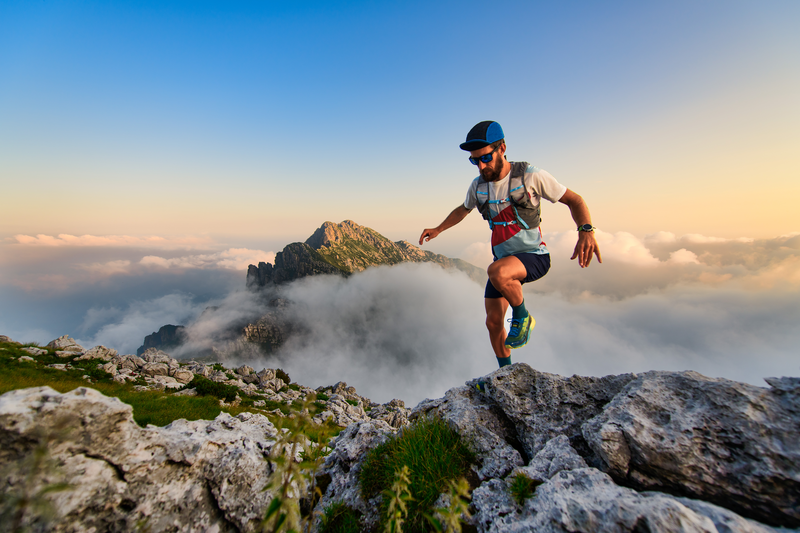
95% of researchers rate our articles as excellent or good
Learn more about the work of our research integrity team to safeguard the quality of each article we publish.
Find out more
ORIGINAL RESEARCH article
Front. Cell. Infect. Microbiol. , 23 December 2022
Sec. Molecular Viral Pathogenesis
Volume 12 - 2022 | https://doi.org/10.3389/fcimb.2022.1071880
This article is part of the Research Topic The interaction between viruses and the host immune system in persistent infection from a metabolic perspective. View all 5 articles
Background: Although γδ T cells play an essential role in immunity against Human Immunodeficiency Virus (HIV) or Mycobacterium tuberculosis (MTB), they are poorly described in HIV infection with tuberculosis (TB).
Methods: The phenotypic and functional properties of peripheral blood γδ T cells in patients with HIV/TB co-infection were analyzed compared to healthy controls and patients with HIV mono-infection or TB by direct intracellular cytokine staining (ICS).
Results: The percentage of Vδ1 subset in HIV/TB group was significantly higher than that in TB group, while the decreased frequency of the Vδ2 and Vγ2Vδ2 subsets were observed in HIV/TB group than in TB group. The percentage of CD4+CD8- Vδ2 subset in HIV/TB group was markedly lower than in TB group. However, the percentage of CD4+CD8+ Vδ2 subset in HIV/TB group was markedly higher than HIV group or TB group. A lower percentage TNF-α and a higher percentage of IL-17A of Vδ2 subset were observed in HIV/TB group than that in HIV mono-infection. The percentage of perforin-producing Vδ2 subset was significantly lower in HIV/TB group than that in HIV group and TB group.
Conclusions: Our data suggested that HIV/TB co-infection altered the balance of γδ T cell subsets. The influence of HIV/TB co-infection on the function of γδ T cells to produce cytokines was complicated, which will shed light on further investigations on the mechanisms of the immune response against HIV and/or MTB infection.
Tuberculosis (TB), caused by Mycobacterium tuberculosis (MTB) infection, is the most common opportunistic infection and the major cause of death among people living with HIV (PLWH) (UNAIDS, 2019). TB harms the immune response to HIV infection, accelerating the progression from HIV infection to AIDS. HIV is also recognized as a crucial risk factor for developing active TB. Therefore, understanding the immunological characteristics in the process of HIV/TB co-infection is crucial for disease control.
γδ T cells are non-conventional T cells that express a T cell receptor (TCR) consisting of a γ and a δ chain with diverse structural and functional heterogeneity. Although the proportion of γδ T cells in total peripheral T lymphocytes is only 1-5%, their rapid cytokine production following activation plays an essential role in patients with tumors, autoimmunity, and infectious diseases (Halary et al., 2005; Zhang et al., 2006; Wu et al., 2014). Depending on the difference in δ chain, γδ T cells can be defined into two major subsets, including Vδ1 and Vδ2. Vδ1 subset, rare in peripheral blood, mainly resides in the epithelium of mucosal tissue and participates in respiratory and intestinal mucosal immunity (Wu et al., 2014). Vδ2 subset is predominately distributed in peripheral blood and represents a significant portion of γδ T cells (accounting for 90%), and the Vγ2Vδ2 (also known as Vγ9Vδ2) subtype is the primary circulating Vδ2 subset (Wu et al., 2014).
Both Vδ1 and Vδ2 subsets are altered after HIV infection. Previous reports have described an increased level of Vδ1 subset and decreased level and function of Vδ2 subset in chronically HIV-infected patients (Li et al., 2008; Zheng et al., 2011). Furthermore, Vδ2 subset is associated with disease progression in HIV infection and can inhibit active viral replication (Poccia et al., 1999; Li et al., 2008). γδ T cells can also enhance human immunity against MTB by secreting cytokines and promoting anti-TB immune response caused by other immune cells such as macrophages (Kawakami et al., 2004; Zhang et al., 2006). Similar to HIV infection, the frequency and function of Vδ2 subset is also decreased in TB patients (Agrati et al., 2011; Negash et al., 2018). Few evidences suggested that HIV/TB co-infection had additive effects on peripheral Vδ2 subset depletion and dysfunction (Rojas et al., 2005; Shao et al., 2008).
