- 1Division of Infectious Diseases, Department of Medicine, University of California Irvine, Irvine, CA, United States
- 2Department of Microbiology and Molecular Genetics, University of California Irvine, Irvine, CA, United States
- 3School of Biological Sciences, University of California Irvine, Irvine, CA, United States
- 4Department of Molecular Biology and Biochemistry, University of California Irvine, Irvine, CA, United States
Auranofin, a reprofiled FDA-approved drug originally designed to treat rheumatoid arthritis, has emerged as a promising anti-parasitic drug. It induces the accumulation of reactive oxygen species (ROS) in parasites, including Toxoplasma gondii. We generated auranofin resistant T. gondii lines through chemical mutagenesis to identify the molecular target of this drug. Resistant clones were confirmed with a competition assay using wild-type T. gondii expressing yellow fluorescence protein (YFP) as a reference strain. The predicted auranofin target, thioredoxin reductase, was not mutated in any of our resistant lines. Subsequent whole genomic sequencing analysis (WGS) did not reveal a consensus resistance locus, although many have point mutations in genes encoding redox-relevant proteins such as superoxide dismutase (TgSOD2) and ribonucleotide reductase. We investigated the SOD2 L201P mutation and found that it was not sufficient to confer resistance when introduced into wild-type parasites. Resistant clones accumulated less ROS than their wild type counterparts. Our results demonstrate that resistance to auranofin in T. gondii enhances its ability to abate oxidative stress through diverse mechanisms. This evidence supports a hypothesized mechanism of auranofin anti-parasitic activity as disruption of redox homeostasis.
Introduction
For more than 50 years, the mainstay of treatment for acute toxoplasmosis has been a combination of a dihydrofolate reductase inhibitor and a sulfa antimicrobial. While this is currently a critical therapeutic strategy, currently approved drugs cannot eliminate latent parasites in cysts that are found in chronically infected individuals. Moreover, these drugs have significant bone marrow toxicity, are suspected teratogens and the emergence of resistance remains a potential threat to treatment. Repurposing FDA-approved drugs will accelerate drug discovery for neglected parasitic diseases. Most recently, auranofin, a reprofiled drug that is FDA-approved for treatment of rheumatoid arthritis, has emerged as a promising anti-parasitic agent. It has antiproliferative activity against Plasmodium falciparum (Sannella et al., 2008), Schistosoma mansoni (Angelucci et al., 2009), Leishmania infantum (Ilari et al., 2012), and Entamoeba histolytica (Debnath et al., 2012), as well as other parasites with public health significance (Martinez-Gonzalez et al., 2010; Debnath et al., 2013; Tejman-Yarden et al., 2013; Bulman et al., 2015; da Silva et al., 2016; Hopper et al., 2016; Peroutka-Bigus and Bellaire, 2019). Despite this promising broad spectrum of anti-parasitic activity, the molecular target of auranofin has only been indirectly implicated as thioredoxin reductase.
Thioredoxin reductase enzymes are found in archaea, bacteria and diverse eukaryotes and play a key role in reduction of disulfide bonds that is essential for cell replication and survival. In humans, inhibition of thioredoxin reductase 1 induces expression of heme oxygenase-1 to repress inflammation (Kobayashi et al., 2006).
We previously demonstrated that auranofin reduces T. gondii infection of host cells in vitro and a single dose of auranofin allows survival of chicken embryos infected with this parasite (Andrade et al., 2014). It is hypothesized that the anti-parasitic activity of auranofin comes from inhibition of the thioredoxin reductase enzyme of these parasites, akin to its action on human cells (Kuntz et al., 2007; Debnath et al., 2012; Tejman-Yarden et al., 2013). This postulated mechanism of action explains why parasites treated with auranofin accumulate ROS (Debnath et al., 2012). Intriguingly, auranofin may improve infection outcome by decreasing pathogenic host inflammatory damage in addition to its direct inhibition of parasite replication.
In this paper we describe our work to investigate resistance to auranofin in T. gondii parasites. This approach illuminates mechanisms of resistance that might arise during its use as an anti-parasitic. In some circumstances, identification of resistance mechanisms validates proposed drug targets [i.e., (McFadden et al., 2000)]. In this study, we found that resistance was not associated with changes to the Toxoplasma thioredoxin reductase gene and no other single locus emerged as a consistent site underlying resistance. However, we observed that resistant clones accumulated less ROS than their wild type counterparts, demonstrating that auranofin resistance enhances oxidative stress responses.
Materials and Methods
Host Cell and Parasite Cultures
Parasite clones and human foreskin fibroblasts (HFF) were grown and maintained in Dulbecco’s modified Eagle’s medium supplemented with 10% fetal bovine serum (Hyclone), penicillin and streptomycin (50 μg/ml each) and 200 μM L-glutamine. We will refer to this complete medium as D10. T. gondii were maintained by serial passage in HFF monolayers at 37°C in a humid 5% CO2 atmosphere, including RH parasites (National Institutes of Health AIDS Reference and reagent Repository, Bethesda, MD), RH tachyzoites expressing cytoplasmic yellow fluorescent protein (YFP) (kindly provided by M.J. Gubbels, Boston College, Boston, Massachusetts) (Gubbels et al., 2003) and Ku80 knock-out parasites (ΔKu80, kindly provided by V. Carruthers, University of Michigan Medical School Dexter, Michigan) (Huynh and Carruthers, 2009; Fox et al., 2009).
Generation of T. gondii Clones Resistant to Auranofin
We mutagenized T. gondii with ENU as previously described (Pfefferkorn and Pfefferkorn, 1979; Nagamune et al., 2007). Briefly, approximately 1.5 × 107 intracellular tachyzoites of wild-type RH strain T. gondii were mutagenized with ENU (N-Ethyl-N-nitrosourea, N3385-1G, Sigma Aldrich; 100–200 µg/ml) in DMEM without FBS for 2 h at 37°C. These parasites were washed and transferred to a new confluent HFF monolayer for selection with auranofin (2.5 μM-3 μM) until normal pace of replication was observed (~2 weeks). Auranofin resistant clones were single cell cloned by limiting dilution in 2 μM auranofin. Isolated clones were propagated in D10 media with 1 μM auranofin to maintain selection pressure without cytotoxic effects on host cells (data not shown).
We selected ten representative T. gondii clones from independent ENU-generated pools to investigate resistance. To assess auranofin resistance, we modified a tachyzoite growth competition assay (Ma et al., 2008). Using wild-type YFP-expressing T. gondii parasites as a control, confluent monolayers of HFF cells were inoculated with approximately equal numbers (2 × 106; 1:1 ratio) of ENU-mutagenized parasites and YFP- expressing wild-type RH parasites. Upon lysis, a new T25 flask with confluent HFF was inoculated with 0.25 ml of lysed parasites. Another 0.25 ml of lysed parasites was used for flow cytometry. The YFP fluorescence allowed us to differentiate wild-type and AurR parasites in the mixed populations to track their growth over serial passages. Host cell debris was removed by filtering of parasites with a 3 μm filter membrane. After parasites were collected by centrifugation (400g for 10 min), the pellet was resuspended in 1 ml PBS. The total number of parasites and the number of parasites expressing YFP were quantified by flow cytometry using a FACSCalibur flow cytometer (Becton Dickinson) and analyzed with FlowJo software (Tree Star). The result of three independent experiments analyzing the replication fitness advantage between each auranofin resistant clone and the wild-type parasites was analyzed with parametric paired t-tests. A two-tailed p-value <0.05 was considered statistically significant.
Identification of Mutations in Auranofin-Resistant T. gondii Clones
We extracted genomic DNA (gDNA) from auranofin resistant parasites lines using the DNeasy Blood and Tissue kit (Qiagen). The thioredoxin reductase gene (TGGT1_309730, ToxoDB.org) was amplified from gDNA samples as two PCR fragments ~3.7 kb each. The 5’ half of the gene was amplified with primers GGACCTATGAGTTGCCTCTCTG and GTCAAATCCCAATTCGCGGAG, and the 3’ half with GTCTGCTCTCTCTGTTTCGCAAG and CCTCCACATCCTCCAGAAGC. Sequencing primers are 5TrxR 1R, 5TrxR 1F and 5TrxR 3F for the 5’ half, and TrxR F 8, 3TrxR 2F, 3TrxR 3F, and 3TrxR 4F for the 3’ half (Table S1).
To determine the presence of other SNVs in the coding region of our T. gondii auranofin resistant clones, gDNA from 10 resistant clones and wild-type RH parasites was submitted for library construction and whole genome sequencing at the Genomic High Throughput Facility at University of California, Irvine. Briefly, genomic DNA (gDNA) was initially quantified with a Qubit DNA High Sensitivity kit. One hundred nanograms of gDNA was sheared to <300–500> bp in size using a Covaris S220 ultrasonicator (shearing conditions: duty Cycle 10%, Cycles/burst 200 and treatment time 55 sec) (Covaris, MA). The size and the quality of the resulting DNA fragments were analyzed with an Agilent DNA High Sensitivity chip. Ten nanograms of each sample’s fragmented DNA was end-repaired, poly adenylated and ligated to a 6bp DNA barcodes (Bioo Scientific NextFlex DNA barcodes) using a PrepX Complete ILMN DNA Library kit (Takara Bio, CA). The genomic library was built using the Apollo 324 system (Takara Bio, CA) to generate 520 bps inserts. To enrich the DNA libraries, 8 cycles of PCR amplification using a Kapa library amplification kit was used (cycling conditions: 98°C for 45 s, 8 cycles of 98°C for 15 sec, 60°C for 30 s 72° C for 30 s and then 72°C for 1 min) in an Apollo 324 system. The ultimate DNA concentration was calibrated using Qubit 2.0 dsDNA HS Assay kit and analyze with an Agilent DNA High Sensitivity chip. Before sequencing, we quantified our DNA libraries using a KAPA Library Quantification kit for Illumina platforms (Roche). Each library was normalized to 2nM and then pooled equimolar amounts in the final multiplex library that was sequenced on the Illumina HiSeq 4000.
