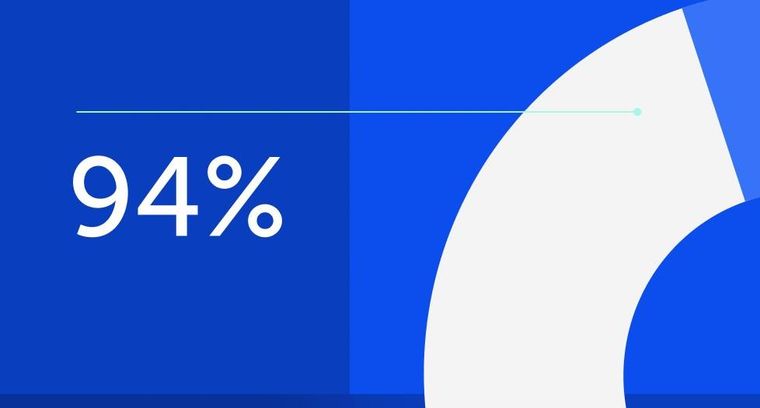
94% of researchers rate our articles as excellent or good
Learn more about the work of our research integrity team to safeguard the quality of each article we publish.
Find out more
BRIEF RESEARCH REPORT article
Front. Cell. Infect. Microbiol., 13 January 2022
Sec. Biofilms
Volume 11 - 2021 | https://doi.org/10.3389/fcimb.2021.809792
This article is part of the Research TopicAdvances in Treatment of Biofilm InfectionsView all 8 articles
Acne vulgaris is the most common dermatological disorder worldwide affecting more than 80% of adolescents and young adults with a global prevalence of 231 million cases in 2019. The involvement of the skin microbiome disbalance in the pathophysiology of acne is recognized, especially regarding the relative abundance and diversity of Propionibacterium acnes a well-known dominant human skin commensal. Biofilms, where bacteria are embedded into a protective polymeric extracellular matrix, are the most prevalent life style for microorganisms. P. acnes and its biofilm-forming ability is believed to be a contributing factor in the development of acne vulgaris, the persistence of the opportunistic pathogen and antibiotic therapy failures. Degradation of the extracellular matrix is one of the strategies used by bacteria to disperse the biofilm of competitors. In this study, we report the identification of an endogenous extracellular nuclease, BmdE, secreted by Propionibacterium granulosum able to degrade P. acnes biofilm both in vivo and in vitro. This, to our knowledge, may represent a novel competitive mechanism between two closely related species in the skin. Antibiotics targeting P. acnes have been the mainstay in acne treatment. Extensive and long-term use of antibiotics has led to the selection and spread of resistant bacteria. The extracellular DNase BmdE may represent a new bio-therapeutical strategy to combat P. acnes biofilm in acne vulgaris.
Biofilm formation is a surviving strategy contributing to the composition and long-term stability of the microbial populations (Rendueles and Ghigo, 2015; Flemming et al., 2016). Extracellular DNA (eDNA) is an important component of the matrix and its degradation is used by bacteria to modify biofilms (Flemming et al., 2016; Rumbaugh and Sauer, 2020). Within biofilms, secretion of nucleases has been described mostly as a strategy used by pathogens to evade the host immune system (Sharma et al., 2019). Whether endogenous matrix-degrading nucleases have any role in the setting of inter-species competition within biofilms is unknown.
Propionibacterium (Cutibacterium) acnes is a part of the normal skin microbiota dominating in the pilosebaceous unit (PSU), a hostile lipid-rich environment, low in oxygen and nutrients (Jahns et al., 2012; Jahns et al., 2013a). P. acnes ability to form biofilms is associated with the persistence of the pathogen and may also represent an adaptive strategy (Coenye et al., 2007; Dessinioti and Katsambas, 2017). The skin commensal Propionibacterium granulosum, while colonizing the same PSU as P. acnes, is commonly seen as a separate community (Jahns et al., 2015) implying a possible existence of a competitive mechanism. P. acnes biofilms are dominant in PSU (Jahns et al., 2012; Jahns et al., 2013a) and we hypothesized that a biofilm-degrading mechanism may be beneficial for P. granulosum colonization. We further speculated that this mechanism should be executed through a component secreted by P. granulosum.
