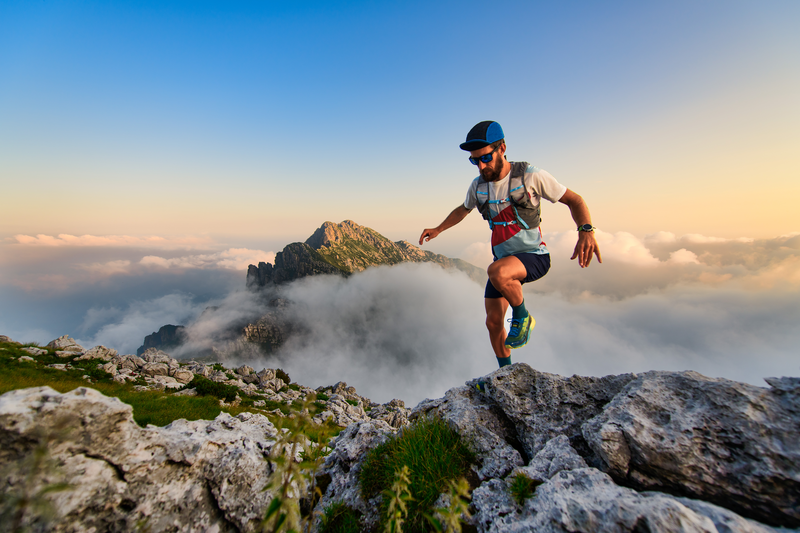
95% of researchers rate our articles as excellent or good
Learn more about the work of our research integrity team to safeguard the quality of each article we publish.
Find out more
ORIGINAL RESEARCH article
Front. Cell. Infect. Microbiol. , 28 January 2022
Sec. Microbiome in Health and Disease
Volume 11 - 2021 | https://doi.org/10.3389/fcimb.2021.787667
This article is part of the Research Topic The Microbial Modulation of Autoimmune Processes and Proinflammatory Pathways View all 10 articles
Introduction: Haploinsufficiency of A20 (HA20) is a form of inborn errors of immunity (IEI). IEIs are genetically occurring diseases, some of which cause intestinal dysbiosis. Due to the dysregulation of regulatory T cells (Tregs) observed in patients with HA20, gut dysbiosis was associated with Tregs in intestinal lamina propria.
Methods: Stool samples were obtained from 16 patients with HA20 and 15 of their family members. Infant samples and/or samples with recent antibiotics use were excluded; hence, 26 samples from 13 patients and 13 family members were analyzed. The 16S sequencing process was conducted to assess the microbial composition of samples. Combined with clinical information, the relationship between the microbiome and the disease activity was statistically analyzed.
Results: The composition of gut microbiota in patients with HA20 was disturbed compared with that in healthy family members. Age, disease severity, and use of immunosuppressants corresponded to dysbiosis. However, other explanatory factors, such as abdominal symptoms and probiotic treatment, were not associated. The overall composition at the phylum level was stable, but some genera were significantly increased or decreased. Furthermore, among the seven operational taxonomic units (OTUs) that increased, two OTUs, Streptococcus mutans and Lactobacillus salivarius, considerably increased in patients with autoantibodies than those without autoantibodies.
Discussion: Detailed interaction on intestinal epithelium remains unknown; the relationship between the disease and stool composition change helps us understand the mechanism of an immunological reaction to microorganisms.
