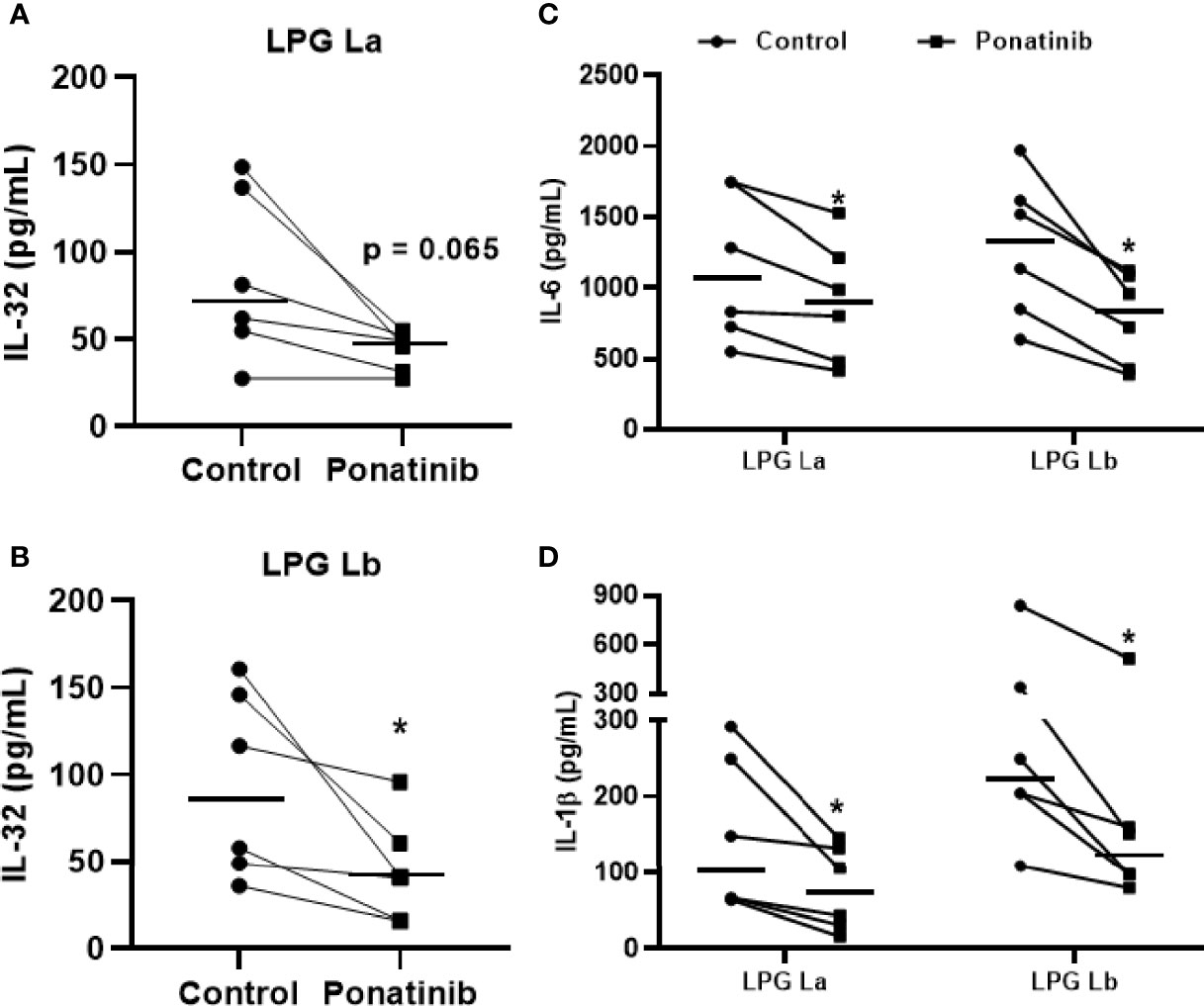
Figure 5 Induction of IL-32 by lipophosphoglycans from Leishmania spp. is dependent on NOD2. PBMCs (5 x 105 cells/200 μL) were treated or not with Ponatinib (100 nM) for 1 h and stimulated with LPG (10 μg/mL) from L. amazonensis (La) or from L. braziliensis (Lb) for 24 h. Cell lysates were obtained for IL-32 evaluation by ELISA, and IL-32 production is shown after exposure to LPG from L. amazonensis (A) or L. braziliensis (B). Supernatants were collected for IL-6 (C) or IL-1β (D) evaluation by ELISA. Data represent individual and median values (n = 6 donors, evaluated in three independent experiments). *p < 0.05 (vs. control) by Wilcoxon test.
Simultaneous antagonism of the TLR4 and inhibition of NOD2 pathway reduced IL-32 production by La LPG (Figure 6A) and Lb LPG (Figure 6B), indicating that both receptors are contributing for LPG effects.
