- 1Key Laboratory of Pathobiology, Ministry of Education, Norman Bethune College of Medicine, Jilin University, Changchun, China
- 2School of Life Sciences, Henan University, Kaifeng, China
Radiotherapy is an important treatment for abdominal tumors. A critical side effect for this therapy is enteritis. In this review, we aim to summarize recent findings in radiation enteritis, in particular the role of gut microbiota dysbiosis in the development and therapy of the disease. Gut microbiota dysbiosis plays an important role in the occurrence of various diseases, such as radiation enteritis. Abdominal radiation results in changes in the composition of microbiota and reduces its diversity, which is mainly reflected in the decrease of Lactobacillus spp. and Bifidobacterium spp. and increase of Escherichia coli and Staphylococcus spp. Gut microbiota dysbiosis aggravates radiation enteritis, weakens intestinal epithelial barrier function, and promotes inflammatory factor expression. Pathogenic Escherichia coli induce the rearrangement and redistribution of claudin-1, occludin, and ZO-1 in tight junctions, a critical component in intestinal epithelial barrier. In view of the role that microbiome plays in radiation enteritis, we believe that intestinal flora could be a potential biomarker for the disease. Correction of microbiome by application of probiotics, fecal microbiota transplantation (FMT), and antibiotics could be an effective method for the prevention and treatment of radiation-induced enteritis.
Highlights
In this article, we reviewed the alteration of intestinal flora and its functional impact on radiation enteritis. Specifically, we summarized changes in the composition and diversity of microbiota in radiation-induced enteritis. We further reviewed the mechanisms by which gut microbiota dysbiosis promotes the development of radiation enteritis. In addition, we summarized recent findings in the treatment of radiation enteritis by correction of microbiome with probiotics and FMT. Our goal is to consolidate the current literature to better delineate the relationship between microbiota and radiation enteritis.
Introduction
Emil Grubbe’s treatment of breast cancer with radiotherapy back in 1896 (Hodges, 1964), pioneered modern cancer radiation therapy. As the number of patients receiving radiation increases steadily, the incidence of radiotherapy-associated complications has risen in parallel (Theis et al., 2010; Li et al., 2020b). While radiotherapy improves the survival of cancer patients (Hale, 2020), the oxidative stress caused by radiotherapy can produce reactive oxide species (ROS), which result in broad DNA damage to normal tissues, e.g., the gastrointestinal mucosa (Kumagai et al., 2018). It was reported that 6% to 78% of the long-term post-radiation survivors suffer from complications, leading to a compromise in patient’s quality of life (Andreyev, 2005). The most common side effect of this therapy is intestinal mucositis caused by injuries of normal intestinal epithelial cells. Intestinal mucosal atrophy and ulcer caused by radiotherapy hinder the renewal of basal epithelial cells (radiation-induced enteritis) (Sonis et al., 2004; Hauer-Jensen et al., 2014). The incidence of radiation-induced enteritis is largely dependent on radiation treatment scheme (Shaw and Taylor, 2012; Siegel et al., 2012). Patients with acute radiotherapy enteritis manifest symptoms, such as diarrhea, abdominal pain, constipation, hematochezia and loss of weight. Severe radiation-induced enteritis can lead to life-threatening systemic infection over a course of 3 months (Lalla et al., 2014; Marin et al., 2014; Lian et al., 2017). Therefore, prevention of adverse side effects associated with radiotherapy has become an urgent priority (Cui et al., 2019). It was reported that 90% of patients receiving abdominal radiotherapy develop gastrointestinal symptoms in a few weeks after the treatment (Andreyev, 2005). The intestinal microecology is disordered, and the infection of Clostridium difficile increases accordingly (Hautmann et al., 2011). In view of the key role of intestinal microecology in diseases, understanding the relationship between radiation enteritis and intestinal microecology and modulating the intestinal microecology to alleviate radiation enteritis may demonstrate a therapeutic benefit.
Microbiome Functions in Human Body
Intestinal mucosal barriers include those induced by mechanical, chemical, immunological and biological factors (Baumgart and Dignass, 2002). The biological factors refer to microbiome parasitizing in gastrointestinal tract of healthy people. The size of population for microbiome in an average adult intestine is around 100 trillion (Xu and Gordon, 2003). In healthy individuals, the intestinal flora maintains a stable and mutualism relationship with human host, and involves in almost all physiological aspects in nutrition, immune, and digestion. Gut microbiota is beneficial to host homeostasis and immune system development, regulating innate and adaptive immune response (Sommer and Backhed, 2013). The physical condition of host depends on the interaction between the microbiome and the immune system (Barroso-Batista et al., 2015). In addition, gut microbiota also regulates host metabolism. Dysbiosis is related to the occurrence of multiple metabolic diseases, such as type 2 diabetes (Amar et al., 2011) and obesity (Sonnenburg and Backhed, 2016), indicating that a stable gut microbiome is essential to human health.
Gut microbiome dysbiosis is also closely related to the occurrence and metastasis of dietary-associated cancers (Schulz et al., 2014; Feng et al., 2015). Intestinal flora may be used as biomarkers for the diagnosis of cancer. For example, the development of gastric cancer is associated with dysbacteriosis. The microbial dysbiosis index improves the sensitivity and specificity in detecting gastric cancer, indicating that changes in the overall flora rather than changes in individual flora lead to gastric cancer (Ferreira et al., 2018). In addition, Fusobacterium and related bacteria may also promote the growth and metastasis of colorectal cancer. Microbiota co-exists in primary and matched metastatic tumors, and is continuously associated with distant metastasis of primary human colorectal cancer (Bullman et al., 2017). Microbiome is also strongly linked to cancer treatment. Reports showed that the composition of gut microbiota can impact anti-cancer treatment by improving antitumor immunity. Targeting gut microbiota strengthens the efficacy of drugs and reduces adverse reactions (Gorjifard and Goldszmid, 2015; Roy and Trinchieri, 2017) (Figure 1).
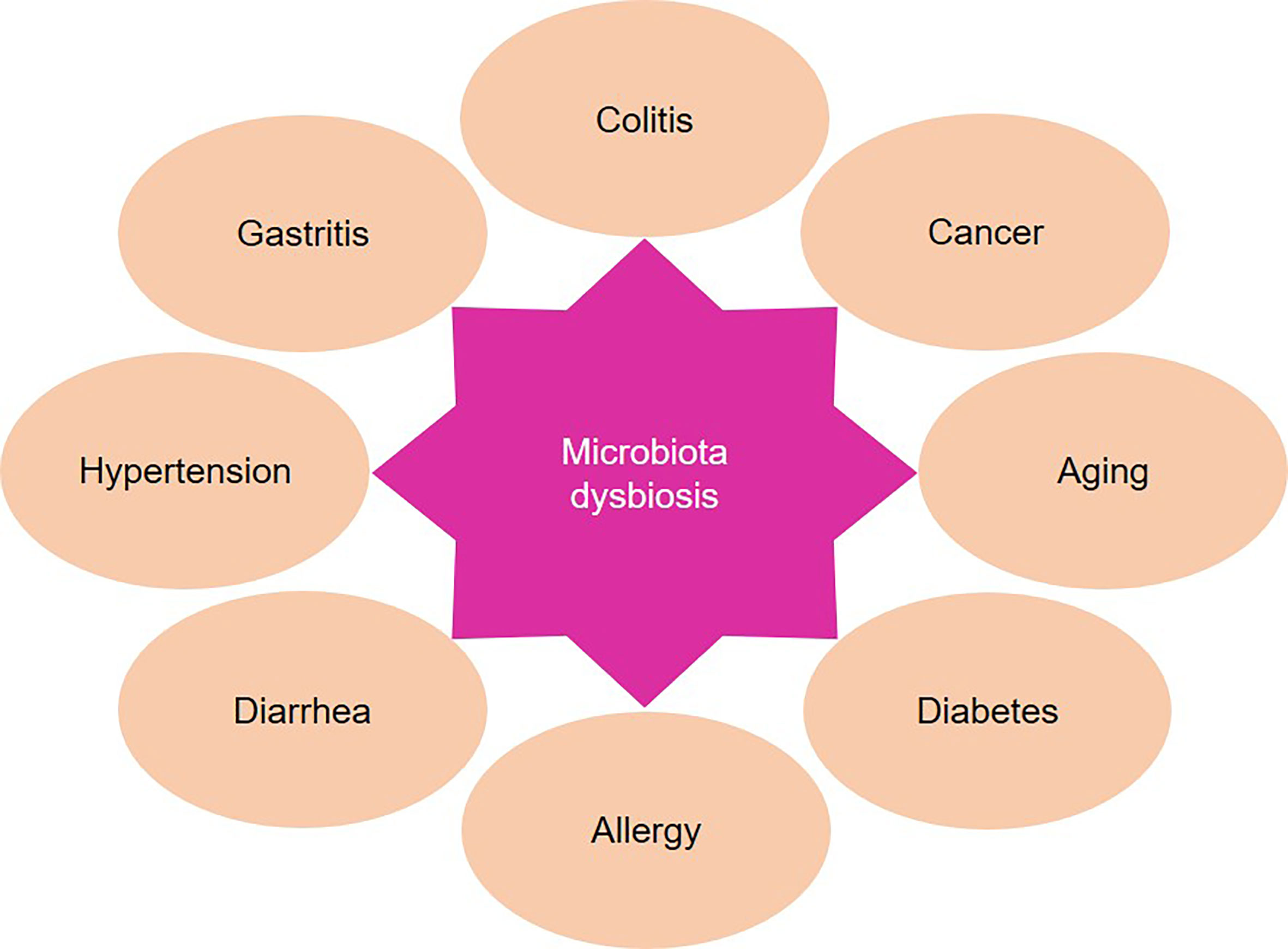
Figure 1 Microbiota dysbiosis is involved in the development of multiple diseases. The intestinal flora affects immune, metabolism and other functions of the host. Microbiota dysbiosis leads to a series of diseases, such as diabetes and colitis.
