- 1Escola de Veterinária e Zootecnia, Universidade Federal de Goiás, Goiânia, Brazil
- 2Instituto de Patologia Tropical e Saúde Pública, Universidade Federal de Goiás, Goiânia, Brazil
- 3Instituto de Ciências Biológicas, Universidade Federal de Goiás, Goiânia, Brazil
- 4Department of Microbiology and Cell Science, University of Florida, Gainesville, FL, United States
Beauveria bassiana holds promise as a feasible biological control agent for tick control. The B. bassiana stress–response transcription factor Msn2 is known to contribute to fungal growth, conidiogenesis, stress–response and virulence towards insects; however, little is known concerning whether Msn2 is involved in infection across Arthropoda classes. We evaluated the effects of Msn2 on B. bassiana virulence against Rhipicephalus microplus (Acari, Ixodidae) using wild-type, targeted gene knockout (ΔBbmsn2) and complemented mutant (ΔBbmsn2/Bbmsn2) strains. Reproductive parameters of R. microplus engorged females treated topically or by an intra-hemocoel injection of conidial suspensions were assessed. Treated cuticles of engorged females were analyzed by microscopy, and proteolytic activity of B. bassiana on cuticles was assessed. Topically treated engorged females showed high mean larval hatching (>84%) in control and ΔBbmsn2 treatments, whereas treatment with the wild-type or ΔBbmsn2/Bbmsn2 strains resulted in significantly decreased (lowered egg viability) larval hatching. Percent control of R. microplus topically treated with ΔBbmsn2 was lower than in the groups treated with wild-type (56.1%) or ΔBbmsn2/Bbmsn2 strains. However, no differences on reproductive parameters were detected when R. microplus were treated by intra-hemocoel injection using low (800 conidia/tick) doses for all strains tested; R. microplus injected with high doses of wild-type or mutant strains (106 conidia/tick) died before laying eggs (~48 h after treatment). SEM analyses of B. bassiana infection showed similar conidial germination and formation of pseudo-appressoria on tick cuticle. Histological sections of ticks treated with the wild-type or ΔBbmsn2/Bbmsn2 strains showed fungal penetration through the cuticle, and into the tick interior. Hyphae of ΔBbmsn2, however, did not appear to penetrate or breach the tick exocuticle 120 h after treatment. Protease activity was lower on tick cuticles treated with ΔBbmsn2 than those treated with the wild-type or ΔBbmsn2/Bbmsn2 strains. These data show that loss of the Msn2 transcription factor reduced B. bassiana virulence against R. microplus, but did not interfere with conidial germination, appressoria formation or sporulation on tick cadavers, and plays only a minimal role once the cuticle is breached. Our results indicate that the BbMsn2 transcription factor acts mainly during the fungal penetration process and that decreased protease production may be one mechanism that contributes to the inability of the mutant strain to breach the tick cuticle.
Introduction
Beauveria bassiana (Hypocreales: Cordycipitaceae) is one of the most widely studied entomopathogenic fungi for applied tick control (Kirkland et al., 2004a; Kirkland et al., 2004b; Fernandes et al., 2012). The potential of this fungus to control Rhipicephalus microplus (Acari: Ixodidae) has been shown in laboratory assays, with variable virulence among B. bassiana isolates (Posadas and Lecuona, 2009; Campos et al., 2010; Fernandes et al., 2011; Sun et al., 2013). According to Fernandes et al. (2011), the mean lethal concentration of B. bassiana to kill 50% (LC50) of R. microplus engorged female can vary from 107 to 109 conidia ml−1.
B. bassiana conidia infect ticks through their cuticle or natural openings (Bernardo et al., 2018). The process of fungal infection on ticks is thought to be similar to that known for insects (Arruda et al., 2005), and includes conidial adhesion on the host cuticle, production of germ tube, differentiation into appressorium (something not seen for all B. bassiana isolates), penetration through the host cuticle by enzymatic action (e.g., lipases, proteases and chitinases) and mechanical pressure, and growth within the host integument and hemocoel (colonization stage) (Ortiz-Urquiza and Keyhani, 2016). The host dies by tissue destruction and by action of toxins from fungi (Schrank and Vainstein, 2010). However, ticks are known to potentially display significantly higher natural resistances to insect pathogenic fungi, and acaricidal specific factors may be produced by these fungi (Kirkland et al., 2004a, Kirkland et al., 2004b, Kirkland et al., 2005).
As mentioned, the virulence of B. bassiana is influenced by the production of proteases, particularly the subtilisin-like protease called Pr1 (Joshi et al., 1995), chitinases and lipases (Fang et al., 2005) and, specifically against ticks, the metabolite oxalic acid has been shown to be important (Kirkland et al., 2005). In the last fifteen years, many studies have contributed to understanding the network of genes which are related to the virulence of B. bassiana (Jin et al., 2010; Ortiz-Urquiza and Keyhani, 2013; Wang et al., 2013; Valero-Jiménez et al., 2016). Fang et al. (2005) demonstrated that overproduction of the Bbchit1 chitinase enhanced the virulence of B. bassiana against aphids, as indicated by the significantly lower LC50 and mean lethal time to kill 50% (LT50) of target insects of the mutant strain compared to the wild-type strain. Another study using hybrid chitinase gene (Bbchit1–BmChBD) showed a 23% reduction of the LT50 to kill aphids (Myzus persicae) when they were treated with the mutant strain of B. bassiana in comparison to its wild-type (Fan et al., 2007).
Transcriptional regulation of effector genes in eukaryotic cells is one of the fundamental mechanisms involved in cellular responses to stress and/or virulence signaling pathways (Ortiz-Urquiza and Keyhani, 2015). In entomopathogenic fungi, the transcription factor Msn2 regulates the conidiogenesis of B. bassiana and Metarhizium robertsii; a knockout strain of each species (ΔBbmsn2 and ΔMrmsn2, respectively) reduced 43 and 39% the conidial production in comparison to their respective wild-type strains (Liu et al., 2013). Also, ΔBbmsn2 and ΔMrmsn2 strains had a reduced cell tolerance to chemical and environmental stresses. Decreased virulence of ΔBbmsn2 and ΔMrmsn2 against Spodoptera litura (Lepidoptera: Noctuidae) second-instar larvae and Tenebrio molitor (Coleoptera: Tenebrionidae) third-instar larvae was recorded; the LT50 values were 28 and 25% longer than the control strains of B. bassiana (5.5 days) and M. robertsii (4.8 days), respectively. In Galleria mellonella (Lepidoptera: Pyralidae) larvae, the LT50 significantly increased when treated with the knockout strain (LT50 = 3.4 ± 0.08 days) in comparison to the wild-type strain (2.71 ± 0.03 days) (Luo et al., 2015). These data confirmed that Msn2 is critical for entomopathogenic fungal infection of insects; however, any similar role towards Acari has not been investigated. Furthermore, to date, no histological investigations related to Msn2 on infection or effects on reproduction have been performed on insects or ticks. Overall, little is known about the molecular basis of virulence in B. bassiana towards ticks. Here, we examined the consequences of loss of Msn2 on B. bassiana infection towards ticks combining genetic characterization with enzymatic and histological approaches. Our data indicate BbMsn2 is critical for penetration but not subsequent growth and proliferation once the tick cuticle has been breached. Furthermore, important effects were seen with respect to lowered female fertility, indicating potential added benefits in the application of B. bassiana for tick control.
Material and Methods
Beauveria bassiana Strains, Conidial Suspensions, and Viability
The strains of B. bassiana knockout ΔBbmsn2 and complemented (ΔBbmsn2/Bbmsn2) were constructed and initially characterized by Luo et al. (2015). ΔBbmsn2 and the complemented strain were obtained from B. bassiana Bb0062. Beauveria bassiana strains were cultivated on potato dextrose agar (PDA, Difco Laboratories, Sparks, MD, USA) supplemented with 1 g L−1 yeast extract (Bacto™ Yeast Extract, Sparks, MD, USA) (PDAY) in Petri plates (90 × 15 mm) and incubated in the dark for 15 days at 26 ± 1°C and relative humidity (RH) ≥90%. Temperature and relative humidity in the incubator were monitored with a data logger HOBO H8® (Onset Computer Corporation, Bourne, MA, USA). Fresh conidia from each strain were harvested using a spatula, suspended in 0.01% (v/v) Tween 80® (Labsynth Prod. Lab. Ltda, Diadema, SP, Brazil) and filtered through cheesecloth to remove mycelia. Conidial suspensions were quantified in hemocytometer at 400× magnification in a Leica DM750 light microscope (Leica Microsystems, Wetzlar, Germany), and the concentration was adjusted to 2.0 × 108 conidia ml−1 or as indicated. To assess conidial viability, 20 µl of each conidial suspension were inoculated onto 8 ml of PDAY medium supplemented with 0.002% (w/v) Benomyl (50% active ingredient; Benlate®, DuPont, São Paulo, SP, Brazil) (Braga et al., 2001) and 0.05% (w/v) chloramphenicol (Sigma-Aldrich, Steinheim, Germany) in Petri plates (35 × 9 ×10 mm). The plates were incubated at 26 ± 1°C for 48 h. After incubation, a drop of lactophenol and cotton blue solution and coverslip were applied over the inoculum; a minimum of 300 conidia per plate was evaluated, and the percent relative germination was determined (Braga et al., 2001). Conidia were considered germinated when the germ tube was longer than the maximum conidial diameter. Conidia were used only if viability was assessed to be >98% in all experiments.