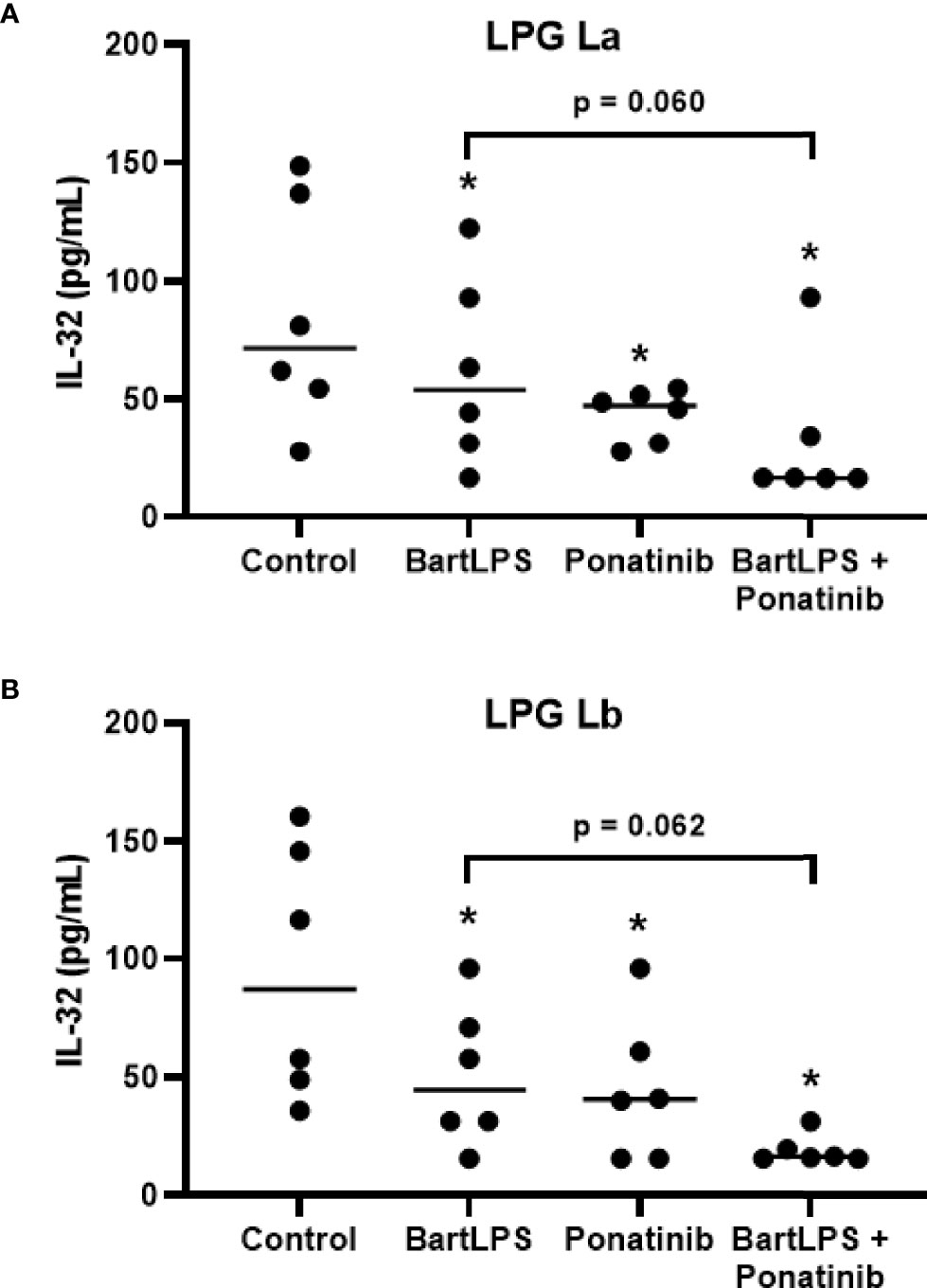
Figure 6 Induction of IL-32 by lipophosphoglycans from Leishmania spp. is dependent on TLR4 and NOD2. PBMCs (5 x 105 cells/200 μL) were treated or not with LPS from Bartonella quintana (100 ng/mL) and/or Ponatinib (100 nM) for 1 h. Then, cells were incubated with LPG (10 μg/mL) from L. amazonensis (La; A) or L. braziliensis (Lb; B) for 24 h. Cell lysates were obtained for the IL-32 evaluation by ELISA. Data represent individual and median values (n = 6 donors, evaluated in three independent experiments). *p < 0.05 (vs. control) by Anova Kruskal-Wallis followed by Dunn’s post hoc test.
Discussion
The results of this study showed that LPGs from L. amazonensis and L. braziliensis induced IL-32 production in human cells, similarly to rhIL-15, a potent IL-32 inducer (Montoya et al., 2014). The amounts of IL-32 produced after stimulation with the LPGs from both species of Leishmania were similar to those induced by live promastigote forms. Whereas the production of IL-32 was not significantly different when comparing the two species, less amount of mRNA IL-32γ was expressed after exposure to L. amazonensis LPG than to L. braziliensis LPG. Dos Santos et al. (2017) showed that promastigote forms of L. amazonensis induced higher expression of mRNA IL32γ and production of IL-32 than metacyclic forms of L. braziliensis in human macrophages differentiated from the monocytic lineage THP-1 (dos Santos et al., 2017). It is noteworthy, therefore, that differences between a differentiated cell line (THP-1) and primary monocytes exist (Chanput et al., 2012) and may explain these differences. Monocytes, the main source of IL-32 in PBMCs (Netea et al., 2006) and THP-1 macrophages can present differential transcriptional and post-transcriptional mechanisms to control the production of IL-32 induced by different Leishmania species. In PBMCs, the data obtained in the present study suggest that the LPG from L. braziliensis induces more efficient transcriptional pathways than L. amazonensis LPG but may be tighter control of translation. Alternatively, the expression/production of other IL-32 isoforms could explain these discrepancies.
The structures of L. amazonensis and L. braziliensis LPGs are polymorphic in composition and displayed different functional properties in murine macrophages (Soaresa et al., 2005; Nogueira et al., 2016; Vieira et al., 2019). Although they are both TLR4 agonists and induced NO, IL-6, and TNF-α, only the LPG of L. braziliensis was able to induce IL-12 and IL-1β (Ibraim et al., 2013; Vieira et al., 2019). Data from the present study suggest that despite their polymorphisms in the sugar motifs, differences in sugar composition of L. amazonensis and L. braziliensis did not significantly influence the amounts of IL-32 induced by both Leishmania species in PBMCs. Consistent with those observations, LPGs from two L. amazonensis strains (PH8 and Josefa) did not differ in their immunomodulatory properties in murine macrophages (Nogueira et al., 2016). However, this appears not to be the case for L. braziliensis LPGs where a larger number of strains from different clinical forms was employed. Thus, for both strains employed in this study, the lipid component may have been determinant for the induction of IL-32, a similar feature to LPS, which uses the lipid A component to stimulate TLR4 pathway and induces IL-32.
