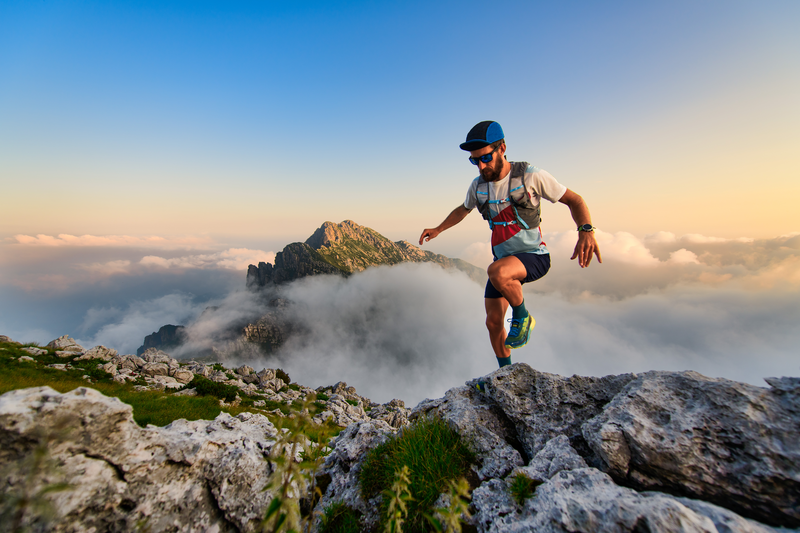
94% of researchers rate our articles as excellent or good
Learn more about the work of our research integrity team to safeguard the quality of each article we publish.
Find out more
ORIGINAL RESEARCH article
Front. Cell. Infect. Microbiol. , 21 September 2021
Sec. Parasite and Host
Volume 11 - 2021 | https://doi.org/10.3389/fcimb.2021.744352
This article is part of the Research Topic Cellular and Molecular Basis in Parasitic Diseases Control: Research Trends View all 42 articles
Background: Schistosomiasis is one of the most important tropical parasitic diseases worldwide. Biomphalaria straminea, the intermediate host of Schistosoma mansoni, has invaded and spread to Southern China since 1974 and may pose enormous threats to public health. Controlling intermediate host snails is an effective strategy in schistosomiasis intervention. However, the only effective chemical molluscicide, niclosamide, currently recommended by WHO may cause environmental pollution, loss of biodiversity, and high costs. Thus, to counter intermediate hosts, a sustainable and environmentally friendly tool is urgently needed. Here, we conducted field investigations to collect and identify a potential snail competitor rotifer and evaluated its molluscicide effect.
Results: In this study, we collected two samples of rotifers from Shenzhen. We found both red and black phenotypic B. straminea snails at the sampling sites. We identified the rotifer population as a species of the genus Philodina according to the amplification and phylogenetic analysis results of coxI gene. We found that rotifer exposure did not significantly affect the hatching rate of B. straminea eggs but promoted the killing of juvenile snails. Meanwhile, rotifer exposure did not significantly alter the fecundity of B. straminea quantified by the number of eggs per egg mass, the number of egg masses per snail, and the number of eggs per snail; but the snails exposed to rotifers showed lower fecundity performance than the control snails. Importantly, rotifer exposure could significantly affect the development of juvenile B. straminea, showing a smaller shell diameter of the exposed snails than that of the control snails. In addition, rotifer exposure affected the life span of B. straminea snails, showing a 16.61% decline in the average life span. After rotifer exposure, the S. mansoni-infected B. straminea snails died significantly faster than those without rotifer exposure. Similar findings were observed in S. mansoni-infected Biomphalaria glabrata snails. These results implied that rotifer exposure significantly promoted the mortality of S. mansoni-infected B. straminea and B. glabrata.
Conclusions: Our study demonstrated the potential molluscicide effect of rotifers on intermediate hosts under laboratory conditions. Our findings may provide new insights into the development of biocontrol strategies for snail-borne disease transmission.
Schistosomiasis is one of the most important human parasitic diseases (Chitsulo et al., 2000), causing almost 240 million people infected worldwide, which may cause huge economic and social burdens globally (Colley et al., 2014). Among all human infected schistosomes, Schistosoma mansoni is the most widespread species. S. mansoni is distributed predominantly in South America, Africa, the Caribbean, and the Middle East (Crompton, 1999; Chitsulo et al., 2000; Colley et al., 2014). Biomphalaria snails, including Biomphalaria glabrata and Biomphalaria straminea, are the main intermediate hosts of S. mansoni (Colley et al., 2014). As an important intermediate host of S. mansoni (Coelho and Caldeira, 2016), the freshwater snail B. straminea has invaded Hong Kong, China, since 1974 and has spread widely in South China (Meier-Brook, 1974; Lin et al., 2020). In addition, this invasive snail can also transmit the zoonotic parasite Angiostrongylus cantonensis (Xu et al., 2019; Zhu et al., 2019). Considering the potential risk of transmission of S. mansoni and threats to human health in China (Colley et al., 2014; Lin et al., 2020), it is necessary to pay more attention to monitoring and controlling B. straminea with close surveillance and control strategies.
The strategy of controlling intermediate hosts has been proven to be an effective approach to interrupt the transmission of S. mansoni (Lardans and Dissous, 1998). The application of chemical molluscicides is a major strategy for snail control. Niclosamide is the only molluscicide recommended by the WHO (Yang et al., 2010). However, the environmental effects, high toxicity to non-target organisms, and high costs in most endemic countries have hampered the widespread use of chemical molluscicides (Ekabo et al., 1996; Oliveira-Filho and Paumgartten, 2000). In addition, the application of niclosamide may induce resistance (Dai et al., 2015). To achieve the UN Sustainable Development Goals (SDGs), alternative tools for intermediate hosts and schistosomiasis control are urgently needed. In recent years, biocontrol strategies have attracted significant research attention due to their low toxicity and environmentally friendly features (de Oliveira et al., 2004; Soberon et al., 2013; Wei et al., 2017). Therefore, low toxicity and environmentally friendly tools are urgently needed and suited for invasive snail control.
