- 1Department of Biotechnology, National Institute of Pharmaceutical Education and Research, Mohali, India
- 2Cellular Immunology and Experimental Therapeutics Laboratory, Department of Zoology, West Bengal State University, Barasat, India
Glutamine synthetase (GS) is one of the most important metabolic enzymes which catalyzes ligation of glutamate and ammonia to form glutamine. Previous studies from our lab had revealed significant differences in parasite and host GS enzyme which warranted us to further work on its relevance in parasite. To analyze glutamine synthetase function in Leishmania, we generated GS overexpressors and knockout mutants and evaluated their ability to grow in vitro in monocyte differentiated macrophage and in vivo by infections in BALB/c mice. GS knocked out strain showed significant growth retardation with delayed cell cycle progression and morphological alteration. Null mutants exhibited attenuated infectivity both in in vitro and in vivo experiments and the effect was reverted back when infected with GS complemented parasites. This indicated that the alterations in phenotype observed were indeed due to GS knockout. GS knockout also made the parasite increasingly sensitive to Miltefosine. Detailed investigation of mode of parasite death upon Miltefosine treatment by dual staining with Annexin-V conjugated FITC and propidium iodide, pointed towards apoptotic or necrotic mode of cell death. This is the first report to confirm that GS is essential for the survivability and infectivity of Leishmania donovani, and can be exploited as a potential drug-target.
Introduction
Leishmaniasis is a major worldwide public health problem, with approximately 0.7 to 1.0 million new cases yearly and mortalities of around 20,000 to 30,000 annually (Burza et al., 2018; Valero and Uriarte, 2020). Visceral leishmaniasis (VL) is the most fatal form of leishmaniasis, caused by Leishmania donovani and Leishmania infantum. (Ready, 2014; Torres-Guerrero et al., 2017). VL is difficult to eradicate from the endemic areas as the vector control and chemotherapy have several limitations. The presently available treatment options are costly, have less efficacy, toxic to humans, have complex administration regimes and are faced with the challenge of resistance. (Uliana et al., 2018). Hence, it is necessary to look out for novel potential molecular or biochemical markers and design more specific and effective inhibitors based on the knowledge of molecular mechanisms employed by the parasite for its survival.
Glutamine synthetase (GS) is a key metabolic pathway enzyme that uses substrate glutamate and ATP to generate glutamine. Glutamine is a part of several metabolic processes which promote cell growth and proliferation. It also helps in the maintenance of acid-base homeostasis. Glutamine has been found to be important in cellular signaling pathways. It also supports infectivity of various pathogens (Newsholme et al., 2003; Haber et al., 2017; Cruzat et al., 2018). GS and its catalytic product glutamine are involved in regulation of several different enzymes such as tryptophan transaminase, asparagine synthetase, glucosamine synthetase, carbamoyl-phosphate synthetase, glutaminyl-tRNA synthetase, glutamate dehydrogenase and ornithine decarboxylase (Fisher, 1999; Burbaeva et al., 2005; Forde and Lea, 2007). In bacteria Mycobacterium tuberculosis and protozoan parasite Plasmodium falciparum, in silico methods identified GS as a viable drug target (Fatumo et al., 2009; Crowther et al., 2010). GS is essential for replication and survival of intracellular forms of Trypanosoma cruzi (Crispim et al., 2018). In Leishmania chagasi, GS stimulates the proliferation of T cells which results in elevation of interferon gamma (Martins et al., 2006). Inhibition of either TCA cycle or glutamine synthetase strongly inhibited amastigote growth and viability in vitro and in infected macrophages in Leishmania mexicana (Saunders et al., 2014). GS acts as a moonlighting protein in Bacillus subtilis and is involved in gene regulation in addition to its enzymatic function (Fisher, 1999). GS is functionally important in many pathogens and thus its viability as a drug target has been extensively explored. Implication of glutamine synthetase in pathogenesis and several metabolic processes makes it an attractive target for drug discovery.
L. donovani glutamine synthetase (LdGS) exists as a single copy gene and the enzyme is expressed in promastigotes as well as in amastigote form (Kumar et al., 2017). Earlier studies from our lab had identified LdGS as potential drug target for VL infections based on structural differences between the host and parasite enzyme indicating possibility of target based drug designing (Kumar et al., 2020). We had also reported the antileishmanial efficacy of methionine sulfoximine (MSO) and phosphinothricin (PPT, Glufosinate) which are well-known glutamine synthetase inhibitors (Kumar et al., 2017).
The current study focuses to explore the essentiality of the GS gene for parasite survival and infectivity. For this we performed targeted gene replacement of LdGS to generate knockout strains and also generated GS overexpression strains. We report that LdGS is vital to growth, in vitro and in vivo parasite infectivity and in cell cycle progression. Apart from this, deletion of GS from the parasite resulted in increased sensitivity to standard antileishmanial drug, miltefosine. The current study validates GS as an enzyme essential for parasite. Hence, targeting this important enzyme could be a promising approach for developing much-needed new inhibitor aimed at controlling Leishmania infection.
Materials and Methods
Materials
Gel extraction and plasmid isolation kits, RPMI 1640 medium without L-glutamine, ampicillin, phleomycin, streptomycin sulfate, Anti-Rabbit IgG antibody conjugated with alkaline phophatase were procured from Sigma-Aldrich, St. Louis, MO, USA. Rabbit was used for raising the customized antibody-polyclonal anti-LdGS (Abgenex, Bhubaneswar, India). Hygromycin B and Geneticin (HiMedia), propidium iodide, Pure Link genomic DNA and RNA isolation Kit (Invitrogen™), Annexin V-FITC apoptosis detection kit (Roche) and Poly-L-lysine coated coverslips was from Corning® BioCoat™. The other chemicals used in the study were available commercially and were of analytical grade.
Plasmids and Strains
L. donovani wild type (MHOM/IN/80/Dd8) promastigotes were used in the present study. Vector psp72αhygroα was a kind gift from Prof. Rentala Madhubala, Jawaharlal Nehru University, New Delhi, India. Leishmania specific expression vectors (pXG B3318, pXG B1288 and pXG B3324), were kindly provided by Dr. Stephen M. Beverly, Washington University Medical School, USA. These vectors were used for making knockout and complementation constructs.
Culture Conditions of Parasite and Mammalian Cells
The promastigotes were grown in RPMI-1640 (pH-7.2) media supplemented with 10% Fetal Bovine Serum. It contained antibiotics- penicillin G, Streptomycin, Gentamycin (100 µg/ml each) and 0.2% sodium bicarbonate. They were cultured at 24°C. The monocytes THP-1 were maintained at 37°C, 5% CO2 environment in the same media.
For selection of the knockout, overexpressor and vector control, parasites were grown in complete RPMI media supplemented with respective antibiotics, i.e. Geneticin G418 (80 μg/ml), Hygromycin B (100 μg/ml) and Phleomycin (25 μg/ml). THP-1 monocytes were also maintained in RPMI 1640 media supplemented with 10% FBS in the humidified atmosphere with 5% CO2 and constant temperature of 37°C.
Generation of Molecular Constructs for GS Gene Overexpression, Knockout and Complementation Studies
LdGS open reading frame (Accession No. KT907048) was cloned in Leishmania specific episomal vector psp72αhygroα between XbaI and HindIII restriction site using gene specific primers H and I as mentioned in (Table S1). Positive clones were confirmed by colony PCR and double digestion of clones. The construct generated was verified by automated DNA sequencing (1st BASE, Axil Scientific Pvt. Ltd, Singapore).
Knockout constructs were generated by cloning the upstream (5′ UTR of ~737 bp) and downstream (3′ UTR of ~785 bp) flanking regions of LdGS present on chromosome number 6. The regions upstream and downstream of the gene were selected and specific primers were designed for the amplification of selected regions. The sequences selected as UTR regions are provided in Supplementary Figure S1. The vectors used for the overexpression and knockout studies are provided in Supplementary Figure S2. The single knockout construct (SKO) was generated by cloning 5′ UTR and 3′ UTR regions in pXG Hygro vector. The restriction enzyme site of NheI and XhoI was selected for cloning of 5′ UTR-LdGS upstream of hygromycin resistance cassette using primers A and C (as mentioned in Supplementary Table S1) to generate 5′ UTR-LdGS-pXG Hygro construct. The confirmed construct (5′ UTR LdGS-pXG Hygro) was further used to clone ~785 bp of 3′ UTR LdGS using primers D and E (as mentioned in Supplementary Table S1) in between restriction sites of NsiI and BamHI respectively to generate SKO construct. The double knockout construct (DKO) was generated using pXG-Neo vector. The primers used to amplify the 5′ UTR LdGS had SalI and XhoI restriction sites. For 3′ UTR, the restriction sites were NsiI and BamHI for cloning in pXG-Neo vector to generate complete DKO construct.
To restore LdGS in the knock out parasites complementation construct was generated, the LdGS gene was amplified using sense and antisense primers F and G respectively (Supplementary Table S1) with BstEII restriction enzyme site. The gene was cloned into pXG Phleo vector. To confirm the orientation and sequence, the constructs were subjected to automated sequencing.
Generation of Genetically Manipulated Parasites
L. donovani GS knockout parasite was generated by transfection of all the constructs with a Bio-Rad Gene Pulsar apparatus and conditions used to electroporate the parasite were at 443 V, 500 µF capacitance, ∞ ohm resistance and 16 ms time constant (Cruz et al., 1991). The harvested cells were washed with PBSG buffer twice. This buffer consisted of 10 mM NaH2PO4, 10 mM Na2HPO4, 145 mM NaCl and 2% glucose. The cells were washed with electroporation buffer containing 21 mM HEPES, 137 mM NaCl, 5 mM KCl, 0.7 mM Na2HPO4, 6 mM glucose. The final resuspension of cells was done in 400μl electroporation buffer to which about ~12 µg of gene replacement SKO cassette linearized with NheI and BamHI (5′UTR LdGS-pXG Hygro-3′UTR) was added along with the cells in the cuvette (2mm). It was kept in ice for 10 minutes and then electroporated. The cells were transferred to 5 ml of RPMI medium with 20% FBS and maintained at 24°C. Antibiotics were added 48 h post transfection for selection of transgenic parasites expressing respective antibiotic resistance gene (Dolai et al., 2008).
LdGS heterozygous mutant (LdGS+/−) were maintained in 100 μg/ml of hygromycin B. The LdGS heterozygous mutant was transfected with LdGS-pXG Phleo vector for episomal expression of GS gene to generate LdGS complementation mutant (LdGS+/−/+) which were maintained in 100 μg/ml of hygromycin along with 25 μg/ml of phleomycin. The LdGS(+/−) and LdGS(+/−/+) mutants were selected for the second round of transfection with BamHI linearized DKO construct 5′ UTR-LdGS-Neo-3′-UTR to replace second allele for null mutant (LdGS−/−) and add back mutant generation (LdGS−/−/+). LdGS(−/−) mutants were maintained in presence of 100 μg/ml of hygromycin and 50 μg/ml of G148 (Genetecin). Add back null mutants (LdGS−/−/+) were maintained in 50 μg/ml of G148 (Genetecin), 100 μg/ml of hygromycin B and 25 μg/ml of phleomycin. The strategy for GS gene knockout is illustrated in Supplementary Figure S3A. GS null mutant failed to grow in media deprived with L-glutamine and could grow only when transferred to RPMI complete media supplemented with L-glutamine.
For overexpression of GS in Leishmania promastigotes (LdGS++/++) transfection of the ~10 µg LdGS psp72αhygroα construct was done through electroporation by same protocol. After selection, the mutant parasites were maintained under constant selection pressure of suitable antibiotic.
