- 1Univ Brest, CNRS, IRD, Ifremer, UMR 6539 LEMAR, Plouzane, France
- 2UMR CNRS 6286 UFIP, University of Nantes, Nantes, France
Vibrio tapetis is a Gram-negative bacterium that causes infections of mollusk bivalves and fish. The Brown Ring Disease (BRD) is an infection caused by V. tapetis that primarily affects the Manila clam Ruditapes philippinarum. Recent studies have shown that a type IV secretion system (T4SS) gene cluster is exclusively found in strains of V. tapetis pathogenic to clams. However, whether the T4SS is implicated or not during the infection process remains unknown. The aim of this study was to create and characterize a V. tapetis T4SS null mutant, obtained by a near-complete deletion of the virB4 gene, in order to determine the role of T4SS in the development of BRD. This study demonstrated that the T4SS is neither responsible for the loss of hemocyte adhesion capacities, nor for the decrease of the lysosomal activity during BRD. Nevertheless, we observed a 50% decrease of the BRD prevalence and a decrease of mortality dynamics with the ΔvirB4 mutant. This work demonstrates that the T4SS of V. tapetis plays an important role in the development of BRD in the Manila clam.
Introduction
Mass mortalities have occurred in the last decades impacting bivalves in hatcheries as well as in natural beds. Many Vibrio species are involved in these plagues and are known to be important pathogens in aquaculture (Travers et al., 2015). Vibrio tapetis (Borrego et al., 1996) is the etiological agent responsible for the Brown Ring Disease (BRD) (Paillard and Maes, 1990; Paillard et al., 1994) primarily affecting the Manila clam Ruditapes philippinarum (Linnaeus, 1758). BRD is characterized by a brown organic deposit, in the inner face of the shell, between the pallial line and the edge of the shell, which can often be observed in Manila clams during the winter and spring periods but is present all year long (Paillard et al., 2014; Paillard, 2016), mostly on the European Northern coast. BRD is a cold water disease based on the fact that V. tapetis is virulent only at low temperatures, with an optimum infection temperature of 14°C (Paillard et al., 1994; Paillard, 2004a).
The effects of BRD infection on R. philippinarum have been well documented. The pathogen enters the pallial cavity of clams and then colonizes and degrades their periostracum to penetrate into extrapallial fluids (EPFs) where they can spread (Paillard et al., 1994; Paillard, 2004a). Hemocytes, which are the clam’s immune system cells, are recruited to the site of infection within the extrapallial fluids to eliminate the pathogen (Allam et al., 2000). During a typical infection, hemocytes phagocytize foreign bacteria and mobilize the lysosome to eliminate them (Al-Khalaifah and Al-Nasser, 2018). These immune cells are characterized by pseudopods, which are actin-rich membrane expansions, that make them able to catch and internalize bacteria. During the development of BRD, V. tapetis cells are phagocytized by hemocytes, but once internalized, they inhibit several cellular functions such as actin polymerization or phagosome–lysosome fusion, thereby avoiding degradation (Choquet et al., 2003; Paillard, 2004a; Al-Khalaifah and Al-Nasser, 2018; Rahmani et al., 2019). Moreover, Reactive Oxygen Species (ROS) production is reduced in infected hemocytes (Paillard, 2017). These bacteria-mediated inhibitions of critical cellular functions lead to the multiplication of V. tapetis within the hemocytes, which are ultimately lysed when bacteria reach high densities, allowing V. tapetis cells to spread in EPFs.
However, while knowledge is improving regarding the behavior of clams and clam hemocytes upon infection by V. tapetis, very little is known about the precise mechanisms linked to the virulence of the pathogen. A characterization of the V. tapetis’ secretome upon exposure to Manila clam hemocytes allowed the identification of several proteins potentially involved in pathogenicity (Madec et al., 2014). V. tapetis is also able to produce several virulence factors potentially involved in its pathogenic capacity such as hemolysins, cytotoxins and exotoxins (Allam and Ford, 2006). It has also been recently demonstrated that the structures of the biofilms formed by different V. tapetis strains vary, depending on whether they are clam- or fish- pathogens (Rodrigues et al., 2015).
The genomes of various strains of V. tapetis have recently been sequenced and compared. Interestingly, this analysis showed that the Type IV Secretion System (T4SS) cluster is exclusively found in strains of V. tapetis reproducing BRD in clams and mainly isolated from infected clams, and not in the ones isolated from fish (Dias et al., 2018). Furthermore, a diagnostic method has recently been developed to quantify V. tapetis in EPFs relying on the presence of the virB4 gene (Bidault et al., 2015). The T4SS is a delivery system, which can transfer proteins, but also DNA, directly into recipient cells (which can be either prokaryotes or eukaryotes) (Christie and Cascales, 2005). T4SSs were first described in the plant pathogen Agrobacterium tumefaciens (Stachel and Nester, 1986) and are well known to be involved in pathogenicity in multiple infections such as Brucellosis (Brucella spp.) or Legionnaires’ disease (Legionella pneumophila) (Voth et al., 2012). One of the characteristics of T4SSs is the presence of a conserved ATPase, called VirB4 in A. tumefaciens. VirB4 is one of the two ATPase of this T4SS, being essential for both T4SS structure and activity (Kerr and Christie, 2010; Waksman, 2019).
Based on the presence of the T4SS cluster only in V. tapetis strains infecting clams together with the demonstrated role of T4SS in the pathogenicity of many bacteria, this secretion system is therefore an attractive candidate to be tested to understand the pathogenicity mechanisms used by V. tapetis during infection of the Manila clam. Furthermore, a recent RNAseq experiment demonstrated the expression of 8 virB genes belonging to V. tapetis CECT4600 T4SS, highlighting that this is an active cluster (Rodrigues et al., 2018). The best way to test such a hypothesis is to delete the corresponding genes. However, despite laborious efforts, only one deletion mutant (δdjlA) of V. tapetis CECT4600 infecting clams has been generated so far (Lakhal et al., 2008) and has shown its role on hemocyte cytotoxicity. This δdjlA mutant was not able to induce mortality or BRD development in clams, but this result may be a consequence of the growth defect phenotype of the mutant (Lakhal et al., 2008).
In this study, we aimed at clarifying the role of the T4SS in the development of the BRD, by deleting the virB4 gene of V. tapetis CECT4600. This gene is surrounded by virB2 (upstream) and virB6 (downstream, preceded by two hypothetical proteins) genes (Figures 1 and 2) (Dias et al., 2018). A targeted mutagenesis approach has been developed, which led to the deletion of the virB4 gene. We could demonstrate that the T4SS, while not being essential to induce vibriosis, plays an important role in BRD development in the Manila clam. This approach and the generation of deletion mutants will help to better understand the genes, and therefore the mechanisms, used by the pathogen during the development of BRD.
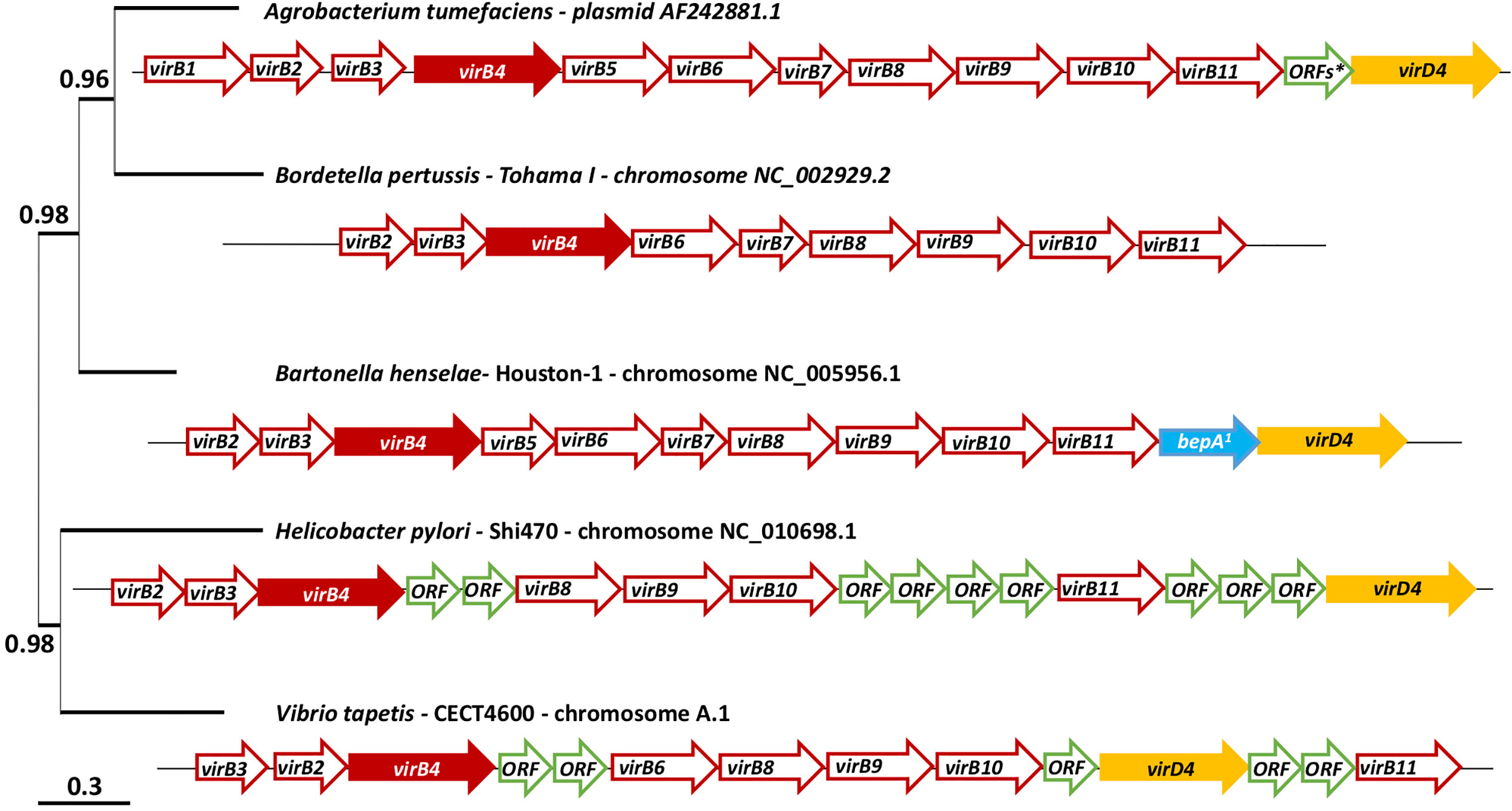
Figure 1 virB/D4 and homologues genes cluster coding the T4SS of Agrobacterium tumefaciens, Bordetella pertussis, Bartonella henselae, Helicobacter pylori and Vibrio tapetis and phylogenetic tree of these five strains. The phylogenetic tree was constructed using the gene virB4 and the software Phylogeny, “One click” mode (Dereeper et al., 2008). *This arrow represents 15 ORFs. a: bepA: bartonella effector protein. A detailed table (Table S1) is available on Supplementary Material with the name of the sequences available on MaGe: MicroScope plateform (Vallenet et al., 2009) and used to represent these cluster.
