- 1Departamento de Microbiología y Parasitología, Instituto de Acuicultura y Facultad de Biología-CIBUS, Universidade de Santiago de Compostela, Santiago de Compostela, Spain
- 2Centro de Investigacións Científicas Avanzadas (CICA) e Departamento de Química, Facultad de Ciencias, Universidade da Coruña, A Coruña, Spain
Vibrio neptunius is an inhabitant of mollusc microbiota and an opportunistic pathogen causing disease outbreaks in marine bivalve mollusc species including oysters and clams. Virulence of mollusc pathogenic vibrios is mainly associated with the production of extracellular products. However, siderophore production is a common feature in pathogenic marine bacteria but its role in fitness and virulence of mollusc pathogens remains unknown. We previously found that V. neptunius produces amphibactin, one of the most abundant siderophores in marine microbes. In this work, synthesis of the siderophore piscibactin was identified as the second siderophore produced by V. neptunius. Single and double mutants in biosynthetic genes of each siderophore system, piscibactin and amphibactin, were constructed in V. neptunius and their role in growth ability and virulence was characterized. Although the High Pathogenicity Island encoding piscibactin is a major virulence factor in vibrios pathogenic for fish, the V. neptunius wild type did not cause mortality in turbot. The results showed that amphibactin contributes more than piscibactin to bacterial fitness in vitro. However, infection challenges showed that each siderophore system contributes equally to virulence for molluscs. The V. neptunius strain unable to produce any siderophore was severely impaired to cause vibriosis in clams. Although the inactivation of one of the two siderophore systems (either amphibactin or piscibactin) significantly reduced virulence compared to the wild type strain, the ability to produce both siderophores simultaneously maximised the degree of virulence. Evaluation of the gene expression pattern of each siderophore system showed that they are simultaneously expressed when V. neptunius is cultivated under low iron availability in vitro and ex vivo. Finally, the analysis of the distribution of siderophore systems in genomes of Vibrio spp. pathogenic for molluscs showed that the gene clusters encoding amphibactin and piscibactin are widespread in the Coralliilyticus clade. Thus, siderophore production would constitute a key virulence factor for bivalve molluscs pathogenic vibrios.
Introduction
Bacteria belonging to the genus Vibrio (Vibrios) are ubiquitously distributed in the marine environment and are also a dominant fraction of bivalve microbiota (Vezzulli et al., 2018). Mollusc hemolymph is a critical site for the host immune response (Potgieter et al., 2015). Bivalve hemocytes can kill vibrios by phagocytosis and production of reactive oxygen species, highly reactive nitric oxide, antimicrobial peptides and hydrolytic enzymes (Destoumieux-Garzón et al., 2020). Interestingly, some Vibrios are part of the resident microbiota of bivalves as they persist in the hemolymph in the absence of an environmental source of population (Potgieter et al., 2015; Lokmer et al., 2016; Vezzulli et al., 2018; Zhang et al., 2018). The microbiota benefits the host as it boosts the immune system, and promotes reproduction, nutrition and defence mechanisms (Engel et al., 2002; McFall-Ngai et al., 2013; Cahill et al., 2016; Utermann et al., 2018). Nonetheless, under unfavourable conditions some bacteria are responsible for disease outbreaks. Among them, vibriosis is a serious epizootic disease caused by some Vibrio spp. that has become the most important limiting factor of the intensive fish and shellfish mariculture industry worldwide (Paillard et al., 2004; Toranzo et al., 2005; Travers et al., 2015; Dubert et al., 2017).
Vibrio species belonging to the Coralliilyticus and Orientalis clades are among the best-known species of bivalve pathogens (Dubert et al., 2017). They include V. neptunius, a marine bacterium that was isolated from marine water samples and animals such as turbot larvae (Scophthalmus maximus), rotifers (Brachiomus plicatilis) and larval stages of cephalopods (Octopus vulgaris) (Thompson et al., 2003; García-Amado et al., 2011; Farto et al., 2019). Notably, this bacterium is a relevant pathogen of aquacultured marine invertebrates, including artemia, oysters and clams (Prado et al., 2005; Kesarcodi-Watson et al., 2009a; Kesarcodi-Watson et al., 2009b; Romalde et al., 2014; Dubert et al., 2017). V. neptunius rapidly invades bivalve larvae tissues by entering them through the filtration feeding process. Its virulence is commonly associated with the production of thermolabile extracellular products with cytotoxic activity for fish and homeothermic animal cell lines (Dubert et al., 2016). Remarkably, vibriolysin-like protease VnpA and collagenase ColA were recently characterized as relevant virulence factors of V. neptunius since their production is required for full virulence in oyster larvae (Ostrea edulis) (Galvis et al., 2021).
It is well established that the production and utilization of siderophores is a key virulence factor for most vertebrate pathogenic bacteria (Kramer et al., 2020). However, although siderophore production would be a common feature of Vibrio pathogens affecting bivalves (Gómez-León et al., 2005; Mechri et al., 2017), its role in bacterial fitness and/or virulence is understudied in this type of bacterial pathogens. Our previous works demonstrated that V. neptunius produces a set of 9 amphibactin forms to overcome low-iron conditions. The gene cluster involved in its production and utilization was identified (genes absABDEF and abtABCDE) and the amphibactin outer membrane transporter gene abtA was characterized. It is noteworthy that abtA was regularly found in mollusc microbiota including some of the most devastating pathogens such as V. coralliilyticus and V. tubiashii. Interestingly, a V. neptunius ΔabsE mutant (impaired to synthesize amphibactins) showed a weak but not null siderophore activity in cell free supernatants, which could imply the production of a second siderophore. Besides, the putative role of amphibactins in virulence for bivalves was not yet evaluated (Galvis et al., 2020).
In the present work the siderophore piscibactin was identified as the second siderophore produced by V. neptunius. Single and double V. neptunius mutants in biosynthetic genes of each siderophore system, piscibactin and amphibactin, were constructed and their role in virulence determined. In addition, the gene expression patterns of each siderophore were studied in vitro and ex vivo. The results showed that both siderophore systems, amphibactin and piscibactin, are expressed during infection, playing a key role in the virulence of V. neptunius for clams.
Materials and Methods
Bacterial Strains, Plasmids, and Media
The bacterial strains and plasmids used, as well as those derived from this study, are listed in Table 1. V. neptunius and V. anguillarum strains were grown at 25°C in Tryptic Soy Agar and Broth (Pronadisa, Madrid, Spain) supplemented with 1% NaCl (TSA-1 and TSB-1, respectively), as well as in M9 minimal medium supplemented with 0.2% Casamino Acids (Difco) (CM9) (Lemos et al., 1988). Escherichia coli strains were grown at 37°C in Luria-Bertani (LB) medium (Pronadisa) or LB supplemented with the appropriate antibiotics. Ampicillin sodium salt was used at 100 µg/mL, kanamycin 50 µg/mL and gentamycin 15 µg/mL (final concentrations).
DNA Manipulations and Bioinformatics Tools
Total genomic DNA from V. neptunius PP-145.98 was purified with the InstaGene™ Matrix (BioRad, Hercules, California, CA, USA). PCR reactions were all carried out with Taq polymerase NZYTaq (Nzytech, Lisboa, Portugal) according to manufacturer protocol in a T-Gradient Thermal Cycler (Biometra, Göttingen, Germany). The extraction of DNA from agarose gels and purification of plasmid DNA were carried out using NucleoSpin Gel and a PCR clean-up kit (Macherey-Nagel, Düren, Germany) and a GeneJET Plasmid Miniprep Kit (Thermo-Fisher, Waltham, MA, USA).
The genome of V. neptunius PP-145.98 strain (accession number JAFHLB000000000) was screened, using antiSMASH 5.0 (Blin et al., 2019), for the presence of siderophore-related sequences. The NCBI services (http://ncbi.nlm.nih.gov) were used to consult the DNA and protein sequence databases with BLAST algorithm. Prediction of protein domains was carried out by using the Pfam protein families database (Finn et al., 2014). The sequences of housekeeping genes ftsZ, gyrB, mreB, pyrH y recA of bivalve pathogenic vibrios were downloaded from the NCBI database and used for multilocus sequence analysis (MLSA). Sequences of each gene were concatenated into a single 3880 bp sequence and aligned using the MUSCLE. The final phylogenetic tree was constructed based on concatenated sequences of the five housekeeping genes by maximum-likelihood (GTR+G model). Sequence alignments and phylogenetic tree were performed using MEGAX (v. 10.2.2) (Kumar et al., 2018).
Construction of absF and irp2 Mutants by Allelic Exchange
In-frame deletions of absF and irp2 in V. neptunius PP-145.98 were constructed by using PCR amplification of two fragments of each gene and flanking regions that, when ligated together, would result in an in-frame (nonpolar) deletion. The oligonucleotides used to amplify the upstream and downstream ends of each gene are shown in Table S1. Once deleted alleles were constructed by sequential cloning of the PCR products into pWKS30 plasmid, they were liberated by digestion with NotI and ApaI and cloned into the suicide vector pCAR109 (Mouriño et al., 2004). The resulting plasmids (Table 1) were mated from E. coli S17-1-λpir into V. neptunius PP-145.98 and transconjugants with the plasmid integrated in the chromosome by homologous recombination, were selected on TSA-1 containing 50 mg/mL of kanamycin (resistance conferred by pNidKan) and 100 mg/mL of ampicillin (antibiotic to select V. neptunius PP-145.98). A second recombination event was obtained by selecting for sucrose (10%) resistance. This process led to the generation of the V. neptunius PP-145.98 single mutants ΔabsF and Δirp2, and the double mutant ΔabsFΔirp2, named FG109, FG113 and FG115, respectively.
Growth Promotion and Siderophore Production Assays
Growth measurement of V. neptunius PP-145.98 strains was performed using 96-well microtiter plates. Each well contained 200 µL of CM9 medium (Lemos et al., 1988) supplemented with FeCl3 at 10 µM (PROBUS) or with the iron chelators ethylenediamine-di(o-hydroxyphenyl-acetic acid) (EDDA) at 5 µM or 2,2’–dipyridyl (dipyridyl) (Sigma) at 50 µM or 30 µM. Each well was inoculated with a 1:50 dilution of an overnight culture of the strain to be tested in TSB-1 at OD600 = 0.5. The plates were incubated at 25°C with shaking at 150 rpm. After 18 h of incubation, growth (OD600) was recorded in an iMACK Microplate reader (Bio-Rad). Bacterial cultures in CM9 with 30 µM 2,2’-dipyridyl and an OD600 ≈0.6 (after 6 h of incubation) were used to measure siderophore production using the chrome azurol-S (CAS) liquid assay (Schwyn and Neilands, 1987). Equal volumes of each cell free supernatant and CAS reagent were mixed and absorbance at 630 nm (A630) was measured in a UV-VIS spectrophotometer (Hitachi) after 15 min of incubation at room temperature.
Cross-Feeding Assays
The biological activities of the supernatants produced by the parental and mutant strains were determined by cross-feeding experiments. We tested the ability of culture supernatants from V. neptunius PP-145.98 mutants defective in piscibactin and amphibactin synthesis to cross-feed different indicator strains defective in the synthesis and/or transport of piscibactin.
To test whether V. neptunius wild type or derivative mutants produce piscibactin, a cross-feeding assay was conducted using two V. anguillarum mutants derived from RV22 strain that lack siderophore synthesis: RV22ΔvabD, a single mutant (strain MB67) that does not produce siderophores and that it is able to use piscibactin as iron source since it carries the piscibactin outer membrane transporter FrpA; and RV22ΔvabDΔfrpA, a double mutant that does not use piscibactin since it has inactivated the piscibactin transporter FrpA. Indicator strains were inoculated into CM9 plates as follows: 0.5 mL of an overnight culture in TSB-1 at an OD600 = 0.5 were mixed with 5 mL of CM9 medium containing 0.5% agarose and 2,2’-dipyridyl 100 µM, a concentration close to the Minimal Inhibitory Concentration (MIC) and at which growth halos can be easily visualized (Balado et al., 2006). The V. neptunius strains to be tested for piscibactin production were cultured in TSA-1 plates and the cells were harvested with a sterile loop and placed on the surface of the plates inoculated with the V. anguillarum strains. A piscibactin producer V. anguillarum strain (RV22 ΔvabF) was used as control. The presence of growth halos of the V. anguillarum indicator strains around cells of V. neptunius after overnight incubation at 25°C was indicative of piscibactin production.