Genotypic Confirmation of GS Knockout by PCR
Knockout strains were confirmed by isolating genomic DNA from wild type and all mutant transgenic parasites and PCR based strategy was employed to show that the gene replacement cassettes had integrated in the genome of the parasite. The primers used are mentioned in Supplementary Table S2 and primer orientation is schematically represented in Supplementary Figure S3B. Correct integration of the hygror or neor gene cassette in heterozygous and null mutant strains was confirmed by PCR of genomic DNA using combination of Neo or Hygro specific sense and antisense primer and the primers for 5′ or 3′ untranslated regions were also used. The genomic DNA of the mutant parasites was subjected to PCR to confirm the presence of the following events: presence of hygror gene, neor gene, knockout of LdGS, using primer combination of P3 + P4, P5 + P6, P1 + P8 and P7 + P2. While integration of 5′UTR to hygror gene, 3′UTR integration to hygror gene, 5′UTR integration to neor gene, integration neor gene and 3′UTR, phleor in episomal construct was confirmed by using primer combination of P1 + P4, P3 + P2, P1 + P6, P5 + P2 and P9 + P10, respectively in separate reaction using genomic DNA as template from all mutant strains keeping wild type genomic DNA as control. Confirmation of complementation and replacement of LdGS gene was also done by PCR.
Reverse Transcriptase PCR of GS Knockouts
The total RNA from wild type and mutant parasites was isolated using Pure Link RNA isolation kit (Invitrogen) and Prime Script™ one step RT-PCR Kit was used to perform RT-PCR. Primers used to perform RT-PCR are represented in Supplementary Table S3. To remove DNA contamination, the RNA was treated with DNAse I enzyme (Thermo Scientific). The expression of LdGS in wild type parasites was considered as the reference for normalization and Ld-actin was taken as control.
Preparation of Crude Lysate and Determination of GS Enzyme Activity
The promastigotes were centrifuged at 3000g and the pellet was washed with PBS (pH -7.4) Cells were resuspended in lysis buffer (20 mM Tris (pH 7.8), 1 mM PMSF, 0.5 µg/ml each of aprotinin and leupeptin). The cells were lysed using repeated freeze-thawing (Padmanabhan et al., 2005). The lysate was centrifuged at 13,000g, 30 min at 4°C. The protein was estimated using Bicinchoninic acid method (BCA) taking BSA (bovine serum albumin) as standard (Smith et al., 1985). To determine the specific activity and to perform western blot, the above cell lysate was used. LdGS enzyme activity was performed by inorganic phosphate (Pi) determination method (Gawronski and Benson, 2004).
Briefly, for measuring the enzyme activity of GS in crude lysate, the reaction mixture containing 5 mM MgCl2, 5 mM NH4Cl, 3 mM ATP and 20 mM L-glutamate in 20 mM Tris-HCl (pH 7.8) and 10 μg of lysate was kept for 20 min at temperature of 37°C. Reaction mixture without crude cell lysate was taken as blank. Blue colored phosphomolybdate complex formation was recorded at 655 nm according to the protocol described previously. Percentage change in specific enzyme activity of all mutant strains were calculated keeping wild type enzyme activity as control.
Immunoblotting of LdGS
The total cell protein was quantified by bicinchoninic acid method and 75 µg protein was run on 10% SDS PAGE and the resolved protein was transferred onto nitrocellulose membrane and blocked with 5% BSA and the membrane was probed with anti-LdGS antibody (1:4000 dilution). The membrane was further incubated with anti-rabbit alkaline phosphatase conjugated secondary antibody (1:7500). The visualization was done using BCIP and NBT (Sigma) as substrate and densitometric analysis was carried out by using Image J software. Alpha tubulin was taken as endogenous control.
Determination of Growth Rate and Infectivity
To analyse growth rate, 1 × 106 cells/ml stationary phase cells were inoculated in 25 cm2 flasks containing RPMI complete medium without addition of particular antibiotic. Cells were maintained at 24°C and cell count of the flask were analyzed at fixed time interval of 24 h by cell counting using Neubauer hemocytometer under a compound microscope for a period of 5 days.
The THP-1 monocyte cells were differentiated into adherent macrophages using phorbol 12-myristate 13-acetate (PMA). Infection was performed taking the ratio of 1:10 (macrophage: parasites) of cells. Infection of stationary stage parasites (WT and mutant) was done for 24 hours to perform parasite rescue transformation assay (Jain et al., 2012). 2×105 THP-1 cells were plated per well of a 96 well plate and differentiated using PMA at 37°C in CO2 incubator with 5% CO2 for 48 h. The adhered monocyte derived macrophage cells were infected with parasite as mentioned above. The non-internalized promastigotes were removed and infected adhered macrophages was washed with serum free media. After cell lysis, RPMI-1640 complete medium was added to each wells and kept at 24°C for 48 h so that the promastigotes would be transformed from amastigotes. Finally, MTT assay was performed as per the previous protocol and absorbance was recorded at 540 nm (Mosmann, 1983). The percentage infectivity was calculated relative to wild type.
Cell Cycle and Scanning Electron Microscopy (SEM) Analysis
L. donovani promastigotes were synchronized using hydroxyurea as per the protocol described earlier with minor modification (Pal et al., 2017). Briefly, 4×106 cells/ml late exponential phase promastigotes were incubated in serum-deprived RPMI-1640 medium containing 100 µg/ml hydroxyurea (HU) for 8 h. After 8 h of cell synchronization, hydroxyurea was removed by low speed centrifugation at 1300g and washed with serum-free media and cultured in RPMI-1640 media (10% FBS) for 12 h and then washed with ice cold PBS. The pellet was resuspended by adding PBS, 300 μl followed by addition of 700 μl of chilled ethanol. and incubated at 20°C overnight. Ethanol was removed by centrifugation at 1300g and cells were washed with ice cold PBS. Next, samples were incubated for 15 min on ice with 500 µl solution containing 0.05% Triton X-100 and 100 μg/ml RNase A prepared in PBS. Propidium iodide (50 μg/ml) was used to stain the cells for 30 minutes and subjected to flow cytometry on FACS Aria Fusion (BD Biosciences)… At last, collection of 20,000 events, separately for each sample was done. The distribution of cells thus obtained were scrutinized using Flow Jo software. This was used to determine the percentage cells in each phase of cell cycle- G0/G1, S and G2/M.
To observe the effect of GS overexpression and deletion on the parasite morphology, SEM was used. 1x 106 promastigote parasites were harvested and washed with ice-cold PBS. Cells were fixed in 2.5% glutaraldehyde (EM-grade glutaraldehyde, Sigma-Aldrich) containing 4% (w/v) paraformaldehyde in 0.1 M phosphate buffer. Cell suspension was added on L-lysine-coated coverslips (Corning® BioCoat™) and incubated for 6 h at room temperature. Unadhered cells were removed and coverslip was washed with sterile filtered water to remove any buffer salts. To dehydrate, graded series of ethanol (v/v) 30%, 50%, 70%, and 90% were added in ascending order. The drying of samples was done at critical point, followed by their mounting on metallic stubs using bioadhesive carbon tape. Sputter coating of gold and palladium was done. The scanning electron microscope imaging was done using S-3400N,(Hitachi, Japan) (Gluenz et al., 2015; Soumya et al., 2017).
Drug Sensitivity Assays
MTT (3-(4,5-dimethylthiazol-2-yl)-2,5-diphenyl tetrazolium bromide) assay was performed to assess the drug susceptibility for Miltefosine (standard anti-leishmanial drug) in wild type parasite and GS mutants (Mosmann, 1983). In a 96-well plate, 2×105 cells were plated in each well followed by 48 h incubation at 24°C. Then, 10-100 µM of Miltefosine was added and incubated for 48h followed by addition of 20 µl MTT reagent of concentration 5mg/ml. The plate was incubated for 4 h at 37°C and then centrifuged at 3000g, 10 min. After the removal of supernatant, 100 µl of 100% DMSO was used to dissolve the formazan crystal. The absorbance was measured at 540 nm. The untreated cells were taken as control and IC50 value was calculated relative to it.
Annexin V-FITC/PI Dual Staining for Determination of Apoptosis or Necrosis
In the event of early apoptosis, the phospholipids on the cell membrane are altered which leads to exposed phosphatidylserine on the surface of cell. In normal parasites, phosphatidylserine are not exposed, while in cells undergoing apoptosis phosphatidylserine are exposed to the cell surface (Mukherjee et al., 2018). 1 x 106 cells/ml were plated in each well of a 24 well plate and kept at 24°C for 24 h. The late apoptotic cells bind to both AnnexinV/FITC and PI. The double positive population contains necrotic cells and can also have late apoptotic cells. Also, PI single positive events may represent pure nucleic acid aggregates. The promastigotes were treated with miltefosine and to detect apoptosis, AnnexinV-FLUOS kit (Roche) was used following the manufactures protocol. They were analysed using flow cytometry. All the knockout strains along with wild type cells (1 x 106/ml) were seeded in 24 well plate and incubated at 24°C. After 24 h, untreated cell lines along with cells treated with IC50 concentration of miltefosine and N-acetyl cysteine preincubated cells treated with IC50 concentration of miltefosine were washed with cold PBS (pH 7.4) at 1300g. The resulting pellets were resuspended in 500 μl of staining buffer and incubated with annexin V-FITC first for 10 min followed by addition of PI. Cultures incubated at 45°C for 2 h were used as positive death controls. Prior to being subjected to flow cytometry, the samples were kept in ice (in dark) for 15 minutes and analyzed by flow cytometry (FACS Aria™ Fusion, Becton Dickinson) with BD FACS Diva™ software. Argon laser (excitation wavelength 488 nm) and 561 nm yellow-green laser was used for detection of Annexin V-FITC stained (early apoptotic) cells in (FITC; 530/30 band pass) filter and PI stained (late apoptotic or necrotic) cells in (PE/PI; 582/15 band pass) filter. 30 000 events in total had been taken for analysis. FITC log (x axis, FITC-fluorescence) versus PI/PE log (y axis, PI) were used for data acquisition. Data was analysed by FlowJo software, Ver. 10.3.1.
Determination of Parasite Infectivity by the Mutant Parasites, In Vivo
For this experiment, male BALB/c mice were procured from Imgenex India, Bhubaneswar. They were kept at 25°C ± 2°C and subjected to a 12 h light dark cycle. They were fed ad libitum (Mukherjee et al., 2020). The experimental groups (5 animals in each group; 4-6 weeks age; weighing approximately 20g) were infected, i.v., with 2 × 107 promastigotes of LdGS(+/+), LdGS(+/−), LdGS(+/−/+), LdGS(−/−), LdGS(−/−/+), LdGS(++/++), and Ld: psp (Vc). Four-week post-infected animals were sacrificed, amastigotes were calculated from the spleen and liver Giemsa-micrographs under phase contrast microscope (Zeiss Axioscope), and parasite burden was extrapolated using Stauber’s formula as described earlier (Dey et al., 2020). The survival percentage of promastigotes maintained under specific antibiotic surveillance was monitored in the flow cytometer (BD FACS Verse) using 7-Aminoactinomycin D (7-AAD) before in vivo infection (Sultana et al., 2018).
As we have focused on the capacity of proliferation and survivability of LdGS(−/−) mutants’ inside the host cells, the time dependent infectivity of LdGS(−/−) parasites was investigated and compared with the LdGS(+/+), in vivo, at day 15 and day 30 post infection. The mRNA was extracted from the spleens of the infected animals and converted to cDNA. The expression of kinetoplast DNA (kDNA) was evaluated by semi-quantitative PCR (L. donovani kDNA; Forward: 5′-AAATCGGCTCCGAGGCGGGAAAC-3′ and Reverse: 5′- GGTACACTCTATCAGTAGCAC-3′), normalized against equal expression of house-keeping gene GAPDH (murine GAPDH; Forward: 5´-GAGCCAAACGGGTCATCATC-3´ and Reverse: 5´-CCTGCTTCACCACCTTCT TG-3´) (Dey et al., 2020). The densitometric quantification was analyzed by Image Lab software and represented as mean ± SE from 3 animals per group. The differences in means among various groups were considered significant as per the one-way analysis of variance using GraphPad Prism v 6.0 software, *p < 0.038 and **p < 0.032.
Ethics Statement
The experiments involving animals had been approved by the institutional Animal Ethics Committee, WBSU, Barasat [File no. WBSU/IAEC/2019-20/8(CP), dated 20.05.2019]. IAEC registration no. 1394/GO/Re/S/10/CPCSEA, dated 09/12/2016). The institutional guidelines for animals had been followed in performing this study.
