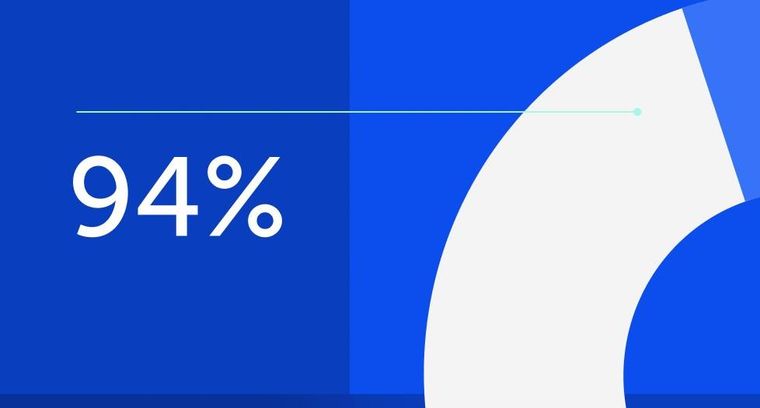
94% of researchers rate our articles as excellent or good
Learn more about the work of our research integrity team to safeguard the quality of each article we publish.
Find out more
REVIEW article
Front. Cell. Infect. Microbiol., 18 October 2022
Sec. Molecular Bacterial Pathogenesis
Volume 12 - 2022 | https://doi.org/10.3389/fcimb.2022.1029178
This article is part of the Research TopicNew Insights in Chlamydia: Host Interactions and PathogenesisView all 8 articles
Chlamydia trachomatis (Ct) is the leading cause of bacterial sexually transmitted infections worldwide. Since the symptoms of Ct infection are often subtle or absent, most people are unaware of their infection until they are tested or develop severe complications such as infertility. It is believed that the primary culprit of Ct-associated tissue damage is unresolved chronic inflammation, resulting in aberrant production of cytokines, chemokines, and growth factors, as well as dysregulated tissue influx of innate and adaptive immune cells. A member of the IL-6 cytokine family, leukemia inhibitory factor (LIF), is one of the cytokines induced by Ct infection but its role in Ct pathogenesis is unclear. In this article, we review the biology of LIF and LIF receptor (LIFR)-mediated signaling pathways, summarize the physiological role of LIF in the reproductive system, and discuss the impact of LIF in chronic inflammatory conditions and its implication in Ct pathogenesis. Under normal circumstances, LIF is produced to maintain epithelial homeostasis and tissue repair, including the aftermath of Ct infection. However, LIF/LIFR-mediated signaling – particularly prolonged strong signaling – can gradually transform the microenvironment of the fallopian tube by altering the fate of epithelial cells and the cellular composition of epithelium. This harmful transformation of epithelium may be a key process that leads to an enhanced risk of infertility, ectopic pregnancy and cancer following Ct infection.
The Chlamydiae family is a diverse group of obligatory intracellular bacteria, comprised of both pathogens and commensals, and found in varying habitats that can extend as far as the bottom of the Arctic Ocean (Dharamshi et al., 2020). Chlamydia trachomatis (Ct) is a human pathogen and the most common bacterial cause of sexually transmitted infections worldwide (WHO, 2021). An uptick in the reporting of chlamydial urogenital infections began in the 1990s following improvements in diagnostic technologies, and the rates have been increasing annually ever since (CDC, 2020; Huai et al., 2020; Control ECfDPa, 2022). Ct affects mostly young women aged 15-24, but can infect both men and women of all age groups (CDC, 2020; Huai et al., 2020; Control ECfDPa, 2022). Urogenital Ct infection is clinically associated with cervicitis, urethritis, endometritis, and salpingitis in women, and urethritis, proctitis and epididymitis in men (CDC, 2020; Control ECfDPa, 2022). However, Ct urogenital infections are notorious for being asymptomatic or sub-clinically symptomatic in as many as 70% of women and 50% of men (CDC, 2020; Gupta et al., 2021; Control ECfDPa, 2022). These “silent” Ct infections are often left untreated, which can last for months to years (McCormack et al., 1979; Morré et al., 2002; Molano et al., 2005; Geisler, 2010). Approximately 17% of Ct-infected women go on to develop pelvic inflammatory disease (PID) and serious complications such as tubal factor infertility (TFI), ectopic pregnancy and chronic pelvic pain (Cherpes et al., 2011; Brunham et al., 2015; Price et al., 2016). Repeated Ct infections and recurrent PID episodes are associated with a greater risk for adverse reproductive outcomes (Hillis et al., 1997; Hosenfeld et al., 2009; Batteiger et al., 2010; Trent et al., 2011; Bautista et al., 2018). Epidemiological evidence indicate that Ct infections significantly increase the risk of cervical (Zhu et al., 2016) and ovarian cancer (Hosseininasab-Nodoushan et al., 2021), presumably due to unresolved chronic inflammation (Clendenen et al., 2011; Peres et al., 2021). While considerable efforts have been made in attempts to elucidate Ct pathogenesis, there are still gaps regarding the definitive molecular and cellular mechanisms that cause Ct-associated tissue damage.
Chlamydia species have a unique biphasic lifecycle consisting of an extracellular form of non-replicative but infectious elementary body (EB), and an intracellular form of replicative reticulate body (RB) (Moulder, 1991; Hackstadt et al., 1997). Infection is normally initiated at the single layer of mucosal epithelium in the lower genital tract, followed by bacterial ascension to the upper genital tract. Once inside the host cells, the non-replicative EB resides within a cytoplasmic inclusion body, where it differentiates into a replicative RB which then multiplies via binary fission. As an inclusion fills with progeny, RBs transform back into infectious EBs that are released from host cells to infect other neighboring cells (Moulder, 1991; Hackstadt et al., 1997). Immediately after intracellular infection, cell-autonomous immunity and innate defence mechanisms are triggered to fight against intracellular Ct infections (Mostowy and Shenoy, 2015; Elwell et al., 2016). These initial molecular and cellular events are followed by induction of Ct-specific adaptive humoral and cellular immune responses that are required for the ultimate elimination of intracellular Ct infection (Kelly, 2003; Batteiger et al., 2010; Helble and Starnbach, 2021). Notably, the inflammatory responses associated with innate and adaptive immunity are also involved in the tissue repair and regeneration processes (Eming et al., 2017), which are critical for removing the cellular debris from the infected tissue site, maintaining the tissue homeostasis, and restoring the normal structure and physiological integrity of the organ after infection (Gurtner et al., 2008). Of note, Chlamydia species are known for their strong ability to exploit various strategies to enhance intracellular survival (Fischer and Rudel, 2018; Chen et al., 2019). Some RBs ultimately differentiate into a morphologically distinct persistence form called an aberrant body (AB) in response to host defense molecules and certain antibiotic treatments (Phillips et al., 1984; Mazzoli et al., 2000; Hogan et al., 2004). While ABs are nonreplicative, they remain dormant inside host cells for a long period of time and are capable of reverting back to replicative RBs when more favorable conditions are present, such as the use of an immune suppressant (Yang et al., 1983; Beatty et al., 1994; Laitinen et al., 1996). As such, the host responses to Ct infection are complex and often modified by persistent infection, reactivation, and/or repeated exposures (Schuchardt and Rupp, 2018). The most widely accepted theory of Ct pathogenesis is that unresolved chronic inflammation is the primary culprit of Ct-associated tissue damage. This involves aberrant production of cytokines, chemokines, and growth factors, as well as the influx of innate and adaptive immune cells that collectively trigger aggravated wound healing and tissue repair processes (Karin and Clevers, 2016). While numerous cytokines have been implicated as part of the host response to Ct infection (Yang, 2003; Xiang et al., 2021), leukemia inhibitory factor (LIF), a member of the interleukin 6 (IL-6) cytokine family, has been largely ignored. Recent studies indicate that LIF is readily induced by Ct infection (Hess et al., 2001; Kessler et al., 2019), and that increased LIF expression is often linked to Ct-associated ectopic pregnancies in humans (Refaat et al., 2016) as well as severe oviductal pathology in mice (Hou et al., 2018). Therefore, LIF likely holds new perspectives of Ct pathogenesis. In this article, we review the biology of LIF and LIF receptor (LIFR)-mediated signaling pathways, summarize the physiological role of LIF in the reproductive system, and discuss the impact of LIF in chronic inflammatory conditions and its implication in Ct pathogenesis.