RNA Purification and RT-PCR
The organization of amphibactin genes into operon(s) was tested by reverse transcription PCR. V. neptunius PP-145.98 was grown until exponential phase (ca. OD600 = 1) in 10 mL CM9 medium containing 2.5 µM EDDA. Cells were pelleted by centrifugation at 10,000 × g for 2 min and total RNA was isolated with Trizol (Invitrogen) following the manufacturer’s recommendations. RT-PCR was performed with 1 µg RNA pre-treated with RQ1 RNase-Free DNase (Promega) by using the M-MLV reverse transcriptase (Invitrogen). A primer located at the 3’-end of absA gene was used to obtain a cDNA that was used as template for subsequent PCR reactions targeted in abtE (PCR2) and in the region between abtC and abtD (PCR1), absE and absF (PCR3) and between abtA and absB (PCR4) (Table S1). A negative control reaction was performed with total RNA treated with DNase without M-MLV reverse transcriptase to confirm the lack of genomic DNA contamination in each reaction mixture. The PCR positive control reaction was done using 100 ng of genomic DNA as template.
lacZ Transcriptional Fusions and β-Galactosidase Assays
The DNA fragments corresponding to the amphibactin and piscibactin promoter regions of V. neptunius PP-145.98 were amplified by PCR. The PCR products spanned from the first nucleotides of the coding sequence to ca. 700-900 bp upstream sequence. These putative promoter regions were fused to a promoterless lacZ gene in the low-copy-number reporter plasmid pHRP309 (Parales and Harwood, 1993). Transcriptional fusions of genes abtC and abtA for amphibactin, and araC1 and frpA for piscibactin were constructed. The resulting transcriptional fusion constructs, abtC::lacZ (pFG156), abtA::lacZ (pFG180), arac1::lacZ (pFG176) and frpA::lacZ (pFG188), were mobilized from E. coli S17-1-λpir into V. neptunius PP-145.98 by conjugation. V. neptunius PP-145.98 derivatives carrying the transcriptional fusions were grown in CM9 at 25°C under conditions of iron availability (CM9 with 10 µM Fe2(SO4)3) and iron deficiency (CM9 with 50 µM 2,2’-dipyridyl). For the ex vivo assay V. neptunius PP-145.98 derivatives carrying the promoter-lacZ fusions were grown at 25°C in the hemolymph of commercial mussels (Mitylus galloprovincialis) under normal or excess iron conditions. Hemolymph was collected from approximately 30 mussels by puncture of the adductor anterior muscle, then centrifuged at 4,000 rpm for 10 min and filtered through a nitrocellulose membrane with a pore size of 0.25 µm. The β-galactosidase (LacZ) activity in CM9 cultures and hemolymph were measured by the method of Miller (Miller, 1992). Results showed are means of three independent experiments, each one measured in triplicate. Statistical significance of differences was determined using t-test. P-values were considered significant when P was <0.05.
Virulence Assays
Experimental infections in healthy clams (Ruditapes philippinarum) were performed with the wild-type and mutants of V. neptunius PP-145.98. For acclimatization the clams (mean size of 10 mm) were kept for 2 days in a seawater tank at 20°C with continuous aeration. The wild-type strain and the mutants ΔabsF, Δirp2 and ΔabsFΔirp2 of V. neptunius PP-145.98 were cultured in CM9 medium with 2,2’-dipyridyl 20 µM for 12 h at 25°C to achieve an OD600 = 0.6. The infection was carried out by bath as follows: groups of 50 clams were introduced in 50 mL of seawater containing the bacterial strain to be tested at a final concentration of 106 cfu/mL, and incubated for 24 h. The clams were then washed twice with abundant seawater and placed in containers with 200 mL of seawater with aeration. The assay was performed by duplicate and a group of clams under the same conditions but without bacterial inoculation was used as negative control. Pathogenicity was evaluated after 96 h and was expressed as a percentage of survival. Mortalities were recorded daily, and the statistical significance of differences in percentage of survival for the different V. neptunius strains was determined using the Kaplan–Meier method with the Mantel–Cox log-rank test using SPSS (version 20; IBM SPSS Inc., Chicago, IL, USA). P-values were considered significant when P was <0.05, <0.01 and <0.001.
Virulence assays on fish were performed using turbot (Scophthalmus maximus) fingerlings weighting 5 g on average. The fish were divided into groups of 10 animals. All groups, one per strain tested and a control group, were maintained in 50 L seawater tanks at 18°C with continuous aeration and water recirculation. The wild-type strain and mutants were grown in CM9 medium for 12 h at 25°C to reach an OD600 = 0.6. The inoculum used was a 10-fold dilution of this suspension in saline solution (0.85% NaCl in distilled H2O). Fish were inoculated intraperitoneally (ip) with 100 µL of bacterial suspensions. A control group was inoculated with 100 µL of saline solution. Mortalities were recorded daily for 15 days after injection. All animal experimentation protocols used in this study were reviewed and approved by the Animal Ethics Committee of the University of Santiago de Compostela (protocol id. 15004/14/003).
Piscibactin Detection by Mass Spectrometry
The presence of piscibactin was studied following the SPE-HLB/HPLC-MS methodology previously described (Souto et al., 2012; Balado et al., 2018). Briefly, V. neptunius ΔabsF was grown in CM9 medium supplemented with 30 µM 2,2’–dipyridyl at 25°C under continuous shaking (150 rpm) for 24 h. Once achieved an OD600 = 1, the bacterial culture was centrifuged at 10,000 × g for 10 min (Beckman J-21 High Speed Centrifuge) and filtrated through a 0.45-μm pore size membrane. 800 mL of the resultant cell-free supernatant were treated with 17 mg of FeCl3, incubated at 4°C for 12 h, and concentrated under reduced pressure to 300 mL. Half of this volume was fractionated using an Oasis® hydrophilic lipophilic balance (HLB) cartridge (Waters) (35 cm3, 6 g), previously conditionated with 60 mL of acetonitrile (ACN, solvent B) and deionized water (H2O, solvent A), in three batches of 75 mL. Each batch was fractionated with 30 mL of the following solvent mixtures: 1:0, 7:3, 1:1, 3:7, and 0:1 of A:B (v/v) obtaining the fractions VNΔabsFH1-5 respectively. VNΔabsFH3 was analysed by HPLC/HRMS in a HPLC Acela (Thermo) coupled to a PDA and HRMS (LQT-Orbitrap Discovery) detector in full positive ion using the Atlantis dC18 (100 x 4.6 mm, 5 µm) column (Waters) and the following method (solvent A: H2O, solvent B: ACN): 35 min from 10% to 100% of B, 5 min isocratic at 100% of B and 10 min from 100 to 10% of B at 1 mL min-1. The results showed the presence of ferri-piscibactin in the chromatographic peak with a Rt=10.69 min displaying the [M+H]+ adduct at m/z 507.0032 (calcd. for C19H21N3O4S3Fe+ m/z 507.0038) and the absorbance maxima at 227, 256, 307, and 388 nm in its UV spectrum.
Results
V. neptunius Genome Harbors a Version of the High Pathogenicity Island (irp-HPI) Encoding Piscibactin Biosynthesis and Transport
In silico analysis of V. neptunius PP-145.98 genome sequence (NZ_JAFHLB010000000) led to the identification of a gene cluster with high homology to irp genes previously identified in P. damselae subsp. piscicida and several Vibrios. These genes are harbored in the High Pathogenicity Island named irp-HPI and confer the ability to produce and use the siderophore piscibactin (Osorio et al., 2015) (Figure 1). The genomic island irp-HPI of V. neptunius (irp-HPIVnep) is a DNA region of approximately 33 kb that is located in the chromosome II between a tRNA gene and the flagellum operon (Figure 1). irp-HPIVnep shares identical structure and genetic organization with the piscibactin gene cluster present in P. damselae subsp. piscicida (KP100338) and V. anguillarum RV22 (AEZB01000000) (Souto et al., 2012; Osorio et al., 2015; Balado et al., 2018). In addition, the irp-HPIVnep predicted products showed an amino acid similarity between 71 and 82% with the irp cluster ortholog from P. damselae subsp. piscicida, and between 54% and 65% with those from V. anguillarum RV22 (Figure 1 and Table S2). Although V. neptunius irp-HPI shows higher similarity to P. damselae subsp. piscicida genomic island than to V. anguillarum, V. neptunius and V. anguillarum irp-HPI genomic islands share a series of characteristics. Among them, they do not include a dahP and probable Fur box motifs are found upstream of araC1 and frpA (Figure 1). To ascertain whether the irp-HPIVnep genes are expressed, a series of retro-transcriptase nested PCR reactions were performed. A PCR targeted to araC1 gene showed the existence of an mRNA covering from araC1 to irp5 (data not shown) indicating that the gene cluster is transcribed in a polycistronic mRNA. The same result was found for the piscibactin gene cluster of P. damselae subsp. piscicida and V. anguillarum (Balado et al., 2018).
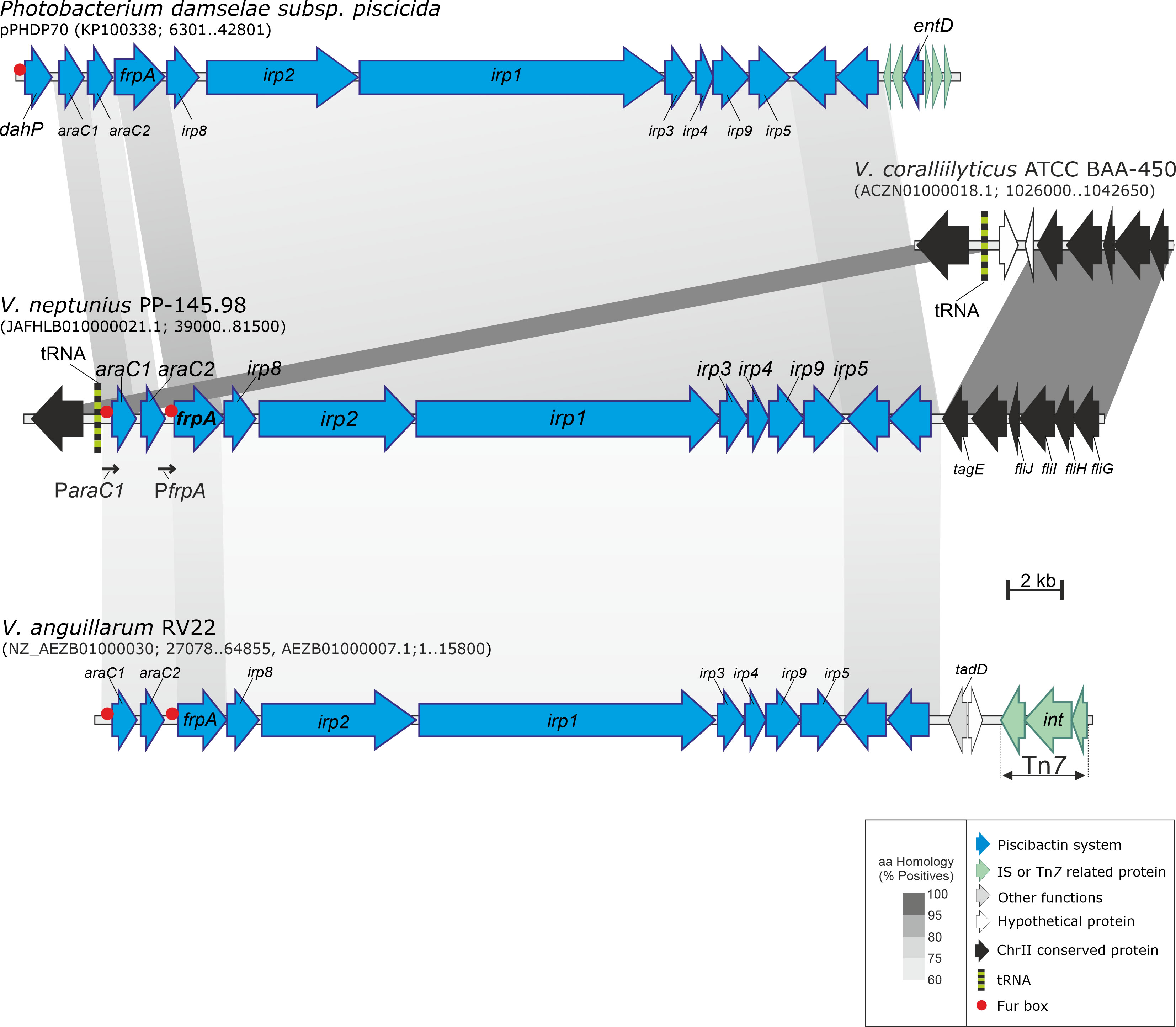
Figure 1 Genetic map of the gene cluster encoding the piscibactin system in V. neptunius. The piscibactin genes, which are part of V. angullarum RV22 chromosome II and the plasmid pPHDP70 of P. damselae subsp. piscicida are included for comparative purposes. Grey blocks indicate percentages of similarity in the proteins sequence.
The non-ribosomal peptide synthetases (NRPSs) require a phosphopantetheinyl transferase (PPTase) to be active (Orikasa et al., 2006) but irp-HPI genomic islands do not include this function (Osorio et al., 2015; Balado et al., 2018). In silico search showed the existence of up to two probable PPTases (loci WP_206370543.1 and WP_206368753.1), whose predicted products are homologous to several groups of PPTases containing all conserved residues required to be functional (Figure 2) (Lambalot et al., 1996; Liu et al., 2005).
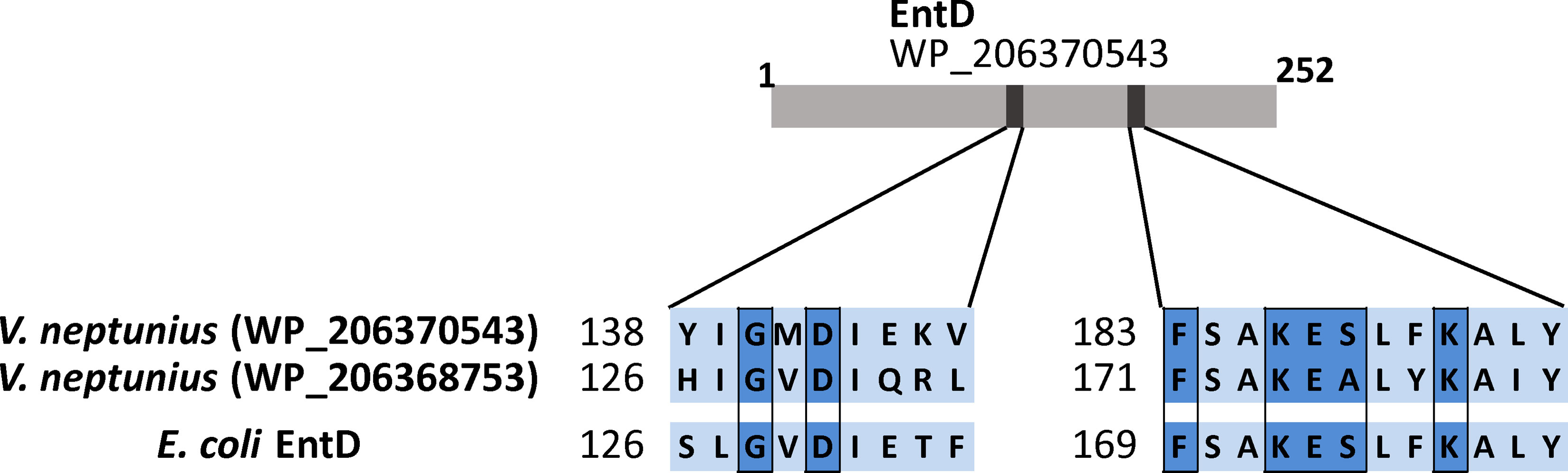
Figure 2 Highly conserved residues of the type-II PPTases shared between the two V. neptunius predicted proteins and the EntD of E. coli.