Radiotherapy remains to be essential for most of cancers. The imbalance in the diversity of microbiome is associated with the occurrence and treatment failure of a variety of diseases, including radiation enteritis (Sokol and Adolph, 2018). It is necessary to clarify the interaction between intestinal flora and radiation-induced enteritis to provide optimal treatment for patients.
Pathogenesis of Radiation-Induced Enteritis: Emerging Roles of Gut Microbiota
The symptoms of radiation-associated enteritis, such as diarrhea, are correlated with the onset time and duration of radiotherapy (Hogan and Kellison, 2002; Keefe, 2007). It has been reported that radiotherapy-induced enteritis is a common reason for reduced dosage of irradiation in radiotherapy. Such reduction can severely compromise body functions and increase mortality among cancer patients (van Vliet et al., 2010; Tarricone et al., 2016). High doses of radiotherapy lead to intestinal villi atrophy, intestinal epithelial damage, increased apoptosis, and spike in inflammation. Moreover, radiotherapy can also result in the dysfunction of intestinal epithelial barrier, leading to increased intestinal permeability, diarrhea, and disruption of water and electrolyte processing. This may ultimately result in hypovolemic shock, hence endangering life (Barnett et al., 2006; Shen et al., 2018). Radiation enteritis includes five phases, (i) the initial phase with the formation of ROS that result in DNA damage, (ii) the primary damage response phase with inflammation and apoptosis, (iii) the signal amplification phase, in which more inflammation and apoptosis occurs, (iv) the phase of ulcer formation, with discontinuity of the epithelial barrier that promotes bacterial translocation, and (v) the healing phase, with cell proliferation once radiotherapy has ceased (Sonis, 2004). Although recent studies have begun to reveal the pathogenesis of radiation enteritis, our current knowledge is far from being able to provide an effective prevention or treatment for the disease.
There is evidence that the pathophysiology of intestinal injury caused by radiation is related to the dysbiosis of gut microbiota. To demonstrate the influence of gut microbiota on intestinal inflammation, García-Lafuente et al. evaluated the effect of colonic microbiota on 2,4,6-trinitrobenzene sulfonic acid (TNBS)-induced intestinal inflammation in rats. Animals whose colon segments were excluded from fecal transport were recolonized with pre-selected bacteria to test the effects of different species on intestinal inflammation and damage. Rats with excluded colon and sterile culture of intracavitary washings showed slight inflammation and low mucosal damage in response to TNBS. Rats colonized with anaerobic bacteria showed significantly higher release of eicosanoids than rats colonized with aerobic bacteria only. In addition, mucosal lesions were mainly observed in rats with anaerobic bacteria, which suggest that colonic anaerobic bacteria may play a role in intestinal inflammation (García-Lafuente et al., 1997). Therefore, it is necessary to clarify the relationship between radiation intestinal injury and intestinal flora. We will give an overview of the characteristics of alteration of intestinal microbiota in radiation enteritis and its possible mechanisms in radiotherapy-induced intestinal injury.
Although limitations exist in studies with animals, the majority of the intestinal flora in mice is unique, with high similarities to those in humans (e.g., phylum level of both mice and humans are mainly dominated by Firmicutes and Bacteroidetes (Ley et al., 2005). Animal models, if used within ethical bounds, are crucial in identifying the relationship between radiotherapy enteritis and gut microbiota (Jones et al., 2020). We will not only summarize the changes of microbiome in clinical patients with radiation enteritis, but will also discuss the relationship between radiation enteritis and intestinal flora in animal models.
Radiation Leads to Alterations in the Composition and Diversity of Gut Microbiota
The development and application of 16S rRNA sequencing greatly promote the study of gut microbiota. 16S rRNA sequencing is a cost-effective method to study the microbiota diversity and composition in a large number of samples. However, this method is limited to the detection in flora composition. In order to obtain a comprehensive understanding of the microbiota, combined applications of 16S rRNA sequencing with metabolomics, transcriptomics, shotgun metagenomics, and other methods are recommended (Goudarzi et al., 2016).
Cui et al. determined the relationship between microbiome and the radiation sensitivity in the radiation enteritis through the 16S rRNA sequencing, and found that 6.5 Gy gamma ray with whole body radiation changed community composition of intestinal microbiota in C57BL/6 mice. The authors further confirmed that alterations in intestinal flora affect post-irradiation survival of mice mainly due to microbiome changes, which deregulate lncRNA expression of the host, hence sensitizing the mice to radiation (Cui et al., 2017). In addition, Johnson et al. found that approximately two hours after radiation, aerobic bacteria and Lactobacillus counts in the intestine become markedly reduced (Johnson et al., 2004). Zhao et al. obtained a similar result, proving that abdominal radiation disrupted the intestinal flora balance and significantly reduced gut microbiota diversity in mice (Zhao et al., 2020). Together, current studies demonstrate that in animal models, abdominal radiation induces gut microbiota dysbiosis and reduces the survival of radioactive mice.
Clinically, the incidence of grade 2 or even worse diarrhea was as high as 17% in patients undergoing radiotherapy before surgery (Bosset et al., 2004). Excessive growth of gram-negative bacilli is detected in radiation-enteritis-induced diarrhea. Comparison of microbiota immediately before, immediately after, and two weeks after radiotherapy indicated that patients with diarrhea showed an increase in Actinobacteria phylum and a reduction in Clostridium. Impairment of intestinal motility after radiation is the main pathogenic factor for colonization of gram-negative bacilli in gastrointestinal tract. During bacterial overgrowth, absence of intestinal migrating motor complex (MMC) correlates with the existence of mass colonization of gram-negative bacilli (Bosset et al., 2004). Thus, abnormal intestinal motility and mass colonization of gram-negative bacilli are important factors for the development of severe late-stage radiation-induced enteropathy. In addition, the susceptibility and protection to diarrhea and other bacterial changes after radiotherapy may also be related to different initial microbiota colonization (Husebye et al., 1995; Manichanh et al., 2008).
Manichanh et al. analyzed the intestinal bacteria of patients receiving radiotherapy (Manichanh et al., 2008; Stringer et al., 2013). In radiation enteritis patients without diarrhea, Actinobacteria phylum was not detected, whereas in patients with diarrhea, Bacilli class showed a higher abundance. Radiation therapy reestablishes the intestinal flora, and acute diarrhea induced by radiation enteritis is associated with the reconstitution of colonized intestinal flora (Manichanh et al., 2008; Stringer et al., 2013). Differences in the level of gut microbiota diversity reveal an obvious variance in microbiota composition and structure between cancer patients and healthy individuals. Stool samples were collected from 18 patients with cervical cancer during radiotherapy. Wang et al. used the Illumina HiSeq platform to characterize the microbiota based on 16S rRNA sequencing. Microbiota dysbiosis was observed in radiation enteritis patients with a significant decrease in α diversity and an increase in β diversity. The abundance of Proteobacteria, Gamma proteobacteria and Coprococcus was increased, while the level of Bacteroides was reduced (Wang et al., 2019).
Manichanh et al. demonstrated that there are different intestinal floras in abdominal cancer patients and in healthy individuals, because shifts in intestinal health status (such as chronic inflammation or abnormal function of epithelial cells) may directly affect microbiome (Manichanh et al., 2008). There is no direct causal relationship between gynecological cancer and intestinal flora, but Nam et al. found that there were significant differences in gut microbiota between gynecological cancer patients and healthy individuals. As compared with healthy individuals, gynecologic cancer patients receiving radiation therapy mainly possess a relative abundance of the phylum. Actinobacteria were 30 times higher than those in healthy individuals whereas Bacteroidetes and Fusobacteria were lower than those in healthy people. At the family level, the relative abundance of Eubacteriaceae was obviously higher, and Prevotellaceae, Oscillospiraceae and Fusobacteriaceae were lower than those in healthy group (Nam et al., 2013). Therefore, even if the location of disease development is far from or not related to the intestinal tract, the alteration in health status of the host will also affect the overall homeostasis of intestinal flora. Gut microbiota in different types of cancer patients may also be different as compared with healthy individuals.