LIF is encoded by a single copy gene on chromosome 22 in humans and chromosome 11 in mice (Gough et al., 1988; Metcalfe, 2011). The coding region is highly conserved among several mammalian species with more than 75% homology (Willson et al., 1992). LIF belongs to the IL-6 cytokine family that consists of IL-6, IL-11, IL-27, LIF, oncostatin M (OSM), ciliary neurotrophic factor (CNTF), cardiotrophin 1 (CT-1) and cardiotrophin-like cytokine factor 1 (CLCF1) (Nicola and Babon, 2015; Rose-John, 2018). All IL-6 family members utilize a receptor complex that is comprised of the shared signal-transducing receptor β-subunit gp130 (also known as IL-6Rβ), together with either a ligand-binding non-signaling receptor α-subunit and/or a signaling receptor β-subunit resembling gp130 for signal transduction. The specific composition of different ligand-receptor complexes underscores both redundant and unique biological activities of IL-6 family cytokines (Rose-John, 2018). In the case of LIF signaling, LIF binds to a receptor complex consisting of gp130 and LIFR, both of which are constitutively associated with receptor-associated JAK molecules, particularly JAK1 (Rodig et al., 1998; Chung et al., 2006; Takahashi et al., 2008), and initiates the signal transduction cascades that lead to activation of JAK/STAT3, MAPK and PI3K/AKT signaling pathways (Nicola and Babon, 2015). Notably, JAK/STAT3 signaling also induces the expression of suppressor of cytokine signaling 3 (SOCS3) (Babon et al., 2014), which binds to both JAK1 and gp130 and induces their ubiquitination and degradation, thereby shutting down entire signaling cascades (Kershaw et al., 2013) (Figure 1). While these pathways collectively contribute to cellular differentiation, survival and self-renewal, the qualitative and quantitative contributions of individual pathways are cell-type specific, leading to the pleiotropic effects of LIF (Nicola and Babon, 2015). Although named for its ability to inhibit proliferation of a myeloid leukemia cell line by inducing its terminal differentiation into macrophages (Gearing et al., 1987), LIF also regulates the growth and differentiation of embryonic stem cells (Smith et al., 1988; Williams et al., 1988), peripheral neurons (Murphy et al., 1991; Li et al., 1995; Cheng and Patterson, 1997), osteoblasts (Matsushita et al., 2014; Sims, 2020), adipocytes (Guo et al., 2021; Zeng et al., 2022), hepatocytes (Baumann and Wong, 1989), and endothelial cells (Ferrara et al., 1992; Li et al., 2022). This multifarious property of LIF has led to several rediscoveries of the protein and a variety of synonyms have been used in the older literature (Alexander et al., 1994; Nicola and Babon, 2015) (Table 1). Since its original discovery, LIF has been shown to display a diverse range of biological activities in embryo implantation, bone metabolism and remodelling, immune regulation, as well as the development of uterine, hematopoietic, and nervous systems (Kurzrock et al., 1991; Mathieu et al., 2012; Nicola and Babon, 2015). The broad effects of LIF also coincide with ubiquitous expression of LIFR in The Human Protein Atlas (Human Protein Atlas, 2022) (Figure 2A). However, contrary to the LIFR expression pattern, LIF expression is enriched in glandular and luminal cells in select healthy human tissues (Human Protein Atlas, 2022) (Figure 2B). Overall, LIF production can be detected in many cell types, such as stromal fibroblasts (Tomida et al., 1984), bone marrow stromal cells (Wetzler et al., 1994), activated monocytes and macrophages (Anegon et al., 1990), astrocytes (Wesselingh et al., 1990), and T cells (Gearing et al., 1987).
Figure 1 LIF signaling. LIF signaling occurs through a receptor complex of gp130 and LIFR. Under resting conditions, both receptor chains are constitutively associated with inactivated JAK1 molecules. Upon LIF stimulation, LIF binds to LIFR and gp130 and initiates signal transduction by transactivation of the gp130-bound and LIFR-bound JAK1 molecules. JAK1 then phosphorylates five tyrosine molecules on each receptor chain, which create the docking sites for the transcription factors STAT3 and SHP-2, leading to activation of JAK/STAT3, MAPK and PI3K/AKT signaling pathways. The docking site also recruits transcription factor SOCS3, which is a predominant negative pathway that inhibits JAK-STAT3 activation. By controlling cellular differentiation, survival and self-renewal, these pathways collectively contribute to specific physiological and immunological processes such as tissue homeostasis, hematopoiesis, pregnancy, bone remodeling, neuromuscular system, inflammatory conditions, and cancer (Nicola et al., 2015 and Rose-John et al., 2018).
Figure 2 LIFR and LIF expression in human tissues. The transcripts of LIFR and LIF in 55 healthy human tissues are displayed at normalized expression levels (nTPM) that are created by combining transcriptomics datasets of The Human Protein Atlas (HPA) project and The Genotype-Tissue Expression (GTEx) project. Figure is available from The Human Protein Atlas website (accessed on August 15, 2022).
Menstruation is a key feature of the mammalian female reproductive system that is characterized by monthly remolding, shedding and regeneration of the endometrium under the influence of ovarian hormones and divided into an estrogen (E2)-dominated proliferative phase and a progesterone (P4)-dominated secretory phase (Reed and Carr, 2000). Cell types in the human endometrium include epithelial cells, stromal fibroblasts, immune cells, vascular endothelium, vascular smooth muscle cells and stem/progenitor cells. The epithelial cells are further divided into three subpopulations: luminal epithelium, glandular epithelium, and ciliated epithelial cells (Wang et al., 2020; Giudice, 2020). Functionally, the glandular and luminal epithelia are required for embryo attachment whereas the ciliated epithelial cells are needed to facilitate sperm transport, fluid movement and removal of debris from the uterine cavity (Giudice, 2020). During the menstrual cycle, the human endometrium undergoes a decidualization process, which is characterized by the transformation of stromal cells to round epithelioid cells capable of secreting growth hormones (e.g., prolactin and insulin-like growth factor binding protein-1), an influx of specialized uterine natural killer cells, and vascular remodeling to support the maternal blood supply to the growing embryo (Okada et al., 2018). The decidua plays a critical role in regulating trophoblast invasion, modulating the local immune response at the fetal-maternal interface, and development of the placenta (Ramathal et al., 2010; Okada et al., 2018). The human decidua is formed routinely and is shed off in the absence of an embryo in the endometrium, whereas the decidua is only formed upon embryo implantation in mice (Ramathal et al., 2010). While the steroid hormones E2 and P4 have paramount effects on the development and differentiation of decidua, the hormone effects are also mediated either directly or indirectly through locally produced growth factors and cytokines (Large and DeMayo, 2012). LIF is one of the most important cytokines essential for decidualization, embryo implantation, and regulation of immune tolerance at the fetal-maternal interface (Stewart et al., 1992; Aghajanova, 2004; Kimber, 2005).
In healthy women, LIF mRNA and protein are expressed by the endometrium throughout the menstrual cycle, with a striking increase in the mid-secretory to late-secretory phase (Charnock-Jones et al., 1994; Arici et al., 1995; Vogiagis et al., 1996) which is a finite period defined as the implantation window (Harper, 1992). Single-cell transcriptomic analysis of the human endometrium during the menstrual cycle has revealed an abrupt and strong transcriptomic activation in epithelial cells, and a widespread decidualization feature in the stromal cells during the implantation window (Wang et al., 2020). LIF mRNA is most abundantly expressed in the glandular and luminal epithelial cells (Human Protein Atlas, 2022) (Figure 3), and the same expression pattern is detected by immunohistochemistry staining (Charnock-Jones et al., 1994; Vogiagis et al., 1996). In parallel with the pattern of LIF expression, LIFR also peaks in human endometrium during the mid-secretory phase (Charnock-Jones et al., 1994; Cullinan et al., 1996), with strong expression in endothelial cells, intermediate levels in glandular epithelial cells, luminal epithelial cells and smooth muscle cells, but very low levels in immune cells (Human Protein Atlas, 2022) (Figure 3). While the tumor suppressor gene p53 is required to maintain both basal and inducible transcription of LIF in the uterus (Hu et al., 2007), endometrial expression of LIF and LIFR are induced by E2 (Chen et al., 2000; Ding et al., 2008; Liang et al., 2014; Yoo et al., 2019; Polim et al., 2022) and P4 (Ding et al., 2008; Yoo et al., 2019), respectively. Interestingly, P4 also induces LIF expression in bovine endometrium epithelial cells in vitro (Feng et al., 2022). As such, the concerted physiological effects of E2 and P4 in the reproductive system are executed, if not entirely, via regulation of LIF/LIFR signaling pathways, particularly the JAK-STAT3 pathway (Cheng et al., 2001). LIF activates over 40 different transcription factors that control a multitude of physiological pathways and signal cascades for epithelial polarity and apoptosis, epithelial-mesenchymal interaction, angiogenesis, stromal decidualization and cell proliferation, creating a favorable environment in the uterus to promote embryo implantation (Rosario et al., 2014; Salleh and Giribabu, 2014; Rosario and Stewart, 2016).
Figure 3 LIF and LIFR mRNA expression in different cell types of the human endometrium. The transcripts of LIF and LIFR in healthy human endometrial tissues are displayed at normalized expression levels (nTPM). Cell types in the endometrium include epithelial cells (blue), stromal fibroblasts (green), immune cells (red), vascular endothelium (purple), and vascular smooth muscle cells (orange). The epithelial cells are further divided into luminal epithelium, glandular epithelium and ciliated epithelial cells. The ciliated epithelial cell clusters are highlighted in yellow boxes. Figure is available from The Human Protein Atlas website (accessed on August 15, 2022).
Functional LIFR and gp130 are expressed on both luminal and granular epithelial cells, human oocytes and preimplantation embryos (van Eijk et al., 1996). This expression pattern suggests that LIF/LIFR-mediated signaling pathways may promote embryo implantation via both autocrine and paracrine pathways. Several studies using gene-deficient mice and embryo-transfer approaches indicate that maternal LIF/LIFR-mediated signaling pathways are essential in embryo implantation (Stewart et al., 1992; Cheng et al., 2002; Cheng et al., 2017). Both LIF-deficient mice (Stewart et al., 1992) and mice with LIFR-deficiency specifically in luminal and glandular epithelial cells (Cheng et al., 2017), fail to support embryo implantation and development. However, the development of LIF-deficient embryos in LIF-deficient mice can be rescued by recombinant LIF injections (Chen et al., 2000). In accordance with the animal studies, reduced LIF production is observed in women with unexplained infertility and recurrent miscarriages compared to healthy fertile women in many studies (Laird et al., 1997; Hambartsoumian, 1998; Wu et al., 2013; Margioula-Siarkou et al., 2016; Margioula-Siarkou et al., 2017; Alzaidi et al., 2021), although there are exceptions (Olivennes et al., 2003; Xu et al., 2012; Karaer et al., 2014). Interestingly, certain LIF polymorphisms have been associated with recurrent implantation failure (Vagnini et al., 2019) and women with LIF mutations also have poor success rates of implantation and fertilization (Giess et al., 1999; Novotný et al., 2009) (Table 2).
Table 2 Influence of LIF in human infertility, miscarriage and assisted pregnancy Brief description of the study.
