- 1Department of Clinical Laboratory, Fourth People’s Hospital of Nanning, Nanning, Guangxi, China
- 2Affiliated Infectious Disease Hospital of Nanning, Guangxi Medical University, Nanning, Guangxi, China
Objectives: This study aimed to investigate the clinical and biochemical profiles of patients with imported malaria infection between 1 January 2011 and 30 April 2022 and admitted to the Fourth People’s Hospital of Nanning.
Methods: This cohort study enrolled 170 patients with conformed imported malaria infection. The clinical and biochemical profiles of these participants were analyzed with malaria parasite clearance, and signs and symptoms related to malaria disappearance were defined as the primary outcome. A multivariable logistic regression model was used to evaluate the odds ratios (ORs) with 95% confidence intervals (CIs) for cerebral malaria. The Cox model was used to estimate the hazard ratios (HRs) with 95% CIs for parasite clearance.
Results: Adenosine deaminase and parasitemia were found to be independent risk factors for severe malaria in patients with imported malaria (OR = 1.0088, 95% CI: 1.0010–1.0167, p = 0.0272 and OR = 2.0700, 95% CI: 1.2584–3.4050, p = 0.0042, respectively). A 0.5–standard deviation (SD) increase of variation for urea (HR = 0.6714, 95% CI: 0.4911–0.9180), a 0.5-SD increase of variation for creatinine (HR = 0.4566, 95% CI: 0.2762–0.7548), a 0.25-SD increase of variation for albumin (HR = 0.4947, 95% CI: 0.3197–0.7653), a 0.25-SD increase of variation for hydroxybutyrate dehydrogenase (HR = 0.6129, 95% CI: 0.3995–0.9402), and a 1.0-SD increase of variation for ferritin (HR = 0.5887, 95% CI: 0.3799–0.9125) were associated with a higher risk for increased parasite clearance duration than a low-level change.
Conclusions: Aspartate aminotransferase, urea, creatinine, albumin, hydroxybutyrate dehydrogenase, and ferritin are useful biochemical indicators in routine clinical practice to evaluate prognosis for imported malaria.
Introduction
Malaria, caused by Plasmodium and transmitted by mosquitoes (Phillips et al., 2017; Walter and John, 2022), is an epidemic infectious disease that predominantly occurs in tropical and subtropical regions (Chaves et al., 2020; van Dorp et al., 2020; Zhou et al., 2020). Globally, there are over 4.72 billion confirmed cases with approximately 11 million deaths over the past two decades, as reported by the World Health Organization (WHO, 2021). China has succeeded in controlling indigenous malaria, and no autochthonous cases have been reported since 2017 (Burki, 2021; Zhou, 2021). However, with the increasing globalization and interconnectedness, an increasing number of people travel to and from malaria-endemic regions. Therefore, the incidence of imported malaria has been gradually increasing and has become a major public health challenge (Feng et al., 2014; Wang et al., 2018; Feng et al., 2020; Li et al., 2021; Liu et al., 2021).
Human malaria, one of the most lethal infectious diseases responsible for high morbidity and mortality (Dos-Santos et al., 2014; Phillips et al., 2017; Ashley et al., 2018; Chaves et al., 2020), is characterized by circulatory inflammatory events and impairment of the microvascular endothelium (Burté et al., 2013; Morrell, 2014; O’Sullivan and O’Donnell, 2018; Erice and Kain, 2019; Knackstedt et al., 2019; Yeo et al., 2019; Mita Mendoza et al., 2020; Neida, 2020; Vasquez et al., 2021). The pathogenesis of malaria is multifactorial and driven predominantly by parasite biomass and modulation by host innate and adaptive immune responses (Yeo et al., 2008; Barber et al., 2015; Barber et al., 2017; Kho et al., 2018; Cao and Vickers, 2021; Dassah et al., 2022). During infection, red blood cells are invaded by merozoites, which grow and develop by schizogony, generating more merozoites during the intraerythrocytic developmental cycle. These merozoites undergo multiple successive rounds of cell division and are released into the blood stream with their metabolites. This triggers the host complex immune response and disrupts the intracellular environment. Immune cell activation triggers host defense, which limits pathogen spread at the site of infection (Vaid, 2010; Miller et al., 2013; Morrell et al., 2014; Niang et al., 2014; Phillips et al., 2017; Mather and Ke, 2018). Host defense to malaria includes a cascade of pathways that are modulated by hundreds of immune modulatory molecules (Miller et al., 2013; Niang et al., 2014; Gramaglia et al., 2017; Phillips et al., 2017). Many of these molecules promote vasculature occlusion for inducing inflammation and endothelial cell activation and induce acute-phase responses to prevent the spread of malaria. Routine laboratory results indicate liver and kidney dysfunction (Nieman et al., 2009; Nantakomol et al., 2011; Mita-Mendoza et al., 2013; Mangal et al., 2017; Cheaveau et al., 2019; Dinkar et al., 2020; Khrapunov et al., 2021; Tona Lutete et al., 2021; Megabiaw et al., 2022), and in some cases, abnormal myocardial enzyme is reported (Herr et al., 2011; Dinkar et al., 2020; Kaiser et al., 2020).
This study aimed to investigate the biochemical characteristics of 170 patients with imported malaria treated with curative intent in the Fourth People’s Hospital of Nanning using a population-based retrospective cohort.
Materials and methods
Study design and participants
In this retrospective study, data were collected and analyzed prospectively from patients with confirmed malaria infection and admitted to the Fourth People’s Hospital of Nanning between 1 January 2011 and 31 May 2022. Only patients with imported malaria were enrolled in this study. Inclusion criteria were as follows: (і) patients with diagnosis of imported malaria, (ii) patients admitted to the hospital within 3 h of onset, and (iii) patients hospitalized with up to three consecutive blood smears being negative with each measurement spaced 24 h, or the disappearance of all clinical evidence of malaria for a minimum of 3 days with no persisting clinical signs and symptoms related to the disease. Imported malaria cases were defined as follows: patients received a diagnosis of malaria, patients had a travel history to a malaria-endemic area, and the onset of symptoms in patients is less than 1 month after returning to China.