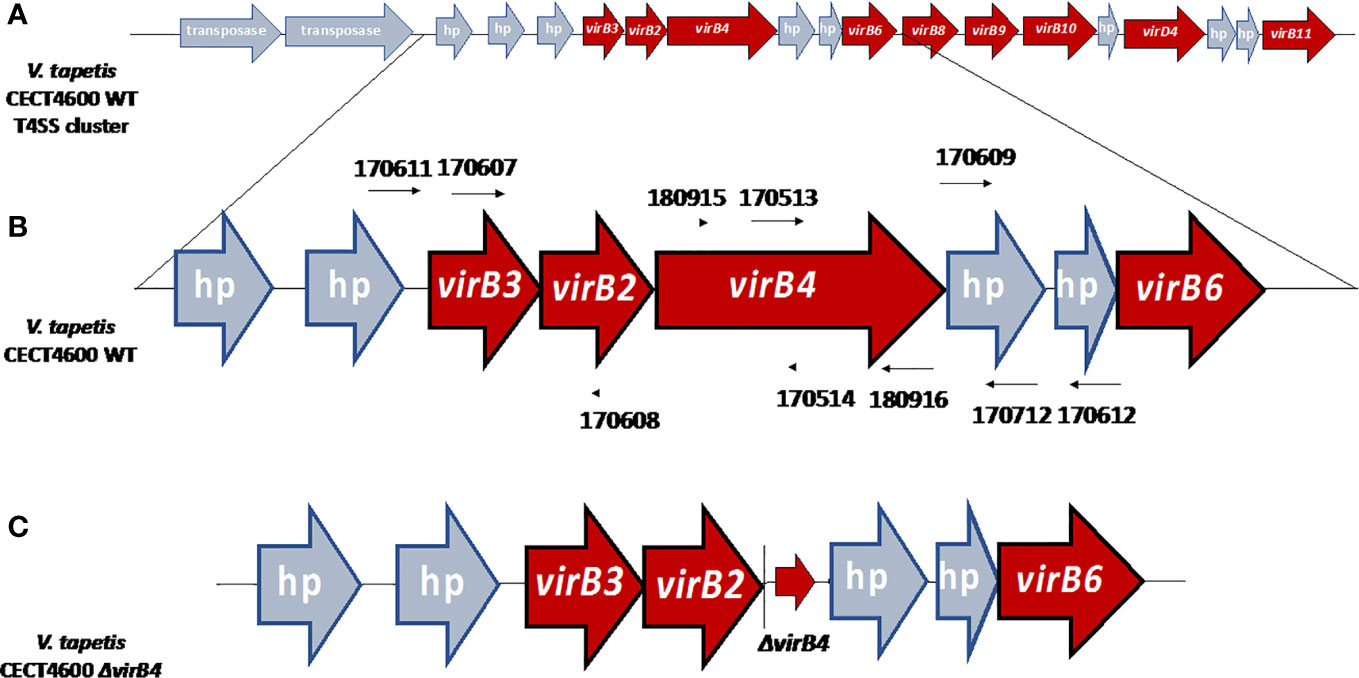
Figure 2 (A) Organization of the T4SS gene cluster of V. tapetis CECT4600 located in the chromosome A (accession number NZ_LT960611.1). (B) Genetic environment before the deletion of the virB4 gene with the positions of primers used in this study. UP and DOWN fragments have been amplified using primers 170,607–170,608 and 170,609–170,712 respectively. (C) Genetic environment after the deletion of virB4 gene, the ΔvirB4 arrow corresponding the three first and the three last codons of virB4 gene, allowing the formation of a non-functional peptide of six amino-acids, limiting the risk of polar effects. hp, hypothetical protein. Based on the description of V. tapetis CECT4600 T4SS in Dias et al., 2018.
Materials and Methods
Bacterial Strains, Plasmids and Culture Conditions
Bacterial strains used in this study are described in Table 1. Plasmids and primers used in this study are listed in Tables 2 and S2, respectively. Escherichia coli strains were routinely grown at 37°C in LB medium. V. tapetis CECT4600 and ΔvirB4 were grown in LB containing 20 g/l (final concentration) of NaCl (LBS) or in Zobell medium (Zobell, 1941) at 18°C ± 2°C. We used LBS for molecular biology because V. tapetis grows better in this rich medium and is therefore better suited for mutagenesis; Zobell was used for in vitro/in vivo experiments, because it allows a good reproducibility, allowing the present results to be compared with previous studies on this strain. When necessary, the following components were added: Kanamycin (Km, 100 µg/ml), Chloramphenicol (Cm, 5 µg/ml for E. coli (Cm5); 1 (Cm1) or 5 µg/ml (Cm5) for V. tapetis), Trimethoprim (Trim, 10 µg/ml), Diaminopimelic acid (DAP, 0.3 mM), D-Glucose (D-Glc 1%), L-Arabinose (L-Ara 0.2%) or agar (15 g/l). Growth parameters of strains CECT4600 and ΔvirB4 were determined by using a microplate reader BioscreenC (Bioscreen, Turku, Finland) in both LBS and Zobell media at 18°C with agitation, in 100-well microplates, after 24 h of growth.
Construction of a ΔvirB4 Deletion Mutant
The virB4 gene was deleted by double homologous recombination using a pLP12-derived suicide plasmid (Luo et al., 2015), under specific culture and mating conditions. Briefly, two fragments of 820 and 799 bp located upstream (UP) and downstream (DOWN) of the virB4 gene of V. tapetis CECT4600, respectively, were PCR-amplified using primers 170,607–170,608 and 170,609–170,712, respectively. The two DNA fragments were fused by Splicing by Overlap Extension (SOEing) PCR using primers 170607 and 170612, giving a single 1.6-kbp fragment UP-DOWN (UD-fragment) that was inserted into the pGEM-T plasmid (Promega, Madison, WI, USA) by ligation with T4 DNA ligase (Promega) according to the manufacturer recommendations. The integrity of the UD-fragment was verified by sequencing (Eurofins Genomics company, Ebersberg, Germany). And then released from pGEM-T using EcoRI and NheI (Promega, Madison) and ligated into the pLP12 plasmid digested with the same enzymes. The ligation mixture was transformed by heat shock into competent cells of E. coli DH5α λpir, and a clone containing the pLP12 plasmid in which the UD-fragment had been inserted (named pFD055) was stored at −80°C. The purified pFD055 plasmid was then transferred to E. coli GEB883 by heat shock transformation (strain 88). E. coli GEB883 was also transformed with the pEVS104 plasmid in order to obtain the helper strain (strain 102, Table 1) used in this study.
The pFD055 plasmid was transferred to V. tapetis CECT4600 by a triparental mating procedure. Briefly, strains 88, 102 and 2 (Table 1) were mixed at a 1:1:5 ratio. Each strain was harvested one after another, centrifuged (7,168×g, 3 min) and the supernatant was removed in order to avoid exposure to antibiotics for the next strain added. The cell pellet was finally resuspended in 20 µl of LBS + D-Glc + DAP and spotted on a cellulose acetate filter (0.22 µm, Sartorius Stedim biotech, Goettingen, Germany) deposited on a LBS + D-Glc + DAP plate. This plate was then incubated at 25°C for 48 h. The spot was then resuspended with 1 ml LBS, and 100 µl were plated on both LBS + D-Glc + Cm5 and LBS + D-Glc + Cm1. The remaining suspension was centrifuged (7,168xg, 3 min), resuspended in 200 µl of LBS, split into two 100 µl fractions which were plated on the same culture media. All plates were incubated at 20°C for 5 days.
The obtained colonies were re-streaked on LBS + D-Glc + Cm1 and the site of chromosomal insertion of pFD055 was determined by PCR, using 170,611–170,902 (for a recombination between UP fragments) and 170,901–170,612 (for a recombination between DOWN fragments).
After confirmation of plasmid integration, the single clone that had integrated pFD055 was cultivated overnight in LBS. The culture was serially diluted, and 100 µl were plated on LBS + L-Ara to induce expression of the Vmi480 toxin encoding gene, located in pLP12 (Luo et al., 2015), to select for excision of pFD055 by a second recombination. Following incubation at 20°C, the obtained colonies were tested for deletion of the virB4 gene by using primers 170,611–170,612 [expected size 1,759 bp in the mutant, 4,285 bp in the wild type (WT)] and 170,513–170,514 (no PCR product in the mutant, 173 bp in the WT). A V. tapetis mutant strain deleted for the virB4 gene was stored at −80°C (strain 177).
Complementation of the ΔvirB4 Deletion Mutant
The full coding sequence of the virB4 gene of V. tapetis CECT4600 (from the ATG start codon to the TGA stop codon) was PCR-amplified using 180,915–180,916, cloned into the pGEM-T vector and verified by sequencing. The obtained construction, as well as the pFD086 plasmid, were then both digested using XbaI and BamHI, the appropriate DNA fragments were purified from the electrophoresis gel (NucleoSpin Gel and PCR Clean-up kit, Macherey-Nagel, Hoerdt, France) and ligated. The replacement of the gfp gene in pFD086 by virB4 was checked by using primers 180,401–170,514 (expected size 2,848 bp). The obtained pAR003 plasmid was then transformed into V. tapetis ΔvirB4 (strain 177) by triparental mating as described earlier, by mixing the strains 234, 102, and 177 (Table 1) at 18°C, for 24 h. The mating mixture was plated on LBS + Trim and the presence of pAR003 in V. tapetis ΔvirB4 was confirmed by using 180,401–170,514 (expected size 2,848 bp) to obtain the strain 268.
In Vitro Virulence Assays on Manila Clams
The in vitro virulence assay was performed, following a standardized protocol developed in order to test the ability of V. tapetis strains to induce a rounding of hemocytes of the Manila clam (Choquet et al., 2003). Briefly, Manila clams from the SATMAR shellfish aquaculture site in Marennes (Charente-Maritime, France) were acclimatized in oxygenated seawater at 14°C. Hemolymph was harvested directly from the adductor muscle of each individual, and the quality of hemocytes was checked by microscopical observation (presence of pseudopods, number of rounded hemocytes). Good quality hemolymph samples were pooled and the hemocytes enumerated by using a Malassez cell. Hemolymph was exposed to a bacterial suspension of V. tapetis CECT4600 or its derivatives (Table 1, plated on Zobell agar) in at least three replicates and two independent experiments. 100 μl of hemolymph were added in 24-well plates, followed by 100 μl of bacterial suspension [in Filter-Sterilized Seawater (FSSW)], to obtain a 25/1:bacteria/hemocyte ratio);. For the controls, the bacterial suspension was replaced by 100 μl of FSSW. The flow cytometry analysis was coupled with the LysoTracker assay described below, and results were expressed as the ratio of non-adherent hemocytes in exposed vs control samples.