The frequency and function of γδ T cells are still poorly understood, especially in HIV/TB co-infection. By directed intracellular cytokine stain (ICS) (Yao et al., 2014; Zou et al., 2022) and flow cytometry, this study was performed to evaluate the phenotypes and function of γδ T cells in peripheral blood of HIV/TB co-infection patients (uninfected controls, patients with HIV or TB infection as control group).
A cross-sectional study was performed involving subjects (≥ 18 years old) divided into four groups from May 2018 to March 2020. (1) Healthy control (HC) group: healthy donors with no history of chronic inflammatory diseases and infection signs in at least 2 weeks before peripheral blood collection were recruited from the physical examination center in Zhongnan Hospital of Wuhan University. They all received tests of HIV antibody screening, chest X-ray, and Interferon Gamma Release Assay (IGRA) to rule out HIV and MTB infection. (2) TB group: individuals recruited from Wuhan Pulmonary Hospital with a confirmed diagnosis of TB by etiological or histopathological methods (smear or culture positive, and/or MTB DNA test positive, and/or Xpert MTB/RIF test positive, and/or histopathological evidence supporting TB) and HIV antibody negative. They were recruited from Wuhan Pulmonary Hospital. All patients had been excluded HIV infection and hadn’t received anti-TB therapy prior to enrollment. (3) HIV group: individuals recruited with confirmed HIV infection by HIV antibody confirmatory testing but excluding MTB infection by chest X-ray and IGRA. They were recruited from AIDS Clinical Guidance and Training Center, Zhongnan Hospital of Wuhan University All patients hadn’t received antiretroviral therapy (ART) prior to enrollment. (4) HIV/TB group: individuals recruited with confirmed HIV infection and TB by the above diagnostic methods. All patients were recruited from department of infectious diseases, Zhongnan Hospital of Wuhan University and hadn’t received ART and anti-TB therapy prior to enrollment.
For all groups, 10ml blood was drawn once with an ethylene diamine tetraacetic acid (EDTA) tube. Peripheral blood mononuclear cells (PBMCs) were isolated from freshly collected EDTA coagulated blood by Lymph prep (Axis-Shield, Norway) with density gradient centrifugation. Cell pellets were treated with 5 ml RBC lysis buffer (Sigma-Aldrich) for 10 min, followed by washing once with 5% FBS-PBS. PBMCs were then counted and cryopreserved until the following step experiments.
The following antibodies (Abs) were used for short-term culture or surface marker and intracellular cytokine staining for flow cytometry (all Abs were from Biolegend): anti-CD3-PerCP-cy5.5 (Clone UCHT1), anti-CD8-APC-Cy7 (Clone SK1), anti-CD4-PE-Cy7 (Clone RPA-T4), anti-Vδ1-APC (Clone REA173), anti-Vδ2-PE (Clone B6), anti-Vγ2-FITC (Clone 7A5), anti-TNF-α-PE (Clone MAb11), anti-IFN-γ-PE-Cy7 (Clone 4S.B3), anti-interleukin-17A (IL-17A)-PE-Cy7 (Clone BL168), anti-Granzyme A-PE (Clone CB9), anti-Perforin-APC (Clone dG9), anti-granulocyte macrophage colony-stimulating factor (GM-CSF)-APC (Clone BVD2-21C11). The isotype control mAbs were purchased from the related company, respectively. The reagents listed below were all commercial products: brefeldin A (GolgiPlug, BD Biosciences), Cytofix/Cytoperm, and Perm buffer (BD Biosciences). The isotype control mAbs were purchased from the related company, respectively.
This procedure was done exactly as we described (Yao et al., 2014; Zou et al., 2022). In brief, PBMCs were incubated for 1h with the medium in the presence of CD28 (1mg/ml) and CD49d (1 mg/ml) mAbs in a 200 ml final volume in round-bottom 96-well plates at 37°C, 5% CO2, followed by a 5-h incubation in the presence of brefeldin A (GolgiPlug; BD Biosciences). At the end of the incubation, cells were washed once with 2% FBS-PBS and stained at room temperature for at least 15-30 min with surface marker Abs (CD3, CD4, CD8, Vδ1, Vδ2 and Vγ2). After the next 45 min permeabilization (Cytofix/Cytoperm; BD Biosciences), another 45 min for intracellular cytokine staining (granzyme A, perforin, IL-17A, GM-CSF, IFN-γ and TNF-α) was performed. Finally, cells were re-suspended in 2% formaldehyde and subjected to flow cytometry analysis. To ensure the specific immune staining in direct or indirect ICS, matched isotype IgG served as negative controls.
Flow cytometric data were analyzed with FlowJo version 7.6.1 for Windows. Statistical analysis of data was performed using GraphPad Prism version for Windows. Data are presented as a median and interquartile range. Analysis of non-parametric data used the Mann-Whitney U test for comparison of the median. Non-parametric rank sum test was used for comparison between groups. Multiple linear regression was employed to identify CD4 count associated with the γδ frequency and subsets. All reported P values were 2-tailed, and a P-value of 0.05 or less was considered significant.
