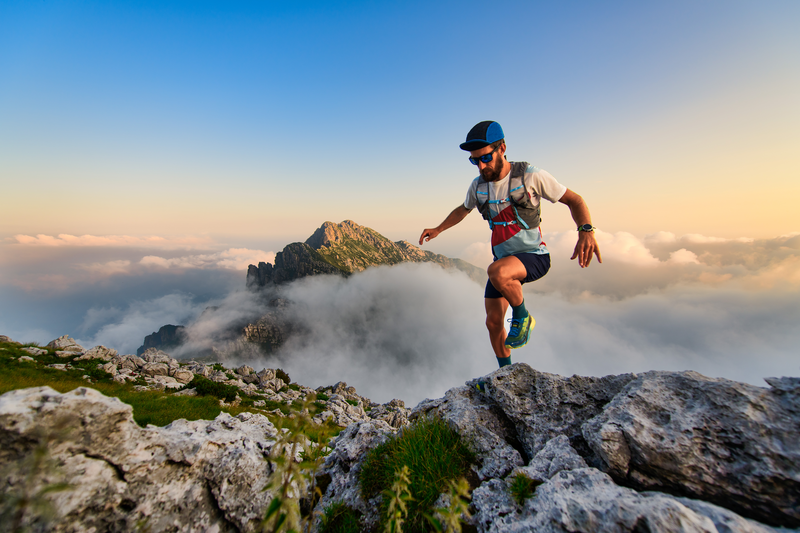
94% of researchers rate our articles as excellent or good
Learn more about the work of our research integrity team to safeguard the quality of each article we publish.
Find out more
ORIGINAL RESEARCH article
Front. Cell. Infect. Microbiol. , 10 December 2020
Sec. Clinical Microbiology
Volume 10 - 2020 | https://doi.org/10.3389/fcimb.2020.561741
This article is part of the Research Topic Enterobacteriaceae Antimicrobial Agents and Resistance: Relationship with the Therapeutic Approach View all 12 articles
Carbapenem-resistant Klebsiella pneumoniae (CRKP) is a prominent cause of nosocomial infections associated with high rates of morbidity and mortality, particularly in oncological patients. The hypermucoviscous (HMV) phenotype and biofilm production are key factors for CRKP colonization and persistence in the host. This study aims at exploring the impact of CRKP virulence factors on morbidity and mortality in oncological patients. A total of 86 CRKP were collected between January 2015 and December 2019. Carbapenem resistance-associated genes, antibiotic susceptibility, the HMV phenotype, and biofilm production were evaluated. The median age of the patients was 71 years (range 40–96 years). Clinically infected patients were 53 (61.6%), while CRKP colonized individuals were 33 (38.4%). The most common infectious manifestations were sepsis (43.4%) and pneumonia (18.9%), while rectal surveillance swabs were the most common site of CRKP isolation (81.8%) in colonized patients. The leading mechanism of carbapenem resistance was sustained by the KPC gene (96.5%), followed by OXA-48 (2.3%) and VIM (1.2%). Phenotypic CRKP characterization indicated that 55.8% of the isolates were strong biofilm-producers equally distributed between infected (54.2%) and colonized (45.8%) patients. The HMV phenotype was found in 22.1% of the isolates, which showed a significant (P<0.0001) decrease in biofilm production as compared to non-HMV strains. The overall mortality rate calculated on the group of infected patients was 35.8%. In univariate analysis, pneumoniae significantly correlated with death (OR 5.09; CI 95% 1.08–24.02; P=0.04). The non-HMV phenotype (OR 4.67; CI 95% 1.13–19.24; P=0.03) and strong biofilm-producing strains (OR 5.04; CI95% 1.39–18.25; P=0.01) were also associated with increased CRKP infection-related mortality. Notably, the multivariate analysis showed that infection with strong biofilm-producing CRKP was an independent predictor of mortality (OR 6.30; CI 95% 1.392–18.248; P=0.004). CRKP infection presents a high risk of death among oncological patients, particularly when pneumoniae and sepsis are present. In infected patients, the presence of strong biofilm-producing CRKP significantly increases the risk of death. Thus, the assessment of biofilm production may provide a key element in supporting the clinical management of high-risk oncological patients with CRKP infection.