LysoTracker Assays on Manila Clams Hemocytes
The LysoTracker probes (Invitrogen™, Paisley, UK) are fluorescent acidotropic probes for labeling and tracking acidic organelles in live cells, according to the manufacturer’s definition. We used this method to characterize the amounts of acidic organelles in hemocytes exposed to the different strains of V. tapetis, or FSSW for control. This LysoTracker assay, performed during the in vitro virulence test, has recently been developed in order to correlate the rounding phenotype to the cell content in acidic organelles by performing both tests (i.e. virulence test and LysoTracker assay) on the same samples (Rahmani et al., 2019). This last study also highlight the highly probable link between the amount of acidic organelles and the fusion between the phagosome and lysosome using cytometry experiment with the LysoTracker coupled with a transcriptomic analysis in the case of BRD (Rahmani et al., 2019).
The virulence test was performed as described above. After 1 h of incubation at 18°C, 4 µl of 50 µM LysoTracker Red DND-99 (Invitrogen™, emission from 550 to 700 nm, final concentration 1 µM) was added to each well. After 2 more hours of incubation at 18°C, the contents of the wells were then transferred to 5-ml cytometry polystyrene Falcon tubes (BD Biosciences, San Jose, CA, USA).
Flow cytometry analyses were performed by using a BD FACSVerse flow cytometer using its blue laser (488 nm) as an excitation source. The mean red fluorescence level (LysoTracker fluorescence linked to acidic organelles) of the selected hemocytes was measured using the PerCP-Cy5.5 detector of the flow cytometer (700/54 nm).
Subsequently, 2 µl of a 100× dilution in ultra-pure water of a commercial solution of SYBR–Green I nucleic acid gel stain 10,000× in DMSO (Molecular Probes by Life Technology, CA, USA) were added in each tube for 10 min at room temperature, in dark conditions, before measurement of the green fluorescence. Addition of SYBR-Green allows the selection of hemocytes by FITC detector of the flow cytometer (527/32 nm) and then the quantification of the number of non-adherent hemocytes in our samples.
This second flow cytometry analysis allowed measurement of both the green and red fluorescences, and was performed by using excitation wavelengths of 488 and 640 nm, respectively. Results were expressed in mean red fluorescence level per hemocyte (arbitrary units, AU) measured with the APC detector of the flow cytometer (660/10 nm) instead of the PerCP-Cy5.5 detector (700/54 nm) to overcome a possible SYBR-Green fluorescence overlap.
To determine whether the differences between the ratio of non-adherent hemocytes and the amount of acidic organelles in exposed and controls samples are statistically significant, a Wilcoxon test was performed.
In Vivo Virulence Assays on Manila Clams
For the in vivo experiments, two modes of infection were used: (i) inoculation of a fixed number of bacteria into the pallial cavity, which results in BRD symptoms after four weeks (Oubella et al., 1994) and (ii) injection of V. tapetis strains into the adductor muscle, which induces clam mortalities that start 3 to 5 days after injection (Allam et al., 2002).
i. The day before injection, clams were removed from water to allow them to open enough for injection the next day. 150 clams were injected with 100 µl of a suspension (5 x 107 CFU/animal) of strains V. tapetis CECT 4600 or ΔvirB4, or with 100 µl FSSW as the control. All injected clams were left for 6 h out of water to promote the colonization of the periostracal lamina by V. tapetis. Animals were then placed into tanks (50 animals per replicate, in triplicate) equipped with a bubbler, at 14°C. Animals were not fed during the experiment and water was changed once after 2 weeks post injection (p.i.). The replicates were injected on 3 independent days. After 4 weeks p.i., animals were sacrificed and shells were kept and analyzed according to the method developed by Paillard and Maes (1994) to determine the BRD prevalence in each condition. To determine whether the BRD prevalence was statistically significant between these conditions, a Chi-2 test with 2 degrees of freedom was performed.
ii. 30 animals (ten clams per replicate) were injected in the adductor muscle with either a bacterial suspension (5 x 107 CFU/animal) of strains V. tapetis CECT4600, V. tapetis ΔvirB4 or V. tapetis ΔvirB4 +pAR003, respectively for the experimental conditions, or with 100 µl FSSW as a control. Animals were then placed into tanks equipped with a bubbler, at 14°C. Dead animals were enumerated and collected throughout the experiment, thus enabling determination of the mortality rates. Data represented survival rates for each condition. Statistical analyses were performed using Kaplan–Meier survival analysis and a Wilcoxon test on R software.
Bioinformatics and Biostatistics
Handling of DNA sequences was performed by using the SnapGene software version 5.1.7. (Insightful Science). Phylogenetic analysis was also performed, based on the virB4 genes of 4 bacterial pathogens that carry the same type of T4SS than V. tapetis CECT4600: Agrobacterium tumefaciens, Bartonella henselae, Helicobacter pylori and Bordetella pertussis. This analysis was conducted using the software Phylogeny.fr (Dereeper et al., 2008) that used for alignment: MUSCLE, for curation: Gblocks, for phylogeny: PhyML and for graphic representation: TreeDyn. The Atlas T4SS tools available at www.t4ss.lncc.br/ (Souza et al., 2012) was also used to highlight the annotation corresponding to the sequence VTAP_v1_a3555, that might correspond to the virB5 gene of V. tapetis CECT4600 T4SS cluster. All biostatistics analyses were performed using the R software (Core Team, R., 2017).
Results
Characterization of T4SS Genes Cluster of 5 Bacterial Pathogens and Phylogeny of the Gene virB4 Between These Strains
Figure 1 schematizes the T4SS gene cluster of V. tapetis CECT4600 as compared to four other pathogenic bacterial species, including the pathogen A. tumefaciens (metadata are also available in Table S1). The phylogenetic tree in Figure 1 also allows the representation of phylogenetic distance between the gene virB4 of each of these strains. V. tapetis CECT4600’s T4SS is composed of genes virB2, 3, 4, 6, 8, 9, 10, 11 and virD4 (Figures 1 and 2) (Dias et al., 2018). In the strain CECT4600 genome, genes virB1 and viB7 are missing as compared to the T4SS of A. tumefaciens. According to our previously published annotation (Dias et al., 2018), the virB5 gene is also missing. As we suspect that the sequence VTAP_v1_a3555 might correspond to the virB5 gene, we further analyzed this sequence and revealed that for this sequence, which is located between virB4 and virB6 genes, the ORF size, position, sequence variability and predicted N-terminal cleavage peptide are good indications that probably this is the virB5 gene. However, we found very low homology between the suspected VirB5 protein sequence and other VirB5 characterized proteins of (the highest homologies being obtained with that of Campylobacter jejuni, i.e. Blast E.value: 0.003, and 28% identity and 49% homology over a 99 aminoacids region) which might also indicate that further physiological analysis should be performed in order to confirm this annotation.
Deletion of the virB4 Gene in V. tapetis CECT4600 and Physiological Characterization of the ΔvirB4 Mutant
In order to determine the role of the T4SS in the virulence of V. tapetis CECT4600, the T4SS-conserved gene virB4 was chosen as a target for gene deletion. V. tapetis death occurs at around 30°C and its optimal growth temperature is 18°C, which is low compared to that of E. coli (Borrego et al., 1996). Based on the recent observation that the growth of Vibrio strains under supra-optimal conditions increases the chance of transferring foreign DNA into marine bacteria (Delavat et al., 2018), matings between V. tapetis CECT4600 and E. coli carrying the suicide plasmid pFD055 were performed at 25°C. After selection of chloramphenicol-resistant clones, one colony was obtained, that had integrated the pFD055 plasmid into its genome. This clone was tested by PCR which showed an integration of pFD055 by recombination within the “DOWN” region. After selection for the excision of the pFD055 plasmid by induction of the Vmi480 toxin with L-Arabinose, some of the obtained colonies were tested by colony-PCR to determine the genotype of the clones, regarding the presence of virB4. Ultimately, clones in which the virB4 gene was deleted in V. tapetis CECT4600 were obtained and one clone (Figure S1) was stored at −80°C for subsequent analyses.
In order to characterize the effect of the virB4 deletion on V. tapetis CECT4600, the doubling time of both strains was compared, under both LBS and Zobell culture conditions in a microplate. The growth rates of both strains in LBS were around two times slower than in Zobell, even though the final cell densities were higher when grown in LBS (Table S3). Statistical analyses of doubling time and lag phase were performed by Wilcoxon tests. The doubling time of the virB4 mutant as compared to the WT, was higher in LBS (p = 0.016) but not in Zobell (p = 0.06) and the lag phase was longer in LBS medium as compared to growth in the Zobell medium (p = 0.0002) for both strains. Therefore, although the absence of the virB4 gene leads to a slight increase in the doubling time of V. tapetis in LBS, this deletion does not significantly modify the growth of the mutant in Zobell medium.
Loss of Hemocytes’ Adhesion Properties, Observed During V. tapetis Infection, Is T4SS Independent
In order to characterize the importance of the T4SS in the virulence of V. tapetis CECT4600 toward R. philippinarum hemocytes, an in vitro adherence test of clam hemocytes was performed, comparing the WT- and the ΔvirB4- strains both in LBS (data not shown) and in Zobell media (Figures 3 and S2). Earlier works showed that V. tapetis CECT4600 induces a loss of hemocyte adhesion properties, by causing rounding of the cells and de-structuration of the actin cytoskeleton (Paillard, 2004b; Rahmani et al., 2019). Our results showed that the ratio of non-adherent hemocytes in exposed versus control samples was increased by 5.6 fold when hemocytes were exposed to V. tapetis CECT4600, as compared to hemocytes exposed to FSSW (Figures 3 and S2). Interestingly, inactivation of the T4SS did not lead to a decrease in this ratio (Figures 3 and S2) neither in Zobell or LBS. These results strongly suggest that the T4SS of V. tapetis does not contribute to the pathogen-induced loss of hemocyte adhesion properties.