Statistical Analysis
The data obtained from indicated number of experiments has been interpretated as mean ± S.D. values. Student’s unpaired t-test was performed in Graph-Pad Prism software (GraphPad, San Diego, CA, USA) for statistical data analysis. The data that showed differences from groups were verified by ANOVA and p values of ≤ 0.05 were considered statistically significant. The levels of statistical significance were indicated as follows: *p ≤ 0.05, **p<0.01, ***p<0.001, **** p ≤ 0.0001 whereas for in vivo animal experimental data analysis only p value of ≤ 0.05 were considered significant.
Results
Generation of LdGS-psp72αhygroα Construct for Overexpression of LdGS in L. donovan is (LdGS++/++) and Functional Characterization of GS Overexpressors
GS overexpressors were generated as described in methodology section. Western blot analysis of whole cell lysate indicated ~1.9 fold increased GS expression in LdGS overexpressor [LdGS(++/++)] compared to wild type [LdGS(+/+)] and Ld: psp vector control (Vc). A poncaeu stained blot was kept as the loading control (Figures 1A, B). The ~1.87 fold higher enzyme specific activity by LdGS(++/++) over LdGS(+/+) and Vc confirmed the GS overexpression in the parasites (Figure 1C).
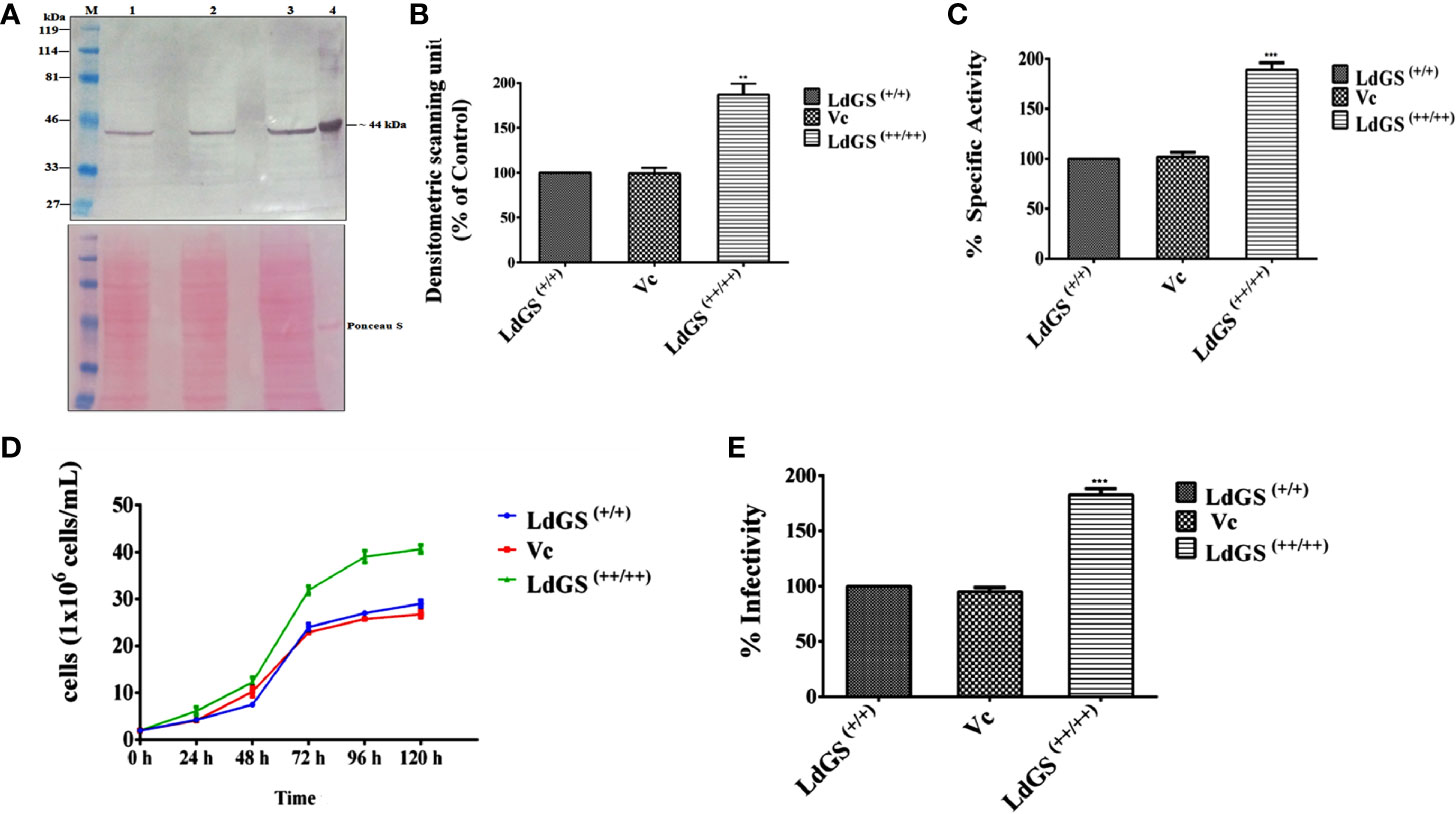
Figure 1 Confirmation of LdGS overexpressor (LdGS++/++) (A) Confirmation of LdGS overexpression by western blotting. Top panel; Lane M: prestained broad range marker (Bio-Rad); Lane 1: crude cell extract of Wild type (WT) promastigote; Lane 2: crude cell extract of vector control (Vc); Lane 3: crude cell extract of GS overexpressor (LdGS++/++); Lane 4: purified recombinant LdGS protein. Bottom panel; The membrane is stained using Ponceau-S as a loading control; (B) The bar graph represents the densitometric analysis of the western blot represented in A; (C) Percentage GS activity was measured in total cell lysate Vc (Ld:psp) and LdGS(++/++) compared to wild-type LdGS(+/+), (D) Effect of LdGS overexpression on parasite growth rate. Analysis of the growth rates of wild type, psp and overexpression promastigotes. (E) Influence of GS gene overexpression on the infectivity of L. donovani parasites in vitro. Data represents mean ± SD of three independent experiments. p values of ≤ 0.05 were considered statistically significant and levels of statistical significance were indicated as follows: **p ≤ 0.01 and ***p ≤ 0.001.
The growth of wild type [LdGS(+/+)], Ld: psp vector control (Vc) and LdGS overexpressor [LdGS(++/++)] promastigotes were followed over five days. Counting of viable cells was done to monitor the growth rate of all transgenic cell lines. (Figure 1D). At 48 h, the parasite cell number was found to be 4 x 106 in both the LdGS(+/+) and Vc however, LdGS(++/++) showed 6 x 106 parasites. At 72 h, the cell number was found to be 24 x 106, 22 x 106 and 31 x 106 respectively for the parasites. At 96 h, the LdGS(++/++) reached a cell density of 41 x106 cells/ml whereas, LdGS(+/+) and Vc reached at a cell density of 29 x106 cells/ml and 27.5 x106 cells/mL respectively. No difference in growth was observed in LdGS(+/+) and Vc parasites up to 120 h. It was clearly observed that though there was no change in growth of LdGS(+/+) and Vc parasites yet the GS overexpressing parasites exhibited higher growth rate compared to the control parasite. The replication rate was observed higher in case of LdGS(++/++) promastigotes, indicating the involvement of GS in growth of Leishmania, at least, in vitro.
Next, to check the consequences of LdGS gene overexpression on robustness of the over expressors in infecting host macrophages in vitro, the parasite rescue and transformation assay was performed with wild type [LdGS(++)], vector control (Vc) and LdGS overexpressor [LdGS(++/++)] promastigote parasites. Phorbol-12-myristate-13-acetate (PMA) differentiated THP-1 cells were infected with wild type, vector control and GS overexpressor promastigotes for 24 h. Non internalized cells were removed and infected macrophages were washed with serum free media. Infected monocyte differentiated macrophage cells were lysed and incubated in complete media for 48 h at 24°C for performing the transformation assay. It was observed that there was no change in the intracellular survival of amastigotes in case of LdGS(++) and Vc. However, LdGS(++/++) parasites exhibited almost ~ 1.8 fold enhanced replication of intracellular amastigotes indicating the role of LdGS in facilitating parasite replication within macrophages (Figure 1E). Thus, LdGS overexpressor parasites exhibited higher infectivity rate to macrophages compared to wild type and vector control parasite. The result clearly indicated that GS affects infective ability of parasites.
Nutritional Assessment of the L. donovani GS Mutant
The amino acid glutamine is an important component of several biochemical pathways such as protein synthesis. After glucose, it is also a source of energy for the cell. Comparing the growth rate of all knockout strains it was found that GS null mutant failed to grow in absence of glutamine, while growth rate of wild type and complementation mutant parasites was almost similar in glutamine or glutamine deficient media (Supplementary Figure S4). Add back null mutant growth rate was also very slow in glutamine deficient media. Indeed, when the mutant cells were transferred to glutamine supplemented media, even though impairment in in vitro growth was observed in case of null mutants but we were able to get sufficient cells to functionally characterize the role of GS gene in parasite.
Genotypic Characterization of LdGS Knockout Strains
The genotype of GS knockout strains was confirmed by performing PCR using different sets of primers (Supplementary Table S2). The template used in the PCR analysis was the genomic DNA of WT parasites with combination of Hygro, Neo and LdGS gene specific primers to show the absence of hygror and neor gene in wild type genomic DNA. Primers 1 and 4 amplified ~2189 bp region of 5′UTR GS and hygror gene while primers 2 and 3 covered the region of 3′UTR and hygror gene with an amplified product of length ~2961 bp from genomic DNA of all mutant promastigotes. No amplified product was obtained from wild type genomic DNA using same set of primer combination which confirmed the integration of the hygromycin cassette in LdGS(+/−). Primers 1 and 6 encompassing 5′UTR and neomycin resistance gene internal region gave an amplified product of ~2001 bp size. Similarly, integration of neor gene to 3′UTR LdGS was confirmed using primers 2 and 5 which gives an amplified product of ~2775 bp size with LdGS(−/−) and LdGS(−/−/+) genomic DNA, while no amplified product was observed with wild type and LdGS(+/−/+) genomic DNA. This confirmed the replacement of second allele of GS gene by neomycin resistance cassette in LdGS(+/−). PCR analysis of the double antibiotic resistant strain with primers 1 and 8 along with 2 and 7 gave no amplified product with GS null mutant, while wild type genomic DNA showed amplified product of ~1,559 bp and ~ 1,500 bp respectively. This revealed that both hygro and neo cassettes replaced both the alleles of GS gene and also got integrated at the appropriate locus [Figure 2A (I–IX)]. P3 (Hygro sense) and P4 (Hygro antisense) pair of primer combination was used to show the presence of hygror gene in LdGS (all GS mutants except wild type) while other set P5 +P6 (Neo sense + neo antisense) combination was used to show the presence of Neo gene in null and add back null mutant cell line. A band of ~538 bp was obtained using primers P9 and P10 which are phleo specific internal primers thus confirming the presence of episomal LdGS-pXG Phleo in the add-back null mutant cell line (Figure 2A X).