The ten auranofin resistant T. gondii clones and wild-type RH T.gondii were sequenced with HiSeq 4000 paired end 100 reads, quality analyzed, adapter and quality trimmed before alignment with the annotated reference genome of GT1 strain from ToxoDB.org (published 7/19/2013) (Gajria et al., 2008). After initial quality control with FASTQC, reads were trimmed using Illumina adapter sequences with the error probability of 0.05 and reads shorter than 20 bases were discarded. Single Nucleotide Variant (SNV) calling for the haploid genome had a coverage threshold of >5 fold. Each of the 10 mutant clones were compared to wild-type RH variant track and shared variants were filtered out. Only SNVs in the coding regions of the 10 mutant clones are reported. Only genes containing SNVs with 100% frequency and ≥50% of read count and read coverage were considered in our final analysis. Genomic DNA from each mutant clone was used to amplify the targeted SNV region of interest. The resulting amplicons ranged between 1.7–4.5 kbp in size. PCR amplicons were purified prior to sequencing via a QiaQuick PCR Purification Kit (Qiagen 28106). Amplicons were sequenced by Sanger sequencing (Genewiz), using sequencing primers targeted to the SNV region (Table S1). Genomic sequences for all 10 mutant lines can be accessed NCBI, under the Bioproject PRJNA679812.
Generation of Modified SOD2 Lines
We generated a T. gondii clone with a 3’ knock-in YFP tag to TgSOD2 using a ligation independent cloning approach as previously described (Huynh and Carruthers, 2009). The mitochondrial distribution of our clone was confirmed by fluorescent microscopy using a Zeiss Axioskop with Axiovision camera and software. Co-localization of the TgSOD2-YFP with mitochondria was done by staining parasite mitochondria with an anti-F1B ATPase antibody (gift from Peter Bradley at UCLA). We subsequently introduced the L201P mutation into the TgSOD2-YFP line using a modified CRISPR plasmid generated using plasmid pSAG1::CAS9-U6::sgUPRT as the scaffold (Shen et al., 2017; Addgene # 54467). Our plasmid had an additional DHFR-TM marker for pyrimethamine selection derived from pLoxP-DHFR-mCherry [(Long et al., 2016), Addgene # 70147], and the two gRNA sequences were replaced with cggtataccggacagatccg and TAGTTTCGCCCAGTTCAAGG, selected by the Benchling software (Benchling.com).
The DNA sequence used for homology directed repair (HDR) was amplified with the primers GATAGTGTGTGAAGAGCAGC and CTGCCGTTACCAACATGG from the LIC-YFP-SOD2 plasmid generated above. PCR amplicon contained the L201P mutation (CTA->CCA) generated with Q5 Site-Directed Mutagenesis kit (New England Biolab), as with the following modifications. To screen for positive CRISPR-Cas9 clones containing L201P, two silent mutations were created to generate new and unique restriction endonuclease sites close to Leucine 201: AflI on Leucine 180 (CTC->CTT, 62 bps upstream of L201P) and XcmI on Serine 208 (TCA->TCT, 22 bps downstream of L201P). The PCR amplicon contained two additional deletions corresponding to the gRNA sites on endogenous SOD2 gene, in order to avoid SpCas9 protein cleaving the amplicon for HDR. The sequence AGAGACCCGGCGG (including the PAM sequence cgg) was deleted on the HDR sequence corresponding to gRNA #1, and sequence CACTTAACAGAGGGTC (including the PAM sequence agg) was deleted on the HDR sequence corresponding to gRNA #2.
The L201P point mutation was introduced into the TgSOD2.YFP line through CRISPR-Cas9 (Figure S1). Transfection of the CRISPR-CAS9 plasmid and the HDR DNA was carried out as published previously, with minor modifications (Shen et al., 2017). Briefly, 107 of freshly lysed parasites with SOD2-YFP were filtered and spun down at 400xg and 18⁰C for 10 min. The pellet was resuspended with Cytomix buffer (10 mM KPO4, 120 mM KCl, 5 mM MgCl2, 25 mM HEPES, 2 mM EDTA, 2µM ATP and 5µM glutathione) up to 800µl, including 7.5µg of CRISPR plasmid and 1.5µg of HDR DNA. The mixture was transfected using the ECM 630 Electro Cell Manipulator (BTX). Parasites were incubated into T25 flasks after electroporation and switched to 2 μM pyrimethamine selection 24 h later. Transfected pools remained in pyrimethamine selection for 3 days, and then switched to D10 media without selection for one more day before single cell cloning. The gDNA for isolated clones were collected by the DNeasy Blood and Tissue kit (Qiagen) and used to PCR amplify SOD2 with either primers SOD2 F/AmpR (for SOD2-YFP) or primers SOD E2/SOD R (for SOD2 only, Table S1). PCR amplicons were digested with XcmI for screening and positive clones were sequence verified.
Measurement of ROS Accumulation in T. gondii
To expose our parasites to oxidative stress, we harvested freshly lysed parasites and treated 5 × 104 parasites with 500 μM hydrogen peroxide (H2O2) for 30 min at 37°C, 5% CO2, with or without 1 μM auranofin After incubation, ROS was detected using H2DCDFC (Invitrogen). Each sample was incubated with 1 µM of H2DCDFC and examined under a Spectramax i5, (Molecular Devices California US; multimode microplate reader SoftMax Pro 7.0.2 Software) multimode microplate reader (excitation 494 nm; emission 522 nm) at 37°C for 15 min. For excitation, a single flash from a UV Xenon lamp was used for each well, and emission signals were recorded with a sensitivity setting of 100. The values are presented as relative fluorescence units. We compared ROS accumulation of each representative auranofin resistant T. gondii clone to that of wild-type parasites by an unpaired t-test in three independent experiments. A two-tailed p-value <0.05 was considered statistically significant.
Protein Alignment and Structural Models
The predicted amino acid sequences for TgSOD2 (TGGT1_316330) and TgRNR (TGGT1_294640) were submitted to the SwissModel site to identify the optimal available protein structures for modeling (Guex et al., 2009; Benkert et al., 2011; Bertoni et al., 2017; Bienert et al., 2017; Waterhouse et al., 2018). The Toxoplasma sequences were threaded onto the structure of Plasmodium knowlesi SOD (PDB 2AWP) (Vedadi et al., 2007) and human RNR (PDB 3HND) (Fairman et al., 2011). Clustal Omega was used to align the amino acid sequences and EMBOSS was used to calculate percent identity and similarity. Information on MS peptide coverage was obtained from the ToxoDB database (Gajria et al., 2008).
Expression Level of the Thioredoxin Reductase Gene in Mutants
We used qPCR to quantify the expression level of thioredoxin reductase gene in both wild type and mutant parasites. RNA was extracted from parasite lines using the RNeasy Mini Kit (Qiagen), and then used for cDNA synthesis via iScript cDNA Synthesis Kit (Bio-Rad). The resulting cDNA samples were then subjected to qPCR with iTaq Universal SYBR Green Supermix (Bio-Rad) with the following gene targets: actin (TGGT1_209030, ToxoDB.org), GAPDH1 (TGGT1_289690, ToxoDB.org), thioredoxin reductase (TGGT1_309730, ToxoDB.org), and superoxide dismutase 2 (TGGT1_316330, ToxoDB.org).
The actin gene was amplified from cDNA samples as one PCR fragment ~60bp, with primers CACGAGAGAGGATACGGCTTC and CGATGTCGCTAGAGTCCTCAG. The GAPDH1 gene was amplified in one PCR fragment ~120bp with primers CACTTTGCCAAGATGGTGTG and GACTGTCTTCAACGAGAAGGAG. The thioredoxin reductase gene was amplified a ~70bp PCR fragment using the primers CACAAACACGAACAACGGCG and GTGGACGGCTGAACAAAGTCG. For the ~50bp fragment in superoxide dismutase 2 gene, primers GGCACACCGTTCGCTGATAAG and CGTGGTTCCATGCTTGTGCTG were used.
In order to quantify the amount of target transcript present, target CT values were normalized against actin CT values. The resulting ΔCT values were then compared through 2-way ANOVA between the different parasite strains surveyed.