Fungal Bioassays Using Rhipicephalus microplus and Measurement of Tick Reproductive Parameters
Rhipicephalus microplus engorged females were collected from artificially infested cattle at Universidade Federal de Goiás (UFG, Goiânia, Brazil). In the laboratory, ticks were washed in tap water, immersed in 1% (v/v) hypochlorite for 1 min, rinsed in sterile distilled water for 1 min and dried with sterile paper towels. The females were homogeneously distributed by weight (160-315 mg) into four treatment groups: control, BbWT, ΔBbmsn2/Bbmsn2 and ΔBbmsn2; each group had 10 individuals.
Two infection protocols were evaluated: (i) topical and (ii) intra-hemocoel injection. For topical assays, ticks were individually immersed in 1 ml of Tween 80® 0.01% (control) or in the conidial suspensions at 2.0 × 108 conidia ml−1 for 3 min. In addition, ticks were individually inoculated by intra-hemocoel injection with 5 µl of Tween 80® 0.01% (control), or 5 µl of conidial suspension at 1.6 × 105 conidia ml−1 (800 conidia/tick), 1.6 × 106 conidia ml−1 (8,000 conidia/tick), 1.6 × 107 conidia ml−1 (80,000 conidia/tick) or 2.0 × 108 conidia ml−1 (106 conidia/tick); injections were performed in the foramen located between the capitulum and the dorsal scutum of engorged females using a stereomicroscope and a 0.3 mm insulin syringe (Angelo et al., 2010). After treatment, ticks were individually placed in each well of 24-well cell culture plates (Corning Brasil Indústria e Comércio Ltda., Suzano, SP, Brazil), incubated at 26 ± 1°C, RH ≥90% and 12 h photophase. The egg mass from each female was collected at the end of oviposition, weighed, transferred to an individual glass tube (16 × 125 mm), closed with a cotton plug, and incubated at 26 ± 1°C and RH ≥90% for assessment of larval hatching. The eggs were observed daily and the larval hatching for each tube was visually estimated through microscopic examination, and values were assigned in percentages ranging from 0 to 100% by intervals of 5% in relation to the total mass of eggs (Drummond et al., 1971; Barreto et al., 2016; Bernardo et al., 2018).
The following reproductive parameters were investigated: estimated reproduction (ER) and percent control (PC) (Drummond et al., 1971). The effectiveness of treatment was measured by the effect on the ER of the engorged females; in this equation, 20,000 is the estimate of the number of larvae that normally hatch from 1 g of eggs of R. microplus; therefore, the percent control of ER estimates the treatment efficacy to decrease the tick population in an infested environment. Bioassays were repeated three times on different days, and with new batches of conidia. The ER and PC were calculated by the Equations (1) and (2), respectively.
Equation (1):
Equation (2):
Scanning Electron and Light Microscopy of Tick’s Cuticle
Initial steps of the B. bassiana infection process were examined by scanning electron microscopy (SEM) and light microscopy. Six R. microplus engorged females were individually treated with BbWT, ΔBbmsn2/Bbmsn2 or ΔBbmsn2 by topical application of 2.5 µl conidial suspension at 2.0 × 108 conidia ml−1. The treated females were incubated at 26 ± 1°C, with RH ≥90% and 12 h photophase, for 48 or 120 h. Then, approximately 50 µl of fixative [2% (v/v) glutaraldehyde (Impex, Labimpex Ind. Com. de Prods. Lab. Ltda., Diadema, SP, Brazil), 2% (v/v) paraformaldehyde (Vetec Química Fina Ltda, Duque de Caxias, RJ, Brazil), 3% (w/v) sucrose (Sigma-Aldrich, Steinheim, Germany) in 0.1 M sodium cacodylate buffer (Sigma-Aldrich, Steinheim, Germany), pH 7.2] was injected into each female using an insulin syringe according to Barreto et al. (2016). Each female was placed in a 15-ml centrifuge tube containing 2 ml of fixative and maintained for 10 days at 4°C in a refrigerator.
Ticks incubated for 48 h were examined by SEM (n = 3). The dorsal cuticle of females was dissected and removed, and then washed three times (15 min each time) in sodium cacodylate buffer (0.1 M, pH 7.2). Cuticles were dehydrated in a graded series of ethanol solutions (30, 50, 70, 80 and 90%), held for 15 min in each solution and passed twice in 100% ethanol for 15 min. Subsequently, the cuticles were individually placed in micro-centrifuge tubes containing 300 µl of hexamethyldisilazane (Electron Microscopy Sciences, Hatfield, PA, USA) and maintained immersed for 5 min (Barreto et al., 2016). After drying, the samples proceeded to metallization. Accordingly, the samples were placed on a stub and coated with gold in a sputter-applicator (Denton Vacuum Desk V). The cuticles were analyzed and electro micrographs were obtained with a scanning electron microscope (Jeol JSM 6610) at an accelerating voltage of 20 kV. The images were analyzed qualitatively (conidial adhesion, size of germinative tubes and presence of appressoria) and quantitatively (number of germinated conidia).
Ticks incubated for 120 h were prepared for histological analyses of their cuticle. After 10 d in fixative, treated ticks (n = 3) were longitudinally cut, dehydrated as described with SEM samples and embedded in resin (Historesin®, Leica Biosystems, Wetzlar, Germany) according to the manufacturer’s instructions. Sections of 4 µm were made in Microtome (Leica Biosystems, Wetzlar, Germany), stained with Periodic Acid-Schiff (PAS) and Green light (Arruda et al., 2005) and assessed by using a light microscope (Nikon E200). Images (n = 5) were captured with a high-definition microscope camera Leica ICC50 HD, with resolution of 1,280 × 720 p (HD ready). The experiments were conducted three times on different days with three replicates in each treatment group.
Protease Assay
Cuticles of R. microplus engorged females were dissected (n = 10), washed in distilled water and immersed in 10 ml conidial suspension of the strain BbWT, ΔBbmsn2/Bbmsn2 or ΔBbmsn2, at 2.0 × 108 conidia ml−1 for 3 min. Cuticles were incubated at 26°C and RH ≥90% for 120 h. After incubation, the pool of cuticles was macerated in 1 ml distilled water and centrifuged at 25,000 RCF for 5 min at 4°C. Protease activity was measured by azocasein hydrolysis as described by Segers et al. (1994) and Phillips et al. (1984) with the following modifications: commercial azocasein (Sigma Chemical Co., St. Louis, MO, USA) was dissolved at 1% (w/v) in 0.1 M Tris–HCl buffer, pH 8.5. Briefly, 1,000 µl of each supernatant sample were incubated with 500 µl de azocasein 1% at 28°C for 60 min. This reaction was stopped by adding 1 ml of 10% TCA and maintaining it for 15 min at 4°C, and followed by centrifugation at 25,000 RCF for 10 min to remove the precipitated protein. Six hundred milliliters of the supernatant were neutralized by adding 700 µl of 1M NaOH, and absorbance at 450 nm was recorded with Enzyme-Linked Immunosorbent Assay (ELISA). One unit of enzyme activity (U) was calculated by the Equation (3):
Equation (3):
Statistical Analyses
All data sets were previously checked for normality and homoscedasticity with Shapiro–Wilk and Bartlett tests, respectively. Normally distributed data (engorged female initial weight, egg mass weight, and conidial germination) were fitted to a parametric model and then were submitted to ANOVA followed by an SNK test for multiple comparisons. Non-normally distributed data (percentage of larval hatch) were fitted to a non-parametric model and then analyzed by a Kruskal–Wallis test, followed by an FDR test. Protease activity of mutant strains was compared with the BbWT strain by applying the Dunnett’s test. Analyses were performed in the statistical environment R (R Team C, 2018). P-values less than 0.05 were considered as significant.