A20, which is encoded by the tumor necrosis factor alpha-induced protein 3 (TNFAIP3) gene, is a negative regulator of the TNF-nuclear factor-κB (NF-κB) signaling pathway. Haploinsufficiency of A20 (HA20) causes autoinflammatory and autoimmune disorders (Kadowaki et al., 2018). Dysbiosis of the intestinal microbiome has been observed in some inborn errors of immunities (IEIs)/primary immunodeficiency disease, such as chronic granulomatous disease (CGD) (Sokol et al., 2016), X-linked inhibitor of apoptosis (XIAP) deficiency (Sokol et al., 2016; Ono et al., 2021), tetratricopeptide repeat domain 7A (TTC7A) deficiency (Sokol et al., 2016), common variable immunodeficiency (CVID) (Jørgensen et al., 2016; Varricchi et al., 2021), and Wiskott–Aldrich syndrome (Zhang et al., 2020). Despite IEIs having a monogenetic nature, they vary in severity relating to the microbiome (Liu et al., 2021). Little is known about the mechanism of dysbiosis in patients with IEI, but the association of gut microbiota and regulatory T cells (Tregs) was reported (Liu et al., 2021). Tregs regulate A20 (Kadowaki et al., 2020); as a result, the gut microbiota of patients with HA20 plays a vital role in association with Tregs. Alternatively, the mouse lacking A20 expression in dendritic cells showed intestinal dysbiosis (Talpin et al., 2019). This is the first report of the microbiome in patients with HA20.
Kadowaki et al. (2018) previously reported that there were 22 patients with HA20 from nine families in Japan. Thirty-six individuals from nine families, including 18 patients, 17 healthy family members living together, and a sibling whose genetic information is unknown, agreed to participate in this study. Next, fecal samples were obtained from 16 patients and 15 healthy relatives from eight corresponding families. Clinical characteristics of the patients were previously reported (patients 3–5, 7–13, and 17–22), and additional information, such as sex, age at the sample collection, symptoms, and medication, was collected (Supplementary Table 1).
We proposed the classification of disease severity for clinical manifestations of patients with HA20. The mild type was defined as asymptomatic or minor phenotype (e.g., mild recurrent stomatitis and/or rash). The moderate type was defined as paroxysmal symptoms (e.g., recurrent fever and/or abdominal pain). The frequency of symptoms is once or more for at least 3 months. The severe type was defined as persistent inflammation (e.g., fever, central nerve lesion, vascular lesion, ocular lesion, intestinal lesion, and/or arthritis) sustained over 2 weeks.
Fecal samples were collected and stored at 4°C under anaerobic conditions until preparation. Fecal samples were stirred until completely homogenized and suspended in phosphate-buffered saline (PBS) containing 20% glycerol (1 g of feces per 5 ml) and poured through a filter to remove large-sized food-derived debris. The fecal suspension was added to EDTA (final concentration of 10 mM, Nacalai) and stored at −80°C until use. After thawing, 100 μl of fecal suspension was gently mixed and incubated in 800 μl of TE10 (10 mM of Tris-HCl and 10 mM of EDTA) buffer containing RNase A (final concentration of 100 μg/ml, Invitrogen) and lysozyme (final concentration of 15 mg/ml, Sigma) for 1 h at 37°C. Purified achromopeptidase (final concentration of 2,000 U/ml, Wako) was added and further incubated for 30 min at 37°C. Sodium dodecyl sulfate (SDS) (final concentration of 1%) and proteinase K (final concentration of 1 mg/ml, Roche) were further added to the mixture and incubated for 1 h at 55°C. High-molecular-weight DNA was extracted with phenol:chloroform:isoamyl alcohol (25:24:1 at pH 7.9), precipitated with isopropanol (equal volume to the aqueous phase), washed with 1 ml of 70% ethanol, and gently resuspended in 30 μl of TE buffer. We used 40 ng of DNA per sample for the sequence.
The sequencing of the 16S ribosomal RNA gene from fecal samples was performed as previously described (Kim et al., 2013; Kakihana et al., 2016). The hypervariable V1–2 region of the 16S rRNA gene was amplified by PCR using barcoded 27Fmod and 338R primers. DNA extracted from Escherichia coli Strain SE 11 (isolated from a healthy adult) was used as the positive control template, whereas a DNA-free sample was used as the negative control. The electrophoresis procedure confirmed the PCR amplicons and negative reaction. Then, an equal amount of purified PCR amplicons was sequenced on a MiSeq platform (Illumina, San Diego, CA). Next, 3,000 high-quality reads were randomly selected per sample and analyzed to minimize the overestimation of species richness during clustering associated with the sequencing error (Kim et al., 2013). Good’s coverage index (Singleton et al., 2001) for the 3,000 reads per sample in the current study was 0.980, indicating a high degree of coverage and a sufficient read number for the fecal microbiome analysis. Furthermore, the reads were sorted into operational taxonomic units (OTUs) using the UCLUST algorithm, at a sequence identity threshold of 97% (Stackebrandt and Goebel, 1994; Konstantinidis and Tiedje, 2005). Taxonomic assignments of each OTU were made by similarity searching against the publicly available 16S (RDP v.10.27 and CORE) and National Center for Biotechnology Information genome database, using GLSEARCH. OTU-based microbial diversity was estimated using the Shannon index with Scikit-bio (v.0.5.5). A comparison of each group (family, HA20) in the unweighted and weighted UniFrac principal coordinate analysis (PCoA) was evaluated by using permutational multivariate ANOVA (PERMANOVA) with Scikit-bio (v.0.5.5) (Anderson, 2017).