Blood samples for microscopic examination and clinical chemistry measurements were collected at the same time, and each collection for microscope examination and clinical chemistry measurement was spaced 24–48 h. Blood samples were centrifuged before routine biochemical tests; biochemical assays were performed using an automatic biochemical analyzer (Roche Modular PPE and Hitachi LABOSPECT 008 AS). Laboratory diagnosis of malaria infection was based on the microscopic examination of Giemsa-stained thin and thick blood smears with confirmed malaria parasites. Additionally, the species of the infecting parasite was confirmed using nested PCR assays.
This study was conducted in accordance with the guidelines of the Declaration of Helsinki and approved by the ethics committee of the Fourth People’s Hospital of Nanning (Nos. [2019]39, [2020]24, and [2021]23).
Data collection
Patient information, including demographics, epidemiological data, comorbidities, symptoms, laboratory results, and treatment measures, was collected from their electronic medical records. A power analysis was conducted that revealed that a cohort of 170 patients was necessary to achieve more than 80% power based on the primary outcome measure with minimum reference interval.
Statistical analysis
Normally distributed continuous variables are presented as means and standard deviations (SDs), and non-normally distributed variables are presented as medians and interquartile ranges. Categorical variables are presented as counts (%). The means of continuous variables were compared using independent-samples t-tests for normally distributed data, and for other variables, the Mann–Whitney U-test was used. Survival analysis was performed using the Kaplan–Meier survival and Cox model analyses. Statistical analyses were conducted using GraphPad Prism software (version 8.0) and MedCalc statistical software (version 15.8). For all statistical tests, p< 0.05 was considered to indicate statistical significance.
Results
Baseline clinical characteristics
Figure 1 presents a flow diagram of the inclusion and exclusion criteria of this study. Demographic, comorbidity, prior malaria history, and treatment variables for patients with imported malaria are summarize in Table 1. During the study period, 170 patients with confirmed imported malaria were tested for all indices of serum biochemistry (Table 2). Of the participants, 95% were male individuals, and 56 participants (33%) were diagnosed with severe malaria. The criteria for severe malaria are presented in Table 3. The results of the analysis of epidemiological data showed that more than 90% cases had recent history of working in an endemic area, primarily in Africa such as Ethiopia, Ghana, and Angola, and more than 70% cases had history of malaria. However, more than 98% cases had history of consuming prophylactic medication with antimalarials.
Laboratory findings
The results of this study suggest that the majority of biochemical outcomes were within the reference range. Moreover, the levels of lactate dehydrogenase, ferritin, adenosine deaminase, and hydroxybutyrate dehydrogenase were observed to be higher than the reference ranges, whereas a slight decrease in the serum calcium ion concentration was observed. Furthermore, ferritin, a unique biochemical parameter, was elevated in all patients with imported malaria (Table 2).
Factors associated with severe malaria in patients with imported malaria
As compared with patients with uncomplicated malaria, the patients with severe malaria had significantly different levels of adenosine deaminase (odds ratio [OR] = 1.0088, 95% CI: 1.0010–1.0167, p = 0.0272), creatine kinase isotype MB (OR = 1.0393, 95% CI: 0.9993–1.0809, p = 0.0500), direct bilirubin (OR = 1.0278, 95% CI: 1.0028–1.0535, p = 0.0293), α-hydroxybutyrate dehydrogenase (OR = 1.0039, 95% CI: 1.0009–1.0069, p = 0.0104), lactate dehydrogenase (OR = 1.0035, 95% CI: 1.0012–1.0058, p = 0.0029), urea (OR = 1.1121, 95% CI: 1.0002–1.2366, p = 0.0495), albumin (OR = 0.9379, 95% CI: 0.8887–0.9897, p = 0.01954), and blood calcium (OR = 0.0697, 95% CI: 0.0074–0.6563, p = 0.01999). A multivariable logistic regression model identified adenosine deaminase (OR = 1.0115, 95% CI: 1.0030–1.0201, p = 0.0080) and parasitemia (OR = 2.0700, 95% CI: 1.2584–3.4050, p = 0.0042) as factors associated with severe malaria. The fitting equation is expressed as Equation 1 below:
where ADA is blood adenosine deaminase (U/L), Parasitemia is blood parasitemia (/µl), and df is the degrees of freedom.
Factors associated with duration of parasite clearance
Analysis of parasite clearance risk was performed by using the Cox proportional hazards model (Figure 2). Patients with a high level of urea needed longer duration for parasite clearance than those with a normal level of urea (median duration: 8 days, 95% CI: 6–14 vs. 6 days, 95% CI: 5–8; p< 0.0001). Thus, urea levels were associated with risk for longer parasite clearance duration (hazard ratio [HR] = 0.5108, 95% CI: 0.3533–0.7386). Patients with a high level of creatinine needed a longer duration for parasite clearance (median duration: 7 days, 95% CI: 5–8 vs. 6 days, 95% CI: 4–7; p = 0.0063) than that of patients who had a normal level of creatinine. Thus, creatinine levels were associated with the risk of a longer length of parasite clearance (HR = 0.6930, 95% CI: 0.4943–0.9718). As compared with patients with normal levels of creatine kinase isoenzyme-MB, patients with higher levels of creatine kinase isoenzyme-MB had a longer duration of parasite clearance (median duration: 8 days, 95% CI: 5–9 vs. 6 days, 95% CI: 5–8; p = 0.0185). Thus, creatine kinase levels were associated with the risk of a longer length of parasite clearance (HR = 0.6639, 95% CI: 0.4543–0.9702). Patients with a higher ratio of aspartate aminotransferase to alanine aminotransferase had a longer duration of parasite clearance (median duration: 8 days, 95% CI: 6–9 vs. 7 days, 95% CI: 5–8; p = 0.0050) than that of patients with imported malaria with a normal level of the ratio of aspartate aminotransferase to alanine aminotransferase. Thus, the ratio of aspartate aminotransferase to alanine aminotransferase was associated with the risk of a longer length of parasite clearance (HR = 0.6016, 95% CI: 0.4055–0.8925). However, patients with a low level of albumin had a longer length of parasite clearance (median duration: 7 days, 95% CI: 5–9 vs. 6 days, 95% CI: 5–8; p = 0.0053) than those with a normal level of albumin. Thus, albumin levels were linked with a higher risk of longer duration of parasite clearance (HR = 0.6850, 95% CI: 0.4985–0.9314). Based on the Cox proportional model, patients with high parasitemia were more likely to have a longer length of parasite clearance (median duration: 7 days, 95% CI: 7–8 vs. 4 days, 95% CI: 4–5; p = 0.0430) than those with low parasitemia. Thus, parasitemia was associated with a higher risk of longer duration of parasite clearance (HR = 1.364, 95% CI: 0.9557–1.947).