Results
Measurement of Reproductive Parameters and Virulence Assays
Reproductive parameters of R. microplus engorged females treated topically with different strains (BbWT, ΔBbmsn2, and ΔBbmsn2/Bbmsn2) of B. bassiana were examined 15 d post-infection after immersion in 2.0 × 108 conidia ml−1 as detailed in the Material and Methods section (Table 1). Because of the difficulty in determining the exact timing of tick death, lethal mortality times could not be accurately determined; however, reproductive parameters that would critically inform on successful biological control efforts were measured. The weight of egg mass from females treated with any of the B. bassiana conidial suspensions tested (i.e., either wild-type, mutant, or complemented mutant) was similar and lower (~50%) than that of control untreated ticks (F3,118 = 18.33; P <0.001). High mean larval hatching (~90%) was observed in the control group, which was slightly reduced after treatment with the ΔBbmsn2 strain (down to ~84%, P = 0.006). However, engorged females treated with BbWT or ΔBbmsn2/Bbmsn2 had significantly decreased larval hatching (10–20%, χ2 = 17.38; df = 3; P = 0.006), indicating lower egg viability using the wild-type and complemented B. bassiana strains. Overall, the percent control of ticks was higher in the groups treated with BbWT (56.1%) or ΔBbmsn2/Bbmsn2 (58.7%) as compared to untreated engorged females or ticks treated with the ΔBbmsn2 mutant strain (39.7%). Despite being unable to determine exact time of death, all ticks showed eventual mycosis and sporulation of the fungus on the tick cadavers.

Table 1 Biological parameters of Rhipicephalus microplus engorged females treated by immersion in Tween 80® 0.01% (control) or in conidial suspension (2.0 × 108 conidia ml−1) of Beauveria bassiana strains (BbWT, ΔBbmsn2/Bbmsn2 or ΔBbmsn2) incubated at 26 ± 1°C and RH ≥ 90%.
For intra-hemocoel injection assays (that would bypass the need to cuticle penetrations), a concentration range of fungal conidia (800, 80,000, 80,000, and 106 conidia/tick) was tested (Table 2). Engorged females of R. microplus treated by intra-hemocoel injection of 800 conidia/tick showed decreased (50–60%) mean egg mass weights for all strains tested (F3,55 = 24.05; P <0.001) as compared to control uninfected ticks, with only slight differences seen between the wild-type, ΔBbmsn2, and ΔBbmsn2/Bbmsn2 strains (Table 2). In addition, a decreased mean oviposition period from 7 to 2 d was seen after injection of 800 conidia/tick of any of the B. bassiana strains tested (F3,55 = 27.66; P <0.001) in comparison to control untreated ticks. Ticks treated by intra-hemocoel injection at concentrations ≥8,000 conidia/tick died before laying their eggs (i.e., within 48 h after treatment), while in control group the oviposition period was 7.9 ± 1 d (Table 2), and thus had essentially no oviposition period. No differences in sporulation were observed on the cadaver of ticks between the wild-type, ΔBbmsn2, and ΔBbmsn2/Bbmsn2 strains at 5 d after infection (Figure 1).
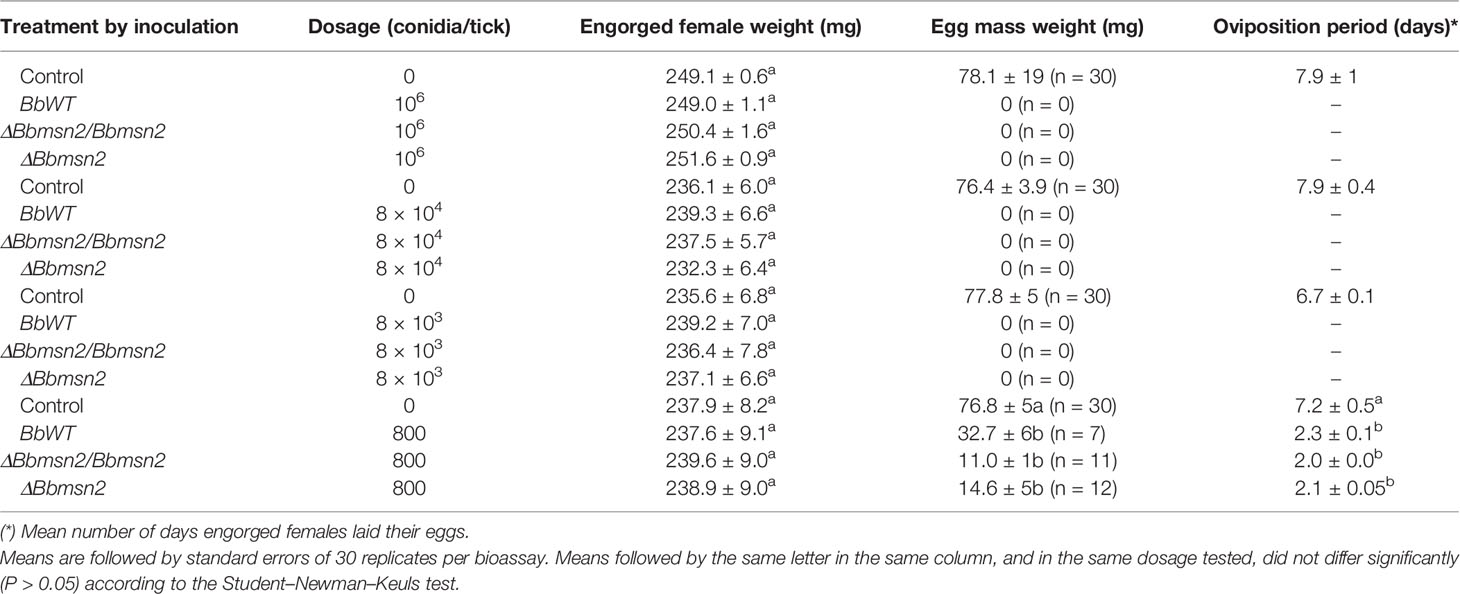
Table 2 Biological parameters of Rhipicephalus microplus engorged females treated by an intra-hemocoel injection with 5 µl of Tween 80® 0.01% (control), or 5 µl of conidial suspension of Beauveria bassiana strains (BbWT, ΔBbmsn2/Bbmsn2 and ΔBbmsn2) at 1.6 × 105 conidia ml−1 (800 conidia/tick), 1.6 × 106 conidia ml−1 (8,000 conidia/tick), 1.6 × 107 conidia ml−1 (80,000 conidia/tick) or 2.0 × 108 conidia ml−1 (106 conidia/tick), and incubated at 26 ± 1°C and RH ≥ 90%.
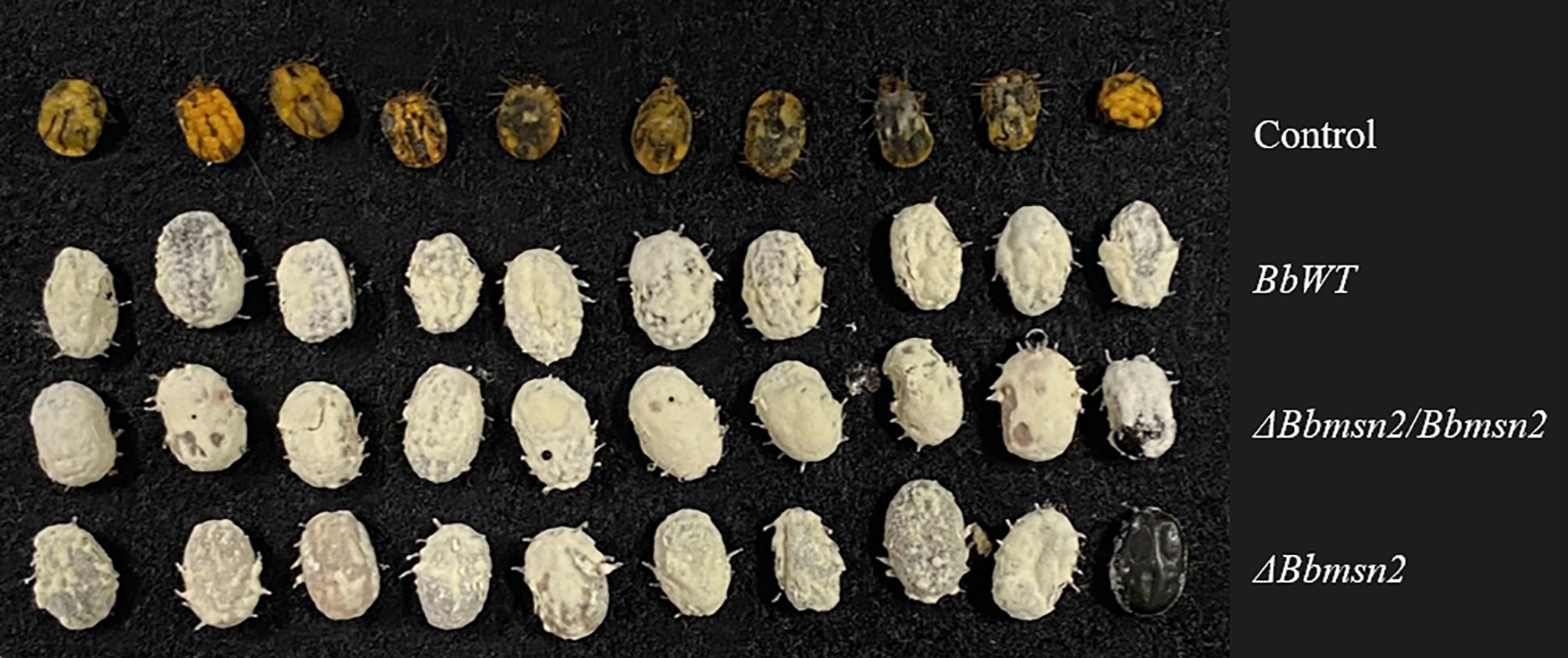
Figure 1 Fungal sporulation on Rhipicephalus microplus engorged females’ cadavers at day 5 post treatment by an intra-hemocoel injection with 5-μl conidial suspension at 2.0 × 108 conidia ml–1.