Klebsiella pneumoniae is a major human pathogen with mortality rates up to 50%, particularly in immune-compromised individuals (Kanj and Kanafani, 2011; David et al., 2019). It causes a broad spectrum of diseases including pneumonia, urinary tract infections, bloodstream infections, skin and soft tissue infections (Melot et al., 2015; Pitout et al., 2015; Paczosa and Mecsas, 2016; David et al., 2019). Carbapenems are often considered the last line therapy for the treatment of multidrug-resistant K. pneumoniae (Tzouvelekis et al., 2012; David et al., 2019). However, global surveillance studies indicate that a significant fraction of nosocomial K. pneumoniae isolates display extended-spectrum β-lactamases (ESBLs) and carbapenemases activities (Molton et al., 2013; Morrissey et al., 2013; Pitout et al., 2015; Brescini et al., 2019). The endemic distribution of carbapenem-resistant K. pneumoniae (CRKP) has been reported worldwide (Munoz-Price et al., 2013). In European countries, the population-weighted mean percentage of CRKP is 7.2%. Greece, Italy, and Romania had the highest rates of CRKP as compared to the rest of Europe (Cassini et al., 2019). Dissemination of CRKP is primarily sustained by the horizontal transfer of carbapenemase genes on mobile elements (Mathers et al., 2011; Martin et al., 2017; Partridge et al., 2018). K. pneumoniae carbapenemase (KPC), imipenemase metallo β-lactamase (IMP), New Delhi metallo β-lactamase (NDM), Verona integron metallo β-lactamase (VIM), and oxacillinase-48 (OXA-48) are the most common carbapenemases in CRKP (Meletis, 2016; Partridge et al., 2018). Treatment for CRKP infections is often limited to colistin, which represents, in many cases, a last-resort option due to its nephrotoxicity and neurotoxicity (Karaiskos et al., 2017). More recently, novel combinations of β-lactam- β-lactamase inhibitors, such as ceftazidime-avibactam and meropenem-vaborbactam, have been found effective against CRKP producing KPC-type and OXA-48-like enzymes, but not for those strains producing metallo carbapenemases (Bassetti et al., 2018).
The production of capsular polysaccharide is the prominent virulence factor of K. pneumoniae that allow this bacterium to overcome innate host immunity (Zhang et al., 2016). Currently, more than 130 different capsule types have been recognized for Klebsiella (Follador et al., 2016). A recent study demonstrated that K. pneumoniae can enhance its pathogenicity by adopting two opposing strategies based on the capsule biosynthesis. The first is related to hypercapsule production, which confers phagocytosis resistance, enhanced dissemination, and higher mortality in animal models (Ernst et al., 2020). Alternatively, K. pneumoniae can acquire mutations impairing capsule production, thus allowing enhanced epithelial cell invasion, increased persistence in urinary tract infections, and biofilm formation (Ernst et al., 2020). Hypervirulent strains of K. pneumoniae can be identified by a hypermucoviscous (HMV) phenotype on agar plates, as a result of a positive string test (Compain et al., 2014). HMV subtypes, initially described in 1986, are characterized by increased production of a capsular substance compared with classic K. pneumoniae, which confers a HMV phenotype (Casanova et al., 1989). Mutations in genes reducing capsule production affect the HMV phenotype and correlate with a substantial reduction in virulence when tested in mice (Walker and Miller, 2020). Thus, the HMV phenotype is directly linked with the amount of capsule production. However, a recent study demonstrated that a mutation in a gene encoding a transcriptional regulator of the mucoid phenotype (RmpC) reduces capsule production but does not affect the HMV phenotype (Walker et al., 2019). This finding suggests that HMV is dependent on the presence of the capsule, but HMV and capsule have to be considered independently (Walker and Miller, 2020). HMV, isolates showed an increased ability to cause both severe community-acquired infections such as pneumonia, liver abscesses, and meningitis in young, healthy individuals, and healthcare-associated invasive infections (Fang et al., 2007; Turton et al., 2010; Decre et al., 2011; Liu and Guo, 2019). Most HMV K pneumoniae strains have been related to the capsular type K1, and, in a lower fraction, with the serotype K2 (Alcantar-Curiel and Giron, 2015; Gu et al., 2018; Cubero et al., 2019) both reported as antibiotic-sensitive (Yeh et al., 2007; Gu et al., 2018). However, in recent years, carbapenem-resistant HMV strains have been reported worldwide (Gu et al., 2018; Huang et al., 2018; Lev et al., 2018; Ferreira et al., 2019; Wang et al., 2020).