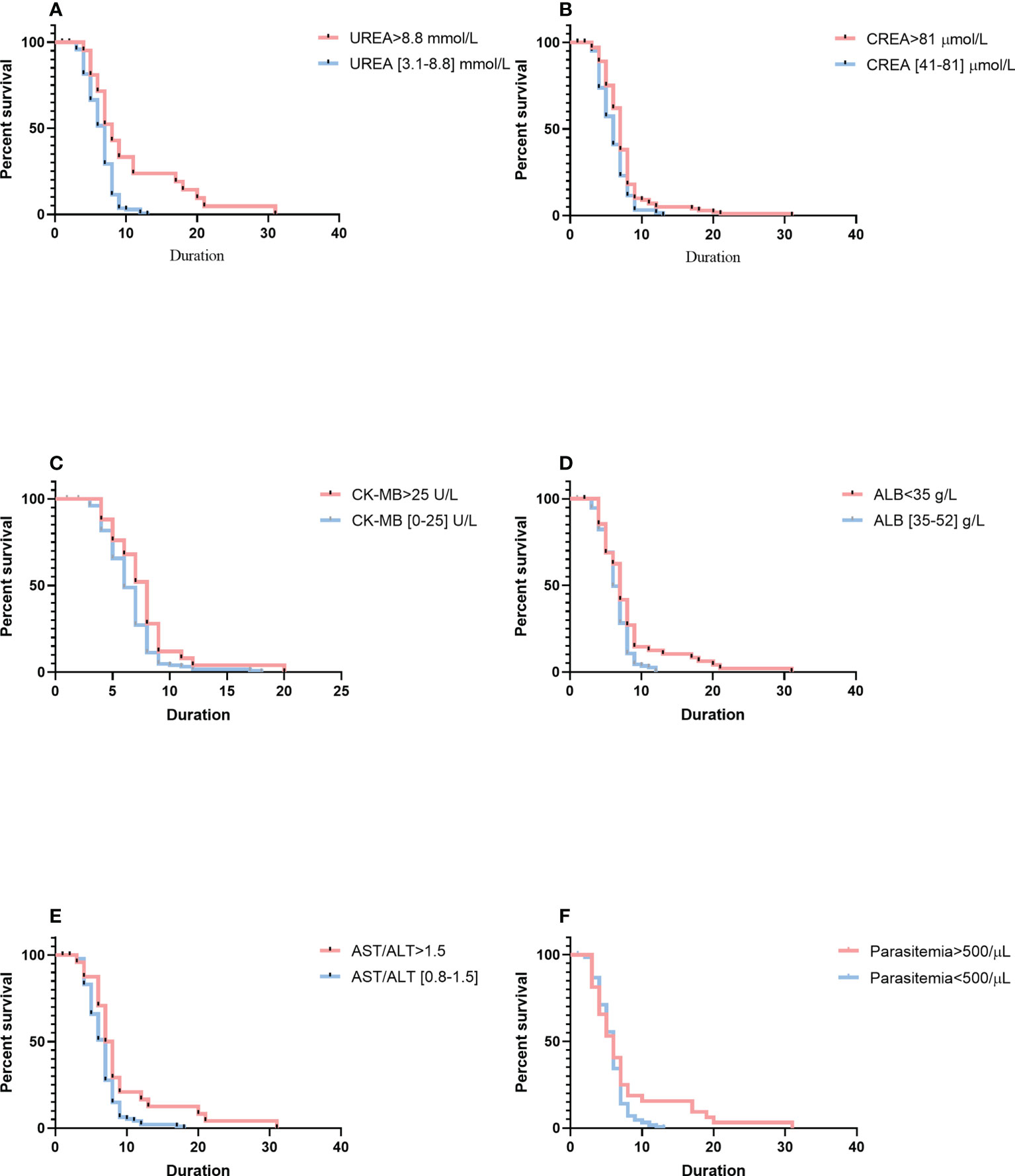
Figure 2 Comparison of survival curves using the log-rank test. (A) Serum higher levels of UREA versus within the normal reference range. (B) of CREA versus within the normal reference range. (C) Serum higher levels of CK-MB versus within the normal reference range. (D) Serum lower levels of ALB versus within the normal reference range. (E) Serum higher levels of AST/ALT versus within the normal reference range. (F) Higher parasitemia versus lower parasitemia. Blue lines show patients with a shorter duration of parasite clearance; red lines show patients with a longer duration of parasite clearance. Duration is indicated in days. CREA, creatinine; CK-MB, creatine kinase isoenzyme-MB; ALB, albumin; AST/ALT, aspartate aminotransferase-to-alanine aminotransferase ratio.