During the study period, 121 individuals (25 in HC group, 40 in TB group, 29 in HIV group and 27 in HIV/TB group) were evaluated and designated in different groups according to the rules described in the methods. Characteristics of these individuals are summarized in Table 1. There was no statistical difference in age and sex composition among all groups. The median CD4+ T lymphocyte count (CD4 count) in HIV group was significantly lower than the HC and TB group, and the HIV/TB group had a lower CD4 count than HIV group (Table 1). All individuals were tested for phenotypes of γδ T cells in PBMC (Table 1), and 85 individuals were tested for function analysis (Table 2).
To characterize the dynamics in γδ T cells during the course of HIV/TB co-infection, the percentage of γδ T cells (The sum of the percentage of Vδ1 subset and the percentage of Vδ2 subset), Vδ1 subset, Vδ2 subset and Vγ2Vδ2 subset in T lymphocytes were examined in HC, TB, HIV and HIV/TB groups. The flow cytometric gating strategy is illustrated in Supplement Figure 1. For the percentage of γδ T cells in T lymphocytes, except HC group, no significant difference was found between the HIV/TB and other groups (Figure 1A). The percentage of γδ T cells in TB was significantly higher than that in HC group (Figure 1A). As shown in Figure 1B, the percentage of Vδ1 subset between TB and HC group did not show statistical significance. In contrast, the percentage of Vδ1 subset in HIV and HIV/TB groups were significantly higher than that in HC and TB groups, respectively.
Figure 1 HIV or TB altered the balance of γδ T cell subsets respectively. (A) Percentages of γδ T cells in CD3+ T cells; (B) Percentages of Vδ1 subset in CD3+ T cells; (C) Percentages of Vδ2 subset in CD3+ T cells; (D) Percentages of Vγ2Vδ2 subset in CD3+ T cells; (E) Ratios of Vδ2/Vδ1. P-value calculated using the Mann–Whitney U test. Statistically significant differences between the groups were indicated as follows: *P<0.05 and **P<0.01. Those that were not statistically significant were not marked intra group or inter group.
Vδ2 subset is the most critical functional subset of γδ T cells in peripheral blood. As shown in Figures 1C, compared with the HC group, the TB, HIV and HIV/TB groups all had significantly lower fractions of Vδ2 subset. Compared to TB group, the percentage of Vδ2 subset in HIV/TB group was significantly lower (Figure 1C). The results of the ratios of Vγ2Vδ2 subset and Vδ2/Vδ1 were similar to Vδ2 subset (Figures 1D, E). No significant difference of the percentage of Vδ2 subset or Vγ2Vδ2 subset was found between the HIV/TB and HIV groups. These results suggested that the damage of Vδ2 subset was prominent in HIV or MTB infection and HIV and MTB co-infection took it further.
The expression of CD4 or CD8 on αβ (CD3+T cells) and Vδ2 subset was analyzed in different groups. The percentage of CD4+CD8- αβ and CD4+CD8- Vδ2 T cells in TB group were significantly higher than in the HC group (Figure 2A). Compared with HC group, the percentage of CD4+CD8- αβ T cells in HIV group was significantly decreased, while the percentage of CD4+CD8- Vδ2 subset didn’t reduce in HIV group (Figure 2A). Furthermore, the percentage of CD4+CD8- αβ and CD4+CD8- Vδ2 T cells in HIV/TB group was markedly lower than that in TB group (Figure 2A).
Figure 2 Comparison of the CD4+CD8- (A) and CD4+CD8+ (B) Vδ2 subset and αβ T cells in each group. P-value calculated using the Mann–Whitney U test. Statistically significant differences between the groups were indicated as follows: *P<0.05 and **P<0.01. Those that were not statistically significant were not marked intra group or inter group.
We also analyzed the CD4+CD8+ αβ T cells and Vδ2 subset in the different groups. The results of CD4+CD8+ αβ T cells and Vδ2 subset were similar. Compared to TB group, the percentage of CD4+CD8+ αβ T cells and Vδ2 subset in HIV, TB and HIV/TB groups were significantly higher. Unexpectedly, the percentage of CD4+CD8+ αβ and Vδ2 subset in HIV/TB group were markedly higher than HIV and TB groups (Figure 2B). Our data suggested that HIV/TB co-infection resulted in a marked increase of CD4+CD8+ T cells.
To further investigate the function of Vδ2 subset, we compared the cytokines production (TNF-α, GM-CSF, IL-17A, IFN-γ, Perforin and Granzyme A) in four groups (Table 3). All percentages represented the ratio of cytokine-secreting cells in Vδ2 subset. As shown in Figure 3A, there were no significant differences observed among the HC, TB, and HIV groups. In comparison with HIV/TB group, the percentage of TNF-α producing Vδ2 subset increased obviously in HIV group. On the contrary, the percentage of GM-CSF producing Vδ2 subset decreased obviously in HIV group compared to HIV/TB group.
Figure 3 Function of Vδ2 subset among each group. Subjects that produce TNF-a and GM-CSF (A), IL-17A and IFN-γ (B), perforin and granzyme A (C) in each group are shown. P-value calculated using the Mann–Whitney U test. Statistically significant differences between the groups were indicated as follows: *P<0.05 and **P<0.01. Those that were not statistically significant were not marked intra group or inter group.
To IL-17, compared to HC group, the percentage of IL-17A producing Vδ2 subset in TB and HIV/TB groups were significantly higher. Similarly, the ratio of IL-17A producing Vδ2 subset in HIV/TB group was significantly higher than HIV group (Figure 3B). These data suggested that TB infection might disturb the production of IL-17A. However, the percentage of IFN-γ producing Vδ2 T cells did not alter among the four groups (Figure 3B).