Infection of B. bassiana Strains on Tick Cuticle
The absence of the Msn2 transcription factor did not interfere with conidial germination or adhesion to the tick cuticle. Electron micrographs showed similar (F2,6 = 0.0638; P = 0.9388) conidial germination between the B. bassiana strains on the tick cuticle at 48 h incubation: BbWT = 84.33 ± 13.2%, ΔBbmsn2/Bbmsn2 = 82.33 ± 11.20% and ΔBbmsn2 = 76.33 ± 22.67% (Figure 2). Most conidia from BbWT (Figure 3A), ΔBbmsn2/Bbmsn2 (Figures 3B, C) and ΔBbmsn2 (Figure 3D) appeared to have germinated, and appressoria-like tip structures could be seen.
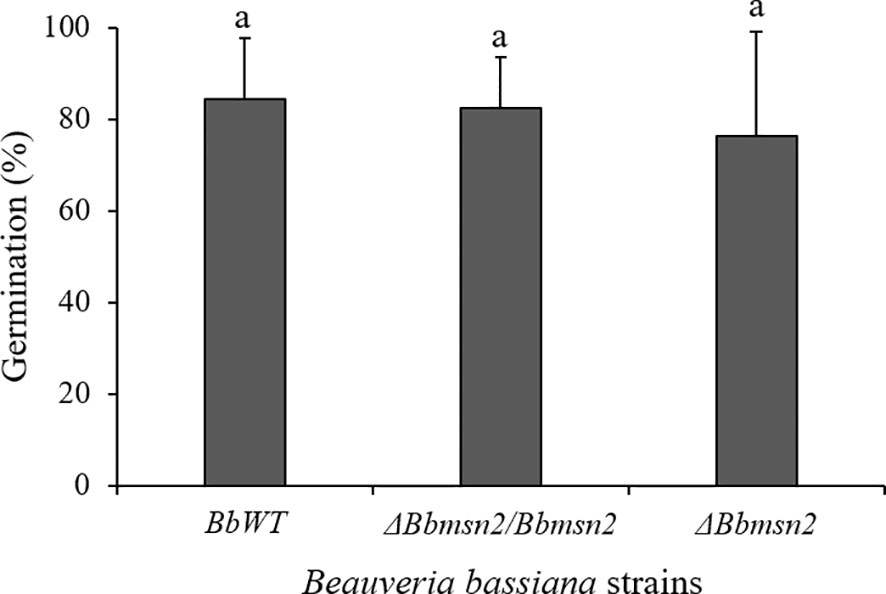
Figure 2 Germination (%) of conidia of Beauveria bassiana strains: BbWT, ΔBbmsn2/Bbmsn2 and ΔBbmsn2 on the cuticle of Rhipicephalus microplus engorged females incubated at 26 ± 1°C and RH ≥90% for 48 h. Bars with the same letter do not differ significantly (P ≥ 0.05) among treatments. Standard errors are based on three independent trials.
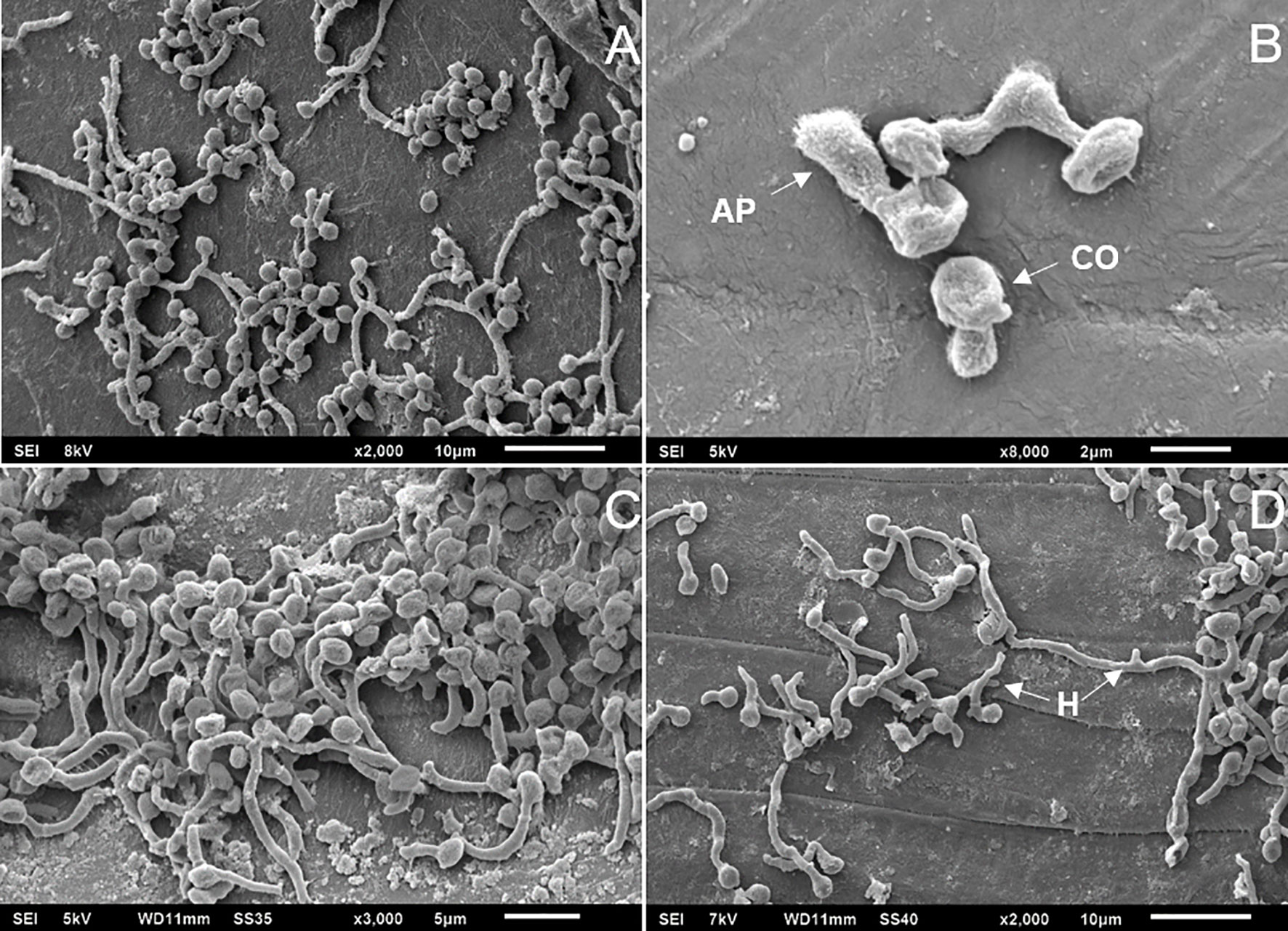
Figure 3 Electron micrographs of Rhipicephalus microplus engorged females treated topically with conidia of Beauveria bassiana strains: BbWT, ΔBbmsn2/Bbmsn2 or ΔBbmsn2, and incubated for 48 h at 26 ± 1°C and RH ≥ 90%. Germinating conidia of BbWT on the tick cuticle (A) Germinating conidia of ΔBbmsn2/Bbmsn2 with developed appressorium on the tick cuticle (B) Germinating conidia of ΔBbmsn2/Bbmsn2 on the tick cuticle (C) Germinated conidia of ΔBbmsn2 [some with long germ tubes (hyphae)] on the tick cuticle (D). CO, conidia; AP, appressorium; H, hyphae.
Conidia from BbWT and ΔBbmsn2/Bbmsn2 were capable of germinating and penetrating through the cuticle (Figures 4A, C, respectively). Hyphae of BbWT (Figures 4A, B) and ΔBbmsn2/Bbmsn2 were also observed inside the tick body, with fungal development in adjacent tissues (Figures 4C, D). However, the hyphae of the ΔBbmsn2 strain appeared to continue to grow on the surface/exocuticle of the tick with fewer instances of penetrative hypha seen at 120 h incubation as compared to the wild-type and complemented strains (Figure 4E).