TLRs are a well-characterized class of pattern recognition receptors (PRRs) used by phagocytes to recognize PAMPs of infectious agents as Leishmania (Ozinsky et al., 2000). Activation of TLRs by parasite molecules triggers the activation of the transcription factor NF-κB and the MAPK pathway to induce the expression of pro-inflammatory cytokine genes that are essential to control parasite replication (T et al., 2008). TLR4 is reported to be an important receptor for the development of the inflammatory response during leishmaniasis (Mukherjee et al., 2016). In the present study, it was observed that blocking of TLR4 with BartLPS, a natural TLR4 antagonist (Popa et al., 2007), as well as with monoclonal antibodies caused a reduction in LPG-induced IL-32 production by both species of Leishmania. These data suggest that TLR4 recognizes LPG and is at least partially responsible for the IL-32 induction. L. braziliensis LPG was able to differentially modulate macrophage functions through activation of TLR2 and TLR4 (de Assis et al., 2012; Ibraim et al., 2013). Regarding the L. mexicana complex, of which L. amazonensis is part, a study demonstrated the inflammatory role of LPG via the binding of TLR2 and TLR4 receptors (Rojas-Bernabé et al., 2014). Furthermore, infection of human cells with L. braziliensis increased the production of TNFα and IL-10 in a TLR4-dependent manner (Galdino et al., 2016), reinforcing the role of TLR4 as an important receptor in Leishmania infections. The data from the present study strengthen those from Dos Santos et al. (2020) where it was shown that TLR4 is an important receptor for IL-32 induction by lysates of L. amazonensis and L. braziliensis promastigote forms (Santos et al., 2020). Therefore, data from the present study suggest that the LPGs from L. amazonensis and L. braziliensis are PAMPs that induce IL-32 in human mononuclear cells in a TLR4-dependent manner.
As TLR4 activation leads to inflammation and it is known that IL-32 can induce pro-inflammatory cytokines (Netea et al., 2005; Netea et al., 2008; dos Santos et al., 2017), and that inflammatory cytokines induce IL-32 (Kim et al., 2005; Kang et al., 2014), we investigated the interactions between these cytokines after cell activation with LPGs. We observed that LPGs from both Leishmania spp. can induce the production of IL-6 and IL-1β, and a positive correlation was detected between the levels of IL-32 and those of both IL-6 and IL-1β. Moreover, blocking of TLR4 decreased the production of IL-6 and IL-1β after stimulation of PBMCs with LPGs. The time course of cytokine production after LPG treatment suggest that LPG induces IL-32, thereby inducing IL-1β and IL-6 in a TLR4-dependent manner. This suggestion is corroborated by our findings in a functional genomics study, which detected IL32 variants influencing cytokine (IL-1β, TNF-α, IL-6) production upon exposure to lysates of both Leishmania species (Santos et al., 2020). Our data confirm the capacity of LPG to induce pro-inflammatory cytokines in a TLR4-dependent manner (Rojas-Bernabé et al., 2014; Nogueira et al., 2016; Vieira et al., 2019) and, in addition, show that this pathway is responsible for IL-32 induction, which is associated with IL-6 and IL-1β production. In fact, L. mexicana, L. braziliensis and L. amazonensis LPGs induce production of pro-inflammatory cytokines via TLR4, both in human and murine macrophages (Rojas-Bernabé et al., 2014; Nogueira et al., 2016; Vieira et al., 2019). However, it is important to mention that despite their abilities to induce NO and cytokine production in murine macrophages, only the LPG from L. braziliensis (strain M2903) was able to translocate NF-KB by CHO cells via TLR2 (Ibraim et al., 2013). A distinguished feature of the LPGs of L. amazonensis (PH8 and Josefa strains) is that they are very pro-inflammatory but unable to translocate NF-kB in CHO cells via TLR2/TLR4 (Nogueira et al., 2016). This paradoxical effect does not seem to be present in murine macrophages or human PBMCs evaluated here, highlighting possible differences in the cell models employed in different studies.
It is reported that TLR4 can be internalized and participate in endosome trafficking, thereby inducing inflammatory cytokines (Galdino et al., 2016). In the present study, it was observed that after treatment of PBMCs with LPG, the expression of total TLR4 (membrane and intracellular) in monocytes was not altered after 2 h or 24 h. However, the TLR4 expression on cell membrane significantly decreased after 2 h of incubation with LPGs from both Leishmania spp. in parallel to an increase of the intracellular TLR4. After 24 h of incubation with LPG, membrane and intracellular TLR4 expression levels were similar, indicating recycling or TLR4 expression. In lung cells activated with LPS via the TLR4 receptor, it has been shown that the responsiveness to LPS is dependent on the levels of TLR4 present on the cell surface membrane, which is determined by the amount of TLR4 that travels from the Golgi complex to the plasma membrane and the amount of TLR4 internalized (McGettrick and O'Neill, 2010). For LPG, as a TLR4 agonist, the same mechanisms can explain the results here; after exposure to LPG, TLR4 has been endocytosed and after a period of 24 h it was replenished on the cell surface. The regulatory pathways of TLR4-induced signaling by endocytosis and the factors that restrict these processes are just beginning to be elucidated. Zanoni et al. (2011) described a LPS-induced endocytic process that is dependent on the CD14 co-receptor, but independent of TLR4 signaling pathways (Zanoni et al., 2011). In that study, it was shown that the response depends on the cell type exposed to LPS and the co-expression of TLR4 and CD14 (Zanoni et al., 2011). Galdino et al. (2016) demonstrated that after exposure of PBMCs to amastigote forms of L. braziliensis, plasma membrane TLR4 molecules decreased on monocyte surface whereas increased intracellularly, suggesting their endocytosis together with the parasite (Galdino et al., 2016). This internalization was necessary for the production of TNFα and IL-10. As it is known that Leishmania amastigote forms do not present LPG on their surface, data suggested that other parasite molecules can interact with TLR4 in order to induce cytokine production as well (Galdino et al., 2016).