Together, current studies suggest that gut microbiota in radiation-associated enteritis patients show marked changes in terms of composition and diversity. In radiation enteritis, the abundance of most bacteria belonging to the phylum Actinobacteria and Proteobacteria increases, and most of these bacteria are conditional pathogens, such as Escherichia coli. In contrast, microorganisms from Firmicutes and Bacteroides are reduced. Most of these bacteria are probiotics, such as Lactobacillus. When the abundance of probiotics decreases, conditional pathogens will reproduce and occupy the niche, which, in return, further inhibit the growth of probiotics and promote the release of endotoxin, and hence enhance intestinal inflammation. A vicious cycle may thus exacerbate radiation enteritis (Table 1).
Mechanisms by Which Dysbiosis of Gut Microbiota Contributes to Radiation-Induced Enteritis
Radiation-induced enteritis refers to the intestinal mucosa damaged by the free radicals derived from ionization. Radiation enteritis is manifested by phenotypes such as impaired intestinal mucosal barrier, increased inflammatory factors, enhanced pathogen invasion and endotoxin release, and decreased immune barrier.
The Gut Microbiota Dysbiosis Weakens the Function of Intestinal Epithelial Barrier
The intestinal epithelial barrier is composed of mucus layer, epithelial glycoglobulin and epithelial cells, and plays a critical role in the prevention of pathogen invasion (Sánchez de Medina et al., 2014). As an important component of the intestinal epithelial barrier, intercellular junctions, which include tight junction, gap junction, adhesion junction and desmosome, form multiple functional complexes (Anderson and Van Itallie, 2009; Groschwitz and Hogan, 2009). Tight junction, as the most important intercellular junction, determines the intestinal epithelial permeability and maintains physiological functions of the intestinal barrier (Zihni et al., 2016). Zonula occludens-1 (ZO-1), occludin, and claudins are three most important tight junction proteins, which play a vital role in maintaining cell polarity and intestinal epithelial barrier (Bazzoni et al., 2000). It was found that tight junction proteins are essential in repairing intestinal epithelial damages. Accumulating evidence shows that intestinal microbiome involves in the intestinal epithelial cell signaling and affects the intestinal barrier function (Ulluwishewa et al., 2011). Gut microbiota regulates intestinal barrier function by maintaining tight junction protein expression and distributions, which are important in intestinal barrier integrity (Ulluwishewa et al., 2011).
The intestinal epithelial mucus is a protective layer, which is regulated by bacteria such as Lactobacilli, Bifidobacteria, and Streptococci that play a positive role in strengthening the intestinal mucosal barrier. In the rat model, microbiota markedly promotes the expression of MUC2 gene and secretion of colonic MUC2 (Caballero-Franco et al., 2007). Akkermansia muciniphila is a type of gram-negative intestinal symbiotic bacteria that also promotes intestinal barrier function by enhancing mucous generation. Short-chain fatty acids (SCFAs) produced by Akkermansia muciniphila enter the intestinal epithelial cells through G protein-coupled receptor (GPCR) 41/43 to increase the expression of tight junction protein claudin-3 and occludin (Le Poul et al., 2003; Maslowski et al., 2009; Grander et al., 2018). Administration of Lactobacillus to healthy subjects significantly increases the scaffold protein ZO-1 and occludin in the vicinity of the tight junction structure, forming a cell side seal between the epithelial cells (Karczewski et al., 2010).
In radiation-associated enteritis patients, probiotics can also maintain micro-ecological stability and protect the intestinal barrier function by interrupting the pathogen’s infection or inhibiting the growth of pathogens. After intestinal pathogenic Escherichia coli infection, changes in host cell cytoskeleton reduce the absorbing surface of intestinal epithelial cells, leading to pathogenic Escherichia coli infection-associated persistent diarrhea. However, Lactobacillus plantarum prevents pathogenic Escherichia coli-induced rearrangement and redistribution of claudin-1, occludin, ZO-1 and JAM-1 (Qin et al., 2009). Lactobacillus competes with pathogens to bind to receptors on the surface of intestinal epithelial cells, inhibits pathogen growth, and reduces the vitality and toxicity of pathogenic Escherichia coli (Sherman et al., 2005). In addition, certain harmful bacteria in Enterobacteriaceae can form a biofilm on the surface of the epithelium, altering and destroying the mucus layer (Hansson and Johansson, 2010). The increase of Citrobacter rodentium digests mucins with glycosidase, and participates in the degradation of mucus barrier (Bergstrom et al., 2010). Thus, the colonization of probiotics decreases, and the pathogenic bacteria multiply without competitors. The imbalance of intestinal flora homeostasis will weaken the intestinal epithelial barrier integrity, resulting in increased intestinal permeability.
Microbiome Dysbiosis Contributes to the Expression of Inflammatory Cytokines
Grander et al. found that recombination with Akkermansia muciniphila reduces expression of IL-1β and TNF-α and decrease the infiltration of MPO+ neutrophils in mice (Grander et al., 2018). Gut probiotics inhibit inflammation via suppressing the activation of NF-κB and TNF-α. This signaling pathway plays an important role in intestinal microbiota balance, which helps to regulate intestinal homeostasis, maintains intestinal barrier function and promotes repair and regeneration of tissues damaged (Stringer, 2013). Ruminococcus, Coprococcus, Dorea, Lachnospira, Roseburia, Bifidobacterium and Clostridium can alleviate inflammation by inhibiting the TLR-NF-κB pathway (Lakhdari et al., 2011). Bifidobacterium inhibits intestinal epithelial cell inflammation by attenuating the inflammatory response induced by TNF-α and lipopolysaccharide (LPS) (Riedel et al., 2006). In addition, increased Citrobacter can activate NF-κB (Wang et al., 2006), and also involve in the degradation of the mucus barrier, thus increasing the inflammatory response. NF-κB and TNF-α are able to increase the production of myosin light-chain kinase, resulting in the disassembly of tight junction proteins. Biffidobactrium diminishes the formation of intestinal endotoxins and increases the production of tight junction proteins, thus decreasing intestinal permeability and bacterial translocation (Ewaschuk et al., 2008). Microbiome dysbiosis induced by radiation enteritis leads to severe side effects in immunodeficiency cancer patients because intestinal flora plays a vital role in innate and adaptive immune responses of hosts (Maynard et al., 2012). Interestingly, less apoptosis was observed in endothelial cells and lymphocytes in small intestine of germ free (GF) mice received a lethal dose of irradiation, demonstrating that GF mice may be resistance to lethal radioactive enteritis (Crawford and Gordon, 2005). Together, these studies suggest that microbiota dysbiosis may be detrimental to the maintenance of effective intestinal barrier function after radiation.
The mechanisms by which changes in the gut microbiota affect intestinal injury in radiation are expected to be extremely complicated. The dynamic changes of the microbial community interact with various components within the intestine rather than a single compound in the system. Current evidence supports and illustrates that intestinal microbiome plays a critical role in radiation-associated enteritis via up-regulating key regulatory factors. Gut microbiota and the body form a symbiotic and stable relationship in the protection of the intestinal epithelium, reduction of inflammatory responses, and maintenance of normal immune tolerance in order to sustain host’s immune homeostasis, growth, and development (Sender et al., 2016). If there is an imbalance in intestinal flora, the relative abundance and diversity of the probiotics will be severely compromised, leading to limited intestinal barrier function, harmful bacteria overgrowth, accumulation of endotoxin in the blood, increased inflammatory factors, and eventually aggravating radiotherapy-induced enteritis. Thus, a stabilized intestinal microbiota is crucial for radiation enteritis (Figure 2).