More analyses using variation of biochemical indices had been conducted to examine the relationships of blood chemistry with the parasite’s clearance (Figure 3). Cox proportional hazard multiple regression analysis suggested that patients with a high level of variation for urea had a longer duration of parasite clearance than those with a low level of variation for urea (median duration: 7 days, 95% CI: 5–9 vs. 6 days, 95% CI: 5–8; p = 0.0182), and variation for urea was associated with a higher risk of a longer length of parasite clearance (HR = 0.6714, 95% CI: 0.4911–0.9180). Patients with a high level of variation for creatinine had a longer duration of parasite clearance (median duration: 8 days, 95% CI: 5–12 vs. 7 days, 95% CI: 5–8; p = 0.0444) than patients with a low level of variation for creatinine, and variation for creatinine was associated with a higher risk of a longer length of parasite clearance (HR = 0.4566, 95% CI: 0.2762–0.7548). As compared with patients with a low-level variation of albumin, patients with a high-level variation of albumin had a longer duration of parasite clearance (median duration: 7 days, 95% CI: 5–9 vs. 6 days, 95% CI: 5–8; p = 0.0285), and albumin levels were associated with a higher risk of a longer length of parasite clearance (HR = 0.4947, 95% CI: 0.3197–0.7653). Patients with a high-level variation of α-hydroxybutyrate dehydrogenase had a longer duration of parasite clearance (median duration: 7 days, 95% CI: 5–8 vs. 6 days, 95% CI: 5–7; p = 0.0357) than patients with imported malaria with a low level of variation for α-hydroxybutyrate dehydrogenase, and variation for α-hydroxybutyrate dehydrogenase levels was associated with a higher risk of a longer length of parasite clearance (HR = 0.6129, 95% CI: 0.3995–0.9402). Patients with a high level of variation for ferritin had a longer length of parasite clearance (median duration: 7 days, 95% CI: 6–8 vs. 6 days, 95% CI: 4–8; p = 0.0211) as compared with those who had a low level of variation for ferritin, and variation for the ferritin level was associated with a higher risk of longer duration of parasite clearance (HR = 0.5887, 95% CI: 0.3799–0.9125). A multivariable model based on the variation and the fitting equation was expressed as Equation 2 below:
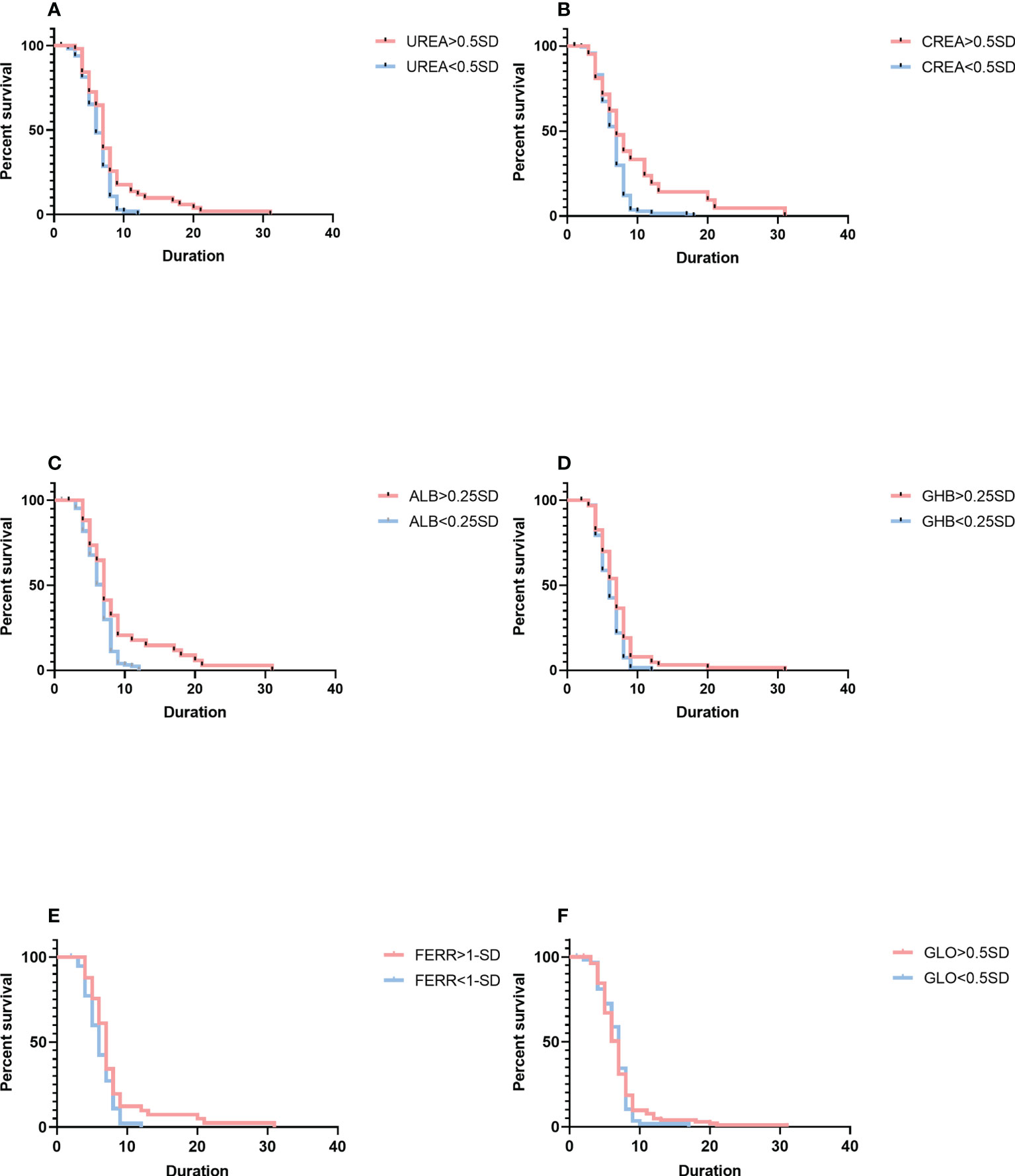
Figure 3 Comparison of survival curves using the log-rank test. (A) The variation for UREA ≥ 0.5 SD versus< 0.5 SD. (B) The change for CREA ≥ 0.5 SD versus< 0.5 SD. (C) The change for ALB ≥ 0.25 SD versus< 0.25 SD. (D) The change for GHB ≥ 0.25 SD versus< 0.25 SD. (E) The change for FERR ≥ 1.0 SD versus< 1.0 SD. (F) The variation for GLO ≥ 0.5 SD versus< 0.5 SD. Blue lines show patients with a shorter duration of parasite clearance; red lines show patients with a longer duration of parasite clearance. Duration is indicated in days. SD, standard deviation; GHB, γ-hydroxybutyrate dehydrogenase; FERR, ferritin; GLO, globin.
where ΔALB is the variation of albumin (g/L), ΔCREA is the variation of creatinine (µmol/L), ΔFERR is the variation of ferritin (ng/ml), ΔGHB is the variation of α-hydroxybutyrate dehydrogenase (U/L), Par is parasitemia (µl), and ΔUREA is the variation of urea (mmol/L).