Inactivation of irp-HPI Genes Reduces the Growth Ability of V. neptunius Under Iron Restriction
To test whether irp-HPI genes mediate siderophore synthesis in V. neptunius PP-145.98, single and double mutants in irp2 and absF genes were constructed. irp2 and absF encode NRPSs required for piscibactin and amphibactin synthesis, respectively (Souto et al., 2012; Galvis et al., 2020). The growth capacity and siderophore production of each mutant were evaluated under different iron availability conditions and their phenotypes were compared to the parental strain (Figure 3). V. neptunius wild type strain and all derivative mutants showed indistinguishable growth ability under iron excess (CM9 plus 10 μM ferric chloride). By contrast, under iron-restricted conditions some differences were observed. Iron-restricted conditions were achieved by adding to the minimal medium CM9 the strong iron chelating agent EDDA or the weaker chelator dipyridyl (McMillan et al., 2010). The V. neptunius Δirp2ΔabsF double mutant showed a MIC of EDDA of 5 µM while the MIC of dipyridyl was 50 µM. By contrast, the growth ability of the wild type strain under weak or strong iron-restriction (CM9 plus dipyridyl 50 μM or EDDA 5 μM, respectively) was almost the same as under iron-excess. Interestingly, some differences were observed between growth of ΔabsF and Δirp2 single mutants under iron-restriction. Addition of the weak iron chelator dipyridyl at 50 μM significantly reduced the growth of the Δirp2 mutant, but it did not affect the growth of the ΔabsF mutant. Under strong iron-restricted conditions both single mutants showed reduced growth, ca. 60% in the Δirp2 mutant and ca. 70% in the ΔabsF mutant. Finally, siderophore activity present in cell free supernatants, measured by the CAS assay, after growth of each V. neptunius strain in non-restrictive conditions (CM9 plus dipyridyl at 30 μM) showed that siderophore production was almost abolished in the Δirp2ΔabsF double mutant. By contrast, although single inactivation of Δirp2 or ΔabsF showed lower siderophore content in supernantants compared to wild type, the differences were not statistically significant (Figure 3).
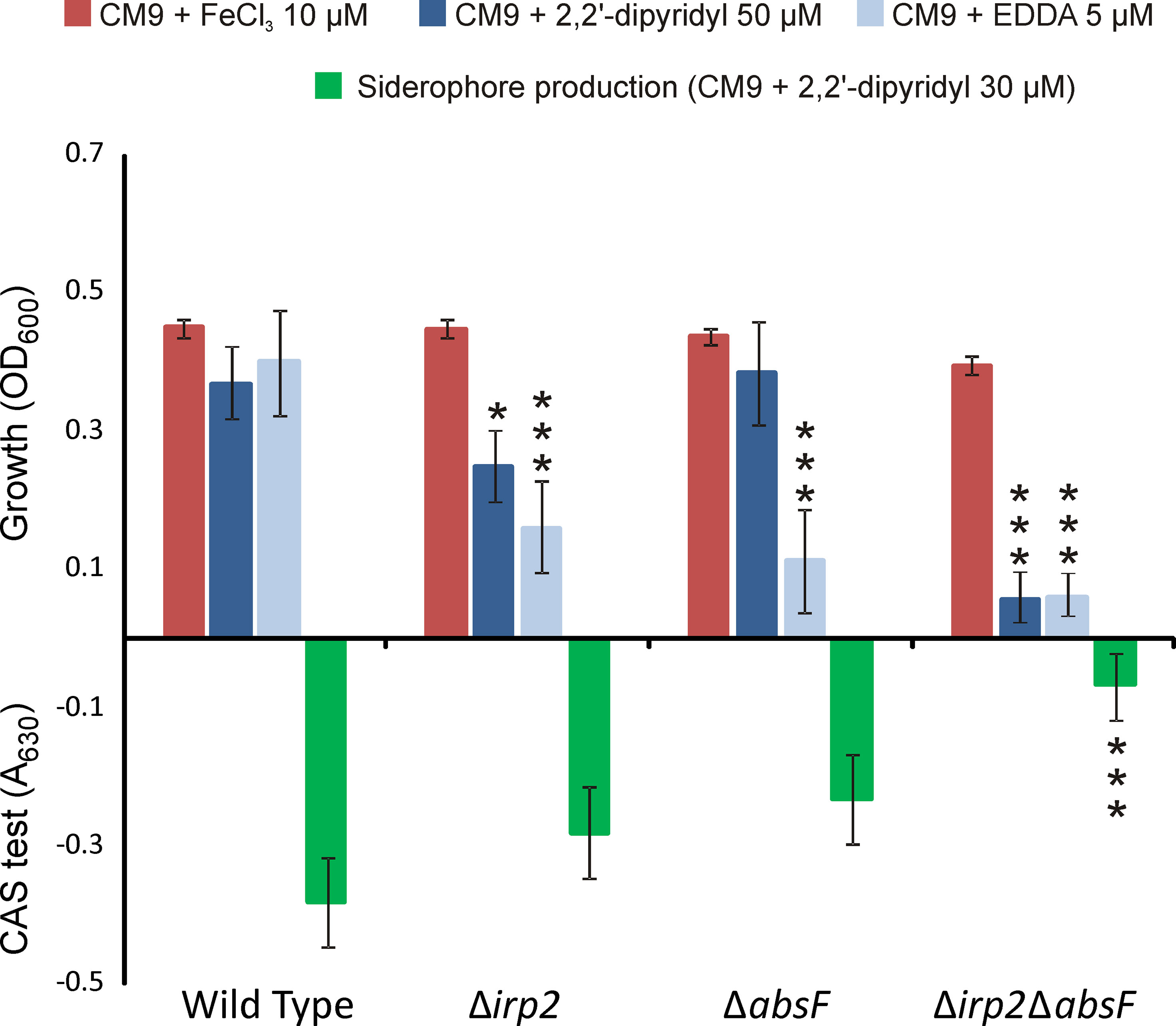
Figure 3 Growth levels under low and high-iron availability and siderophore production (CAS assay) of V. neptunius PP-145.98 wild type and its derivative mutants ΔabsF, Δirp2 and Δirp2-ΔabsF. Asterisks denote statistically significant differences with the wild type strain (*P < 0.05 and ***P < 0.001).
To test whether V. neptunius wild type and mutant strains produced piscibactin, a series of cross-feeding assays were performed. A V. anguillarum FrpA+ (ΔvabD) strain that has a functional piscibactin transporter FrpA, (can use piscibactin as iron source) and a FrpA– (ΔvabDΔfrpA double mutant) that cannot use piscibactin since it lacks FrpA, were used as indicator strains. Both indicator strains do not produce any siderophore due to the inactivation of the PPTase gene vabD (Balado et al., 2018). The results showed that the V. neptunius wild-type strain and the ΔabsF single mutant promoted the growth of V. anguillarum FrpA+, but they did not cross-feed the FrpA– indicator strain. Congruently, neither V. neptunius Δirp2 nor Δirp2ΔabsF mutants induced the growth of FrpA+ (Figure 4).
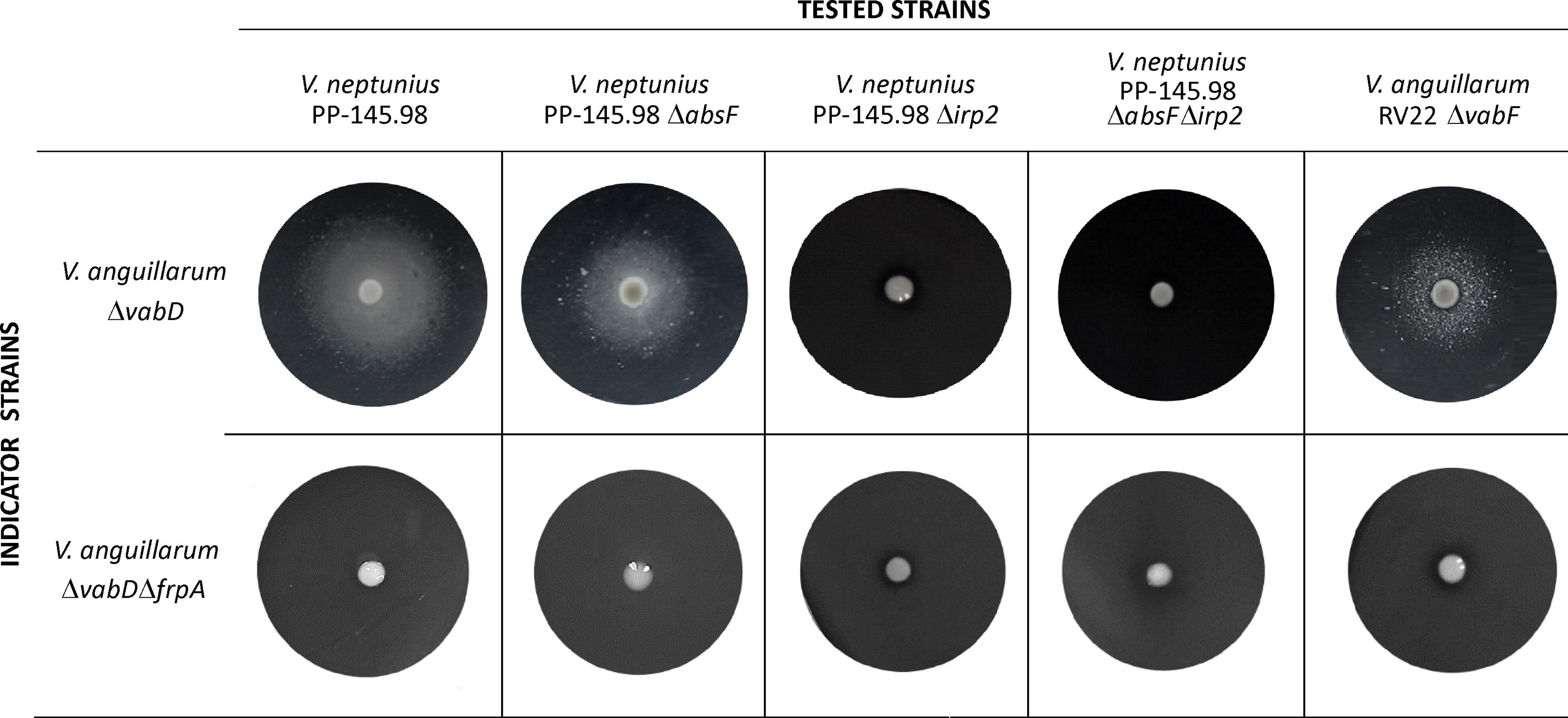
Figure 4 Cross feeding assay to test the production of piscibactin by V. neptunius 145.98 wild type strain and its derivative ΔabsF and Δirp2 single mutants and Δirp2ΔabsF double mutant. Indicator strains were inoculated within the CM9 plates containing 100 µM of 2,2’-dipyridyl. Tested strains were placed as a loop full of bacterial biomass on the agar surface. The presence of a growth halo shows that the indicator strains can use the siderophores produced by the tested strains to overcome the iron limitation.
To prove that V. neptunius 145.98 synthesizes piscibactin in addition to amphibactin, cell free supernatants from ΔabsF single mutant were studied following the SPE-HLB/HPLC-HRMS methodology previously described by our research group (Souto et al., 2012). Cell-free supernatant was treated with FeCl3, to obtain the stable ferri-siderophores, and fractionated using an HLB cartridge. HPLC/HRMS analysis of the CAS-positive fraction VNΔabsFH3, eluted with 1:1 of ACN:H2O, confirmed the presence of ferri-piscibactin in the chromatographic peak at Rt=10.69 min (Figures 5A, B) by comparison of its MS and UV spectra with the previously described data (Souto et al., 2012). Specifically, the mass spectrum (MS) of this peak showed the [M + H]+ adduct at m/z 507.0032 (calcd. for C19H21N3O4S3Fe+, m/z 507.0038) along with the isotopic distribution of Fe (Mr = 54, 56, 57, 58; ratio 0.6:9.2:0.2:0.02) (Figure 5D); while the UV spectrum displayed the absorbance maxima at 227, 256, 307, and 388 nm (Figure 5C).