We have shown that NOD2 is receptor relevant for the control of Leishmania spp (Dos Santos et al., 2017). In addition, we reported that LPG was shown to activate NOD-like receptor NLRP3 in a non-canonical pathway (de Carvalho et al., 2019). Thus, in the present study we have inhibited the RIPK2 phosphorylation pathway, a critical kinase for the NOD2 signaling cascade and production of inflammatory cytokines (Park et al., 2007). Inhibition of the RIPK2 phosphorylation pathway caused a reduction in the IL-32 production induced by L. amazonensis LPG or L. braziliensis LPG, which in turn reduced the production of IL-1β and IL-6 in human cells. We have previously demonstrated that the NOD2 receptor in human macrophages is crucial for immune responses and intracellular control of infections caused by the same Leishmania species studied here. In individuals with mutations in the NOD2 gene, the production of cytokines such as TNFα, IL-1β, IL-6, IL-8, IFNγ was significantly decreased in PBMCs after exposure to lysates or promastigote forms of L. amazonensis and L. braziliensis (Dos Santos et al., 2017). Additionally, in a study on visceral leishmaniasis, NOD2-RIPK2 activation contributed to the induction of a potent Th1 but inhibited the Th17 response, due to modulation of cytokine produced by DCs (Nascimento et al., 2016). How LPG accesses the cytoplasm to activate NOD2 remains unclear, but it could be possible that LPG escapes the endosome, after endocytosis with TLR4, and gains the cytosol, thereby activating NOD2. Production of IL-1β has been shown to be dependent on NLRP3 after LPG stimulation (de Carvalho et al., 2019). As the data from the present study suggested a synergism between TLR4 and NOD2 to induce IL-32 after exposure to LPG, it can be speculated that these receptors work together to induce IL-32 and IL-1β during Leishmania spp. infection.
Most of the studies on functional properties of LPGs have been focused on murine macrophages and the studies with human cells are still scarce. A previous study with human neutrophils has shown the ability of L. amazonensis LPG to induce the production of LTB4 (Tavares et al., 2014). The present study also confirms the functional ability of LPGs from L. amazonensis and L. braziliensis as important PAMPs inducers of IL-32, IL-1β, and IL-6 production in human PBMCs. The induction of the cytokines is dependent on TLR4 and NOD2 receptors. In addition, data suggest that LPG-induced IL-32 is at least partially responsible for the IL-6 and IL-1β production induced by the LPGs. A landscape of the results is summarized in Figure 7. To identify the Leishmania PAMPs responsible for IL-32 induction is crucial to control the effects of this cytokine in leishmaniases and to the development of therapeutic and/or vaccine strategies for this important neglected disease.