There is a vast amount of zooplankton in rivers and oceans. As an important type of zooplankton, rotifers are widely distributed in freshwater bodies (Gilbert, 2017). Rotifers are an important food source of fishes in aquaculture (Stelzer, 2009; Dabrowski and Miller, 2018) and can also be indicators of environmental toxicity (Stelzer, 2009; Dabrowski and Miller, 2018; Colvin et al., 2021; Xu et al., 2021) and water quality (Jose et al., 2008; Picapedra et al., 2021). Nevertheless, rotifers can promote mortality by affecting the ingestion of shrimp (Yan et al., 2004; Yan et al., 2007) and cause tissue injury and fish death by attaching to the gills (Imai et al., 1991; Xu et al., 1999; Xu et al., 2000). Mass rotifers may rob food and nutrition from aquatic animals, inducing unhealthy status and mortality among aquatics (Meyabeme et al., 2010; Reyes-Prieto et al.2014; Ranasinghe and Amarasinghe 2020). B. straminea and B. glabrata are important freshwater snails and invasive species globally. In addition, the identification of microbiota as food competitors, such as rotifers, could be a potential additional tool for mosquito control (Ranasinghe and Amarasinghe, 2020). However, whether rotifers can be competitors of freshwater snails is unclear. Few studies have focused on controlling intermediate hosts by rotifers. Therefore, we hypothesized that rotifers could affect the development and survival of Biomphalaria snails.
In the present study, we collected rotifer samples from field studies in South China from 2016 to 2017 and investigated the influence and survival of Biomphalaria snails affected by rotifer exposure. Our findings may promote the development of biocontrol strategies for intermediate hosts.
To collect the rotifer samples in Guangdong Province, we conducted systematic field surveys from 2016 to 2017. We collected about 100 snails from each sampling site. We found some rotifers were attaching to the surface of the B. straminea shell. Then, we collected samples and transferred alive rotifers to the laboratory. The name of the locality, Global Positioning System (GPS) coordinates, and date were recorded. We took pictures of the surroundings using a camera. The living specimens were maintained under laboratory conditions. We finally preserved several samples in 95% ethanol and stored them in −80°C for further processing.
We removed the shell from the snail before genomic DNA extraction. Total DNA was extracted separately from approximately 30 mg of head–foot or the entire rotifer. All samples were individually crushed using a bead mill in an enzyme-free Eppendorf tube with 1-mm-diameter inox beads (Qiagen, Germany). After removing the beads, we extracted total DNA using the hipure DNA mini kit (Magen, China) as previously described (Lin et al., 2020). Briefly, genomic DNA was extracted according to the protocol of the kit, and finally, total DNA was suspended in 30 μl of nuclease-free buffer and stored at −80°C until further processing. The DNA quality and quantity were examined using a NanoDrop instrument (Thermo Fisher Scientific, USA).
The DNA samples were amplified for identification as described in the previous study (Lin et al., 2020). The universal cytochrome oxidase subunit (cox) I primer set for rotifer identification was used: LCO1490 5′-GGTCAACAAATCATAAAGATATTGG-3′ and HCO2198 5′-TAAACTTCAGGGTGACCAAAAAATCA-3′. The PCR amplification system for the target gene comprises 1 μl of cDNA, 12.5 μl of a mixture, 1 μl of forward primer, 1 μl of reverse primer, and 9.5 μl of double deionized water. The PCR cycling conditions were carried out: initial denaturation step at 94°C for 5 min followed by 30 cycles of 94°C for 45 s, 48°C for 45 s, and 72°C for 45 s with a final extension step at 72°C for 10 min. In addition, The universal coxI primer set was also used for the Biomphalaria species identification. The PCR conditions for the marker amplification were performed: denaturation at 94°C for 5 min, 30 cycles of 94°C for 50 s, 55°C for 50 s, 72°C for 50 s, and final extension at 72°C for 10 min. The PCR products were detected on 3% agarose gel electrophoresis and purified according to the protocol of the Qiagen gel extraction kit (Qiagen, Germany). The purified PCR products were sequenced on an ABI-3730 platform (Applied Biosystems) by the Majorbio company (Guangzhou, China).
The sequences obtained from sequencing and the National Center for Biotechnology Information (NCBI) databases (https://www.ncbi.nlm.nih.gov/) were aligned and concatenated by the neighbor-joining method using the molecular evolutionary genetics analysis (MEGA) 7 (Kumar et al., 2016). We performed the parsimony analysis by generating 1,500 bootstrap replicates.
The Biomphalaria snails were raised under laboratory conditions as described in the previous study (Lin et al., 2020). Each snail was exposed to 10 S. mansoni miracidia. The procedures for infecting snails with miracidia were described in the previous study (Keiser et al., 2014). The S. mansoni-exposed snails were maintained with shading treatment. The infection rate was measured as described in the previous study (Fernandez and Thiengo, 2002). The release of cercaria from Biomphalaria was previously described (Lin et al., 2020).
To investigate the effect of rotifers, we used eggs, juvenile, and mature snails to perform further experiments. i) We randomly divided eggs or snails on the same developmental stage into two groups: normal snails being exposed with or without rotifers. ii) S. mansoni miracidia-infected snails were also randomly divided into two groups: snails being exposed with or without rotifers. We selected the 2-week-old Biomphalaria snails for S. mansoni-infected experiments. After S. mansoni miracidia exposure experiments, both the exposed and unexposed snails were maintained under the same conditions. The survival rate was measured. The snail releasing S. mansoni cercaria was considered an infected snail.
The fecundity (the number of eggs per egg mass, the number of egg masses per snail, and the number of eggs per snail) and fertility (rate of eggs hatched per mass) were measured as previously described (Costa et al., 2004). The number of hatching embryos was examined in 2 weeks, and subsequently, the egg hatchability was calculated. The survival and growth rates (shell diameter) were measured. The snails being measured for the shell diameter were randomly picked out from the alive juvenile snails.
We calculated the results using GraphPad Prism version 6.0 (GraphPad Software, USA). Data are expressed as the mean ± standard error of the mean (SEM). The differences between groups were analyzed by Student’s t-test using SPSS 19.0 software (SPSS Inc., USA). The survival rates between groups were analyzed using the chi-square test. *p < 0.05 was considered statistically significant.