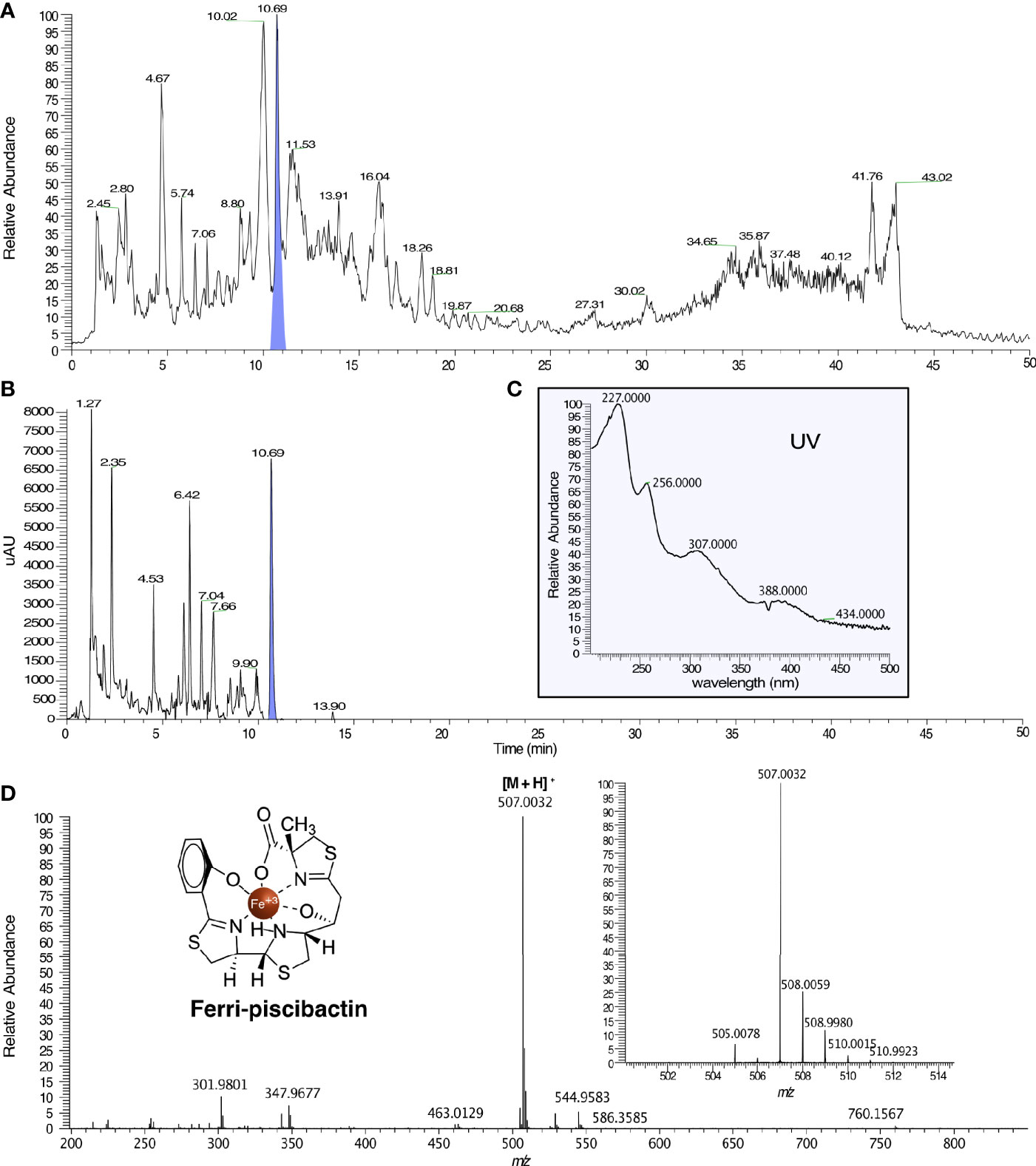
Figure 5 HPLC/HRMS analysis for the detection of ferri-piscibactin in the fraction VNΔabsFH3, from the V. neptunius ΔabsF mutant, eluted with 1:1 H2O:ACN from a HLB cartridge. (A) Total Ion Current (TIC) chromatogram of VNΔabsFH3 in which ferri-piscibactin was detected at Rt = 10.69 min, highlighted in blue. (B) HPLC-DAD chromatogram of VNΔabsFH3 in which ferri-piscibactin was detected at Rt = 10.69 min, highlighted in blue. (C) UV spectrum of the peak at Rt = 10.69 min, where ferri-piscibactin was detected, showing the absorption maxima at 207, 256, 307 and 388 nm. (D) (+)-HRESIMS of the peak at Rt 10.69 min, where ferri-piscibactin was identified, displaying: m/z 507.0032 (calcd. for C19H21N3O4S3Fe+, m/z 507.0038), expanded region of MS in the range m/z 501-514, showing the presence of the characteristic Fe isotopic distribution, and structure of ferri-piscibactin.
All these results demonstrate that each one of the V. neptunius single mutants (Δirp2 or ΔabsF) produce one unique siderophore when cultivated under iron-restricted conditions. Thus, while V. neptunius Δirp2 mutant produces only amphibactin and ΔabsF produces only piscibactin, the Δirp2ΔabsF double mutant does not produce any of these siderophores.
Piscibactin and Amphibactin Significantly Contribute to V. neptunius Virulence for Clams
To study the role of piscibactin and/or amphibactin production in virulence of the bivalve mollusc pathogen V. neptunius, experimental infection challenges were performed. For this purpose, groups of 50 clams larvae were inoculated with the V. neptunius wild type or with one of the mutants. A 100% mortality was obtained in the group inoculated with V. neptunius wild-type strain 4 days after the challenge (Figure 6A) and no mortality was observed in the non-challenged control group. Interestingly, mortality registered after inoculation with ΔabsF or Δirp2 single mutants decreased up to 75-80% (Figure 6A), which represents statistically significant differences with respect to the group inoculated with the wild type strain. Most notably, mortality was strongly reduced in the clams group inoculated with the ΔabsFΔirp2 double mutant, where almost 80% of larvae survived to the challenge (Figure 6A). To test the possible virulence of V. neptunius wild type for fish, an experimental infection was done also in fish, using turbot fingerlings. In this case, there was not registered any mortality (Figure 6B), which showed that V. neptunius PP-145.98 wild type strain is pathogenic only for bivalve molluscs.
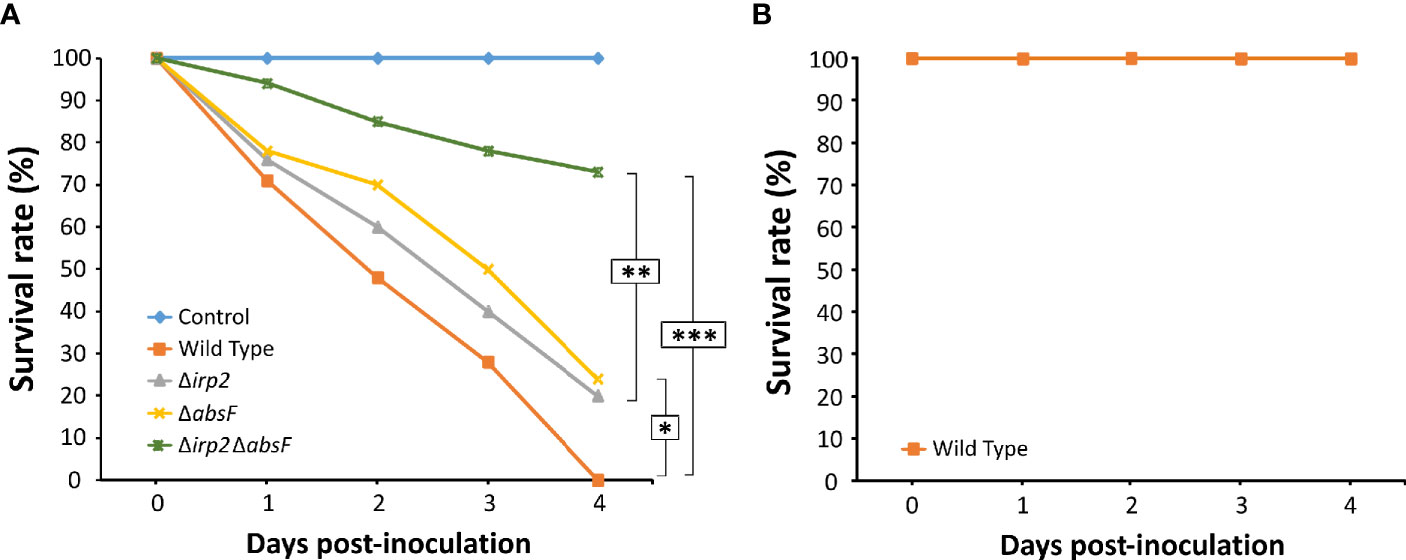
Figure 6 Survival curves after infection challenge in clams (Ruditapes philippinarum) (A) and turbot (B) with V. neptunius wild-type strain and its derivatives ΔabsF, Δirp2 and Δirp2ΔabsF mutants. Asterisks denote statistically significant differences between strains (*P < 0.05; **P < 0.01 and ***P < 0.001).
Amphibactin Gene Cluster Transcriptional Organization
Although amphibactin is one of the most abundant siderophores in the seawater (Boiteau et al., 2016; Gauglitz et al., 2021), transcriptional organization of amphibactin genes was not studied to date. To define the transcriptional organization of amphibactin genes, a series of reverse transcriptase PCR reactions (RT-PCRs) were performed. Results are shown in Figure 7. Since all amphibactin genes are encoded in the same DNA strand (Figure 7A), a reverse transcriptase reaction was done by using a primer targeted on absA, the last gene of the cluster (Figure 7A). Positive amplification was found in a PCR targeted into the abtC gene using previously obtained cDNA as template. This result clearly shows that amphibactin genes are transcribed into a polycistronic mRNA spanning from abtC to absA genes. Consequently, they must be transcribed from a divergent promoter located in the entD-abtC intergenic region (Figure 7B). Nevertheless, this result does not rule out the possibility that additional promoters exist within the gene cluster driving independent transcription of some genes. In silico analysis of the intergenic sequences of the amphibactin cluster suggests the existence of two putative FUR box motifs: one located 113 bp upstream of abtC start codon (GCAAACCATTTTCATTTGC) and another one located 83 bp upstream of abtA (absF-abtA intergenic region) (GATAACCATTATTATCATTAGC) (Figure 7A). AbtA was previously characterized as the TonB-dependent outer membrane transporter required for ferri-amphibactin internalization (Galvis et al., 2020). These Fur box motifs showed an identity of 58 and 79%, respectively, to the FUR box motif consensus sequence of E. coli (GATAATGATAATCATTATCATTATC) (Ochsner and Vasil, 1996; Payne et al., 2016). Thus, the existence of a promoter upstream of abtA controlling the expression of amphibactin transporter will be further studied.
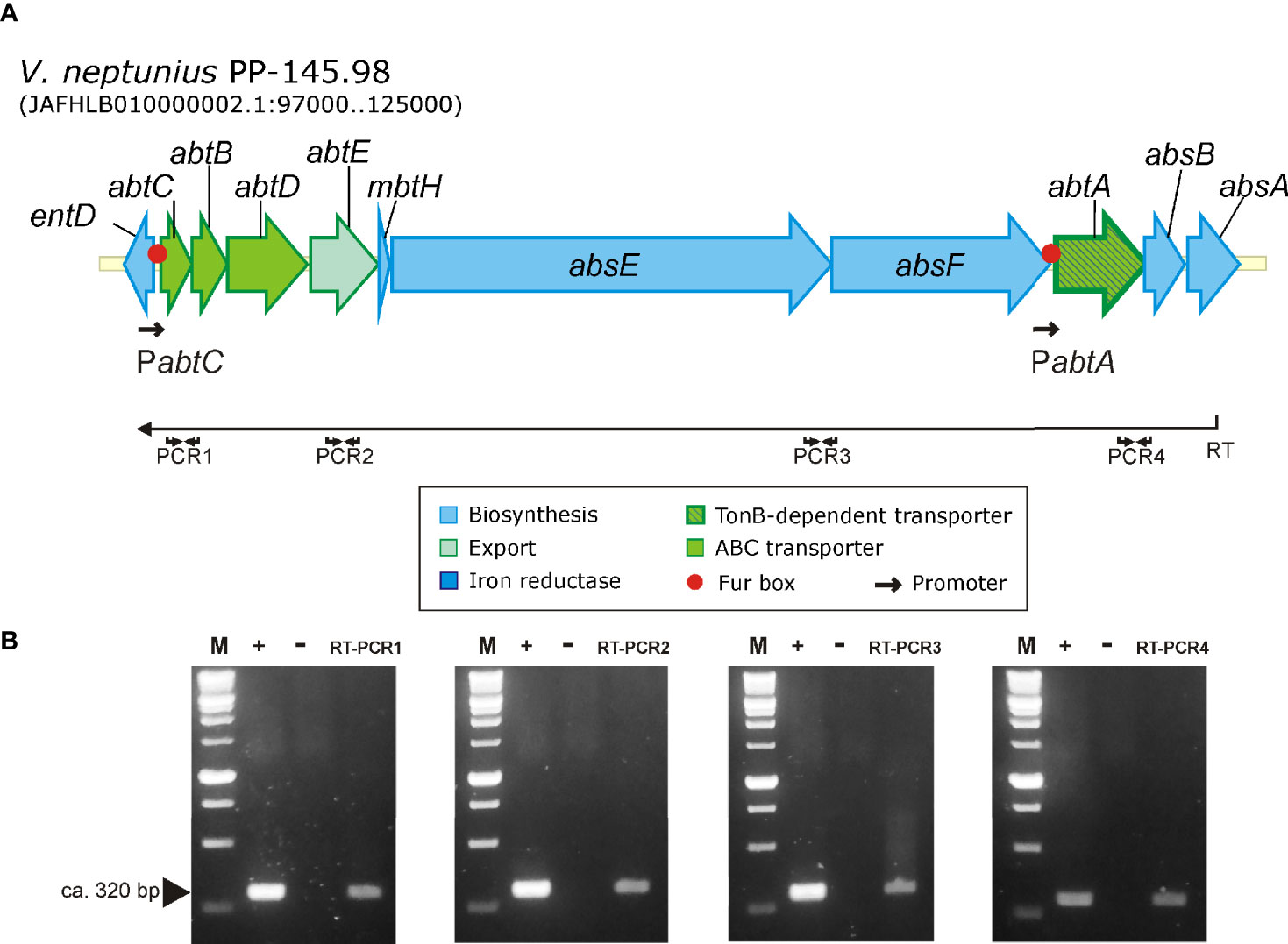
Figure 7 Results of RT-PCR reactions designed to analyze the transcription of the amphibactin gene cluster in V. neptunius PP-145.98. (A) Genetic map of the gene cluster encoding the amphibactin in V. neptunius PP-145.98. (B) An appropriate primer (Primer RT, Table S1), located at the 3’-end of absA gene, was used to obtain a cDNA (denoted as RT in the figure) that was then used as template for 4 PCR reactions targeted between abtC 3’ -end and 5’ -end abtD (PCR1), abtE (PCR2), between absE 3’ -end and absF 5’ -end (PCR3), and between abtA 3’ -end and absB 5’ -end (PCR4). M, size marker from 100 to 1,000 bp; +, positive control PCR using genomic DNA as template; −, negative control PCR using total RNA without reverse transcriptase. RT-PCR1-4 shows the amplification result of the 4 different RT-PCRs.