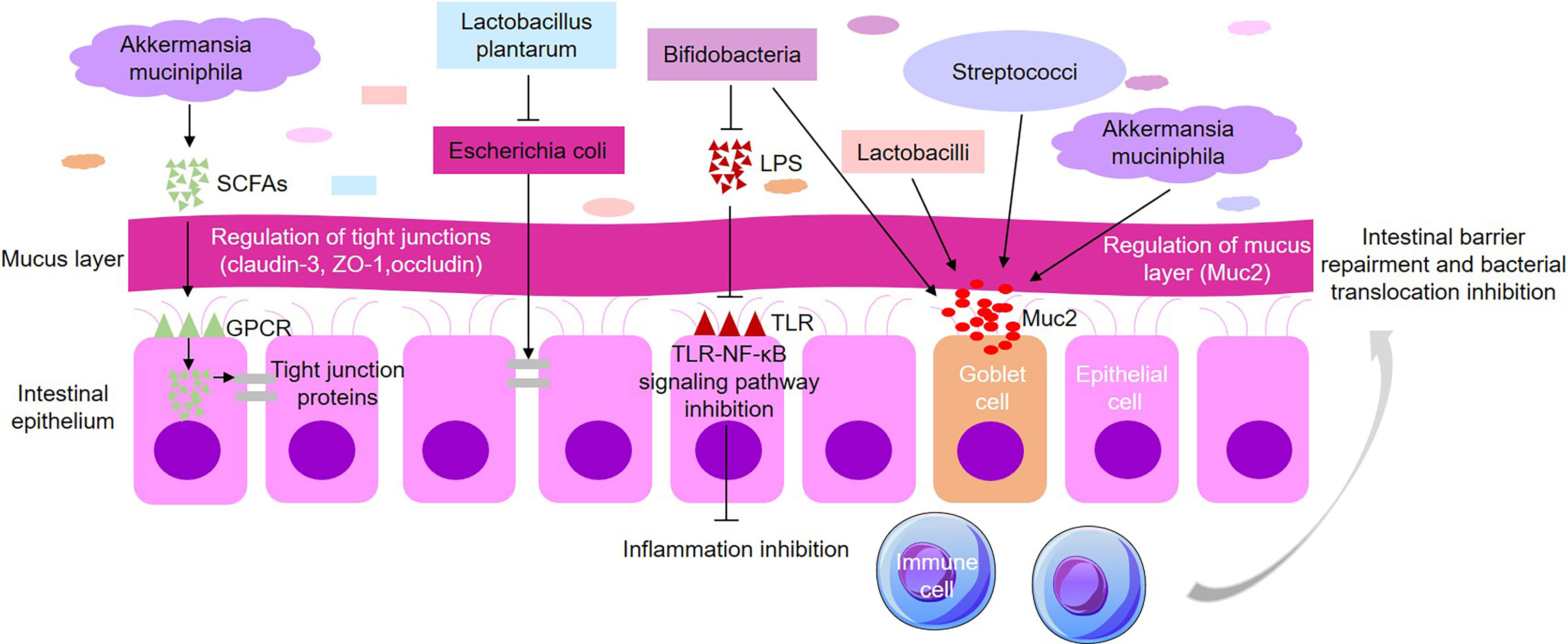
Figure 2 Gut microbiota affects the function of intestinal epithelial barrier. Different types of microbiota act on various targets associated with intestinal epithelial barrier. Biffidobactrium, Lactobacilli, Streptococci and Akkermansia muciniphila stimulate the secretion of MUC2. SCFAs produced by Akkermansia muciniphila enter into the intestinal epithelial cells through GPCR to increase the expression of tight junction protein claudin-3 and occludin. Lactobacillus plantarum inhibits pathogenic Escherichia coli growth and increases the expression of ZO-1 and occludin. Biffidobactrium diminishes the formation of LPS and inhibits the TLR-NF-κB signaling to alleviate inflammation, hence decreasing intestinal permeability and bacterial translocation.
The Role of Intestinal Flora in the Treatment of Radiotherapy-Induced Enteritis
Probiotics Regulate the Intestinal Microbiota to Improve Radiation Enteritis
Recent studies have shown that probiotics are beneficial in strengthening the number and activity of host T cells, directly affecting the immune response mediated by T cells and improving immune functions (Redman et al., 2014). Furthermore, probiotics promote the diversity of gut microbiota, and increase the species of intestinal flora, ensuring the integrity of intestinal mucosal barrier so as to avoid bacterial translocation (Reiff and Kelly, 2010). The probiotic VSL# 3 is a mixture of 8 probiotics, which has been used broadly for many years with high safety. Clinically, it was proved that VSL#3 alleviates intestinal inflammation and enhances intestinal barrier functions by improving intestinal flora balance (Mimura et al., 2004; Sartor, 2006; Tankou et al., 2018). Treatment with VSL# 3 probiotics increases the diversity of bacterial community in patients, reduces fungal variety, and elevates the abundance of Lactobacillus and Bifidobacterium, and hence reduces the incidence and severity of radiotherapy enteritis-associated diarrhea (Kuhbacher et al., 2006; Chitapanarux et al., 2010).
A randomized double-blind controlled trial showed that a standard dose of Lactobacillus acidophilus LAC-361 and Bifidobacterium longum BB-536 decreased level 2, 3, and 4 diarrhea caused by radiation enteritis in patients with surgery. Demers et al. analyzed a total of 229 patients and found that for patients who had undergone surgery, the incidence of diarrhea was reduced after probiotic treatment. For patients who did not undergo surgery, there was no statistical difference (Demers et al., 2014). In fact, for patients without surgery, patients treated with standard doses of probiotics had fewer moderate to severe diarrhea than the placebo group at the end of radiotherapy. For patients with surgery before radiotherapy, administration of probiotics tends to reduce all degrees of diarrhea, especially the most severe grade 4 diarrhea. These results may be attributable to the individualized nutritional interventions during the treatment, and these interventions were adjusted as the treatment progressed. It is difficult to compare these results with other published studies due to different treatment options. In addition, compared to probiotic treatment group, the placebo group showed a significant destruction of gut microbiota after radiotherapy enteritis (e.g., through an increase in Enterobacteriaceae (Osterlund et al., 2007). The intragastric administration of Lactobacillus rhamnosus increased the crypts survival in radiation-induced enteritis by approximately two-fold and reduced epithelial cell apoptosis at the crypt basement, which depends on intact Toll-like receptor 2 (TLR2) and MyD88-dependent signals that involve in the relocation and expression of cyclooxygenase 2 (COX-2) in mesenchymal stem cells of crypt region (Ciorba et al., 2012; Uribe et al., 2018; Riehl et al., 2019). Bifidobacterium infantis produces indole-3-lactic acid (ILA) to protect intestinal epithelial cells in culture via activation of the aryl hydrogen receptor (AhR) and nuclear factor erythroid 2-related factor 2 (Nrf2) (Ehrlich et al., 2020). Thus, prebiotics and synbiotics can also promote the growth and reproduction of probiotics and hence reduce diarrhea in radiotherapy patients (Garcia-Peris et al., 2016).
Probiotics-mediated homeostasis of intestinal microbiota may also prevent other symptoms from radiation enteritis, but more studies are needed to clarify the observation, which is the key to maintaining the biological strategies of healthy intestine during radiotherapy of cancer (Bentzen, 2006; Fuccio and Guido, 2013; Florez et al., 2016).
Fecal Microbiota Transplantation Maintains the Balance of Gut Microbiota to Improve Radiotherapy-Induced Enteritis
FMT is a treatment method to reestablish the gut microbiota of patients by separating and transplanting the intestinal flora of healthy persons into patient’s intestine tract (Xu et al., 2016). In 1958, Eiseman et al. applied FMT to treat Pseudomembranous enteritis caused by the treatment of antibiotics, leading to an improvement of patient’s condition (Eiseman et al., 1958). With the analysis of the 16S rRNA sequencing, Cui et al. confirmed that FMT treatment increases the relative abundance of the intestinal microbiota, such as escalation (or stabilization) of Bacteroidetes (or Lactobacillus), augment of Prevotella at the genus level. In addition, FMT-improved gut microbiota can reduce the intestinal leakage and enhance the intestinal functions and epithelial integrity in radiotherapy-induced enteritis (Cui et al., 2017). Ding et al. proved that the intestinal flora diversity of all patients underwent radiotherapy increased after FMT treatment. Radiation-induced intestinal edema was strikingly alleviated after eight weeks of FMT, and the beneficial bacteria such as Alistipes, Phascolarctobacterium, Streptococcus and Bacteroides expanded, whereas the abundance of Faecalibacterium decreased (Ding et al., 2020). FMT of gut microbes from healthy donors may reduce the toxicity caused by radiotherapy (Cui et al., 2017). In addition, FMT could elevate the level of microbial-derived indole 3-propionic acid (IPA) in the feces of irradiated mice, so that the mice had a lower level of systemic inflammation, a reduced hematopoietic organ injury and catabatic myelosuppression, and improved gastrointestinal tract functions and epithelial integrity (Xiao et al., 2020). Li et al. proved that FMT increased the level of SCFAs in feces and valeric acid (VA) produced by microbiota exerted the most important radioprotective effect by increasing the expression of keratin 1 (KRT1). VA supplementation increased the survival of irradiated mice, protected their hematopoietic organs, and alleviated gastrointestinal injury of irradiated mice (Li et al., 2020a).