The percentage of perforin produced in the Vδ2 subset was significantly lower in HIV/TB group than in the HC, HIV and TB groups (Figure 3C). However, there was no significant difference between HIV, TB and HC groups, respectively (Figure 3C). This indicated that HIV/TB co-infection suppressed perforin production in Vδ2 subset by certain mechanisms, but the mono-infection of these two pathogens did not show the depression of perforin in Vδ2 subset. However, the percentage of granzyme A producing Vδ2 T cells did not alter among the four groups (Figure 3C).
γδ T cells have been proven to have anti-viral capability by lysing HIV-infected cells in vitro (Gan and Malkovsky, 1996). They can also enhance human immunity against HIV or MTB by secreting cytokines with the stimulation of phosphoantigens (Poccia et al., 1999; Zhang et al., 2006). However, the phenotype and function of γδ T cells during HIV/TB co-infection were poorly understood. The aim of this study was to evaluate the roles of γδ T cells in the peripheral blood of patients with HIV/TB co-infection, thus offering new insights into the role played by these cells in immunity against MTB and HIV.
In agreement with previous reports that the destruction of γδ T cells was in accordance with HIV infection progression (Zheng et al., 2011; Li et al., 2015), the present study confirmed that HIV infection caused an increased percentage of Vδ1 subset and a decreased percentage of Vδ2 subset. Several studies proved that Vδ2 subset could inhibit and directly kill the intracellular MTB, thus preventing disease progression (Li et al., 1996; Dieli et al., 2001). In the present study, a lower proportion of Vδ2 subset was observed in TB group compared with HC group, which was consistent with other studies (Li et al., 1996; Carvalho et al., 2002). Whether the reduction of Vδ2 subset is a predisposing factor to the development of TB or is a consequence of MTB infection itself is still unknown. These results suggested HIV or TB infection could alter the balance of γδ T cells subsets (especially the decrease of Vδ2 subset), which may deteriorate the status of patients with HIV or MTB infection. Moreover, in HIV/TB co-infection patients, the proportion of Vδ1 and Vδ2 subset had a further increased and decrease, respectively. Vδ1 expansion may be linked to microbial translocation and may impact coinfection with several herpesviruses. Vδ2 subset plays an important role in controlling HIV replication and disease progression (Poccia et al., 1999; Li et al., 2008). The decrease of Vδ2 in the HIV/TB group may indicate a decrease in the body's ability to control HIV (Halary et al., 2005; Harris et al., 2010). The reduction in Vδ2 subset observed in our study may attribute to the presence of specific HIV and MTB ligands inducing a sustained activation of Vδ2 T cells, followed by a reduction in this cell subset by spontaneous and activation-induced apoptosis (Poccia et al., 1996; Li et al., 1998). Our results also indicated that HIV/TB co-infection might synergistically affect escaping the immune attack. In addition, some metabolites may influence the phenotype and function of γδ T cells. Various manipulations affecting isoprenoid metabolism can lead to the stimulation of Vγ2Vδ2 T cells (Wang et al., 2011). In vitro, the metabolic conversion of isopentenyl pyrophosphate (IPP) was blocked by zoledronate, resulting in the selective activation and expansion of Vγ2Vδ2 T cells (Roelofs et al., 2009; Wang et al., 2011). Moreover, mycobacterial metabolites, such as 3-Formyl-1-butyl-PP, were demonstrated to activate human γδ T cells (Belmant et al., 1999). How HIV/TB co-infection affects the metabolism is still unclear. Further studies on the function of Vδ2 subset need to explain why the HIV/TB co-infection progresses more quickly and is much more complicated to control than single pathogen infection from the immunological and metabolic aspect.
Most γδ T cells are CD4 and CD8 double negative, while only a tiny part express CD4 or CD8 molecules (Zheng et al., 2011). Our study revealed characteristics of CD4 or CD8 expression on γδ T cells in patients with HIV infection and TB. As is known, CD4+ T lymphocytes are attacked and destroyed by HIV. In our study, the proportion of CD4+CD8- αβ T cells was significantly lower in HIV and HIV/TB group than that in HC and TB groups, respectively. However, the comparison between HIV and HC groups in our study revealed no significant difference in the percentage of CD4+CD8- Vδ2 T cells. This result might raise doubt about that HIV possibly infecting γδ T cells by CD4 molecules and co-receptors (CXCR4 and CCR5) to cause the depletion of Vδ2 subset (Imlach et al., 2003; Spencer et al., 2013). This result illustrated that CD4+CD8- Vδ2 T cells could survive during HIV infection and might be able to resist HIV infection but not HIV/TB co-infection. Recent studies suggested that double-positive cells (DP) T cells which accounted for only about 1%-2% of circulating humans are mature memory cells and may participate in the adaptive immune response against some infections (Overgaard et al., 2015; Zou et al., 2022). Our study described a significant increase in the percentage of DP T cells among Vδ2 subset and αβ T cells in the peripheral blood of HIV and TB group and incredibly high DP cells in HIV/TB co-infection group. These data showed that DP cells might play a certain role in the immune response of HIV or TB infection, particularly the co-infection of two pathogens.