Both Siderophore Systems, Amphibactin and Piscibactin, Are Significantly Expressed When V. neptunius Is Cultivated Under Iron Deficiency
To evaluate the transcription levels of both amphibactin and piscibactin siderophore systems, the promoter regions of each siderophore gene cluster were cloned into the promoterless plasmid pHRP309 upstream of the lacZ gene. Resulting plasmids were mobilized into V. neptunius PP-145.98 wild type strain and the transcription levels of each promoter were evaluated in vitro and ex vivo (Figure 8A). To identify amphibactin promoters, fusions of the putative promoters abtA, abtC, and the sequence upstream of absE were evaluated by measuring β-galactosidase activity. These regions were named PabtA, PabtC and PabsE, respectively (Figure 8A). On the other hand, since ParaC1 and PfrpA were previously characterized as the promoters that control piscibactin expression in V. anguillarum (Balado et al., 2018), lacZ fusions of the V. neptunius sequences upstream of frpA and araC1 (PfrpA and ParaC1) were also obtained. The PabtA promoter cloned in reverse orientation was used as negative control and its transcriptional levels were almost undetectable under all the growth conditions tested (data not shown).
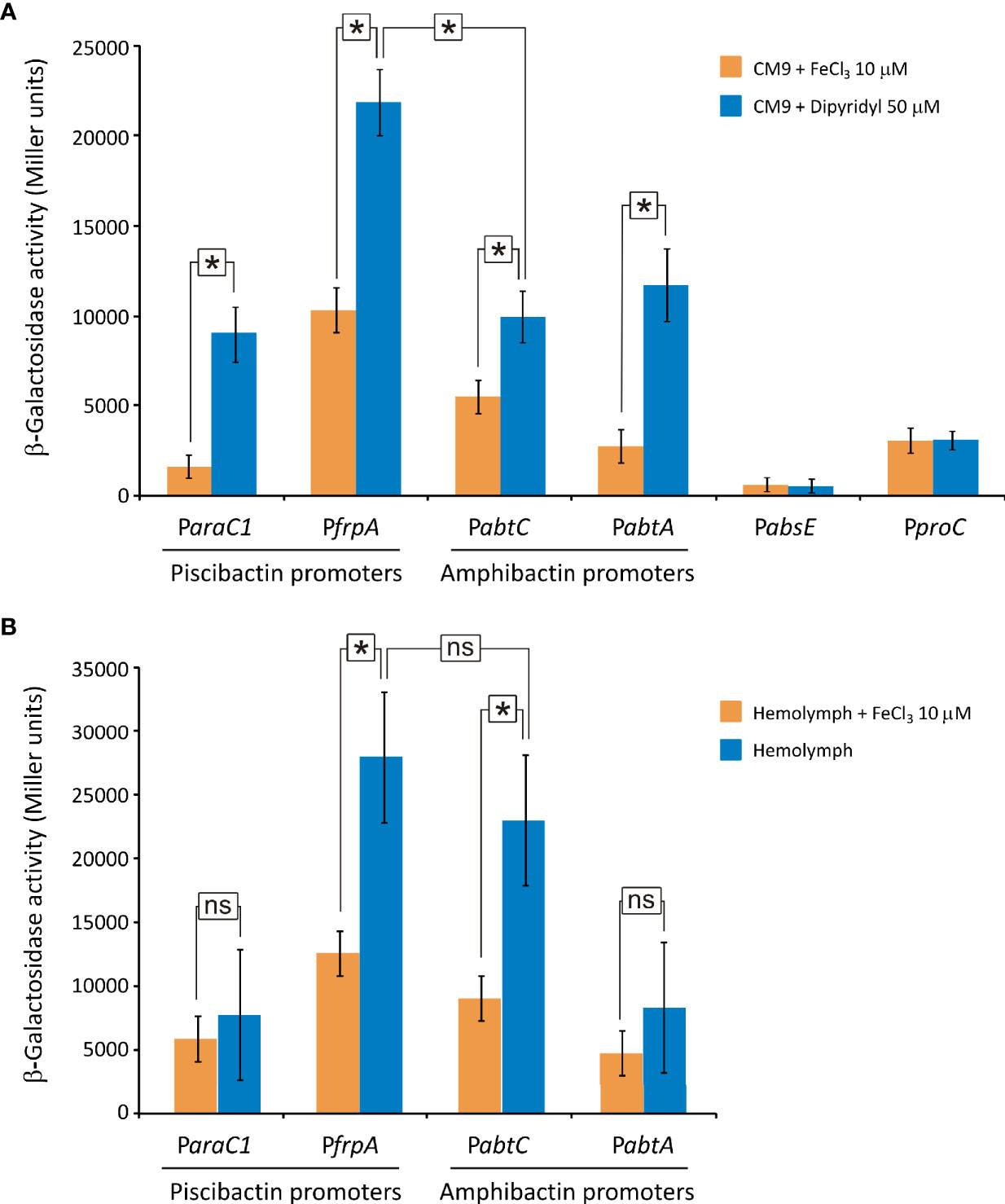
Figure 8 Transcriptional activity (β-galactosidase units) of piscibactin (ParaC1 and PfrpA) and amphibactin (PabtC and PabtA) promoters carried out using lacZ fusions. Expression assay was performed in vitro by cultivating V. neptunius PP-145.98 in CM9 minimal medium supplemented with FeCl3 10 µM (iron excess) or 2,2’-dipyridyl 50 µM (iron deficiency) (A) and ex vivo by cultivating bacteria in mussel hemolymph (B). PabtA promoter cloned in reverse orientation upstream of the lacZ gene was used as a negative control. A lacZ fusion with the constitutive promoter proC (PproC) was used as control. Asterisks indicate statistically significant differences between bars (P < 0.05); ns, no statistically significant differences. Error bars denote standard deviations.
Piscibactin promoters (ParaC1 and PfrpA) as well as amphibactin promoters PabtC and PabtA were assayed under low-iron availability in vitro (CM9 with 50 μM dipyridyl). The results show that all these promoters displayed high β-galactosidase activities (Figure 8A). However, the sequence upstream of absE showed an almost undetectable β-galactosidase activity under all the conditions tested (Figure 8A). Thus, the presence of an independent promoter upstream of the biosynthetic gene absE was discarded. It is noteworthy that while piscibactin promoter PfrpA achieved an activity of ca. 22,000 U under low iron availability, the activity of PabtC, PabtA and ParaC1 were 50% lower (ca. 10,000 U). These results would suggest that, under iron deficiency, the expression of piscibactin genes would be predominant. As expected, the addition of 10 μM FeCl3 to the culture medium greatly reduced (>40%) the transcriptional activity of amphibactin and piscibactin promoters, indicating that the two siderophore systems are significantly less expressed when iron availability increase (Figure 8A). The transcriptional activity of the constitutive promoter proC was independent of iron concentration.
To assess the expression of the siderophore systems during bacteria-bivalve interaction, an ex vivo assay was performed by the incubation of V. neptunius lacZ fusion carrier strains in mussel hemolymph (Mytilus galloprovincialis). Interestingly, the expression pattern registered ex vivo (Figure 8B) showed some differences with that observed in vitro (Figure 8A). Specifically, the transcriptional activity of the amphibactin promoter PabtC was 2-fold higher when incubated in hemolymph, achieving ca. 25,000 U. Thus, while the piscibactin promoter PfrpA showed in vivo a notable higher expression than amphibactin promoter PabtC, there were no statistically significant differences between them ex vivo. Addition of 10 μM FeCl3 to the hemolymph also significantly reduced transcriptional activity of both siderophore systems, which suggests that iron limitation must be a signal that bacteria detect when entering the host (Figure 8B).
Piscibactin and Amphibactin Are Present in Most Pathogenic Vibrio Species of the Corallilyticus Clade
To evaluate the distribution of amphibactin and piscibactin siderophore systems in species of Vibrio with importance in bivalve aquaculture, a total of 234 genomes of Vibrio species belonging to the Coralliilyticus, Anguillarum, Harveyi, Orientalis, Pectenecida and Splendidus clades were tested in silico for the presence of complete amphibactin and piscibactin gene clusters. These species were selected since they are commonly reported as bacteria associated with mortality events in larvae and spats of bivalves hatcheries and their virulence for bivalves is well-established (Beaz-Hidalgo et al., 2010; Dubert et al., 2017). The results (Figure 9 and Table S3) showed that most Vibrio genomes belonging to the Coralliilyticus clade harbor complete gene clusters encoding amphibactin and piscibactin siderophore systems. All V. neptunius, V. ostreicida and V. coralliilyticus genomes available in GenBank harbor close homologues of amphibactin genes, sharing identical structure and an average identity of 99%, 67% and 84%, respectively, with those found in V. neptunius PP-145.98 strain. The genomic island irp-HPI is present in all V. neptunius and V. ostreicida genomes sequenced and also in most V. coralliilyticus (18 of the 29). These results altogether suggest that most Vibrio species that belong to the Coralliilyticus clade harbor close homologues of both amphibactin and piscibactin siderophore systems. In addition, the amphibactin system is also widespread among vibrios of the Orientalis clade (V. bivalvicida, V. europeus and V. tubiashii). Finally, among vibrios of the Splendidus clade, 9.5% of V. splendidus and 25% of V. tasmaniensis genomes also harbor amphibactin genes.
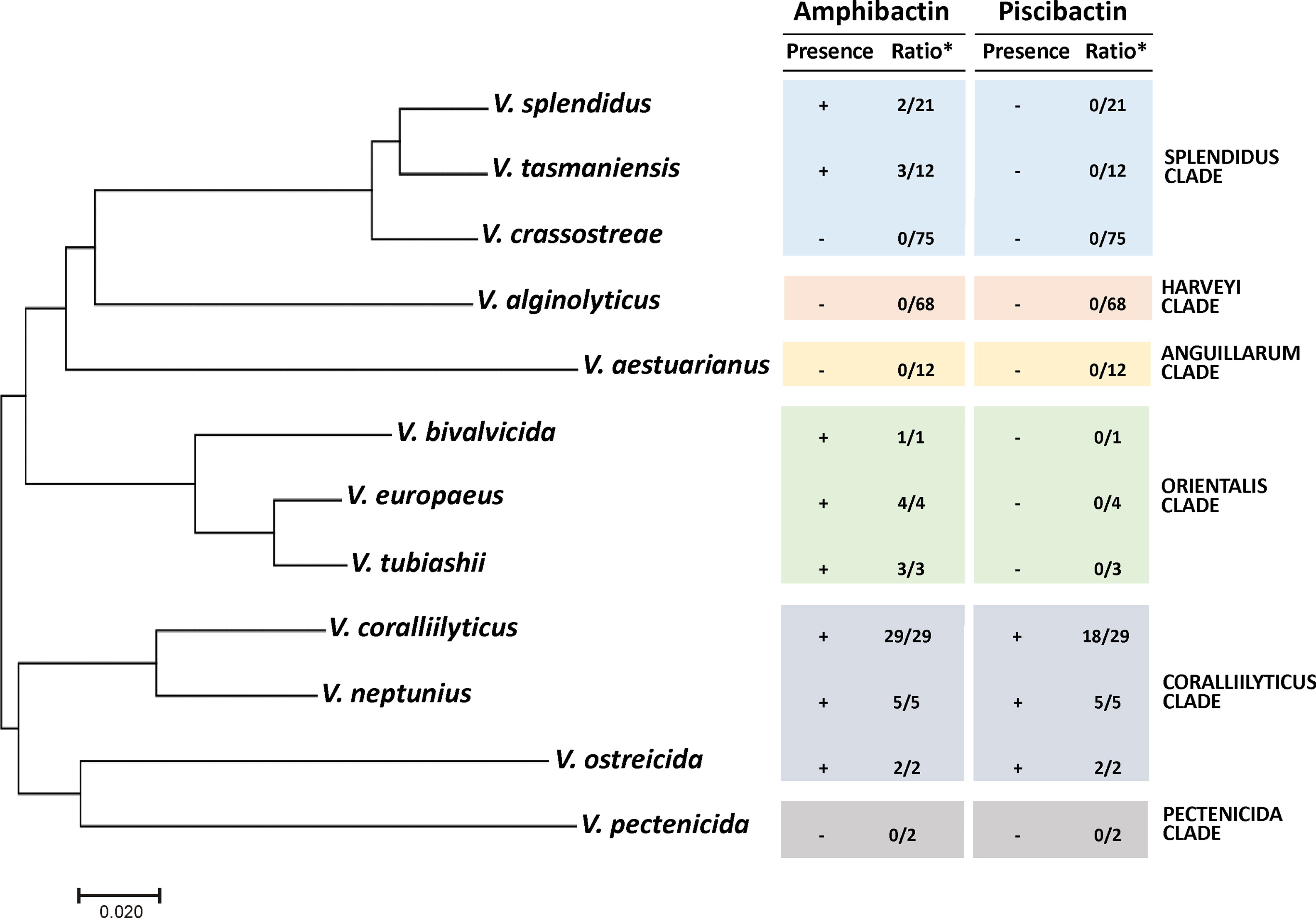
Figure 9 Distribution of amphibactin and piscibactin siderophore systems in Vibrio species responsible for outbreaks in bivalves hatcheries. Presence (+) or absence (-) of complete piscibactin/amphibactin gene cluster determined by Blast search in WGS database indexed in the NCBI database using the nucleotide sequences of V. neptunius PP-145.98 as query. The ratio (*) indicates the number of genomes of a Vibrio sp. harboring close homologues with respect to the total number of genomes analyzed of that species. Phylogenetic tree was constructed by maximum-likelihood (GTR+G model) with the concatenated sequences of the five housekeeping genes ftsZ, gyrB, mreB, pyrH and recA.