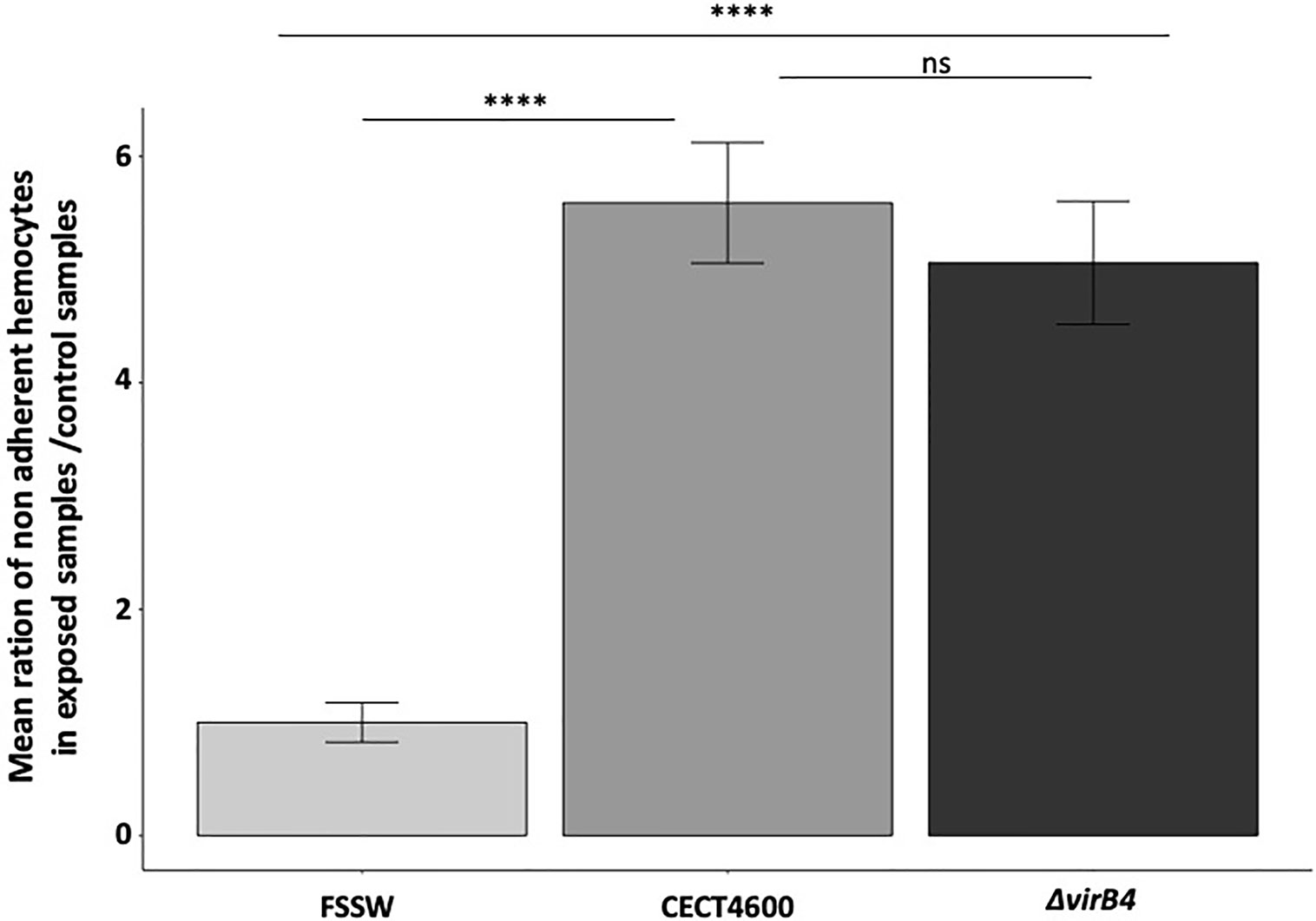
Figure 3 Effect of the ΔvirB4 mutation on the adhesion properties of clam hemocytes. Results are presented by mean ratio of non-adherent hemocytes (i.e. round hemocytes) on exposed samples/control samples. ****p value <10−4 (student test), ns, not significative. Cytograms and fluorescence pics are available in Figure S2. Flow repository ID: FR-FCM-Z3WT.
The T4SS Is Not Solely Responsible for the Inhibition of the Lysosomal Activity in V. tapetis Infected Hemocytes
To test whether the T4SS is involved in the inhibition of the phagosome–lysosome fusion, we characterized the amounts of acidic organelles in hemocytes exposed to V. tapetis or the virB4 mutant in comparison with exposure to FSSW, by using the LysoTracker Red DND-99 in flow cytometry. Hemocytes exposed to V. tapetis ΔvirB4 show significantly more acidic organelles as compared to CECT4600 (p = 0.004), highlighting a role of the T4SS in the decrease of lysosomal activity (Figures 4 and S2). However, the T4SS is not solely responsible, since the virB4 mutant still shows a 2-fold decrease in the amount of acidic organelles, as compared to the control hemocytes (p = 0.003).
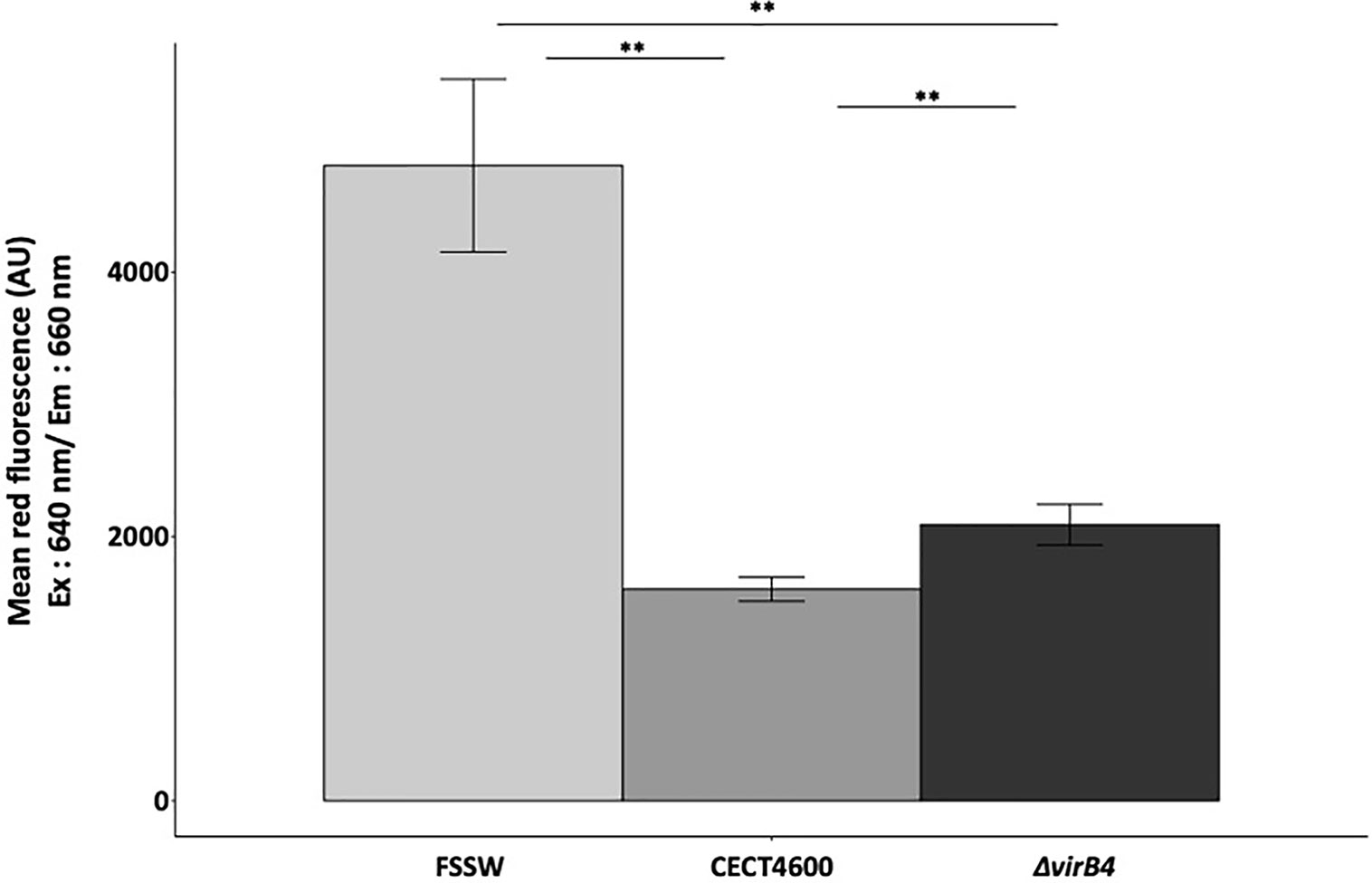
Figure 4 Measurement of acidic organelles amount by flow cytometry using LysoTracker Red DND-99®. Excitation of the probe has been done at 640 nm, as specified in the x axis. Mean fluorescence measured in this range of excitation laser in y axis for each strain tested. **p.value <0.004 (Wilcoxon test). Cytograms and fluorescence pics are available in Figure S2. Flow repository ID: FR-FCM-Z3WT.
The T4SS of V. tapetis Is Involved in BRD Development in the Manila Clam
Our in vitro assays revealed that the T4SS does not seem to be involved in the cytotoxic effect (loss of hemocytes adhesion properties) and is not strictly required in the phagosome–lysosome inhibition of V. tapetis within hemocytes. To test whether the T4SS is important for BRD development in the Manila clam, injections of V. tapetis CECT4600 or V. tapetis ΔvirB4 into the pallial cavity of clams were performed. BRD prevalence was determined after 4 weeks post-injection (Figures 5 and S3). The prevalence of BRD in control clams was very low (2%), confirming that clams were not infected before this experiment. After four weeks of infection, the prevalence of BRD observed in clams infected by V. tapetis CECT4600 was close to 60%, which is the expected prevalence already reported in several studies (Maes, 1992; Maes and Paillard, 1992; Paillard, 2004a; Paillard, 2004b). Interestingly, clams injected with a suspension of the ΔvirB4 mutant displayed a prevalence of BRD close to 30%, thus decreasing two-fold the prevalence of BRD in Manila clams. Consequently, our results show that the T4SS, although not essential, is a key contributor to BRD development.