Although the collective evidence support a critical role for LIF in promoting embryo implantation, recombinant human LIF did not yield any beneficial effects in one randomized, double-blind, placebo-controlled, multicenter study (Brinsden et al., 2009). Surprisingly, recombinant LIF, administered to infertile women subcutaneously for 7 days starting on the day of embryo transfer, significantly reduced clinical pregnancy rates (Brinsden et al., 2009). Although the reason behind this unexpected result remains to be investigated, it is possible that a balanced LIF/LIFR signal is critical for successful pregnancies; both too little and too much LIF production can cause infertility. A prolonged strong LIF signal may trigger activation of a negative feedback pathway mediated by SOCS, subsequently promoting degradation of receptors, leading to implantation failure (Carvalho et al., 2014; Zhao et al., 2021). Furthermore, implantation is a complex and highly organized process that involves active crosstalk between a receptive uterus and a competent blastocyte in a time- and location-specific manner (Kim and Kim, 2017). Thus, dysregulation of LIF/LIFR may lead to ectopic pregnancy and potentially other fertility-related issues (Krishnan et al., 2013).
In addition to its physiological roles, the LIF/LIFR axis has been broadly discussed in the context of chronic inflammation, including chronic airway inflammation (Knight, 2001), cutaneous inflammation (Zhu et al., 2001), neuroinflammation (Linker et al., 2008; Pan et al., 2008), and cancer-associated inflammation (Christianson et al., 2021), mostly as an anti-inflammatory cytokine. In infection models, LIF is recognized as a vital stem cell growth factor that protects the lung from collateral damage during inflammatory attack against viral infections (Quinton et al., 2012; Foronjy et al., 2014).
The production of LIF during chlamydial infection has been reported by several in vitro and in vivo studies (Hess et al., 2001; Peters et al., 2005; Refaat et al., 2016; Hou et al., 2018; Kessler et al., 2019). It was first identified through comparing the transcriptomic profile of 1176 genes in DNA arrays of Ct-infected and mock-infected epithelial HeLa cells, in which 18 genes, including LIF, were up-regulated by Ct infection (Hess et al., 2001). This observation has been recently confirmed in human fallopian tube organoid cultures (Kessler et al., 2019), which provide a novel in vitro system for recapitulating Ct infection in vivo. It was demonstrated that LIF is readily induced by Ct infection, along with robust activation of type I interferon (IFN-β) signaling and upregulation of inducible nitric oxide synthase (NOS2) to control Ct replication in organoids. In addition, LIF/LIFR signaling is involved in tissue injury and repair responses that are needed for maintaining epithelial homeostasis and organoid renewal (Kessler et al., 2019). Therefore, LIF seems to be part of cell-autonomous immunity, and its production is a protective response. Notably, LIF production is only triggered in mice by a pathological, plasmid-containing Chlamydia muridarum, but not a plasmid-free one. The level of LIF is closely associated with the degree of bacterial ascension in the upper genital tract and the formation of hydrosalpinx (Hou et al., 2018), a common tissue pathology associated with Ct infection in both humans and mice that can cause infertility. Similarly, LIF mRNA and protein are most detected at high levels in human fallopian tube samples obtained from ectopic pregnancies associated with Ct infections but not non-Ct infections (Refaat et al., 2016). While these experimental results support a potential role of LIF in Ct pathogenesis, it is intriguing why a protective response is highly associated with tissue pathology.
The human fallopian tube is a conduit that has a major functional role in oocyte pickup, fertilization, and embryo transport. The fallopian tube mucosa contains two major histologic cell types: ciliated epithelial cells and secretory epithelial cells, which work together for effective tubal transport of ova, sperm, and embryos for successful spontaneous pregnancy (Lyons et al., 2006). Propulsion of gametes and embryos is achieved by complex interactions between muscle contractions, ciliary activity, and the flow of tubal secretions. Proper density of ciliated cells is required to avoid ectopic pregnancy (Lyons et al., 2006). It has been demonstrated that fallopian tubes containing an ectopic pregnancy have a marked reduction in the number of ciliated cells in comparison with those of women with an intrauterine gestation (Vasquez et al., 1983; Lyons et al., 2006). Detailed ultrastructural analysis of hydrosalpinx of infertile women also demonstrates severe abnormalities in epithelial cells, including flattening of the epithelial layer and severe loss of ciliated cells (Ajonuma et al., 2005). Notably, ciliated cells and secretory cells are developmentally connected. A study using in vivo genetic cell lineage tracing in mice demonstrated that secretory epithelial cells not only self-renew, but also give rise to ciliated epithelial cells (Ghosh et al., 2017). LIF/LIFR signaling has been shown to alter the fate of epithelial cells during Ct infection (Kessler et al., 2019). The density of ciliated cells in organoids chronically infected with Ct is markedly reduced compared to non-infected controls (Kessler et al., 2019). This is likely mediated by LIF, as addition of recombinant LIF protein to non-infected organoids potently inhibits the frequency of ciliated cells (Kessler et al., 2019). The results from the organoid cultures provide strong experimental evidence supporting a potential role of LIF in epithelial transformation during Ct infection.
LIF is also recognized as a cell-autonomous molecule during intracellular viral infections caused by HIV (Patterson et al., 2002; Tjernlund et al., 2006) and HPV (Bay et al., 2011). We were unable to find any reports on LIF production caused by extracellular pathogens, such as Neisseria gonorrhoeae. Therefore, it is likely that LIF is produced preferentially, if not exclusively, upon intracellular infections for combating intracellular pathogens and assisting in tissue repair and epithelium homeostasis. While this is a protective response in nature, repeated Ct infections and/or persistent Ct infections may lead to aberrant expression of LIF and LIF-mediated alterations of the epithelium, which has been demonstrated in in vitro models of persistent chlamydial infections (Peters et al., 2005). Clinical observations indicate that most women with TFI and serological evidence of Ct infection lack a history of clinical PID (Brunham et al., 1985). Therefore, it is likely that the regulation of LIF and LIF-mediated responses are operating for long periods of time, during which, LIF levels are constantly being modified by surrounding proinflammatory cytokines and growth factors (Table 3). LIF expression is robustly induced by proinflammatory signals (e.g., LPS, IL-1 and TNF-α) (Wetzler et al., 1991; Ishimi et al., 1992; Hamilton et al., 1993; Wetzler et al., 1994; Arici et al., 1995; Perrier d'Hauterive et al., 2004) and signaling molecules active in tissue growth and development, including platelet-derived growth factor (PDGF) (Arici et al., 1995), epidermal growth factor (EGF) (Arici et al., 1995), human chorionic gonadotropin (hCG) (Perrier d'Hauterive et al., 2004), insulin-like growth factor (IGF) (Perrier d'Hauterive et al., 2004), and transforming growth factor-β (TGF-β) (Wetzler et al., 1991; Arici et al., 1995; Perrier d'Hauterive et al., 2004; Ruan et al., 2010; Ota et al., 2013). Many of these molecules are concurrently induced by Ct infection, which collectively amplify LIF expression and LIFR-mediated responses, and eventually lead to a marked reduction in ciliated epithelial cells and an increase of secretory epithelial cells in the fallopian tube. In comparison, IFN-γ potently supresses LIF expression in endometrial epithelial cells and stromal cells in vitro (Arici et al., 1995). Of note, IL-4 is a typical type 2 cytokine that regulates LIF in a cell type-dependent manner. IL-4 downregulates LIF in cultured bone marrow stromal cells, synovial fibroblasts and liver myofibroblasts (Wetzler et al., 1994; Denizot et al., 1999), whereas it upregulates LIF secretion in type 2 helper T-cells (Piccinni et al., 1998). Given that IFN-γ and IL-4 are primarily produced by T cells, it is possible that LIF production may diminish upon the establishment of adaptive immune responses. While LIF has been shown to promote regulatory T cells and inhibit the differentiation of type 17 helper T-cells (Metcalfe, 2011), it is unclear how T cell responses are regulated by prolonged LIF production during Ct infection. Although only limited knowledge is available, the crosstalk between LIF and T cells is likely an integral part of Ct pathogenesis and additional studies are warranted.
Based on the collective evidence discussed above, it is appealing to suggest that the LIF-mediated reduction in ciliated epithelial cell density is a key process of Ct pathogenesis, which can lead to reduced opportunities for fertilization and increased risk of ectopic pregnancy. Although it remains to be demonstrated experimentally, increased secretory cell density may lead to over-production and accumulation of fluids inside the fallopian tube, a characteristic feature of hydrosalpinx (Ng and Cheong, 2019). Consistent with this notion, LIF is found to be expressed in human fallopian tubes (Keltz et al., 1996) and is markedly elevated in chronically inflamed fallopian tubes (Ji et al., 2009). Furthermore, ectopic pregnancies are mostly associated with intracellular infections caused by Ct and Mycoplasma genitalium, and less frequently linked to extracellular pathogens like Neisseria gonorrhoeae (Ashshi et al., 2015; Refaat et al., 2016), despite its ability to cause a similar spectrum of pelvic inflammatory diseases (Xu and Gray-Owen, 2021).
The Human Protein Atlas dataset shows that high expression of LIF is a poor prognostic marker for human cervical cancer (Human Protein Atlas, 2022), for which HPV and Ct are well-known risk factors (Zhu et al., 2016; Liao et al., 2022). Ct infection is also a risk factor for ovarian cancer (Hosseininasab-Nodoushan et al., 2021). While HPV promotes cervical cancer via oncogenic transformation (Baedyananda et al., 2022), Ct may promote cervical cancer and ovarian cancer via aberrant LIF signaling, which has been shown to regulate multiple hallmarks of cancer, including proliferation, metastasis and chemoresistance (Jones and Jenkins, 2018; Jorgensen and de la Puente, 2022). LIF also has a significant role in enriching and maintaining cancer stem cells, epithelial to mesenchymal transition, de-differentiation, and re-differentiation of cancer cells (Halder et al., 2022).