Discussion
In this study, biochemical parameters in imported malaria cases were evaluated, and most of these indicators were found to be associated with the severity of the malaria infection. Consistent with previous studies of different biochemical indicators, we found that most parameters in imported malaria are of high abundance, although the opposite is true for a few measurements, and these measures are associated with parasite clearance. Some biochemical parameters were not associated with parasite clearance; however, a high-level variation of these indices is significantly correlated with a longer duration of parasite clearance. Furthermore, a 0.25–1.0 SD increase or decrease of these indicators is associated with a higher risk of a longer length of parasite clearance.
Ferritin, an acute-phase protein in inflammatory responses of various kinds (Damron et al., 2016; Leligdowicz et al., 2021), is the main intracellular iron storage protein in mammals (Li et al., 2012; Truman-Rosentsvit et al., 2018; Fang et al., 2021), plants (Li et al., 2012; Truman-Rosentsvit et al., 2018), and bacteria (Li et al., 2012; Bauckman and Mysorekar, 2016; Truman-Rosentsvit et al., 2018). Moreover, the serum levels of ferritin are strongly associated with infective and non-infective inflammatory conditions (Gabay, 1999; Burté et al., 2013). This study indicated that serum ferritin concentrations did not correlate significantly with parasite clearance, although they were elevated in all patients. Conventionally, serum ferritin has been used as the key iron marker as it reflects the total body iron storage. However, ferritin is also an acute phase protein, so in an acute inflammatory situation, such as during malaria, serum ferritin levels may not accurately reflect body iron stores only. However, a 1-SD increase of variation for serum ferritin levels was associated with a higher risk of duration for parasite clearance, a 1-SD variation of which associates very strongly with malaria parasitemia. Our observations for ferritin are consistent with the findings of previous studies that positively correlated elevated ferritin with the increased risk of malaria parasitemia (Wessells et al., 2017).
In contrast to an increase in the liver enzyme that was observed in previous studies, we observed no elevation of liver enzyme in this study (Viriyavejakul et al., 2014; Rocha et al., 2015; Dinkar et al., 2020; Megabiaw et al., 2022); however, abnormalities in the ratio of aspartate aminotransferase to alanine aminotransferase were observed in 43% of the patients, and higher ratios of aspartate aminotransferase to alanine aminotransferase were significantly associated with malaria parasitemia. The liver enzyme profiles are distinct from prior studies (Viriyavejakul et al., 2014; Dinkar et al., 2020; Megabiaw et al., 2022). Based on the findings on the clinical history of prior prophylactic administration, we speculate that adherence to prophylactic treatment with antimalarial medications before and after infection has a hepatoprotective role. Furthermore, serum albumin values and a 0.25-SD increase of albumin were associated with a significant increased risk of malaria parasitemia. Thus, serum albumin could be a better prognostic risk factor or biomarker for imported malaria than liver enzyme, as abnormal liver function impaired synthesis and acute kidney injury, resulting in an increased albuminuria (Viriyavejakul et al., 2014; Doltario et al., 2016; Leopold et al., 2019; Dinkar et al., 2020).
Multiple studies have shown that malaria is closely associated with hemolysis-induced endothelial activation, resulting in acute kidney injury (Sriboonvorakul et al., 2018; Brown et al., 2020; Kaur et al., 2020; Ouma et al., 2020; Katsoulis et al., 2021). Consistent with previous studies, an increase in the level of creatinine and urea in serum was observed in the case of malaria (Sriboonvorakul et al., 2018; Brown et al., 2020; Kaur et al., 2020; Ouma et al., 2020; Katsoulis et al., 2021). Here, we have described that the plasma levels of renal injury biomarkers correlated with the duration of parasite clearance. Furthermore, in agreement with previous studies of myocardial injury in malaria patients (Dinkar et al., 2020; Watts et al., 2020), we found that most cardiac enzymes in human normal and lactate dehydrogenase increased in malarial infection, with no significant association between lactate dehydrogenase and the duration of parasite clearance. However, the serum levels of creatine kinase isoenzyme-MB, but not creatine kinase isoenzyme, showed a significant association with the duration of parasite clearance. Furthermore, this study provides the first description of a statistically significant association between hydroxybutyrate dehydrogenase and the duration of parasite clearance.
Data availability statement
The original contributions presented in the study are included in the article/Supplementary Material. Further inquiries can be directed to the corresponding author.
Ethics statement
This study was conducted according to the guidelines of the Declaration of Helsinki and approved by The Ethics Committee of The Fourth People’s Hospital of Nanning (No. [2019]39, [2020]24, [2021]23). Written informed consent for participation was not required for this study in accordance with the national legislation and the institutional requirements.
Author contributions
Conceptualization, XH. Clinical validation, JL, LL and XT. Investigation, YL and XL. Data curation and original draft preparation, DB. Review, XL. All authors contributed to the article and approved the submitted version.
Funding
This study was supported by the Key Research and Development Program of Nanning Municipal Science and Technology Department (grant number 20193008), the Guangxi Zhuang Region Health Department (grant number Z20200979), and the Fourth People’s Hospital of Nanning Youth Foundation (grant number NNSY2021010).
Acknowledgments
The authors would like to thank the staff of the Nanning Center for Disease Control and Prevention and the Guangxi Center for Disease Control and Prevention.
Conflict of interest
The authors declare that the research was conducted in the absence of any commercial or financial relationships that could be construed as a potential conflict of interest.
Publisher’s note
All claims expressed in this article are solely those of the authors and do not necessarily represent those of their affiliated organizations, or those of the publisher, the editors and the reviewers. Any product that may be evaluated in this article, or claim that may be made by its manufacturer, is not guaranteed or endorsed by the publisher.
Supplementary material
The Supplementary Material for this article can be found online at: https://www.frontiersin.org/articles/10.3389/fcimb.2022.1008430/full#supplementary-material.