Results
AurRT. Gondii Lines Have a Growth Advantage Over Wild Type Parasites
To verify auranofin resistance of the selected clones, we adapted a previously described competition assay (Ma et al., 2008). This was used in place of a normal dose-response assay because at auranofin concentrations higher than 3 μM, the fibroblast monolayer begins to disrupt (data not shown), making the plaque assays not feasible. To confirm resistance to auranofin in the AurR T. gondii lines, we assessed their growth in competition with YFP-expressing RH strain parasites in control media and in media with 2.5–3.0 μM auranofin. In competition assays, the inoculating parasite population is composed of 50% YFP-expressing wild type (sensitive) parasites and 50% of an AurR line verified by flow cytometry. The relative contribution of the individual populations was measured after each cycle of lytic growth in serial culture. If a parasite line increases to >50% of the population, it exhibits a growth advantage over the competing line. In the absence of auranofin, AurR lines grew comparably or less well than the parental RH line that does not express YFP (Figure 1A). However, in the presence of 2.5 μM auranofin, all AurR lines displayed significant growth advantage over YFP-expressing wild-type RH parasites (Figure 1B). The observations indicated that day 6 is the optimal day to capture the growth advantage of resistant lines under auranofin selection because YFP-expressing wild-type parasites were eliminated from the culture. Given these results, we measured the ability of a larger set of AurR lines to compete with wild-type YFP-expressing RH strain parasites in the absence or presence of auranofin by quantifying their contribution to the culture at 6 days (Figure 2). These results show that 9 of the 10 AurR lines outcompete the sensitive YFP-expressing RH line in the presence of 2.5 μM auranofin. Line 6 has a distinct profile: it grows slowly in both control and auranofin media, suggesting that it may evade auranofin-mediated killing by protracted replication.
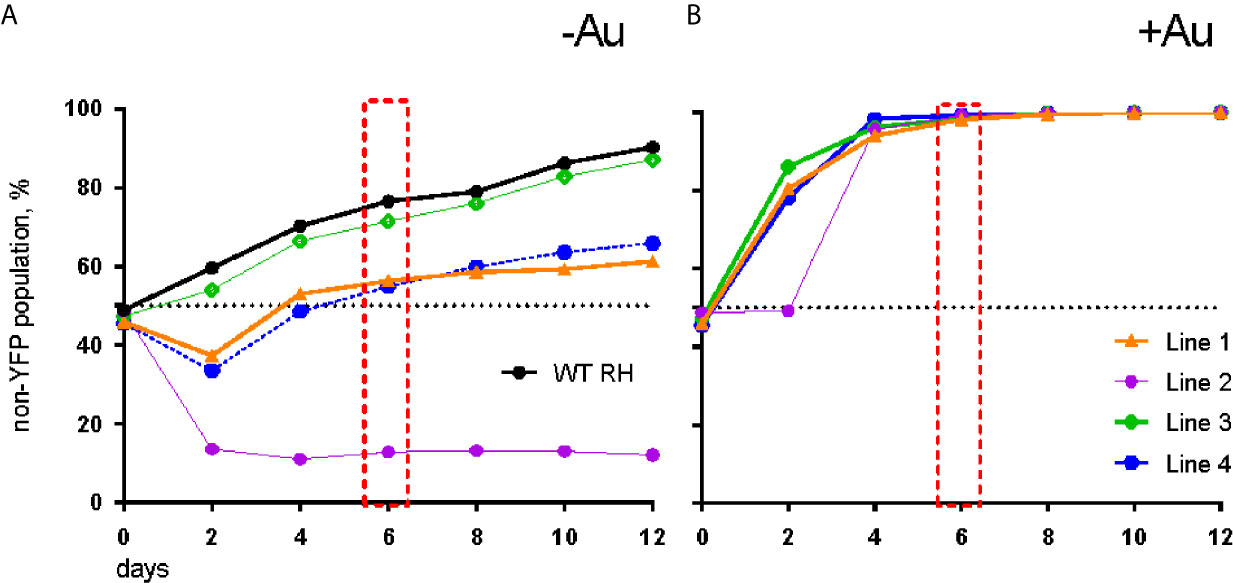
Figure 1 AurR lines have a growth advantage under selective pressure with auranofin. (A) In the absence of auranofin, individual resistant lines grow as well or less well than the parental RH strain. (B) In the presence of 3 µM auranofin, wild-type parasites are eliminated by day 6 (red box).
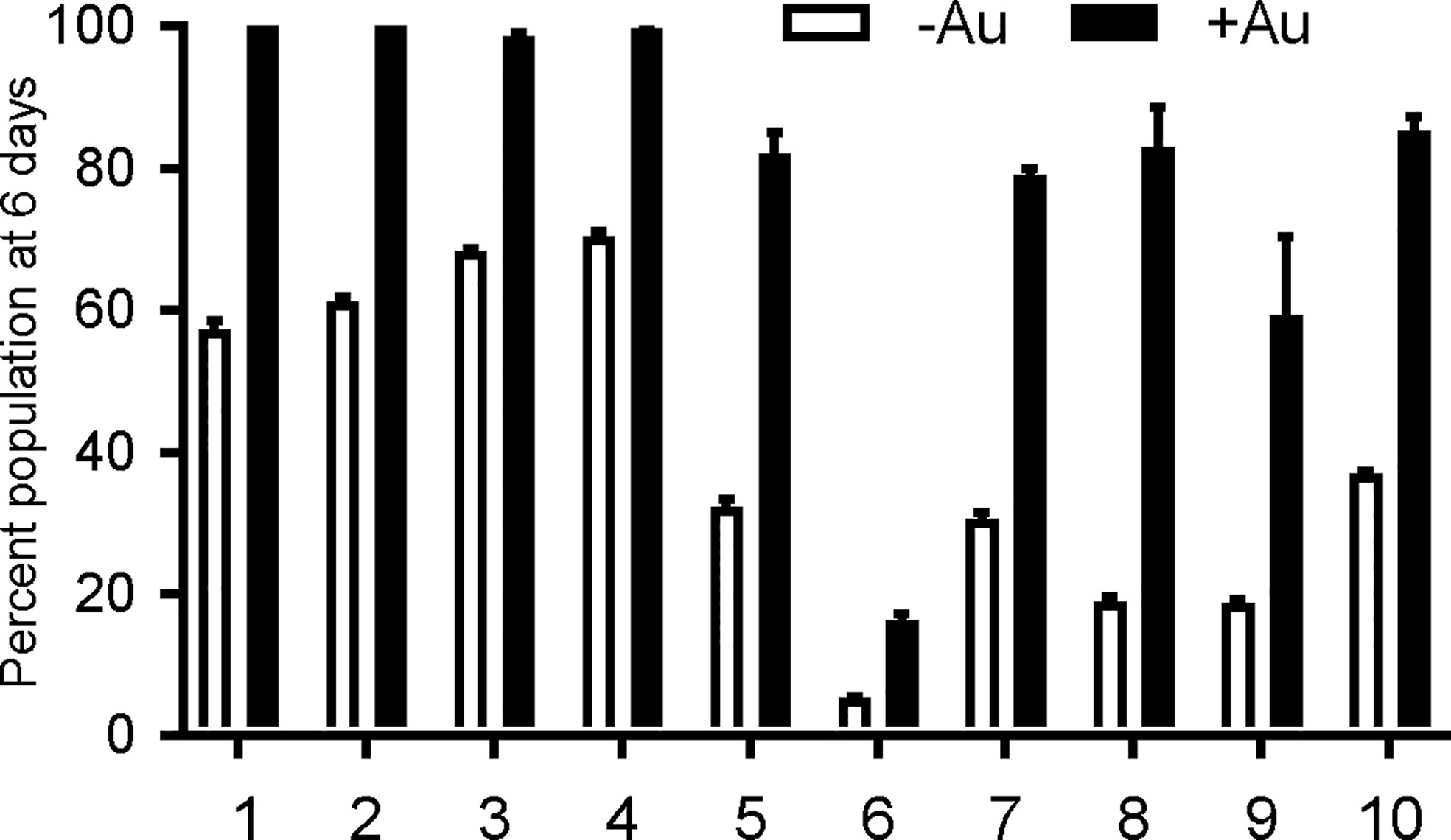
Figure 2 Resistant T. gondii lines outcompete wild type parasites under auranofin selection. AurR lines are represented as percentage of the total population of parasites after 6 days in competition with YFP-expressing wild type (sensitive) parasites, no drug media (white) and 2.5 uM auranofin (black). Mean difference between parasite population in the absence of auranofin and in the presence of auranofin: 40.6; SD 14.6; two-tailed unpaired t-test p < 0.001.
AurR Lines Do Not Harbor Mutations in the Thioredoxin Reductase Gene
Considering that thioredoxin reductase (Sannella et al., 2008; Angelucci et al., 2009; Debnath et al., 2012; Ilari et al., 2012) is the proposed target of auranofin, we hypothesized that resistance in T. gondii would develop through mutations to the parasite thioredoxin reductase gene (TgTrxR, TGGT1_309730). Therefore, we amplified and sequenced the TgTrxR gene in 53 lines derived from parallel (independent) selections (not shown). We did not detect mutations (SNVs) in the thioredoxin reductase gene of any of the lines, indicating that auranofin resistance does not arise through modifications to TgTrxR that directly influence auranofin binding or TgTrxR enzyme activity.