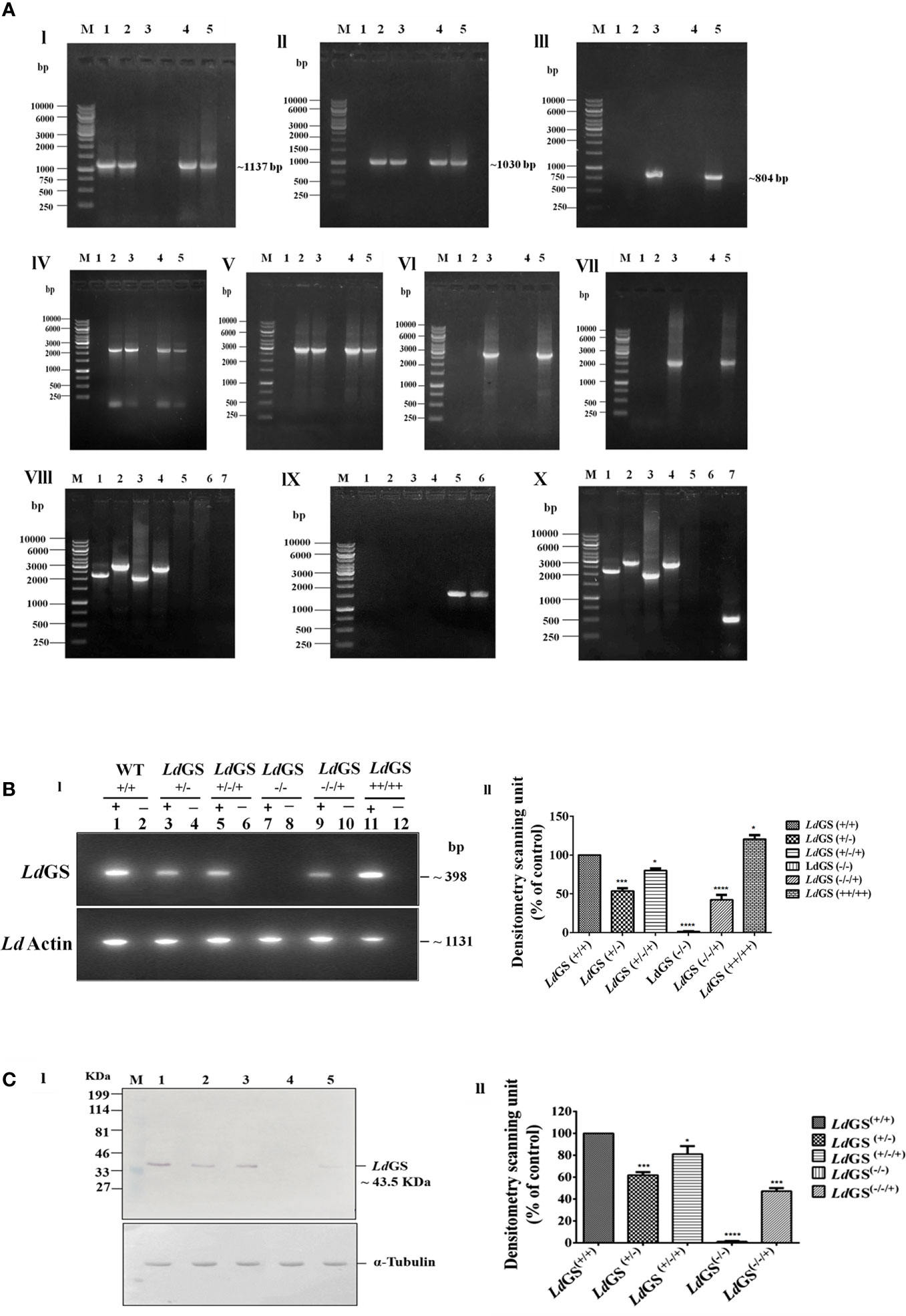
Figure 2 Confirmation of GS knockout. (A) Genotypic analysis of genetically manipulated promastigotes by PCR. Template for PCR analysis were the genomic DNA of the following: 1. wild type, LdGS(+/+); 2. heterozygous mutant, LdGS(+/−); 3. null mutant, LdGS(−/−); 4. complementation mutant, LdGS(+/−/+); 5. add back null mutant, LdGS(−/−/+) (I) Primers encompassing complete LdGS ORF was used in the amplification of GS gene (~1137 bp) (II) The amplified product of primer P3+P4 to show presence of hygromycin resistance gene (~1030 bp) (III) primer P5+P6 to show presence of neomycin resistance gene (~804 bp) (IV)) primer P1+P4 to show integration of 5′ flanking sequence to hygromycin gene(~2189 bp). (V) primer P2+P3 to show integration of hygromycin to 3′ flanking sequence (~2961 bp). (VI) primer P1+P6 to show integration of 5′ flanking sequence to neomycin gene (~2001 bp) (VII) primer P2+P5 to show integration of neomycin to 3′ flanking sequence (~2775 bp). (VIII) Genomic DNA from GS null mutant cells were taken as PCR template. Internal primers specific to hygro, neo and wild type were used to validate the recombination. The specificity of recombination event was checked with Hygro, Neo and LdGS (WT) gene specific internal primers. M: indicates the DNA molecular size marker in kb. Lane 1: amplified product of P1+P4; Lane 2: amplified product of P3+P2; Lane 3: amplified product of P1+P6; Lane 4: amplified product of P5+P2; Lane 5: amplified product of P1+P8 (5′UTR-LdGS sense and LdGS internal antisense primer); Lane 6: amplified product of P7+P2 LdGS internal sense and 3′UTR-LdGS antisense primer; Lane 7: negative control without DNA template (IX) Genomic DNA from wild type promastigotes was used as a template for PCR analysis with Hygro, Neo and LdGS gene specific primer. M: denotes 1 Kb DNA ladder. molecular size marker in kb. Lane 1: amplified product of P1+P4; Lane 2: amplified product of P2+P3; Lane 3: amplified product of P1+P6 primer; Lane 4: amplified product of P2+P5; Lane 5: amplified product of P1+P8; Lane 6: amplified product of P2+P7 primer (X) PCR analysis of add back null mutant parasites using genomic DNA. To validate the recombination event, primers specific to hygro, neo, wild type and phleo were used., M: represents the DNA ladder. Lane 1-6: same set of primers in respective lane as represented in IX; Lane 7: amplified product of phleo gene to show presence of episomal construct using primer set P9+P10. (B). RT-PCR of total cell RNA from genetically manipulated parasites. (I) Expression analysis of LdGS mRNA in wild type (LdGS+/+), GS heterozygous (LdGS+/−), complementation mutant (LdGS+/−/+), null mutant (LdGS−/−), add-back null mutant (LdGS−/−/+) and LdGS overexpressor (LdGS++/++) in L. donovani promastigotes using semi-quantitative reverse transcription PCR. Internal primer specific for the gene were used.(lanes have been marked as ‘+’). The negative control reactions are marked as ‘−’. The product sizes of (~398 bp) is indicated. As an endogenous control of L. donovani Actin gene was also amplified (1131bp). The bar graph shows the relative expression of GS using densitometric analysis of each sample. The house keeping actin gene was the control. The expression of GS in wild type was consider as standard for data normalization. The significance differences in expression are marked using asterisks. Two independent experiments were performed and the erroe bars show the mean ± SD value. (C) Analysis of LdGS protein expression using immunoblots. (I) Lane M: prestained broad range marker (Bio-Rad); Equal amount of total cell proteins (100 μg) from Wild type (Lane 1), LdGS(+/−) (Lane 2), LdGS(+/−/+) (Lane 3); LdGS(−/−) (Lane 4); LdGS(−/−/+) (Lane 5) detected using anti-LdGS antibody. Alpha tubulin was used as loading control. (II) A bar graph representing the densitometry analysis of the western blot is represented in (C) Data represents mean ± SD of two independent experiments. p values of > 0.05 were considered statistically non significant and levels of statistical significance were as follows: *p ≤ 0.05, ***p ≤ 0.001 and ****p ≤ 0.0001.
Alteration in Expression of LdGS mRNA in GS Knockout Strains by Reverse Transcriptase PCR
To confirm the expression of GS at the mRNA level, gene-specific sense internal primers were designed. Amplification was seen at ~398 bp in RT-PCR using gene specific internal primer as mentioned in methodology section. Negative control for each reaction did not contain any RNA template, which was included to show that the reaction system was not contaminated (Supplementary Table S3 and Figure 2B top panel). Transcription of LdGS gene was quantified by densitometric analysis of cDNA amplified product from all transgenic strains by Image J software (Figure 2B bottom panel). The results revealed significant reduction in GS mRNA levels of LdGS(+/−) and LdGS(−/−/+) strains. LdGS(++/++) showed ~ 20% higher level of mRNA expression while LdGS(+/−) mutant showed ~45.3% reduction and LdGS(−/−/+) mutant showed significantly higher level of 57.7% reduction in level of mRNA expression compared to LdGS(+/+). Null mutant (LdGS(−/−)) showed no amplification. Reaction without RNA template also showed no amplified product.
Confirmation of GS Gene Knockout by Western Blot Analysis
To check the alteration in LdGS expression in all cell types: LdGS heterozygous mutant, LdGS complementation mutant, LdGS null mutant and LdGS add back null mutant western blot analysis was performed keeping expression of GS in wild-type as control. The NC membrane was incubated with the customized anti-GS antibody. Expression of GS in all transgenic promastigotes was observed by Western blot: wild type expressed endogenous levels of enzyme while LdGS(+/−) mutant showed reduction in GS expression by 38%, The protein expression in LdGS complementation was similar to WT cells which implies the restoration of gene expression. Alpha tubulin was taken as endogenous control (Figure 2C I). Western blot analysis confirmed the almost complete loss of GS protein expression (~98%) in LdGS null mutant promastigotes. Surprisingly, LdGS(−/−/+) add back mutant showed 67% reduction in protein expression compared to the wild type parasites (Figure 2C II).
Functional Characterization of GS Mutant Cell Lines
To confirm the level of LdGS protein expression in mutant cell line, enzyme activity was performed with crude cell lysate of the Leishmania cell lines using inorganic phosphate (Pi) determination method as discussed in methodology section. Higher specific activity of GS in the LdGS(+/+) parasites (311.2 ± 12.4 µmol.min-1.mg-1) was seen in comparison to mutants. LdGS(−/−) showed ~82% reduction in specific activity whereas LdGS(−/−/+) showed almost two fold (~46%) reduction in enzyme activity as compared to the LdGS(+/+) (Figure 3A).
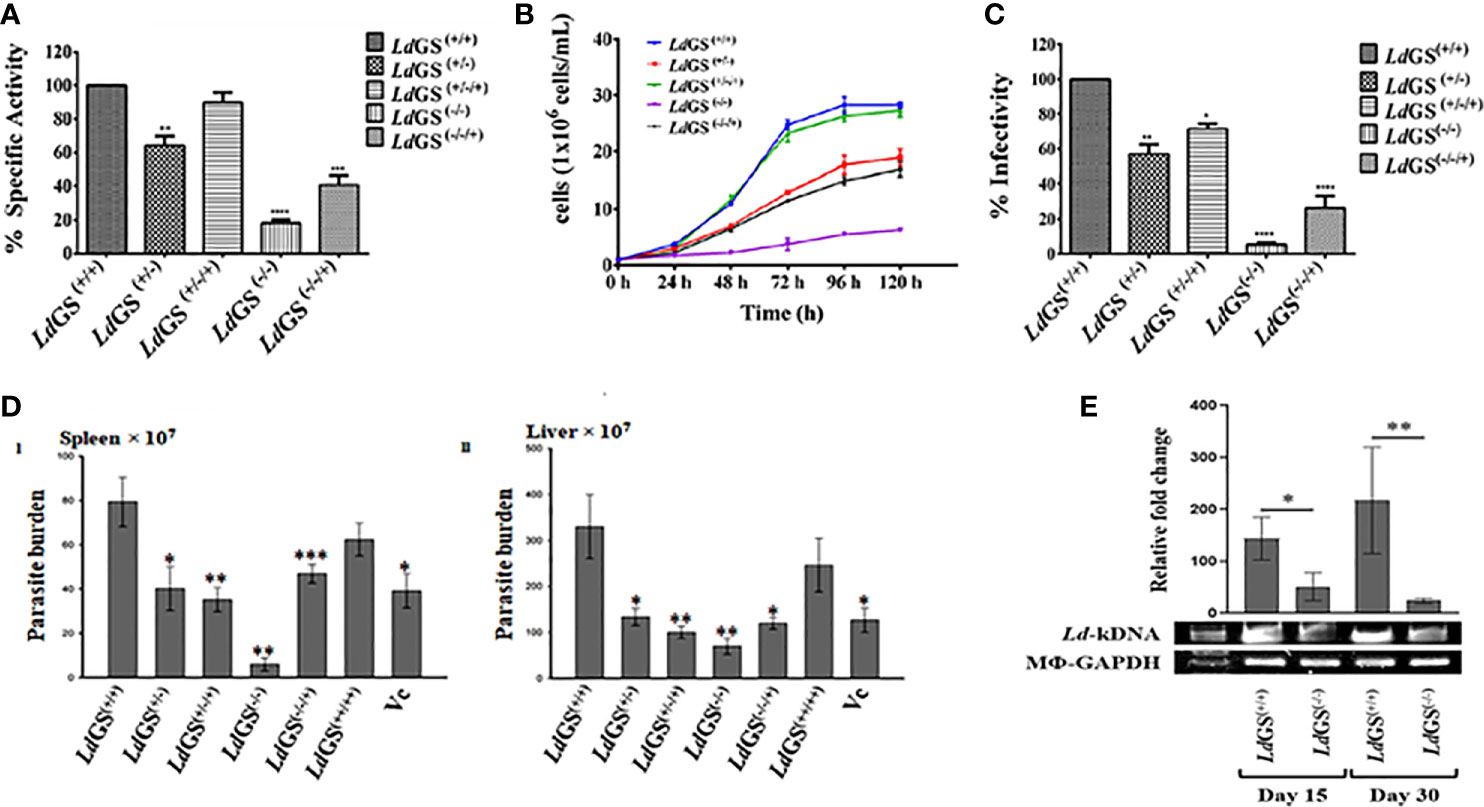
Figure 3 Characterization of knockout strains. (A) Confirmation of LdGS gene knockout strains by enzyme activity. GS enzyme activity measured in total cell lysate extracted from wild type and different GS mutant cell lines by inorganic phosphate (Pi) determination method, (B) Comparative Growth profile of wild type with GS heterozygous promastigotes, LdGS(+/−), complementation, LdGS(+/−/+), Null LdGS(−/−) and LdGS(−/−/+) add back null mutant promastigotes, (C) Effect of GS gene knockout on parasite infectivity. the percentage infectivity was analyzed for LdGS mutant cells compared to wild type promastigotes. The Data has been calculated from three independent experiments and represented as mean± SD, (D) Effect of GS gene knockout on the infectivity of L. donovani amastigotes, in vivo: Burden of parasite in spleen and liver of the infected BALB/c mice with different mutant strains of L. donovani Dd8, in vivo. Data are representative of Mean ± SE from three independent experiments with 5 animals per group (*p < 0.002, **p < 0.001, ***p < 0.009, vs WT-infected control. (E) Leishmania-kDNA expression against the normalized equal expression of Mϕ-GAPDH in spleens of LdGS(+/+)and LdGS(−/−) infected animals at day 15 and day 30, post infected. The densitometric quantification was analyzed by Image Lab software and represented as mean ± SE from 3 animals per group. The differences in means among various groups were considered significant as per the one-way analysis of variance using GraphPad Prism v 6.0 software. *p < 0.038 and **p < 0.032.