In conclusion, available evidence supports a novel aspect of Ct pathogenesis controlled by the pleiotropic cytokine LIF (Figure 4). Despite the intended purpose of LIF production as a part of the host defense against intracellular Ct infection and host protective tissue healing, LIF-mediated signaling, particularly prolonged strong signaling, gradually transforms the microenvironment of the fallopian tube by diminishing the density of ciliated epithelial cells and increasing the population of less differentiated secretory epithelial cells. This harmful transformation of epithelium might be a key process leading to an enhanced risk of infertility, ectopic pregnancy and cancer.
Figure 4 A potential role of LIF in Chlamydia pathogenesis. LIF is produced by epithelial cells upon Ct infection, which is required for maintaining epithelium homeostasis and normal tissue repair via autocrine LIF/LIFR signaling pathways. This would occur in many Ct-infected women without leaving serious complications. However, aberrant tissue repair is triggered by repeated or persistent infections, which results in marked reductions of ciliated epithelial cells and increases in secretory cells over time. The alteration in the cellular composition creates a microenvironment in fallopian tubes that may promote infertility, ectopic pregnancy and cancer.
All authors listed have made a substantial, direct, and intellectual contribution to the work and approved it for publication.
The study is supported by operating grant of the Canadian Institute of Health Research (CIHR) (Grant number: 201803PJT-159700) to JW. KW is supported by a Dalhousie Medical Research Foundation (DMRF) – Infection, Immunity, Inflammation & Vaccinology (I3V) Graduate Studentship.
The authors declare that the research was conducted in the absence of any commercial or financial relationships that could be construed as a potential conflict of interest.
All claims expressed in this article are solely those of the authors and do not necessarily represent those of their affiliated organizations, or those of the publisher, the editors and the reviewers. Any product that may be evaluated in this article, or claim that may be made by its manufacturer, is not guaranteed or endorsed by the publisher.
Aghajanova, L. (2004). Leukemia inhibitory factor and human embryo implantation. Ann. N Y Acad. Sci. 1034, 176–183. doi: 10.1196/annals.1335.020
Ajonuma, L. C., Ng, E. H., Chan, L. N., Chow, P. H., Kung, L. S., Cheung, A. N., et al. (2005). Ultrastructural characterization of whole hydrosalpinx from infertile Chinese women. Cell Biol. Int. 29 (10), 849–856. doi: 10.1016/j.cellbi.2005.05.012
Alexander, H. R., Billingsley, K. G., Block, M. I., Fraker, D. L. (1994). D-factor/leukaemia inhibitory factor: evidence for its role as a mediator in acute and chronic inflammatory disease. Cytokine. 6 (6), 589–596. doi: 10.1016/1043-4666(94)90045-0
Alzaidi, Z., Yildiz, ŞM, Saatçi, Ç, Akalin, H. Ü., Muderris, I. I., Aynekin, B., et al. (2021). The effect of cytokine leukemia-inhibitory factor (LIF) and interleukin-11 (IL-11) gene expression on the primary infertility related to polycystic ovary syndrome, tubal factor, and unexplained infertility in Turkish women. Egypt J. Med. Hum. Genet. 22 (1), 85. doi: 10.1186/s43042-021-00201-9
Anegon, I., Moreau, J. F., Godard, A., Jacques, Y., Peyrat, M. A., Hallet, M. M., et al. (1990). Production of human interleukin for DA cells (HILDA)/leukemia inhibitory factor (LIF) by activated monocytes. Cell Immunol. 130 (1), 50–65. doi: 10.1016/0008-8749(90)90161-J
Arici, A., Engin, O., Attar, E., Olive, D. L. (1995). Modulation of leukemia inhibitory factor gene expression and protein biosynthesis in human endometrium. J. Clin. Endocrinol. Metab. 80 (6), 1908–1915. doi: 10.1210/jcem.80.6.7775640
Ashshi, A. M., Batwa, S. A., Kutbi, S. Y., Malibary, F. A., Batwa, M., Refaat, B. (2015). Prevalence of 7 sexually transmitted organisms by multiplex real-time PCR in fallopian tube specimens collected from Saudi women with and without ectopic pregnancy. BMC Infect. Dis. 15, 569. doi: 10.1186/s12879-015-1313-1
Babon, J. J., Varghese, L. N., Nicola, N. A. (2014). Inhibition of IL-6 family cytokines by SOCS3. Semin. Immunol. 26 (1), 13–19. doi: 10.1016/j.smim.2013.12.004
Baedyananda, F., Sasivimolrattana, T., Chaiwongkot, A., Varadarajan, S., Bhattarakosol, P. (2022). Role of HPV16 E1 in cervical carcinogenesis. Front. Cell Infect. Microbiol. 12, 955847. doi: 10.3389/fcimb.2022.955847
Batteiger, B. E., Tu, W., Ofner, S., Van Der Pol, B., Stothard, D. R., Orr, D. P., et al. (2010). Repeated chlamydia trachomatis genital infections in adolescent women. J. Infect. Dis. 201 (1), 42–51. doi: 10.1086/648734
Batteiger, B. E., Xu, F., Johnson, R. E., Rekart, M. L. (2010). Protective immunity to chlamydia trachomatis genital infection: evidence from human studies. J. Infect. Dis. 201 Suppl 2 (S2), S178–S189. doi: 10.1086/652400
Baumann, H., Wong, G. G. (1989). Hepatocyte-stimulating factor III shares structural and functional identity with leukemia-inhibitory factor. J. Immunol. 143 (4), 1163–1167.
Bautista, C. T., Hollingsworth, B. P., Sanchez, J. L. (2018). Repeat chlamydia diagnoses increase the hazard of pelvic inflammatory disease among US army women: A retrospective cohort analysis. Sex Transm Dis. 45 (11), 770–773. doi: 10.1097/OLQ.0000000000000878
Bay, J. M., Patterson, B. K., Teng, N. N. (2011). Leukemia inhibitory factor downregulates human papillomavirus-16 oncogene expression and inhibits the proliferation of cervical carcinoma cells. Infect. Dis. Obstet Gynecol 2011, 463081. doi: 10.1155/2011/463081
Beatty, W. L., Morrison, R. P., Byrne, G. I. (1994). Persistent chlamydiae: from cell culture to a paradigm for chlamydial pathogenesis. Microbiol. Rev. 58 (4), 686–699. doi: 10.1128/mr.58.4.686-699.1994
Brinsden, P. R., Alam, V., de Moustier, B., Engrand, P. (2009). Recombinant human leukemia inhibitory factor does not improve implantation and pregnancy outcomes after assisted reproductive techniques in women with recurrent unexplained implantation failure. Fertil Steril 91 (4 Suppl), 1445–1447. doi: 10.1016/j.fertnstert.2008.06.047
Brunham, R. C., Gottlieb, S. L., Paavonen, J. (2015). Pelvic inflammatory disease. N Engl. J. Med. 372 (21), 2039–2048. doi: 10.1056/NEJMra1411426
Brunham, R. C., Maclean, I. W., Binns, B., Peeling, R. W. (1985). Chlamydia trachomatis: its role in tubal infertility. J. Infect. Dis. 152 (6), 1275–1282. doi: 10.1093/infdis/152.6.1275
Carvalho, A. V., Reinaud, P., Forde, N., Healey, G. D., Eozenou, C., Giraud-Delville, C., et al. (2014). SOCS genes expression during physiological and perturbed implantation in bovine endometrium. Reproduction. 148 (6), 545–557. doi: 10.1530/rep-14-0214
CDC (2020) Sexually transmitted disease surveillance 2020. Available at: https://www.cdc.gov/std/statistics/2020/data.zip.
Charnock-Jones, D. S., Sharkey, A. M., Fenwick, P., Smith, S. K. (1994). Leukaemia inhibitory factor mRNA concentration peaks in human endometrium at the time of implantation and the blastocyst contains mRNA for the receptor at this time. J. Reprod. Fertil 101 (2), 421–426. doi: 10.1530/jrf.0.1010421
Chen, J. R., Cheng, J. G., Shatzer, T., Sewell, L., Hernandez, L., Stewart, C. L. (2000). Leukemia inhibitory factor can substitute for nidatory estrogen and is essential to inducing a receptive uterus for implantation but is not essential for subsequent embryogenesis. Endocrinology. 141 (12), 4365–4372. doi: 10.1210/endo.141.12.7855
Cheng, J. G., Chen, J. R., Hernandez, L., Alvord, W. G., Stewart, C. L. (2001). Dual control of LIF expression and LIF receptor function regulate Stat3 activation at the onset of uterine receptivity and embryo implantation. Proc. Natl. Acad. Sci. U.S.A. 98 (15), 8680–8685. doi: 10.1073/pnas.151180898
Cheng, J. G., Patterson, P. H. (1997). LIF is an autocrine factor for sympathetic neurons. Mol. Cell Neurosci. 9 (5-6), 372–380. doi: 10.1006/mcne.1997.0635
Cheng, J. G., Rodriguez, C. I., Stewart, C. L. (2002). Control of uterine receptivity and embryo implantation by steroid hormone regulation of LIF production and LIF receptor activity: towards a molecular understanding of "the window of implantation". Rev. Endocr. Metab. Disord. 3 (2), 119–126. doi: 10.1023/A:1015402811650
Cheng, J., Rosario, G., Cohen, T. V., Hu, J., Stewart, C. L. (2017). Tissue-specific ablation of the LIF receptor in the murine uterine epithelium results in implantation failure. Endocrinology. 158 (6), 1916–1928. doi: 10.1210/en.2017-00103
Chen, H., Wen, Y., Li, Z. (2019). Clear victory for chlamydia: The subversion of host innate immunity. Front. Microbiol. 10, 1412. doi: 10.3389/fmicb.2019.01412
Cherpes, T. L., Rice, P. A., Sweet, R. L. (2011). Pelvic inflammatory disease. Infect. Dis. Obstet Gynecol 2011, 714289. doi: 10.1155/2011/714289
Christianson, J., Oxford, J. T., Jorcyk, C. L. (2021). Emerging perspectives on leukemia inhibitory factor and its receptor in cancer. Front. Oncol. 11, 693724. doi: 10.3389/fonc.2021.693724
Chung, B. M., Kang, H. C., Han, S. Y., Heo, H. S., Lee, J. J., Jeon, J., et al. (2006). Jak2 and Tyk2 are necessary for lineage-specific differentiation, but not for the maintenance of self-renewal of mouse embryonic stem cells. Biochem. Biophys. Res. Commun. 351 (3), 682–688. doi: 10.1016/j.bbrc.2006.10.081
Clendenen, T. V., Lundin, E., Zeleniuch-Jacquotte, A., Koenig, K. L., Berrino, F., Lukanova, A., et al. (2011). Circulating inflammation markers and risk of epithelial ovarian cancer. Cancer Epidemiol. Biomarkers Prev. 20 (5), 799–810. doi: 10.1158/1055-9965.EPI-10-1180
Control ECfDPa. (2022). Facts about chlamydia. Available at: https://www.ecdc.europa.eu/en/chlamydia/facts#:~:text=At%20least%2070%25%20of%20genital,asymptomatic%20infections%20is%20not%20uncommon (Accessed May 17, 2022).