AurR Lines Accumulate Less ROS
The anti-parasitic activity of auranofin relies on its ability to induce accumulation of ROS in parasites (Debnath et al., 2012). We exposed wild type RH strain parasites and four representative AurR lines to hydrogen peroxide (H2O2) for 30 min before measuring ROS accumulation with dichloro-fluorescein (H2DCDFC). All AurR lines displayed decreased accumulation of ROS relative to the parental RH line in both the absence and presence of auranofin (Figure 3). For control, we also measured ROS accumulation in the absence of H2O2 and observed no fluorescence signal (data not shown). This negative control is consistent with a prior study measuring ROS accumulation using the same dichloro-fluorescein dye without the H2O2 (Lee et al., 2006).
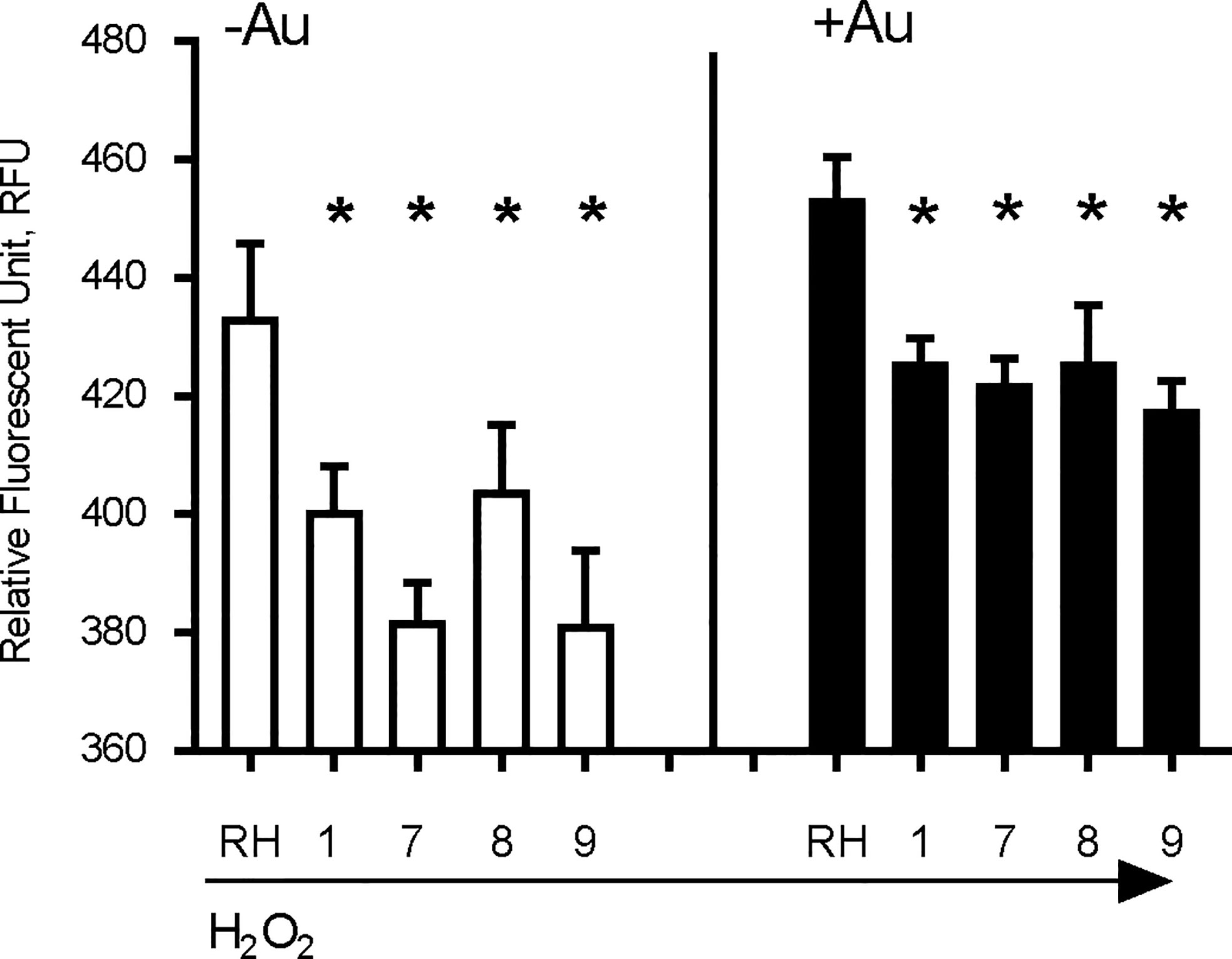
Figure 3 Auranofin-resistant T. gondii parasites accumulate less ROS. Freshly lysed wild-type parasites were treated with 500 μM hydrogen peroxide (H2O2) for 30 min and accumulation of reactive oxygen species (ROS) was assayed with dichloro-fluorescein H2DCDFC. Auranofin-resistant clones accumulated less ROS after H2O2 treatment compared to the wild-type RH parasites in the presence of H2O2 (white) or H2O2 + 1 µM auranofin (black). (*) p < 0.05 when compared to RH or to RH+ Auranofin (Au).
AurR Lines Harbor Diverse Mutations That Introduce Point Mutations
Given that we did not observe mutations in T. gondii thioredoxin reductase, we performed whole genome sequencing (WGS) analysis of 10 independent T. gondii AurR lines. Our goal was to determine whether these T. gondii parasites harbor a common mutation(s) that underlies resistance to auranofin. Analysis of coding sequences with 100% SNVs frequency and at least 50-fold coverage identified a variety of ~5 non-synonymous SNVs in the exons of each line. Selected SNVs observed in the WGS were validated by PCR amplification and Sanger sequencing (primers in Table S1). We chose to specifically investigate the AurR line 8 to consider which SNV was most likely to underlie auranofin resistance. Line 8 has 12 SNVs that introduce amino acid substitutions to diverse protein coding genes (Table 1). We used the community annotated ToxoDB database and BLAST analysis to investigate these loci. In particular, the availability of RNA-seq datasets permitted us to evaluate whether loci were expressed in the tachyzoite stage. In order to assess the significance of expressed loci, we used BLAST analysis to look for protein homologs and significant motifs and collected RNA-seq values and CRISPR screen scores for available loci. This information led us to focus on 2 of the loci (TGGT1_316330 and TGGT1_294640) as most likely resistance conferring. These loci encode a superoxide dismutase (SOD2) and the large subunit (R1) of ribonucleotide reductase (RNR). Both enzymes have strong fitness conferring effects in the deposited CRISPR screen data and well-conserved metabolic roles.
RNR removes the 2’-hydroxyl group of the ribose ring of nucleoside diphosphates to form deoxyribonucleotides from ribonucleotides which are used in DNA synthesis while SOD inactivates damaging superoxide radicals by converting them into molecular oxygen and hydrogen peroxide. Since both TgRNR and TgSOD2 are conserved proteins, we threaded the amino acid sequence onto homologous crystal structures to locate the position of the amino acid substitutions. TgSOD2 is a Fe-type SOD and was previously demonstrated to localize to the tachyzoite mitochondrion (Brydges and Carruthers, 2003; Pino et al., 2007). SODs form dimers with shared coordination of two iron (FeIII) cofactors. The L201P mutation is located near to the key iron-coordinating residues: H111, E249 and H160 (Figure 4A) and is located in an α-helix. Substitution of a proline at this site likely causes the helix to bend, perhaps altering SOD enzymatic properties. TgRNR aligns well with human ribonucleotide reductase 1 (61.7% identity and 78.2% similarity). However, there is a 59 amino acid N-terminal extension to the Toxoplasma protein, with the conserved sequence beginning with M60 (Figure 4B). This leader does not represent mis-annotation of the N-terminus of the protein: there are MS peptides mapping to this sequence, especially in samples that are from monomethylarginine and phosphopeptide-enriched datasets in ToxoDB. This is particularly interesting as arginine methylation is a modification associated with proteins that have roles in transcriptional regulation, RNA metabolism and DNA repair. RNR use free-radical chemistry to convert ribonucleotides into deoxyribonucleotides. Significantly, ribose reduction requires generation of a free radical and RNR requires electrons donated from thioredoxin. Moreover, superoxide has been shown to inactivate RNR and yeast lacking either cytoplasmic or mitochondrial SODs have increased susceptibility to oxidative stress and RNR inactivation. The L166Q mutation is in a solvent exposed loop (Figure 4C), that is poorly conserved and not known to contribute to enzyme interactions.