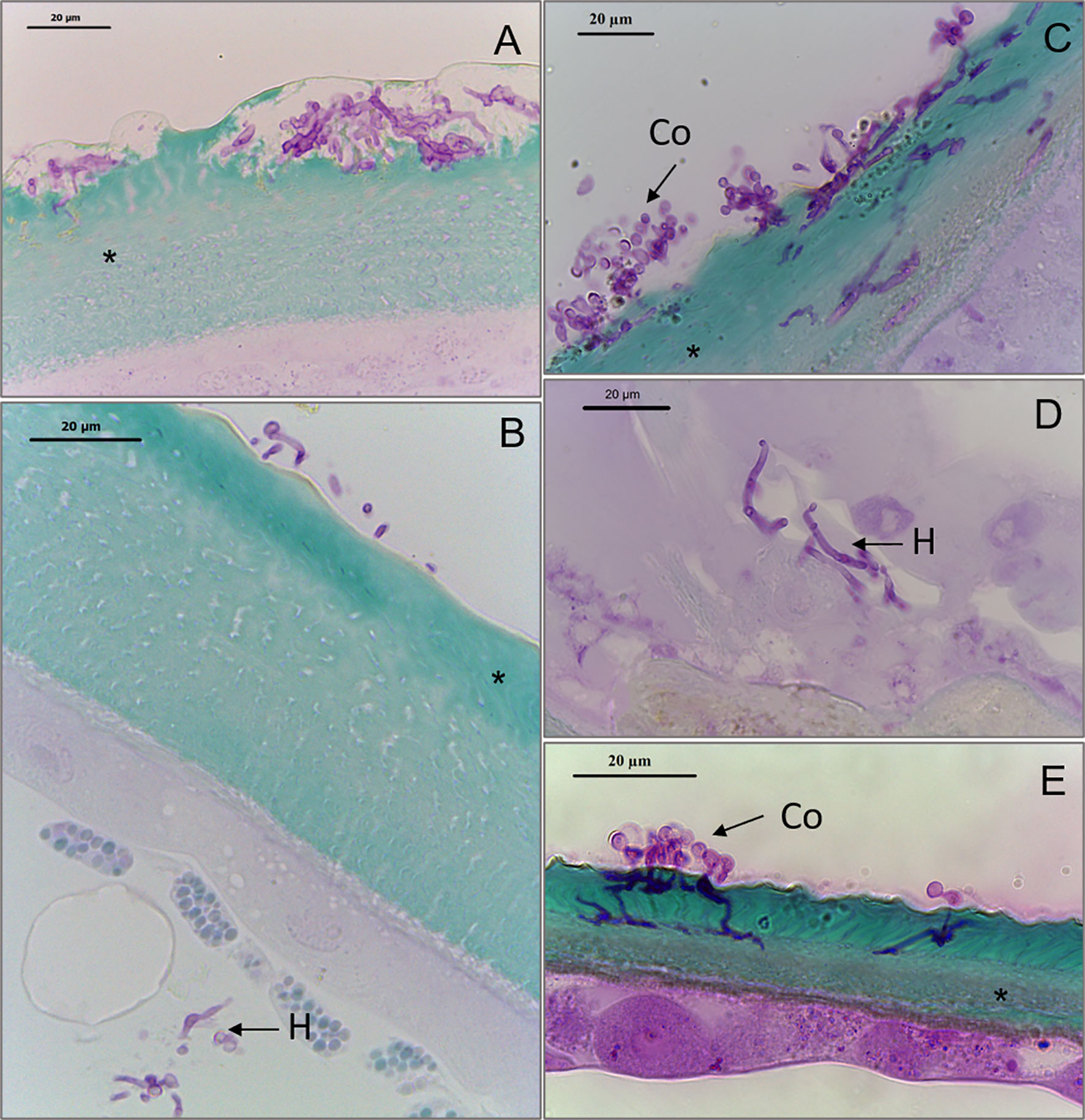
Figure 4 Sagittal sections of Rhipicephalus microplus engorged females treated with conidia of Beauveria bassiana strains: BbWT, ΔBbmsn2/Bbmsn2 or ΔBbmsn2, and incubated for 120 h at 26 ± 1°C and RH ≥90%. Germinating conidia of BbWT penetrates through the tick cuticle (A) Germinating conidia of BbWT attaches to the tick cuticle and fungal hyphae infecting the tick interior (B) Germinating conidia of ΔBbmsn2/Bbmsn2 penetrates through all the layers of the tick cuticle (C) Hyphae of ΔBbmsn2/Bbmsn2 infects the interior tick tissues (D) Incomplete penetration of ΔBbmsn2 hyphae through the tick cuticle (E). Co, germinated conidia; H, hyphae inside tick tissue; asterisk (*) cuticle.
Protease Activity
The proteolytic activity of the B. bassiana strains on R. microplus cuticle was measured using azoalbumin as detailed in the Material and Methods section. Protease activity was significantly lower in the ΔBbmsn2 strain (1.91 ± 0.29 U ml−1) than in the BbWT (9.88 ± 4.08 U ml−1; P = 0.0113) and ΔBbmsn2/Bbmsn2 strains (8.16 ± 1.59 U ml−1; P = 0.0150) (Figure 5).
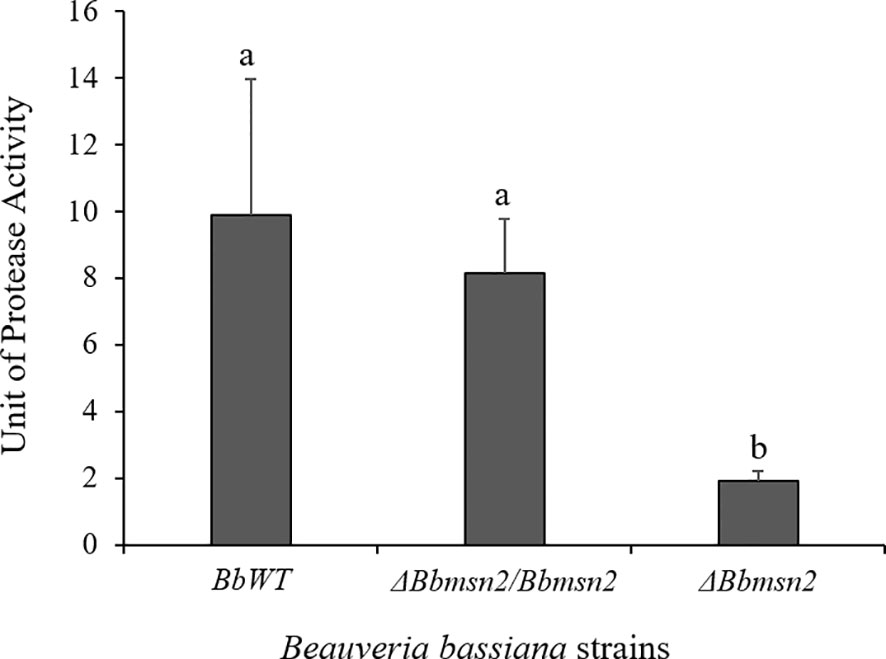
Figure 5 Protease activity on the cuticle of Rhipicephalus microplus treated by an immersion in a conidial suspension of Beauveria bassiana strains: BbWT, ΔBbmsn2/Bbmsn2 or ΔBbmsn2, and incubated for 120 h at 26 ± 1°C and RH ≥90%. Bars with different letters, i.e. “a” and “b”, indicate significant difference (P < 0.05). Standard errors are based on three independent trials.
Discussion
Our data indicate that the Msn2 transcription factor significantly contributes to the ability of B. bassiana to infect R. microplus via the “natural” cuticle-penetration requiring route. Engorged females treated topically with the ΔBbmsn2 strain showed a significantly decreased percent control of ticks in comparison to the wild-type and complemented strains. However, the mortality of ticks reached 100% when engorged females were injected, thus by-passing the requirement for cuticle penetration, with ΔBbmsn2 (≥8,000 conidia/tick) conidial suspension by intra-hemocoel injection. Even at the lowest dose injected (800 conidia/tick), reduced reproductive parameters of engorged females was seen for all of the B. bassiana strains tested. In a previous study, S. litura and T. molitor larvae treated with ΔBbmsn2 conidia had, respectively, LT50 values 28 and 25% higher (decreased virulence) than the complemented strain (Liu et al., 2013). In addition, G. mellonella larvae treated topically with ΔBbmsn2 conidia also had an increased LT50 detected; however, no difference in LT50 values among the mutant and wild-type strains was observed when G. mellonella larvae were treated by injecting conidial suspension into the hemocoel (Luo et al., 2015). These results and our data lead us to conclude that the transcription factor Msn2 acts predominantly during the penetration of B. bassiana through the tick cuticle, with retention of the ability of the fungus to evade immune systems once inside the host.