Activated γδ T cells can secrete a variety of cytokines, including TNF-α, interleukin, IFN-γ, perforin, granzyme, and so on, which function as effector molecules in controlling infectious microorganisms (Li et al., 2008; Spencer et al., 2013). In this study, we investigated the function of γδ T cells in HIV and/or TB infection by analyzing cytokines produced by Vδ2 subset. TNF-α is the main component of the natural defense mechanism of the host. TNF-α can recruit monocytes, granulocytes and other innate immune cells to the location of infection and helps to form tuberculous granulomas in the infected tissues, which is beneficial to prevent the dissemination of TB bacteria in the body (Saunders et al., 2004). The levels of TNF-α also positively correlate with the progression of HIV infection (Benjamin et al., 2013; Vaidya et al., 2014), suggesting the critical role of TNF-α in HIV or/and TB. In the present study, we showed that the percentage of TNF-α producing γδ T cells was significantly lower in HIV/TB group compared to HIV group. However, there were no significant differences between the HC and TB group. Our data implied that HIV infection impaired the ability of γδ T cells to secrete TNF-α in active TB. Some studies also showed lower TNF production and cellular proliferation in MTB-specific peripheral T cells in HIV/MTB co-infection individuals than in individuals with HIV infection (Mendonca et al., 2007; Geldmacher et al., 2008), and HIV/MTB co-infected macrophages released fewer TNF-α than macrophages infected with only MTB (Kumawat et al., 2016). The reason might be that the expression of TNF-α in cells was down-regulated by HIV/MTB co-infection through some mechanism. Or the TNF-α-secreting cells may die through certain pathways in the case of HIV/MTB co-infection.
By inducing the expression of chemokines, recruiting monocytes and granulocytes and promoting the formation of granuloma, several studies demonstrated that IL-17 contributes to protective immunity in TB infection (Khader and Cooper, 2008; Torrado and Cooper, 2010). Nevertheless, some studies found that TB patients resistant to multiple drugs express high levels of IL-17 accompanied by severe tissue damage (Basile et al., 2011). Agree with another study (Peng et al., 2008), in the present study, we found that the percentage of IL-17A (referred to as IL-17) producing γδ T cells in TB patients was significantly higher than that in HC group, and there was a similar feature of change between HIV/TB and HIV patients. Our data not only further proved that γδ T cells might be involved in the immunity to MTB infection by secreting IL-17, but also suggests that HIV infection does not reduce the function of γδ T cells in producing IL-17. Considering the double-edged effect of IL-17, it is critical to elucidate further the mechanisms of IL-17 producing γδ T cells in the aspect of protective immunity and immunopathology during tuberculosis.
More studies show that effective cytotoxic T lymphocytes (CTL) responses require a coordinated expression of perforin and granzymes (Andersson et al., 1999; Chattopadhyay et al., 2009; Wu et al., 2014). A study describes that in TB, MTB-specific CD8+T cells express a low level of perforin but a relatively high level of granzyme A and B (Rozot et al., 2013). It is also reported that perforin-granzyme A+ CD8+ T cells had a weak capacity to kill target cells (Takata and Takiguchi, 2006). In our study, there was no significant difference in the percentage of γδ T cells secreting granzyme A among the four groups. However, the percentage of γδ T cells secreting perforin in HIV/TB group was significantly lower than that in HIV and TB group, and there was no significant difference between HIV and TB group and HC group. In addition, granzyme A secreted by Vδ2 subset has been found to induce TNF-α production in MTB-infected macrophages and inhibit the growth of intracellular MTB independently of perforin (Spencer et al., 2013). These results suggested that the anti-HIV and anti-TB effect of granzyme A and perforin secreted by Vδ2 subset was independent. Moreover, HIV/TB co-infection but not HIV or TB mono-infection could influence the ability of γδ T cells to secrete perforin.
This study has several limitations. First, the sample size was small. In addition, antigen-specific assays were not performed in our study. Although direct ICS represents the actual immune response in the human body, there is seldom such a high concentration of antigens in blood, and our results could not be compared with those of other studies using antigen-specific stimulation.
In conclusion, our results demonstrated a significant decrease in Vδ2 T cells in HIV/TB co-infection. Further studies on CD4+CD8- Vδ2 T cells will be needed to clarify their ability to resist HIV infection. The influence of HIV and /or MTB on the function of γδ T cells to produce cytokines is very complicated, which will shed light on further investigations on the mechanisms of the immune response against HIV and/or MTB infection.
The original contributions presented in the study are included in the article/Supplementary Material. Further inquiries can be directed to the corresponding authors.
The studies involving human participants were reviewed and approved by Research and Ethics Committee of Zhongnan Hospital, Wuhan University, P. R. China (2016009-1K). The patients/participants provided their written informed consent to participate in this study.
LS, YF, and KL conceived and designed this investigation. SZ, YX, and WG helped to design the scheme of the investigation and collected the original data. YT and QZ performed the experiments. YY and SW analyzed the data. SZ, YX, and KL contributed to the writing of the paper. All authors contributed to the article and approved the submitted version.
This work was supported by the Medical Science and Technology Innovation Platform Support Project of Zhongnan Hospital, Wuhan University (PTXM2020008), Science and Technology Innovation Cultivation Fund of Zhongnan Hospital, Wuhan University (cxpy2017043), Medical Science Advancement Program (Basic Medical Sciences) of Wuhan University (TFJC2018004) and the Non-profit Central Research Institute Fund of Chinese Academy of Medical Sciences (2020-PT320-004), Discipline Cultivation Project of Department of Infectious Diseases, Zhongnan Hospital, Wuhan University (ZNXKPY2021027).
The authors declare that the research was conducted in the absence of any commercial or financial relationships that could be construed as a potential conflict of interest.