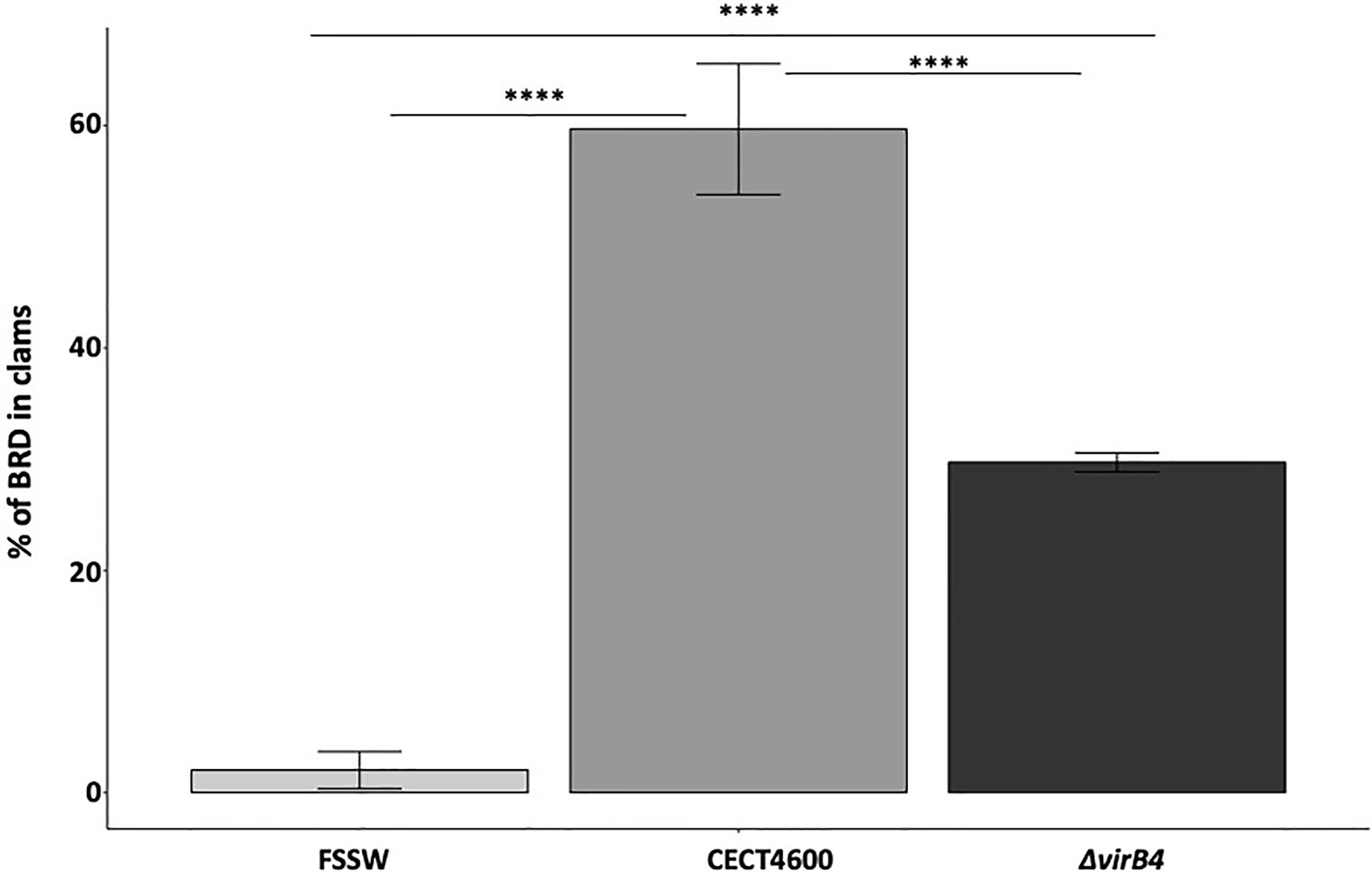
Figure 5 BRD prevalence after injection of V. tapetis strains CECT4600 and ΔvirB4 in the pallial cavity of the Manila clam. ****p value <10−4 (Chi-2 test, 2df).
The T4SS of V. tapetis Is Involved in the Mortality Dynamics Observed After Injection of V. tapetis in the Adductor Muscle
The T4SS is implicated in BRD formation, but it remained unknown whether this system is also important for the direct mortality of Manila clams. To answer this question, mortalities were monitored for 4 weeks after injection in the adductor muscle of Manila clams with V. tapetis strains. In this experiment, we expected mortality because the injection was performed in tissues (i.e. adductor muscle) and this protocol allows mortality within weeks as has been demonstrated previously (Allam et al., 2002; Choquet, 2004). Mortality rates observed in this experiment are presented in Figure 6. By using the Kaplan–Meier survival analysis, we showed that the mortality rates significantly differed among conditions (p = 0.002). Expectedly, the highest mortalities were observed when clams were injected with V. tapetis CECT4600. By contrast, V. tapetis ΔvirB4 displayed a lower virulence, as the mortality dynamics significantly differed from that of strains CECT4600 (p = 0.013). Specifically, the proportion of dead animals at the end of the experiment decreased from 43% to 23% following exposure to CECT4600 and ΔvirB4, respectively. In order to confirm that this phenotype was effectively due to the ΔvirB4 deletion, we performed genetic complementation on V. tapetis ΔvirB4 by introducing into this mutant a plasmid carrying the virB4 gene (from the ATG start codon to the TGA stop codon) cloned downstream of the Plac promotor and ribosome binding site (RBS) of the vector. The functional complementation of virB4 in the ΔvirB4 mutant partially restored the virulence of this strain (Figure 6), with 30% of clam mortality after 5 weeks of infection, and a mortality dynamics that did not significantly differ from that observed for V. tapetis CECT4600 (p = 0.52). Thus, the functional complementation of V. tapetis ΔvirB4 reproduced the mortality dynamics observed after injection in the adductor muscle of the V. tapetis CECT4600. This demonstrated that the attenuated virulence phenotype of V. tapetis ΔvirB4, in the case of mortalities dynamics caused by injection into the adductor muscle, is related to the deletion of the virB4 gene, thus demonstrating an important role for the T4SS in the virulence of V. tapetis.
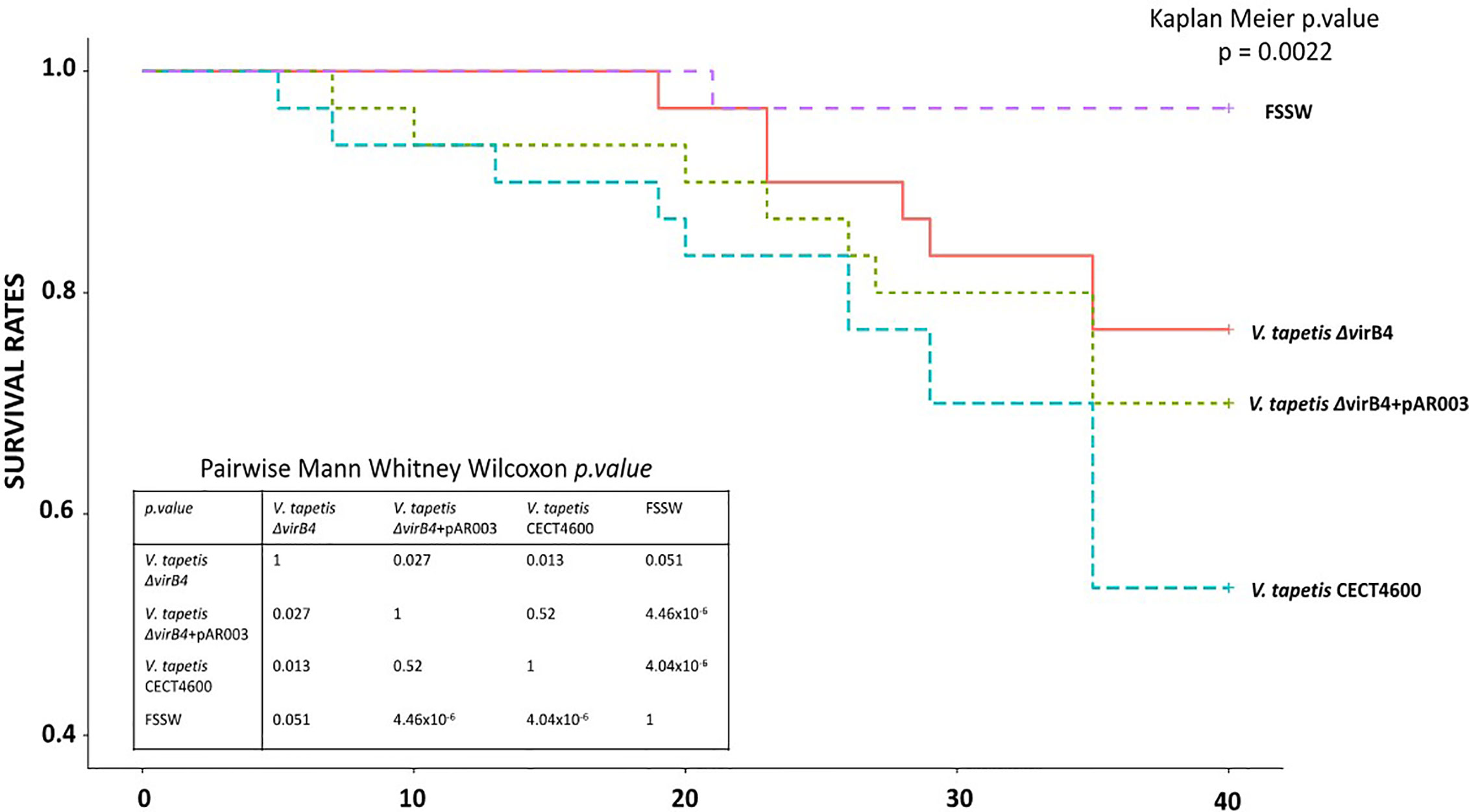
Figure 6 Mortality rates, using Kaplan–Meier analysis, observed after injection of strains V. tapetis strains CECT4600, ΔvirB4 and ΔvirB4+pAR003 (30 clams/conditions) in the adductor muscle of the Manila clam R. philippinarum.
Discussion
Brown Ring Disease (BRD) primarily affects the Manila clam R. philippinarum and is due to an infection by V. tapetis, a bacterium that acts as an external microparasite to induce this disease (Paillard, 2004a). V. tapetis colonizes and degrades the periostracal lamina, in order to enter the extrapallial fluids (EPFs). During this process, a brown organic deposit is produced that results from the inhibition of shell biomineralization. This inhibition leads to accumulation of the organic part of the shell, the conchyoline that forms a brown ring inside the shell, giving its name to BRD (Paillard et al., 1994). Invasion of V. tapetis into EPFs also leads to several perturbations in the immune response capacities of the Manila clam, exemplified by a phenotype of rounded hemocytes (Choquet et al., 2003; Le Bris et al., 2015). Indeed, it has been demonstrated that V. tapetis induces a loss of adhesion properties of infected hemocytes and a standardized test have been developed in order to show and measure this phenotype in vitro (Choquet et al., 2003). Studies have also demonstrated that V. tapetis is able to modify processes such as phagocytosis, ROS production and resistance capacities of the hemocyte cells during infection (Paillard, 2004b; Allam and Ford, 2006; Jeffroy and Paillard, 2011). These modifications of hemocyte physiology are accompanied by actin cytoskeleton re-organization, as has been shown by analyses of deregulated genes in clams during infection or physiological assay (Paillard, 2004b; Brulle et al., 2012; Allam et al., 2014; Rahmani et al., 2019).