Statistical analysis of OTUs and Shannon index was performed using the Wilcoxon rank-sum test. Values are represented as median (minimum–maximum). The threshold for significance was p < 0.05. Also, stratified analysis was performed using the two-tailed t-test for ordinal scale or regression analysis for continuous scale. Statistical analyses were conducted using R package (exactRankTests) or JMP Pro v.16.0.0 (SAS Institute Inc., NC, USA). The linear discriminant analysis (LDA) effect size (LEfSe) method was performed on the Huttenhower lab Galaxy server (https://huttenhower.sph.harvard.edu/galaxy/) by importing the bacterial relative abundance values at the genus level with Kruskal–Wallis test p < 0.05 and LDA score (log10) > 2 (Segata et al., 2011).
Thirty-one fecal samples from 16 patients and 15 healthy family members were sequenced. However, samples from three patients who received antibiotic treatment within 4 weeks of stool collection were excluded from statistical analysis. Also, two samples from children under the age of 3 years were excluded from statistical analysis because their gut microbiome is naturally different from the older’s microbiome (Yatsunenko et al., 2012). Nobody but an excluded younger brother (P22-sib) has food allergies. The median (min–max) age of 13 patients with HA20 and their healthy family members of 13 individuals were 19 (3–43) and 36 (4–49) years (p = 0.09, Wilcoxon rank-sum test), respectively. Patients with HA20 consist of four mild, four moderate, and five severe patients. Eleven of 13 patients and eight of 13 presented with stomatitis and abdominal symptoms, respectively. One patient was treated with probiotics and 5 with immunosuppressants (Supplementary Table 1).
Ten thousand reads were used from an average (and SD) of 53,935 (± 9,383) raw reads. An average of 9,848 reads passed the filter, and every 3,000 Passed Filter reads were used for OTU analysis. The number of OTUs in patients’ samples was considerably less than their healthy members (median 123 vs. 150, p = 0.049, Wilcoxon rank-sum test) (Figure 1A). Likewise, the Shannon index in patients’ samples was significantly less than their healthy members (median 4.68 vs. 5.26, p = 0.009, Wilcoxon rank-sum test) (Figure 1B), whereas the principal component analysis showed a significant difference in the composition of the intestinal microbiome in unweighted UniFrac analysis (p = 0.008, PERMANOVA) (Figure 2A), although not significant in weighted UniFrac analysis (p = 0.059) (Figure 2B). Thus, the stratified analysis suggested that any sex, stomatitis, abdominal symptoms, and use of probiotics do not affect the microbiome diversity (Tables 1 and 2). Lower age was significantly associated with a lower Shannon index although insignificantly with OTUs. Both disease severity and immunosuppressant use were associated with a lower number of OTUs with significance, and they showed lower Shannon index although not significantly.
Figure 1 The numbers of OTUs and Shannon index. The numbers of OTUs (median 123 vs. 150, p = 0.049) (A) and Shannon index (median 4.68 vs. 5.26, p = 0.009) (B) of patients with HA20 and their family members. Box plot indicates median value, and lower and upper quartiles. p-Value is calculated using the Wilcoxon rank-sum test. *p < 0.05, **p < 0.01. OTU, operational taxonomic unit; HA20, haploinsufficiency of A20.