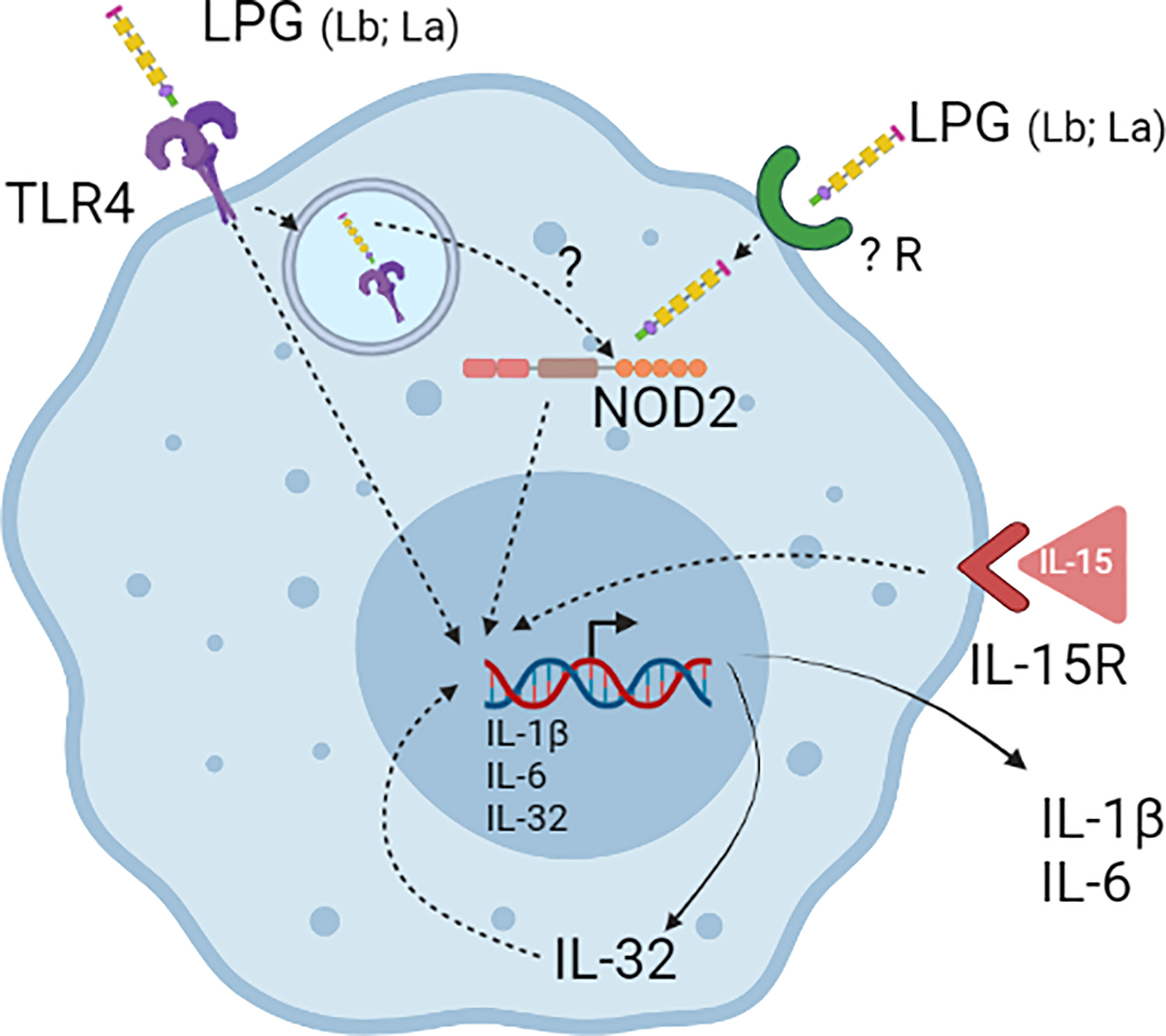
Figure 7 Lipophosphoglycans (LPGs) from L. amazonensis and L. braziliensis are molecules responsible for inducing IL-32 production. LPGs induce IL-32 in a TLR4- and NOD2-dependent manner in human PBMCs. After LPG endocytosis by TLR4 it can escape the endosomes and activate NOD2. IL-32 can increase the production of pro-inflammatory cytokines such as IL-6 and IL-1β induced by LPGs.
Data Availability Statement
The original contributions presented in the study are included in the article/Supplementary Material. Further inquiries can be directed to the corresponding author.
Ethics Statement
This study was approved by the Research Ethics Committee of Hospital das Clínicas/UFG, protocol CAAE: 44033514.0.0000.5078. Blood was collected from blood donors from Instituto Goiano de Oncologia e Hematologia (INGOH); individuals were over 18 years old and of both sexes. Before blood collection, patients read and signed the Informed Consent Form. The patients/participants provided their written informed consent to participate in this study.
Author Contributions
MBS, RG, MTS, JR, LP, RS, and FR-D participated in planning and/or performing the experiments. RG, RS, and FR-D conceived, planned the experiments, analyzed the results, and wrote the manuscript. All authors approved the submitted version.
Funding
This work was supported by CAPES (Finance Code 001) and CNPq/FAPEG (grant n. 465771/2014-9 - INCT/HPI - National Institute of Science and Technology for the strategies in host-pathogen interaction), Brazil. FR-D and RS are researcher fellows of CNPq. RS is supported by CNPq (302972/2019-6), FAPEMIG (PPM-XII-00202-18 ) and FAPESP (2021/01243-0).
Conflict of Interest
The authors declare that the research was conducted in the absence of any commercial or financial relationships that could be construed as a potential conflict of interest.
Publisher’s Note
All claims expressed in this article are solely those of the authors and do not necessarily represent those of their affiliated organizations, or those of the publisher, the editors and the reviewers. Any product that may be evaluated in this article, or claim that may be made by its manufacturer, is not guaranteed or endorsed by the publisher.
Acknowledgments
Thanks to Dr. Leo AB Joosten, Radboud University of Nijmegen for kindly supplying BartLPS and Ponatinib. Thanks to Iara BN Oliveira for the figure design. This work was supported by CAPES (Finance Code 001) and CNPq/FAPEG (grant n. 465771/2014-9 - INCT/HPI - National Institute of Science and Technology for the strategies in host-pathogen interaction), Brazil. FR-D and RS are researcher fellows of CNPq. We thank Dr. Ana Cláudia Torrecilhas, Universidade Federal de São Paulo (UNIFESP), for kindly supplying anti-TLR4 mAb.