Fresh stool used for bacterial transplantation can effectively treat Clostridium difficile infection. For patients with severe Clostridium difficile infection, multiple stool transplantation may effectively relieve diarrhea (Hui et al., 2019). FMT capsules were also used to treat pediatric diarrhea and other diseases. It significantly improves both overall and gastrointestinal health after FMT (Youngster et al., 2014). FMT also has an obvious effect on the treatment of pediatric inflammatory bowel disease (IBD). Patients prior to FMT treatment exhibited reduced biodiversity and significantly differed in gut microbiota composition characterized by an increase in Enterobacteriaceae, Enterococcus, Haemophilus, and Fusobacterium compared to donors, and an augment in species diversity at 30 days post-FMT treatment (Goyal et al., 2018). Paramsothy et al. demonstrated that microbiota diversity increased with the persistence of FMT, which could alleviate the clinical symptoms of ulcerative colitis, and was mainly associated with the improvement of microbial diversity (Paramsothy et al., 2017). Ishikawa et al. also confirmed that FMT is a potential treatment for restoring normal intestinal flora in patients with ulcerative colitis (Ishikawa et al., 2017). Selection of healthy donors for FMT may avoid potential adverse effects transmitted from donor’s feces in the therapy of radiation-induced enteritis. Standardization of this technology and humanization of FMT are two key factors in the usage of the therapy to meet the needs of patients (Ren et al., 2016).
Antibiotics Reconstruct the Intestinal Microbiota to Alleviate Radiation-Induced Enteritis
Abdominal radiation disrupts the intestinal microbial balance. It reduces microbiota diversity, and increases the relative abundance of pathogenic bacteria, such as Proteobacteria in mice. Antibiotic cocktail (ABX) and metronidazole pretreatment are beneficial to the reconstruction of gut microbes in irradiated mice. It has been reported that Abx pretreatment effectively reduces the level of LPS in the ileum and inhibits the TLR4/MyD88/NF-κB signaling, thereby reducing intestinal inflammation. In addition, Abx pretreatment regulates macrophage polarization in the ileum and downregulates the expression of TGF-β1 and phosphorylated Smad-3 and α-SMA thereby preventing intestinal fibrosis and ultimately improving the survival of mice with radiation-induced intestinal injury (Cui et al., 2017; Zhao et al., 2020). These results indicate that antibiotic pretreatment can effectively alleviate gut microbial disorders and intestinal injury caused by abdominal radiation. In general, these findings have increased our understanding of the radiation enteritis pathogenesis.
Conclusions and Prospects
The intestinal flora is affected by multiple factors, as well as original diseases, such as cancers. Although the location of a disease is far from or not related to the intestinal tract, alterations in health status of the host may also affect the overall homeostasis of intestinal flora. Thus, gut microbiota in various cancer patients may be different as compared with healthy individuals. Studies with strictly selected healthy people for comparison may help to uncover bacterial genera changed in radiation enteritis. In terms of treatment, with intestinal flora in healthy people as references, those in patients with radiation-induced intestinal injury can be manipulated to a comparable level in healthy individuals.
In the current review, we emphasized the relationship between microbiota and radiation enteritis. Radiation reduces the diversity of intestinal flora and modifies gut microbiota composition. We summarized the types of bacteria, which have been changed in radiation enteritis. Alterations in the composition of the intestinal flora aggravate radiation enteritis, which may form a vicious circle and amplify the changes in microbiota. Radiation enteritis could weaken the intestinal epithelial barrier and increase the expression of inflammatory factors. Mounting evidence shows that intestinal microbiota is the initiator for the pathogenesis of radiation enteritis. Targeted treatment of gut microbiota with probiotics, fecal bacteria transplantation and antibiotics can alleviate radiation enteritis. In addition, intestinal flora could be a potential biomarker for radiation enteritis and may help to establish a personalized radiation treatment plan. Further studies regarding the role of intestinal flora in radiation enteritis are needed in order to set up a more reliable preventive strategy for the disease. In the future, on the basis of 16S rRNA sequencing, metabolomics, transcriptomics, shotgun metagenomics, and other state-of-art methods, functions of gut microbiota in radiation enteritis are expected to be explored more extensively. Reversing the changes in microbiome may eventually prove to be an effective therapeutic strategy for patients with life-threatening radiation enteritis.
Author Contributions
YJ wrote the manuscript. Z-XX, YW, DZ, and ML contributed to critical revision of the paper. All authors contributed to the article and approved the submitted version.
Funding
This work was supported by the National Natural Science Foundation of China (Nos. 81573087, 81772924, and 82020108024) and International Cooperation Foundation Grant of Jilin Province (20190701006GH).
Conflict of Interest
The authors declare that the research was conducted in the absence of any commercial or financial relationships that could be construed as a potential conflict of interest.
Publisher’s Note
All claims expressed in this article are solely those of the authors and do not necessarily represent those of their affiliated organizations, or those of the publisher, the editors and the reviewers. Any product that may be evaluated in this article, or claim that may be made by its manufacturer, is not guaranteed or endorsed by the publisher.
References
Amar, J., Chabo, C., Waget, A., Klopp, P., Vachoux, C., Bermudez-Humaran, L. G., et al. (2011). Intestinal Mucosal Adherence and Translocation of Commensal Bacteria at the Early Onset of Type 2 Diabetes: Molecular Mechanisms and Probiotic Treatment. EMBO Mol. Med. 3, 559–572. doi: 10.1002/emmm.201100159
Anderson, J. M., Van Itallie, C. M. (2009). Physiology and Function of the Tight Junction. Cold Spring Harb. Perspect. Biol. 1, 002584. doi: 10.1101/cshperspect.a002584
Andreyev, J. (2005). Gastrointestinal Complications of Pelvic Radiotherapy: Are They of Any Importance? Gut 54, 1051–1054. doi: 10.1136/gut.2004.062596
Barnett, D. J., Parker, C. L., Blodgett, D. W., Wierzba, R. K., Links, J. M. (2006). Understanding Radiologic and Nuclear Terrorism as Public Health Threats: Preparedness and Response Perspectives. J. Nucl. Med. 47, 1653–1661. doi: 10.1038/nrc1950
Barroso-Batista, J., Demengeot, J., Gordo, I. (2015). Adaptive Immunity Increases the Pace and Predictability of Evolutionary Change in Commensal Gut Bacteria. Nat. Commun. 6, 8945. doi: 10.1038/ncomms9945
Baumgart, D. C., Dignass, A. U. (2002). Intestinal Barrier Function. Curr. Opin. Clin. Nutr. Metab. Care 5, 685–694. doi: 10.1097/00075197-200211000-00012
Bazzoni, G., Martinez-Estrada, O. M., Orsenigo, F., Cordenonsi, M., Citi, S., Dejana, E. (2000). Interaction of Junctional Adhesion Molecule With the Tight Junction Components ZO-1, Cingulin, and Occludin. J. Biol. Chem. 275, 20520–20526. doi: 10.1074/jbc.M905251199
Bentzen, S. M. (2006). Preventing or Reducing Late Side Effects of Radiation Therapy: Radiobiology Meets Molecular Pathology. Nat. Rev. Cancer 6, 702–713. doi: 10.1038/nrc1950
Bergstrom, K. S., Kissoon-Singh, V., Gibson, D. L., Ma, C., Montero, M., Sham, H. P., et al. (2010). Muc2 Protects Against Lethal Infectious Colitis by Disassociating Pathogenic and Commensal Bacteria From the Colonic Mucosa. PLoS Pathog. 6, 1000902. doi: 10.1371/journal.ppat.1000902
Bosset, J. F., Calais, G., Daban, A., Berger, C., Radosevic-Jelic, L., Maingon, P., et al. (2004). Preoperative Chemoradiotherapy Versus Preoperative Radiotherapy in Rectal Cancer Patients: Assessment of Acute Toxicity and Treatment Compliance. Report of the 22921 Randomised Trial Conducted by the EORTC Radiotherapy Group. Eur. J. Cancer 40, 219–224. doi: 10.1016/j.ejca.2003.09.032
Bullman, S., Pedamallu, C. S., Sicinska, E., Clancy, T. E., Zhang, X., Cai, D., et al. (2017). Analysis of Fusobacterium Persistence and Antibiotic Response in Colorectal Cancer. Science 358, 1443–1448. doi: 10.1126/science.aal5240
Caballero-Franco, C., Keller, K., De Simone, C., Chadee, K. (2007). The VSL3 Probiotic Formula Induces Mucin Gene Expression and Secretion in Colonic Epithelial Cells. Am. J. Physiol. Gastrointest Liver Physiol. 292, 315–322. doi: 10.1152/ajpgi.00265.2006
Chitapanarux, I., Chitapanarux, T., Traisathit, P., Kudumpee, S., Tharavichitkul, E., Lorvidhaya, V. (2010). Randomized Controlled Trial of Live Lactobacillus Acidophilus Plus Bifidobacterium Bifidum in Prophylaxis of Diarrhea During Radiotherapy in Cervical Cancer Patients. Radiat. Oncol. 5, 31. doi: 10.1186/1748-717X-5-31
Ciorba, M. A., Riehl, T. E., Rao, M. S., Moon, C., Ee, X., Nava, G. M., et al. (2012). Lactobacillus Probiotic Protects Intestinal Epithelium From Radiation Injury in a TLR-2/Cyclo-Oxygenase-2-Dependent Manner. Gut 61, 829–838. doi: 10.1136/gutjnl-2011-300367
Crawford, P. A., Gordon, J. I. (2005). Microbial Regulation of Intestinal Radiosensitivity. Proc. Natl. Acad. Sci. U. S. A. 102, 13254–13259. doi: 10.1073/pnas.0504830102
Cui, M., Xiao, H., Li, Y., Zhang, S., Dong, J., Wang, B., et al. (2019). Sexual Dimorphism of Gut Microbiota Dictates Therapeutics Efficacy of Radiation Injuries. Adv. Sci. (Weinh) 6, 1901048. doi: 10.1002/advs.201901048
Cui, M., Xiao, H., Li, Y., Zhou, L., Zhao, S., Luo, D., et al. (2017). Faecal Microbiota Transplantation Protects Against Radiation-Induced Toxicity. EMBO Mol. Med. 9, 448–461. doi: 10.15252/emmm.201606932
Demers, M., Dagnault, A., Desjardins, J. (2014). A Randomized Double-Blind Controlled Trial: Impact of Probiotics on Diarrhea in Patients Treated With Pelvic Radiation. Clin. Nutr. 33, 761–767. doi: 10.1016/j.clnu.2013.10.015
Ding, X., Li, Q., Li, P., Chen, X., Xiang, L., Bi, L., et al. (2020). Fecal Microbiota Transplantation: A Promising Treatment for Radiation Enteritis? Radiother. Oncol. 143, 12–18. doi: 10.1016/j.radonc.2020.01.011
Ehrlich, A. M., Pacheco, A. R., Henrick, B. M., Taft, D., Xu, G., Huda, M. N., et al. (2020). Indole-3-Lactic Acid Associated With Bifidobacterium-Dominated Microbiota Significantly Decreases Inflammation in Intestinal Epithelial Cells. BMC Microbiol. 20, 357. doi: 10.1186/s12866-020-02023-y
Eiseman, B., Silen, W., Bascom, G. S., Kauvar, A. J. (1958). Fecal Enema as an Adjunct in the Treatment of Pseudomembranous Enterocolitis. Surgery 44, 854–859.