Nevertheless, almost nothing is known about the molecular mechanisms used by the pathogen in order to induce the phenotypes described above. Interestingly, a recent genomic comparison of V. tapetis strains highlighted the probable involvement of T4SS in BRD (Dias et al., 2018). Dias et al. compared the genomes of seventeen strains of V. tapetis isolated from either bivalves or fish. They highlighted that strains of V. tapetis pathogenic to clams are the only ones that carry a cluster of 11 genes encoding a VirB/D4 T4SS. It is therefore likely that the T4SS is involved in this ability of strains that are pathogenic to Manila clams, to induce BRD. T4SSs are found in nearly half of bacterial genomes (Guglielmini et al., 2011) and are implicated in the conjugation of DNA and/or the secretion of effectors into prokaryotic and/or eukaryotic cells. Its molecular structure is also very different depending on the infection strategy implemented by the species that carries this cluster, and on the bacterial species itself. The T4SS genes organization of V. tapetis CECT4600 is very close to the VirB/D4 system of A. tumefaciens (Dias et al., 2018).
Given that T4SSs are present in many pathogens and that a T4SS is present only in V. tapetis strains pathogenic to clams (Bidault et al., 2015; Dias et al., 2018), we decided to inactivate this system in V. tapetis CECT4600. To achieve this goal, we focused our effort on the deletion of the virB4 gene of V. tapetis CECT4600. The VirB4 protein is one of the two ATPase of this T4SS and is involved in pilus biogenesis, as it provides the energy required for this process. The other ATPase, VirB11, functions with VirB4 to induce a structural change in the pilin and was thus suggested to modulate the VirB4 dislocase activity (Waksman, 2019).
Unfortunately, no efficient technique of gene deletion was available for V. tapetis, with only one study that was able to perform a gene deletion on V. tapetis. In that study Lakhal et al. (2008) succeeded more than 10 years ago to delete the djlA gene (849 bp), encoding a membrane-anchored DnaJ-Like protein that is involved in the rounding phenotype observed and measured by in vitro assay. V. tapetis δdjlA was not able to induce BRD in infected clams. However, the fact that this strain exhibits a significantly reduced growth rates makes its role in the virulence of V. tapetis in vivo undetermined (Lakhal et al., 2008). In this study, we succeeded in deleting the virB4 gene, using a derivative of the pLP12 suicide plasmid (Luo et al., 2015). To achieve this, we developed a mutagenesis protocol specifically designed for V. tapetis, by identifying the appropriate mating temperature allowing recombination of the suicide plasmid into the V. tapetis genome. Despite this being a low frequency occurrence, performing the matings at 25°C was key to obtain one transconjugant in which the suicide plasmid has recombined within the genome of V. tapetis CECT4600. This temperature is sublethal for V. tapetis CECT4600, as this strain can survive but is not able to grow at this temperature. This stressful condition is proposed to alter potential restriction/modification systems (Rolfe and Holloway, 1969), making these stressed cells more prone to accept foreign DNA. An electroporation protocol has recently been developed to introduce foreign DNA in various marine bacteria, and successful transformation was only obtained if cells were grown at supraoptimal temperature (Delavat et al., 2018).
V. tapetis is able to grow and develop in clam hemocytes after phagocytosis (Paillard, 2004a; Balbi et al., 2018). This ability to grow in hemocytes may be due to the activity of the T4SS, as demonstrated with various pathogens. Indeed, Legionella pneumophila, that induces the Legionnaire’s disease in humans, carries a specialized Dot/Icm T4SS. This T4SS induces the formation of a Legionella containing vacuole (LCV) and escapes fusion with the lysosome (Grohmann et al., 2018). The L. pneumophila T4SS structure is close to the Helicobacter pylori Cag T4SS (Ghosal et al., 2017), and the former system allows the translocation of more than 300 effectors in eukaryotic cells, affecting pathways controlling membrane transport processes (Sherwood and Roy, 2016; Grohmann et al., 2018). The LCV is able to fuse with endoplasmic reticulum and to move along microtubules to deregulate factors for host signaling. Nevertheless, in this study we demonstrated that the amount of acidic organelles, which might be a proxy of lysosomal activity in this model (Rahmani et al., 2019), decreases after challenge of hemocytes by V. tapetis in the WT strain but also in the virB4 mutant (Figure 3). Thus, although there is a probable link between intracellular survival and decrease of lysosomal activity, this process is not strictly dependent on the T4SS in the case of V. tapetis (Figure 4), which might reveal that a synergy of different mechanisms could be used by the pathogen to achieve this function.
The diversion of the actin cytoskeleton has already been shown in clam hemocytes following exposure to V. tapetis (Paillard, 2004b; Rahmani et al., 2019). Such a phenomenon has also been reported in other pathogenic species, forming a vacuole and encoding a T4SS. For example, H. pylori, that carries a cag pathogenicity island, and causes gastric ulcers and gastric adenocarcinoma in humans, secretes a protein, CagA, associated with the surface-exposed portion of the pilus (Backert et al., 2015). This effector is associated with the protein HtrA to open cell-to-cell junctions and to bind to the basolateral host receptor integrin. These proteins disorganize the apical junctions in stomach cells by a disorganization of the actin cytoskeleton (Selbach et al., 2003; Grohmann et al., 2018). Bartonella henselae carries a VirB/D4 type of T4SS which is involved in a reorganization of the cytoskeleton that leads to uptake of bacteria into endothelial cells (Grohmann et al., 2018). Finally, Coxiella burnetii, that causes Q-fever in humans, forms a Coxiella-containing-vacuole (CCV), using a Dot/Icm-like T4SS similar to that of L. pneumophila. It modifies host endocytic transport systems and replicates in CCVs interacting with the autophagosome (Kohler et al., 2016). Unlike V. tapetis, C. burnetii grows in a CCV that has acquired lysosomal characteristics such as acidic pH, acid hydrolases and cationic peptides (Voth and Heinzen, 2007). Interestingly, in vitro incubation of R. philippinarum hemocytes with V. tapetis heated cultures had revealed that the severe changes that affect hemocytes during exposure to V. tapetis (i.e. changes in structure and decrease in hemocyte phagocytic activity and viability) are related to a thermosensitive molecule (Allam and Ford, 2006). Thus, V. tapetis seems to possess thermosensitive cytotoxic factor(s) mediated by intact bacterial cells, which are also present as extracellular secretions (Allam and Ford, 2006) and might be secreted through the T4SS or even maybe a thermosensitive export system. In the dynamics of BRD, disorganization of hemocyte adhesion capacities is followed by an absence of phagolysosome fusion, thus allowing V. tapetis growth in the infected hemocytes in a vacuole formed after phagocytosis (Paillard, 2004b). Nevertheless, we demonstrated in this study that T4SS is not implicated in the cytotoxic effect of V. tapetis toward hemocytes, as in vitro assays showed that a virB4 null mutant still induces a loss of hemocyte adhesion properties (Figure 3). Furthermore, since the VirB4 protein is involved in the pilus formation (Waksman, 2019), the predicted absence of a pilus in the virB4 mutant shows that the T4SS pilus is probably not involved in this phenotype.
Finally, we could demonstrate that the deletion of the virB4 gene in V. tapetis CECT4600, and therefore inactivation of its T4SS, significantly reduced BRD prevalence by 50% when compared to the WT strain (Figure 5). Furthermore, clam mortality dynamics and rates after injection of the adductor muscle significantly decreased in V. tapetis ΔvirB4, as compared to the WT strain (Figure 6). We did not test whether the complemented strain is able to restore the prevalence of BRD phenotype induced by the WT (Figure 5), because we wanted to avoid the massive overuse (and sacrifice) of animals in our study (150 animals for an experiment reproducing BRD against 30 animals for an experiment reproducing septicemic mortalities, for each condition). However, we did construct a complemented mutant of virB4, which restored the mortality dynamics and partially this mortality rates (Figure 6). We hypothesize that this only partial complementation of mortality rate is due to the ectopic expression of the virB4 gene in our complemented strain.
Indeed, due to the lack of other genetic tools (like mini-transposons) available for this strain, we cloned the virB4 gene in a replicative plasmid. Complementation using a replicative plasmid as the caveat that the number of plasmid copies cannot be controlled, influencing the regulation of the virB4 gene expression and the number of VirB4 molecules. This, together with the production of virB4 using the Plac promotor and RBS of the plasmid, may explain this only partial restoration of the virulence phenotype. All in all, we showed that the T4SS has a direct involvement in the virulence and BRD formation by V. tapetis. Interestingly, previous studies focusing on V. tapetis LP2, a strain that does not carry the T4SS cluster, might help us to understand the role of T4SS in the development of BRD. The LP2 strain has been isolated from the fish Symphodus melops (Jensen et al., 2003) and is not able to induce BRD in the classical way of infection (i.e. injection into the pallial cavity or balneation) (Choquet, 2004; Bidault et al., 2015; Dias et al., 2018). However, if this strain is injected directly into the extrapallial cavity of Manila clams, it can induce BRD at rates of 75%, as compared to strain CECT4600 injected into extrapallial cavity that had induced 100% of BRD is this particular study (Le Bris et al., 2015).
Considering the knowledge about other bacterial species encoding T4SS, it is likely that V. tapetis is able to secrete effector proteins during the dynamics of BRD. Nevertheless, because a virB4 mutant is not able to reduce loss of adhesion properties in infected hemocytes, and because prevalence of BRD is reduced in the absence of the virB4 gene, we hypothesize that V. tapetis T4SS would probably be involved in the colonization of the host, thus suggesting that T4SS-pilus-mediated colonization is a key factor for the specificity of these pathogenic strains of V. tapetis, and in our study the strain CECT4600, toward the Manila clam. The BRD owes its name from the brown organic deposit observed during infection by V. tapetis. This reaction of the Manila clams to infection occurs in two steps: conchiolin deposit and shell process repair to enrobe bacteria (Paillard and Maes, 1995). It has also been shown that V. tapetis is able to disrupt the host biomineralization process to facilitate colonization (Trinkler et al., 2011). Indeed, V. tapetis colonizes the periostracal lamina and the epithelia of mantle fold (Paillard, 2004b). The mantle edge cells are involved in pathogen recognition and the “nacrezation” defense mechanism with the secretion of melanized matrices (Paillard et al., 1994; Allam et al., 2014; Allam and Raftos, 2015). Thus, we could hypothesize that the T4SS interacts with the shell biomineralization process by injecting effectors into the cytosol of mantle edge cells. Considering our study, the ΔvirB4 strain is able to colonize (at least partially) the periostracal blade and epithelium of the mantle edge, but it might induce a weaker immune response because this strain might be less recognized by the mantle cell receptors and therefore induces less brown deposit characterizing BRD.
Future analyses will focus on colonization of the periostracal lamina as observed by light and electronic microscopy but also on phenoloxydase production in mantle and extrapallial fluids, in order to test these hypotheses (Paillard, 2004a; Le Bris et al., 2015).
Conclusions
In this study, we showed that the T4SS plays an important role in the dynamics of BRD but is not essential for BRD development. Considering our results and the knowledge on other pathogens carrying T4SSs, we hypothesize that this system might be involved in colonization steps or in the pathogen’s induction of the immune response in the development of BRD. Both of these hypotheses might be consistent with the reduced prevalence of BRD observed in vivo.