We found that some rotifers were attached to the surface of the shell of Biomphalaria snails in field studies. Then, we collected rotifer samples from the sites of the Guanlan River (22°40′18" N and 114°2′25" E) and Donghu Park (22°33′26" N and 114°8′38" E) in Shenzhen in South China (Figure 1A). Pictures of the surroundings of these sampling sites are shown (Figures 1B, C). In addition, maximum-likelihood trees showed that both the red and black phenotypic Biomphalaria snails collected from Shenzhen were similar to the South American B. straminea strain (Figures 1D, E).
Figure 1 Sampling site study. The map showing sampling sites in Shenzhen (A) and pictures of rotifer habitats in the Guanlan River (B) and Donghu Park (C). The red spot represents the rotifer sampling site. Both red and black phenotypic Biomphalaria snails were found in sampling site 1 (D), and black snails were collected in site 2. The red arrow shows the red Biomphalaria snail observed, and the black arrow shows the black Biomphalaria snail observed. (E) Neighbor-joining tree constructed based on the K2P+G model for coxI sequences obtained from National Center for Biotechnology Information (NCBI) database and Biomphalaria samples collected from Shenzhen. This map was created using ArcGIS.
We found that the rotifers were mainly attached to the navels of Biomphalaria snails (Figure 2A). To determine the species identification of rotifers from sampling sites, coxI gene was amplified and sequenced for phylogenetic reconstruction. The PCR fragments of coxI gene of rotifer were amplified and resolved in an agarose gel (Figure 2B). The five referenced sequences of mitochondrial genes obtained from the NCBI database included EF650549.1 (uncultured bdelloid rotifer), DQ078567.1 (Philodina sp.), HM032977.1 (Philodina sp. Pha3), DQ078584.1 (Philodina sp.), and MT895717.1 (Culex quinquefasciatus) (Figure 2C). We found that our samples (Isolations 1 and 2) clustered on the same branch, similar to the branches of uncultured bdelloid rotifers and Philodina sp. (Figure 2C). The sequence similarity between these two clusters was greater than 97% according to the BLAST results (https://blast.ncbi.nlm.nih.gov/Blast.cgi). These results showed that our rotifer samples belonged to the genus Philodina. Therefore, we named our rotifer samples collected from Shenzhen Philodina sp. sz1 and Philodina sp. sz2.
Figure 2 Amplification and phylogenetic analysis of rotifers collected in fields. (A) Pictures of rotifers attaching to navels of Biomphalaria straminea snail compared with control snail. The red arrow shows the rotifers observed. (B) Picture of PCR amplification based on coxI sequence extracted from rotifers (Lane 1 and 2). #, Rotifer isolation. Negative control (Blank). Marker: about 750 bp. (C) Neighbor-joining tree constructed based on K2P+G model for coxI sequences obtained from National Center for Biotechnology Information (NCBI) database and rotifer samples (Isolations 1 and 2) collected from Shenzhen.
To investigate the effect of rotifers on the hatching rate of B. straminea, we randomly divided egg masses of B. straminea into two groups. We observed gelatinous intima and extima on the egg masses (Figure 3A). Rotifers were only attached on the edge of the Biomphalaria egg mass and segregated into eggs by the extima and intima (Figure 3A). We found no significant difference in the hatching rates between groups infected with (0.7839 ± 0.03658) or without (0.8267 ± 0.02927) rotifers, but on average, the hatching rate declined in the rotifer-infected masses (Figure 3B).
Figure 3 The effect of rotifer exposure on Biomphalaria straminea egg masses. (A) Pictures of rotifers attaching to the egg mass of B straminea compared with control snail (left). The green arrow shows the extima of the egg mass. The black arrow shows the intima of egg mass. The red arrow shows rotifers. (B) The difference of hatching rate of egg mass between with and without rotifer exposure. ns, Not statistically significant.
As our results showed before, there was no significantly different effect on the hatchability. However, the required times for juvenile snails to hatch from the egg mass were not similar, ranging from 5 to 14 days. The juvenile snails hatching from egg masses were exposed to rotifers (Figure 4A), and the juvenile snails immediately become infected with rotifers after hatching. We found that the survival rate of juveniles in the infected group declined significantly compared with that of the control snails (Figure 4B). In addition, rotifers affected the development of juveniles, which showed a significantly smaller shell diameter than the control snails (Figure 4C).
Figure 4 The effect of rotifer exposure on juvenile Biomphalaria straminea snails. (A) Pictures of juvenile B. straminea being exposed to rotifers (right) and control group with rotifer exposure (left). (B) The difference of survival rate of juvenile B. straminea between with and without rotifer exposure in 6 weeks. (C) The influence on the development of juvenile B. straminea exposed to rotifers. This result was quantified by the shell diameter of B. straminea.
We found no significant differences in the number of egg masses per snail per day (Figure 5A), the number of eggs per snail (Figure 5B), or the number of eggs per mass (Figure 5C). However, rotifer exposure significantly affected the oviposition time of juvenile B. straminea, indicating that rotifers may delay the sexual development of B. straminea (Figure 5D and Table 1). Our study showed that there was no significant difference in the fecundity of snails infected with or without rotifers.
Figure 5 The effect of rotifer exposure on the fecundity of Biomphalaria straminea. (A) The difference in the number of egg mass per snail per day. (B) The difference in the number of eggs per snail. (C) The difference in the number of eggs per mass. (D) The difference in oviposition time of juvenile B. straminea snails exposed to rotifers or without rotifers. ns, Not statistically significant.
Table 1 The average differences in the oviposition time of Biomphalaria straminea exposed to rotifers or without rotifers (control group).
To test whether rotifer exposure could impact aging and affect life span, we treated juvenile B. straminea with rotifers. We measured the life spans of both exposed snails and control snails (without rotifer exposure). Since our previous results showed that rotifer exposure significantly affected the survival rate of juvenile B. straminea, we selected 5-week-old snails for further studies. We found that exposed B. straminea experienced dramatic life span shortening as compared with the control group (Figure 6, Table 2), showing a 16.61% decline in median life span after rotifer exposure.
Figure 6 The survival analysis of Biomphalaria straminea exposed to rotifers or without rotifers (control group).
Table 2 The average difference in the life spans of Biomphalaria straminea exposed to rotifers or without rotifers (control group).