References
Ashley, E. A., Pyae Phyo, A., Woodrow, C. J. (2018). Malaria. Lancet 391, 1608–1621. doi: 10.1016/S0140-6736(18)30324-6
Barber, B. E., Grigg, M. J., William, T., Piera, K. A., Boyle, M. J., Yeo, T. W., et al. (2017). Effects of aging on parasite biomass, inflammation, endothelial activation, microvascular dysfunction and disease severity in plasmodium knowlesi and plasmodium falciparum malaria. J. Infect. Dis. 215, 1908–1917. doi: 10.1093/infdis/jix193
Barber, B. E., William, T., Grigg, M. J., Parameswaran, U., Piera, K. A., Price, R. N., et al. (2015). Parasite biomass-related inflammation, endothelial activation, microvascular dysfunction and disease severity in vivax malaria. PloS Pathog. 11, e1004558. doi: 10.1371/journal.ppat.1004558
Bauckman, K. A., Mysorekar, I. U. (2016). Ferritinophagy drives uropathogenic escherichia coli persistence in bladder epithelial cells. Autophagy 12, 850–863. doi: 10.1080/15548627.2016.1160176
Brown, D. D., Solomon, S., Lerner, D., Del Rio, M. (2020). Malaria and acute kidney injury. Pediatr. Nephrol. 35, 603–608. doi: 10.1007/s00467-018-4191-0
Burki, T. (2021). Triumph in China as it is certified malaria-free by WHO. Lancet Infect. Dis. 21, 1220–1221. doi: 10.1016/S1473-3099(21)00491-6
Burté, F., Brown, B. J., Orimadegun, A. E., Ajetunmobi, W. A., Afolabi, N. K., Akinkunmi, F., et al. (2013). Circulatory hepcidin is associated with the anti-inflammatory response but not with iron or anemic status in childhood malaria. Blood 121, 3016–3022. doi: 10.1182/blood-2012-10
Cao, H., Vickers, M. A. (2021). Oxidative stress, malaria, sickle cell disease, and innate immunity. Trends Immunol. 42, 849–851. doi: 10.1016/j.it.2021.08.008
Chaves, L. S. M., Fry, J., Malik, A., Geschke, A., Sallum, M. A. M., Lenzen, M. (2020). Global consumption and international trade in deforestation-associated commodities could influence malaria risk. Nat. Commun. 12(1):3713. doi: 10.1038/s41467-020-14954-1
Cheaveau, J., Marasinghe, D., Akakpo, S., Deardon, R., Naugler, C., Chin, A., et al. (2019). The impact of malaria on liver enzymes: A retrospective cohort study, (2010–2017). Open Forum Infect. Dis. Open Forum Infect. 6, ofz234. doi: 10.1093/ofid/ofz234
Damron, F. H., Oglesby-Sherrouse, A. G., Wilks, A., Barbier, M. (2016). Dual-seq transcriptomics reveals the battle for iron during pseudomonas aeruginosa acute murine pneumonia. Sci. Rep-Uk. Sci. Rep. 6, 39172. doi: 10.1038/srep39172
Dassah, S., Adu, B., Tiendrebeogo, R. W., Singh, S. K., Arthur, F. K. N., Sirima, S. B., et al. (2022). GMZ2 vaccine-induced antibody responses, naturally acquired immunity and the incidence of malaria in burkinabe children. Front. Immunol. 13. doi: 10.3389/fimmu.2022.899223
Dinkar, A., Singh, J., Singh, S. (2020). Acute myocardial infarction associated with severe plasmodium vivax malaria. J. Vector Borne Dis. 57, 193–196. doi: 10.4103/0972-9062.310872
Doltario, A. B., Menon, L. J. B., Bollela, V. R., Martinez, R., de Almeida E Araújo, D. C., da Fonseca, B. A. L., et al. (2016). Malaria and other febrile diseases among travellers: the experience of a reference centre located outside the Brazilian Amazon region. Malar. J. 15, 294. doi: 10.1186/s12936-016-1347-x
Dos-Santos, J. C. K., Angerami, R. N., Castiñeiras, C. M. S., Lopes, S. C. P., Albrecht, L., Garcia, M. T., et al. (2014). Imported malaria in a non-endemic area: the experience of the university of campinas hospital in the Brazilian southeast. Malar. J. 13, 280. doi: 10.1186/1475-2875-13-280
Erice, C., Kain, K. C. (2019). New insights into microvascular injury to inform enhanced diagnostics and therapeutics for severe malaria. Virulence 10, 1034–1046. doi: 10.1080/21505594.2019.1696621
Fang, Y., Chen, X., Tan, Q., Zhou, H., Xu, J., Gu, Q. (2021). Inhibiting ferroptosis through disrupting the NCOA4–FTH1 interaction: A new mechanism of action. ACS Cent. Sci. 7, 980–989. doi: 10.1021/acscentsci.0c01592
Feng, J., Tu, H., Zhang, L., Xia, Z., Zhou, S. (2020). Imported malaria cases - China 2012–2018. China CDC Wkly. 2, 277–283. doi: 10.46234/ccdcw2020.072
Feng, J., Yan, H., Feng, X. Y., Zhang, L., Li, M., Xia, Z. G., et al. (2014). Imported malaria in China 2012. Emerg. Infect. Dis. 20, 1778–1780. doi: 10.3201/eid2010.140595
Gabay, C. (1999). Acute-phase protein and other systemic responses to inflammation. New Engl. J. Med. 340, 448–455. doi: 10.1056/NEJM199902113400607
Gramaglia, I., Velez, J., Combes, V., Grau, G. E., Wree, M., van der Heyde, H. C. (2017). Platelets activate a pathogenic response to blood-stage plasmodium infection but not a protective immune response. Blood 129, 1669–1679. doi: 10.1182/blood-2016-08
Herr, J., Mehrfar, P., Schmiedel, S., Wichmann, D., Brattig, N. W., Burchard, G. D., et al. (2011). Reduced cardiac output in imported plasmodium falciparum malaria. Malar. J. 10, 160. doi: 10.1186/1475-2875-10-160
Kaiser, A., Heiss, K., Mueller, A. K., Fimmers, R., Matthes, J., Njuguna, J. T. (2020). Inhibition of EIF-5A prevents apoptosis in human cardiomyocytes after malaria infection. Amino Acids 52, 693–710. doi: 10.1007/s00726-020-02843-2
Katsoulis, O., Georgiadou, A., Cunnington, A. J. (2021). Immunopathology of acute kidney injury in severe malaria. Front. Immunol. 12. doi: 10.3389/fimmu.2021.651739