Figure 2 Principal component analysis of gut flora. Clustering of patients (red triangle) and their families (blue circle) following unweighted UniFrac analysis (p = 0.008). (A) Weighted UniFrac analysis (p = 0.059). (B) Explained variation is represented as a percentage.
At the phylum level, there was no significant difference between patients with HA20 and their family members (Figure 3 and Supplementary Table 2). Conversely, at the genus level, five genera (Streptococcus, Lactobacillus, Butyricicoccus, Haemophilus, and Enterococcus) increased, and another four (Ruminococcus, Clostridium, Parabacteroides, and Alistipes) decreased in patients with HA20 compared with their family members (LDA score greater than two at p < 0.05 with LEfSe analysis) (Figure 4A). At the OTU level, seven OTUs significantly increased in patients with HA20 compared with their family members (p < 0.001: closest species/strain name Haemophilus parainfluenzae, Streptococcus mutans, and Veillonella sp. oral taxon 158, p < 0.05: Bifidobacterium dentium, Lactobacillus salivarius, Enterococcus avium, and Eubacterium eligens, Wilcoxon rank-sum test). Most of these OTUs are indigenous bacteria and are undetected in the intestinal microbiota. As a representative, only one of two healthy child siblings under 10 years and none of 11 healthy adult members had H. parainfluenzae, when all six child patients with HA20 and two of seven adult patients with HA20 had it (Figure 4B). None of these seven OTUs showed a significant correlation with either stomatitis or abdominal symptoms. However, two of seven OTUs showed a significant difference between patients with and without autoantibodies (four and five patients, respectively; four patients were not analyzed) (Figure 4C).
Figure 3 The composition of gut bacterial microbiota. The global composition of bacterial microbiota at the phylum level for the indicated groups. The healthy family members (left) and patients (middle) are represented in order of age. The two bars on the right are average values. P, patient; fa, Father; mo, mother; sib, sibling.
Figure 4 Significant increase or decrease of bacteria at the genus level and increase at the OTU level. The LDA score of patients with HA20 compared with their family members (Kruskal–Wallis test p < 0.05 and LDA score (log10) > 2) are represented. (A) The increased OTUs in patients with HA20 compared with their family members. (B) and the increased OTUs in patients with autoantibodies compared with those without antibodies. (C) Their closest species or strain and percentage similarity (%) in the Ribosomal Database Project (RDP) database are indicated. Box plot indicates the median value, and lower and upper quartiles. p-Values are calculated using the Wilcoxon rank-sum test. *p < 0.05, **p < 0.01. OTU, operational taxonomic unit; LDA, linear discriminant analysis; HA20, haploinsufficiency of A20.
This study demonstrated that patients with HA20 show intestinal dysbiosis. Despite HA20 being a genetically occurring disease, previous studies report bacterial composition changes in other IEIs. XIAP deficiency is a rare IEI, and it is characterized by recurrent hemophagocytic lymphohistiocytosis and refractory inflammatory bowel disease (IBD) similar to Crohn’s disease. Furthermore, patients with XIAP deficiency showed intestinal dysbiosis and IBD, which are rescued by allogeneic hematopoietic cell transplantation (Ono et al., 2021). These data further establish the hypothesis that monogenic disease is the cause of gut dysbiosis. From previous studies, there are 118 of 354 IEIs that exhibit gastrointestinal symptoms (Hartono et al., 2019), and the interaction between bacteria and the immune system might vary with the disease. For instance, immunoglobulin A secreted at the mucosal surface of the gastrointestinal tract was associated with selective IgA deficiency (Berbers et al., 2019), and the absence of various subsets of T cells in intestinal lamina propria was associated with X-linked severe combined immunodeficiency (Clarke et al., 2018).