In the present study we focused on identifying a possible antagonistic compound produced by P. granulosum facilitating colonization of PSU in the presence of P. acnes.
P. acnes KPA171202 and P. granulosum DSM 20700 (DSMZ, Braunschweig, Germany) were cultured under anaerobic conditions at 37°C and rotation 250 rpm as previously described (Bronnec and Alexeyev, 2021).
Total DNA from P. granulosum was extracted as previously described (Bronnec and Alexeyev, 2021). Paired-end sequencing was performed using Illumina HiSeq2000 2x100 bp runs. Reads were mapped against the reference genome GCA_900186975.1 and checked using Kraken 2. Quality control was performed using FastQC v0.11.9. De novo assembly and annotation were performed using RAPT release v0.3.2 on the reads filtered with KrakenTools. Data were analyzed and edited using NCBI Genome Workbench software version 3.6.
Sessile bacteria were grown in brain heart infusion broth supplemented with 2 g/L of glucose (BHIg) either in a volume of 10 mL in T-25 cell culture flasks (Sarstedt AB, Helsingborg, Sweden) or 200 µL in a 96-well polystyrene culture plate with flat bottom (Sarstedt AB). Biofilms were kept for 6 days with medium changes every other day. Incubations were done under anaerobic conditions at 37°C.
To prepare cell‐free supernatant (CFS), 10 mL of bacterial culture were centrifuged 10 minutes at 3 500 g at room temperature. The supernatant was filtered through a 0.22 μm syringe filter (Sarstedt AB). CFS was fractionated according to the following molecular weights: < 30 kDa, < 50 kDa, > 30 kDa and > 50 kDa using a size exclusion centrifugation device (Amicon® Ultra-15 Centrifugal Filter Units, Merck Chemicals and Life Science AB, Solna, Sweden) according to the manufacturer protocol.
The culture medium of a six-days old P. acnes biofilm was removed. The biofilm was washed with PBS 1X and incubated with P. granulosum CFS at 37°C for 24- and 48 hours. Controls included P. acnes biofilm incubated with either BHIg, P. acnes CFS or fresh planktonic cultures of P. granulosum or P. acnes. Effect of P. granulosum CFS on P. acnes biofilm was performed at least in triplicates with three biological replicates.
The sequence L860_000289 (Table 1), was amplified by PCR using the Phusion™ High-Fidelity DNA Polymerase (Fisher Scientific, Göteborg Sweden) with the forward primer 5’-GTACGAAGACTCCATGGGCCCAGCTCCCCACCC-3’ (with added NcoI restriction site) and reverse primer 5’-GTACAAGCTTCAGATGCCGGTGGCCG-3’(with added HindIII restriction site). The amplified sequence was inserted in frame into the pET HisZZ-TEV vector and the construct was confirmed by Sanger sequencing. The protein was overexpressed in Escherichia coli strain Rosetta (DE3) and purified thought Ni-NTA column followed by ion exchange and size-exclusion chromatography. Both His- an ZZ- tags were removed by enzymatic cleavage.
P. granulosum CFS containing protein fractions with different molecular weights were tested. P. acnes biofilm grown in 96-well plate was treated with 200 µL of P. granulosum CSF fractions for 24 hours at 37°C. Dispersal activity of the recombinant protein r-BmdE was evaluated with DNase I (Sigma-Aldrich Sweden AB, Stockholm, Sweden) as a positive control. An equal molar concentration of both enzymes (1 x 10-11 mol/mL) was tested in 10 mL. Biofilms were incubated for 24 hours. PBS 1X and r-BmdE buffer (50 mM NaP, 50 mM NaCl, 10 % glycerol, 2 mM β-Mercaptoethanol) were used as a negative control. The dispersal effect was visually evaluated. The experiment was performed in triplicate.