Discussion
Bacterial virulence is context-dependent and mainly depends on the interaction among diverse virulence factors functioning at the same time or sequentially when bacteria enter the host (Won and Park, 2008). The main virulence factors characterized to date in bacteria pathogenic for marine bivalve molluscs are those related to motility, chemotaxis and, in a major extent, to extracellular products (de O Santos et al., 2011; Kimes et al., 2012; Ushijima et al., 2014; Ushijima et al., 2018). In mollusc tissues, iron is mainly associated to ferritins, whose role includes transport, detoxification and iron storage (Webb et al., 1991). Hemolymph bacterial inhabitants include both mutualistic and pathogenic bacteria that must overcome hemolymph antibacterial activity and compete for the nutrients present in the host sources (Vezzulli et al., 2018).
Although the V. neptunius pathogenicity is not yet well known, some studies made with V. coralliilyticus could shed some light to the V. neptunius virulence factors, since these are two close-related bacteria (Thompson et al., 2003; Sawabe et al., 2013; Dubert et al., 2017). V. coralliilyticus pathogenicity depends on the coordinated expression of multiple virulence factors such as flagella, quorum sensing (QS), T6SS, and extracellular products including hemolysins and proteases (Jeffries, 1982; Austin et al., 2005; de O Santos et al., 2011; Kimes et al., 2012; Richards et al., 2015; Guillemette et al., 2020). It has been suggested that siderophore production should play a main role in Vibrio infection disease affecting bivalve molluscs (Soto-Rodriguez et al., 2003; Gómez-León et al., 2005; Mechri et al., 2017; Zhang et al., 2020). However, this hypothesis was based on the high frequency of siderophore producer strains found in mollusc microbiota and not in functional analysis (Desriac et al., 2014; Hernández-Robles et al., 2016; Leite et al., 2017).
The present work shows that V. neptunius PP-145.98 genome harbours a close homologue of the piscibactin system (irp genes), which is part of the highly pathogenicity island irp-HPI (Osorio et al., 2006; Balado et al., 2018). This genomic island harbours the genes that encode most functions required for piscibactin biosynthesis (irp123459) and uptake (frpABC), but lacks a gene homolog to entD, which encodes an 4’-phosphopantetheinyl transferase (PPTases) (Osorio et al., 2015; Balado et al., 2018). PPTases are required to activate the non-ribosomal peptide synthetases (NRPSs) assembly lines enabling siderophore synthesis (Orikasa et al., 2006). This function must be supplied by one of the two PPTases found in V. neptunius genome (loci WP_206370543.1 and WP_206368753.1). Genetic and chemical data proved that irp genes of V. neptunius PP-145.98 are expressed and that piscibactin is being synthesized. Since our previous works demonstrated that V. neptunius PP-145.98 also produces the siderophore amphibactin (Galvis et al., 2020), V. neptunius PP-145.98 would produce two different siderophores, amphibactin and piscibactin, simultaneously. This is not a rare characteristic, since the same feature has been reported in other bacteria such as Pseudomonas aeruginosa (Cornelis and Dingemans, 2013) or Escherichia coli (Valdebenito et al., 2006), or in the fish pathogens V. anguillarum (Balado et al., 2018) and Aeromonas salmonicida (Balado et al., 2015). The synthesis of siderophores demands a high energetic cost to the bacterium (Cornelis et al., 2011). Thus, production of redundant siderophore systems by a cell is deleterious (Cordero et al., 2012). However, in some cases, switching between siderophore systems would enhance niche flexibility (Sandy and Butler, 2009; Dumas et al., 2013; Zhang et al., 2019) and pathogenesis (Garcia et al., 2011; Balado et al., 2018).
Inactivation of both siderophore systems, piscibactin and amphibactin, greatly reduced growth capacity of V. neptunius under low-iron conditions. Interestingly, amphibactin and piscibactin are siderophores with different chemical characteristics and different affinity for Fe(III). Amphibactin is an amphiphilic siderophore produced by the addition of a variable fatty acid moiety to a head group that coordinates a strong affinity for iron. Thus, amphibactin is mainly associated to the cell membrane, which would minimize the diffusive loss of siderophore in the marine environment (Martinez et al., 2003; Boiteau et al., 2016). On the other hand, piscibactin is a labile yersiniabactin-like siderophore with low affinity for iron (Souto et al., 2012). Congruently, the V. neptunius Δirp2 single mutant, that only produces amphibactin, showed higher growth under strong iron restriction than the piscibactin-only producer V. neptunius ΔabtF mutant. The affinity of a siderophore for iron does not predict their impact in virulence, e.g. highly virulent V. anguillarum strains produce piscibactin and also vanchrobactin, a siderophore with higher affinity for iron, but piscibactin production is the most relevant for virulence (Balado et al., 2018).
Infection challenges showed that inactivation of siderophore synthesis strongly reduced the mortality caused by V. neptunius in clams. Thus, our results proved that siderophore production constitutes a key virulence factor of pathogenic Vibrios in molluscs. Interestingly, inactivation of one of the two siderophore systems (either amphibactin or piscibactin) significantly reduced virulence compared to the wild type strain. This finding showed that the ability to produce both siderophores maximises virulence of V. neptunius. In this concern, piscibactin a yersiniabactin-like siderophore, belongs to a type of siderophores that could have other roles during infection besides iron uptake, since they could promote bacterial colonization, dissemination and resistance against phagocytosis (Chaturvedi et al., 2012; Bobrov et al., 2014; Holden and Bachman, 2015; Koh and Henderson, 2015). Thus, piscibactin and amphibactin could have specialized role(s) in the survival of V. neptunius within the host and the environment. However, the fact that V. neptunius single mutants deficient in each one of these siderophores have showed indistinguishable virulence properties, suggests that both siderophore systems contribute equally to V. neptunius virulence.
Although amphibactin is one of the most abundant siderophores in seawater (Boiteau et al., 2016; Gauglitz et al., 2021), transcriptional organization of amphibactin genes was not studied to date. The results demonstrated that amphibactin and piscibactin siderophore systems are significantly expressed when V. neptunius is cultivated under low-iron availability in vitro. More notably, both siderophore systems are also simultaneously expressed at similar levels when V. neptunius is cultivated in bivalve hemolymph (ex vivo). The co-expression of siderophores with different chemical properties could contribute to enhance bacterial fitness during host-pathogen interactions (Dumas et al., 2013; Lages et al., 2019). Thus, these results confirm that the high virulence for clams showed by V. neptunius wild-type strain must depend on the simultaneous production of piscibactin and amphibactin.
Austin and col. (2005) showed that the V. neptunius LMG 20536 and LMG 20610 strains are pathogenic to rainbow trout causing up to 100% mortality at a dose of 106 cells per fish inoculated intraperitoneally. The present work showed that, although PP-145.98 strain harbors the Highly Pathogenicity Island irp-HPI and produces the siderophore piscibactin, wild type strain does not cause mortality in turbot (Scophthalmus maximus). Interestingly, Austin and col. (2005) also showed that while extracellular products (ECPs) of V. neptunius LMG 20610 were highly toxic for fish, ECPs of V. neptunius LMG 20536 were almost non toxic (Austin et al., 2005). These findings greatly suggest the existence of a high heterogeneity among V. neptunius strains and are in agreement with previous works showing that the pathogenicity mechanisms necessary for infecting fish or shellfish are different (Won and Park, 2008; Zhang et al., 2020).
In a previous work it was found that a high proportion of the Vibrio-like isolates from mussel hemolymph were PCR positive for a target in amphibactin outer membrane transporter abtA (Galvis et al., 2020). Those abtA-positive isolates were mainly non-pathogenic vibrios belonging to the Splendidus clade (Galvis et al., 2020). The in silico analysis of 234 genome sequences of well characterized mollusc pathogens belonging to the clades Coralliilyticus, Anguillarum, Harveyi, Orientalis, Pectenecida and Splendidus showed that amphibactin is widespread not only in the Splendidus clade, but also in Orientalis and Coralliilyticus clades. However, irp-HPI element encoding piscibactin seems to be limited to the Coralliilyticus clade, since it is present in all V. neptunius and V. ostreicida genomes and in most V. coralliilyticus genomes available. Thus, simultaneous production of both siderophores would be a widespread character in the well-recognized mollusc pathogens V. coralliilyticus, V. neptunius and V. ostreicida. According to the available data, siderophores could have a dual function since they can be key drivers of the microbial community structure and would be key virulence factors that enable infection in an animal host (Vezzulli et al., 2008; Cordero et al., 2012; Kramer et al., 2020; Gauglitz et al., 2021). Rubio-Portillo and col. (2020) showed that siderophore production has a central role in competition among bacteria of mollusc microbiota, enhancing the ability of Vibrio coral pathogens such as V. coralliilyticus and V. mediterranei to invade the host and cause tissue necrosis.
It is known that some bacterial diseases emerged after rapid spread of selectively favoured virulence factors by horizontal gene transfer (HGT) (Bruto et al., 2017; Le Roux and Blokesch, 2018). Molluscs are a major group of marine animals that play key roles in marine ecosystems including water clarification and providing an habitat for other organisms (Schatte Olivier et al., 2020). In addition, molluscs serve as alternative/reservoir hosts for pathogenic bacteria, including human pathogens (Destoumieux-Garzón et al., 2020). Although mollusc microbiota itself would not be a primary source of pathogens for higher animals, the high prevalence of siderophore systems in this niche would imply that the microbiota could serve as test beds (reservoir) of virulence factors like the High-Pathogenicity Island irp-HPI encoding the piscibactin system, which is a proved virulence factor in some fish pathogenic bacteria (Osorio et al., 2015; Balado et al., 2018).
In conclusion, synthesis of the siderophores amphibactin and piscibactin, which are widespread in several different Vibrio species pathogenic for marine bivalve molluscs, would constitute key virulence factors for disease outbreaks in molluscs aquaculture systems.
Data Availability Statement
Publicly available datasets were analyzed in this study. This data can be found here: NCBI, accession number: JAFHLB000000000.
Ethics Statement
The animal study was reviewed and approved by Bioethics Committee of the University of Santiago de Compostela.
Author Contributions
FG and LA performed the lab experiments. MB, JR, and CJ analyzed the data. FG, LA, CJ, and MB wrote the first draft of the manuscript. MB and ML corrected the draft and built the final version of the manuscript. All authors conceived and designed the study, contributed to manuscript revision, and read and approved the submitted version.
Funding
This work was supported by grants AGL2017-86183-R (AEI/FEDER, EU), RTI2018-093634-B-C21/C22 (AEI/FEDER, EU) and PID2019-103891RJ-100 (AEI) from the State Agency for Research (AEI) of Spain. AGL2017-86183-R and RTI2018-093634-B-C21/C22 were co-funded by the FEDER Programme from the European Union. Work in University of Santiago de Compostela was also supported by grants GRC2018/018 and 2021-CP112, and in University of A Coruña by grant GRC2018/039 from Xunta de Galicia (Spain). FG was financed with a fellowship ‘Programa de formación de recurso humano de alto nivel doctorado en el exterior’ granted by Colciencias and the government of Norte de Santander, Colombia. LA was financed with a fellowship (ED481A-2019/081) from Xunta de Galicia (Spain), co-financed by ESF (European Social Fund).
Conflict of Interest
The authors declare that the research was conducted in the absence of any commercial or financial relationships that could be construed as a potential conflict of interest.
Publisher’s Note
All claims expressed in this article are solely those of the authors and do not necessarily represent those of their affiliated organizations, or those of the publisher, the editors and the reviewers. Any product that may be evaluated in this article, or claim that may be made by its manufacturer, is not guaranteed or endorsed by the publisher.
Acknowledgments
The authors acknowledge Proameixa Fernández S.L. and IGAFA (Instituto Galego de Formación en Acuicultura) from Galicia, Spain, for providing the seed and larvae of molluscs for the pathogenesis assays. FG also acknowledges a fellowship from ‘Programa de formación de recurso humano de alto nivel doctorado en el exterior’ granted by Colciencias and the government of Norte de Santander, Colombia; and ‘Pasaporte a la Ciencia’ granted by Icetex, Colombia.