As an intermediate host of S. mansoni, B. straminea plays an important role in the transmission of S. mansoni. Therefore, reducing the transmission risk of S. mansoni can be helpful for disease control. However, we found that rotifer exposure did not significantly alter the infection rate of S. mansoni-exposed B. straminea snails (Figure 7A). To conduct further experiments, we used positive snails that can release the cercaria of S. mansoni. After rotifer exposure, the S. mansoni-infected B. straminea died faster than the unexposed snails (Figure 7B and Table 3), showing a decline in the release time of cercaria from intermediate hosts.
Figure 7 The effect of rotifer exposure on the survival of Schistosoma mansoni-infected Biomphalaria straminea snails. (A) Rotifer exposure did not significantly affect the infection rate of B straminea to S. mansoni. (B) Rotifer exposure decreased the survival rate of S. mansoni-infected B. straminea. Unexposed: the S. mansoni-infected snail was not exposed to rotifers. Exposed: the S. mansoni-infected snail was exposed to rotifers. ns, Not statistically significant.
Table 3 The average difference in the life span of Schistosoma mansoni-infected Biomphalaria straminea after rotifer exposure.
B. glabrata is an important model organism for researching the interaction mechanism between S. mansoni and mollusks. We also detected the effect of rotifer exposure on the survival of S. mansoni-infected B. glabrata. We found that rotifer exposure did not significantly alter the infection rate of S. mansoni-exposed B. glabrata snails (Figure 8A). Since the infection rate was not 100% after S. mansoni miracidia exposure, we used positive snails that could release the cercaria of S. mansoni for further studies. We found that the S. mansoni-infected B. glabrata died significantly more than unexposed snails after 15 weeks of S. mansoni infection (Figure 8B). Rotifer exposure may accelerate the death of Biomphalaria snails infected with S. mansoni. Our results showed that rotifer exposure did not significantly alter the infection rate but significantly promoted the mortality of S. mansoni-exposed Biomphalaria snails, indicating the potential use of rotifer exposure on snail-borne disease transmission.
Figure 8 The effect of rotifer exposure on the survival of Schistosoma mansoni-infected Biomphalaria glabrata snails. (A) Rotifer exposure did not significantly affect the infection rate of B. glabrata to S. mansoni. (B) Rotifer exposure decreased the survival rate of S. mansoni-infected B glabrata in 15 weeks. ns, Not statistically significant.
The freshwater snail B. straminea, which plays an important role in the transmission of S. mansoni, is one of the most widely distributed species in the genus Biomphalaria and originated from the southeastern part of South America (Colley et al., 2014; Yang et al., 2018). During the last decades, B. straminea has been reported in tropical countries, including Brazil, Paraguay, Argentina, Uruguay, Colombia, and Costa Rica (Lin et al., 2020). B. straminea was first reported to be introduced into Hong Kong in 1974 and has now spread to Shenzhen, Dongguan, Huizhou, and Puning in South China (Colley et al., 2014; Yang et al., 2018). Historically, China has been a non-endemic area for blood flukes of S. mansoni. However, with the increasing imported schistosomiasis cases in China (Dai et al., 2020; Wang et al., 2020) and the spread of the intermediate host (Meier-Brook, 1974; Lin et al., 2020), the potential risk of transmission of S. mansoni is increasing. Considering the potential threats to human health, we should pay more attention and make efforts to manage these snails. Controlling intermediate hosts is considered an effective approach to interrupt the transmission of S. mansoni and control snail-borne disease schistosomiasis (Lu et al., 2018). Using chemical molluscicides to control snails was the major strategy. However, chemical molluscicides such as niclosamide are highly toxic to other aquatic animals. Therefore, environmentally friendly tools are urgently needed for intermediate host control. In the present study, we detected potential biocontrol strategies for the intermediate host of S. mansoni.
We reported the isolation of populations of rotifers collected from Shenzhen for biocontrol tools to the intermediate host of S. mansoni. According to coxI gene sequence analysis, we positioned these rotifers within the genus Philodina. Since these identity levels are greater than the genomic definition of a species based on coxI gene, we proposed to name our rotifer samples collected from Shenzhen rotifer Philodina sp. sz1 and rotifer Philodina sp. sz2. As important zooplankton, rotifers are distributed in all kinds of water bodies, though mainly in freshwater bodies (Lu et al., 2018). Rotifers naturally coexist with aquatic organisms and are an important food source of fish and shrimp (Stelzer, 2009; Dabrowski and Miller, 2018). However, previous studies have shown that rotifers can also affect the development and survival of fish and shrimp (Imai et al., 1991; Xu et al., 1999; Xu et al., 2000; Yan et al., 2004; Yan et al., 2007). Yet whether rotifers affect the development and survival of Gastropoda, such as B. straminea snails, is unknown.
B. straminea has already spread to Hong Kong and Guangdong provinces in South China (Lin et al., 2020). Our findings revealed that both the red and black phenotypic Biomphalaria snails collected from Shenzhen were similar to the South American B. straminea strain, implying that these two sites exhibited two kinds of invasive freshwater snail phenotypes, B. straminea. The population level of the intermediate host B. straminea may be associated with the number of snails exposed to S. mansoni in the field: the more snails that exist, the more snails that are infected (de Souza et al., 1981; Fernandez and Pieri, 2001; Gandasegui et al., 2018). We found that rotifer exposure did not significantly affect the hatching rate of Biomphalaria eggs, and we hypothesized that a gelatinous membrane may cover the eggs, protecting them from pathogens. The hatching rate of B. straminea in our study was similar to that in previous studies (Scherrer et al., 1976; Costa et al., 2004) but was lower than that of Biomphalaria pfeifferi (Kengne-Fokam et al., 2016). In addition, rotifer exposure did not significantly affect the fecundity of B. straminea, showing no difference, but declines in the number of eggs per egg mass, the number of egg masses per snail, and the number of eggs per snail. We hypothesized that the reason for these declines was that there was enough food to supply snails and protect the fecundity of snails. The fecundity performance of B. straminea in our work was lower than that of B. glabrata (Rozemberg et al., 1992; Costa et al., 2004). These results suggested that the fertility of the genus Biomphalaria snails may be associated with genotype.