The mechanism that causes dysbiosis in patients with HA20 is mediated by Tregs because it is a key factor of immunoregulatory disorders in patients with HA20 (Liu et al., 2021), and in the mouse model of Treg-associated monogenic autoimmune disorders, Aire knockout mouse showed significant dysbiosis (Dobeš et al., 2015). Mouse models, Aire-deficient mice (Gray et al., 2007), and Foxp3 knockout mice (Chinen et al., 2010) exhibit an autoimmune phenotype even in germ-free conditions, and it was concluded that these diseases are independent of commensal microbial regulation (Liu et al., 2021). Conversely, the Treg-depleted model showed more severe inflammation in the small intestine of specific pathogen-free mice than germ-free mice, which indicates that the disease severity is associated with the microbiome (Liu et al., 2021). These findings indicate the possible association between disease severity and gut dysbiosis. Additionally, data from this study showed a significant correlation between disease severity and dysbiosis. The use of immunosuppressants, an alternative indicator of disease severity, also correlated with dysbiosis.
Another possible mechanism of dysbiosis is metabolism. A mouse model lacking A20 in specific dendritic cells reported aberrant expression of antibacterial peptides and luminal dysbiosis (Talpin et al., 2019).
Ten of 18 patients with HA20 were treated with immunosuppressants (Kadowaki et al., 2020), and one refractory patient successfully received hematopoietic cell transplantation (Shiraki et al., 2021). However, less invasive therapy would be required. Successful fecal microbiota transplantation (FMT) is reported in patients with HIV (Serrano-Villar et al., 2021) and the mouse model of Behcet’s disease (Ye et al., 2018), while there is no evidence of FMT in patients with IEIs including HA20. Further study is required to clarify the mechanism of dysbiosis in patients with HA20 and IEIs, to determine the biomarker of the disease, and to discover if FMT works or not.
Several monogenic IEIs inform us how the disease-specific immune dysfunction causes disease-specific dysbiosis. Collectively, we can easily understand how the complex immune systems respond to various microorganisms. This study showed that S. mutans and L. salivarius significantly increased in both patients with HA20, those with autoantibodies compared with family members and patients without autoantibodies, respectively. Since the sample size was small for statistical analysis (13 vs. 13, and 4 vs. 5, respectively), statistical significance in two manners was rare enough to reject the null hypothesis. Also, although the close mechanism remains unclear, it was suggested that these two OTUs might be associated with autoimmune onset.
In the context of statistical analysis, we excluded patients younger than 3 years and/or patients with antibiotics use because they present a confounding bias for microbiota (Hopkins et al., 2002; Yatsunenko et al., 2012; Ramirez et al., 2020). However, our data suggested that lower age is the explanatory variable for lower Shannon index, not for OTU numbers. This potential confounding bias is statistically a problem but not clinically crucial. Considering clinical management, both lower age and antibiotics use affect disease severity as well as microbiome diversity. Indeed, the excluded patients based on antibiotics use [previously described as P4, P9, and P21 (Kadowaki et al., 2018)] were relatively younger (4, 1, and 3 years old, respectively) and more severe (P4 and P9 are moderate; P21 is severe) than the included patients. Early-onset patients could be regarded as more strongly influenced by genetics and so potentially more severe than late-onset patients.
This study has several limitations. Firstly, clinical heterogeneity exists among medications, such as probiotics and antibiotics. The potential confounding factor is underestimated because of the small sample size. Secondly, because of rare diseases, it is difficult to collect more fecal samples for statistical analysis of the potential confounding factor described. Multivariate analysis was unfeasible due to small samples. Thirdly, not all patients with HA20 received gastrointestinal endoscopy, and so alternative indicators such as the disease severity or abdominal symptoms do not necessarily reflect the exact intestinal condition.
We investigated the intestinal microbiome composition in patients with HA20 and identified that seven OTUs significantly increased in the patients. In particular, S. mutans and L. salivarius significantly increased in patients with HA20 and autoantibodies, and these OTUs were associated with autoimmune onset.
The datasets for this article are not publicly available as regards participant/patient anonymity. Requests to access the datasets should be forwarded to the corresponding author.
Written informed consent was obtained from patients or their parents. This study was conducted following the Declaration of Helsinki and approved by the ethics boards of Tokyo Medical and Dental University and Keio University.
ET, KT, and YK contributed to data management. KT, YK, WS, HM, WS, and MH conducted DNA sequencing and bioinformatics analysis. HO and HK managed sample recruitment. HO, TK, RN, KN, CK, TE, TS, and YI contributed to sample collection. ET, KT, and HK wrote the manuscript. TM and KH provided critical discussion. HK designed and managed the project. All authors read and approved the final manuscript.