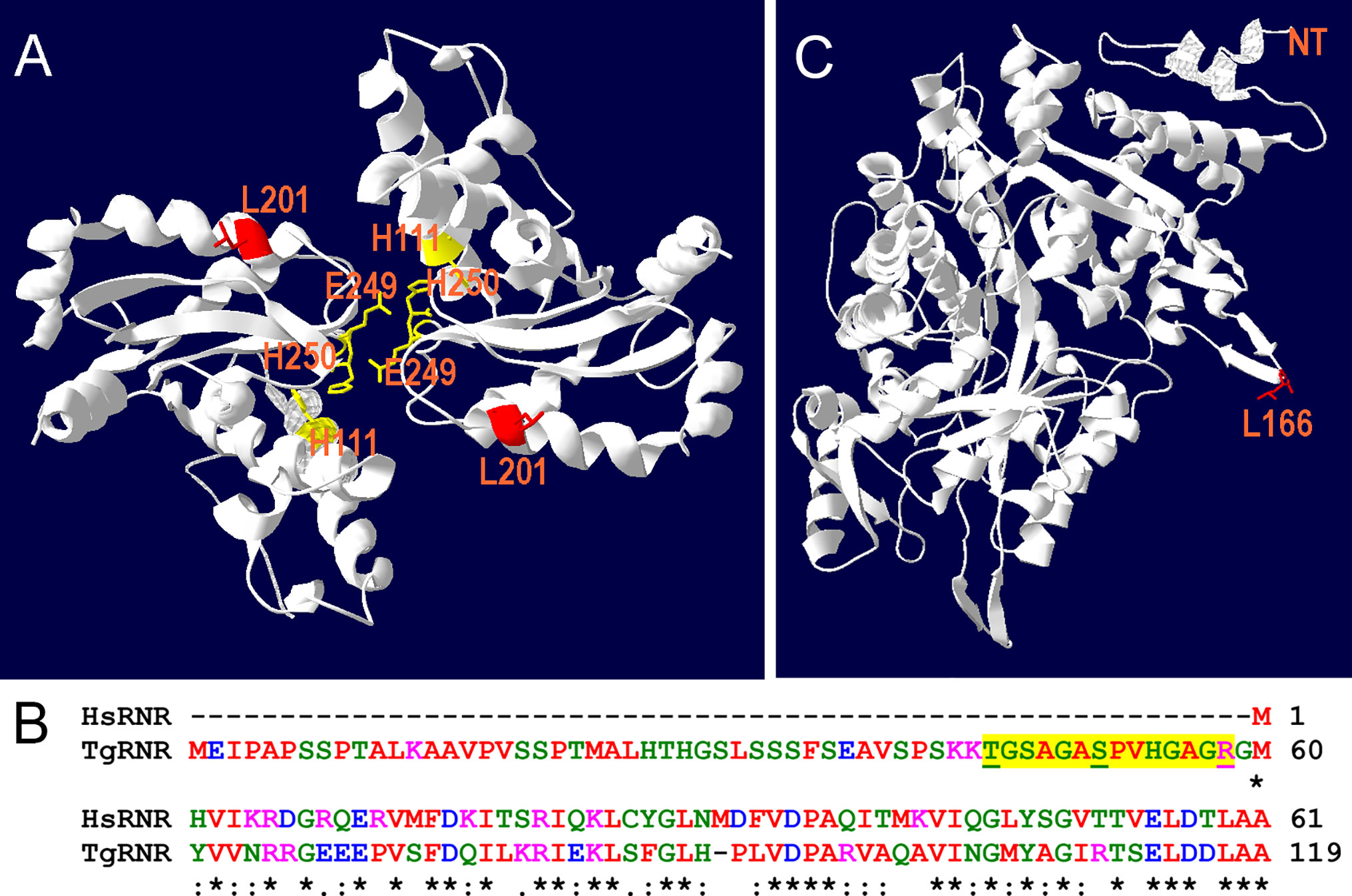
Figure 4 Location of substitutions in TgSOD2 and TgRNR. (A) SOD forms dimers that coordinately bind to iron cofactors at their interface. The Toxoplasma amino acid sequence was threaded onto a Plasmodium knowlesi protein structure (PDB 2AWP) to create a model for TgSOD2. The mutation at position 201 (red) is near to the end of an α-helix; substitution of a proline for leucine at this location likely causes the helix to bend. This mutation is also proximal to key iron-coordinating residues H111, E249 and H160. (B) Alignment of the Toxoplasma and human RNR sequences indicates that TgRNR has a novel 59 amino acid N-terminal extension. MS peptides (yellow highlight) in ToxoDB map to this sequence with S and T phosphorylated, and R monomethylated (underlined). (C) The Toxoplasma amino acid sequence for RNR was threaded onto the human RNR structure (PDB 3HND) to create a model for TgRNR. The L166Q mutation is in a solvent exposed loop, that is poorly conserved and not known to contribute to enzyme interactions.
Resistance to Auranofin Is Not Conferred by an Isolated Mutation in TgSOD2
The anti-parasitic effect of auranofin appears to stem from its molecule of gold, which is known to induce the accumulation of ROS in parasites (Debnath et al., 2012; Adeyemi et al., 2017). Since we hypothesized that a single mutation (SNV) suffices to generate resistance to auranofin, we analyzed the effect of the TgSOD2 L201P mutation on auranofin sensitivity. SOD2 is a bona fide enzymatic ROS scavenger and SOD2 is the sole mitochondrial superoxide dismutase in tachyzoites. To analyze the role of mutant SOD2 in auranofin resistance, we generated a T. gondii clone with a knock-in YFP tag for SOD2 that localizes to the tachyzoite mitochondrion as confirmed by co-localization with the mitochondrial marker F1B ATPase (Figure 5A). Subsequently, we generated two T. gondii SOD2.L201P lines: one lacking the YFP tag and one tagged with a C-terminal YFP fusion (Figure S1). Both lines were corroborated by Sanger sequencing. In the absence of Auranofin, both L201P lines grew less well than the TgSOD2-YFP wild-type line but were more fit than AurR line 8 (Figure 5B). Previous attempts to create SOD1 and SOD2 gene deletions failed (Odberg-Ferragut et al., 2000) and SOD2 is a fitness-conferring locus in a genome-wide CRISPR/Cas screen (Sidik et al., 2016). It is noteworthy that the L201P mutation is associated with reduced fitness in the absence of superoxide stress (control conditions). In the presence of auranofin, only line 8 grows well, indicating that the single L201P mutation to TgSOD2 does not confer resistance. This may indicate that this substitution does not influence superoxide metabolism at all or that auranofin resistance also requires the mutant TgRNR enzyme. It is unlikely that these enzymes directly interact, as RNR enzymes are cytosolic while TgSOD2 is in the mitochondrial compartment. However, the SOD2.L201P T. gondii line accumulated ROS similarly to wild-type parasites, consistent with its auranofin-sensitive growth (Figure 5C).
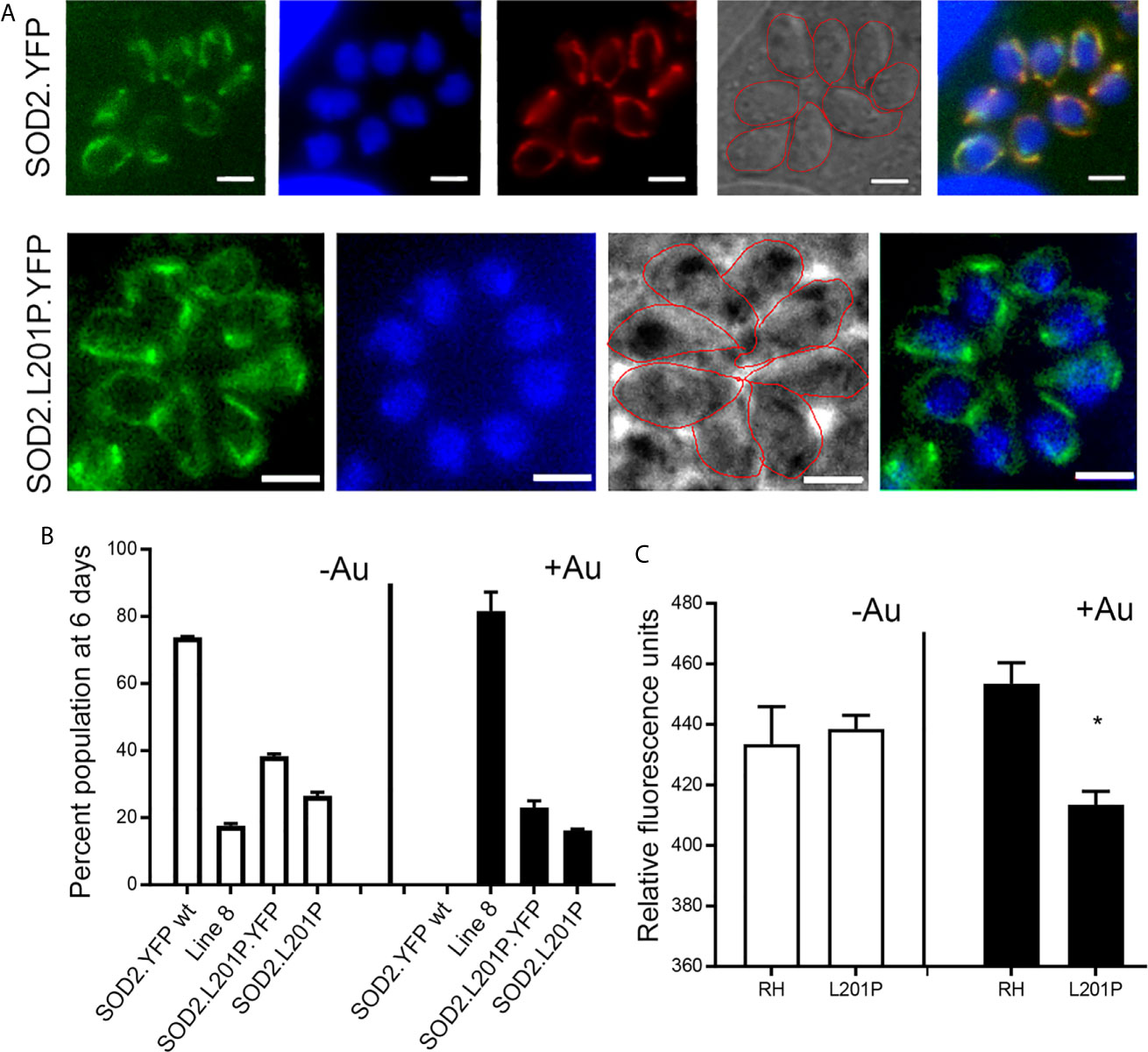
Figure 5 A L201P mutation in TgSOD2 does not alter localization of TgSOD2 or confer resistance to auranofin. (A) Wild type and mutant SOD2-YFP exhibit a mitochondrial distribution. Mitochondrion was stained red with the anti-F1B ATPase. Scale bar: 5 µm. (B) Growth competition assays indicate that the L201P mutation is associated with reduced fitness in the absence of auranofin (control conditions, white bars). In the presence of auranofin (black bars), only line 8 shows growth advantage, indicating that the single L201P mutation to TgSOD2 does not confer resistance. (C) In the absence of auranofin, the SOD2.L201P line accumulated ROS similarly to wild type parasites, consistent with its auranofin-sensitive growth; however, it accumulated less ROS in the presence of auranofin. *p < 0.05 when compared to RH in auranofin (+Au).