Cullinan, E. B., Abbondanzo, S. J., Anderson, P. S., Pollard, J. W., Lessey, B. A., Stewart, C. L. (1996). Leukemia inhibitory factor (LIF) and LIF receptor expression in human endometrium suggests a potential autocrine/paracrine function in regulating embryo implantation. Proc. Natl. Acad. Sci. U.S.A 93 (7), 3115–3120. doi: 10.1073/pnas.93.7.3115
Denizot, Y., Besse, A., Raher, S., Nachat, R., Trimoreau, F., Praloran, V., et al. (1999). Interleukin-4 (IL-4), but not IL-10, regulates the synthesis of IL-6, IL-8 and leukemia inhibitory factor by human bone marrow stromal cells. Biochim. Biophys. Acta 1449 (1), 83–92. doi: 10.1016/S0167-4889(98)00177-3
Dharamshi, J. E., Tamarit, D., Eme, L., Stairs, C.W., Martijn, J., Homa, F., et al. (2020). Marine sediments illuminate chlamydiae diversity and evolution. Curr. Biol. 30 (6), 1032–1048.e1037. doi: 10.1016/j.cub.2020.02.016
Ding, T., Song, H., Wang, X., Khatua, A., Paria, B. C. (2008). Leukemia inhibitory factor ligand-receptor signaling is important for uterine receptivity and implantation in golden hamsters (Mesocricetus auratus). Reproduction. 135 (1), 41–53. doi: 10.1530/REP-07-0013
Elwell, C., Mirrashidi, K., Engel, J. (2016). Chlamydia cell biology and pathogenesis. Nat. Rev. Microbiol. 14 (6), 385–400. doi: 10.1038/nrmicro.2016.30
Eming, S. A., Wynn, T. A., Martin, P. (2017). Inflammation and metabolism in tissue repair and regeneration. Science. 356 (6342), 1026–1030. doi: 10.1126/science.aam7928
Feng, R., Qin, X., Li, Q., Olugbenga Adeniran, S., Huang, F., Li, Y., et al. (2022). Progesterone regulates inflammation and receptivity of cells via the NF-κB and LIF/STAT3 pathways. Theriogenology. 186, 50–59. doi: 10.1016/j.theriogenology.2022.04.005
Ferrara, N., Winer, J., Henzel, W. J. (1992). Pituitary follicular cells secrete an inhibitor of aortic endothelial cell growth: identification as leukemia inhibitory factor. Proc. Natl. Acad. Sci. U.S.A 89 (2), 698–702. doi: 10.1073/pnas.89.2.698
Fischer, A., Rudel, T. (2018). Subversion of cell-autonomous host defense by chlamydia infection. Curr. Top. Microbiol. Immunol. 412, 81–106. doi: 10.1007/82_2016_13
Foronjy, R. F., Dabo, A. J., Cummins, N., Geraghty, P. (2014). Leukemia inhibitory factor protects the lung during respiratory syncytial viral infection. BMC Immunol. 15, 41. doi: 10.1186/s12865-014-0041-4
Gearing, D. P., Gough, N. M., King, J. A., Hilton, D. J., Nicola, N. A., Simpson, R. J., et al. (1987). Molecular cloning and expression of cDNA encoding a murine myeloid leukaemia inhibitory factor (LIF). EMBO J. 6 (13), 3995–4002. doi: 10.1002/j.1460-2075.1987.tb02742.x
Geisler, W. M. (2010). Duration of untreated, uncomplicated chlamydia trachomatis genital infection and factors associated with chlamydia resolution: a review of human studies. J. Infect. Dis. 201 Suppl 2, S104–S113. doi: 10.1086/652402
Ghosh, A., Syed, S. M., Tanwar, P. S. (2017). In vivo genetic cell lineage tracing reveals that oviductal secretory cells self-renew and give rise to ciliated cells. Development. 144 (17), 3031–3041. doi: 10.1242/dev.149989
Giess, R., Tanasescu, I., Steck, T., Sendtner, M. (1999). Leukaemia inhibitory factor gene mutations in infertile women. Mol. Hum. Reprod. 5 (6), 581–586. doi: 10.1093/molehr/5.6.581
Giudice, L. C. (2020). Multidimensional transcriptomic mapping of human endometrium at single-cell resolution. Nat. Med. 26 (10), 1513–1514. doi: 10.1038/s41591-020-1075-1
Gough, N. M., Gearing, D. P., King, J. A., Willson, T. A., Hilton, D. J., Nicola, N. A., et al. (1988). Molecular cloning and expression of the human homologue of the murine gene encoding myeloid leukemia-inhibitory factor. Proc. Natl. Acad. Sci. U.S.A 85 (8), 2623–2627. doi: 10.1073/pnas.85.8.2623
Guo, T., Gupta, A., Yu, J., Granados, J. Z., Gandhi, A. Y., Evers, B. M., et al. (2021). LIFR-α-dependent adipocyte signaling in obesity limits adipose expansion contributing to fatty liver disease. iScience. 24 (3), 102227. doi: 10.1016/j.isci.2021.102227
Gupta, K., Harrison, S. A., Davis, N. A., Culp, M. L., Hand, S. C., Simpson, T., et al. (2021). Prevalence of chlamydia trachomatis infection in young women and associated predictors. Sex Transm Dis. 48 (8), 529–535. doi: 10.1097/OLQ.0000000000001372
Gurtner, G. C., Werner, S., Barrandon, Y., Longaker, M. T. (2008). Wound repair and regeneration. Nature. 453 (7193), 314–321. doi: 10.1038/nature07039
Hackstadt, T., Fischer, E. R., Scidmore, M. A., Rockey, D. D., Heinzen, R. A. (1997). Origins and functions of the chlamydial inclusion. Trends Microbiol. 5 (7), 288–293. doi: 10.1016/S0966-842X(97)01061-5
Halder, S., Parte, S., Kshirsagar, P., Muniyan, S., Nair, H. B., Batra, S. K., et al. (2022). The pleiotropic role, functions and targeted therapies of LIF/LIFR axis in cancer: Old spectacles with new insights. Biochim. Biophys. Acta (BBA) - Rev. Cancer 1877 (4), 188737. doi: 10.1016/j.bbcan.2022.188737
Hambartsoumian, E. (1998). Endometrial leukemia inhibitory factor (LIF) as a possible cause of unexplained infertility and multiple failures of implantation. Am. J. Reprod. Immunol. 39 (2), 137–143. doi: 10.1111/j.1600-0897.1998.tb00345.x
Hamilton, J. A., Waring, P. M., Filonzi, E. L. (1993). Induction of leukemia inhibitory factor in human synovial fibroblasts by IL-1 and tumor necrosis factor-alpha. J. Immunol. 150 (4), 1496–1502.
Harper, M. J. (1992). The implantation window. Baillieres Clin. Obstet Gynaecol 6 (2), 351–371. doi: 10.1016/S0950-3552(05)80092-6
Helble, J. D., Starnbach, M. N. (2021). T Cell responses to chlamydia. Pathog. Dis. 79 (4), ftab014. doi: 10.1093/femspd/ftab014
Hess, S., Rheinheimer, C., Tidow, F., Bartling, G., Kaps, C., Lauber, J., et al. (2001). The reprogrammed host: Chlamydia trachomatis-induced up-regulation of glycoprotein 130 cytokines, transcription factors, and antiapoptotic genes. Arthritis Rheumatol. 44 (10), 2392–2401. doi: 10.1002/1529-0131(200110)44:10<2392::AID-ART404>3.0.CO;2-I
Hillis, S. D., Owens, L. M., Marchbanks, P. A., Amsterdam, L. F., Mac Kenzie, W. R. (1997). Recurrent chlamydial infections increase the risks of hospitalization for ectopic pregnancy and pelvic inflammatory disease. Am. J. Obstet Gynecol 176 (1 Pt 1), 103–107. doi: 10.1016/S0002-9378(97)80020-8
Hogan, R. J., Mathews, S. A., Mukhopadhyay, S., Summersgill, J. T., Timms, P. (2004). Chlamydial persistence: beyond the biphasic paradigm. Infect. Immun. 72 (4), 1843–1855. doi: 10.1128/IAI.72.4.1843-1855.2004
Hosenfeld, C. B., Workowski, K. A., Berman, S., Zaidi, A., Dyson, J., Mosure, D., et al. (2009). Repeat infection with chlamydia and gonorrhea among females: a systematic review of the literature. Sex Transm Dis. 36 (8), 478–489. doi: 10.1097/OLQ.0b013e3181a2a933
Hosseininasab-Nodoushan, S. A., Ghazvini, K., Jamialahmadi, T., Keikha, M., Sahebkar, A. (2021). Association of chlamydia and mycoplasma infections with susceptibility to ovarian cancer: A systematic review and meta-analysis. Semin. Cancer Biol. doi: 10.1016/j.semcancer.2021.07.016
Hou, S., Yue, L., Xu, R., Zhu, C., Shan, S., Wang, H., et al. (2018). Chlamydia muridarum plasmid induces mouse oviduct pathology by promoting chlamydial survival and ascending infection and triggering host inflammation. Eur. J. Dermatol. 28 (5), 628–636. doi: 10.1684/ejd.2018.3399
Huai, P., Li, F., Chu, T., Liu, D., Liu, J., Zhang, F. (2020). Prevalence of genital chlamydia trachomatis infection in the general population: a meta-analysis. BMC Infect. Dis. 20 (1), 589. doi: 10.1186/s12879-020-05307-w
Hu, W., Feng, Z., Teresky, A. K., Levine, A. J. (2007). p53 regulates maternal reproduction through LIF. Nature. 450 (7170), 721–724. doi: 10.1038/nature05993
Human Protein Atlas. (2022). Available at: http://www.proteinatlas.org (accessed on August 2022).