The first step to successful infection of entomopathogenic fungi is the adhesion of fungal propagules (conidia or blastospores) on the host cuticle. Accordingly, high fungal virulence is directly related to increased adhesion to the host cuticle (Butt and Goettel, 2000). Adhesion is considered to follow a two-step process involving initial attachment followed by consolidation of adhesion, influenced by the surface characteristics of the fungal cells and the target substrata (Holder et al., 2007). The B. bassiana conidial surface is composed of a layer of hydrophobic rod-like proteins (hydrophobins, hyd1 and hyd2), which mediate, in part, attachment to the host cuticle that is also hydrophobic (Holder and Keyhani, 2005; Ortiz-Urquiza and Keyhani, 2016). The deletion of hyd1 gene in B. bassiana decreased the conidia hydrophobicity and virulence, although it did not interfere in its adhesion capacity. The deletion of the hyd2 gene caused changes in the structure of the conidia cell wall, and consequently diminished its adhesion capacity and hydrophobicity but without altering its virulence (Zhang et al., 2011). Liu et al. (2013) have shown that the hydrophobin genes (hyd1 and hyd2) were repressed by 66–68% in a ΔBbmsn2 mutant strain, but it did not affect the mad1 and mad2 expressions, additional factors important for facilitating conidial adhesion to arthropods and plants, respectively (Broetto et al., 2010). Microscopic analyses were used in order to probe effects of BbMsn2 on adhesion to tick surfaces, and these data showed that conidia of ΔBbmsn2 were equivalent to the wild-type (BbWT) and complemented strains (ΔBbmsn2/Bbmsn2) in size and ability to adhere to the tick cuticle. These data indicate that the impaired virulence seen in topical assays is not likely due to impaired adhesion to the host surface.
The tick cuticle is divided in several layers; from the outside to inside: epicuticle, exocuticle, endocuticle and epidermis (Hackman, 1982). The epicuticle layer is composed of lipids, long chain alkenes, esters and fatty acids (Ali et al., 2009). In particular, R. microplus females, during the engorgement process, have altered epicuticle composition that includes an almost 20% increase in surface lipids; with the most commonly found including alcohol and fatty acids combined by esterification (Hackman, 1982). To penetrate the host cuticle, entomopathogenic fungi use mechanical pressure and secrete proteases, chitinases, and lipases, which degrade their main constituents (proteins, chitin, and lipids) to allow the hyphae to penetrate through the exoskeleton. B. bassiana secretes lipases which potentiate the degradation of the arthropod wax layer (Sánchez-Pérez et al., 2014). However, Feng (1998) indicated that lipase activity of several B. bassiana s.l. isolates had little correlation with their virulence. Luo et al. (2015) demonstrated that proteinase and lipase activities were similar in ΔBbmsn2 colonies grown on skim milk agar plates and in the wild-type. However, in the present study, our data show that the protease activity of B. bassiana ΔBbmsn2 on R. microplus cuticle decreased in the Msn2 mutant. It is known that the production of (total) protease activity can vary significantly according to the composition of host cuticle (Cito et al., 2016), which may induce or inhibit fungal development (Ment et al., 2012). Additionally, Santi et al. (2019) identified 50 proteins involved in the infection process, which were produced by B. bassiana cultured in media supplemented with cuticles derived from R. microplus, but not produced in media supplemented with glucose only. Our results showed a decreased penetration of ΔBbmsn2 hyphae through the cuticle in comparison to the wild-type and complemented strains at 120 h post-infection. This inability to trespass the tick cuticle may be due to the reduced capacity to degrade chitin, proteins, and/or lipids that constitute the cuticle composition of R. microplus (Hackman, 1982). In addition, impairments related to the fungal response to the osmotic, oxidative and/or nutrient stress that occurs on the cuticle, and are mediated by Msn2, may also contribute to the decreased virulence (Luo et al., 2015).
The production of oxalic acid is also an important virulence factor in B. bassiana against ticks. Direct treatment of ticks with oxalate at pH 4.0 resulted in almost 80% mortality in adults of the tick Amblyomma americanum within 14 days after treatment (Kirkland et al., 2005). Oxalate production reduces extracellular pH and, consequently, acts to facilitate degradation of components of the host cuticle, e.g., chitin, elastin and collagen (Bidochka and Khachatourians, 1991). M. anisopliae mutants unable to acidify the culture medium (i.e., that produced less metabolic acids) also show a decreased protease activity (St Leger et al., 1999). In B. bassiana, Luo et al. (2015) demonstrated that the ΔBbmsn2 strain had little to no radial growth at pH 4.1 or 4.7 (although conidiation still occurred), and its growth was reduced in comparison to the wild-type strain. In addition, oosporein production is impaired in the Msn2 mutant (Luo et al., 2015), however its contribution to infection may be limited (Fan et al., 2017). A critical difference with respect to Msn2 function between insects and ticks may be that in insects Msn2 appears to contribute to both topical and intra-hemocoel infection (Luo et al., 2015), whereas our data indicate that for ticks, Msn2 is essentially only required for full virulence via the topical route of infection. While topical infection is the “natural” route of infection, these data imply important downstream immune system differences in dealing with invading (fungi) microbes between ticks and insects.
Effects on reproduction can be critical to the biological control potential of insect pathogenic fungi. Reduced fecundity and lack of resistance development have been reported in using Cordyceps fumosorosea (formerly, Isaria fumosorosea) against Bemisia tabaci (whitefly) (Gao et al., 2017), as well as in B. bassiana (Bernardo et al., 2018) or M. anisopliae (Bittencourt et al., 1994; Muniz et al., 2020) infecting R. microplus engorged females. After penetrating the cuticle of R. microplus, M. anisopliae reaches the hemocoel and can be found colonizing the hemolymph (Bittencourt et al., 1995) and internal organs, including the ovary tissues (Paulo et al., 2018) thus contributing to the reduced fecundity of engorged females seen after infection. B. bassiana infection towards Argas persicus (Acari: Argasidae) also directly affects the female reproductive system and causes damages to the ovaries, inhibiting vitellogenesis (Marzouk et al., 2020). Our data show that B. bassiana infection reduced fecundity of R. microplus engorged females by reducing ovipositing and larval hatching, with loss of Msn2 impairing these effects. For successful control, even if entomopathogenic fungi may not (quickly) kill engorged females, any reduction in fecundity can be crucial for controlling R. microplus infestations, because fully engorged females naturally drop off the host and lay thousands of eggs on the ground before dying and completing their life cycle.
In recent years, studies on the identification of genes and their functions in B. bassiana have revealed a wide range of mechanisms involved in the infection process (Butt et al., 2016); however, most of these studies have focused on insect larvae that are naturally more susceptible to infection by these fungi (Ortiz-Urquiza and Keyhani, 2016), and the extent to which these results can be extrapolated to other targets within Arthropoda remains to be determined. Our data indicate some shared contributions as well as potential differences. In addition, our results indicate that conidial germination, appressorial differentiation, and even fungal colonization on the host surface may be poor indicators of successful tick control (mortality), which requires host penetration (Ment et al., 2012).
Conclusions
Our results indicate that the absence of Msn2 transcription factor reduced the virulence of B. bassiana s.l. against R. microplus demonstrated by the delayed fungal penetration and decreased protease production on the tick cuticle. Results on tick reproduction revealed potential effects beyond direct virulence that can impact biological control efforts.
Data Availability Statement
The raw data supporting the conclusions of this article will be made available by the authors, without undue reservation.
Ethics Statement
The animal study was reviewed and approved by Ethics Commission on Animal Use of Universidade Federal de Goiás (CEUA, protocol #057/16). The access to Brazilian genetic heritage was approved by the Genetic Heritage Management Council (CGen) of Brazil (protocol #A420934).
Author Contributions
EM, NK and ÉF designed the experiments and wrote the manuscript. EM and CR-S performed the bioassays, histology of ticks and protease activity assays. EM and WA performed scanning electron microscopy and histology of ticks. NK inspired co-authors to investigate this subject and produced the mutant strains of B. bassiana. All authors contributed to the article and approved the submitted version.
Funding
This study was supported by the Coordenação de Aperfeiçoamento de Pessoal de Nível Superior (CAPES) of Brazil for providing PhD scholarship for EM and CR-S. This research was supported by grants from the Conselho Nacional de Desenvolvimento Científico e Tecnológico (CNPq) of Brazil (431928/2016-9 to ÉF). CNPq also provided the grant 306319/2018-7 for ÉF. This research was also supported in part by US-NSF grant IOS-1557704 to NK. The publication costs were covered by grant from the Fundação de Amparo à Pesquisa do Estado de Goiás (FAPEG; CC 11233).
Conflict of Interest
ÉF is Associate Editor for the section Fungi-Animal Interactions in Frontiers in Fungal Biology, and Guest Associate Editor for the section Invertebrate Physiology, in the research topic: Entomopathogenic Fungi for the Control of Arthropods - Frontiers in Physiology.
The remaining authors declare that the research was conducted in the absence of any commercial or financial relationships that could be construed as a potential conflict of interest.
Acknowledgments
We thank Dr. Filippe Elias de Freitas Soares (Visiting professor at UFG, and current professor at Universidade Federal de Lavras), and MSc. Juliana Marques Ferreira (PhD student at UFG) for teaching EM the protocol used for the protease assays.