Supplementary Material
The Supplementary Material for this article can be found online at: https://www.frontiersin.org/articles/10.3389/fcimb.2022.805720/full#supplementary-material
References
Alvar, J., Vélez, I. D., Bern, C., Herrero, M., Desjeux, P., Cano, J., et al. (2012). Leishmaniasis Worldwide and Global Estimates of its Incidence. PLoS One 7, e35671. doi: 10.1371/journal.pone.0035671
Borovsky, D., Schlein, Y. (1987). Trypsin and Chymotrypsin-Like Enzymes of the Sandfly Phlebotomus Papatasi Infected With Leishmania and Their Possible Role in Vector Competence. Med. Vet. Entomol. 1, 235–242. doi: 10.1111/J.1365-2915.1987.TB00349.X
Brittingham, A., Mosser, D. M. (1996). Exploitation of the Complement System by Leishmania Promastigotes. Parasitol. Today 12, 444–447. doi: 10.1016/0169-4758(96)10067-3
Cardoso, C. A., Araujo, G. V., Sandoval, C. M., Nogueira, P. M., Zúniga, C., Sosa-Ochoa, W. H., et al. (2020). Lipophosphoglycans From Dermotropic Leishmania Infantum are More Pro-Inflammatory Than Those From Viscerotropic Strains. Mem. Inst. Oswaldo Cruz 115, 1–6. doi: 10.1590/0074-02760200140
Chanput, W., Reitsma, M., Kleinjans, L., Mes, J. J., Savelkoul, H. F., Wichers, H. J. (2012). β-Glucans are Involved in Immune-Modulation of THP-1 Macrophages. Mol. Nutr. Food Res. 56, 822–833. doi: 10.1002/MNFR.201100715
da Silva Vieira, T., Arango Duque, G., Ory, K., Gontijo, C. M., Soares, R. P., Descoteaux, A. (2019). Leishmania Braziliensis: Strain-Specific Modulation of Phagosome Maturation. Front. Cell Infect. Microbiol. 9. doi: 10.3389/FCIMB.2019.00319
de Assis, R. R., Ibraim, I. C., Nogueira, P. M., Soares, R. P., Turco, S. J. (2012). Glycoconjugates in New World Species of Leishmania: Polymorphisms in Lipophosphoglycan and Glycoinositolphospholipids and Interaction With Hosts. Biochim. Biophys. Acta 1820, 1354–1365. doi: 10.1016/J.BBAGEN.2011.11.001
de Carvalho, R. V. H., Andrade, W. A., Lima-Junior, D. S., Dilucca, M., de Oliveira, C. V., Wang, K., et al. (2019). Leishmania Lipophosphoglycan Triggers Caspase-11 and the Non-Canonical Activation of the NLRP3 Inflammasome. Cell Rep. 26, 429–437.e5. doi: 10.1016/J.CELREP.2018.12.047
de Veer, M. J., Curtis, J. M., Baldwin, T. M., DiDonato, J. A., Sexton, A., McConville, M. J., et al. (2003). MyD88 is Essential for Clearance of Leishmania Major: Possible Role for Lipophosphoglycan and Toll-Like Receptor 2 Signaling. Eur. J. Immunol. 33, 2822–2831. doi: 10.1002/EJI.200324128
Dos Santos, J. C., Damen, M. S. M. A., Oosting, M., De Jong, D. J., Heinhuis, B., Gomes, R. S., et al. (2017). The NOD2 Receptor is Crucial for Immune Responses Towards New World Leishmania Species. Sci. Rep. 7 (1), 15219. doi: 10.1038/s41598-017-15412-7
dos Santos, J. C., Heinhuis, B., Gomes, R. S., Damen, M. S. M. A., Real, F., Mortara, R. A., et al. (2017). Cytokines and Microbicidal Molecules Regulated by IL-32 in THP-1-Derived Human Macrophages Infected With New World Leishmania Species. PLoS Negl. Trop. Dis. 11. doi: 10.1371/journal.pntd.0005413
Dos Santos, J. C., Leite Quixabeira, V. B., Teodoro Silva, M. V., Damen, M. S. M. A., Schraa, K., Jaeger, M., et al. (2020). Genetic Variation in Interleukin-32 Influence the Immune Response Against New World Leishmania Species and Susceptibility to American Tegumentary Leishmaniasis. PLoS Negl. Trop. Dis. 14 (12), e0008029. doi: 10.1371/journal.pntd.0008029
Galdino, H., Maldaner, A., Pessoni, L., Soriani, F. M., Pereira, L., Pinto, S., et al. (2014). Interleukin 32γ (IL-32γ) Is Highly Expressed in Cutaneous and Mucosal Lesions of American Tegumentary Leishmaniasis Patients: Association With Tumor Necrosis Factor (TNF) and IL-10. BMC Infect. Dis. 14, 249. doi: 10.1186/1471-2334-14-249
Galdino, H., Saar Gomes, R., dos Santos, J. C., Pessoni, L. L., Maldaner, A. E., Marques, S. M., et al. (2016). Leishmania (Viannia) Braziliensis Amastigotes Induces the Expression of Tnfα and IL-10 by Human Peripheral Blood Mononuclear Cells in vitro in a TLR4-Dependent Manner. Cytokine 88, 184–192. doi: 10.1016/j.cyto.2016.09.009
Gomes, R. S., Silva, M. V. T., dos Santos, J. C., de Lima Silva, L. L., Batista, A. C., Machado, J. R., et al. (2017). IL-32γ Promotes the Healing of Murine Cutaneous Lesions Caused by Leishmania Braziliensis Infection in Contrast to Leishmania Amazonensis. Parasit. Vectors 10, 336. doi: 10.1186/s13071-017-2268-4
Gontijo, B., de Carvalho M de, L. R. (2013). American Cutaneous Leishmaniasis. Rev. Soc. Bras. Med. Trop. 36, 71–80. doi: 10.1590/s0037-86822003000100011
Guimarães-Costa, A. B., Nascimento, M. T. C., Froment, G. S., Soares, R. P. P., Morgado, F. N., Conceição-Silva, F., et al. (2009). Leishmania Amazonensis Promastigotes Induce and are Killed by Neutrophil Extracellular Traps. Proc. Natl. Acad. Sci. U. S. A. 106, 6748–6753. doi: 10.1073/pnas.0900226106