Ishimi, Y., Abe, E., Jin, C. H., Miyaura, C., Hong, M. H., Oshida, M., et al. (1992). Leukemia inhibitory factor/differentiation-stimulating factor (LIF/D-factor): regulation of its production and possible roles in bone metabolism. J. Cell Physiol. 152 (1), 71–78. doi: 10.1002/jcp.1041520110
Ji, Y. F., Chen, L. Y., Xu, K. H., Yao, J. F., Shi, Y. F. (2009). Locally elevated leukemia inhibitory factor in the inflamed fallopian tube resembles that found in tubal pregnancy. Fertil Steril 91 (6), 2308–2314. doi: 10.1016/j.fertnstert.2008.01.110
Jones, S. A., Jenkins, B. J. (2018). Recent insights into targeting the IL-6 cytokine family in inflammatory diseases and cancer. Nat. Rev. Immunol. 18 (12), 773–789. doi: 10.1038/s41577-018-0066-7
Jorgensen, M. M., de la Puente, P. (2022). Leukemia inhibitory factor: An important cytokine in pathologies and cancer. Biomolecules. 12 (2), 217. doi: 10.3390/biom12020217
Karaer, A., Cigremis, Y., Celik, E., Urhan Gonullu, R. (2014). Prokineticin 1 and leukemia inhibitory factor mRNA expression in the endometrium of women with idiopathic recurrent pregnancy loss. Fertil Steril 102 (4), 1091–1095.e1091. doi: 10.1016/j.fertnstert.2014.07.010
Karin, M., Clevers, H. (2016). Reparative inflammation takes charge of tissue regeneration. Nature. 529 (7586), 307–315. doi: 10.1038/nature17039
Kelly, K. A. (2003). Cellular immunity and chlamydia genital infection: induction, recruitment, and effector mechanisms. Int. Rev. Immunol. 22 (1), 3–41. doi: 10.1080/08830180305229
Keltz, M. D., Attar, E., Buradagunta, S., Olive, D. L., Kliman, H. J., Arici, A. (1996). Modulation of leukemia inhibitory factor gene expression and protein biosynthesis in the human fallopian tube. Am. J. Obstet Gynecol 175 (6), 1611–1619. doi: 10.1016/S0002-9378(96)70114-X
Kershaw, N. J., Murphy, J. M., Liau, N. P., Varghese, L. N., Laktyushin, A., Whitlock, E. L., et al. (2013). SOCS3 binds specific receptor-JAK complexes to control cytokine signaling by direct kinase inhibition. Nat. Struct. Mol. Biol. 20 (4), 469–476. doi: 10.1038/nsmb.2519
Kessler, M., Hoffmann, K., Fritsche, K., Brinkmann, V., Mollenkopf, H. J., Thieck, O., et al. (2019). Chronic chlamydia infection in human organoids increases stemness and promotes age-dependent CpG methylation. Nat. Commun. 10 (1), 1194. doi: 10.1038/s41467-019-09144-7
Kimber, S. J. (2005). Leukaemia inhibitory factor in implantation and uterine biology. Reproduction. 130 (2), 131–145. doi: 10.1530/rep.1.00304
Kim, S. M., Kim, J. S. (2017). A review of mechanisms of implantation. Dev. Reprod. 21 (4), 351–359. doi: 10.12717/DR.2017.21.4.351
Knight, D. (2001). Leukaemia inhibitory factor (LIF): a cytokine of emerging importance in chronic airway inflammation. Pulm Pharmacol. Ther. 14 (3), 169–176. doi: 10.1006/pupt.2001.0282
Krishnan, T., Winship, A., Sonderegger, S., Menkhorst, E., Horne, A.W., Brown, J., et al. (2013). The role of leukemia inhibitory factor in tubal ectopic pregnancy. Placenta. 34 (11), 1014–1019. doi: 10.1016/j.placenta.2013.09.003
Kurzrock, R., Estrov, Z., Wetzler, M., Gutterman, J. U., Talpaz, M. (1991). LIF: not just a leukemia inhibitory factor. Endocr. Rev. 12 (3), 208–217. doi: 10.1210/edrv-12-3-208
Laird, S. M., Tuckerman, E. M., Dalton, C. F., Dunphy, B. C., Li, T. C., Zhang, X. (1997). The production of leukaemia inhibitory factor by human endometrium: presence in uterine flushings and production by cells in culture. Hum. Reprod. 12 (3), 569–574. doi: 10.1093/humrep/12.3.569
Laitinen, K., Laurila, A. L., Leinonen, M., Saikku, P. (1996). Reactivation of chlamydia pneumoniae infection in mice by cortisone treatment. Infect. Immun. 64 (4), 1488–1490. doi: 10.1128/iai.64.4.1488-1490.1996
Large, M. J., DeMayo, F. J. (2012). The regulation of embryo implantation and endometrial decidualization by progesterone receptor signaling. Mol. Cell Endocrinol. 358 (2), 155–165. doi: 10.1016/j.mce.2011.07.027
Liang, X. H., Deng, W. B., Li, M., Zhao, Z. A., Wang, T. S., Feng, X. H., et al. (2014). Egr1 protein acts downstream of estrogen-leukemia inhibitory factor (LIF)-STAT3 pathway and plays a role during implantation through targeting Wnt4. J. Biol. Chem. 289 (34), 23534–23545. doi: 10.1074/jbc.M114.588897
Liao, C. I., Francoeur, A. A., Kapp, D. S., Caesar, M. A. P., Huh, W. K., Chan, J. K., et al. (2022). Trends in human papillomavirus-associated cancers, demographic characteristics, and vaccinations in 2001-2017. JAMA Netw. Open 5 (3), e222530. doi: 10.1001/jamanetworkopen.2022.2530
Li, P., Li, Q., Biswas, N., Xin, H., Diemer, T., Liu, L., et al. (2022). LIF, a mitogen for choroidal endothelial cells, protects the choriocapillaris: implications for prevention of geographic atrophy. EMBO Mol. Med. 14 (1), e14511. doi: 10.15252/emmm.202114511
Linker, R. A., Kruse, N., Israel, S., Wei, T., Seubert, S., Hombach, A., et al. (2008). Leukemia inhibitory factor deficiency modulates the immune response and limits autoimmune demyelination: a new role for neurotrophic cytokines in neuroinflammation. J. Immunol. 180 (4), 2204–2213. doi: 10.4049/jimmunol.180.4.2204
Li, M., Sendtner, M., Smith, A. (1995). Essential function of LIF receptor in motor neurons. Nature. 378 (6558), 724–727. doi: 10.1038/378724a0
Lyons, R. A., Saridogan, E., Djahanbakhch, O. (2006). The reproductive significance of human fallopian tube cilia. Hum. Reprod. Update. 12 (4), 363–372. doi: 10.1093/humupd/dml012
Margioula-Siarkou, C., Prapas, Y., Petousis, S., Milias, S., Ravanos, K., Kalogiannidis, I., et al. (2016). LIF and LIF−R expression in the endometrium of fertile and infertile women: A prospective observational case−control study. Mol. Med. Rep. 13 (6), 4721–4728. doi: 10.3892/mmr.2016.5142
Margioula-Siarkou, C., Prapas, Y., Petousis, S., Milias, S., Ravanos, K., Dagklis, T., et al. (2017). LIF endometrial expression is impaired in women with unexplained infertility while LIF-r expression in all infertility sub-groups. Cytokine. 96, 166–172. doi: 10.1016/j.cyto.2017.04.009
Mathieu, M. E., Saucourt, C., Mournetas, V., Gauthereau, X., Thézé, N., Praloran, V., et al. (2012). LIF-dependent signaling: new pieces in the Lego. Stem Cell Rev. Rep. 8 (1), 1–15. doi: 10.1007/s12015-011-9261-7
Matsushita, K., Itoh, S., Ikeda, S., Yamamoto, Y., Yamauchi, Y., Hayashi, M. (2014). LIF/STAT3/SOCS3 signaling pathway in murine bone marrow stromal cells suppresses osteoblast differentiation. J. Cell Biochem. 115 (7), 1262–1268. doi: 10.1002/jcb.24777
Mazzoli, S., Bani, D., Salvi, A., Ramacciotti, I., Romeo, C., Bani, T. (2000). In vivo evidence of chlamydia trachomatis miniature reticulary bodies (MRB) as persistence markers in patients with chronic chlamydial prostatitis. Proc. Eur. Soc. Chlamydia Res. 4, 40.