Growth curve analysis of the five populations at different selected time interval of 24 h was performed for complete five days. During exponential phase of growth at 96 h time point, LdGS(+/−) showed ~1.9 fold reduction in growth. It was observed that in case of LdGS(+/−/+) mutant the parasite numbers was almost same for all selected time point and restored to the levels comparable to that of wild type. LdGS(−/−) failed to grow in media without L-glutamine so was provided with exogenous supplementation of glutamine and showed ~7.0 fold reduction in growth rate compared to wild type cells. LdGS(−/−/+) showed compromised growth pattern but only ~2.2 fold reduction in growth was observed at 96 h time point which indicated recovery of growth (Figure 3B).
It was observed that LdGS(+/−) mutant parasites exhibited almost ~43% reduced in vitro infectivity compared to LdGS(+/+). Complete reversal of effect was not observed in LdGS(+/−/+) mutant cell line which showed ~28% reduction in infection. While, LdGS(−/−) mutant showed ~95% reduction of in vitro infectivity and replication of intracellular amastigotes compared to wild type [LdGS(+/+)] promastigotes. LdGS(−/−/+) mutant parasite showed ~73% reduction in infectivity to macrophages relative to the wild type control. These results indicated that the GS mutant parasites showed impairment in infectivity and survival inside the macrophages and the effect was reversed by complementing with GS expression (Figure 3C).
Role of GS Deletion in Parasite Infectivity In Vivo
The impact of the deletion of GS in Leishmania has been verified for the capacity of its infection, in vivo, as only amastigotes are the pathogenic morphs in mammalian hosts. LdGS(−/−) were found most incompetent in infecting the hosts. As evidenced, a reduced parasite-burden was found by 96.4 ± 3.38% (P<0.001) and 77.83 ± 3.52% (P<0.001) in the spleen and liver, respectively, in comparison to the wild type L. donovani (MHOM/IN/1980/Dd8) infection. However, the LdGS(+/−) were more infective and the proliferation restriction was found by 47.2 ± 11.69% (P<0.002) and 56.80 ± 8.36% (P<0.003) in the spleen and liver, respectively. Complete reversal of the effect was not achieved in infected animals by LdGS(+/−/+) [inhibition in the spleen by 55.5 ± 2.50% (P<0.001) and liver 67.86 ± 6.89% (P<0.001)] and LdGS(−/−/+) [inhibition in spleen by 39.67 ± 6.26% (P<0.009) and liver 62.03 ± 5.03% (P<0.002)]. The role of GS was further verified for its importance in parasite survival, in vivo, by overexpressing it in L. donovani. LdGS(++/++) were found efficient over both LdGS(−/−) and LdGS(+/−) as evidenced in comparison to the wild type parasite infection at one-month post infected animals [inhibition only by 23.5 ± 15.05% and 23.97 ± 16.21% in the spleen and liver, respectively] as -represented in (Figure 3D I and II). The presence of Leishmania-kDNA in respect to Mϕ-GAPDH determined the degree of infection, in vivo (Dey et al., 2020). Expression of kDNA further confirmed the result that LdGS(−/−) parasites had low infection rate, in vivo, as evidenced from the significantly reduced expression of Leishmania-kDNA in LdGS(−/−) infected animals in comparison to LdGS(+/+) at both 15 days and 30 days post infection against normalized equal expression of Mϕ-GAPDH (Figure 3E).
Effect of GS in Parasite Cell Cycle Regulation and Morphology
Propidium iodide (PI) was used to analyse the DNA content of the cells. The resulting distribution of cells was analyzed by FlowJo software to determine the percentage of cells in G0/G1, S and G2/M phases of the cell cycle. Result suggested that parasites synchronized in the G1/S cell cycle phase were able to proceed through cell cycle, except null mutant but percentage of cells in G2/M phases was less compared to wild type promastigotes. LdGS(+/−) mutant showed ~1.25 fold less percentage of cells whereas LdGS(+/−/+) mutant showed cell percentage in G2/M phases almost similar to LdGS(+/+). LdGS(−/−) mutants were able to complete cell division at a very slow rate and most of the cell population (~70%) was stuck to G0/G1 phase with entry to S (~ 6.1%) and G2/M phases (~17%) of the cell cycle at very low rate. Null mutant showed ~2.1 fold decreased cell population compared to wild type while add back null mutant showed ~1.31 fold decreased cell percentage as demonstrated by flow cytometric analysis (Figure 4A I and II).
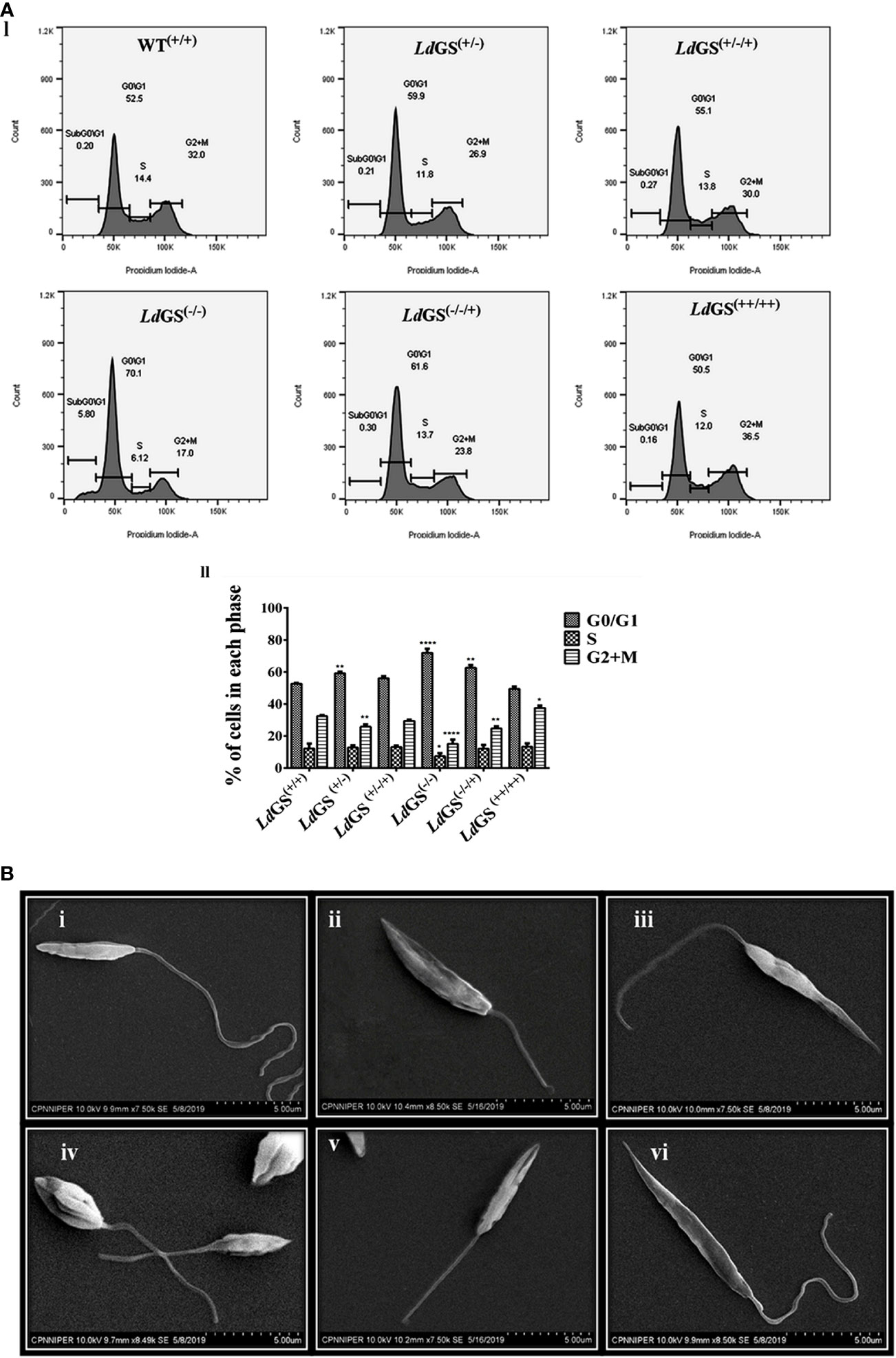
Figure 4 Role of GS in parasite cell cycle and morphology. (A) (I) Analysis of DNA content in the wild type and Knockout L. donovani parasites after cell synchronization. Fraction of cells in each phase are depicted using histograms. II. Bar graph showing the percentage of cells post synchronization and nutrient supplementation in G0/G1, S and G2/M phases of the cell cycle for wild type and all GS mutant promastigote cell lines. Data represents the mean ± S.D from three independent experiments compared to wild type. *p ≤ 0.05, **p ≤ 0.01, ***p ≤ 0.001 and ****p ≤ 0.0001. (B) Morphological alterations of GS mutant promastigotes observed under SEM micrographs (i) SEM micrograph of wild type promastigotes (LdGS(+/+)) showing a slender and elongated structure with a healthy flagella, (ii) image of LdGS(+/−) showing cell morphology almost similar to LdGS(+/+) but with shortened flagella, (iii) LdGS+/−/+) complementation mutant cellular morphology almost similar to wild type, (iv) image of null mutant, LdGS(−/−) demonstrating a much altered morphology and a shorter flagella (v) add back null mutant, LdGS(−/−/+) show shrinking morphological alteration (vi), LdGS(++/++) mutants show similar healthy appearance like LdGS(+/+) promastigotes and typically more elongated than LdGS(+/+) cells.
SEM analysis of LdGS(+/+) and LdGS(++/++) cells showed normal parasite morphology. They had a longer flagellum. The outline was slender and cell surface looked normal (Figure 4B).