References
Ali, S., Huang, Z., Ren, S. (2009). Production and Extraction of Extracellular Lipase From the Entomopathogenic Fungus Isaria Fumosoroseus (Cordycipitaceae; Hypocreales). Biocontrol Sci. Technol. 19 (1), 81–89. doi: 10.1080/09583150802588524
Angelo, I. C., Gôlo, P. S., Camargo, M. G., Kluck, G. E. G., Folly, E., Bittencourt, V. R. E. P. (2010). Haemolymph Protein and Lipid Profile of Rhipicephalus (Boophilus) microplus Infected by Fungi. Transbound Emerg. Dis. 57 (1-2), 79–83. doi: 10.1111/j.1865-1682.2010.01119.x
Arruda, W., Lubeck, I., Schrank, A., Vainstein, M. H. (2005). Morphological Alterations of Metarhizium anisopliae During Penetration of Boophilus microplus Ticks. Exp. Appl. Acarol. 37 (3-4), 231–244. doi: 10.1007/s10493-005-3818-6
Barreto, L. P., Luz, C., Mascarin, G. M., Roberts, D. W., Arruda, W., Fernandes, É. K. K. (2016). Effect of Heat Stress and Oil Formulation on Conidial Germination of Metarhizium anisopliae s.s. on Tick Cuticle and Artificial Medium. J. Invertebr. Pathol. 138, 94–103. doi: 10.1016/j.jip.2016.06.007
Bernardo, C. C., Barreto, L. P., Ribeiro-Silva, C. S., Luz, C., Arruda, W., Fernandes, É. K. K. (2018). Conidia and Blastospores of Metarhizium spp. And Beauveria bassiana s.l.: Their Development During the Infection Process and Virulence Against the Tick Rhipicephalus microplus. Ticks Tick-Borne Dis. 9 (5), 1334–1342. doi: 10.1016/j.ttbdis.2018.06.001
Bidochka, M. J., Khachatourians, G. G. (1991). The Implication of Metabolic Acids Produced by Beauveria bassiana in Pathogenesis of the Migratory Grasshopper, Melanoplus sanguinipes. J. Invertebr. Pathol. 58, 106–117. doi: 10.1016/0022-2011(91)90168-P
Bittencourt, V. R. E. P., Massard, C. L., Lima, A. F. (1994). Ação do Fungo Metarhizium anisopliae Sobre a Fase Não Parasitária do Ciclo Biológico de Boophilus microplus. Rev. Univ. Rur. Sér. Ciênc. Vida 16:1-2, 49–55.
Bittencourt, V. R. E. P., Massard, C. L., Lima, A. F. (1995). Dinâmica Da Infecção do Fungo Metarhizium anisopliae (Metschnikof) Sorokin Sobre O Carrapato Boophilus microplus (Canestrini). Rev. Univer. Rur. Sér. Ciênc. Vida. 17, 83–88.
Braga, G. U. L., Flint, S. D., Miller, C. D., Anderson, A. J., Roberts, D. W. (2001). Variability in Response to UV-B Among Species and Strains of Metarhizium Isolated From Sites at Latitudes From 61°N to 54°S. J. Invertebr. Pathol. 78 (2), 98–108. doi: 10.1006/jipa.2001.5048
Broetto, L., Da Silva, W. O. B., Bailão, A. M., Soares, C. A., Vainstein, M. V., Schrank, A. (2010). Glyceraldehyde-3-Phosphate Dehydrogenase of the Entomopathogenic Fungus Cell-Surface Localization and Role in Host Adhesion. FEMS Microbiol. Let. 312 (2), 101–109. doi: 10.1111/j.1574-6968.2010.02103.x
Butt, T. M., Coates, C. J., Dubovskiy, I. M., Ratcliffe, N. A. (2016). Entomopathogenic Fungi: New Insights Into Host–Pathogen Interaction. Adv. Genet. 94, 307–364. doi: 10.1016/bs.adgen.2016.01.006
Butt, T. M., Goettel, M. S. (2000). “Bioassays of Entomogenous Fungi,” in Bioassays of Entomopathogenic Microbes and Nematodes. Eds. Navon, A., Ascher, K. R. S. (Wallingford, UK: CAB International), 141–195.
Campos, R. A., Boldo, J. T., Pimentel, I. C., Dalfovo, V., Araújo, W. L., Azevedo, J. L., et al. (2010). Endophytic and Entomopathogenic Strains of Beauveria sp. to Control the Bovine Tick Rhipicephalus (Boophilus) microplus. Genet. Mol. Res. 9 (3), 1421–1430 doi: 10.4238/vol9-3gmr884
Cito, A., Barzanti, G. P., Strangi, A., Francardi, V., Zanfini, A., Dreassi, E. (2016). Cuticle-Degrading Proteases and Toxins as Virulence Markers of Beauveria bassiana (Balsamo) Vuillemin. J. Basic Microbiol. 56 (9), 941–948. doi: 10.1002/jobm.201600022
Drummond, R. O., Gladney, W. J., Whetstone, T. M., Ernst, S. E. (1971). Laboratory Testing of Insecticides for Control of the Winter Tick. J. Econ. Entomol. 64 (3), 686–688. doi: 10.1093/jee/64.3.686
Fan, Y., Fang, W., Guo, S., Pei, X., Zhang, Y., Xiao, Y., et al. (2007). Increased Insect Virulence in Beauveria bassiana Strains Overexpressing an Engineered Chitinase. Appl. Environ. Microbiol. 73 (1), 295–302. doi: 10.1128/AEM.01974-06
Fang, W., Leng, B., Xiao, Y., Jin, K., Ma, J., Fan, Y., et al. (2005). Cloning of Beauveria bassiana Chitinase Gene Bbchit1 and Its Application to Improve Fungal Strain Virulence. Appl. Environ. Microbiol. 71 (1), 363–370. doi: 10.1128/AEM.71.1.363-370.2005
Fan, Y., Liu, X., Keyhani, N. O., Tang, G., Pei, Y., Zhang, W., et al. (2017). Regulatory Cascade and Biological Activity of Beauveria bassiana Oosporein That Limits Bacterial Growth After Host Death. Proc. Natl. Acad. Sci. 114, E1578–E1586. doi: 10.1073/pnas.1616543114
Feng, M. G. (1998). Reliability of Extracellular Protease and Lipase Activities of Beauveria bassiana Isolates Used as Their Virulence Indices. Acta Microbiol. Sin. 38 (6), 461–467.
Fernandes, É. K. K., Angelo, I. C., Rangel, D. E. N., Bahiense, T. C., Moraes, A. M. L., Roberts, D. W., et al. (2011). An Intensive Search for Promising Fungal Biological Control Agents of Ticks, Particularly Rhipicephalus microplus. Vet. Parasitol. 182 (4), 307–318. doi: 10.1016/j.vetpar.2011.05.046
Fernandes, É. K. K., Bittencourt, V. R. E. P., Roberts, D. W. (2012). Perspectives on the Potential of Entomopathogenic Fungi in Biological Control of Ticks. Exp. Parasitol. 130 (3), 300–305. doi: 10.1016/j.exppara.2011.11.004
Gao, T., Wang, Z., Huang, Y., Keyhani, N. O., Huang, Z. (2017). Lack of Resistance Development in Bemisia tabaci to Isaria fumosorosea After Multiple Generations of Selection. Sci. Rep. 7, 42727. doi: 10.1038/srep42727
Hackman, R. H. (1982). Structure and Function in Tick Cuticle. Ann. Rev. Entomol. 27, 75–95. doi: 10.1146/annurev.en.27.010182.000451
Holder, D. J., Keyhani, N. O. (2005). Adhesion of the Entomopathogenic Fungus Beauveria (Cordyceps) bassiana to Substrata. Appl. Environ. Microbiol. 71 (9), 5260–5266. doi: 10.1128/aem.71.9.5260-5266.2005
Holder, D. J., Kirkland, B. H., Lewis, M. W., Keyhani, N. O. (2007). Surface Characteristics of the Entomopathogenic Fungus Beauveria (Cordyceps) bassiana. Microbiology 153, 3448–3457. doi: 10.1099/mic.0.2007/008524-0
Jin, K., Zhang, Y., Fang, W., Luo, Z., Zhou, Y., Pei, Y. (2010). Carboxylate Transporter Gene JEN1 From the Entomopathogenic Fungus Beauveria bassiana Is Involved in Conidiation and Virulence. Appl. Environ. Microbiol. 76, 254–263. doi: 10.1128/AEM.00882-09
Joshi, L., St leger, R. J., Bidochka, M. J. (1995). Cloning of a Cuticle-Degrading Protease From the Entomopathogenic Fungus, Beauveria bassiana. FEMS Microbiol. Lett. 125 (2-3), 211–218. doi: 10.1111/j.1574-6968.1995.tb07360.x
Kirkland, B. H., Cho, E., Keyhani, N. O. (2004a). Differential Susceptibility of Amblyomma maculatum and Amblyomma americanum (Acari: Ixodidae) to the Entomopathogenic Fungi Beauveria bassiana and Metarhizium anisopliae. Biol. Control 31 (3), 414–421. doi: 10.1016/j.biocontrol.2004.07.007