Ewaschuk, J. B., Diaz, H., Meddings, L., Diederichs, B., Dmytrash, A., Backer, J., et al. (2008). Secreted Bioactive Factors From Bifidobacterium Infantis Enhance Epithelial Cell Barrier Function. Am. J. Physiol. Gastrointest Liver Physiol. 295, 1025–1034. doi: 10.1152/ajpgi.90227.2008
Feng, Q., Liang, S., Jia, H., Stadlmayr, A., Tang, L., Lan, Z., et al. (2015). Gut Microbiome Development Along the Colorectal Adenoma-Carcinoma Sequence. Nat. Commun. 6, 6528. doi: 10.1038/ncomms7528
Ferreira, R. M., Pereira-Marques, J., Pinto-Ribeiro, I., Costa, J. L., Carneiro, F., Machado, J. C., et al. (2018). Gastric Microbial Community Profiling Reveals a Dysbiotic Cancer-Associated Microbiota. Gut 67, 226–236. doi: 10.1136/gutjnl-2017-314205
Florez, A. B., Sierra, M., Ruas-Madiedo, P., Mayo, B. (2016). Susceptibility of Lactic Acid Bacteria, Bifidobacteria and Other Bacteria of Intestinal Origin to Chemotherapeutic Agents. Int. J. Antimicrob. Agents 48, 547–550. doi: 10.1016/j.ijantimicag.2016.07.011
Fuccio, L., Guido, A. (2013). Probiotics Supplementation for the Prevention of Gastrointestinal Radiation-Induced Side Effects: The Time Is Now. Am. J. Gastroenterol. 108, 277. doi: 10.1038/ajg.2012.418
García-Lafuente, A., Antolín, M., Guarner, F., Crespo, E., Salas, A., Forcada, P., et al. (1997). Incrimination of Anaerobic Bacteria in the Induction of Experimental Colitis. Am. J. Physiol. 272, 10–15. doi: 10.1152/ajpgi.1997.272.1.G10
Garcia-Peris, P., Velasco, C., Hernandez, M., Lozano, M. A., Paron, L., de la Cuerda, C., et al. (2016). Effect of Inulin and Fructo-Oligosaccharide on the Prevention of Acute Radiation Enteritis in Patients With Gynecological Cancer and Impact on Quality-of-Life: A Randomized, Double-Blind, Placebo-Controlled Trial. Eur. J. Clin. Nutr. 70, 170–174. doi: 10.1038/ejcn.2015.192
Gorjifard, S., Goldszmid, R. S. (2015). Beating Cancer With a Gut Feeling. Cell Host Microbe 18, 646–648. doi: 10.1016/j.chom.2015.11.014
Goudarzi, M., Mak, T. D., Jacobs, J. P., Moon, B. H., Strawn, S. J., Braun, J., et al. (2016). An Integrated Multi-Omic Approach to Assess Radiation Injury on the Host-Microbiome Axis. Radiat. Res. 186, 219–234. doi: 10.1667/RR14306.1
Goyal, A., Yeh, A., Bush, B. R., Firek, B. A., Siebold, L. M., Rogers, M. B., et al. (2018). Safety, Clinical Response, and Microbiome Findings Following Fecal Microbiota Transplant in Children With Inflammatory Bowel Disease. Inflamm. Bowel Dis. 24, 410–421. doi: 10.1093/ibd/izx035
Grander, C., Adolph, T. E., Wieser, V., Lowe, P., Wrzosek, L., Gyongyosi, B., et al. (2018). Recovery of Ethanol-Induced Akkermansia Muciniphila Depletion Ameliorates Alcoholic Liver Disease. Gut 67, 891–901. doi: 10.1136/gutjnl-2016-313432
Groschwitz, K. R., Hogan, S. P. (2009). Intestinal Barrier Function: Molecular Regulation and Disease Pathogenesis. J. Allergy Clin. Immunol. 124, 3–20; quiz 21-22. doi: 10.1016/j.jaci.2009.05.038
Hale, M. F. (2020). Radiation Enteritis: From Diagnosis to Management. Curr. Opin. Gastroenterol. 36, 208–214. doi: 10.1097/MOG.0000000000000632
Hansson, G. C., Johansson, M. E. (2010). The Inner of the Two Muc2 Mucin-Dependent Mucus Layers in Colon is Devoid of Bacteria. Gut Microbes 1, 51–54. doi: 10.4161/gmic.1.1.10470
Hauer-Jensen, M., Denham, J. W., Andreyev, H. J. (2014). Radiation Enteropathy–Pathogenesis, Treatment and Prevention. Nat. Rev. Gastroenterol. Hepatol. 11, 470–479. doi: 10.1038/nrgastro.2014.46
Hautmann, M. G., Hipp, M., Kölbl, O. (2011). Clostridium Difficile-Associated Diarrhea in Radiooncology: An Underestimated Problem for the Feasibility of the Radiooncological Treatment? Radiat. Oncol. 6, 89. doi: 10.1186/1748-717X-6-89
Hodges, P. C. (1964). Dr. Emil H. Grubbe, Pioneer Chicago Radiologist. Postgrad. Med. 35, 85–87. doi: 10.1080/00325481.1964.11695172
Hogan, D. E., Kellison, T. (2002). Nuclear Terrorism. Am. J. Med. Sci. 323, 341–349. doi: 10.1097/00000441-200206000-00006
Hui, W., Li, T., Liu, W., Zhou, C., Gao, F. (2019). Fecal Microbiota Transplantation for Treatment of Recurrent C. Difficile Infection: An Updated Randomized Controlled Trial Meta-Analysis. PLoS One 14, 0210016. doi: 10.1371/journal.pone.0210016
Husebye, E., Skar, V., Hoverstad, T., Iversen, T., Melby, K. (1995). Abnormal Intestinal Motor Patterns Explain Enteric Colonization With Gram-Negative Bacilli in Late Radiation Enteropathy. Gastroenterology 109, 1078–1089. doi: 10.1016/0016-5085(95)90565-0
Ishikawa, D., Sasaki, T., Osada, T., Kuwahara-Arai, K., Haga, K., Shibuya, T., et al. (2017). Changes in Intestinal Microbiota Following Combination Therapy With Fecal Microbial Transplantation and Antibiotics for Ulcerative Colitis. Inflammation Bowel Dis. 23, 116–125. doi: 10.1097/MIB.0000000000000975
Johnson, L. B., Riaz, A. A., Adawi, D., Wittgren, L., Bäck, S., Thornberg, C., et al. (2004). Radiation Enteropathy and Leucocyte-Endothelial Cell Reactions in a Refined Small Bowel Model. BMC Surg. 4, 10. doi: 10.1186/1471-2482-4-10
Jones, C. B., Davis, C. M., Sfanos, K. S. (2020). The Potential Effects of Radiation on the Gut-Brain Axis. Radiat. Res. 193, 209–222. doi: 10.1667/RR15493.1
Karczewski, J., Troost, F. J., Konings, I., Dekker, J., Kleerebezem, M., Brummer, R. J., et al. (2010). Regulation of Human Epithelial Tight Junction Proteins by Lactobacillus Plantarum In Vivo and Protective Effects on the Epithelial Barrier. Am. J. Physiol. Gastrointest Liver Physiol. 298, 851–859. doi: 10.1152/ajpgi.00327.2009
Keefe, D. M. (2007). Intestinal Mucositis: Mechanisms and Management. Curr. Opin. Oncol. 19, 323–327. doi: 10.1097/CCO.0b013e3281214412
Kuhbacher, T., Ott, S. J., Helwig, U., Mimura, T., Rizzello, F., Kleessen, B., et al. (2006). Bacterial and Fungal Microbiota in Relation to Probiotic Therapy (VSL#3) in Pouchitis. Gut 55, 833–841. doi: 10.1136/gut.2005.078303
Kumagai, T., Rahman, F., Smith, A. M. (2018). The Microbiome and Radiation Induced-Bowel Injury: Evidence for Potential Mechanistic Role in Disease Pathogenesis. Nutrients 10, 1405. doi: 10.3390/nu10101405
Lakhdari, O., Tap, J., Beguet-Crespel, F., Le Roux, K., De Wouters, T., Cultrone, A., et al. (2011). Identification of NF-KappaB Modulation Capabilities Within Human Intestinal Commensal Bacteria. J. BioMed. Biotechnol. 2011, 282356. doi: 10.1155/2011/282356