All claims expressed in this article are solely those of the authors and do not necessarily represent those of their affiliated organizations, or those of the publisher, the editors and the reviewers. Any product that may be evaluated in this article, or claim that may be made by its manufacturer, is not guaranteed or endorsed by the publisher.
The Supplementary Material for this article can be found online at: https://www.frontiersin.org/articles/10.3389/fcimb.2022.1071880/full#supplementary-material
Agrati, C., D'Offizi, G., Gougeon, M. L., Malkovsky, M., Sacchi, A., Casetti, R., et al. (2011). Innate gamma/delta T-cells during HIV infection: Terra relatively incognita in novel vaccination strategies? AIDS Rev. 13 (1), 3–12.
Andersson, J., Behbahani, H., Lieberman, J., Connick, E., Landay, A., Patterson, B., et al. (1999). Perforin is not co-expressed with granzyme a within cytotoxic granules in CD8 T lymphocytes present in lymphoid tissue during chronic HIV infection. AIDS 13 (11), 1295–1303. doi: 10.1097/00002030-199907300-00005
Basile, J. I., Geffner, L. J., Romero, M. M., Balboa, L., Sabio, Y. G. C., Ritacco, V., et al. (2011). Outbreaks of mycobacterium tuberculosis MDR strains induce high IL-17 T-cell response in patients with MDR tuberculosis that is closely associated with high antigen load. J. Infect. Dis. 204 (7), 1054–1064. doi: 10.1093/infdis/jir460
Belmant, C., Espinosa, E., Poupot, R., Peyrat, M. A., Guiraud, M., Poquet, Y., et al. (1999). 3-Formyl-1-butyl pyrophosphate a novel mycobacterial metabolite-activating human gammadelta T cells. J. Biol. Chem. 274 (45), 32079–32084. doi: 10.1074/jbc.274.45.32079
Benjamin, R., Banerjee, A., Sunder, S. R., Gaddam, S., Valluri, V. L., Banerjee, S. (2013). Discordance in CD4+T-cell levels and viral loads with co-occurrence of elevated peripheral TNF-alpha and IL-4 in newly diagnosed HIV-TB co-infected cases. PloS One 8 (8), e70250. doi: 10.1371/journal.pone.0070250
Carvalho, A. C., Matteelli, A., Airo, P., Tedoldi, S., Casalini, C., Imberti, L., et al. (2002). Gammadelta T lymphocytes in the peripheral blood of patients with tuberculosis with and without HIV co-infection. Thorax 57 (4), 357–360. doi: 10.1136/thorax.57.4.357
Chattopadhyay, P. K., Betts, M. R., Price, D. A., Gostick, E., Horton, H., Roederer, M., et al. (2009). The cytolytic enzymes granyzme a, granzyme b, and perforin: expression patterns, cell distribution, and their relationship to cell maturity and bright CD57 expression. J. Leukoc. Biol. 85 (1), 88–97. doi: 10.1189/jlb.0208107
Dieli, F., Troye-Blomberg, M., Ivanyi, J., Fournie, J. J., Krensky, A. M., Bonneville, M., et al. (2001). Granulysin-dependent killing of intracellular and extracellular mycobacterium tuberculosis by Vgamma9/Vdelta2 T lymphocytes. J. Infect. Dis. 184 (8), 1082–1085. doi: 10.1086/323600
Gan, Y. H., Malkovsky, M. (1996). Mechanisms of simian gamma delta T cell cytotoxicity against tumor and immunodeficiency virus-infected cells. Immunol. Lett. 49 (3), 191–196. doi: 10.1016/0165-2478(96)02508-4
Geldmacher, C., Schuetz, A., Ngwenyama, N., Casazza, J. P., Sanga, E., Saathoff, E., et al. (2008). Early depletion of mycobacterium tuberculosis-specific T helper 1 cell responses after HIV-1 infection. J. Infect. Dis. 198 (11), 1590–1598. doi: 10.1086/593017
Halary, F., Pitard, V., Dlubek, D., Krzysiek, R., de la Salle, H., Merville, P., et al. (2005). Shared reactivity of V{delta}2(neg) {gamma}{delta} T cells against cytomegalovirus-infected cells and tumor intestinal epithelial cells. J. Exp. Med. 201 (10), 1567–1578. doi: 10.1084/jem.20041851
Harris, L. D., Klatt, N. R., Vinton, C., Briant, J. A., Tabb, B., Ladell, K., et al. (2010). Mechanisms underlying gammadelta T-cell subset perturbations in SIV-infected Asian rhesus macaques. Blood 116 (20), 4148–4157. doi: 10.1182/blood-2010-05-283549
Imlach, S., Leen, C., Bell, J. E., Simmonds, P. (2003). Phenotypic analysis of peripheral blood gammadelta T lymphocytes and their targeting by human immunodeficiency virus type 1 in vivo. Virology 305 (2), 415–427. doi: 10.1006/viro.2002.1759
Kawakami, K., Kinjo, Y., Uezu, K., Miyagi, K., Kinjo, T., Yara, S., et al. (2004). Interferon-gamma production and host protective response against mycobacterium tuberculosis in mice lacking both IL-12p40 and IL-18. Microbes Infect. 6 (4), 339–349. doi: 10.1016/j.micinf.2004.01.003
Khader, S. A., Cooper, A. M. (2008). IL-23 and IL-17 in tuberculosis. Cytokine 41 (2), 79–83. doi: 10.1016/j.cyto.2007.11.022
Kumawat, K., Pathak, S. K., Spetz, A. L., Kundu, M., Basu, J. (2016). Exogenous nef is an inhibitor of mycobacterium tuberculosis -induced tumor necrosis factor-alpha production and macrophage apoptosis. J. Biol. Chem. 291 (2), 665–666. doi: 10.1074/jbc.A109.073320
Li, B., Bassiri, H., Rossman, M. D., Kramer, P., Eyuboglu, A. F., Torres, M., et al. (1998). Involvement of the Fas/Fas ligand pathway in activation-induced cell death of mycobacteria-reactive human gamma delta T cells: a mechanism for the loss of gamma delta T cells in patients with pulmonary tuberculosis. J. Immunol. 161 (3), 1558–1567.