Kaur, C., Pramanik, A., Kumari, K., Mandage, R., Dinda, A. K., Sankar, J., et al. (2020). Renal detection of plasmodium falciparum, plasmodium vivax and plasmodium knowlesi in malaria associated acute kidney injury: a retrospective case–control study. BMC Res. Notes 13, 37. doi: 10.1186/s13104-020-4900-1
Kho, S., Barber, B. E., Johar, E., Andries, B., Poespoprodjo, J. R., Kenangalem, E., et al. (2018). Platelets kill circulating parasites of all major plasmodium species in human malaria. Blood 132, 1332–1344. doi: 10.1182/blood-2018-05-849307
Khrapunov, S., Waterman, A., Persaud, R., Chang, E. P. (2021). Structure, function, and thermodynamics of lactate dehydrogenases from humans and the malaria parasite p. falciparum. Biochemistry 60, 3582–3595. doi: 10.1021/acs.biochem.1c00470
Knackstedt, S. L., Georgiadou, A., Apel, F., Abu-Abed, U., Moxon, C. A., Cunnington, A. J., et al. (2019). Neutrophil extracellular traps drive inflammatory pathogenesis in malaria. Sci. Immunol. 4 (40), eaaw0336. doi: 10.1126/sciimmunol.aaw0336
Leligdowicz, A., Conroy, A. L., Hawkes, M., Richard-Greenblatt, M., Zhong, K., Opoka, R. O., et al. (2021). Risk-stratification of febrile African children at risk of sepsis using sTREM-1 as basis for a rapid triage test. Nat. Commun. 12, 6832. doi: 10.1038/s41467-021-27215-6
Leopold, S. J., Ghose, A., Allman, E. L., Kingston, H. W. F., Hossain, A., Dutta, A. K., et al. (2019). Identifying the components of acidosis in patients with severe plasmodium falciparum malaria using metabolomics. J. Infect. Dis. 219, 1766–1776. doi: 10.1093/infdis/jiy727
Li, Y., Fang, Y., Xue, J., Yang, L., Liu, Q., Shi, W., et al. (2021). Surveillance of malaria vectors –18 sites, 12 PLADs, China 2018–2020. China CDC Wkly. 3, 741–745. doi: 10.46234/ccdcw2021.183
Li, Y., Lin, L., Li, Z., Ye, X., Xiong, K., Aryal, B., et al. (2012). Iron homeostasis regulates the activity of the microRNA pathway through poly(C)-binding protein 2. Cell Metab. 15, 895–904. doi: 10.1016/j.cmet.2012.04.021
Liu, P., Shen, L., Wang, S., Qin, P., Si, Y., Pan, M., et al. (2021). Increasing proportions of relapsing parasite species among imported malaria in china's guangxi province from Western and central Africa. Travel Med. Infect. Di 43, 102130. doi: 10.1016/j.tmaid.2021.102130
Mangal, P., Mittal, S., Kachhawa, K., Agrawal, D., Rath, B., Kumar, S. (2017). Analysis of the clinical profile in patients with plasmodium falciparum malaria and its association with parasite density. J. Glob. Infect. Dis. 9, 60–65. doi: 10.4103/0974-777X.201626
Mather, M. W., Ke, H. (2018). Novel defense peptides from platelets kill malaria parasites. Trends Parasitol. 34, 729–731. doi: 10.1016/j.pt.2018.07.013
Megabiaw, F., Eshetu, T., Kassahun, Z., Aemero, M. (2022). Liver enzymes and lipid profile of malaria patients before and after antimalarial drug treatment at dembia primary hospital and teda health center, Northwest, Ethiopia. Res. Rep. Trop. Med. 13, 11–23. doi: 10.2147/RRTM.S351268
Miller, L. H., Ackerman, H. C., Su, X. Z., Wellems, T. E. (2013). Malaria biology and disease pathogenesis: insights for new treatments. Nat. Med. 19, 156–167. doi: 10.1038/nm.3073
Mita-Mendoza, N. K., Magallon-Tejada, A., Parmar, P., Furtado, R., Aldrich, M., Saidi, A., et al. (2020). Dimethyl fumarate reduces TNF and plasmodium falciparum induced brain endothelium activation in vitro. Malar. J. 19, 376. doi: 10.1186/s12936-020-03447-7
Mita-Mendoza, N. K., van de Hoef, D. L., Lopera-Mesa, T. M., Doumbia, S., Konate, D., Doumbouya, M., et al. (2013). A potential role for plasma uric acid in the endothelial pathology of plasmodium falciparum malaria. PloS One 8, e54481. doi: 10.1371/journal.pone.0054481
Morrell, C. N. (2014). Understanding platelets in malaria infection. Curr. Opin. Hematol. 21, 445–449. doi: 10.1097/MOH.0000000000000073
Morrell, C. N., Aggrey, A. A., Chapman, L. M., Modjeski, K. L. (2014). Emerging roles for platelets as immune and inflammatory cells. Blood 123, 2759–2767. doi: 10.1182/blood
Nantakomol, D., Dondorp, A. M., Krudsood, S., Udomsangpetch, R., Pattanapanyasat, K., Combes, V., et al. (2011). Circulating red cell-derived microparticles in human malaria. J. Infect. Dis. 203, 700–706. doi: 10.1093/infdis/jiq104
Neida, K. (2020). Mita Mendoza AMTP. dimethyl fumarate reduces TNF and plasmodium falciparum induced brain endothelium activation in vitro. Malaria J. 19(1):376. doi: 10.1186/s12936-020-03447-7
Niang, M., Bei, A. K., Madnani, K. G., Pelly, S., Dankwa, S., Kanjee, U., et al. (2014). STEVOR is a plasmodium falciparum erythrocyte binding protein that mediates merozoite invasion and rosetting. Cell Host Microbe 16, 81–93. doi: 10.1016/j.chom.2014.06.004
Nieman, A. E., de Mast, Q., Roestenberg, M., Wiersma, J., Pop, G., Stalenhoef, A., et al. (2009). Cardiac complication after experimental human malaria infection: a case report. Malar. J. 8, 277. doi: 10.1186/1475-2875-8-277
O’Sullivan, J. M., O’Donnell, J. S. (2018). Platelets in malaria pathogenesis. Blood 132, 1222–1224. doi: 10.1182/blood-2018-08-865618