Gene deletion has been reported before, but in that study, only in vitro virulence assays were interpretable. Therefore, this study is the first gene deletion approach on V. tapetis that unveils an important molecular mechanism involved in the pathogenic activity of this bacterium in the BRD and not only on in vitro markers. Furthermore, it is the first study that confirms the involvement of T4SS in the development of BRD.
Data Availability Statement
The raw data supporting the conclusions of this article will be made available by the authors, without undue reservation.
Author Contributions
CP and VP acquired funds and coordinated the study. AR performed the experimental mutagenesis with the knowledge and help of FD. AR performed in vitro tests with the help of NL and CL for the flow cytometry analyses. AR performed in vivo infections technical design with the help of ED, NL, and CP. AR, FD, VP, and CP wrote the article (the original draft was written by AR). The article was carefully reviewed by other co-authors, who all approved the final version. All authors contributed to the article and approved the submitted version.
Funding
This project received grants from the H2020 European project “VIVALDI” (grant agreement N°678589). This work was also supported by the University of Western Brittany (UBO), the “investment for the future” programs LabexMER (ANR-10-LABX-19) and ISblue (ANR-17-EURE-0015).
Conflict of Interest
The authors declare that the research was conducted in the absence of any commercial or financial relationships that could be construed as a potential conflict of interest.
Acknowledgments
We warmly thank Jean-François Auvray (SATMAR, Marennes, Charentes-Maritimes) for providing the clams, and Adeline Bidault and Morgan Perennou for helping with clam dissections.
Supplementary Material
The Supplementary Material for this article can be found online at: https://www.frontiersin.org/articles/10.3389/fcimb.2021.634427/full#supplementary-material
Supplementary Figure 1 | PCR with primers 170513-170514 that amplified virB4 gene. A fragment of 173 bp is expected if the gene virB4 is present, no amplification is expected if the gene virB4 is absent. T-: negative control of PCR with sterile water.
Supplementary Figure 2 | Cytograms and Fluorescence Peaks corresponding to results of count of non-adherent hemocytes (laser FITC, Figure 3) and amount of acidic organelles (laser APC, Figure 4) in the conditions: control FSSW, V. tapetis CECT4600 and V. tapetis ΔvirB4. Flow repository ID: FR-FCM-Z3WT. (A–C): Green fluorescence (FITC) in function of granularity (SSC) of the cells that belong to a region corresponding of total non-adherent hemocytes in the conditions. This region was defined to target hemocytes. Since our experiment only harvested non-adherent hemocytes, this region includes all the non-adherent hemocytes of the sample in the condition tested. (A) control FSSW, (B) V. tapetis CECT4600 and (C) V. tapetis ΔvirB4. (E) Distribution of the green fluorescence (FITC) of the total hemocytes count (same region as in figures A–C), in the conditions: control FSSW, V. tapetis CECT4600 and V. tapetis ΔvirB4. (F) Distribution of the red fluorescence (APC) of the total hemocytes count (same region as in figures A–C), in the conditions: control FSSW, V. tapetis CECT4600 and V. tapetis ΔvirB4.
Supplementary Figure 3 | Picture of a Brown Ring Disease deposit on an infected Manila clam of the experiment described in Figure 5. (A) 1 valve pictured without a zoom. (B, C) Picture realized with a binocular loupe. The black arrows point the brown deposit that characterizes BRD at different zoom.
Abbreviations
BRD, Brown Ring Disease; CFU, Colony Forming Unit; EPFs, Extrapallial Fluids; FSSW, Filter Sterilized Sea Water; LBS, Luria Broth Salted; PCR, Polymerase Chain Reaction; ROS, Reactive Oxygen Species; T4SS, Type IV Secretion System; WT, Wild Type.
References
Al-Khalaifah H., Al-Nasser A. (2018). “Immune Response of Molluscs”. In: Diarte-Plata D., Escamilla-Montes R., editors. Molluscs. IntechOpen. doi: 10.5772/intechopen.81778
Allam B., Ford S. E. (2006). Effects of the pathogenic Vibrio tapetis on defence factors of susceptible and non-susceptible bivalve species: I. Haemocyte changes following in vitro challenge. Fish Shellfish Immunol. 20, 374–383. doi: 10.1016/j.fsi.2005.05.012
Allam B., Raftos D. (2015). Immune responses to infectious diseases in bivalves. J. Invertebr. Pathol. 131, 121–136. doi: 10.1016/j.jip.2015.05.005
Allam B., Paillard C., Auffret M. (2000). Alterations in hemolymph and extrapallial fluid parameters in the Manila clam, Ruditapes philippinarum, challenged with the pathogen Vibrio tapetis. J. Invertebr. Pathol. 76, 63–69. doi: 10.1006/jipa.2000.494
Allam B., Paillard C., Ford S. E. (2002). Pathogenicity of Vibrio tapetis, the etiological agent of brown ring disease in clams. Dis. Aquat. Organ. 48, 221–231. doi: 10.3354/dao048221
Allam B., Pales Espinosa E., Tanguy A., Jeffroy F., Le Bris C., Paillard C. (2014). Transcriptional changes in Manila clam (Ruditapes philippinarum) in response to Brown Ring Disease. Fish Shellfish Immunol. 41, 2–11. doi: 10.1016/j.fsi.2014.05.022
Backert S., Tegtmeyer N., Fischer W. (2015). Composition, structure and function of the Helicobacter pylori cag pathogenicity island encoded type IV secretion system. Future Microbiol. 10, 955–965. doi: 10.2217/fmb.15.32
Balbi T., Cortese K., Ciacci C., Bellese G., Vezzulli L., Pruzzo C., et al. (2018). Autophagic processes in Mytilus galloprovincialis hemocytes: Effects of Vibrio tapetis. Fish Shellfish Immunol. 73, 66–74. doi: 10.1016/j.fsi.2017.12.003
Bidault A., Richard G. G., Le Bris C., Paillard C. (2015). Development of a Taqman real-time PCR assay for rapid detection and quantification of Vibrio tapetis in extrapallial fluids of clams. PeerJ 3, e1484. doi: 10.7717/peerj.1484
Borrego J. J., Castro D., Luque A., Paillard C., Maes P., Garcia M. T., et al. (1996). Vibrio tapetis sp. nov., the causative agent of the brown ring disease affecting cultured clams. Int. J. Syst. Evol. Microbiol. 46, 480–484. doi: 10.1099/00207713-46-2-480
Brulle F., Jeffroy F., Madec S., Nicolas J.-L., Paillard C. (2012). Transcriptomic analysis of Ruditapes philippinarum hemocytes reveals cytoskeleton disruption after in vitro Vibrio tapetis challenge. Dev. Comp. Immunol. 38, 368–376. doi: 10.1016/j.dci.2012.03.003
Choquet G., Soudant P., Lambert C., Nicolas J.-L., Paillard C. (2003). Reduction of adhesion properties of Ruditapes philippinarum hemocytes exposed to Vibrio tapetis. Dis. Aquat. Organ. 57, 109–116. doi: 10.3354/dao057109
Choquet G. (2004). Caractérisation et pathogénie des isolats de Vibrio tapetis, bactérie responsable de la maladie de l’anneau brun chez la palourde japonaise (Brest). Available at: http://www.theses.fr/2004BRES2029 (Accessed September 10, 2018).
Christie P. J., Cascales E. (2005). Structural and dynamic properties of bacterial Type IV secretion systems (Review). Mol. Membr. Biol. 22, 51–61. doi: 10.1080/09687860500063316
Core Team, R. (2017). R: A language and environment for statistical computing. R Foundation for Statistical Computing (Vienna Austria). Available at: https://wwwR-Proj.OrgGoogleSch.
Delavat F., Bidault A., Pichereau V., Paillard C. (2018). Rapid and efficient protocol to introduce exogenous DNA in Vibrio harveyi and. Pseudoalteromonas sp. J. Microbiol. Methods 154, 1–5. doi: 10.1016/j.mimet.2018.09.022
Dereeper A., Guignon V., Blanc G., Audic S., Buffet S., Chevenet F., et al. (2008). Phylogeny.fr: robust phylogenetic analysis for the non-specialist. Nucleic Acids Res. 36, W465–W469. doi: 10.1093/nar/gkn180
Dias G. M., Bidault A., Le Chevalier P., Choquet G., Der Sarkissian C., Orlando L., et al. (2018). Vibrio tapetis Displays an Original Type IV Secretion System in Strains Pathogenic for Bivalve Molluscs. Front. Microbiol. 9, 227. doi: 10.3389/fmicb.2018.00227
Dunn A. K., Millikan D. S., Adin D. M., Bose J. L., Stabb E. V. (2006). New rfp- and pES213-Derived Tools for Analyzing Symbiotic Vibrio fischeri Reveal Patterns of Infection and lux Expression In Situ. Appl. Environ. Microbiol. 72, 802–810. doi: 10.1128/AEM.72.1.802-810.2006
Ghosal D., Chang Y.-W., Jeong K. C., Vogel J. P., Jensen G. J. (2017). In situ structure of the Legionella Dot/Icm type IV secretion system by electron cryotomography. EMBO Rep. 18, 726–732. doi: 10.15252/embr.201643598
Grohmann E., Christie P. J., Waksman G., Backert S. (2018). Type IV secretion in Gram-negative and Gram-positive bacteria: Type IV secretion. Mol. Microbiol. 107, 455–471. doi: 10.1111/mmi.13896
Guglielmini J., Quintais L., Garcillán-Barcia M. P., de la Cruz F., Rocha E. P. C. (2011). The Repertoire of ICE in Prokaryotes Underscores the Unity, Diversity, and Ubiquity of Conjugation. PloS Genet. 7, e1002222. doi: 10.1371/journal.pgen.1002222
Jeffroy F., Paillard C. (2011). Involvement of nitric oxide in the in vitro interaction between Manila clam, Ruditapes philippinarum, hemocytes and the bacterium Vibrio tapetis. Fish Shellfish Immunol. 31, 1137–1141. doi: 10.1016/j.fsi.2011.10.008
Jensen S., Samuelsen O. B., Andersen K., Torkildsen L., Lambert C., Choquet G., et al. (2003). Characterization of strains of Vibrio splendidus and V. tapetis isolated from corkwing wrasse Symphodus melops suffering vibriosis. Dis. Aquat. Organ. 53, 25–31. doi: 10.3354/dao053025
Kerr J. E., Christie P. J. (2010). Evidence for VirB4-Mediated Dislocation of Membrane-Integrated VirB2 Pilin during Biogenesis of the Agrobacterium VirB/VirD4 Type IV Secretion System. J. Bacteriol. 192, 4923–4934. doi: 10.1128/JB.00557-10
Kohler L. J., Reed S. R., Sarraf S. A., Arteaga D. D., Newton H. J., Roy C. R. (2016). Effector Protein Cig2 Decreases Host Tolerance of Infection by Directing Constitutive Fusion of Autophagosomes with the Coxiella-Containing Vacuole. mBio 7, e01127–e01116. doi: 10.1128/mBio.01127-16
Lakhal F., Bury-Mone S., Nomane Y., Le Goic N., Paillard C., Jacq A. (2008). DjlA, a Membrane-Anchored DnaJ-Like Protein, Is Required for Cytotoxicity of Clam Pathogen Vibrio tapetis to Hemocytes. Appl. Environ. Microbiol. 74, 5750–5758. doi: 10.1128/AEM.01043-08
Le Bris C., Richard G., Paillard C., Lambert C., Seguineau C., Gauthier O., et al. (2015). Immune responses of phenoloxidase and superoxide dismutase in the manila clam Venerupis philippinarum challenged with Vibrio tapetis – Part I: Spatio-temporal evolution of enzymes’ activities post-infection. Fish Shellfish Immunol. 42, 16–24. doi: 10.1016/j.fsi.2014.10.021
Luo P., He X., Liu Q., Hu C. (2015). Developing universal genetic tools for rapid and efficient deletion mutation in Vibrio species based on suicide T-Vectors carrying a novel counterselectable marker, vmi480. PloS One 10, e0144465. doi: 10.1145/2818302
Madec S., Pichereau V., Jacq A., Paillard M., Boisset C., Guérard F., et al. (2014). Characterization of the Secretomes of Two Vibrios Pathogenic to Mollusks. PloS One 9, e113097. doi: 10.1371/journal.pone.0113097
Maes P., Paillard C. (1992). “Effect du Vibrio P1, pathogene de Ruditapes philippinarum, sur d’autres espèces de bivalves,” in Les Mollusques Marins: Biologie et Aquaculture (Brest: ACtes colloq, IFREMER).