Lalla, R. V., Bowen, J., Barasch, A., Elting, L., Epstein, J., Keefe, D. M., et al. (2014). MASCC/ISOO Clinical Practice Guidelines for the Management of Mucositis Secondary to Cancer Therapy. Cancer 120, 1453–1461. doi: 10.1002/cncr.28592
Le Poul, E., Loison, C., Struyf, S., Springael, J. Y., Lannoy, V., Decobecq, M. E., et al. (2003). Functional Characterization of Human Receptors for Short Chain Fatty Acids and Their Role in Polymorphonuclear Cell Activation. J. Biol. Chem. 278, 25481–25489. doi: 10.1074/jbc.M301403200
Ley, R. E., Backhed, F., Turnbaugh, P., Lozupone, C. A., Knight, R. D., Gordon, J. I. (2005). Obesity Alters Gut Microbial Ecology. Proc. Natl. Acad. Sci. U. S. A. 102, 11070–11075. doi: 10.1073/pnas.0504978102
Lian, Q., Xu, J., Yan, S., Huang, M., Ding, H., Sun, X., et al. (2017). Chemotherapy-Induced Intestinal Inflammatory Responses are Mediated by Exosome Secretion of Double-Strand DNA via AIM2 Inflammasome Activation. Cell Res. 27, 784–800. doi: 10.1038/cr.2017.54
Li, Y., Dong, J., Xiao, H., Zhang, S., Wang, B., Cui, M., et al. (2020a). Gut Commensal Derived-Valeric Acid Protects Against Radiation Injuries. Gut Microbes 11, 789–806. doi: 10.1080/19490976.2019.1709387
Li, Y., Xiao, H., Dong, J., Luo, D., Wang, H., Zhang, S., et al. (2020b). Gut Microbiota Metabolite Fights Against Dietary Polysorbate 80-Aggravated Radiation Enteritis. Front. Microbiol. 11, 1450. doi: 10.3389/fmicb.2020.01450
Manichanh, C., Varela, E., Martinez, C., Antolin, M., Llopis, M., Dore, J., et al. (2008). The Gut Microbiota Predispose to the Pathophysiology of Acute Postradiotherapy Diarrhea. Am. J. Gastroenterol. 103, 1754–1761. doi: 10.1111/j.1572-0241.2008.01868.x
Marin, M., Gudiol, C., Ardanuy, C., Garcia-Vidal, C., Calvo, M., Arnan, M., et al. (2014). Bloodstream Infections in Neutropenic Patients With Cancer: Differences Between Patients With Haematological Malignancies and Solid Tumours. J. Infection 69, 417–423. doi: 10.1016/j.jinf.2014.05.018
Maslowski, K. M., Vieira, A. T., Ng, A., Kranich, J., Sierro, F., Yu, D., et al. (2009). Regulation of Inflammatory Responses by Gut Microbiota and Chemoattractant Receptor GPR43. Nature 461, 1282–1286. doi: 10.1038/nature08530
Maynard, C. L., Elson, C. O., Hatton, R. D., Weaver, C. T. (2012). Reciprocal Interactions of the Intestinal Microbiota and Immune System. Nature 489, 231–241. doi: 10.1038/nature11551
Mimura, T., Rizzello, F., Helwig, U., Poggioli, G., Schreiber, S., Talbot, I., et al. (2004). Once Daily High Dose Probiotic Therapy (VSL#3) for Maintaining Remission in Recurrent or Refractory Pouchitis. Gut 53, 108–114. doi: 10.1136/gut.53.1.108
Nam, Y. D., Kim, H. J., Seo, J. G., Kang, S. W., Bae, J. W. (2013). Impact of Pelvic Radiotherapy on Gut Microbiota of Gynecological Cancer Patients Revealed by Massive Pyrosequencing. PLoS One 8, 82659. doi: 10.1371/journal.pone.0082659
Osterlund, P., Ruotsalainen, T., Korpela, R., Saxelin, M., Ollus, A., Valta, P., et al. (2007). Lactobacillus Supplementation for Diarrhoea Related to Chemotherapy of Colorectal Cancer: A Randomised Study. Br. J. Cancer 97, 1028–1034. doi: 10.1038/sj.bjc.6603990
Paramsothy, S., Kamm, M. A., Kaakoush, N. O., Walsh, A. J., Van Den Bogaerde, J., Samuel, D., et al. (2017). Multidonor Intensive Faecal Microbiota Transplantation for Active Ulcerative Colitis: A Randomised Placebo-Controlled Trial. Lancet 389, 1218–1228. doi: 10.1016/S0140-6736(17)30182-4
Qin, H., Zhang, Z., Hang, X., Jiang, Y. (2009). L. Plantarum Prevents Enteroinvasive Escherichia Coli-Induced Tight Junction Proteins Changes in Intestinal Epithelial Cells. BMC Microbiol. 9, 63. doi: 10.1186/1471-2180-9-63
Redman, M. G., Ward, E. J., Phillips, R. S. (2014). The Efficacy and Safety of Probiotics in People With Cancer: A Systematic Review. Ann. Oncol. 25, 1919–1929. doi: 10.1093/annonc/mdu106
Reiff, C., Kelly, D. (2010). Inflammatory Bowel Disease, Gut Bacteria and Probiotic Therapy. Int. J. Med. Microbiol. 300, 25–33. doi: 10.1016/j.ijmm.2009.08.004
Ren, R. R., Sun, G., Yang, Y. S., Peng, L. H., Wang, S. F., Shi, X. H., et al. (2016). Chinese Physicians’ Perceptions of Fecal Microbiota Transplantation. World J. Gastroenterol. 22, 4757–4765. doi: 10.3748/wjg.v22.i19.4757
Riedel, C. U., Foata, F., Philippe, D., Adolfsson, O., Eikmanns, B. J., Blum, S. (2006). Anti-Inflammatory Effects of Bifidobacteria by Inhibition of LPS-Induced NF-KappaB Activation. World J. Gastroenterol. 12, 3729–3735. doi: 10.3748/wjg.v12.i23.3729
Riehl, T. E., Alvarado, D., Ee, X., Zuckerman, A., Foster, L., Kapoor, V., et al. (2019). Lactobacillus Rhamnosus GG Protects the Intestinal Epithelium From Radiation Injury Through Release of Lipoteichoic Acid, Macrophage Activation and the Migration of Mesenchymal Stem Cells. Gut 68, 1003–1013. doi: 10.1136/gutjnl-2018-316226
Roy, S., Trinchieri, G. (2017). Microbiota: A Key Orchestrator of Cancer Therapy. Nat. Rev. Cancer 17, 271–285. doi: 10.1038/nrc.2017.13
Sánchez de Medina, F., Romero-Calvo, I., Mascaraque, C., Martínez-Augustin, O. (2014). Intestinal Inflammation and Mucosal Barrier Function. Inflamm. Bowel Dis. 20, 2394–2404. doi: 10.1097/MIB.0000000000000204
Sartor, R. B. (2006). Mechanisms of Disease: Pathogenesis of Crohn’s Disease and Ulcerative Colitis. Nat. Clin. Pract. Gastroenterol. Hepatol. 3, 390–407. doi: 10.1038/ncpgasthep0528
Schulz, M. D., Atay, C., Heringer, J., Romrig, F. K., Schwitalla, S., Aydin, B., et al. (2014). High-Fat-Diet-Mediated Dysbiosis Promotes Intestinal Carcinogenesis Independently of Obesity. Nature 514, 508–512. doi: 10.1038/nature13398
Sender, R., Fuchs, S., Milo, R. (2016). Are We Really Vastly Outnumbered? Revisiting the Ratio of Bacterial to Host Cells in Humans. Cell 164, 337–340. doi: 10.1016/j.cell.2016.01.013
Shaw, C., Taylor, L. (2012). Treatment-Related Diarrhea in Patients With Cancer. Clin. J. Oncol. Nurs. 16, 413–417. doi: 10.1188/12.CJON.413-417
Shen, Z., Wang, J., Huang, Q., Shi, Y., Wei, Z., Zhang, X., et al. (2018). Genetic Modification to Induce CXCR2 Overexpression in Mesenchymal Stem Cells Enhances Treatment Benefits in Radiation-Induced Oral Mucositis. Cell Death Dis. 9, 229. doi: 10.1038/s41419-018-0310-x