Supplementary Material
The Supplementary Material for this article can be found online at: https://www.frontiersin.org/articles/10.3389/fcimb.2021.750567/full#supplementary-material
References
Austin, B., Austin, D., Sutherland, R., Thompson, F., Swings, J. (2005). Pathogenicity of Vibrios to Rainbow Trout (Oncorhynchus mykiss, Walbaum) and Artemia nauplii. Environ. Microbiol. 7, 1488–1495. doi: 10.1111/j.1462-2920.2005.00847.x
Balado, M., Lages, M. A., Fuentes-Monteverde, J. C., Martínez-Matamoros, D., Rodríguez, J., Jiménez, C., et al (2018). The Siderophore Piscibactin is a Relevant Virulence Factor for Vibrio Anguillarum Favored at Low Temperatures. Front. Microbiol. 9, 1766. doi: 10.3389/fmicb.2018.01766
Balado, M., Osorio, C. R., Lemos, M. L. (2006). A Gene Cluster Involved in the Biosynthesis of Vanchrobactin, a Chromosome-Encoded Siderophore Produced by Vibrio anguillarum. Microbiology 152, 3517–3528. doi: 10.1099/mic.0.29298-0
Balado, M., Souto, A., Vences, A., Careaga, V. P., Valderrama, K., Segade, Y., et al (2015). Two Catechol Siderophores, Acinetobactin and Amonabactin, Are Simultaneously Produced by Aeromonas salmonicida subsp. salmonicida Sharing Part of the Biosynthetic Pathway. ACS Chem. Biol. 10, 2850–2860. doi: 10.1021/acschembio.5b00624
Beaz-Hidalgo, R., Balboa, S., Romalde, J. L., Figueras, M. J. (2010). Diversity and Pathogenecity of Vibrio Species in Cultured Bivalve Molluscs. Environ. Microbiol. Rep. 2, 34–43. doi: 10.1111/j.1758-2229.2010.00135.x
Blin, K., Shaw, S., Steinke, K., Villebro, R., Ziemert, N., Lee, S. Y., et al (2019). antiSMASH 5.0: Updates to the Secondary Metabolite Genome Mining Pipeline. Nucleic Acids Res. 47, W81–W87. doi: 10.1093/nar/gkz310
Bobrov, A. G., Kirillina, O., Fetherston, J. D., Miller, M. C., Burlison, J. A., Perry, R. D. (2014). The Yersinia Pestis Siderophore, Yersiniabactin, and the ZnuABC System Both Contribute to Zinc Acquisition and the Development of Lethal Septicaemic Plague in Mice. Mol. Microbiol. 93, 759–775. doi: 10.1111/mmi.12693
Boiteau, R. M., Mende, D. R., Hawco, N. J., McIlvin, M. R., Fitzsimmons, J. N., Saito, M. A., et al (2016). Siderophore-Based Microbial Adaptations to Iron Scarcity Across the Eastern Pacific Ocean. Proc. Natl. Acad. Sci. U. S. A. 113, 14237–14242. doi: 10.1073/pnas.1608594113
Bruto, M., James, A., Petton, B., Labreuche, Y., Chenivesse, S., Alunno-Bruscia, M., et al (2017). Vibrio Crassostreae, a Benign Oyster Colonizer Turned Into a Pathogen After Plasmid Acquisition. ISME J. 11, 1043–1052. doi: 10.1038/ismej.2016.162
Cahill, P. L., Fidler, A. E., Hopkins, G. A., Wood, S. A. (2016). Geographically Conserved Microbiomes of Four Temperate Water Tunicates. Environ. Microbiol. Rep. 8, 470–478. doi: 10.1111/1758-2229.12391
Chaturvedi, K. S., Hung, C. S., Crowley, J. R., Stapleton, A. E., Henderson, J. P. (2012). The Siderophore Yersiniabactin Binds Copper to Protect Pathogens During Infection. Nat. Chem. Biol. 8, 731–736. doi: 10.1038/nchembio.1020
Cordero, O. X., Ventouras, L.-A., DeLong, E. F., Polz, M. F. (2012). Public Good Dynamics Drive Evolution of Iron Acquisition Strategies in Natural Bacterioplankton Populations. Proc. Natl. Acad. Sci. U. S. A. 109, 20059–20064. doi: 10.1073/pnas.1213344109
Cornelis, P., Dingemans, J. (2013). Pseudomonas Aeruginosa Adapts its Iron Uptake Strategies in Function of the Type of Infections. Front. Cell. Infect. Microbiol. 3, 75. doi: 10.3389/fcimb.2013.00075
Cornelis, P., Wei, Q., Andrews, S. C., Vinckx, T. (2011). Iron Homeostasis and Management of Oxidative Stress Response in Bacteria. Metallomics 3, 540. doi: 10.1039/c1mt00022e
de O Santos, E., Alves, N., Dias, G. M., Mazotto, A. M., Vermelho, A., Vora, G. J., et al (2011). Genomic and Proteomic Analyses of the Coral Pathogen Vibrio coralliilyticus Reveal a Diverse Virulence Repertoire. ISME J. 5, 1471–1483. doi: 10.1038/ismej.2011.19
Desriac, F., Le Chevalier, P., Brillet, B., Leguerinel, I., Thuillier, B., Paillard, C., et al (2014). Exploring the Hologenome Concept in Marine Bivalvia: Haemolymph Microbiota as a Pertinent Source of Probiotics for Aquaculture. FEMS Microbiol. Lett. 350, 107–116. doi: 10.1111/1574-6968.12308
Destoumieux-Garzón, D., Canesi, L., Oyanedel, D., Travers, M. A., Charrière, G. M., Pruzzo, C., et al (2020). Vibrio–bivalve Interactions in Health and Disease. Environ. Microbiol. 22, 4323–4341. doi: 10.1111/1462-2920.15055
Dubert, J., Barja, J. L., Romalde, J. L. (2017). New Insights Into Pathogenic Vibrios Affecting Bivalves in Hatcheries: Present and Future Prospects. Front. Microbiol. 8, 762. doi: 10.3389/fmicb.2017.00762
Dubert, J., Nelson, D. R., Spinard, E. J., Kessner, L., Gomez-Chiarri, M., Costa, F., et al (2016). Following the Infection Process of Vibriosis in Manila Clam (Ruditapes philippinarum) Larvae Through GFP-Tagged Pathogenic Vibrio Species. J. Invertebr. Pathol. 133, 27–33. doi: 10.1016/j.jip.2015.11.008
Dumas, Z., Ross-Gillespie, A., Kümmerli, R. (2013). Switching Between Apparently Redundant Iron-Uptake Mechanisms Benefits Bacteria in Changeable Environments. Proc. Biol. Sci. 280, 20131055. doi: 10.1098/rspb.2013.1055
Engel, S., Jensen, P. R., Fenical, W. (2002). Chemical Ecology of Marine Microbial Defense. J. Chem. Ecol. 28, 1971–1985. doi: 10.1023/A:1020793726898
Farto, R., Fichi, G., Gestal, C., Pascual, S., Nieto, T. P. (2019). “Bacteria-Affecting Cephalopods,” in Handbook of Pathogens and Diseases in Cephalopods (Cham: Springer International Publishing), 127–142. doi: 10.1007/978-3-030-11330-8_8
Finn, R. D., Bateman, A., Clements, J., Coggill, P., Eberhardt, R. Y., Eddy, S. R., et al (2014). Pfam: The Protein Families Database. Nucleic Acids Res. 42, D222–D230. doi: 10.1093/nar/gkt1223
Galvis, F., Ageitos, L., Martínez-Matamoros, D., Barja, J. L., Rodríguez, J., Lemos, M. L., et al (2020). The Marine Bivalve Molluscs Pathogen Vibrio neptunius Produces the Siderophore Amphibactin, Which Is Widespread in Molluscs Microbiota. Environ. Microbiol. 22, 5467–5482. doi: 10.1111/1462-2920.15312
Galvis, F., Barja, J. L., Lemos, M. L., Balado, M. (2021). The Vibriolysin-Like Protease VnpA and the Collagenase ColA Are Required for Full Virulence of the Bivalve Mollusks Pathogen Vibrio neptunius. Antibiotics 10, 391. doi: 10.3390/antibiotics10040391
García-Amado, M. A., Bozo-Hurtado, L., Astor, Y., Suárez, P., Chistoserdov, A. (2011). Denaturing Gradient Gel Electrophoresis Analyses of the Vertical Distribution and Diversity of Vibrio Spp. Populations in the Cariaco Basin. FEMS Microbiol. Ecol. 77, 347–356. doi: 10.1111/j.1574-6941.2011.01116.x
Garcia, E. C., Brumbaugh, A. R., Mobley, H. L. T. (2011). Redundancy and Specificity of Escherichia Coli Iron Acquisition Systems During Urinary Tract Infection. Infect. Immun. 79, 1225–1235. doi: 10.1128/IAI.01222-10
Gauglitz, J. M., Boiteau, R. M., McLean, C., Babcock-Adams, L., McIlvin, M. R., Moran, D. M., et al (2021). Dynamic Proteome Response of a Marine Vibrio to a Gradient of Iron and Ferrioxamine Bioavailability. Mar. Chem. 229, 103913. doi: 10.1016/j.marchem.2020.103913
Gómez-León, J., Villamil, L., Lemos, M. L., Novoa, B., Figueras, A. (2005). Isolation of Vibrio alginolyticus and Vibrio splendidus From Aquacultured Carpet Shell Clam (Ruditapes decussatus) Larvae Associated With Mass Mortalities. Appl. Environ. Microbiol. 71, 98–104. doi: 10.1128/AEM.71.1.98-104.2005
Guillemette, R., Ushijima, B., Jalan, M., Häse, C. C., Azam, F. (2020). Insight Into the Resilience and Susceptibility of Marine Bacteria to T6SS Attack by Vibrio cholerae and Vibrio coralliilyticus. PLoS One 15 (1), e0227864. doi: 10.1371/journal.pone.0227864
Hernández-Robles, M. F., Álvarez-Contreras, A. K., Juárez-García, P., Natividad-Bonifacio, I., Curiel-Quesada, E., Vázquez-Salinas, C., et al (2016). Virulence Factors and Antimicrobial Resistance in Environmental Strains of Vibrio alginolyticus. Int. Microbiol. 19, 191–198. doi: 10.2436/20.1501.01.277
Herrero, M., de Lorenzo, V., Timmis, K. N. (1990). Transposon Vectors Containing Non-Antibiotic Resistance Selection Markers for Cloning and Stable Chromosomal Insertion of Foreign Genes in Gram-Negative Bacteria. J. Bacteriol. 172, 6557–6567. doi: 10.1128/jb.172.11.6557-6567.1990
Holden, V. I., Bachman, M. A. (2015). Diverging Roles of Bacterial Siderophores During Infection. Metallomics 7, 986–995. doi: 10.1039/c4mt00333k
Jeffries, V. E. (1982). Three Vibrio Strains Pathogenic to Larvae of Crassostrea Gigas and Ostrea Edulis. Aquaculture 29, 201–226. doi: 10.1016/0044-8486(82)90136-3
Kesarcodi-Watson, A., Kaspar, H., Lategan, M., Gibson, L. (2009a). Challenge of New Zealand GreenshellTM Mussel Perna canaliculus Larvae Using Two Vibrio Pathogens: A Hatchery Study. Dis. Aquat. Organ. 86, 15–20. doi: 10.3354/dao02100
Kesarcodi-Watson, A., Kaspar, H., Lategan, M. J., Gibson, L. (2009b). Two Pathogens of GreenshellTM Mussel Larvae, Perna canaliculus: Vibrio splendidus and a V. coralliilyticus/neptunius -Like Isolate. J. Fish Dis. 32, 499–507. doi: 10.1111/j.1365-2761.2009.01006.x
Kimes, N. E., Grim, C. J., Johnson, W. R., Hasan, N. A., Tall, B. D., Kothary, M. H., et al (2012). Temperature Regulation of Virulence Factors in the Pathogen Vibrio coralliilyticus. ISME J. 6, 835–846. doi: 10.1038/ismej.2011.154
Koh, E.-I., Henderson, J. P. (2015). Microbial Copper-Binding Siderophores at the Host-Pathogen Interface. J. Biol. Chem. 290, 18967–18974. doi: 10.1074/jbc.R115.644328
Kramer, J., Özkaya, Ö., Kümmerli, R. (2020). Bacterial Siderophores in Community and Host Interactions. Nat. Rev. Microbiol. 18, 152–163. doi: 10.1038/s41579-019-0284-4
Kumar, S., Stecher, G., Li, M., Knyaz, C., Tamura, K. (2018). MEGA X: Molecular Evolutionary Genetics Analysis Across Computing Platforms. Mol. Biol. Evol. 35, 1547–1549. doi: 10.1093/molbev/msy096
Lages, M. A., Balado, M., Lemos, M. L. (2019). The Expression of Virulence Factors in Vibrio Anguillarum Is Dually Regulated by Iron Levels and Temperature. Front. Microbiol. 10, 2335. doi: 10.3389/FMICB.2019.02335
Lambalot, R. H., Gehring, A. M., Flugel, R. S., Zuber, P., LaCelle, M., Marahiel, M. A., et al (1996). A New Enzyme Superfamily - the Phosphopantetheinyl Transferases. Chem. Biol. 3, 923–936. doi: 10.1016/s1074-5521(96)90181-7
Leite, L., Jude-Lemeilleur, F., Raymond, N., Henriques, I., Garabetian, F., Alves, A. (2017). Phylogenetic Diversity and Functional Characterization of the Manila Clam Microbiota: A Culture-Based Approach. Environ. Sci. Pollut. Res. 24, 21721–21732. doi: 10.1007/s11356-017-9838-z
Lemos, M. L., Salinas, P., Toranzo, A. E., Barja, J. L., Crosa, J. H. (1988). Chromosome-Mediated Iron Uptake System in Pathogenic Strains of Vibrio anguillarum. J. Bacteriol. 170, 1920–1925. doi: 10.1128/jb.170.4.1920-1925.1988
Le Roux, F., Blokesch, M. (2018). Eco-Evolutionary Dynamics Linked to Horizontal Gene Transfer in Vibrios. Annu. Rev. Microbiol. 72, 89–110. doi: 10.1146/annurev-micro-090817-062148
Liu, Q., Ma, Y., Zhou, L., Zhang, Y. (2005). Gene Cloning, Expression and Functional Characterization of a Phosphopantetheinyl Transferase From Vibrio anguillarum Serotype O1. Arch. Microbiol. 183, 37–44. doi: 10.1007/s00203-004-0745-6
Lokmer, A., Goedknegt, M. A., Thieltges, D. W., Fiorentino, D., Kuenzel, S., Baines, J. F., et al (2016). Spatial and Temporal Dynamics of Pacific Oyster Hemolymph Microbiota Across Multiple Scales. Front. Microbiol. 7, 1367. doi: 10.3389/fmicb.2016.01367
Martinez, J. S., Carter-franklin, J. N., Mann, E. L., Martin, J. D., Haygood, M. G., Butler, A. (2003). Structure and Membrane Affinity of a Suite of Amphiphilic Siderophores Produced by a Marine Bacterium. Proc. Natl. Acad. Sci. 100, 3754–3759. doi: 10.1073/pnas.0637444100
McFall-Ngai, M., Hadfield, M. G., Bosch, T. C. G., Carey, H. V., Domazet-Lošo, T., Douglas, A. E., et al (2013). Animals in a Bacterial World, a New Imperative for the Life Sciences. Proc. Natl. Acad. Sci. 110, 3229–3236. doi: 10.1073/pnas.1218525110
McMillan, D. G. G., Velasquez, I., Nunn, B. L., Goodlett, D. R., Hunter, K. A., Lamont, I., et al (2010). Acquisition of Iron by Alkaliphilic Bacillus species. Appl. Environ. Microbiol. 76, 6955–6961. doi: 10.1128/AEM.01393-10
Mechri, B., Monastiri, A., Medhioub, A., Medhioub, M. N., Aouni, M. (2017). Molecular Characterization and Phylogenetic Analysis of Highly Pathogenic Vibrio alginolyticus Strains Isolated During Mortality Outbreaks in Cultured Ruditapes decussatus Juvenile. Microb. Pathog. 111, 487–496. doi: 10.1016/J.MICPATH.2017.09.020
Miller, J. H. (1992). A Short Course in Bacterial Genetics (Plainview, N.Y: Cold Spring Harbor Laboratory Press).