This work was partly supported by MEXT/JSPS KAKENHI (Grant Number: JP17K10099) and Naoki Tsuchida Research Grant to HK.
KH is a scientific advisory board member of Vedanta Biosciences and 4BIO CAPITAL. YK is an employee of JSR Corporation.
The remaining author declares that the research was conducted in the absence of any commercial or financial relationships that could be construed as a potential conflict of interest.
All claims expressed in this article are solely those of the authors and do not necessarily represent those of their affiliated organizations, or those of the publisher, the editors and the reviewers. Any product that may be evaluated in this article, or claim that may be made by its manufacturer, is not guaranteed or endorsed by the publisher.
We appreciate the patients and their families for their participation in this study. We express gratitude to Mayako Asakawa for technical support and members of the JSR-Keio University Medical and Chemical Innovation Center for conducting the meta-16S rRNA gene sequencing. We also express gratitude to Kazushi Izawa at the Department of Pediatrics, Kyoto University Hospital, for collecting clinical information for revision.
The Supplementary Material for this article can be found online at: https://www.frontiersin.org/articles/10.3389/fcimb.2021.787667/full#supplementary-material
Anderson, M. J. (2017). Permutational Multivariate Analysis of Variance (PERMANOVA) (Wiley StatsRef), 1–15. Stat Ref Online. doi: 10.1002/9781118445112.stat07841
Berbers, R. M., Franken, I. A., Leavis, H. L. (2019). Immunoglobulin A and Microbiota in Primary Immunodeficiency Diseases. Curr. Opin. Allergy Clin. Immunol. 19, 563–570. doi: 10.1097/ACI.0000000000000581
Chinen, T., Volchkov, P. Y., Chervonsky, A. V., Rudensky, A. Y. (2010). A Critical Role for Regulatory T Cell-Mediated Control of Inflammation in the Absence of Commensal Microbiota. J. Exp. Med. 207, 2323–2330. doi: 10.1084/jem.20101235
Clarke, E. L., Connell, A. J., Six, E., Kadry, N. A., Abbas, A. A., Hwang, Y., et al. (2018). T Cell Dynamics and Response of the Microbiota After Gene Therapy to Treat X-Linked Severe Combined Immunodeficiency. Genome Med. 10, 70. doi: 10.1186/s13073-018-0580-z
Dobeš, J., Neuwirth, A., Dobešová, M., Vobořil, M., Balounová, J., Ballek, O., et al. (2015). Gastrointestinal Autoimmunity Associated With Loss of Central Tolerance to Enteric α-Defensins. Gastroenterology 149, 139–150. doi: 10.1053/j.gastro.2015.05.009
Gray, D. H. D., Gavanescu, I., Benoist, C., Mathis, D. (2007). Danger-Free Autoimmune Disease in Aire-Deficient Mice. Proc. Natl. Acad. Sci. U. S. A. 104, 18193–18198. doi: 10.1073/pnas.0709160104
Hartono, S., Ippoliti, M. R., Mastroianni, M., Torres, R., Rider, N. L. (2019). Gastrointestinal Disorders Associated With Primary Immunodeficiency Diseases. Clin. Rev. Allergy Immunol. 57, 145–165. doi: 10.1007/s12016-018-8689-9
Hopkins, M. J., Sharp, M., Macfarlane, G. T. (2002). Variation in Human Intestinal Microbiota With Age. Dig. Liver Dis. 24, S12–S18. doi: 10.1016/s1590-8658(02)80157-8
Jørgensen, S. F., Trøseid, M., Kummen, M., Anmarkrud, J. A., Michelsen, A. E., Osnes, L. T., et al. (2016). Altered Gut Microbiota Profile in Common Variable Immunodeficiency Associates With Levels of Lipopolysaccharide and Markers of Systemic Immune Activation. Mucosal Immunol. 9, 1455–1465. doi: 10.1038/mi.2016.18