Li, Z., Jiao, Y., Hu, Y., Cui, L., Chen, D., Wu, H., et al. (2015). Distortion of memory Vdelta2 gammadelta T cells contributes to immune dysfunction in chronic HIV infection. Cell Mol. Immunol. 12 (5), 604–614. doi: 10.1038/cmi.2014.77
Li, H., Luo, K., Pauza, C. D. (2008). TNF-alpha is a positive regulatory factor for human Vgamma2 Vdelta2 T cells. J. Immunol. 181 (10), 7131–7137. doi: 10.4049/jimmunol.181.10.7131
Li, H., Peng, H., Ma, P., Ruan, Y., Su, B., Ding, X., et al. (2008). Association between Vgamma2Vdelta2 T cells and disease progression after infection with closely related strains of HIV in China. Clin. Infect. Dis. 46 (9), 1466–1472. doi: 10.1086/587107
Li, B., Rossman, M. D., Imir, T., Oner-Eyuboglu, A. F., Lee, C. W., Biancaniello, R., et al. (1996). Disease-specific changes in gammadelta T cell repertoire and function in patients with pulmonary tuberculosis. J. Immunol. 157 (9), 4222–4229.
Mendonca, M., Tanji, M. M., Silva, L. C., Silveira, G. G., Oliveira, S. C., Duarte, A. J., et al. (2007). Deficient in vitro anti-mycobacterial immunity despite successful long-term highly active antiretroviral therapy in HIV-infected patients with past history of tuberculosis infection or disease. Clin. Immunol. 125 (1), 60–66. doi: 10.1016/j.clim.2007.06.002
Negash, M., Tsegaye, A., Wassie, L., Howe, R. (2018). Phenotypic and functional heterogeneity of peripheral gammadelta T cells in pulmonary TB and HIV patients in Addis Ababa, Ethiopia. BMC Infect. Dis. 18 (1), 464. doi: 10.1186/s12879-018-3361-9
Overgaard, N. H., Jung, J. W., Steptoe, R. J., Wells, J. W. (2015). CD4+/CD8+ double-positive T cells: more than just a developmental stage? J. Leukoc. Biol. 97 (1), 31–38. doi: 10.1189/jlb.1RU0814-382
Peng, M. Y., Wang, Z. H., Yao, C. Y., Jiang, L. N., Jin, Q. L., Wang, J., et al. (2008). Interleukin 17-producing gamma delta T cells increased in patients with active pulmonary tuberculosis. Cell Mol. Immunol. 5 (3), 203–208. doi: 10.1038/cmi.2008.25
Poccia, F., Battistini, L., Cipriani, B., Mancino, G., Martini, F., Gougeon, M. L., et al. (1999). Phosphoantigen-reactive Vgamma9Vdelta2 T lymphocytes suppress in vitro human immunodeficiency virus type 1 replication by cell-released antiviral factors including CC chemokines. J. Infect. Dis. 180 (3), 858–861. doi: 10.1086/314925
Poccia, F., Boullier, S., Lecoeur, H., Cochet, M., Poquet, Y., Colizzi, V., et al. (1996). Peripheral V gamma 9/V delta 2 T cell deletion and anergy to nonpeptidic mycobacterial antigens in asymptomatic HIV-1-infected persons. J. Immunol. 157 (1), 449–461.