The DNase activity of P. granulosum CFS was evaluated as follows. The largest fraction > 50 kDa was concentrated 50 times using ultrafiltration devices according to the manufacturer protocol (Amicon®). Several volumes (1 µL, 3 µL and 5 µL) of this faction were incubated with 500 ng of P. acnes DNA for two hours at 37°C. P. acnes DNA was extracted as previously described (Bronnec and Alexeyev, 2021). As controls, reaction mix were supplemented with 3 mM MgCl2 and 10 mM EDTA. Samples were run on a 1 % (w/v) agarose gel containing GelGreen® Nucleic Acid Stain (VWR) to visualize DNA degradation.
A semi-quantitative analysis of biofilm dispersal activity was performed with crystal violet assay. P. acnes biofilms grown in a 96-well plate were washed with PBS 1X and treated with 200 µL of serial dilutions of enzymes ranging from 500 pmol to 3 x 10-9 pmol per well. After two hours incubation at 37°C crystal violet assay was performed as previously described (Peeters et al., 2008) with some modifications. Biofilms were stained with 0.1% (w/v) of crystal violet (Fisher Scientific). The solubilization was performed with 200 µL of a solution composed of 80% ethanol and 20% acetone (v/v). The absorbance was read at 560 nm. This experiment was performed with at least three technical and three biological replicates.
The DNase activity of the recombinant protein on P. acnes DNA was tested in the same way using different quantity of r-BmdE ranging from 5 µg to 0.2 µg per µg of DNA and incubated for 2 hours at 37°C. A six-days old preformed biofilm of P. acnes was incubated with 0.001 mg/mL of r-BmdE. The dispersal activity was visually observed after 24 hours of incubation. r-BmdE activity was also tested after pre-incubation of the enzyme with 10 % and 5 % (v/v) Intralipid® solution (Fresenius Kabi, Uppsala, Sweden) for 2 hours at room temperature followed by incubation with 1 µg of P. acnes DNA for 2 hours at 37°C. Lipids were removed after two successive centrifugations at 13 000 rpm for 10 min at 4°C.
The in vivo model of P. acnes biofilm in Drosophila melanogaster (Bronnec and Alexeyev, 2021) has been used to evaluate the dispersal activity of BmdE. Briefly, the feeding infection method has been used to develop a mature biofilm of P. acnes in the fruit fly gut. The biofilm was treated for three and five days with 0.65 nmol of r-BmdE. The fruit fly diet has been modified and supplemented at 11 % with Intralipid® solution (Bronnec and Alexeyev, 2021). A treatment with BHIg was used as a control. To evaluate the biofilm dispersion, formalin fixed, paraffin embedded flies were sectioned and observed with a light microscope. Biofilm positive sections were previously defined as sections containing large microbial structures attached to the gut wall in the abdominal part of the fly (Bronnec and Alexeyev, 2021). Other sections are considered as biofilm negative and correspond to a partial or a totally dispersed biofilm. These criteria were followed to evaluate P. acnes biofilm dispersion with r-BmdE.
To evaluate if P. acnes viability was affected by r-BmdE treatment, we used a live/dead staining kit as well as bacterial culture. First, P. acnes planktonic cultures were incubated with 50 %, 33 %, 10 % and 1% of r-BmdE for 30 minutes and 16 hours at 37°C, with r-BmdE buffer and BHIg as controls. LIVE/DEAD™ BacLight™ Bacterial Viability Kit (Thermo Fisher Scientific, Upsala, Sweden) was used according to the manufacturer protocol. Then, fruit flies excretions containing P. acnes released from the biofilm were collected after the treatment. The Whatman filter of the fruit fly vial was resuspended in 100 µL of buffered peptone water and the suspension was plated and incubated in anaerobically at 37°C.