Discussion
Auranofin, is an FDA-approved drug for treatment of rheumatoid arthritis. More recently it has been proposed to be a promising anti-parasitic agent with activity against diverse parasites with public health significance. Despite this promising broad spectrum of anti-parasitic activity, the molecular target of auranofin has only been indirectly implicated as thioredoxin reductase.
We previously demonstrated that auranofin decreases the parasite burden during a systemic infection with T. gondii using a chicken embryo model of acute toxoplasmosis (Andrade et al., 2014). Our preliminary observations suggest that auranofin exerts a dual effect: it modulates both parasite proliferation and host immunopathology. Herein, we report isolation and characterization of auranofin-resistant T. gondii lines. None of our resistant lines have mutations in the thioredoxin reductase gene. This may indicate that this enzyme is not the molecular target of auranofin in T. gondii; alternately, it may indicate that thioredoxin reductase cannot tolerate resistance-conferring mutations or is not the sole significant target in this organism. During these investigations, we monitored the activity of auranofin on wild type parasites as a control condition relative to the resistant lines. Our observations suggest that auranofin enhances cumulative free radical damage, ultimately causing parasite death. Each of our auranofin resistant T. gondii lines carry several mutations (SNVs), suggesting a high threshold to develop auranofin resistance. This is a desirable characteristic for drug development. Importantly, resistant lines accumulate less reactive oxygen species (ROS) than wild-type parasites.
Chemical mutagenesis is a well-established and robust approach [i.e., (McFadden et al., 2000; Nagamune et al., 2007)] to identify the molecular targets of candidate anti-T. gondii drugs. This approach is also relevant to anticipating mechanisms of drug resistance. We isolated auranofin-resistant T. gondii lines derived from the RH strain. Resistant lines have increased replication relative to wild type RH parasites in competition assays carried out in the presence of auranofin. Lines with ≤5 accumulated SNVs (lines 1–4) appear to have a growth advantage over wild-type parasites in the absence auranofin. This probably reflects a fitness defect induced by expression of YFP in wild type parasites (Ma et al., 2008); these lines likely grow comparably to non-YFP expressing wild type parasites without auranofin present. In contrast, resistant lines that have >5 SNVs each compete poorly with wild type parasites in the absence of selection, suggesting that accumulation of SNVs comes at a cost to parasite fitness. An alternative explanation to the isolation of multiple SNVs in each resistant clone is that auranofin interferes with many processes and development of resistance requires alterations to more than one target. Another possibility to consider is that the expression level of thioredoxin reductase could change with auranofin treatment. We measured the transcript levels of two redox genes, TrxR and SOD2, in both wild type and mutant line 8 (Figure S2). Auranofin did not induce significant changes in the expression level of either TrxR or SOD2. While changes in TrxR transcription were not detected in the mutant line, it is possible that overexpression could lead to development of resistance. Future studies could investigate whether overexpression of thioredoxin reductase alone is sufficient to confer resistance to auranofin.
Auranofin contains a molecule of gold in a 3,4,5-Triacetyloxy-6-sulfanyl-oxan-2-yl methyl ethanoate scaffold and has been proposed to be a pro-drug (Chircorian and Barrios, 2004) that delivers gold to dithiol groups, like those found in proteins that bear thioredoxin-containing domains (Saccoccia et al., 2012). Although auranofin inhibits mammalian thioredoxin reductase (Gromer et al., 1998), the source of its anti-inflammatory activity remains ill-defined and may reflect inhibition of multiple targets. For example, macrophages decrease production of nitric oxide and pro-inflammatory cytokines in response to auranofin, which also inhibits cyclooxygenase-2 -dependent prostaglandin E2 production (Yamashita et al., 2003). Previous studies using the parasite enzymes trypanothione reductase and glutathione-thioredoxin reductase incubated with auranofin demonstrated that co-crystals harbor gold bound to reactive cysteines in these proteins (Angelucci et al., 2009; Ilari et al., 2012; Parsonage et al., 2016). In addition, S. mansoni worms with decreased expression of thioredoxin glutathione reductase (SmTGR) exhibit diminished parasite viability upon exposure to auranofin. E. histolytica treated with auranofin upregulate transcripts of a gene encoding a protein resembling an arsenite-inducible RNA-associated protein (AIRAP) (Debnath et al., 2012). Interestingly, both arsenite and auranofin are inhibitors of thioredoxin reductase, implying that EhTrxR is a target of auranofin. Studies in both S. mansoni and E. histolytica corroborated the interaction of thioredoxin reductase with auranofin by mobility shift assays or other in vitro measures (Kuntz et al., 2007; Debnath et al., 2012). In these cases, the approach involved target validation guided by the chemical properties of gold rather than target identification through genetic resistance.
It was surprising to not identify changes to the thioredoxin reductase gene, given previous studies that implicate antioxidant enzymes containing thioredoxin or thioredoxin-like domains as a target for auranofin in parasites. However, although S. mansoni and E. histolytica thioredoxin reductase antioxidant systems are essential for survival, a previous study indicates that T. gondii thioredoxin reductase is necessary for ROS metabolism (Xue et al., 2017). It should be noted that infection with 106 TgTrxR knockout parasites increases survival in mice by approximately 2 weeks relative to the parental RH line (Xue et al., 2017). In vitro, the null line exhibits reduced antioxidant capacity, invasion efficiency, and proliferation (Xue et al., 2017). These observations are in-line with the CRISPR fitness score for thioredoxin reductase (-1.98) which places it as fitness-conferring but not essential.
Among the significant SNVs found in our auranofin resistant T. gondii clones, we directed our attention at a point mutation to the superoxide dismutase 2 (SOD2) gene. SOD2 is a bona fide enzymatic antioxidant system that catalyzes the dismutation of superoxide anions into hydrogen peroxide and oxygen. The Toxoplasma genome contains three SOD genes. TgSOD1 is expressed in the cytoplasm of tachyzoites and TgSOD2 is located in the mitochondrion; TgSOD3 is specifically expressed in oocysts. TgSOD2 is a critical enzyme for tachyzoite survival: previous efforts to generate a TgSOD2 knockout line were not successful and its CRISPR fitness score for (-4.09) indicates that it is strongly fitness conferring and likely essential (Odberg-Ferragut et al., 2000). We hypothesized that the L201P mutation in TgSOD2 might increase its capacity to convert superoxide into hydrogen peroxide and oxygen to confer auranofin resistance. To test this hypothesis, we generated several lines that harbor knock-in tag to wild type SOD2 and engineered both YFP-tagged and untagged lines that bear the L201P mutation. Consistent with previous characterization of TgSOD2 (Pino et al., 2007), both wild type and L201P TgSOD2-YFP lines exhibit a mitochondrial distribution. However, neither the tagged or untagged L201P TgSOD2 lines exhibit increased growth in 2.5 uM auranofin, indicating that the single TgSOD2 mutation cannot confer resistance to this level of auranofin or reduce accumulation of ROS in our assay.
In addition to the L201P TgSOD2 mutation, AurR line 8 harbors a mutation in the large subunit of TgRNR, an essential enzyme which generates deoxyribonucleotides that are required for genome duplication and parasite replication. TgRNR has a 59 amino acid N-terminal extension relative to other RNRs. Peptides from this extension are represented in phosphorylated and monomethylated MS datasets, suggesting that this domain may regulate RNR activity. RNRs perform free-radical chemistry, require electrons donated from thioredoxin and are susceptible to superoxide inactivation. Although the L166Q mutation is in an area that is not known to contribute to enzyme interactions, it may play a role diminishing TgRNR susceptibility to superoxide inactivation. While this mutation may indirectly contribute to resistance to superoxide, RNR enzymes do not directly contribute to redox homeostasis and the observed reduced accumulation of ROS in the AurR lines.