Ouma, B. J., Ssenkusu, J. M., Shabani, E., Datta, D., Opoka, R. O., Idro, R., et al. (2020). Endothelial activation, acute kidney injury, and cognitive impairment in pediatric severe malaria. Crit. Care Med. 48, e734–e743. doi: 10.1097/CCM.0000000000004469
Phillips, M. A., Burrows, J. N., Manyando, C., van Huijsduijnen, R. H., Van Voorhis, W. C., Wells, T. N. C. (2017). Malaria. Nat. Rev. Dis. Primers 3, 17050. doi: 10.1038/nrdp.2017.50
Rocha, B. C., Marques, P. E., Leoratti, F. M. S., Junqueira, C., Pereira, D. B., Antonelli, L. R. D. V., et al. (2015). Type I interferon transcriptional signature in neutrophils and low-density granulocytes are associated with tissue damage in malaria. Cell Rep. 13, 2829–2841. doi: 10.1016/j.celrep.2015.11.055
Sriboonvorakul, N., Ghose, A., Hassan, M. M. U., Hossain, M. A., Faiz, M. A., Pukrittayakamee, S., et al. (2018). Acidosis and acute kidney injury in severe malaria. Malar. J. 17, 128. doi: 10.1186/s12936-018-2274-9
Tona Lutete, G., Mombo-Ngoma, G., Assi, S. B., Bigoga, J. D., Koukouikila-Koussounda, F., Ntamabyaliro, N. Y., et al. (2021). Pyronaridine–artesunate real-world safety, tolerability, and effectiveness in malaria patients in 5 African countries: A single-arm, open-label, cohort event monitoring study. PloS Med. 18, e1003669. doi: 10.1371/journal.pmed.1003669
Truman-Rosentsvit, M., Berenbaum, D., Spektor, L., Cohen, L. A., Belizowsky-Moshe, S., Lifshitz, L., et al. (2018). Ferritin is secreted via 2 distinct nonclassical vesicular pathways. Blood 131, 342–352. doi: 10.1182/blood-2017-02-768580
Vaid, A., Ranjan, R., Smythe, W. A., Hoppe, H. C., Sharma, P. (2010). PfPI3K, a phosphatidylinositol-3 kinase from plasmodium falciparum, is exported to the host erythrocyte and is involved in hemoglobin trafficking. Blood 115, 2500–2507. doi: 10.1182/blood
van Dorp, L., Gelabert, P., Rieux, A., de Manuel, M., De-Dios, T., Gopalakrishnan, S., et al. (2020). Plasmodium vivax malaria viewed through the lens of an eradicated European strain. Mol. Biol. Evol. 37, 773–785. doi: 10.1093/molbev/msz264
Vasquez, M., Zuniga, M., Rodriguez, A. (2021). Oxidative stress and pathogenesis in malaria. Front. Cell. Infect. Microbiol. 11. doi: 10.3389/fcimb.2021.768182
Viriyavejakul, P., Khachonsaksumet, V., Punsawad, C. (2014). Liver changes in severe plasmodium falciparum malaria: histopathology, apoptosis and nuclear factor kappa b expression. Malar. J. 13, 106. doi: 10.1186/1475-2875-13-106
Wang, Y., Wang, X., Liu, X., Ren, R., Zhou, L., Li, C., et al. (2018). Epidemiology of imported infectious diseases, China 2005–2016. Emerg. Infect. Dis. 25, 33–41. doi: 10.3201/eid2501.180178
Watts, R. E., Odedra, A., Marquart, L., Webb, L., Abd-Rahman, A. N., Cascales, L., et al. (2020). Safety and parasite clearance of artemisinin-resistant plasmodium falciparum infection: A pilot and a randomised volunteer infection study in Australia. PloS Med. 17, e1003203. doi: 10.1371/journal.pmed.1003203
Wessells, K. R., Ouédraogo, C. T., Young, R. R., Faye, M. T., Brito, A., Hess, S. Y. (2017). Micronutrient status among pregnant women in zinder, Niger and risk factors associated with deficiency. Nutrients 9, 430. doi: 10.3390/nu9050430
WHO (2021). WORLD MALARIA REPORT World Health Organization. Available at: https://www.who.int/publications/i/item/9789240040496.
Yeo, T. W., Lampah, D. A., Gitawati, R., Tjitra, E., Kenangalem, E., Piera, K., et al. (2008). Angiopoietin-2 is associated with decreased endothelial nitric oxide and poor clinical outcome in severe falciparum malaria. Proc. Natl. Acad. Sci. U. S. A. 105, 17097–17102. doi: 10.1073/pnas.0805782105
Yeo, T. W., Weinberg, J. B., Lampah, D. A., Kenangalem, E., Bush, P., Chen, Y., et al. (2019). Glycocalyx breakdown is associated with severe disease and fatal outcome in plasmodium falciparum malaria. Clin. Infect. Dis. 69, 1712–1720. doi: 10.1093/cid/ciz038
Zhou, X. N. (2021). China Declared malaria-free: a milestone in the world malaria eradication and Chinese public health. Infect. Dis. Poverty 10, 98. doi: 10.1186/s40249-021-00882-9
Keywords: imported malaria, biochemical outcomes, risk factor, parasite clearance, prognostic model
Citation: Bi D, Lin J, Luo X, Lin L, Tang X, Luo X, Lu Y and Huang X (2022) Biochemical characteristics of patients with imported malaria. Front. Cell. Infect. Microbiol. 12:1008430. doi: 10.3389/fcimb.2022.1008430
Received: 31 July 2022; Accepted: 13 September 2022;
Published: 10 November 2022.
Edited by:
Penghua Wang, University of Connecticut Health Center, United StatesReviewed by:
Myat Htut Nyunt, Department of Medical Research, MyanmarDebarati Mukherjee, Institute of Post Graduate Medical Education And Research, India
Copyright © 2022 Bi, Lin, Luo, Lin, Tang, Luo, Lu and Huang. This is an open-access article distributed under the terms of the Creative Commons Attribution License (CC BY). The use, distribution or reproduction in other forums is permitted, provided the original author(s) and the copyright owner(s) are credited and that the original publication in this journal is cited, in accordance with accepted academic practice. No use, distribution or reproduction is permitted which does not comply with these terms.
*Correspondence: Xiaodong Huang, xiaodonghuang976@163.com