Heinhuis, B., Plantinga, T. S., Semango, G., Küsters, B., Netea, M. G., Dinarello, C. A., et al. (2016). Alternatively Spliced Isoforms of IL-32 Differentially Influence Cell Death Pathways in Cancer Cell Lines. Carcinogenesis 37, 197–205. doi: 10.1093/CARCIN/BGV172
Ibraim, I. C., de Assis, R. R., Pessoa, N. L., Campos, M. A., Melo, M. N., Turco, S. J., et al. (2013). Two Biochemically Distinct Lipophosphoglycans From Leishmania Braziliensis and Leishmania Infantum Trigger Different Innate Immune Responses in Murine Macrophages. Parasit. Vectors 6, 54. doi: 10.1186/1756-3305-6-54
Kamhawi, S., Ramalho-Ortigao, M., Pham, V. M., Kumar, S., Lawyer, P. G., Turco, S. J., et al. (2004). A Role for Insect Galectins in Parasite Survival. Cell 119, 329–341. doi: 10.1016/J.CELL.2004.10.009
Kang, J.-W., Park, Y. S., Lee, D. H., Kim, M. S., Bak, Y., Ham, S. Y., et al. (2014). Interaction Network Mapping Among IL-32 Isoforms. Biochimie 101, 248–251. doi: 10.1016/j.biochi.2014.01.013
Kim, S. H., Han, S. Y., Azam, T., Yoon, D. Y., Dinarello, C. A. (2005). Interleukin-32: A Cytokine and Inducer of Tnfα. Immunity 22, 131–142. doi: 10.1016/j.immuni.2004.12.003
Kumar, P., Nagarajan, A., Uchil, P. D. (2018). Analysis of Cell Viability by the MTT Assay. Cold Spring Harb. Protoc. 2018, 469–471. doi: 10.1101/PDB.PROT095505
Lima, J. B., Araújo-Santos, T., Lázaro-Souza, M., Carneiro, A. B., Ibraim, I. C., Jesus-Santos, F. H., et al. (2017). Leishmania Infantum Lipophosphoglycan Induced-Prostaglandin E 2 Production in Association With PPAR-γ Expression via Activation of Toll Like Receptors-1 and 2. Sci. Rep. 7 (1), 14321. doi: 10.1038/S41598-017-14229-8
Marques, F. A., Soares, R. P., Almeida, G. G., Souza, C. C., Melo, M. N., Pinto, S. A., et al. (2017). Effectiveness of an Immunohistochemical Protocol for Leishmania Detection in Different Clinical Forms of American Tegumentary Leishmaniasis. Parasitol. Int. 66, 884–888. doi: 10.1016/J.PARINT.2016.10.003
McGettrick, A. F., O'Neill, L. (2010). Localisation and Trafficking of Toll-Like Receptors: An Important Mode of Regulation. Curr. Opin. Immunol. 22, 20–27. doi: 10.1016/J.COI.2009.12.002
Montoya, D., Inkeles, M. S., Liu, P. T., Realegeno, S., Teles, R. M. B., Vaidya, P., et al. (2014). IL-32 is a Molecular Marker of a Host Defense Network in Human Tuberculosis. Sci. Transl. Med. 6, 250ra114. doi: 10.1126/scitranslmed.3009546
Mukherjee, S., Karmakar, S., Babu, S. P. (2016). TLR2 and TLR4 Mediated Host Immune Responses in Major Infectious Diseases: A Review. Braz. J. Infect. Dis. 20, 193–204. doi: 10.1016/J.BJID.2015.10.011
Nascimento, M. S., Ferreira, M. D., Quirino, G. F., Maruyama, S. R., Krishnaswamy, J. K., Liu, D., et al. NOD2-RIP2-Mediated Signaling Helps Shape Adaptive Immunity in Visceral Leishmaniasis. J. Infect. Dis. 214 (11), 1647–1657. doi: 10.1093/infdis/jiw446
Netea, M. G., Azam, T., Ferwerda, G., Girardin, S. E., Walsh, M., Park, J. S., et al. (2005). IL-32 Synergizes With Nucleotide Oligomerization Domain (NOD) 1 and NOD2 Ligands for IL-1β and IL-6 Production Through a Caspase 1-Dependent Mechanism. Proc. Natl. Acad. Sci. U. S. A. 102, 16309–16314. doi: 10.1073/pnas.0508237102
Netea, M. G., Azam, T., Lewis, E. C., Joosten, L. A. B., Wang, M., Langenberg, D., et al. (2006). Mycobacterium Tuberculosis Induces Interleukin-32 Production Through a Caspase- 1/IL-18/Interferon-γ-Dependent Mechanism. PLoS Med. 3, e277. doi: 10.1371/journal.pmed.0030277
Netea, M. G., Lewis, E. C., Azam, T., Joosten, L. A. B., Jaekal, J., Bae, S.-Y., et al. (2008). Interleukin-32 Induces the Differentiation of Monocytes Into Macrophage-Like Cells. Proc. Natl. Acad. Sci. 105, 3515–3520. doi: 10.1073/pnas.0712381105
Nogueira, P. M., Assis, R. R., Torrecilhas, A. C., Saraiva, E. M., Pessoa, N. L., Campos, M. A., et al. (2016). Lipophosphoglycans From Leishmania Amazonensis Strains Display Immunomodulatory Properties via TLR4 and Do Not Affect Sand Fly Infection. PLoS Negl. Trop. Dis. 10 (8), e0004848. doi: 10.1371/JOURNAL.PNTD.0004848
Nogueira, P. M., Guimarães, A. C., Assis, R. R., Sadlova, J., Myskova, J., Pruzinova, K., et al. (2017). Lipophosphoglycan Polymorphisms do Not Affect Leishmania Amazonensis Development in the Permissive Vectors Lutzomyia Migonei and Lutzomyia Longipalpis. Parasit. Vectors 10 (1), 608. doi: 10.1186/S13071-017-2568-8
Ozinsky, A., Underhill, D. M., Fontenot, J. D., Hajjar, A. M., Smith, K. D., Wilson, C. B., et al. (2000). The Repertoire for Pattern Recognition of Pathogens by the Innate Immune System is Defined by Cooperation Between Toll-Like Receptors. Proc. Natl. Acad. Sci. U. S. A. 97, 13766–13771. doi: 10.1073/PNAS.250476497