Our study revealed that rotifer exposure can significantly affect the development of B. straminea snails according to the shell diameter results, implying a potential influence on the reproduction and maturity of Biomphalaria snails. Previous studies revealed that there was an increase in growth inhibition in S. mansoni-infected snails (Looker and Etges, 1979; Meier and Meier-Brook, 1981; Cardoso and Coelho, 1990). Therefore, the growth of rotifer-exposed snails was similar to that of S. mansoni-infected snails. However, the association between growth alterations and parasite infection is unclear. Further studies on the mechanism of growth inhibition by rotifers are needed.
Rotifer exposure affected the survival rate of B. straminea snails, mainly juvenile snails. Importantly, rotifer exposure caused a significant decline in the average life span of B. straminea snails. Although previous studies have attempted to explore control strategies for intermediate hosts in China, they have mainly focused on chemical molluscicides, including salicylanilidate (He et al., 2017) and pyridylphenylurea derivatives (Wang et al., 2018). We focused on environmentally friendly tools to control intermediate hosts. Our findings suggested that rotifers may become a potential biocontrol tool for the intermediate host of S. mansoni. As one of the biocontrol strategies, pathogenic bacteria, including Candidatus Paenibacillus glabratella (Duval et al., 2015), Bacillus thuringiensis (Soberon et al., 2013), and Beauveria bassiana (Wei et al., 2017), have been further studied and have become potential alternative tools in disease intervention. Although the application of chemical molluscicides such as niclosamide is the most widely used method for snail control (Lardans and Dissous, 1998), we believe that environmentally friendly tools for intermediate hosts will be obtained with increasing research on biocontrol strategies.
Our work revealed that rotifers did not significantly affect the survival rate of adult Biomphalaria snails or the infection rates of S. mansoni-exposed snails. The susceptibility of the Biomphalaria snails mainly depends on their immune system, not foreign organisms (Hanington et al., 2010; Pila et al., 2016). Our findings demonstrated that rotifers promoted the killing of S. mansoni-infected Biomphalaria snails, including B. straminea and B. glabrata, implying that rotifer exposure may decrease the releasing cercaria of S. mansoni over time and contribute to disease control. Trematode parasites and their molluscan hosts produce antioxidants and oxidants to maintain the cellular redox balance, which may explain their survival in the late stage of parasite infection (Bayne et al., 2001; Bayne, 2009; Mourao et al., 2009). Rotifers not only grab food from other species but also receive foreign DNA from the animal kingdom, fungi, plants, and bacteria (Gladyshev et al., 2008; Boschetti et al., 2012; Szydlowski et al., 2015). Therefore, rotifer exposure may increase the burden on the survival of Biomphalaria snails and ultimately induce an imbalance. These results implied that rotifer exposure may interrupt the immune balance between S. mansoni and host snails, leading to snail mortality. However, the mechanisms of these findings are unclear, and further studies are needed.
In our study, we identified a species of the genus Philodina rotifer collected from Shenzhen, South China. Rotifer exposure can alter the fecundity and significantly affect the fertility and life span of B. straminea, promote the death of juvenile snails, and significantly promote the mortality of S. mansoni-infected B. straminea and B. glabrata. Overall, our study demonstrated that rotifers may contribute to snail control and disease intervention by affecting the development and population quantity of Biomphalaria snails, in addition to S. mansoni-infected snails. Our results implied that rotifers may be a potential use and supplement in controlling snail-borne schistosomiasis transmission.
The data presented in the study are deposited in the GenBank repository, accession number (OK156495-OK156499). Please contact the author for additional data requests.
ZW, XS, and DL conceived and designed the study. DL carried out the experiments, prepared the manuscript, and handled the statistical analysis and interpretation of the data. DL, SX, BS, and YL critically revised the draft version of the paper. All authors contributed to the article and approved the submitted version.
This work was supported by the National Key R&D Program of China (Nos. 2020YFC1200100 and 2016YFC1200500), the Natural Science Foundation of Guangdong Province (Nos. 2019A1515012068 and 2021A1515010976), the 111 Project (No. B12003), the Pearl River Nova Program of Guangzhou (No.201710010030), the Fundamental Research Funds for the Central University (No. 17ykpy09), the National Natural Science Foundation of China (Nos. 81802036 and 81871682), and the Natural Science Foundation of Guangdong Province (No. 2020A1515010896).
The authors declare that the research was conducted in the absence of any commercial or financial relationships that could be construed as a potential conflict of interest.
All claims expressed in this article are solely those of the authors and do not necessarily represent those of their affiliated organizations, or those of the publisher, the editors and the reviewers. Any product that may be evaluated in this article, or claim that may be made by its manufacturer, is not guaranteed or endorsed by the publisher.
Thanks to the Jiangsu Institute of Parasitic Diseases, Jiangsu Province, China, for offering us the Schistosoma mansoni strain.
SDGs, Sustainable Development Goals; COX, cytochrome oxidase subunit; GPS, Global Positioning System; MEGA, molecular evolutionary genetics analysis.