Mouriño, S., Osorio, C. R., Lemos, M. L. (2004). Characterization of Heme Uptake Cluster Genes in the Fish Pathogen Vibrio Anguillarum. J. Bacteriol. 186, 6159–6167. doi: 10.1128/JB.186.18.6159-6167.2004
Ochsner, U. A., Vasil, M. L. (1996). Gene Repression by the Ferric Uptake Regulator in Pseudomonas Aeruginosa: Cycle Selection of Iron-Regulated Genes. Proc. Natl. Acad. Sci. U. S. A. 93, 4409–4414. doi: 10.1073/pnas.93.9.4409
Orikasa, Y., Nishida, T., Hase, A., Watanabe, K., Morita, N., Okuyama, H. (2006). A Phosphopantetheinyl Transferase Gene Essential for Biosynthesis of N - 3 Polyunsaturated Fatty Acids From Moritella marina Strain MP-1. FEBS Lett. 580, 4423–4429. doi: 10.1016/j.febslet.2006.07.008
Osorio, C. R., Juiz-Rio, S., Lemos, M. L. (2006). A Siderophore Biosynthesis Gene Cluster From the Fish Pathogen Photobacterium Damselae Subsp. Piscicida Is Structurally and Functionally Related to the Yersinia High-Pathogenicity Island. Microbiology 152, 3327–3341. doi: 10.1099/mic.0.29190-0
Osorio, C. R., Rivas, A. J., Balado, M., Fuentes-Monteverde, J. C., Rodríguez, J., Jiménez, C., et al (2015). A Transmissible Plasmid-Borne Pathogenicity Island Confers Piscibactin Biosynthesis in the Fish Pathogen Photobacterium damselae Subsp. Piscicida. Appl. Environ. Microbiol. 81, 5867–5879. doi: 10.1128/AEM.01580-15
Paillard, C., Le Roux, F., Borrego, J. J. (2004). Bacterial Disease in Marine Bivalves, a Review of Recent Studies: Trends and Evolution. Aquat. Living Resour. 17, 477–498. doi: 10.1051/alr:2004054
Parales, R. E., Harwood, C. S. (1993). Construction and Use of a New Broad-Host-Range lacZ Transcriptional Fusion Vector, pHRP309, for Gram- Bacteria. Gene 133, 23–30. doi: 10.1016/0378-1119(93)90220-W
Payne, S. M., Mey, A. R., Wyckoff, E. E. (2016). Vibrio Iron Transport: Evolutionary Adaptation to Life in Multiple Environments. Microbiol. Mol. Biol. Rev. 80, 69–90. doi: 10.1128/MMBR.00046-15
Potgieter, M., Bester, J., Kell, D. B., Pretorius, E. (2015). The Dormant Blood Microbiome in Chronic, Inflammatory Diseases. FEMS Microbiol. Rev. 39, 567–591. doi: 10.1093/femsre/fuv013
Prado, S., Romalde, J., Montes, J., Barja, J. (2005). Pathogenic Bacteria Isolated From Disease Outbreaks in Shellfish Hatcheries. First Description of Vibrio neptunius as an Oyster Pathogen. Dis. Aquat. Organ. 67, 209–215. doi: 10.3354/dao067209
Richards, G. P., Watson, M. A., Needleman, D. S., Church, K. M., Häse, C. C. (2015). Mortalities of Eastern and Pacific Oyster Larvae Caused by the Pathogens Vibrio Coralliilyticus and Vibrio Tubiashii. Appl. Environ. Microbiol. 81, 292–297. doi: 10.1128/AEM.02930-14
Romalde, J. L., Dieguez, A. L., Lasa, A., Balboa, S. (2014). New Vibrio Species Associated to Molluscan Microbiota: A Review. Front. Microbiol. 4, 413. doi: 10.3389/fmicb.2013.00413
Rubio-Portillo, E., Martin-Cuadrado, A. B., Caraballo-Rodríguez, A. M., Rohwer, F., Dorrestein, P. C., Antón, J. (2020). Virulence as a Side Effect of Interspecies Interaction in Vibrio Coral Pathogens. mbio 11, 201–221. doi: 10.1128/mBio.00201-20
Sandy, M., Butler, A. (2009). Microbial Iron Acquisition: Marine and Terrestrial Siderophores. Chem. Rev. 109, 4580–4595. doi: 10.1021/cr9002787
Sawabe, T., Ogura, Y., Matsumura, Y., Feng, G., Amin, A. R., Mino, S., et al (2013). Updating the Vibrio Clades Defined by Multilocus Sequence Phylogeny: Proposal of Eight New Clades, and the Description of Vibrio tritonius Sp. Nov. Front. Microbiol. 4, 414. doi: 10.3389/fmicb.2013.00414
Schatte Olivier, A., Jones, L., Vay, L., Christie, M., Wilson, J., Malham, S. K. (2020). A Global Review of the Ecosystem Services Provided by Bivalve Aquaculture. Rev. Aquacult. 12, 3–25. doi: 10.1111/raq.12301
Schwyn, B., Neilands, J. B. (1987). Universal Chemical Assay for the Detection and Determination of Siderophores. Anal. Biochem. 160, 47–56. doi: 10.1016/0003-2697(87)90612-9
Soto-Rodriguez, S., Roque, A., Lizarraga-Partida, M., Guerra-Flores, A., Gomez-Gil, B. (2003). Virulence of Luminous Vibrios to Artemia franciscana Nauplii. Dis. Aquat. Organ. 53, 231–240. doi: 10.3354/dao053231
Souto, A., Montaos, M. A. M. A., Rivas, A. J. A. J., Balado, M., Osorio, C. R., Rodríguez, J., et al (2012). Structure and Biosynthetic Assembly of Piscibactin, a Siderophore From Photobacterium damselae Subsp. Piscicida, Predicted From Genome Analysis. Eur. J. Org. Chem. 2012, 5693–5700. doi: 10.1002/ejoc.201200818
Thompson, F. L., Li, Y., Gomez-Gil, B., Thompson, C. C., Hoste, B., Vandemeulebroecke, K., et al (2003). Vibrio Neptunius Sp. Nov., Vibrio brasiliensis Sp. Nov. And Vibrio xuii Sp. Nov., Isolated From the Marine Aquaculture Environment (Bivalves, Fish, Rotifers and Shrimps). Int. J. Syst. Evol. Microbiol. 53, 245–252. doi: 10.1099/ijs.0.02447-0
Toranzo, A. E., Magariños, B., Romalde, J. L. (2005). A Review of the Main Bacterial Fish Diseases in Mariculture Systems. Aquaculture 246, 37–61. doi: 10.1016/j.aquaculture.2005.01.002
Travers, M.-A., Boettcher Miller, K., Roque, A., Friedman, C. S. (2015). Bacterial Diseases in Marine Bivalves. J. Invertebr. Pathol. 131, 11–31. doi: 10.1016/j.jip.2015.07.010
Ushijima, B., Richards, G. P., Watson, M. A., Schubiger, C. B., Häse, C. C. (2018). Factors Affecting Infection of Corals and Larval Oysters by Vibrio Coralliilyticus. PLoS One 13, e0199475. doi: 10.1371/journal.pone.0199475
Ushijima, B., Videau, P., Burger, A. H., Shore-Maggio, A., Runyon, C. M., Sudek, M., et al (2014). Vibrio Coralliilyticus Strain OCN008 Is an Etiological Agent of Acute Montipora White Syndrome. Appl. Environ. Microbiol. 80, 2102–2109. doi: 10.1128/AEM.03463-13
Utermann, C., Parrot, D., Breusing, C., Stuckas, H., Staufenberger, T., Blümel, M., et al (2018). Combined Genotyping, Microbial Diversity and Metabolite Profiling Studies on Farmed Mytilus Spp. From Kiel Fjord. Sci. Rep. 8, 7983. doi: 10.1038/s41598-018-26177-y
Valdebenito, M., Crumbliss, A. L., Winkelmann, G., Hantke, K. (2006). Environmental Factors Influence the Production of Enterobactin, Salmochelin, Aerobactin, and Yersiniabactin in Escherichia coli Strain Nissle 1917. Int. J. Med. Microbiol. 296, 513–520. doi: 10.1016/j.ijmm.2006.06.003
Vezzulli, L., Guzmán, C. A., Colwell, R. R., Pruzzo, C. (2008). Dual Role Colonization Factors Connecting Vibrio cholerae’s Lifestyles in Human and Aquatic Environments Open New Perspectives for Combating Infectious Diseases. Curr. Opin. Biotechnol. 19, 254–259. doi: 10.1016/j.copbio.2008.04.002
Vezzulli, L., Stagnaro, L., Grande, C., Tassistro, G., Canesi, L., Pruzzo, C. (2018). Comparative 16S rDNA Gene-Based Microbiota Profiles of the Pacific Oister (Crassostrea Gigas) and the Mediterranean Mussel (Mytilus Galloprovincialis) From a Shellfish Farm (Ligurian Sea, Italy). Microb. Ecol. 75, 495–504. doi: 10.1007/s00248-017-1051-6
Wang, R. F., Kushner, S. R. (1991). Construction of Versatile Low-Copy-Number Vectors for Cloning, Sequencing and Gene Expression in Escherichia Coli. Gene 100, 195–199. doi: 10.1016/0378-1119(91)90366-J
Webb, J., St.Pierre, T. G., Macey, D. J. (1991). “Iron Biomineralization in Invertebrates,” in Iron Biominerals (Boston, MA: Springer US), 193–220. doi: 10.1007/978-1-4615-3810-3_14
Won, K. M., Park, S. (2008). Pathogenicity of Vibrio Harveyi to Cultured Marine Fishes in Korea. Aquaculture 285, 8–13. doi: 10.1016/j.aquaculture.2008.08.013
Zhang, X., Baars, O., Morel, F. M. M. (2019). Genetic, Structural, and Functional Diversity of Low and High-Affinity Siderophores in Strains of Nitrogen Fixing Azotobacter Chroococcum. Metallomics 11, 201–212. doi: 10.1039/C8MT00236C
Zhang, X.-H., He, X., Austin, B. (2020). Vibrio Harveyi: A Serious Pathogen of Fish and Invertebrates in Mariculture. Mar. Life Sci. Technol. 2, 231–245. doi: 10.1007/s42995-020-00037-z
Keywords: Coralliilyticus, Vibrio neptunius, bivalve molluscs pathogens, virulence factors, siderophores, piscibactin, amphibactin, aquaculture
Citation: Galvis F, Ageitos L, Rodríguez J, Jiménez C, Barja JL, Lemos ML and Balado M (2021) Vibrio neptunius Produces Piscibactin and Amphibactin and Both Siderophores Contribute Significantly to Virulence for Clams. Front. Cell. Infect. Microbiol. 11:750567. doi: 10.3389/fcimb.2021.750567
Received: 30 July 2021; Accepted: 07 October 2021;
Published: 25 October 2021.
Edited by:
Cristian Oliver, Austral University of Chile, ChileReviewed by:
Javier Santander, Memorial University of Newfoundland, CanadaJorge Alberto Giron, University of Puebla, Mexico
Copyright © 2021 Galvis, Ageitos, Rodríguez, Jiménez, Barja, Lemos and Balado. This is an open-access article distributed under the terms of the Creative Commons Attribution License (CC BY). The use, distribution or reproduction in other forums is permitted, provided the original author(s) and the copyright owner(s) are credited and that the original publication in this journal is cited, in accordance with accepted academic practice. No use, distribution or reproduction is permitted which does not comply with these terms.
*Correspondence: Manuel L. Lemos, manuel.lemos@usc.es; Miguel Balado, miguel.balado@usc.es