Kadowaki, T., Ohnishi, H., Kawamoto, N., Hori, T., Nishimura, K., Kobayashi, C., et al. (2018). Haploinsufficiency of A20 Causes Autoinflammatory and Autoimmune Disorders. J. Allergy Clin. Immunol. 141, 1485–1488.e11. doi: 10.1016/j.jaci.2017.10.039
Kadowaki, T., Ohnishi, H., Kawamoto, N., Kadowaki, S., Hori, T., Nishimura, K., et al. (2020). Immunophenotyping of A20 Haploinsufficiency by Multicolor Flow Cytometry. Clin. Immunol. 216, 108441. doi: 10.1016/j.clim.2020.108441
Kakihana, K., Fujioka, Y., Suda, W., Najima, Y., Kuwata, G., Sasajima, S., et al. (2016). Fecal Microbiota Transplantation for Patients With Steroid-Resistant Acute Graft-Versus-Host Disease of the Gut. Blood 128, 2083–2088. doi: 10.1182/blood-2016-05-717652
Kim, S. W., Suda, W., Kim, S., Oshima, K., Fukuda, S., Ohno, H., et al. (2013). Robustness of Gut Microbiota of Healthy Adults in Response to Probiotic Intervention Revealed by High-Throughput Pyrosequencing. DNA Res. 20, 241–253. doi: 10.1093/dnares/dst006
Konstantinidis, K. T., Tiedje, J. M. (2005). Genomic Insights That Advance the Species Definition for Prokaryotes. Proc. Natl. Acad. Sci. U. S. A. 102, 2567–2572. doi: 10.1073/pnas.0409727102
Liu, Y., Freeborn, J., Armbrister, S. A., Tran, D. Q., Rhoads, J. M. (2021). Treg-Associated Monogenic Autoimmune Disorders and Gut Microbial Dysbiosis. Pediatr. Res. 91(1), 35–43. doi: 10.1038/s41390-021-01445-2
Liu, Y., Tran, D. Q., Lindsey, J. W., Rhoads, J. M. (2021). The Association of Gut Microbiota and Treg Dysfunction in Autoimmune Diseases. Adv. Exp. Med. Biol. 1278, 191–203. doi: 10.1007/978-981-15-6407-9_10
Ono, S., Takeshita, K., Kiridoshi, Y., Kato, M., Kamiya, T., Hoshino, A., et al. (2021). Hematopoietic Cell Transplantation Rescues Inflammatory Bowel Disease and Dysbiosis of Gut Microbiota in XIAP Deficiency. J. Allergy Clin. Immunol. Pract. 9 (10), 3767–3780. doi: 10.1016/j.jaip.2021.05.045
Ramirez, J., Guarner, F., Bustos Fernandez, L. B., Maruy, A., Sdepanian, V. L., Cohen, H. (2020). Antibiotics as Major Disruptors of Gut Microbiota. Front. Cell. Infect. Microbiol. 10, 572912. doi: 10.3389/fcimb.2020.572912
Segata, N., Izard, J., Waldron, L., Gevers, D., Miropolsky, L., Garrett, W. S., et al. (2011). Metagenomic Biomarker Discovery and Explanation. Genome Biol. 12, R60. doi: 10.1186/gb-2011-12-6-r60
Serrano-Villar, S., Talavera-Rodríguez, A., Gosalbes, M. J., Madrid, N., Pérez-Molina, J. A., Elliott, R. J., et al. (2021). Fecal Microbiota Transplantation in HIV: A Pilot Placebo-Controlled Study. Nat. Commun. 12, 1139. doi: 10.1038/s41467-021-21472-1
Shiraki, M., Williams, E., Yokoyama, N., Shinoda, K., Nademi, Z., Matsumoto, K., et al. (2021). Hematopoietic Cell Transplantation Ameliorates Autoinflammation in A20 Haploinsufficiency. J. Clin. Immunol. 41 (8), 1954–1956. doi: 10.1007/s10875-021-01124-1
Singleton, D. R., Furlong, M. A., Rathbun, S. L., Whitman, W. B. (2001). Quantitative Comparisons of 16S rRNA Gene Sequence Libraries From Environmental Samples. Appl. Environ. Microbiol. 67, 4374–4376. doi: 10.1128/AEM.67.9.4374-4376.2001