A co-culture of P. granulosum as well as cell‐free supernatant (CFS) of P. granulosum with a six-days old P. acnes biofilm resulted in total dispersion of the biofilm after 48 hours (Figure 1A). Size-fractionation of the CFS further showed that P. acnes biofilm is degraded by a fraction size exceeding 50 kDa (Figure 1B). The absence of biofilm dispersion using P. acnes CFS indicated that the secreted protein is not conserved within P. acnes strains.
Figure 1 P. acnes biofilm dispersion by P. granulosum cell‐free supernatant (CFS). (A) Dispersal of 6 days old P. acnes biofilms in flask after 24 and 48 hours CFS treatment. (B) P. acnes biofilm dispersion in 96-well plate after 24 hours treatment with different P. granulosum CFS fractions.
We further sequenced the P. granulosum DSM 20700 genome. This Whole Genome Shotgun project has been deposited at DDBJ/ENA/GenBank under the accession JNBU00000000. The version described in this paper is version JNBU02000000. A bioinformatical analysis of the genome was performed using the following criteria: (i) a size ≥ 50 kDa, (ii) prediction of secretion-tag from amino acid sequence, (iii) a gene annotation keywords search “extracellular protein”, “secreted protein” or “uncharacterized protein” and (iv) sequence not conserved among P. acnes genomes. The first-choice candidate after the in-silico search corresponded to an uncharacterized extracellular nuclease (L860_000289) encoding for a 939 aa protein (Table 1). BLAST sequence analysis showed a high conservation of the sequence encoding this protein in P. granulosum strains (Table 1).
We further demonstrated that the CFS fraction > 50 kDa exhibited a DNase activity by degrading P. acnes DNA. The activity was enhanced with cations and inhibited with EDTA. We named this extracellular nuclease as BmdE (biofilm matrix degrading enzyme, eDNase BmdE) according to International Protein Nomenclature Guidelines from NCBI (NCBI, Last updated: 02-MAR-2020) (Table 1). Recombinant BmdE was produced and showed the ability to degrade P. acnes DNA (Figure 2A) as well as preformed biofilm of P. acnes in vitro (Figure 2B). A quantitative biofilm dispersion analysis was performed in 96-well plate with serial dilutions of r-BmdE (500 pmol to 3 x 10-9 pmol of enzyme/well). A quantity of 0.03 ng of r-BmdE was the lowest one used to disperse a mature biofilm of P. acnes in vitro compared to 0.25 ng for the control with DNase I. In the conditions tested, no lethal effect of r-BmdE was observed using the viability assay kit. Pre-incubation of r-BmdE in a lipid-rich environment showed enhanced DNA hydrolysis (Figure 2C).
Figure 2 In vitro deoxyribonuclease activity of r-BmdE. (A) P. acnes DNA treated with r-BmdE. Control 1 consisted of r-BmdE buffer at the highest volume. (B) Mature biofilm of P. acnes incubated r-BmdE. An equal molar ratio of DNase I (0.0003 mg/mL) was used as a positive control and 10 µL of PBS 1X and r-BmdE buffer were used as negative controls. (C) r-BmdE activity in lipids. Controls 2 and 3, consisted of r-BmdE buffer and water respectively.
We recently reported a successful development of an in vivo model of P. acnes biofilm in fruit fly on a lipid rich diet at an attempt to mimic a lipid rich PSU environment (Bronnec and Alexeyev, 2021). A 3- and 5 day in vivo treatment of P. acnes biofilm with r-BmdE resulted in biofilm degradation in both lipids and non-lipids environment (Table 2).
Results show that r-BmdE treatment does not impact P. acnes viability since the bacteria released from biofilm could be regrown on agar plate supporting results obtained with the viability assay kit.