Our previous and current findings suggest that auranofin decreases T. gondii viability through oxidative stress (Andrade et al., 2014). AurR lines exhibit an increased ability to scavenge ROS. We carried out preliminary studies to evaluate whether auranofin resistant clones were resilient to the hydrogen peroxide damage. Since our observations were highly variable, we are currently generating parasites expressing redox sensors. The genetic studies presented here did not identify a consistent mechanism for acquisition of resistance by point mutations to a specific protein target. Our future studies will examine whether there are transcriptional, translational or post-translational changes that contribute to auranofin resistance in these lines. For example, difference-gel electrophoresis and mass spectrometry have been used to identify differentially expressed proteins in sulfadiazine-resistant T. gondii isolates. While it was disappointing to not identify a single molecular target for auranofin in T. gondii in our studies, this result likely indicates that an enhanced ability to scavenge ROS in the presence of auranofin requires multiple changes to the parasite genome. Therefore, if auranofin is repurposed as an anti-parasitic treatment, it would likely present an increased threshold to resistance in clinical contexts.
Data Availability Statement
The sequencing data are publicly available at NCBI’s BioProject, with the accession # PRJNA679812.
Author Contributions
CM and JT collected, contributed, and performed the analysis of the data and drafted the paper. SJ and SS collected and contributed the data. SR, YD, and JZ collected the data. NM conceived the analysis and edited the paper. RA conceived and designed the analysis, collected the data, performed the analysis and wrote the paper, and obtained funding. All authors contributed to the article and approved the submitted version.
Funding
RA was supported by NIHK08 5K08AI102989-04; AMFDP RWJF 70642 (the views expressed here do not necessarily reflect the views of the Foundation) and UCI DOM Chair Research Award 60244. This work was made possible, in part, through access to the Genomics High Throughput Facility Shared Resource of the Cancer Center Support Grant (P30CA-062203) at the University of California, Irvine and NIH shared instrumentation grants 1S10RR025496-01, 1S10OD010794-01, and 1S10OD021718-01.
Conflict of Interest
The authors declare that the research was conducted in the absence of any commercial or financial relationships that could be construed as a potential conflict of interest.
Acknowledgments
Special gratitude to Dr. Melissa Lodoen (UCI) for her advice and critical review of this article and to Dr. Edward Brignole (MIT) for his thoughts on the TgRNR mutation. We also thank Dr. Peter Bradley (UCLA) for sharing the F1B ATPase antibody for mitochondria microscopy.
Supplementary Material
The Supplementary Material for this article can be found online at: https://www.frontiersin.org/articles/10.3389/fcimb.2021.618994/full#supplementary-material
Supplementary Figure 1 | CRISPR-Cas9 strategy for generating the T. gondii SOD2.L201P line. TgSOD2-YFP parasites incorporating the LIC plasmid (blue line) undergo CRISPR-Cas9 mediated homologous directed repair (HDR). The DNA amplicon used for HDR contains the L201P mutation (gold vertical line in exon 3) and two silent and unique restriction sites AflI and XcmI (black vertical lines in exon 3), which allow for screening of positive clones. Sequences corresponding to the two gRNA were deleted from the DNA for HDR (Δg1 and Δg2, golden triangles). Each of the gRNA was present twice in TgSDO2-YFP parasites (g1a/b, g2a/b; red font), resulting in two T. gondii SOD2.L201P lines. For HDR after g1a/g2a cuts, a T. gondii SOD2.YFP.L201P line is generated (lower left). For HDR after g1a/g2b cuts, a T. gondii SOD2.L201P line without YFP or LIC plasmid (blue) is generated (lower right). Positive clones were screened with XcmI digest before sequence verification.
Supplementary Figure 2 | Auranofin treatment does not change the expression of thioredoxin reductase nor superoxide dismutase 2 transcripts. RNA was harvested from freshly lysed parasites incubated in either control or 1µM auranofin containing media. Relative expression of SOD2 and TrxR genes normalized to actin. Parasites with or without exposure to auranofin, expressed similar transcripts amounts of TrxR and SOD2 (p>0.05, Mann-Whitney U-Test). There were no statistical differences. This manuscript has been released as a pre-print at https://www.biorxiv.org/as (Ma C et al., 2020).
Supplementary Table 1 | Primers for SNV validation.
References
Adeyemi, O. S., Murata, Y., Sugi, T., Kato, K. (2017). Inorganic nanoparticles kill Toxoplasma gondii via changes in redox status and mitochondrial membrane potential. Int. J. Nanomed. 12, 1647–1661. doi: 10.2147/IJN.S122178
Andrade, R. M., Chaparro, J. D., Capparelli, E., Reed, S. L. (2014). Auranofin is highly efficacious against Toxoplasma gondii in vitro and in an in vivo experimental model of acute toxoplasmosis. PloS Negl. Trop. Dis. 8, e2973. doi: 10.1371/journal.pntd.0002973
Angelucci, F., Sayed, A. A., Williams, D. L., Boumis, G., Brunori, M., Dimastrogiovanni, D., et al. (2009). Inhibition of Schistosoma mansoni thioredoxin-glutathione reductase by auranofin: structural and kinetic aspects. J. Biol. Chem. 284, 28977–28985. doi: 10.1074/jbc.M109.020701
Benkert, P., Biasini, M., Schwede, T. (2011). Toward the estimation of the absolute quality of individual protein structure models. Bioinformatics 27, 343–350. doi: 10.1093/bioinformatics/btq662
Bertoni, M., Kiefer, F., Biasini, M., Bordoli, L., Schwede, T. (2017). Modeling protein quaternary structure of homo- and hetero-oligomers beyond binary interactions by homology. Sci. Rep. 7, 10480. doi: 10.1038/s41598-017-09654-8
Bienert, S., Waterhouse, A., de Beer, T. A., Tauriello, G., Studer, G., Bordoli, L., et al. (2017). The SWISS-MODEL Repository-new features and functionality. Nucleic Acids Res. 45, D313–D319. doi: 10.1093/nar/gkw1132
Brydges, S. D., Carruthers, V. B. (2003). Mutation of an unusual mitochondrial targeting sequence of SODB2 produces multiple targeting fates in Toxoplasma gondii. J. Cell Sci. 116, 4675–4685. doi: 10.1242/jcs.00750
Bulman, C. A., Bidlow, C. M., Lustigman, S., Cho-Ngwa, F., Williams, D., Rascon, A. A., Jr., et al. (2015). Repurposing auranofin as a lead candidate for treatment of lymphatic filariasis and onchocerciasis. PloS Negl. Trop. Dis. 9, e0003534. doi: 10.1371/journal.pntd.0003534
Chircorian, A., Barrios, A. M. (2004). Inhibition of lysosomal cysteine proteases by chrysotherapeutic compounds: a possible mechanism for the antiarthritic activity of Au(I). Bioorg Med. Chem. Lett. 14, 5113–5116. doi: 10.1016/j.bmcl.2004.07.073
da Silva, M. T., Silva-Jardim, I., Portapilla, G. B., de Lima, G. M., Costa, F. C., Anibal Fde, F., et al. (2016). In vivo and in vitro auranofin activity against Trypanosoma cruzi: Possible new uses for an old drug. Exp. Parasitol. 166, 189–193. doi: 10.1016/j.exppara.2015.05.012
Debnath, A., Parsonage, D., Andrade, R. M., He, C., Cobo, E. R., Hirata, K., et al. (2012). A high-throughput drug screen for Entamoeba histolytica identifies a new lead and target. Nat. Med. 18, 956–960. doi: 10.1038/nm.2758
Debnath, A., Ndao, M., Reed, S. L. (2013). Reprofiled drug targets ancient protozoans: drug discovery for parasitic diarrheal diseases. Gut Microbes 4, 66–71. doi: 10.4161/gmic.22596
Fairman, J. W., Wijerathna, S. R., Ahmad, M. F., Xu, H., Nakano, R., Jha, S., et al. (2011). Structural basis for allosteric regulation of human ribonucleotide reductase by nucleotide-induced oligomerization. Nat. Struct. Mol. Biol. 18, 316–322. doi: 10.1038/nsmb.2007
Fox, B. A., Ristuccia, J. G., Gigley, J. P., Bzik, D. J. (2009). Efficient gene replacements in Toxoplasma gondii strains deficient for nonhomologous end joining. Eukaryot Cell 8, 520–529. doi: 10.1128/EC.00357-08
Gajria, B., Bahl, A., Brestelli, J., Dommer, J., Fischer, S., Gao, X., et al. (2008). ToxoDB: an integrated Toxoplasma gondii database resource. Nucleic Acids Res. 36, D553–D556. doi: 10.1093/nar/gkm981
Gromer, S., Arscott, L. D., Williams, C. H., Jr., Schirmer, R. H., Becker, K. (1998). Human placenta thioredoxin reductase. Isolation of the selenoenzyme, steady state kinetics, and inhibition by therapeutic gold compounds. J. Biol. Chem. 273, 20096–20101. doi: 10.1074/jbc.273.32.20096
Gubbels, M. J., Li, C., Striepen, B. (2003). High-throughput growth assay for Toxoplasma gondii using yellow fluorescent protein. Antimicrob. Agents Chemother. 47, 309–316. doi: 10.1128/AAC.47.1.309-316.2003
Guex, N., Peitsch, M. C., Schwede, T. (2009). Automated comparative protein structure modeling with SWISS-MODEL and Swiss-PdbViewer: a historical perspective. Electrophoresis 30 Suppl 1, S162–S173. doi: 10.1002/elps.200900140
Hopper, M., Yun, J. F., Zhou, B., Le, C., Kehoe, K., Le, R., et al. (2016). Auranofin inactivates Trichomonas vaginalis thioredoxin reductase and is effective against trichomonads in vitro and in vivo. Int. J. Antimicrob. Agents 48, 690–694. doi: 10.1016/j.ijantimicag.2016.09.020
Huynh, M. H., Carruthers, V. B. (2009). Tagging of endogenous genes in a Toxoplasma gondii strain lacking Ku80. Eukaryot Cell 8, 530–539. doi: 10.1128/EC.00358-08
Ilari, A., Baiocco, P., Messori, L., Fiorillo, A., Boffi, A., Gramiccia, M., et al. (2012). A gold-containing drug against parasitic polyamine metabolism: the X-ray structure of trypanothione reductase from Leishmania infantum in complex with auranofin reveals a dual mechanism of enzyme inhibition. Amino Acids 42, 803–811. doi: 10.1007/s00726-011-0997-9
Kobayashi, H., Takeno, M., Saito, T., Takeda, Y., Kirino, Y., Noyori, K., et al. (2006). Regulatory role of heme oxygenase 1 in inflammation of rheumatoid arthritis. Arthritis Rheum. 54, 1132–1142. doi: 10.1002/art.21754
Kuntz, A. N., Davioud-Charvet, E., Sayed, A. A., Califf, L. L., Dessolin, J., Arner, E. S., et al. (2007). Thioredoxin glutathione reductase from Schistosoma mansoni: an essential parasite enzyme and a key drug target. PloS Med. 4, e206. doi: 10.1371/journal.pmed.0040206
Lee, D. H., Lim, B. S., Lee, Y. K., Yang, H. C. (2006). Effects of hydrogen peroxide (H2O2) on alkaline phosphatase activity and matrix mineralization of odontoblast and osteoblast cell lines. Cell Biol. Toxicol. 22, 39–46. doi: 10.1007/s10565-006-0018-z
Long, S., Wang, Q., Sibley, L. D. (2016). Analysis of Noncanonical Calcium-Dependent Protein Kinases in Toxoplasma gondii by Targeted Gene Deletion Using CRISPR/Cas9. Infect. Immun. 84, 1262–1273. doi: 10.1128/IAI.01173-15
Ma CI, T. J., Jan, S., Schweizer, S. S., Rosario, S. A. C., Du, Y., Zhang, J. J., et al. (2020). Auranofin resistance in Toxoplasma gondii decreases the accumulation of reactive oxygen species but does not target parasite thioredoxin reductase. bioRxiv [Preprint]. doi: 10.1101/2020.04.23.058842 20200423058842.