Bayne, C. J. (2009). Successful Parasitism of Vector Snail Biomphalaria Glabrata by the Human Blood Fluke (Trematode) Schistosoma Mansoni: A 2009 Assessment. Mol. Biochem. Parasitol. 165 (1), 8–18. doi: 10.1016/j.molbiopara.2009.01.005
Bayne, C. J., Hahn, U. K., Bender, R. C. (2001). Mechanisms of Molluscan Host Resistance and of Parasite Strategies for Survival. Parasitol. 123 (Suppl), S159–S167. doi: 10.1017/s0031182001008137
Boschetti, C., Carr, A., Crisp, A., Eyres, I., Wang-Koh, Y., Lubzens, E., et al. (2012). Biochemical Diversification Through Foreign Gene Expression in Bdelloid Rotifers. PloS Genet. 8 (11), e1003035. doi: 10.1371/journal.pgen.1003035
Cardoso, G. S., Coelho, P. M. (1990). [Schistosoma Mansoni: Quantitative Aspects of the Fertility and Survival of Worms of Irradiated Cercariae (3 Krad), in Mice]. Rev. Inst Med. Trop. Sao Paulo. 32 (1), 28–35. doi: 10.1590/S0036-46651990000100005
Chitsulo, L., Engels, D., Montresor, A., Savioli, L. (2000). The Global Status of Schistosomiasis and its Control. Acta Trop. 77 (1), 41–51. doi: 10.1016/s0001-706x(00)00122-4
Coelho, P., Caldeira, R. L. (2016). Critical Analysis of Molluscicide Application in Schistosomiasis Control Programs in Brazil. Infect. Dis. Poverty. 5 (1), 57. doi: 10.1186/s40249-016-0153-6
Colley, D. G., Bustinduy, A. L., Secor, W. E., King, C. H. (2014). Human Schistosomiasis. Lancet. 383 (9936), 2253–2264. doi: 10.1016/S0140-6736(13)61949-2
Colvin, K. A., Parkerton, T. F., Redman, A. D., Lewis, C., Galloway, T. S. (2021). Miniaturised Marine Tests as Indicators of Aromatic Hydrocarbon Toxicity: Potential Applicability to Oil Spill Assessment. Mar. Pollut. Bull. 165:112151. doi: 10.1016/j.marpolbul.2021.112151
Costa, M. J., Grault, C. E., Confalonieri, U. E. (2004). Comparative Study of the Fecundity and Fertility of Biomphalaria Glabrata (Say 1818) and Biomphalaria Straminea (Dunker 1848) in a Laboratory Through Self-Fertilization and Cross-Fertilization. Rev. Inst Med. Trop. Sao Paulo. 46 (3), 157–163. doi: 10.1590/s0036-46652004000300007
Crompton, D. W. (1999). How Much Human Helminthiasis is There in the World? J. Parasitol. 85 (3), 397–403. doi: 10.2307/3285768
Dabrowski, K., Miller, M. (2018). Contested Paradigm in Raising Zebrafish (Danio Rerio). Zebrafish. 15 (3), 295–309. doi: 10.1089/zeb.2017.1515
Dai, S. M., Guan, Z., Zhang, L. J., Lv, S., Cao, C. L., Li, S. Z., et al. (2020). Imported Schistosomiasis, China 2010-2018. Emerg. Infect. Dis. 26 (1), 179–180. doi: 10.3201/eid2601.191250
Dai, J. R., Li, Y. Z., Wang, W., Xing, Y. T., Qu, G. L., Liang, Y. S. (2015). Resistance to Niclosamide in Oncomelania Hupensis, the Intermediate Host of Schistosoma Japonicum: Should We be Worried? Parasitology 142 (2), 332–340. doi: 10.1017/S0031182014000870
de Oliveira, E. J., Rabinovitch, L., Monnerat, R. G., Passos, L. K., Zahner, V. (2004). Molecular Characterization of Brevibacillus Laterosporus and its Potential Use in Biological Control. Appl. Environ. Microbiol. 70 (11), 6657–6664. doi: 10.1128/AEM.70.11.6657-6664.2004
de Souza, C. P., Rodrigues, M. S., de Azevedo, M. L., Araujo, N. (1981). [Susceptibility of Populations of Biomphalaria Straminea (Dunker 1848) From Minas Gerais, to Schistosoma Mansoni Infection]. Rev. Inst Med. Trop. Sao Paulo. 23 (5), 212–216.
Duval, D., Galinier, R., Mouahid, G., Toulza, E., Allienne, J. F., Portela, J., et al. (2015). A Novel Bacterial Pathogen of Biomphalaria Glabrata: A Potential Weapon for Schistosomiasis Control? PloS Negl. Trop. Dis. 9 (2), e3489. doi: 10.1371/journal.pntd.0003489
Ekabo, O. A., Farnsworth, N. R., Henderson, T. O., Mao, G., Mukherjee, R. (1996). Antifungal and Molluscicidal Saponins From Serjania Salzmanniana. J. Nat. Prod. 59 (4), 431–435. doi: 10.1021/np960208r
Fernandez, M. A., Pieri, O. S. (2001). Infection by Schistosoma Mansoni Sambon 1907 in the First Four Months of Life of Biomphalaria Straminea (Dunker 1848) in Brazil. Mem Inst Oswaldo Cruz. 96 (Suppl), 185–192. doi: 10.1590/s0074-02762001000900029
Fernandez, M. A., Thiengo, S. C. (2002). Susceptibility of Biomphalaria Straminea (Dunker 1848) From Serra Da Mesa Dam, Goias, Brazil to Infection With Three Strains of Schistosoma Mansoni Sambon 1907. Mem Inst Oswaldo Cruz. 97 (Suppl 1), 59–60. doi: 10.1590/s0074-02762002000900013
Gandasegui, J., Fernandez-Soto, P., Muro, A., Simoes, B. C., Lopes, D. M. F., Loyo, R., et al. (2018). A Field Survey Using LAMP Assay for Detection of Schistosoma Mansoni in a Low-Transmission Area of Schistosomiasis in Umbuzeiro, Brazil: Assessment in Human and Snail Samples. PloS Negl. Trop. Dis. 12 (3), e6314. doi: 10.1371/journal.pntd.0006314
Gilbert, J. J. (2017). Non-Genetic Polymorphisms in Rotifers: Environmental and Endogenous Controls, Development, and Features for Predictable or Unpredictable Environments. Biol. Rev. Camb Philos. Soc 92 (2), 964–992. doi: 10.1111/brv.12264
Gladyshev, E. A., Meselson, M., Arkhipova, I. R. (2008). Massive Horizontal Gene Transfer in Bdelloid Rotifers. Science 320 (5880), 1210–1213. doi: 10.1126/science.1156407
Hanington, P. C., Forys, M. A., Dragoo, J. W., Zhang, S. M., Adema, C. M., Loker, E. S. (2010). Role for a Somatically Diversified Lectin in Resistance of an Invertebrate to Parasite Infection. Proc. Natl. Acad. Sci. U. S. A. 107 (49), 21087–21092. doi: 10.1073/pnas.1011242107
He, P., Wang, W., Sanogo, B., Zeng, X., Sun, X., Lv, Z., et al. (2017). Molluscicidal Activity and Mechanism of Toxicity of a Novel Salicylanilide Ester Derivative Against Biomphalaria Species. Parasit Vectors. 10 (1), 383. doi: 10.1186/s13071-017-2313-3
Imai, S., Miyazaki, H., Nomura, K. (1991). Trichodinid Species From the Gill of Cultured Japanese Eel, Anguilla Japonica, With the Description of a New Species Based on Light and Scanning Electron Microscopy. Eur. J. Protistol. 27 (1), 79–84. doi: 10.1016/S0932-4739(11)80430-X
Jose, D. P. S., Paggi, J., Collins, P., Collins, J., Graciela, B. (2008). Water Quality and Zooplankton Composition in a Receiving Pond of the Stormwater Runoff From an Urban Catchment. J. Environ. Biol. 29 (5), 693–700.