Our results indicate that P. granulosum is able to secret BmdE, an eDNase disrupting P. acnes biofilm. Highly conserved within P. granulosum strains BmdE can represent an antagonistic compound facilitating colonization in the presence of P. acnes. In vitro, DNase activity of r-BmdE appears to be enhanced in lipids solution and retain activity in vivo in D. melanogaster on lipid-rich diet. This is in line with the observation that P. granulosum producing BmdE, naturally inhabits the PSU, a sebum/lipids-rich environment.
During bacterial colonization, competition for nutrients or adhesion sites require that one of the interacting microorganisms inhibits the development of other species through the production of antagonistic compounds. In bacterial biofilms, eDNA facilitates adhesion, helps to maintain the structural integrity of the biofilm. It is assumed that eDNA contributes to antimicrobial resistance and protects from the host immune system (Okshevsky and Meyer, 2015; Flemming et al., 2016). Microorganisms are also able to secrete enzymes to modulate their own biofilm. Based on this mechanism, several strategies are available to change the matrix stability using enzymes targeting eDNA at different stages of the biofilm life cycle (Tetz et al., 2009). The commensal Staphylococcus epidermidis has been reported to secret a protease interrupting the release of eDNA which consequently inhibits biofilm formation and nasal colonization by Staphylococcus aureus (Iwase et al., 2010; Chen et al., 2013; Stubbendieck and Straight, 2016). Our data suggest that the ability to produce a DNase can represent a novel competitive mechanism targeting biofilm within the same genus. This mechanism can probably explain our earlier observation that P. acnes and P. granulosum form separate communities while colonizing the same PSU (Jahns et al., 2015).
P. acnes biofilm formation is believed to be an important pathogenic mechanism in acne vulgaris (Jahns et al., 2012; Jahns et al., 2013b). Biofilms are thought to be able to directly block hair follicles, contribute to antibiotic resistance and inflammation. The use of BmdE in acne treatment for controlling the colonization and biofilm formation by P. acnes might be an innovative strategy. The use of this non-biocidal molecule, fully functional in its natural lipid-rich environment both in vitro and in vivo, may alter the biofilm structure by degrading eDNA and lead to the release of planktonic P. acnes susceptible to antibiotics. The usage of nucleases such as the recombinant human DNase is an effective and safe approach already described for Pseudomonas aeruginosa biofilm treatment in cystic fibrosis infection (Patel et al., 2020; Yang and Montgomery, 2021). Using enzymes that can weaken or destroy the biofilm structure can represent a new strategy to combat bacterial biofilms in acne vulgaris.
The datasets presented in this study can be found in online repositories. The names of the repository/repositories and accession number(s) can be found in the article/supplementary material.
VB conceived, designed, and performed experiments, and wrote the manuscript. HE, AJ, and HO conceived, designed, and performed experiments. OA conceived and designed experiments, and wrote the manuscript. All authors contributed to the article and approved the submitted version.
Financial support was provided through a regional agreement between Umeå University and Västerbotten County Council on cooperation in the field of Medicine, Odontology and Health (ALF), the Kempe Foundation and Umeå Biotech Incubator.
Based on these findings an international patent application has been filed (patent #WO2020190203A1: “New compositions and methods for the treatment of acne vulgaris”, 19 March 2020) by Vakona AB where OA is a part owner. This does not alter the authors’ adherence to all the Frontiers Journals policies on sharing data and materials.
The remaining authors declare that the research was conducted in the absence of any commercial or financial relationships that could be construed as a potential conflict of interest.
All claims expressed in this article are solely those of the authors and do not necessarily represent those of their affiliated organizations, or those of the publisher, the editors and the reviewers. Any product that may be evaluated in this article, or claim that may be made by its manufacturer, is not guaranteed or endorsed by the publisher.
Authors are grateful to Pär Larsson from the Department of Medical Biosciences - Pathology (Umeå University), to Mikael Lindberg from the Protein Expertise Platform (Umeå University), to Maria Kim from the Department of Molecular Biology (Umeå University) for their technical advices and assistance. Authors are also thankful to the staff at Clinical Pathology (Umeå University Hospital) for help with proceeding samples.