Ma, C., Tran, J., Li, C., Ganesan, L., Wood, D., Morrissette, N. (2008). Secondary mutations correct fitness defects in Toxoplasma gondii with dinitroaniline resistance mutations. Genetics 180, 845–856. doi: 10.1534/genetics.108.092494
Martinez-Gonzalez, J. J., Guevara-Flores, A., Alvarez, G., Rendon-Gomez, J. L., Del Arenal, I. P. (2010). In vitro killing action of auranofin on Taenia crassiceps metacestode (cysticerci) and inactivation of thioredoxin-glutathione reductase (TGR). Parasitol. Res. 107, 227–231. doi: 10.1007/s00436-010-1867-1
McFadden, D. C., Tomavo, S., Berry, E. A., Boothroyd, J. C. (2000). Characterization of cytochrome b from Toxoplasma gondii and Q(o) domain mutations as a mechanism of atovaquone-resistance. Mol. Biochem. Parasitol. 108, 1–12. doi: 10.1016/S0166-6851(00)00184-5
Nagamune, K., Moreno, S. N., Sibley, L. D. (2007). Artemisinin-resistant mutants of Toxoplasma gondii have altered calcium homeostasis. Antimicrob. Agents Chemother. 51, 3816–3823. doi: 10.1128/AAC.00582-07
Odberg-Ferragut, C., Renault, J. P., Viscogliosi, E., Toursel, C., Briche, I., Engels, A., et al. (2000). Molecular cloning, expression analysis and iron metal cofactor characterisation of a superoxide dismutase from Toxoplasma gondii. Mol. Biochem. Parasitol. 106, 121–129. doi: 10.1016/S0166-6851(99)00211-X
Parsonage, D., Sheng, F., Hirata, K., Debnath, A., McKerrow, J. H., Reed, S. L., et al. (2016). X-ray structures of thioredoxin and thioredoxin reductase from Entamoeba histolytica and prevailing hypothesis of the mechanism of Auranofin action. J. Struct. Biol. 194, 180–190. doi: 10.1016/j.jsb.2016.02.015
Peroutka-Bigus, N., Bellaire, B. H. (2019). Antiparasitic Activity of Auranofin against Pathogenic Naegleria fowleri. J. Eukaryot. Microbiol. 66, 684–688. doi: 10.1111/jeu.12706
Pfefferkorn, E. R., Pfefferkorn, L. C. (1979). Quantitative studies of the mutagenesis of Toxoplasma gondii. J. Parasitol. 65, 364–370. doi: 10.2307/3280274
Pino, P., Foth, B. J., Kwok, L. Y., Sheiner, L., Schepers, R., Soldati, T., et al. (2007). Dual targeting of antioxidant and metabolic enzymes to the mitochondrion and the apicoplast of Toxoplasma gondii. PloS Pathog. 3, e115. doi: 10.1371/journal.ppat.0030115
Saccoccia, F., Angelucci, F., Boumis, G., Brunori, M., Miele, A. E., Williams, D. L., et al. (2012). On the mechanism and rate of gold incorporation into thiol-dependent flavoreductases. J. Inorg. Biochem. 108, 105–111. doi: 10.1016/j.jinorgbio.2011.11.005
Sannella, A. R., Casini, A., Gabbiani, C., Messori, L., Bilia, A. R., Vincieri, F. F., et al. (2008). New uses for old drugs. Auranofin, a clinically established antiarthritic metallodrug, exhibits potent antimalarial effects in vitro: Mechanistic and pharmacological implications. FEBS Lett. 582, 844–847. doi: 10.1016/j.febslet.2008.02.028
Shen, B., Brown, K., Long, S., Sibley, L. D. (2017). Development of CRISPR/Cas9 for Efficient Genome Editing in Toxoplasma gondii. Methods Mol. Biol. 1498, 79–103. doi: 10.1007/978-1-4939-6472-7_6
Sidik, S. M., Huet, D., Ganesan, S. M., Huynh, M. H., Wang, T., Nasamu, A. S., et al. (2016). A Genome-wide CRISPR Screen in Toxoplasma Identifies Essential Apicomplexan Genes. Cell 166, 1423–1435 e12. doi: 10.1016/j.cell.2016.08.019
Tejman-Yarden, N., Miyamoto, Y., Leitsch, D., Santini, J., Debnath, A., Gut, J., et al. (2013). A reprofiled drug, auranofin, is effective against metronidazole-resistant Giardia lamblia. Antimicrob. Agents Chemother. 57, 2029–2035. doi: 10.1128/AAC.01675-12
Vedadi, M., Lew, J., Artz, J., Amani, M., Zhao, Y., Dong, A., et al. (2007). Genome-scale protein expression and structural biology of Plasmodium falciparum and related Apicomplexan organisms. Mol. Biochem. Parasitol. 151, 100–110. doi: 10.1016/j.molbiopara.2006.10.011
Waterhouse, A., Bertoni, M., Bienert, S., Studer, G., Tauriello, G., Gumienny, R., et al. (2018). SWISS-MODEL: homology modelling of protein structures and complexes. Nucleic Acids Res. 46, W296–W303. doi: 10.1093/nar/gky427
Xue, J., Jiang, W., Chen, Y., Gong, F., Wang, M., Zeng, P., et al. (2017). Thioredoxin reductase from Toxoplasma gondii: an essential virulence effector with antioxidant function. FASEB J. 31, 4447–4457. doi: 10.1096/fj.201700008R
Keywords: gold, repurposing, anti-parasitic, resistance, redox, Toxoplasma, auranofin, superoxide
Citation: Ma CI, Tirtorahardjo JA, Jan S, Schweizer SS, Rosario SAC, Du Y, Zhang JJ, Morrissette NS and Andrade RM (2021) Auranofin Resistance in Toxoplasma gondii Decreases the Accumulation of Reactive Oxygen Species but Does Not Target Parasite Thioredoxin Reductase. Front. Cell. Infect. Microbiol. 11:618994. doi: 10.3389/fcimb.2021.618994
Received: 19 October 2020; Accepted: 07 January 2021;
Published: 19 March 2021.
Edited by:
Loïc Riviere, Université de Bordeaux, FranceReviewed by:
Nathaniel Gadsby Jones, University of York, United KingdomTravis Bourret, Creighton University, United States
Copyright © 2021 Ma, Tirtorahardjo, Jan, Schweizer, Rosario, Du, Zhang, Morrissette and Andrade. This is an open-access article distributed under the terms of the Creative Commons Attribution License (CC BY). The use, distribution or reproduction in other forums is permitted, provided the original author(s) and the copyright owner(s) are credited and that the original publication in this journal is cited, in accordance with accepted academic practice. No use, distribution or reproduction is permitted which does not comply with these terms.
*Correspondence: Rosa M. Andrade, cm1hbmRyYTFAdWNpLmVkdQ==
†These authors have contributed equally to this work