Removal of LdGS Gene Increases the Sensitivity of Parasite to Drug, Ultimately Leading to Apoptotic or Necrotic Cell Death
Data suggested increase in sensitivity of knockout cells to standard drug. LdGS(++/++) showed ~1.3 fold increase in IC50 value of miltefosine (IC50:15.1 ± 0.17 µM) compared to LdGS(+/+) cells (IC50:11.5 ± 0.70 µM). LdGS(−/−) mutants showed ~2.4 fold decrease in IC50 value (IC50: 4.8 ± 0.21µM) compared to LdGS(+/+). LdGS(−/−/+) mutant showed reduction in IC50 value (IC50: 5.6 ± 0.61 µM) comparable to GS heterozygous mutant (Figure 5A). The data suggested that GS gene deletion is rendering parasite sensitive to miltefosine and the effect is reversed upon complementation with GS gene. We had tested other antileishmanial drug (Amphotericin B) in our study. DKO and the other knockout strains were not able to sustain the drug selection pressure so further detailed study could not be carried out (data not shown).
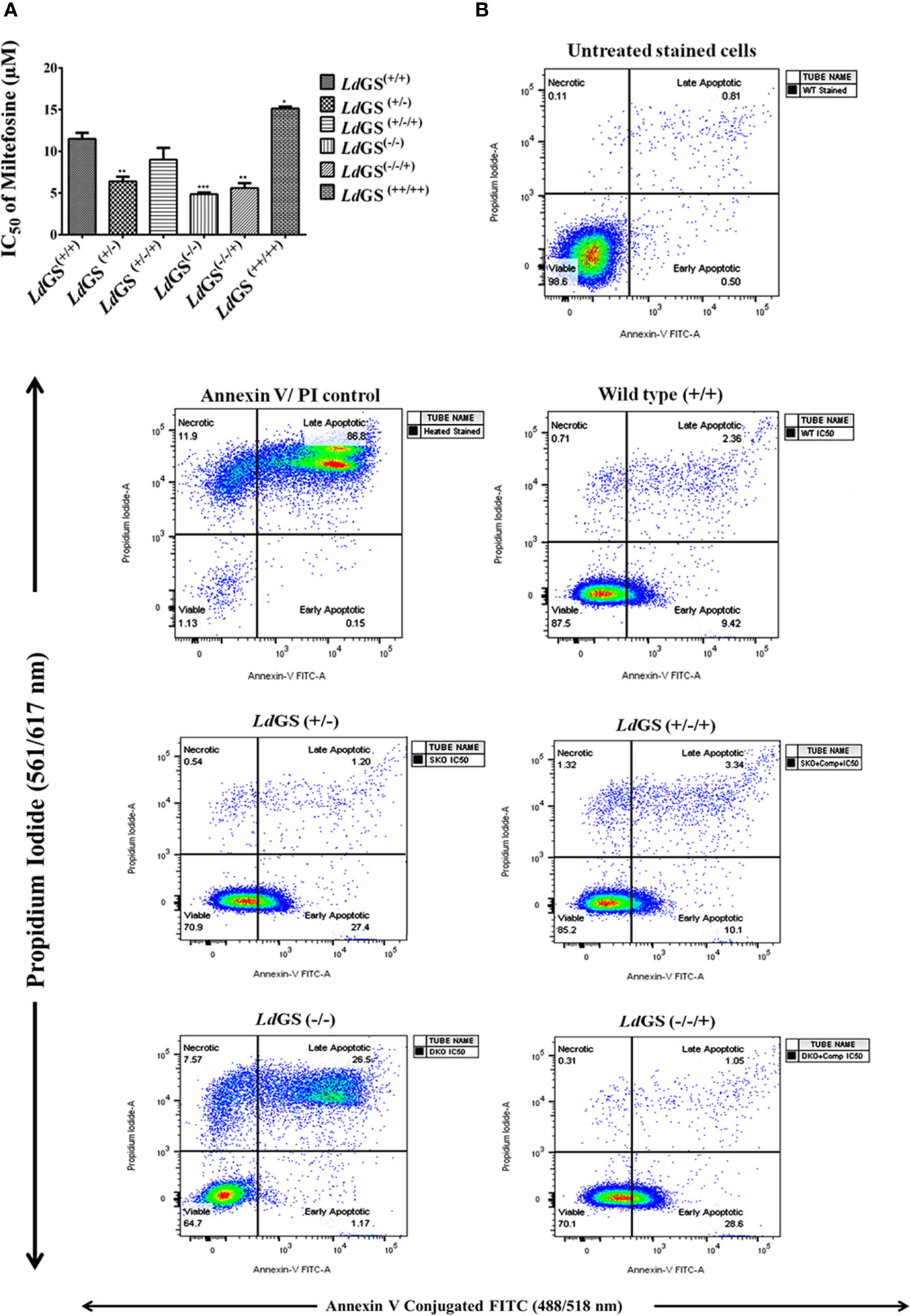
Figure 5 Sensitivity of L. donovani glutamine synthetase knockout and overexpressing promastigote parasite to standard drug miltefosine. (A) Promastigote growth in the presence of increasing concentration of miltefosine and IC50 value of miltefosine was calculated. Data represents the mean ± S.D. from three independent experiments. *p ≤ 0.05, **p ≤ 0.01 and ***p ≤ 0.001. (B) Mode of cell death due to action of standard drug in Leishmania GS knockout promastigotes. The cells treated with IC50 of miltefosine and had been subjected to annexin V-FITC and propidium iodide (PI) staining prior to cell sorting The cells which had not been miltefosine treated but had been dual stained were used as negative control. Flow cytometric data of wild type (LdGS+/+), GS heterozygous mutant (LdGS+/−), complementation mutant (LdGS+/−/+), null mutant (LdGS−/−) and add back null mutant (LdGS−/−/+) treated with miltefosine followed by 24 h incubation.
Percentage of early apoptotic cells were increased in LdGS(+/−) mutant (27.5%) and LdGS(−/−/+) mutant cells (28.6%) upon treatment with IC50 concentration of miltefosine compared to wild type (9.42%) and complementation mutant (LdGS+/−/+) cells (10.1%) in FACS analysis performed with Annexin V-FITC and PI. LdGS(−/−) mutants undergo late apoptosis or necrosis upon treatment with similar concentration of miltefosine and the percentage of late apoptotic cells or necrotic cells was found to be 26.5% (Figure 5B).
Discussion
Extensive study of the metabolic pathways in L. donovani has been done however the knowledge regarding the amino acid metabolizing enzymes in Leishmania promastigotes and amastigotes including regulation of the amino acid metabolism throughout the course of infection of the host, is rudimentary. A more detailed knowledge of amino acid metabolic network including uptake, degradation and biosynthesis is quiet essential to enrich our knowledge of Leishmania biology. Exploring the kinetoplastid amino acid metabolism may open a new array of therapeutic targets. One such amino acid metabolic pathway enzyme which has been explored in Leishmania parasite in this study is glutamine synthetase. It is a vital metabolic enzyme facilitating many biochemical reactions. It exists in all kingdoms of life including prokaryotes, extra thermophilic archeons, fungi, protozoans, plants as well as in humans.
Previous reports from our lab had identified GS from Leishmania and in silico analysis had provided structural aspects of variations in host and parasite enzyme. The biochemical and structural studies had pointed towards its potential as antileishmanial drug target (Kumar et al., 2020). The present study provides molecular and functional evidence of the role of glutamine synthetase in growth and infectivity of L. donovani and its essentiality for the parasites. Two strategies were designed to study glutamine synthetase expression by gene replacement and by episomal gene over expression. GS Overexpression was confirmed by immunoblotting and enzyme activity. Overexpression studies revealed increase in growth and infective ability of the parasites. Confirmation of LdGS knockout in transgenic strains at genomic level was done by PCR and changes in LdGS expression at the transcriptional and translational level was studied by RT-PCR, western blot and enzyme activity. Null mutants were generated which could survive only when exogenously supplied with glutamine. In LdGS mutants, the parasite grows slowly and was less infective. Here we show that glutamine limitation hinders the growth of LdGS null mutant, but can be rescued by nutrient supplementation under in vitro conditions. Our observations further strengthens the fact that media composition is an important parameter which influences the study of gene knockout of metabolic pathway enzymes (Boitz et al., 2017). There is no evidence till date that Leishmania can use host L-glutamine (taken as dietary supplement) for their growth and survivability, however it has been reported that carbon skeletons of the amino acids, especially in promastigotes acts as an energy source. In promastigotes more amino acid utilization is seen than in amastigotes. Glutamine is synthesized using environmental glutamate by interlinked pathway through glycosomal GMP synthase, finally utilized for synthesis of trypanothione (Glew et al., 1988; Subramanian and Sarkar, 2017).
The effect of GS gene deletion on in vitro infectivity of monocyte differentiated macrophage was observed. Among GS knockouts, heterozygous mutant parasite [(LdGS (+/−)] had almost 56% reduction in infectivity while null mutant [(LdGS (−/−)] showed ~93% of drastic reduction in infectivity to macrophage as compared to wild type cells. On episomal expression of GS gene in heterozygous mutants [(LdGS (+/−/+)] parasite infectivity was restored almost comparable to wild type. Unlike null mutant, GS add back null mutant [(LdGS (−/−/+)] parasites showed less alteration in the growth pattern and infectivity as compared to null mutants. Therefore, it may be said that the knockout parasite attempts to recover when episomal complementation is done. However, complete recovery is not seen. Residual LdGS activity of null mutant is probably due to the fact that, apart from GS there are several other ATP dependent enzymes in total cell lysate that catalyzes dephosphorylation of ATP which results in formation of blue color phosphomolybdate complex. GS first needs ATP to get active catalytic confirmation which facilitates binding of Glutamate that finally result in release of inorganic phosphate and Glutamine. Glutamine has been known to modulate immune response and play essential role for the control of L. donovani infection to macrophages. The gene of glutamine metabolism have been found to be upregulated in macrophages infected by L. donovani, as revealed by transcriptomic analysis (Ferreira et al., 2020). It is known that the protein expression is more when the gene integration happens in the genome, as compared to episomal addition. This data correlates with previously reported result in case of T. cruzi where GS was reported to be responsible for host-cell invasion establishment of infection (Crispim et al., 2018). In T. cruzi, GS facilitates cellular replication and infection to host cell by helping it escape parasitophorous vacuole. This established the link between GS activity and how the parasite grows and establishes infection inside host (Crispim et al., 2018). GS is involved in pathogenesis of M. tuberculosis, S. typhimurium, S. suis, S. pneumoniae and S. enterica (Klose and Mekalanos, 1997; Harth et al., 2000; Hendriksen et al., 2008; Si et al., 2009; Aurass et al., 2018). Also glnA-1 mutant strain of M. tuberculosis has less ability to infect THP-1 cells (Tullius et al., 2003). Glutamine has been found to regulate virulence gene expression responsible for invasion of host cell by T. gondii and L. monocytogenes (Lee et al., 2014; Haber et al., 2017). Similar result was obtained in in vivo infection with knockout strains in mice. Parasite burden was checked in spleen and liver of infected mice. However, low parasitic burden in LdGS (+/−/+) mutant than LdGS (+/−) mutant was observed. The parasites that overexpress LdGS are more infective and grow better in vitro, but in vivo, mice infected with parasites that overexpress the enzyme do not present increased parasite load compared to animals infected with WT parasites. The outcomes of infectivity, in vitro and in vivo may vary due to the presence of different cytokine milieu that can affect infection vividly. Active segregation of episomal vectors does not take place and so, the daughter cells do not inherit equal copy number of the constructs. All transfected parasites do not have the same number of copies due to which their expression varies from cell to cell. Therefore, if the copy number is too high, it may be toxic to parasite. If the copy number is too low, it may not be enough to complement gene deletion. Episomal vectors lack active segregation mechanisms so possibly distribution between daughter cells is not always equal. Furthermore, the gene expression level from an episome cannot be controlled and fluctuates among transfected parasites depending upon the copy number of episome. Thus, the target protein expression may be low and insufficient for rescue or too high and have deleterious effects on parasite growth (Kushnir et al., 2011; Roberts, 2011). Cell cycle experiment with synchronized cells shows that glutamine is essential and is required for progression through the restriction point in mid-to late G1 and through S phase into cell division (G2/M). For cell cycle progress to mid to late G1, glucose and glutamine both are essential. But only glutamine is required for transition through S phase (Colombo et al., 2011). Amino acid metabolism plays an important role in critical life processes of parasites such as pathogens and resistance to stress inside the host. In critical biological processes during the life cycle of these parasites, such as the establishment of infection within the mammalian and insect hosts, differentiation, modulation of the cell cycle and resistance to extreme stress conditions metabolism of amino acid play very important role (Marchese et al., 2018). Morphological changes were observed in GS mutant cells which can be inferred to the fact that either LdGS maintains the cell morphology or mutating the gene pushes the cells under biochemical and oxidative stress which in turn affects the morphology.