McCormack, W. M., Alpert, S., McComb, D. E., Nichols, R. L., Semine, D. Z., Zinner, S. H. (1979). Fifteen-month follow-up study of women infected with chlamydia trachomatis. N Engl. J. Med. 300 (3), 123–125. doi: 10.1056/NEJM197901183000305
Metcalfe, S. M. (2011). LIF in the regulation of T-cell fate and as a potential therapeutic. Genes Immun. 12 (3), 157–168. doi: 10.1038/gene.2011.9
Molano, M., Meijer, C. J., Weiderpass, E., Arslan, A., Posso, H., Franceschi, S., et al. (2005). The natural course of chlamydia trachomatis infection in asymptomatic Colombian women: a 5-year follow-up study. J. Infect. Dis. 191 (6), 907–916. doi: 10.1086/428287
Morré, S. A., van den Brule, A. J., Rozendaal, L., Boeke, A. J., Voorhorst, F. J., de Blok, S., et al. (2002). The natural course of asymptomatic chlamydia trachomatis infections: 45% clearance and no development of clinical PID after one-year follow-up. Int. J. STD AIDS 13 Suppl 2, 12–18. doi: 10.1258/095646202762226092
Mostowy, S., Shenoy, A. R. (2015). The cytoskeleton in cell-autonomous immunity: structural determinants of host defence. Nat. Rev. Immunol. 15 (9), 559–573. doi: 10.1038/nri3877
Moulder, J. W. (1991). Interaction of chlamydiae and host cells in vitro. Microbiol. Rev. 55 (1), 143–190. doi: 10.1128/mr.55.1.143-190.1991
Murphy, M., Reid, K., Hilton, D. J., Bartlett, P. F. (1991). Generation of sensory neurons is stimulated by leukemia inhibitory factor. Proc. Natl. Acad. Sci. U.S.A 88 (8), 3498–3501. doi: 0.1073/pnas.88.8.3498
Ng, K. Y. B., Cheong, Y. (2019). Hydrosalpinx - salpingostomy, salpingectomy or tubal occlusion. Best Pract. Res. Clin. Obstet Gynaecol 59, 41–47. doi: 10.1016/j.bpobgyn.2019.01.011
Nicola, N. A., Babon, J. J. (2015). Leukemia inhibitory factor (LIF). Cytokine Growth factor Rev. 26 (5), 533–544. doi: 10.1016/j.cytogfr.2015.07.001
Novotný, Z., Krízan, J., Síma, R., Síma, P., Uher, P., Zech, N., et al. (2009). Leukaemia inhibitory factor (LIF) gene mutations in women diagnosed with unexplained infertility and endometriosis have a negative impact on the IVF outcome. a pilot study. Folia Biol. (Praha) 55 (3), 92–97.
Okada, H., Tsuzuki, T., Murata, H. (2018). Decidualization of the human endometrium. Reprod. Med. Biol. 17 (3), 220–227. doi: 10.1002/rmb2.12088
Olivennes, F., Lédée-Bataille, N., Samama, M., Kadoch, J., Taupin, J. L., Dubanchet, S., et al. (2003). Assessment of leukemia inhibitory factor levels by uterine flushing at the time of egg retrieval does not adversely affect pregnancy rates with in vitro fertilization. Fertil Steril 79 (4), 900–904. doi: 10.1016/S0015-0282(02)04949-X
Ota, K., Quint, P., Weivoda, M. M., Ruan, M., Pederson, L., Westendorf, J. J., et al. (2013). Transforming growth factor beta 1 induces CXCL16 and leukemia inhibitory factor expression in osteoclasts to modulate migration of osteoblast progenitors. Bone. 57 (1), 68–75. doi: 10.1016/j.bone.2013.07.023
Pan, W., Yu, C., Hsuchou, H., Zhang, Y., Kastin, A. J. (2008). Neuroinflammation facilitates LIF entry into brain: role of TNF. Am. J. Physiol. Cell Physiol. 294 (6), C1436–C1442. doi: 10.1152/ajpcell.00489.2007
Patterson, B. K., Tjernlund, A., Andersson, J. (2002). Endogenous inhibitors of HIV: potent anti-HIV activity of leukemia inhibitory factor. Curr. Mol. Med. 2 (8), 713–722. doi: 10.2174/1566524023361817
Peres, L. C., Townsend, M. K., Birmann, B. M., Conejo-Garcia, J. R., Kim, Y., Kubzansky, L. D., et al. (2021). Circulating biomarkers of inflammation and ovarian cancer risk in the nurses' health studies. Cancer Epidemiol. Biomarkers Prev. 30 (4), 710–718. doi: 10.1158/1055-9965.EPI-20-1390
Perrier d'Hauterive, S., Charlet-Renard, C., Berndt, S., et al. (2004). Human chorionic gonadotropin and growth factors at the embryonic-endometrial interface control leukemia inhibitory factor (LIF) and interleukin 6 (IL-6) secretion by human endometrial epithelium. Hum. Reprod. 19 (11), 2633–2643. doi: 10.1093/humrep/deh450
Peters, J., Hess, S., Endlich, K., et al. (2005). Silencing or permanent activation: host-cell responses in models of persistent chlamydia pneumoniae infection. Cell Microbiol. 7 (8), 1099–1108. doi: 10.1111/j.1462-5822.2005.00534.x
Phillips, D. M., Swenson, C. E., Schachter, J. (1984). Ultrastructure of chlamydia trachomatis infection of the mouse oviduct. J. Ultrast Res. 88 (3), 244–256. doi: 10.1016/S0022-5320(84)90122-9
Piccinni, M. P., Beloni, L., Livi, C., Maggi, E., Scarselli, G., Romagnani, S. (1998). Defective production of both leukemia inhibitory factor and type 2 T-helper cytokines by decidual T cells in unexplained recurrent abortions. Nat. Med. 4 (9), 1020–1024. doi: 10.1038/2006
Polim, A. A., Abdullah, N., Hatta, M., Natzir, R., Soebijanto, S., Hutomo, C., et al. (2022). Positive correlation of oestradiol level on trigger day with the secretion level of endometrial kisspeptin and leukaemia inhibitory factor in the mid-luteal stimulated cycle. J. Hum. Reprod. Sci. 15 (1), 72–77. doi: 10.4103/jhrs.jhrs_120_21
Price, M. J., Ades, A. E., Soldan, K., Welton, N. J., Macleod, J., Simms, I., et al. (2016). The natural history of chlamydia trachomatis infection in women: a multi-parameter evidence synthesis. Health Technol. Assess 20 (22), 1–250. doi: 10.3310/hta20220
Quinton, L. J., Mizgerd, J. P., Hilliard, K. L., Jones, M. R., Kwon, C. Y., Allen, E. (2012). Leukemia inhibitory factor signaling is required for lung protection during pneumonia. J. Immunol. 188 (12), 6300–6308. doi: 10.4049/jimmunol.1200256
Ramathal, C. Y., Bagchi, I. C., Taylor, R. N., Bagchi, M. K. (2010). Endometrial decidualization: of mice and men. Semin. Reprod. Med. 28 (1), 17–26. doi: 10.1055/s-0029-1242989
Reed, B. G., Carr, B. R. (2000). “The normal menstrual cycle and the control of ovulation,” in Endotext. Eds. Feingold, K. R., Anawalt, B., Boyce, A., et al (South Dartmouth (MA: MDText.com, Inc).