Roelofs, A. J., Jauhiainen, M., Monkkonen, H., Rogers, M. J., Monkkonen, J., Thompson, K. (2009). Peripheral blood monocytes are responsible for gammadelta T cell activation induced by zoledronic acid through accumulation of IPP/DMAPP. Br. J. Haematol. 144 (2), 245–250. doi: 10.1111/j.1365-2141.2008.07435.x
Rojas, R. E., Chervenak, K. A., Thomas, J., Morrow, J., Nshuti, L., Zalwango, S., et al. (2005). Vdelta2+ gammadelta T cell function in mycobacterium tuberculosis- and HIV-1-positive patients in the united states and Uganda: application of a whole-blood assay. J. Infect. Dis. 192 (10), 1806–1814. doi: 10.1086/497146
Rozot, V., Vigano, S., Mazza-Stalder, J., Idrizi, E., Day, C. L., Perreau, M., et al. (2013). Mycobacterium tuberculosis-specific CD8+ T cells are functionally and phenotypically different between latent infection and active disease. Eur. J. Immunol. 43 (6), 1568–1577. doi: 10.1002/eji.201243262
Saunders, B. M., Briscoe, H., Britton, W. J. (2004). T Cell-derived tumour necrosis factor is essential, but not sufficient, for protection against mycobacterium tuberculosis infection. Clin. Exp. Immunol. 137 (2), 279–287. doi: 10.1111/j.1365-2249.2004.02518.x
Shao, L., Zhang, W., Zhang, S., Chen, C. Y., Jiang, W., Xu, Y., et al. (2008). Potent immune responses of Ag-specific Vgamma2Vdelta2+ T cells and CD8+ T cells associated with latent stage of mycobacterium tuberculosis coinfection in HIV-1-infected humans. AIDS 22 (17), 2241–2250. doi: 10.1097/QAD.0b013e3283117f18
Spencer, C. T., Abate, G., Sakala, I. G., Xia, M., Truscott, S. M., Eickhoff, C. S., et al. (2013). Granzyme a produced by gamma(9)delta(2) T cells induces human macrophages to inhibit growth of an intracellular pathogen. PloS Pathog. 9 (1), e1003119. doi: 10.1371/journal.ppat.1003119
Takata, H., Takiguchi, M. (2006). Three memory subsets of human CD8+ T cells differently expressing three cytolytic effector molecules. J. Immunol. 177 (7), 4330–4340. doi: 10.4049/jimmunol.177.7.4330
Torrado, E., Cooper, A. M. (2010). IL-17 and Th17 cells in tuberculosis. Cytokine Growth Factor. Rev. 21 (6), 455–462. doi: 10.1016/j.cytogfr.2010.10.004
UNAIDS (2019) Tuberculosis and HIV — progress towards the 2020 target. Available at: https://www.unaids.org/en/resources/documents/2019/tuberculosis-and-hiv-progress-towards-the-2020-target.
Vaidya, S. A., Korner, C., Sirignano, M. N., Amero, M., Bazner, S., Rychert, J., et al. (2014). Tumor necrosis factor alpha is associated with viral control and early disease progression in patients with HIV type 1 infection. J. Infect. Dis. 210 (7), 1042–1046. doi: 10.1093/infdis/jiu206
Wang, H., Sarikonda, G., Puan, K. J., Tanaka, Y., Feng, J., Giner, J. L., et al. (2011). Indirect stimulation of human Vgamma2Vdelta2 T cells through alterations in isoprenoid metabolism. J. Immunol. 187 (10), 5099–5113. doi: 10.4049/jimmunol.1002697
Wu, Y. L., Ding, Y. P., Tanaka, Y., Shen, L. W., Wei, C. H., Minato, N., et al. (2014). Gammadelta T cells and their potential for immunotherapy. Int. J. Biol. Sci. 10 (2), 119–135. doi: 10.7150/ijbs.7823
Yao, S., Huang, D., Chen, C. Y., Halliday, L., Wang, R. C., Chen, Z. W. (2014). CD4+ T cells contain early extrapulmonary tuberculosis (TB) dissemination and rapid TB progression and sustain multieffector functions of CD8+ T and CD3- lymphocytes: mechanisms of CD4+ T cell immunity. J. Immunol. 192 (5), 2120–2132. doi: 10.4049/jimmunol.1301373
Zhang, R., Zheng, X., Li, B., Wei, H., Tian, Z. (2006). Human NK cells positively regulate gammadelta T cells in response to mycobacterium tuberculosis. J. Immunol. 176 (4), 2610–2616. doi: 10.4049/jimmunol.176.4.2610
Zheng, N. N., McElrath, M. J., Sow, P. S., Mesher, A., Hawes, S. E., Stern, J., et al. (2011). Association between peripheral gammadelta T-cell profile and disease progression in individuals infected with HIV-1 or HIV-2 in West Africa. J. Acquir. Immune Defic. Syndr. 57 (2), 92–100. doi: 10.1097/QAI.0b013e318215a877
Keywords: HIV, tuberculosis (TB), γδ T cells, TNF-α, IL-17A
Citation: Zou S, Xiang Y, Guo W, Zhu Q, Wu S, Tan Y, Yan Y, Shen L, Feng Y and Liang K (2022) Phenotype and function of peripheral blood γδ T cells in HIV infection with tuberculosis. Front. Cell. Infect. Microbiol. 12:1071880. doi: 10.3389/fcimb.2022.1071880
Received: 17 October 2022; Accepted: 12 December 2022;
Published: 23 December 2022.
Edited by:
Sergio Arce, University of South Carolina, United StatesReviewed by:
Cai Kun, Hubei Provincial Center for Diseases Control and Prevention, ChinaCopyright © 2022 Zou, Xiang, Guo, Zhu, Wu, Tan, Yan, Shen, Feng and Liang. This is an open-access article distributed under the terms of the Creative Commons Attribution License (CC BY). The use, distribution or reproduction in other forums is permitted, provided the original author(s) and the copyright owner(s) are credited and that the original publication in this journal is cited, in accordance with accepted academic practice. No use, distribution or reproduction is permitted which does not comply with these terms.
*Correspondence: Ling Shen, bHNoZW5AdWljLmVkdQ==; Yong Feng, eW9uZ2ZlbmdAd2h1LmVkdS5jbg==; Ke Liang, a2VsaWFuZ0B3aHUuZWR1LmNu
†These authors have contributed equally to this work and share first authorship
Disclaimer: All claims expressed in this article are solely those of the authors and do not necessarily represent those of their affiliated organizations, or those of the publisher, the editors and the reviewers. Any product that may be evaluated in this article or claim that may be made by its manufacturer is not guaranteed or endorsed by the publisher.
Research integrity at Frontiers
Learn more about the work of our research integrity team to safeguard the quality of each article we publish.