GS knockout made L. donovani parasites more sensitive to standard drug miltefosine and mode of cell death was evaluated by annexin V-FITC/PI staining. Wild type parasites were unstained whereas IC50 treated cells showed less population of early apoptotic cells stained with annexin V-FITC (externalization of phosphatidylserine). Assessment of flow cytometric data of null mutants treated with similar drug concentration showed significant population of cells undergoing late apoptosis or necrosis which were stained by both annexin V and PI. GS null mutant pre-treated with NAC (N -acetyl cysteine) showed recovery of cells with a smaller number of both annexin V and PI-stained cells indicating role of oxidative stress leading to apoptotic signals (externalization of phosphatidylserine) in GS null mutants compared to wild-type cells. Glutamine synthetase is also essential for combating oxidative stress as the GS null mutants upon miltefosine treatment were observed to have higher sensitivity to miltefosine as compared to wild type cells. Treatment time with miltefosine vary in case of both cell based study and FACS data. It was 48 h in case of cell-based study while in case of FACS based analysis cells were incubated with drug for 24 h. Incubating cells with drug for 48 h resulted in cellular morphological alteration and large number of dead cells specially in case of null mutants which made the comparative analysis of data with different cell lines difficult. The hypothetical model of miltefosine sensitivity of LdGS mutants is depicted in Figure 6.
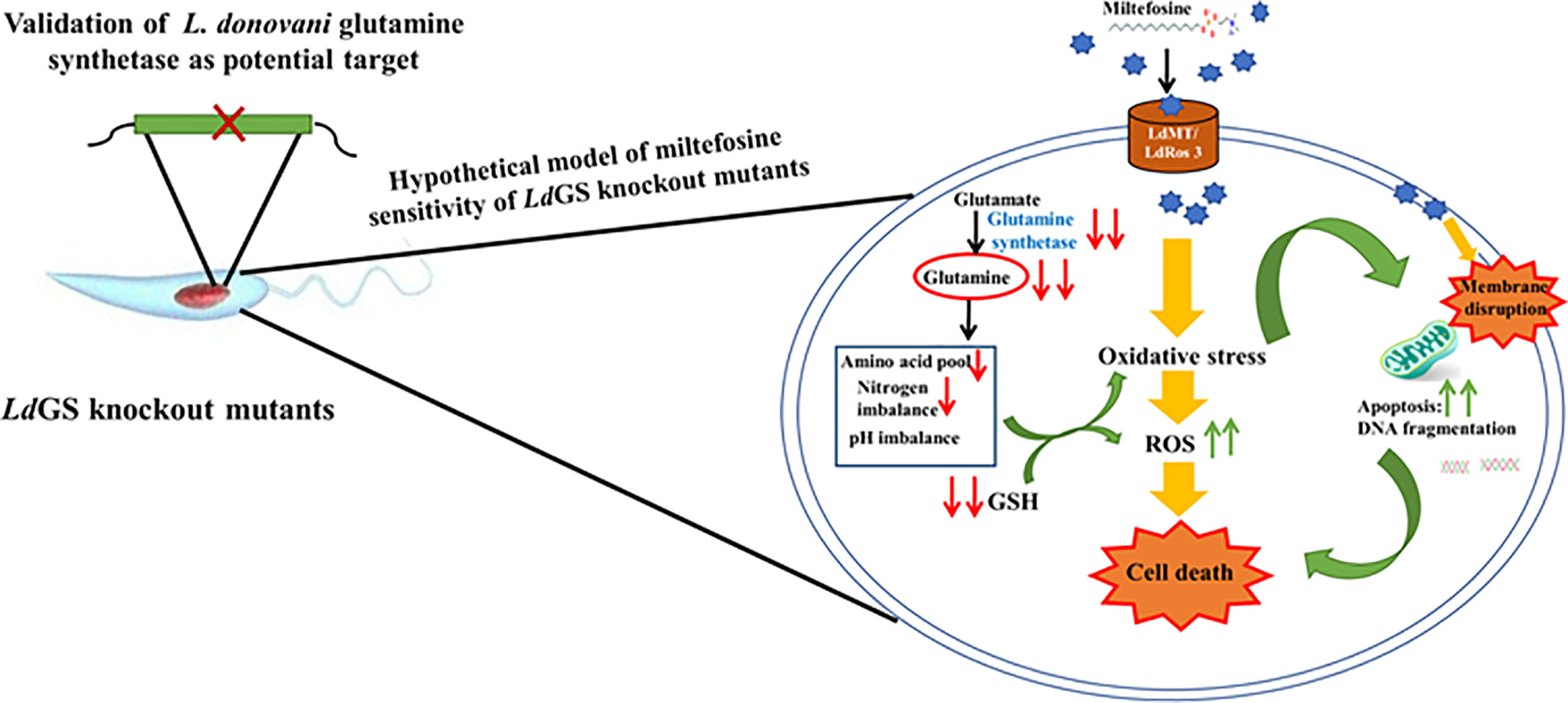
Figure 6 Hypothetical model explaining sensitivity of LdGS knockout strains to Miltefosine. Based on experimental results a model was proposed to understand the link between GS expression and miltefosine sensitivity.
Overall findings of our data provide the role of GS gene overexpression and deletion of alleles leading to alteration in the growth pattern and infectivity of promastigotes. This work deals with the impact of GS deletion of L. donovani on the survivability and infectivity of the parasites, in vitro and in vivo conditions. The GS null mutant parasites failed to grow in glutamine deprived media and could survive only when maintained in glutamine supplemented media, pointing towards the essential role of this gene in Leishmania parasites. Hence, experimental results indicate that LdGS is pivotal to cell survival and its metabolic regulation. The above studies validate the conserved glutamine synthetase as promising therapeutic target and can be used for Leishmania specific designing of new drug candidates.
Data Availability Statement
The original contributions presented in the study are included in the article/Supplementary Material. Further inquiries can be directed to the corresponding author.
Ethics Statement
The study was performed in accordance with appropriate institutional guidelines for animals. All animal experiments were approved by the Institutional Animal Ethics Committee, WBSU, Barasat [File no. WBSU/IAEC/2019-20/8(CP), dated 20.05.2019]. IAEC registration no. 1394/GO/Re/S/10/CPCSEA, dated 09/12/2016) approved by, Committee for the Purpose of Control and Supervision of Experiments on Animals, Ministry of Environment and Forest, Govt. of India.
Author Contributions
SS and CP conceived and designed the experiments. VK, SG, and KR performed the research. SS and CP wrote the manuscript. All authors contributed to the article and approved the submitted version.
Funding
We acknowledge the DST-FIST, Govt. of India [Ref: SR/FST/LS1-001/2014] and DBT-BOOST, Govt. of West Bengal [Ref: 49 (11)/BT (Estt)/1P-4/2013 (Part-1)] for providing the research infrastructures in WBSU, Barasat. VK received SRF in the project funded by Department of Pharmaceuticals, India [KA-01(2013-14)-Sushma]. SG received SRF from ICMR, Govt. of India [Ref: Fellowship/65/20I9-ECD-II dated 17/07/2019], and KR received the fellowship from INSPIRE-Department of Science and Technology, Govt. of India [Ref: Dy. No. C/3321/IFD/2019-20 dated 25/09/2019].
Conflict of Interest
The authors declare that the research was conducted in the absence of any commercial or financial relationships that could be construed as a potential conflict of interest.
Acknowledgments
We acknowledge the support of Department of Pharmaceuticals, India for providing financial support (Grant KA01). We thank Director, NIPER, S.A.S Nagar, India for providing research infrastructure. Special thanks to Mr. Prateek Arora, IISER, Mohali, India for assisting with the flow cytometry work.
Supplementary Material
The Supplementary Material for this article can be found online at: https://www.frontiersin.org/articles/10.3389/fcimb.2021.622266/full#supplementary-material
Supplementary Figure 1 | The 5′UTR and 3′UTR sequences of LdGS used for cloning in knockout vectors are depicted.
Supplementary Figure 2 | (A) Vector map of pXG Hygro, used to generate heterozygous construct (B) Vector map of pXG-Neo, used to generate null mutant construct (C) Vector map of pXG-Phleo, used to generate complementation construct (kind gift from Prof. Stephen M. Beverley, Department of Molecular Microbiology, Washington University, S. Louis, USA). (D) Vector map of psp72αhygroα, used to generate overexpression construct (kind gift from Prof. Rentala Madhubala, JNU, New Delhi).
Supplementary Figure 3 | Scheme for GS gene Knockout in L. donovani parasites. (A) The Knockout was done using targeted gene replacement(UTR represents untranslated region); (B) Schematic representation to highlight set of primers along with their position of binding for confirmation of appropriate integration of LdGS gene replacement cassette with the genes for neomycin resistance (neor-neomycin phosphotransferase) or hygromycin resistance (hygror-hygromycin phosphotransferase) and 5′ and 3′ UTR were present in flanking region.
Supplementary Figure 4 | Comparison of the growth rate of all knockout strains in glutamine deprived media.
Supplementary Table 1 | Primers used for generating LdGS overexpressor and knockout constructs.
Supplementary Table 2 | List of primers used to confirm appropriate integration of LdGS knockout cassettes.
Supplementary Table 3 | List of primers used for RT-PCR analysis of GS mutant parasite.
Supplementary Table 4 | (A, B) Parasite load in infected male BALB/c mice (A) Spleen (B) Liver of mice infected with different mutant strains of L. donovani Dd8, in vivo. Data are representative of Mean ± SE from three independent experiments with 5 animals per group.