Biofilm production is also important to the virulence of K. pneumoniae because the biofilm matrix facilitates the transfer of antibiotic-resistance mobile elements while physically protecting bacteria, thus increasing microbial tolerance to antibiotics, bacterial persistence, and dissemination (Clegg and Murphy, 2016; Ribeiro et al., 2016; Cubero et al., 2019). Biofilm eradication requires high antimicrobial concentrations, which are often impossible to achieve due to drug-related toxicity. Thus, relapses are frequent even after targeted and prolonged therapies (Clegg and Murphy, 2016; Di Domenico et al., 2019). Despite its role in microbial virulence, biofilm is not routinely assessed in clinical microbiology, and diagnosis of biofilm-related infection, in most cases, can only be presumed based on clinical signs and symptoms (Di Domenico et al., 2016).
This study analyzes the impact of different CRKP virulence determinants to assess their predictivity in supporting clinical decision-making in high-risk oncological patients.
This retrospective study was performed at the San Gallicano Dermatological Institute and Regina Elena National Cancer Institute, Rome, Italy, between January 2015 and December 2019.
The Central Ethics Committee I.R.C.C.S. Lazio, approved the study (Prot. CE/1016/15—15 December 2015, trials registry N. 730/15).
The samples were collected from a total of 86 oncological patients colonized or infected with CRKP. Bacterial identification was performed by matrix-assisted laser desorption/ionization-time of flight mass spectrometry (MALDI-TOF MS) system (Bruker Daltonik, Bremen, Germany). The antimicrobial susceptibility was assessed by the VITEK® 2 system (bioMérieux, Marcy l’Étoile, France) (Lucarelli et al., 2017). Susceptibility for colistin and ceftazidime/avibactam was determined by the Sensititre broth microdilution method (Thermo Scientific, New Jersey, USA), and results were interpreted according to the European Committee on Antimicrobial Susceptibility Testing (EUCAST) clinical breakpoints (http://www.eucast.org/clinical_breakpoints). The presence of blaKPC, blaVIM, blaOXA-48, blaIMP-1, blaNDM types was determined by the Cepheid Xpert® Carba-R assay and the GeneXpert® device (Cepheid, Sunnyvale, USA).
Biofilm production was assessed by the clinical BioFilm Ring Test (cBRT) (Biofilm Control, Saint Beauzire, France), as described in Di Domenico et al., 2016. Briefly, an overnight culture of K. pneumoniae grown on a blood agar plate was used to inoculate 2 ml of 0.45% saline solution to 1.0 ± 0.3 McFarland turbidity standard. The bacterial suspension was used to inoculate a 96-well polystyrene plate with 200 μl/well. The test was performed using the toner solution (TON004) containing magnetic beads 1% (v/v) mixed in the Brain Heart Infusion medium. Ten-fold serial dilutions were performed in a volume of 200 μl BHI/TON mix. K. pneumoniae ATCC700603 and K. pneumoniae ATCC 13883 were included in each plate as standard reference and internal control. After 5 h of incubation at 37°C in a static condition, wells were covered with contrast liquid, placed for 1 min on the block carrying 96 mini-magnets, and scanned with a plate reader (Pack BIOFILM, Biofilm Control, Saint Beauzire, France). The adhesion strength of each strain was expressed as BioFilm Index (BFI). Each K. pneumoniae strain was classified as weak moderate and high biofilm producers (Di Domenico et al., 2016; Di Domenico et al., 2017). Besides, moderate and high biofilm producers were grouped and classified as strong biofilm producers (Di Domenico et al., 2019). Each K. pneumoniae isolate was analyzed in duplicate, and experiments were repeated three times.