Bronnec, V., Alexeyev, O. A. (2021). In Vivo Model of Propionibacterium (Cutibacterium) Spp. Biofilm in Drosophila Melanogaster. Anaerobe 72, 102450. doi: 10.1016/j.anaerobe.2021.102450
Chen, C., Krishnan, V., Macon, K., Manne, K., Narayana, S. V., Schneewind, O. (2013). Secreted Proteases Control Autolysin-Mediated Biofilm Growth of Staphylococcus Aureus. J. Biol. Chem. 288, 29440–29452. doi: 10.1074/jbc.M113.502039
Coenye, T., Peeters, E., Nelis, H. J. (2007). Biofilm Formation by Propionibacterium Acnes Is Associated With Increased Resistance to Antimicrobial Agents and Increased Production of Putative Virulence Factors. Res. Microbiol. 158, 386–392. doi: 10.1016/j.resmic.2007.02.001
Dessinioti, C., Katsambas, A. (2017). Propionibacterium Acnes and Antimicrobial Resistance in Acne. Clin. Dermatol. 35, 163–167. doi: 10.1016/j.clindermatol.2016.10.008
Flemming, H.-C., Wingender, J., Szewzyk, U., Steinberg, P., Rice, S. A., Kjelleberg, S. (2016). Biofilms: An Emergent Form of Bacterial Life. Nat. Rev. Microbiol. 14, 563–575. doi: 10.1038/nrmicro.2016.94
Iwase, T., Uehara, Y., Shinji, H., Tajima, A., Seo, H., Takada, K., et al. (2010). Staphylococcus Epidermidis Esp Inhibits Staphylococcus Aureus Biofilm Formation and Nasal Colonization. Nature 465, 346–349. doi: 10.1038/nature09074
Jahns, A. C., Golovleva, I., Palmer, R. H., Alexeyev, O. A. (2013a). Spatial Distribution of Bacterial-Fungal Communities in Facial Skin. J. Dermatol. Sci. 70, 71–73. doi: 10.1016/j.jdermsci.2012.11.592
Jahns, A. C., Lundskog, B., Ganceviciene, R., Palmer, R. H., Golovleva, I., Zouboulis, C. C., et al. (2012). An Increased Incidence of Propionibacterium Acnes Biofilms in Acne Vulgaris: A Case-Control Study. Br. J. Dermatol. 167, 50–58. doi: 10.1111/j.1365-2133.2012.10897.x
Jahns, A. C., Lundskog, B., Nosek, D., Killasli, H., Emtestam, L., Alexeyev, O. A. (2015). Microbiology of Folliculitis Decalvans: A Histological Study of 37 Patients. J. Eur. Acad. Dermatol. Venereol 29, 1025–1026. doi: 10.1111/jdv.12448
Jahns, A. C., Oprica, C., Vassilaki, I., Golovleva, I., Palmer, R. H., Alexeyev, O. A. (2013b). Simultaneous Visualization of Propionibacterium Acnes and Propionibacterium Granulosum With Immunofluorescence and Fluorescence In Situ Hybridization. Anaerobe 23, 48–54. doi: 10.1016/j.anaerobe.2013.07.002
Ncbi (Last updated: 02-MAR-2020) Genome Database - International Protein Nomenclature Guidelines [Online]. Available at: https://www.ncbi.nlm.nih.gov/genome/doc/internatprot_nomenguide/#3-choosing-protein-names (Accessed Febuary 2021).