Maes P. (1992). Pathologie bactérienne chez deux invertébrés marins d’intérêt commercial, Ruditapes philippinarum et Paracentrotus lividus. Brest Univ. Bretagne Occident.
Nguyen A. N., Disconzi E., Charrière G. M., Destoumieux-Garzón D., Bouloc P., Le Roux F., et al. (2018). csrB Gene Duplication Drives the Evolution of Redundant Regulatory Pathways Controlling Expression of the Major Toxic Secreted Metalloproteases in Vibrio tasmaniensis LGP32. mSphere 3, e00582–e00518. doi: 10.1128/mSphere.00582-18 msphere/3/6/mSphere582-18.atom.
Oubella R., Paillard C., Maes P., Auffret M. (1994). Changes in hemolymph parameters in the Manila clam Ruditapes philippinarum (Mollusca, Bivalvia) following bacterial challenge. J. Invertebr. Pathol. 64, 33–38. doi: 10.1006/jipa.1994.1065
Paillard C., Maes P. (1990). Aetiology of brown ring disease in Tapes philippinarum: pathogenicity of a. Vibrio sp. Comptes Rendus Acad. Sci. 310, 15–20.
Paillard C., Maes P. (1994). Brown ring disease in the Manila clam Ruditapes philippinarum : establishment of a classification system. Dis. Aquat. Organ. 19, 137–146. doi: 10.3354/dao019137
Paillard C., Maes P. (1995). The brown ring disease in the Manila clam, Ruditapes philippinarum: I. Ultrastructural alterations of the periostracal lamina. J. Invertebr. Pathol. 65, 91–100. doi: 10.1006/jipa.1995.1015
Paillard C., Maes P., Oubella R. (1994). Brown ring disease in clams. Annu. Rev. Fish Dis. 4, 219–240. doi: 10.1016/0959-8030(94)90030-2
Paillard C., Jean F., Ford S. E., Powell E. N., Klinck J. M., Hofmann E. E., et al. (2014). A theoretical individual-based model of Brown Ring Disease in Manila clams, Venerupis philippinarum. J. Sea Res. 91, 15–34. doi: 10.1016/j.seares.2014.03.005
Paillard C. (2004a). A short-review of brown ring disease, a vibriosis affecting clams, Ruditapes philippinarum and Ruditapes decussatus. Aquat. Living Resour. 17, 467–475. doi: 10.1051/alr:2004053
Paillard C. (2004b). Rôle de l’environnement dans les interactions hôtes-pathogènes; développement d’un modèle de vibriose chez les bivalves. Habilit. À Dir. Rech. HDR Univ. Bretagne Occident. Brest 180, 1–180.
Paillard C. (2016).An ecological approach to understanding host-pathogen-environment interactions: the case of Brown Ring Disease in clams. In: Oysters and Clams: Cultivation, Habitat Threats and Ecological Impact (Microbiology research advances, Nova Science Publishers). Available at: https://hal.archives-ouvertes.fr/hal-01426301 (Accessed February 22, 2017).
Paillard C. (2017). Brown ring disease: a vibriosis affecting clams Ruditapes philippinarum and R. decussatus. ICES Identif. Leafl. Dis. Parasites Fish Shellfish 12, 1–12. doi: 10.17895/ices.pub.1924
Rahmani A., Corre E., Richard G., Bidault A., Lambert C., Oliveira L., et al. (2019). Transcriptomic analysis of clam extrapallial fluids reveals immunity and cytoskeleton alterations in the first week of Brown Ring Disease development. Fish Shellfish Immunol. 93, 940–948. doi: 10.1016/j.fsi.2019.08.025
Rodrigues S., Paillard C., Le Pennec G., Dufour A., Bazire A. (2015). Vibrio tapetis, the Causative Agent of Brown Ring Disease, Forms Biofilms with Spherical Components. Front. Microbiol. 6, 1384. doi: 10.3389/fmicb.2015.01384
Rodrigues S., Paillard C., Van Dillen S., Tahrioui A., Berjeaud J.-M., Dufour A., et al. (2018). Relation between Biofilm and Virulence in Vibrio tapetis: A Transcriptomic Study. Pathogens 7, 92. doi: 10.3390/pathogens7040092
Rolfe B., Holloway B. W. (1969). Host Specificity of DNA and Conjugation in Pseudomonas aeruginosa. Genetics 61, 341–349. doi: 10.1093/genetics/61.2.341
Selbach M., Moese S., Hurwitz R., Hauck C. R., Meyer T. F., Backert S. (2003). The Helicobacter pylori CagA protein induces cortactin dephosphorylation and actin rearrangement by c-Src inactivation. EMBO J. 22, 515–528. doi: 10.1093/emboj/cdg050
Sherwood R. K., Roy C. R. (2016). Autophagy Evasion and Endoplasmic Reticulum Subversion: The Yin and Yang of Legionella Intracellular Infection. Annu. Rev. Microbiol. 70, 413–433. doi: 10.1146/annurev-micro-102215-095557
Souza R. C., Saji G., del R. Q., Costa M. O., Netto D. S., Lima N. C., et al. (2012). AtlasT4SS: a curated database for type IV secretion systems. BMC Microbiol. 12, 172. doi: 10.1186/1471-2180-12-172
Stabb E. V., Ruby E. G. (2002). RP4-based plasmids for conjugation between Escherichia coli and members of the Vibrionaceae. Methods Enzymol. 358, 413–426. doi: 10.1016/S0076-6879(02)58106-4
Stachel S. E., Nester E. W. (1986). The genetic and transcriptional organization of the vir region of the A6 Ti plasmid of Agrobacterium tumefaciens. EMBO J. 5, 1445–1454. doi: 10.1002/j.1460-2075.1986.tb04381.x
Stoudenmire J. L., Essock-Burns T., Weathers E. N., Solaimanpour S., Mrázek J., Stabb E. V. (2018). An Iterative, Synthetic Approach To Engineer a High-Performance PhoB-Specific Reporter. Appl. Environ. Microbiol. 84, e00603–e00618. doi: 10.1128/AEM.00603-18
Travers M.-A., Boettcher Miller K., Roque A., Friedman C. S. (2015). Bacterial diseases in marine bivalves. J. Invertebr. Pathol. 131, 11–31. doi: 10.1016/j.jip.2015.07.010
Trinkler N., Bardeau J., Marin F., Labonne M., Jolivet A., Crassous P., et al. (2011). Mineral phase in shell repair of Manila clam Venerupis philippinarum affected by brown ring disease. Dis. Aquat. Organ. 93, 149–162. doi: 10.3354/dao02288
Vallenet D., Engelen S., Mornico D., Cruveiller S., Fleury L., Lajus A., et al. (2009). MicroScope: a platform for microbial genome annotation and comparative genomics. Database 2009. doi: 10.1093/database/bap021
Voth D. E., Heinzen R. A. (2007). Lounging in a lysosome: the intracellular lifestyle of Coxiella burnetii. Cell. Microbiol. 9, 829–840. doi: 10.1111/j.1462-5822.2007.00901.x
Voth D. E., Broederdorf L. J., Graham J. G. (2012). Bacterial Type IV secretion systems: versatile virulence machines. Future Microbiol. 7, 241–257. doi: 10.2217/fmb.11.150
Waksman G. (2019). From conjugation to T4S systems in Gram-negative bacteria: a mechanistic biology perspective. EMBO Rep. 20 (2), e47012. doi: 10.15252/embr.201847012
Keywords: Brown Ring Disease, Vibrio tapetis, Ruditapes philippinarum, hemocytes, type four secretion system, rounding phenotype, colonization, mutagenesis
Citation: Rahmani A, Delavat F, Lambert C, Le Goic N, Dabas E, Paillard C and Pichereau V (2021) Implication of the Type IV Secretion System in the Pathogenicity of Vibrio tapetis, the Etiological Agent of Brown Ring Disease Affecting the Manila Clam Ruditapes philippinarum. Front. Cell. Infect. Microbiol. 11:634427. doi: 10.3389/fcimb.2021.634427
Received: 03 December 2020; Accepted: 23 March 2021;
Published: 29 April 2021.
Edited by:
William D. Picking, University of Kansas, United StatesReviewed by:
Anastasia D. Gazi, Institut Pasteur, FranceMichael John Calcutt, University of Missouri, United States
Copyright © 2021 Rahmani, Delavat, Lambert, Le Goic, Dabas, Paillard and Pichereau. This is an open-access article distributed under the terms of the Creative Commons Attribution License (CC BY). The use, distribution or reproduction in other forums is permitted, provided the original author(s) and the copyright owner(s) are credited and that the original publication in this journal is cited, in accordance with accepted academic practice. No use, distribution or reproduction is permitted which does not comply with these terms.
*Correspondence: Vianney Pichereau, dmlhbm5leS5waWNoZXJlYXVAdW5pdi1icmVzdC5mcg==; Alexandra Rahmani, YWxleGFuZHJhLnJhaG1hbmlAdW5pdi1icmVzdC5mcg==; Christine Paillard, Y2hyaXN0aW5lLnBhaWxsYXJkQHVuaXYtYnJlc3QuZnI=
†These authors have contributed equally to this work