The HMV phenotype of the CRKP isolates was revealed by the string test as described previously (Zhan et al., 2017; Liu et al., 2019).
Overnight cultures were pelleted by centrifugation at 9,000×g and resuspended in PBS to an OD600 of 1. The suspensions were centrifuged at 1,000×g for 5 min, and the OD600 of the supernatants was measured. Readings were normalized to the OD600 of the strains before centrifugation (Bachman et al., 2015; Walker et al., 2019).
Continuous variables were compared by Student’s t-test for normally distributed variables and the Mann-Whitney U test for non-normally distributed variables. Categorical variables were evaluated using the χ2 or two-tailed Fisher’s exact test. Univariate and multivariate analyses were carried by a logistic regression model to identify independent risk factors for 30-days mortality. Statistical analyses were carried out using IBM SPSS v.21 statistics software.
From January 2015 to December 2019, 86 consecutive patients infected or colonized with CRKP were included in the study. Patients’ demographic and clinical characteristics are described in Table 1. The most represented underlying malignancy was hepato-bilio-pancreatic cancer (27.9%), urinary tract cancer (24.4%), hematologic malignancy (12.8%), and gastrointestinal cancer (12.8%) (Table 1). Infected patients were 61.6% (N53), while colonized patients accounted for 38.4% (N33). A concomitant fungal infection was detected in 5.8% (N5) of patients. Among infected patients, the most frequent manifestation caused by CRKP was sepsis (N23; 43.4%) followed by pneumoniae (N10; 18.9%), urinary tract infections (N7; 13.2%) and intra-abdominal infection (N5; 9.4%). CRKP caused 9 cases of catheter-related bloodstream infections and one case of catheter-acquired urinary infection. Among colonized patients, rectal surveillance swabs (RSS) were the most common site of CRKP isolation (N27; 81.8%) followed by urine samples (N4; 12.1%).
Based on genotypic characterization, the leading mechanism of carbapenem resistance was related to the KPC gene (N83, 96.5%), followed by OXA-48 (N2, 2.3%) and VIM (N1, 1.2%). None of the strains analyzed were positive for the class B metallo-β-lactamases IMP and NDM. The OXA-48 and VIM were only isolated from RSS in colonized patients, while all CRKP from infected patients were KPC-producing K. pneumoniae strains. The antimicrobial susceptibility profile confirmed that almost all the CRKP strains were resistant to three carbapenems with a high level of resistance to all tested beta-lactams (Table 2). Among the CRKP strains, 10.5% (N9) were also resistant to colistin. Notably, only one strain were found resistant to ceftazidime-avibactam. The only CRKP isolate resistant to ceftazidime-avibactam was the VIM-positive strain. In the colistin-resistant group, seven strains were isolated from infected patients and two from colonized individuals. Among aminoglycosides, 25.6% (N22) of CRKP strains were susceptible to amikacin, and 17.4% (N15) were susceptible to gentamycin. Trimethoprim/sulfamethoxazole-susceptible isolates were 23.3% (N20), while fosfomycin and tigecycline were below the breakpoints in only 13.9% (N12) and 12.7% (N11) of cases, respectively. Notably, only 2.3% (N2) of the CRKP strains were found to be susceptible to ciprofloxacin.