Refaat, B., Ashshi, A. M., Batwa, S. A., Ahmad, J., Idris, S., Kutbi, S. Y., et al. (2016). The prevalence of chlamydia trachomatis and mycoplasma genitalium tubal infections and their effects on the expression of IL-6 and leukaemia inhibitory factor in fallopian tubes with and without an ectopic pregnancy. Innate Immun. 22 (7), 534–545. doi: 10.1177/1753425916662326
Rodig, S. J., Meraz, M. A., White, J. M., Lampe, P. A., Riley, J. K., Arthur, C. D., et al. (1998). Disruption of the Jak1 gene demonstrates obligatory and nonredundant roles of the jaks in cytokine-induced biologic responses. Cell. 93 (3), 373–383. doi: 10.1016/S0092-8674(00)81166-6
Rosario, G. X., Hondo, E., Jeong, J. W., Mutalif, R., Ye, X., Yee, L. X., et al. (2014). The LIF-mediated molecular signature regulating murine embryo implantation. Biol. Reprod. 91 (3), 66. doi: 10.1095/biolreprod.114.118513
Rosario, G. X., Stewart, C. L. (2016). The multifaceted actions of leukaemia inhibitory factor in mediating uterine receptivity and embryo implantation. Am. J. Reprod. Immunol. 75 (3), 246–255. doi: 10.1111/aji.12474
Rose-John, S. (2018). Interleukin-6 family cytokines. Cold Spring Harbor Perspect. Biol. 10 (2), a028415. doi: 10.1101/cshperspect.a028415
Ruan, M., Pederson, L., Bradley, E. W., Bamberger, A. M., Oursler, M. J. (2010). Transforming growth factor-{beta} coordinately induces suppressor of cytokine signaling 3 and leukemia inhibitory factor to suppress osteoclast apoptosis. Endocrinology. 151 (4), 1713–1722. doi: 10.1210/en.2009-0813
Salleh, N., Giribabu, N. (2014). Leukemia inhibitory factor: roles in embryo implantation and in nonhormonal contraception. Sci. World J. 2014, 201514. doi: 10.1155/2014/201514
Schuchardt, L., Rupp, J. (2018). Chlamydia trachomatis as the cause of infectious infertility: Acute, repetitive or persistent long-term infection? Curr. Top. Microbiol. Immunol. 412, 159–182. doi: 10.1007/82_2016_15
Sims, N. A. (2020). The JAK1/STAT3/SOCS3 axis in bone development, physiology, and pathology. Exp. Mol. Med. 52 (8), 1185–1197. doi: 10.1038/s12276-020-0445-6
Smith, A. G., Heath, J. K., Donaldson, D. D., Wong, G. G., Moreau, J., Stahl, M., et al. (1988). Inhibition of pluripotential embryonic stem cell differentiation by purified polypeptides. Nature. 336 (6200), 688–690. doi: 10.1038/336688a0
Stewart, C. L., Kaspar, P., Brunet, L. J., Bhatt, H., Gadi, I., Köntgen, F., et al. (1992). Blastocyst implantation depends on maternal expression of leukaemia inhibitory factor. Nature. 359 (6390), 76–79. doi: 10.1038/359076a0
Takahashi, Y., Takahashi, M., Carpino, N., Jou, S. T., Chao, J. R., Tanaka, S., et al. (2008). Leukemia inhibitory factor regulates trophoblast giant cell differentiation via janus kinase 1-signal transducer and activator of transcription 3-suppressor of cytokine signaling 3 pathway. Mol. Endocrinol. 22 (7), 1673–1681. doi: 10.1210/me.2008-0058
Tjernlund, A., Barqasho, B., Nowak, P., Kinloch, S., Thorborn, D., Perrin, L., et al. (2006). Early induction of leukemia inhibitor factor (LIF) in acute HIV-1 infection. Aids. 20 (1), 11–19. doi: 10.1097/01.aids.0000198082.16960.94
Tomida, M., Yamamoto-Yamaguchi, Y., Hozumi, M. (1984). Purification of a factor inducing differentiation of mouse myeloid leukemic M1 cells from conditioned medium of mouse fibroblast L929 cells. J. Biol. Chem. 259 (17), 10978–10982. doi: 10.1016/S0021-9258(18)90609-5
Trent, M., Bass, D., Ness, R. B., Haggerty, C. (2011). Subsequent STI, and reproductive health outcomes: findings from the PID evaluation and clinical health (PEACH) study. Sex Transm Dis. 38 (9), 879–881. doi: 10.1097/OLQ.0b013e31821f918c
Vagnini, L. D., Renzi, A., Petersen, B., Canas, M., Petersen, C. G., Mauri, A. L., et al. (2019). Association between estrogen receptor 1 (ESR1) and leukemia inhibitory factor (LIF) polymorphisms can help in the prediction of recurrent implantation failure. Fertil Steril 111 (3), 527–534. doi: 10.1016/j.fertnstert.2018.11.016
van Eijk, M. J., Mandelbaum, J., Salat-Baroux, J., Belaisch-Allart, J., Plachot, M., Junca, A. M., et al. (1996). Expression of leukaemia inhibitory factor receptor subunits LIFR beta and gp130 in human oocytes and preimplantation embryos. Mol. Hum. Reprod. 2 (5), 355–360. doi: 10.1093/molehr/2.5.355
Vasquez, G., Winston, R. M., Brosens, I. A. (1983). Tubal mucosa and ectopic pregnancy. Br. J. Obstet Gynaecol 90 (5), 468–474. doi: 10.1111/j.1471-0528.1983.tb08946.x
Vogiagis, D., Marsh, M. M., Fry, R. C., Salamonsen, L. A. (1996). Leukaemia inhibitory factor in human endometrium throughout the menstrual cycle. J. Endocrinol. 148 (1), 95–102. doi: 10.1677/joe.0.1480095
Wang, W., Vilella, F., Alama, P., Moreno, I., Mignardi, M., Isakova, A., et al. (2020). Single-cell transcriptomic atlas of the human endometrium during the menstrual cycle. Nat. Med. 26 (10), 1644–1653. doi: 10.1038/s41591-020-1040-z
Wesselingh, S. L., Gough, N. M., Finlay-Jones, J. J., McDonald, P. J. (1990). Detection of cytokine mRNA in astrocyte cultures using the polymerase chain reaction. Lympho Res. 9 (2), 177–185.
Wetzler, M., Estrov, Z., Talpaz, M., Kim, K. J., Alphonso, M., Srinivasan, R., et al. (1994). Leukemia inhibitory factor in long-term adherent layer cultures: increased levels of bioactive protein in leukemia and modulation by IL-4, IL-1 beta, and TNF-alpha. Cancer Res. 54 (7), 1837–1842.
Wetzler, M., Talpaz, M., Lowe, D. G., Baiocchi, G., Gutterman, J. U., Kurzrock, R. (1991). Constitutive expression of leukemia inhibitory factor RNA by human bone marrow stromal cells and modulation by IL-1, TNF-alpha, and TGF-beta. Exp. Hematol. 19 (5), 347–351.
WHO (2021) Sexually transmitted infections (STIs). Available at: https://www.who.int/news-room/fact-sheets/detail/sexually-transmitted-infections-(stis) (Accessed May 12, 2022).
Williams, R. L., Hilton, D. J., Pease, S., Willson, T. A., Stewart, C. L., Gearing, D. P., et al. (1988). Myeloid leukaemia inhibitory factor maintains the developmental potential of embryonic stem cells. Nature. 336 (6200), 684–687. doi: 10.1038/336684a0
Willson, T. A., Metcalf, D., Gough, N. M. (1992). Cross-species comparison of the sequence of the leukaemia inhibitory factor gene and its protein. Eur. J. Biochem. 204 (1), 21–30. doi: 10.1111/j.1432-1033.1992.tb16601.x
Wu, M., Yin, Y., Zhao, M., Hu, L., Chen, Q. (2013). The low expression of leukemia inhibitory factor in endometrium: possible relevant to unexplained infertility with multiple implantation failures. Cytokine. 62 (2), 334–339. doi: 10.1016/j.cyto.2013.03.002
Xiang, W., Yu, N., Lei, A., Li, X., Tan, S., Huang, L., et al. (2021). Insights into host cell cytokines in chlamydia infection. Front. Immunol. 12, 639834. doi: 10.3389/fimmu.2021.639834
Xu, S. X., Gray-Owen, S. D. (2021). Gonococcal pelvic inflammatory disease: Placing mechanistic insights into the context of clinical and epidemiological observations. J. Infect. Dis. 224 (12 Suppl 2), S56–s63. doi: 10.1093/infdis/jiab227
Xu, B., Sun, X., Li, L., Wu, L., Zhang, A., Feng, Y. (2012). Pinopodes, leukemia inhibitory factor, integrin-β3, and mucin-1 expression in the peri-implantation endometrium of women with unexplained recurrent pregnancy loss. Fertil Steril 98 (2), 389–395. doi: 10.1016/j.fertnstert.2012.04.032
Yang, X. (2003). Role of cytokines in chlamydia trachomatis protective immunity and immunopathology. Curr. Pharm. Des. 9 (1), 67–73. doi: 10.2174/1381612033392486
Yang, Y. S., Kuo, C. C., Chen, W. J. (1983). Reactivation of chlamydia trachomatis lung infection in mice by cortisone. Infect. Immun. 39 (2), 655–658. doi: 10.1128/iai.39.2.655-658.1983
Yoo, I., Chae, S., Han, J., Lee, S., Kim, H. J., Ka, H. (2019). Leukemia inhibitory factor and its receptor: expression and regulation in the porcine endometrium throughout the estrous cycle and pregnancy. Asian-Australas J. Anim. Sci. 32 (2), 192–200. doi: 10.5713/ajas.18.0429
Zeng, R., Tong, C., Xiong, X. (2022). The molecular basis and therapeutic potential of leukemia inhibitory factor in cancer cachexia. Cancers (Basel) 14 (12), 2955. doi: 10.3390/cancers14122955
Zhao, Y., He, D., Zeng, H., Luo, J., Yang, S., Chen, J., et al. (2021). Expression and significance of miR-30d-5p and SOCS1 in patients with recurrent implantation failure during implantation window. Reprod. Biol. Endocrinol. 19 (1), 138. doi: 10.1186/s12958-021-00820-2
Zhu, M., Oishi, K., Lee, S. C., Patterson, P. H. (2001). Studies using leukemia inhibitory factor (LIF) knockout mice and a LIF adenoviral vector demonstrate a key anti-inflammatory role for this cytokine in cutaneous inflammation. J. Immunol. 166 (3), 2049–2054. doi: 10.4049/jimmunol.166.3.2049
Keywords: Chlamydia, leukemia inhibitory factor (LIF), ectopic pregnancy, infertility, cervical cancer, ovarian cancer, pelvic inflammatory disease (PID)
Citation: Wang J and Wang K (2022) New insights into Chlamydia pathogenesis: Role of leukemia inhibitory factor. Front. Cell. Infect. Microbiol. 12:1029178. doi: 10.3389/fcimb.2022.1029178
Received: 26 August 2022; Accepted: 27 September 2022;
Published: 18 October 2022.
Edited by:
Guangming Zhong, The University of Texas Health Science Center at San Antonio, United StatesReviewed by:
Kevin Hybiske, University of Washington, United StatesCopyright © 2022 Wang and Wang. This is an open-access article distributed under the terms of the Creative Commons Attribution License (CC BY). The use, distribution or reproduction in other forums is permitted, provided the original author(s) and the copyright owner(s) are credited and that the original publication in this journal is cited, in accordance with accepted academic practice. No use, distribution or reproduction is permitted which does not comply with these terms.
*Correspondence: Jun Wang, anVuLndhbmdAZGFsLmNh
Disclaimer: All claims expressed in this article are solely those of the authors and do not necessarily represent those of their affiliated organizations, or those of the publisher, the editors and the reviewers. Any product that may be evaluated in this article or claim that may be made by its manufacturer is not guaranteed or endorsed by the publisher.
Research integrity at Frontiers
Learn more about the work of our research integrity team to safeguard the quality of each article we publish.