Sherman, P. M., Johnson-Henry, K. C., Yeung, H. P., Ngo, P. S. C., Goulet, J., Tompkins, T. A. (2005). Probiotics Reduce Enterohemorrhagic Escherichia Coli O157: H7-And Enteropathogenic E-Coli O127: H6-Induced Changes in Polarized T84 Epithelial Cell Monolayers by Reducing Bacterial Adhesion and Cytoskeletal Rearrangements. Infect. Immun. 73, 5183–5188. doi: 10.1128/IAI.73.8.5183-5188.2005
Siegel, R., Desantis, C., Virgo, K., Stein, K., Mariotto, A., Smith, T., et al. (2012). Cancer Treatment and Survivorship Statistic. CA Cancer J. Clin. 62, 220–241. doi: 10.3322/caac.21149
Sokol, H., Adolph, T. E. (2018). The Microbiota: An Underestimated Actor in Radiation-Induced Lesions? Gut 67, 1–2. doi: 10.1136/gutjnl-2017-314279
Sommer, F., Backhed, F. (2013). The Gut Microbiota - Masters of Host Development and Physiology. Nat. Rev. Microbiol. 11, 227–238. doi: 10.1038/nrmicro2974
Sonis, S. T. (2004). The Pathobiology of Mucositis. Nat. Rev. Cancer 4, 277–284. doi: 10.1038/nrc1318
Sonis, S. T., Elting, L. S., Keefe, D., Peterson, D. E., Schubert, M., Hauer-Jensen, M., et al. (2004). Perspectives on Cancer Therapy-Induced Mucosal Injury: Pathogenesis, Measurement, Epidemiology, and Consequences for Patients. Cancer 100, 1995–2025. doi: 10.1002/cncr.20162
Sonnenburg, J. L., Backhed, F. (2016). Diet-Microbiota Interactions as Moderators of Human Metabolism. Nature 535, 56–64. doi: 10.1038/nature18846
Stringer, A. M. (2013). Interaction Between Host Cells and Microbes in Chemotherapy-Induced Mucositis. Nutrients 5, 1488–1499. doi: 10.3390/nu5051488
Stringer, A. M., Al-Dasooqi, N., Bowen, J. M., Tan, T. H., Radzuan, M., Logan, R. M., et al. (2013). Biomarkers of Chemotherapy-Induced Diarrhoea: A Clinical Study of Intestinal Microbiome Alterations, Inflammation and Circulating Matrix Metalloproteinases. Support Care Cancer 21, 1843–1852. doi: 10.1007/s00520-013-1741-7
Tankou, S. K., Regev, K., Healy, B. C., Cox, L. M., Tjon, E., Kivisakk, P., et al. (2018). Investigation of Probiotics in Multiple Sclerosis. Mult. Scler. 24, 58–63. doi: 10.1177/1352458517737390
Tarricone, R., Abu Koush, D., Nyanzi-Wakholi, B., Medina-Lara, A. (2016). A Systematic Literature Review of the Economic Implications of Chemotherapy-Induced Diarrhea and Its Impact on Quality of Life. Crit. Rev. Oncol. Hematol. 99, 37–48. doi: 10.1016/j.critrevonc.2015.12.012
Theis, V. S., Sripadam, R., Ramani, V., Lal, S. (2010). Chronic Radiation Enteritis. Clin. Oncol. (R Coll. Radiol) 22, 70–83. doi: 10.1016/j.clon.2009.10.003
Ulluwishewa, D., Anderson, R. C., Mcnabb, W. C., Moughan, P. J., Wells, J. M., Roy, N. C. (2011). Regulation of Tight Junction Permeability by Intestinal Bacteria and Dietary Components. J. Nutr. 141, 769–776. doi: 10.3945/jn.110.135657
Uribe, G., Villéger, R., Bressollier, P., Dillard, R. N., Worthley, D. L., Wang, T. C., et al. (2018). Lactobacillus Rhamnosus GG Increases Cyclooxygenase-2 Expression and Prostaglandin E2 Secretion in Colonic Myofibroblasts via a MyD88-Dependent Mechanism During Homeostasis. Cell Microbiol. 20, 12871. doi: 10.1111/cmi.12871
van Vliet, M. J., Harmsen, H. J., De Bont, E. S., Tissing, W. J. (2010). The Role of Intestinal Microbiota in the Development and Severity of Chemotherapy-Induced Mucositis. PLoS Pathog. 6, 1000879. doi: 10.1371/journal.ppat.1000879
Wang, Z., Wang, Q., Wang, X., Zhu, L., Chen, J., Zhang, B., et al. (2019). Gut Microbial Dysbiosis is Associated With Development and Progression of Radiation Enteritis During Pelvic Radiotherapy. J. Cell Mol. Med. 23, 3747–3756. doi: 10.1111/jcmm.14289
Wang, Y., Xiang, G. S., Kourouma, F., Umar, S. (2006). Citrobacter Rodentium-Induced NF-KappaB Activation in Hyperproliferating Colonic Epithelia: Role of P65 (Ser536) Phosphorylation. Br. J. Pharmacol. 148, 814–824. doi: 10.1038/sj.bjp.0706784
Xiao, H. W., Cui, M., Li, Y., Dong, J. L., Zhang, S. Q., Zhu, C. C., et al. (2020). ). Gut Microbiota-Derived Indole 3-Propionic Acid Protects Against Radiation Toxicity via Retaining Acyl-CoA-Binding Protein. Microbiome 8, 69. doi: 10.1186/s40168-020-00845-6
Xu, J., Gordon, J. I. (2003). Honor Thy Symbionts. Proc. Natl. Acad. Sci. U. S. A. 100, 10452–10459. doi: 10.1073/pnas.1734063100
Xu, L., Zhang, T., Cui, B., He, Z., Xiang, J., Long, C., et al. (2016). Clinical Efficacy Maintains Patients’ Positive Attitudes Toward Fecal Microbiota Transplantation. Medicine (Baltimore) 95, 4055. doi: 10.1097/MD.0000000000004055
Youngster, I., Russell, G. H., Pindar, C., Ziv-Baran, T., Sauk, J., Hohmann, E. L. (2014). Oral, Capsulized, Frozen Fecal Microbiota Transplantation for Relapsing Clostridium Difficile Infection. JAMA 312, 1772–1778. doi: 10.1001/jama.2014.13875
Zhao, Z., Cheng, W., Qu, W., Shao, G., Liu, S. (2020). Antibiotic Alleviates Radiation-Induced Intestinal Injury by Remodeling Microbiota, Reducing Inflammation, and Inhibiting Fibrosis. ACS Omega 5, 2967–2977. doi: 10.1021/acsomega.9b03906
Keywords: microbiota, radiation enteritis, intestinal epithelial barrier, inflammatory cytokines, probiotics
Citation: Jian Y, Zhang D, Liu M, Wang Y and Xu Z-X (2021) The Impact of Gut Microbiota on Radiation-Induced Enteritis. Front. Cell. Infect. Microbiol. 11:586392. doi: 10.3389/fcimb.2021.586392
Received: 23 July 2020; Accepted: 12 July 2021;
Published: 29 July 2021.
Edited by:
Ganwu Li, Iowa State University, United StatesReviewed by:
Philip Sherman, University of Toronto, CanadaLeilei Yu, Jiangnan University, China
Xin Cong, Peking University, China
Copyright © 2021 Jian, Zhang, Liu, Wang and Xu. This is an open-access article distributed under the terms of the Creative Commons Attribution License (CC BY). The use, distribution or reproduction in other forums is permitted, provided the original author(s) and the copyright owner(s) are credited and that the original publication in this journal is cited, in accordance with accepted academic practice. No use, distribution or reproduction is permitted which does not comply with these terms.
*Correspondence: Zhi-Xiang Xu, emhpeGlhbmd4dTA4QGdtYWlsLmNvbQ==; Yishu Wang, d2FuZ3lzQGpsdS5lZHUuY24=
†ORCID: Yongping Jian, orcid.org/0000-0002-0676-2247
Yishu Wang, orcid.org/0000-0002-4759-8498
Zhi-Xiang Xu, orcid.org/0000-0002-8209-9678