Keiser, J., Vargas, M., Rubbiani, R., Gasser, G., Biot, C. (2014). In Vitro and In Vivo Antischistosomal Activity of Ferroquine Derivatives. Parasit Vectors. 7:424. doi: 10.1186/1756-3305-7-424
Kengne-Fokam, A. C., Nana-Djeunga, H. C., Djuikwo-Teukeng, F. F., Njiokou, F. (2016). Analysis of Mating System, Fecundity, Hatching and Survival Rates in Two Schistosoma Mansoni Intermediate Hosts (Biomphalaria Pfeifferi and Biomphalaria Camerunensis) in Cameroon. Parasit Vectors. 9:10. doi: 10.1186/s13071-015-1285-4
Kumar, S., Stecher, G., Tamura, K. (2016). MEGA7: Molecular Evolutionary Genetics Analysis Version 7.0 for Bigger Datasets. Mol. Biol. Evol. 33 (7), 1870–1874. doi: 10.1093/molbev/msw054
Lardans, V., Dissous, C. (1998). Snail Control Strategies for Reduction of Schistosomiasis Transmission. Parasitol Today 14 (10), 413–417. doi: 10.1016/s0169-4758(98)01320-9
Lin, D., Zeng, X., Sanogo, B., He, P., Xiang, S., Du, S., et al. (2020). The Potential Risk of Schistosoma Mansoni Transmission by the Invasive Freshwater Snail Biomphalaria Straminea in South China. PloS Negl. Trop. Dis. 14 (6), e8310. doi: 10.1371/journal.pntd.0008310
Looker, D. L., Etges, F. J. (1979). Effect of Schistosoma Mansoni Infection on Fecundity and Perivitelline Fluid Composition in Biomphalaria Glabrata. J. Parasitol. 65 (6), 880–885. doi: 10.2307/3280241
Lu, X. T., Gu, Q. Y., Limpanont, Y., Song, L. G., Wu, Z. D., Okanurak, K., et al. (2018). Snail-Borne Parasitic Diseases: An Update on Global Epidemiological Distribution, Transmission Interruption and Control Methods. Infect. Dis. Poverty. 7 (1), 28. doi: 10.1186/s40249-018-0414-7
Meier-Brook, C. (1974). A Snail Intermediate Host of Schistosoma Mansoni Introduced Into Hong Kong. Bull. World Health Organ. 51 (6), 661.
Meier, M., Meier-Brook, C. (1981). Schistosoma Mansoni: Effect on Growth, Fertility, and Development of Distal Male Organs in Biomphalaria Glabrata Exposed to Miracidia at Different Ages. Z Parasitenkd. 66 (2), 121–131. doi: 10.1007/BF00925719
Meyabeme, E. A., Liess, M., Duquesne, S. (2010). Influence of Competing and Predatory Invertebrate Taxa on Larval Populations of Mosquitoes in Temporary Ponds of Wetland Areas in Germany. J. Vector Ecol. 35 (2), 419–427. doi: 10.1111/j.1948-7134.2010.00101.x
Mourao, M. M., Dinguirard, N., Franco, G. R., Yoshino, T. P. (2009). Role of the Endogenous Antioxidant System in the Protection of Schistosoma Mansoni Primary Sporocysts Against Exogenous Oxidative Stress. PloS Negl. Trop. Dis. 3 (11), e550. doi: 10.1371/journal.pntd.0000550
Oliveira-Filho, E. C., Paumgartten, F. J. (2000). Toxicity of Euphorbia Milii Latex and Niclosamide to Snails and Nontarget Aquatic Species. Ecotoxicol. Environ. Saf. 46 (3), 342–350. doi: 10.1006/eesa.2000.1924
Picapedra, P., Fernandes, C., Baumgartner, G., Sanches, P. V. (2021). Zooplankton Communities and Their Relationship With Water Quality in Eight Reservoirs From the Midwestern and Southeastern Regions of Brazil. Braz. J. Biol. 81 (3), 701–713. doi: 10.1590/1519-6984.230064
Pila, E. A., Gordy, M. A., Phillips, V. K., Kabore, A. L., Rudko, S. P., Hanington, P. C. (2016). Endogenous Growth Factor Stimulation of Hemocyte Proliferation Induces Resistance to Schistosoma Mansoni Challenge in the Snail Host. Proc. Natl. Acad. Sci. U. S. A. 113 (19), 5305–5310. doi: 10.1073/pnas.1521239113
Ranasinghe, H., Amarasinghe, L. D. (2020). Naturally Occurring Microbiota in Dengue Vector Mosquito Breeding Habitats and Their Use as Diet Organisms by Developing Larvae in the Kandy District, Sri Lanka. BioMed. Res. Int. 2020:5830604. doi: 10.1155/2020/5830604
Reyes-Prieto, M., Oceguera-Figueroa, A., Snell, S., Negredo, A., Barba, E., Fernandez, L., et al. (2014). DNA Barcodes Reveal the Presence of the Introduced Freshwater Leech Helobdella Europaea in Spain. Mitochondrial DNA. 25 (5), 387–393. doi: 10.3109/19401736.2013.809426
Rozemberg, B., Rey, L., Pieri, O. S. (1992). Fecundity of Biomphalaria Straminea and B. Glabrata in the Laboratory: A 12-Month Comparative Study. Mem Inst Oswaldo Cruz. 87 (Suppl 1), 223–232. doi: 10.1590/s0074-02761992000500042
Scherrer, J. F., Chquiloff, M. A., de Freitas, J. R. (1976). [Comparative Study on the Reproduction in 4 Genetic Variations of Biomphalaria Glabrata (Say 1818) I. Fertility. Rev. Inst. Med. Trop. Sao Paulo. 18 (5), 315-321.