Paranaíba, L. F., de Assis, R. R., Nogueira, P. M., Torrecilhas, A. C., Campos, J. H., Silveira, A. C., et al. (2015). Leishmania Enriettii: Biochemical Characterisation of Lipophosphoglycans (LPGs) and Glycoinositolphospholipids (GIPLs) and Infectivity to Cavia Porcellus. Parasit. Vectors 8, 31. doi: 10.1186/S13071-015-0633-8
Park, J. H., Kim, Y. G., Shaw, M., Kanneganti, T. D., Fujimoto, Y., Fukase, K., et al. (2007). Nod1/RICK and TLR Signaling Regulate Chemokine and Antimicrobial Innate Immune Responses in Mesothelial Cells. J. Immunol. 179, 514–521. doi: 10.4049/JIMMUNOL.179.1.514
Peters, N. C., Egen, J. G., Secundino, N., Debrabant, A., Kimblin, N., Kamhawi, S., et al. (2008). In Vivo Imaging Reveals an Essential Role for Neutrophils in Leishmaniasis Transmitted by Sand Flies. Science (80-) 321, 970–974. doi: 10.1126/science.1159194
Popa, C., Abdollahi-Roodsaz, S., Joosten, L. A.B., Takahashi, N., Sprong, T., Matera, G., et al. (2007). Bartonella Quintana Lipopolysaccharide is a Natural Antagonist of Toll-Like Receptor 4. Infect. Immun. 75, 4831–4837. doi: 10.1128/IAI.00237-07
Rogers, M., Kropf, P., Choi, B.-S., Dillon, R., Podinovskaia, M., Bates, P., et al. (2009). Proteophosophoglycans Regurgitated by Leishmania-Infected Sand Flies Target the L-Arginine Metabolism of Host Macrophages to Promote Parasite Survival. PLoS Pathog. 5 (8), e1000555. doi: 10.1371/JOURNAL.PPAT.1000555
Rojas-Bernabé, A., Garcia-Hernández, O., Maldonado-Bernal, C., Delegado-Dominguez, J., Ortega, E., Gutiérrez-Kobeh, L., et al. (2014). Leishmania Mexicana Lipophosphoglycan Activates ERK and P38 MAP Kinase and Induces Production of Proinflammatory Cytokines in Human Macrophages Through TLR2 and TLR4. Parasitology 141, 788–800. doi: 10.1017/S0031182013002187
Silva LL de, L., Gomes, R. S., Silva, M. V. T., Joosten, L. A. B., Ribeiro-Dias, F. (2020). IL-15 Enhances the Capacity of Primary Human Macrophages to Control Leishmania Braziliensis Infection by IL-32/Vitamin D Dependent and Independent Pathways. Parasitol. Int. 76, 102097. doi: 10.1016/j.parint.2020.102097
Soares, R. P., Cardoso, T. L., Barrona, T., Araújob, M. S., Pimentab, P. F., Turco, S. J. (2005). Leishmania Braziliensis: A Novel Mechanism in the Lipophosphoglycan Regulation During Metacyclogenesis. Int. J. Parasitol. 35, 245–253. doi: 10.1016/J.IJPARA.2004.12.008
Tuon, F. F., Amato, V. S., Bacha, H. A., Almusawi, T., Duarte, M. I., Amato Neto, V. (2008). Toll-Like Receptors and Leishmaniasis. Infect. Immun. 76, 866–872. doi: 10.1128/IAI.01090-07
Tavares, N. M., Araújo-Santos, T., Afonso, L., Nogueira, P. M., Lopes, U. G., Soares, R. P., et al. (2014). Understanding the Mechanisms Controlling Leishmania Amazonensis Infection In Vitro: The Role of LTB4 Derived From Human Neutrophils. J. Infect. Dis. 210, 656–666. doi: 10.1093/INFDIS/JIU158
Vieira, T. da S., Rugani, J. N., Nogueira, P. M., Torrecilhas, A. C., Gontijo, C. M. F., Descoteaux, A., et al. (2019). Intraspecies Polymorphisms in the Lipophosphoglycan of L. Braziliensis Differentially Modulate Macrophage Activation via TLR4. Front. Cell Infect. Microbiol. 9. doi: 10.3389/FCIMB.2019.00240
Vivarini, Á. de C., Meirelles Santos Pereira, R. de, Luiza Dias Teixeira, K., Calegari-Silva, T. C., Bellio, M., Laurenti, M. D., et al. (2011). Human Cutaneous Leishmaniasis: Interferon-Dependent Expression of Double-Stranded RNA-Dependent Protein Kinase (PKR) via TLR2. FASEB J. 25, 4162–4173. doi: 10.1096/FJ.11-185165
Keywords: IL-32, lipophosphoglycan, Leishmania braziliensis, Leishmania amazonensis, TLR4, NOD2
Citation: Silveira MB, Gomes RS, Shio MT, Rugani JN, Paranaiba LF, Soares RP and Ribeiro-Dias F (2022) Lipophosphoglycan From Dermotropic New World Leishmania Upregulates Interleukin-32 and Proinflammatory Cytokines Through TLR4 and NOD2 Receptors. Front. Cell. Infect. Microbiol. 12:805720. doi: 10.3389/fcimb.2022.805720
Received: 30 October 2021; Accepted: 28 February 2022;
Published: 23 March 2022.
Edited by:
Ricardo Goncalves, Universidade Federal de Minas Gerais, BrazilReviewed by:
Surya Prakash Pandey, University of Pittsburgh, United StatesMaritza Jaramillo, Université du Québec, Canada
Copyright © 2022 Silveira, Gomes, Shio, Rugani, Paranaiba, Soares and Ribeiro-Dias. This is an open-access article distributed under the terms of the Creative Commons Attribution License (CC BY). The use, distribution or reproduction in other forums is permitted, provided the original author(s) and the copyright owner(s) are credited and that the original publication in this journal is cited, in accordance with accepted academic practice. No use, distribution or reproduction is permitted which does not comply with these terms.
*Correspondence: Fátima Ribeiro-Dias, fatimardias@gmail.com; fdias@ufg.br