Sokol, H., Mahlaoui, N., Aguilar, C., Bach, P., Lambert, O. J., Garraffo, A., et al. (2016). Intestinal Dysbiosis in Inflammatory Bowel Disease Associated With Primary Immunodeficiency. J. Allergy Clin. Immunol. 143, 775–778.e6. doi: 10.1016/j.jaci.2018.09.021
Stackebrandt, E., Goebel, B. M. (1994). Taxonomic Note: A Place for DNA-DNA Reassociation and 16S rRNA Sequence Analysis in the Present Species Definition in Bacteriology. Int. J. Syst. Bacteriol. 44, 846–849. doi: 10.1099/00207713-44-4-846
Talpin, A., Kattah, M. G., Advincula, R., Fadrosh, D., Lynch, K., LaMere, B., et al. (2019). A20 in Dendritic Cells Restrains Intestinal Anti-Bacterial Peptide Expression and Preserves Commensal Homeostasis. PloS One 14, e0218999. doi: 10.1371/journal.pone.0218999
Varricchi, G., Poto, R., Ianiro, G., Punziano, A., Marone, G., Gasbarrini, A., et al. (2021). Gut Microbiome and Common Variable Immunodeficiency: Few Certainties and Many Outstanding Questions. Front. Immunol. 12, 712915. doi: 10.3389/fimmu.2021.712915
Yatsunenko, T., Rey, F. E., Manary, M. J., Trehan, I., Dominguez-Bello, M. G., Contreras, M., et al. (2012). Human Gut Microbiome Viewed Across Age and Geography. Nature 486, 222–227. doi: 10.1038/nature11053
Ye, Z., Zhang, N., Wu, C., Zhang, X., Wang, Q., Huang, X., et al. (2018). A Metagenomic Study of the Gut Microbiome in Behcet’s Disease. Microbiome 6, 135. doi: 10.1186/s40168-018-0520-6
Keywords: haploinsufficiency of A20, intestinal microbiome, regulatory T cells, inborn errors of immunity, Streptococcus mutans, Lactobacillus salivarius
Citation: Toyofuku E, Takeshita K, Ohnishi H, Kiridoshi Y, Masuoka H, Kadowaki T, Nishikomori R, Nishimura K, Kobayashi C, Ebato T, Shigemura T, Inoue Y, Suda W, Hattori M, Morio T, Honda K and Kanegane H (2022) Dysregulation of the Intestinal Microbiome in Patients With Haploinsufficiency of A20. Front. Cell. Infect. Microbiol. 11:787667. doi: 10.3389/fcimb.2021.787667
Received: 01 October 2021; Accepted: 28 December 2021;
Published: 28 January 2022.
Edited by:
Elias Adel Rahal, American University of Beirut, LebanonReviewed by:
Daniel Alford Powell, University of Arizona, United StatesCopyright © 2022 Toyofuku, Takeshita, Ohnishi, Kiridoshi, Masuoka, Kadowaki, Nishikomori, Nishimura, Kobayashi, Ebato, Shigemura, Inoue, Suda, Hattori, Morio, Honda and Kanegane. This is an open-access article distributed under the terms of the Creative Commons Attribution License (CC BY). The use, distribution or reproduction in other forums is permitted, provided the original author(s) and the copyright owner(s) are credited and that the original publication in this journal is cited, in accordance with accepted academic practice. No use, distribution or reproduction is permitted which does not comply with these terms.
*Correspondence: Hirokazu Kanegane, aGthbmVnYW5lLnBlZEB0bWQuYWMuanA=
†These authors have contributed equally to this work
Disclaimer: All claims expressed in this article are solely those of the authors and do not necessarily represent those of their affiliated organizations, or those of the publisher, the editors and the reviewers. Any product that may be evaluated in this article or claim that may be made by its manufacturer is not guaranteed or endorsed by the publisher.
Research integrity at Frontiers
Learn more about the work of our research integrity team to safeguard the quality of each article we publish.