Kirkland, B. H., Eisa, A., Keyhani, N. O. (2005). Oxalic Acid as a Fungal Acaracidal Virulence Factor. J. Med. Entomol. 42 (3), 346–351. doi: 10.1093/jmedent/42.3.346
Kirkland, B. H., Westwood, G. S., Keyhani, N. O. (2004b). Pathogenicity of Entomopathogenic Fungi Beauveria bassiana and Metarhizium anisopliae to Ixodidae Tick Species Dermacentor variabilis, Rhipicephalus sanguineus, and Ixodes scapularis. J. Med. Entomol. 41 (4), 705–711. doi: 10.1603/0022-2585-41.4.705
Liu, Q., Ying, S. H., Li, J. G., Tian, C. G., Feng, M. G. (2013). Insight Into the Transcriptional Regulation of Msn2 Required for Conidiation, Multi-Stress Responses and Virulence of Two Entomopathogenic Fungi. Fungal Genet. Biol. 54, 42–51. doi: 10.1016/j.fgb.2013.02.008
Luo, Z., Li, Y., Mousa, J., Bruner, S., Zhang, Y., Pei, Y., et al. (2015). Bbmsn2 Acts as a pH-Dependent Negative Regulator of Secondary Metabolite Production in the Entomopathogenic Fungus Beauveria bassiana. Environ. Microbiol. 17 (4), 1189–1202. doi: 10.1111/1462-2920.12542
Marzouk, A. S., Swelim, H. H., Ali, A. A. B. (2020). Ultrastructural Changes Induced by the Entomopathogenic Fungus Beauveria bassiana in the Ovary of the Tick Argas (Persicargas) persicus (Oken). Ticks Tick-Borne Dis. 11 (6), 101507. doi: 10.1016/j.ttbdis.2020.101507
Ment, D., Churchill, A. C. L., Gindin, G., Belausov, E., Glazer, I., Rehner, S. A., et al. (2012). Resistant Ticks Inhibit Metarhizium Infection Prior to Haemocoel Invasion by Reducing Fungal Viability on the Cuticle Surface. Environ. Microbiol. 14, 1570–1583. doi: 10.1111/j.1462-2920.2012.02747.x
Muniz, E. R., Paixão, F. R. S., Barreto, L. P., Luz, C., Arruda, W., Angelo, I. C., et al. (2020). Efficacy of Metarhizium anisopliae Conidia in Oil-In-Water Emulsion Against the Tick Rhipicephalus microplus Under Heat and Dry Conditions. BioControl 65, 339–351. doi: 10.1007/s10526-020-10002-5
Ortiz-Urquiza, A., Keyhani, N. O. (2013). Action on the Surface: Entomopathogenic Fungi Versus the Insect Cuticle. Insects 4 (3), 357–374. doi: 10.3390/insects4030357
Ortiz-Urquiza, A., Keyhani, N. O. (2015). Stress Response Signaling and Virulence: Insights From Entomopathogenic Fungi. Curr. Genet. 61 (3), 239–249. doi: 10.1007/s00294-014-0439-9
Ortiz-Urquiza, A., Keyhani, N. O. (2016). Molecular Genetics of Beauveria bassiana Infection of Insects. Adv. Genet. 94, 165–249. doi: 10.1016/bs.adgen.2015.11.003
Paulo, J. F., Camargo, M. G., Coutinho-Rodrigues, C. J. B., Marciano, A. F., Freitas, M. C., da Silva, E. M., et al. (2018). Rhipicephalus microplus Infected by Metarhizium: Unveiling Hemocyte Quantification, GFP-Fungi Virulence, and Ovary Infection. Parasitol. Res. 117 (6), 1847–1856. doi: 10.1007/s00436-018-5874-y
Phillips, P. K., Prior, D., Dawes, B. (1984). A Modified Azoalbumin Technique for the Assay of Proteolytic Enzymes for Use in Blood Group Serology. J. Clin. Pathol. 37 (3), 329–331. doi: 10.1136/jcp.37.3.329
Posadas, J. B., Lecuona, R. E. (2009). Selection of Native Isolates of Beauveria bassiana (Ascomycetes: Clavicipitaceae) for the Microbial Control of Rhipicephalus (Boophilus) microplus (Acari: Ixodidae). J. Med. Entomol. 46 (2), 284–291. doi: 10.1603/033.046.0213
R Team C (2018). R: A Language and Environment for Statistical Computing (Vienna: R Foundation for Statistical Computing).
Sánchez-Pérez, L. D. C., Barranco-Florido, J. B., Rodriguez-Navarro, S., Cervantes-Mayagoitia, J. F., Ramos-Lopez, M. A. (2014). Enzymes of Entomopathogenic Fungi, Advances and Insights. Adv. Enzyme Res. 2, 65–76. doi: 10.4236/aer.2014.22007
Santi, L., Coutinho-Rodrigues, C. J. B., Berger, M., Klein, L. A. S., De Souza, E., Rosa, R. L., et al. (2019). Secretomic Analysis of Beauveria bassiana Related to Cattle Tick, Rhipicephalus microplus, Infection. Folia Microbiol. 64, 361–372. doi: 10.1007/s12223-018-0659-3
Schrank, A., Vainstein, M. H. (2010). Metarhizium anisopliae Enzymes and Toxins. Toxicon. 56 (7), 1267–1274. doi: 10.1016/j.toxicon.2010.03.008
Segers, R., Butt, T. M., Kerry, B. R., Peberdy, J. F. (1994). The Nematophagous Fungus Verticillium chlamydosporium Produces a Chymoelastase-Like Protease Which Hydrolyses Host Nematode Proteins In Situ. Microbiology 140 (Pt 10), 2715–2723. doi: 10.1099/00221287-140-10-2715
St Leger, R. J., Nelson, J. O., Screen, S. E. (1999). The Entomopathogenic Fungus Metarhizium anisopliae Alters Ambient pH, Allowing Extracellular Protease Production and Activity. Microbiology 145, 2691–2699. doi: 10.1099/00221287-145-10-2691
Sun, M., Ren, Q., Guan, G., Li, Y., Han, X., Ma, C., et al. (2013). Effectiveness of Beauveria bassiana sensu lato Strains for Biological Control Against Rhipicephalus (Boophilus) microplus (Acari: Ixodidae) in China. Parasitol. Int. 62 (5), 412–415. doi: 10.1016/j.parint.2013.04.008
Valero-Jiménez, C. A., Wiegers, H., Zwaan, B. J., Koenrradt, C. J. M., Kan, J. A. (2016). Genes Involved in Virulence of the Entomopathogenic Fungus Beauveria bassiana. J. Invertebr. Pathol. 133, 41–49. doi: 10.1016/j.jip.2015.11.011
Wang, X. X., Ji, X. P., Li, J. X., Keyhani, N., Feng, M. G., Ying, S. H. (2013). A Putative Alpha-Glucoside Transporter Gene BbAGT1 Contributes to Carbohydrate Utilization, Growth, Conidiation and Virulence of Filamentous Entomopathogenic Fungus Beauveria bassiana. Res. Microbiol. 164 (5), 480–489. doi: 10.1016/j.resmic.2013.02.008
Zhang, S., Xia, Y. X., Kim, B., Keyhani, N. O. (2011). Two Hydrophobins are Involved in Fungal Spore Coat Rodlet Layer Assembly and Each Play Distinct Roles in Surface Interactions, Development and Pathogenesis in the Entomopathogenic Fungus, Beauveria bassiana. Mol. Microbiol. 80 (3), 811–826. doi: 10.1111/j.1365-2958.2011.07613.x
Keywords: tick, biological control, entomopathogenic fungi, cuticle, virulence, Beauveria bassiana, Rhipicephalus microplus, Msn2
Citation: Muniz ER, Ribeiro-Silva CS, Arruda W, Keyhani NO and Fernandes ÉKK (2021) The Msn2 Transcription Factor Regulates Acaricidal Virulence in the Fungal Pathogen Beauveria bassiana. Front. Cell. Infect. Microbiol. 11:690731. doi: 10.3389/fcimb.2021.690731
Received: 04 April 2021; Accepted: 18 June 2021;
Published: 20 July 2021.
Edited by:
Guilhem Janbon, Institut Pasteur, FranceReviewed by:
Almudena Ortiz-Urquiza, Swansea University, United KingdomHokyoung Son, Seoul National University, South Korea
Copyright © 2021 Muniz, Ribeiro-Silva, Arruda, Keyhani and Fernandes. This is an open-access article distributed under the terms of the Creative Commons Attribution License (CC BY). The use, distribution or reproduction in other forums is permitted, provided the original author(s) and the copyright owner(s) are credited and that the original publication in this journal is cited, in accordance with accepted academic practice. No use, distribution or reproduction is permitted which does not comply with these terms.
*Correspondence: Éverton K. K. Fernandes, ZXZlcnRvbmtvcnRAdWZnLmJy