References
Aurass P., Düvel J., Karste S., Nübel U., Rabsch W., Flieger A. (2018). glnA Truncation in Salmonella enterica results in a small colony variant phenotype, attenuated host cell entry, and reduced expression of flagellin and SPI-1-associated effector genes. Appl. Environ. Microbiol. 84, e01838–e01817. doi: 10.1128/AEM.01838-17
Boitz J. M., Gilroy C. A., Olenyik T. D., Paradis D., Perdeh J., Dearman K., et al. (2017). Arginase is essential for survival of Leishmania donovani promastigotes but not intracellular amastigotes. Infect. Immun. 85, e00554–e00516. doi: 10.1128/IAI.00554-16
Burbaeva G. S., Boksha I. S., Tereshkina E. B., Savushkina O. K., Starodubtseva L. I., Turishcheva M. S. (2005). Glutamate metabolizing enzymes in prefrontal cortex of Alzheimer’s disease patients. Neurochem. Res. 30, 1443–1451. doi: 10.1007/s11064-005-8654-x4
Burza S., Croft S. L., Boelaert M. (2018). Leishmaniasis [seminar]. Lancet 392, 951–70. doi: 10.1016/S0140-6736(18)31204-2
Colombo S. L., Palacios-Callender M., Frakich N., Carcamo S., Kovacs I., Tudzarova S., et al. (2011). Molecular basis for the differential use of glucose and glutamine in cell proliferation as revealed by synchronized HeLa cells. Proc. Natl. Acad. Sci. 108, 21069–21074. doi: 10.1073/pnas.1117500108
Crispim M., Damasceno F. S., Hernández A., Barisón M. J., Sauter I. P., Pavani R. S., et al. (2018). The glutamine synthetase of Trypanosoma cruzi is required for its resistance to ammonium accumulation and evasion of the parasitophorous vacuole during host-cell infection. PloS Negl. Trop. Dis. 12, e0006170. doi: 10.1371/journal.pntd.0006170
Crowther G. J., Shanmugam D., Carmona S. J., Doyle M. A., Hertz-Fowler C., Berriman M., et al. (2010). Identification of attractive drug targets in neglected-disease pathogens using an in silico approach. PloS Negl. Trop. Dis. 4, e804. doi: 10.1371/journal.pntd.0000804
Cruz A., Coburn C. M., Beverley S. M. (1991). Double targeted gene replacement for creating null mutants. Proc. Natl. Acad. Sci. 88, 7170–7174. doi: 10.1073/pnas.88.16.7170
Cruzat V., Macedo Rogero M., Noel Keane K., Curi R., Newsholme P. (2018). Glutamine: Metabolism and immune function, supplementation and clinical translation. Nutrients 10, 1564. doi: 10.3390/nu10111564
Dey S., Mukherjee D., Sultana S. S., Mallick S., Dutta A., Ghosh J., et al. (2020). Combination of Mycobacterium indicus pranii and heat-induced promastigotes cures drug-resistant Leishmania infection: Critical role of IL-6 producing classical dendritic cells. Infect. Immun. 88 (6), e00222–19. doi: 10.1128/IAI.00222-19
Dolai S., Yadav R. K., Pal S., Adak S. (2008). Leishmania major ascorbate peroxidase overexpression protects cells against reactive oxygen species-mediated cardiolipin oxidation. Free Radic. Biol. Med. 45, 1520–1529. doi: 10.1016/j.freeradbiomed.2008.08.029
Fatumo S., Plaimas K., Mallm J.-P., Schramm G., Adebiyi E., Oswald M., et al. (2009). Estimating novel potential drug targets of Plasmodium falciparum by analysing the metabolic network of knock-out strains in silico. Infect. Genet. Evol. 9, 351–358. doi: 10.1016/j.meegid.2008.01.007
Ferreira C., Mesquita I., Barbosa A. M., Osório N. S., Torrado E., Beauparlant C.-J., et al. (2020). Glutamine supplementation improves the efficacy of miltefosine treatment for visceral leishmaniasis. PloS Negl. Trop. Dis. 14, e0008125. doi: 10.1371/journal.pntd.0008125
Fisher S. H. (1999). Regulation of nitrogen metabolism in Bacillus subtilis: vive la difference! Mol. Microbiol. 32, 223–232. doi: 10.1046/j.1365-2958.1999.01333.x
Forde B. G., Lea P. J. (2007). Glutamate in plants: metabolism, regulation, and signalling. J. Exp. Bot. 58, 2339–2358. doi: 10.1093/jxb/erm121
Gawronski J. D., Benson D. R. (2004). Microtiter assay for glutamine synthetase biosynthetic activity using inorganic phosphate detection. Anal. Biochem. 327, 114–118. doi: 10.1016/j.ab.2003.12.024
Glew R. H., Saha A. K., Das S., Remaley A. T. (1988). Biochemistry of the Leishmania species. Microbiol. Rev. 52, 412. doi: 10.1128/MR.52.4.412-432.1988
Gluenz E., Wheeler R. J., Hughes L., Vaughan S. (2015). “Scanning and three-dimensional electron microscopy methods for the study of Trypanosoma brucei and Leishmania mexicana flagella,” in Methods in cell biology (Elsevier) 127, 509–542. doi: 10.1016/bs.mcb.2014.12.011
Haber A., Friedman S., Lobel L., Burg-Golani T., Sigal N., Rose J., et al. (2017). l-Glutamine induces expression of Listeria monocytogenes virulence genes. PloS Pathog. 13, e1006161. doi: 10.1371/journal.ppat.1006161
Harth G., Zamecnik P. C., Tang J.-Y., Tabatadze D., Horwitz M. A. (2000). Treatment of Mycobacterium tuberculosis with antisense oligonucleotides to glutamine synthetase mRNA inhibits glutamine synthetase activity, formation of the poly-L-glutamate/glutamine cell wall structure, and bacterial replication. Proc. Natl. Acad. Sci. 97, 418–423. doi: 10.1073/pnas.97.1.418
Hendriksen W. T., Kloosterman T. G., Bootsma H. J., Estevão S., De Groot R., Kuipers O. P., et al. (2008). Site-specific contributions of glutamine-dependent regulator GlnR and GlnR-regulated genes to virulence of Streptococcus pneumoniae. Infect. Immun. 76, 1230–1238. doi: 10.1128/IAI.01004-07
Jain S. K., Sahu R., Walker L. A., Tekwani B. L. (2012). A Parasite Rescue and Transformation Assay for Antileishmanial Screening Against Intracellular Leishmania donovani Amastigotes in THP1 Human Acute Monocytic Leukemia Cell Line. J. Vis. Exp. 70, 1–14. doi: 10.3791/4054
Klose K. E., Mekalanos J. J. (1997). Simultaneous prevention of glutamine synthesis and high-affinity transport attenuates Salmonella typhimurium virulence. Infect. Immun. 65, 587–596. doi: 10.1128/IAI.65.2.587-596.1997
Kumar V., Yadav S., Soumya N., Kumar R., Babu N. K., Singh S. (2017). Biochemical and inhibition studies of glutamine synthetase from Leishmania donovani. Microb. Pathog. 107, 164–174. doi: 10.1016/j.micpath.2017.03.024
Kumar V., Sri N. S., Tripathi N., Sharma V. K., Bharatam P. V., Garg P., et al. (2020). Structural exploration of glutamine synthetase from Leishmania donovani: Insights from in silico and in vitro analysis. Int. J. Biol. Macromol. 146, 860–874. doi: 10.1016/j.ijbiomac.2019.09.209
Kushnir S., Cirstea I. C., Basiliya L., Lupilova N., Breitling R., Alexandrov K. (2011). Artificial linear episome-based protein expression system for protozoon Leishmania tarentolae. Mol. Biochem. Parasitol. 176, 69–79. doi: 10.1016/j.molbiopara.2010.12.002
Lee I.-P., Evans A. K., Yang C., Works M. G., Kumar V., De Miguel Z., et al. (2014). Toxoplasma gondii is dependent on glutamine and alters migratory profile of infected host bone marrow derived immune cells through SNAT2 and CXCR4 pathways. PloS One 9, e109803. doi: 10.1371/journal.pone.0109803
Marchese L., Bringaud F., Damasceno F., Silber A., Michels P., Nascimento J. (2018). The Uptake and Metabolism of Amino Acids, and Their Unique Role in the Biology of Pathogenic Trypanosomatids. Pathogens 7, 36. doi: 10.3390/pathogens7020036
Martins D. R. A., Jeronimo S. M. B., Donelson J. E., Wilson M. E. (2006). Leishmania chagasi T-cell antigens identified through a double library screen. Infect. Immun. 74, 6940–6948. doi: 10.1128/IAI.02032-05
Mosmann T. (1983). Rapid colorimetric assay for cellular growth and survival: Application to proliferation and cytotoxicity assays. J. Immunol. Methods 65, 55–63. doi: 10.1016/0022-1759(83)90303-4
Mukherjee A., Adhikari A., Das P., Biswas S., Mukherjee S., Adak S. (2018). Loss of virulence in NAD (P) H cytochrome b5 oxidoreductase deficient Leishmania major. Biochem. Biophys. Res. Commun. 503, 371–377. doi: 10.1016/j.bbrc.2018.06.037
Mukherjee D., Yousuf M., Dey S., Chakraborty S., Chaudhuri A., Kumar V., et al. (2020). Targeting the Trypanothione Reductase of Tissue-Residing Leishmania in Hosts’ Reticuloendothelial System: A Flexible Water-Soluble Ferrocenylquinoline-Based Preclinical Drug Candidate. J. Med. Chem. doi: 10.1021/acs.jmedchem.0c00690
Newsholme P., Lima M. M. R., Procópio J., Pithon-Curi T. C., Bazotte R. B., Curi R. (2003). Glutamine and glutamate as vital metabolites. Braz. J. Med. Biol. Res. 36, 153–163. doi: 10.1590/S0100-879X2003000200002
Padmanabhan P. K., Mukherjee A., Singh S., Chattopadhyaya S., Gowri V. S., Myler P. J., et al. (2005). Glyoxalase I from Leishmania donovani: A potential target for anti-parasite drug. Biochem. Biophys. Res. Commun. 337, 1237–1248. doi: 10.1016/j.bbrc.2005.09.179
Pal D. S., Abbasi M., Mondal D. K., Varghese B. A., Paul R., Singh S., et al. (2017). Interplay between a cytosolic and a cell surface carbonic anhydrase in pH homeostasis and acid tolerance of Leishmania. J. Cell Sci. 130, 754–766. doi: 10.1242/jcs.199422
Ready P. D. (2014). Epidemiology of visceral leishmaniasis. Clin. Epidemiol. 6, 147. doi: 10.2147/CLEP.S44267
Roberts S. C. (2011). The genetic toolbox for Leishmania parasites. Bioeng. Bugs 2, 320–326. doi: 10.4161/bbug.2.6.18205
Saunders E. C., Ng W. W., Kloehn J., Chambers J. M., Ng M., McConville M. J. (2014). Induction of a stringent metabolic response in intracellular stages of Leishmania mexicana leads to increased dependence on mitochondrial metabolism. PloS Pathog. 10, e1003888. doi: 10.1371/journal.ppat.1003888
Si Y., Yuan F., Chang H., Liu X., Li H., Cai K., et al. (2009). Contribution of glutamine synthetase to the virulence of Streptococcus suis serotype 2. Vet. Microbiol. 139, 80–88. doi: 10.1016/j.vetmic.2009.04.024
Smith P. K., Krohn R. I., Hermanson G. T., Mallia A. K., Gartner F. H., Provenzano M. D., et al. (1985). Measurement of protein using bicinchoninic acid. Anal. Biochem. 150, 76–85. doi: 10.1016/0003-2697(85)90442-7
Soumya N., Panara M. N., Neerupudi K. B., Singh S. (2017). Functional analysis of an AMP forming acetyl CoA synthetase from Leishmania donovani by gene overexpression and targeted gene disruption approaches. Parasitol. Int. 66, 992–1002. doi: 10.1016/j.parint.2016.11.001
Subramanian A., Sarkar R. R. (2017). Revealing the mystery of metabolic adaptations using a genome scale model of Leishmania infantum. Sci. Rep. 7, 1–12. doi: 10.1038/s41598-017-10743-x
Sultana S. S., Ghosh J., Chakraborty S., Mukherjee D., Dey S., Mallick S., et al. (2018). Selective in vitro inhibition of Leishmania donovani by a semi-purified fraction of wild mushroom Grifola frondosa. Exp. Parasitol. 192, 73–84. doi: 10.1016/j.exppara.2018.07.006
Torres-Guerrero E., Quintanilla-Cedillo M. R., Ruiz-Esmenjaud J., Arenas R. (2017). Leishmaniasis: a review. F1000Research 6, 750. doi: 10.12688/f1000research.11120.1
Tullius M. V., Harth G., Horwitz M. A. (2003). Glutamine synthetase GlnA1 is essential for growth of Mycobacterium tuberculosis in human THP-1 macrophages and guinea pigs. Infect. Immun. 71, 3927–3936. doi: 10.1128/IAI.71.7.3927-3936.2003
Uliana S. R. B., Trinconi C. T., Coelho A. C. (2018). Chemotherapy of leishmaniasis: present challenges. Parasitology 145, 464–480. doi: 10.1017/S0031182016002523
Keywords: leishmaniasis, glutamine synthetase, knockout, essentiality, infectivity
Citation: Kumar V, Ghosh S, Roy K, Pal C and Singh S (2021) Deletion of Glutamine Synthetase Gene Disrupts the Survivability and Infectivity of Leishmania donovani. Front. Cell. Infect. Microbiol. 11:622266. doi: 10.3389/fcimb.2021.622266
Received: 28 October 2020; Accepted: 25 January 2021;
Published: 26 February 2021.
Edited by:
Christy Petersen, The University of Iowa, United StatesReviewed by:
Diego Luis Costa, University of São Paulo, BrazilJohn Mina, Teesside University, United Kingdom
Copyright © 2021 Kumar, Ghosh, Roy, Pal and Singh. This is an open-access article distributed under the terms of the Creative Commons Attribution License (CC BY). The use, distribution or reproduction in other forums is permitted, provided the original author(s) and the copyright owner(s) are credited and that the original publication in this journal is cited, in accordance with accepted academic practice. No use, distribution or reproduction is permitted which does not comply with these terms.
*Correspondence: Sushma Singh, c3VzaG1hc2luZ2hAbmlwZXIuYWMuaW4=