Okshevsky, M., Meyer, R. L. (2015). The Role of Extracellular DNA in the Establishment, Maintenance and Perpetuation of Bacterial Biofilms. Crit. Rev. Microbiol. 41, 341–352. doi: 10.3109/1040841X.2013.841639
Patel, K. K., Agrawal, A. K., Anjum, M. M., Tripathi, M., Pandey, N., Bhattacharya, S., et al. (2020). Dnase-I Functionalization of Ciprofloxacin-Loaded Chitosan Nanoparticles Overcomes the Biofilm-Mediated Resistance of Pseudomonas Aeruginosa. Appl. Nanosci. 10, 563–575. doi: 10.1007/s13204-019-01129-8
Peeters, E., Nelis, H. J., Coenye, T. (2008). Comparison of Multiple Methods for Quantification of Microbial Biofilms Grown in Microtiter Plates. J. Microbiol. Methods 72, 157–165. doi: 10.1016/j.mimet.2007.11.010
Rendueles, O., Ghigo, J.-M. (2015). Mechanisms of Competition in Biofilm Communities. Microbiol. Spectr. 3, 319–342. doi: 10.1128/microbiolspec.MB-0009-2014
Rumbaugh, K. P., Sauer, K. (2020). Biofilm Dispersion. Nat. Rev. Microbiol. 18, 571–586. doi: 10.1038/s41579-020-0385-0
Sharma, P., Garg, N., Sharma, A., Capalash, N., Singh, R. (2019). Nucleases of Bacterial Pathogens as Virulence Factors, Therapeutic Targets and Diagnostic Markers. Int. J. Med. Microbiol. 309, 151354. doi: 10.1016/j.ijmm.2019.151354
Stubbendieck, R. M., Straight, P. D. (2016). Multifaceted Interfaces of Bacterial Competition. J. Bacteriol 198, 2145–2155. doi: 10.1128/JB.00275-16
Tetz, G. V., Artemenko, N. K., Tetz, V. V. (2009). Effect of Dnase and Antibiotics on Biofilm Characteristics. Antimicrob. Agents Chemother. 3, 1204–1209. doi: 10.1128/AAC.00471-08
Keywords: Propionibacterium, Cutibacterium, biofilm, matrix-degrading enzyme, extracellular nuclease, inter-species competition, acne vulgaris
Citation: Bronnec V, Eilers H, Jahns AC, Omer H and Alexeyev OA (2022) Propionibacterium (Cutibacterium) granulosum Extracellular DNase BmdE Targeting Propionibacterium (Cutibacterium) acnes Biofilm Matrix, a Novel Inter-Species Competition Mechanism. Front. Cell. Infect. Microbiol. 11:809792. doi: 10.3389/fcimb.2021.809792
Received: 05 November 2021; Accepted: 08 December 2021;
Published: 13 January 2022.
Edited by:
Oana Ciofu, University of Copenhagen, DenmarkReviewed by:
Raymond Allan, De Montfort University, United KingdomCopyright © 2022 Bronnec, Eilers, Jahns, Omer and Alexeyev. This is an open-access article distributed under the terms of the Creative Commons Attribution License (CC BY). The use, distribution or reproduction in other forums is permitted, provided the original author(s) and the copyright owner(s) are credited and that the original publication in this journal is cited, in accordance with accepted academic practice. No use, distribution or reproduction is permitted which does not comply with these terms.
*Correspondence: Oleg A. Alexeyev, b2xlZy5hbGV4ZXlldkB1bXUuc2U=
†Present address: Hinnerk Eilers, Clinical Microbiology, University Hospital of Umeå, Umeå, Sweden
Anika C. Jahns, Promicol B.V., Geleen, Netherlands
Hélène Omer, Nutrition and Immunology, Technical University of Munich, Munich, Germany
Disclaimer: All claims expressed in this article are solely those of the authors and do not necessarily represent those of their affiliated organizations, or those of the publisher, the editors and the reviewers. Any product that may be evaluated in this article or claim that may be made by its manufacturer is not guaranteed or endorsed by the publisher.
Research integrity at Frontiers
Learn more about the work of our research integrity team to safeguard the quality of each article we publish.