Soberon, M., Lopez-Diaz, J. A., Bravo, A. (2013). Cyt Toxins Produced by Bacillus Thuringiensis: A Protein Fold Conserved in Several Pathogenic Microorganisms. Peptides. 41, 87–93. doi: 10.1016/j.peptides.2012.05.023
Stelzer, C. P. (2009). Automated System for Sampling, Counting, and Biological Analysis of Rotifer Populations. Limnol Oceanogr Methods 7, 856–864. doi: 10.4319/lom.2009.7.856
Szydlowski, L., Boschetti, C., Crisp, A., Barbosa, E. G., Tunnacliffe, A. (2015). Multiple Horizontally Acquired Genes From Fungal and Prokaryotic Donors Encode Cellulolytic Enzymes in the Bdelloid Rotifer Adineta Ricciae. Gene 566 (2), 125–137. doi: 10.1016/j.gene.2015.04.007
Wang, W., Mao, Q., Yao, J., Yang, W., Zhang, Q., Lu, W., et al. (2018). Discovery of the Pyridylphenylureas as Novel Molluscicides Against the Invasive Snail Biomphalaria Straminea, Intermediate Host of Schistosoma Mansoni. Parasit Vectors. 11 (1), 291. doi: 10.1186/s13071-018-2868-7
Wang, L., Wu, X., Li, X., Zheng, X., Wang, F., Qi, Z., et al. (2020). Imported Schistosomiasis: A New Public Health Challenge for China. Front. Med. (Lausanne). 7, 553487. doi: 10.3389/fmed.2020.553487
Wei, G., Lai, Y., Wang, G., Chen, H., Li, F., Wang, S. (2017). Insect Pathogenic Fungus Interacts With the Gut Microbiota to Accelerate Mosquito Mortality. Proc. Natl. Acad. Sci. U. S. A. 114 (23), 5994–5999. doi: 10.1073/pnas.1703546114
Xu, X., Chen, T., Xie, A., Yang, X., Wei, X. (2021). Chronic Effects of Bromate on Sexual Reproduction of Freshwater Rotifer Brachionus Calyciflorus. Bull. Environ. Contam. Toxicol. 106 (2), 270–277. doi: 10.1007/s00128-021-03103-z
Xu, K., Meng, F., Song, W. (2000). Scanning Electron Microscopic Observations on the Histopathology of Trichodiniasis of the Mariculture Fish, Lateolabrax Japonicus (In Chinese). J. Ocean Univ. Qingdao (03), 418–422. doi: 10.16441/j.cnki.hdxb.2000.03.009
Xu, K., Song, W., Warren, A. (1999). Trichodinid Ectoparasites (Ciliophora: Peritrichida) From the Gills of Cultured Marine Fishes in China, With the Description of Trichodinella Lomi N. Sp. Syst. Parasitol. 42 (3), 219–227. doi: 10.1023/a:1006067005936
Xu, L., Xu, M., Sun, X., Xu, J., Zeng, X., Shan, D., et al. (2019). The Genetic Basis of Adaptive Evolution in Parasitic Environment From the Angiostrongylus Cantonensis Genome. PloS Negl. Trop. Dis. 13 (11), e7846. doi: 10.1371/journal.pntd.0007846
Yan, D. C., Dong, S. L., Huang, J., Yu, X. M., Feng, M. Y., Liu, X. Y. (2004). White Spot Syndrome Virus (WSSV) Detected by PCR in Rotifers and Rotifer Resting Eggs From Shrimp Pond Sediments. Dis. Aquat. Organ. 59 (1), 69–73. doi: 10.3354/dao059069
Yang, Y., Cheng, W., Wu, X., Huang, S., Deng, Z., Zeng, X., et al. (2018). Prediction of the Potential Global Distribution for Biomphalaria Straminea, an Intermediate Host for Schistosoma Mansoni. PloS Negl. Trop. Dis. 12 (5), e6548. doi: 10.1371/journal.pntd.0006548
Yang, G. J., Li, W., Sun, L. P., Wu, F., Yang, K., Huang, Y. X., et al. (2010). Molluscicidal Efficacies of Different Formulations of Niclosamide: Result of Meta-Analysis of Chinese Literature. Parasit Vectors. 3, 84. doi: 10.1186/1756-3305-3-84
Yan, D., Yan, J., Deng, Y. (2007). Advances in the Research of Rotifer Diseases (In Chinese). Reservoir Fisheries (05), 105–107. doi: 10.3969/j.issn.1003-1278.2007.05.044
Keywords: Biomphalaria straminea, Biomphalaria glabrata, Schistosoma mansoni, rotifer, Philodina, biocontrol strategy
Citation: Lin D, Xiang S, Sanogo B, Liang Y, Sun X and Wu Z (2021) Molecular Characterization of Rotifers and Their Potential Use in the Biological Control of Biomphalaria. Front. Cell. Infect. Microbiol. 11:744352. doi: 10.3389/fcimb.2021.744352
Received: 20 July 2021; Accepted: 11 August 2021;
Published: 21 September 2021.
Edited by:
Gaoqian Feng, Burnet Institute, AustraliaReviewed by:
Chaoming Xia, Soochow University Medical College (SUMC), ChinaCopyright © 2021 Lin, Xiang, Sanogo, Liang, Sun and Wu. This is an open-access article distributed under the terms of the Creative Commons Attribution License (CC BY). The use, distribution or reproduction in other forums is permitted, provided the original author(s) and the copyright owner(s) are credited and that the original publication in this journal is cited, in accordance with accepted academic practice. No use, distribution or reproduction is permitted which does not comply with these terms.
*Correspondence: Zhongdao Wu, d3V6aGRAbWFpbC5zeXN1LmVkdS5jbg==; Xi Sun, c3VueGkyQG1haWwuc3lzdS5lZHUuY24=
Disclaimer: All claims expressed in this article are solely those of the authors and do not necessarily represent those of their affiliated organizations, or those of the publisher, the editors and the reviewers. Any product that may be evaluated in this article or claim that may be made by its manufacturer is not guaranteed or endorsed by the publisher.
Research integrity at Frontiers
Learn more about the work of our research integrity team to safeguard the quality of each article we publish.