Phenotypic CRKP characterization indicated that 22.1% (N19) of the isolates were HMV, and 77.9% (N67) were classified as non-HMV. The HMV isolates showed a positive string test result (Figure 1A). The median length of the string was 7 mm (ranging from 5–25 mm). The mucoviscosisty levels were determined by the sedimentation assay. HMV strains do not sediment properly during low-speed centrifugation, and the supernatant remains turbid, while the non-HMV strains produce compact pellets with clear supernatants. The turbidity of supernatant can be measured by the optical density at 600 nm (OD600) (Walker and Miller, 2020). The OD600 of HMV strains was 0.32±0.12 and, non-HMV was 0.13±0.08 (P<0.001) (Figure 1B).
Figure 1 String test for identification of the HMV phenotype. A positive string test (A) is defined as the formation of viscous strings of >5 mm in length on an agar plate. (B) Sedimentation assay for HMV and non-HMV isolates.
One HMV isolate was found colistin-resistant and eight were non-HMV. Due to the low number of colistin-resistant compared to colistin-susceptible strains the difference was no statistically significant. Besides, HMV and non-HMV isolates did not show any significant association to infected or colonized patients as well as to a specific site of isolation.
Among the 86 CRKP isolates, 55.8% (N48) were classified as strong biofilm producers, while 44.2% (N38) showed a weak production (Table 1). Strong biofilm-producing CRKP were equally distributed in both infected (N26) and colonized (N22) patients, while weak biofilm-producing strains were more abundant in infected (N27) as compared to colonized patients (N11). Although the level of biofilm was not significantly related to the site of isolation, strong biofilm producers were detected in 80% of BAL from patients with pneumoniae, 63% of urine samples, 63% of RSS, and 43.5% of blood cultures of septic patients (Figure 2). Among the colistin-resistant isolates, six were classified as strong and three as weak biofilm producers. The degree of biofilm was not significantly associated with colistin resistance. Noteworthy, biofilm production was significantly different in HMV and non-HMV strains (P=0.0002), with the former being mostly weak biofilm producers (88.2%) as compared to non-HMV (33.3%) isolates (Figure 2). Confocal microscopy analysis of the biofilms was performed after 24 h of incubation (Figure 3). The strong biofilm-producing CRKP isolates (Figure 3A) formed a compact 15–25 μm thick multi-layered structure. Conversely, weak biofilm-producing strains, including non-HMV (Figure 3B) and HMV (Figure 3C) isolates, were scattered over the polystyrene slide surface and no three-dimensional structure could be observed.
Figure 2 Biofilm formation of CRKP clinical isolates according to (A) the site of isolation and (B) the HMV and non-HMV phenotype.
Figure 3 Representative confocal microscopy images of CRKP biofilms developed on polystyrene slides for 24 h at 37°C. (A) Strong biofilm-producing non-HMV isolates. (B) Weak biofilm-producing non-HMV and (C) Weak biofilm-producing HMV strain. Orthogonal sections displaying horizontal (z) and side views (x and y) of reconstructed 3D biofilm images are shown.
None of the patients colonized with CRKP died in the study period. Therefore, the 30-day mortality rate was calculated on the group of infected patients (Table 3). Infection-related mortality in this group was 35.8% (N19). In univariate analysis, a significantly high proportion of patients dying within 30 days had pneumoniae (OR 5.09; CI 95% 1.08–24.02; P=0.04). The presence of colistin resistance was not significantly related to increased attributable mortality in this group of patients (OR 3.08; CI 95% 0.33–28.77; P=0.32). Likewise, a concomitant fungal infection was not correlated with increased 30-day mortality (OR 4.00; CI 95% 0.33–47.73; P=0.27). Among the CRKP virulence factors, either the presence of a non-HMV phenotype (OR 4.67; CI 95% 1.13–19.24; P=0.03) or the presence of strong biofilm-producing isolates (OR 5.04; CI 95% 1.39–18.25; P=0.01) represents a significant predictive element for 30-day mortality. Further assessment of CRKP virulence factors by multivariate analysis gave a strong biofilm-producing phenotype as the only independent predictor of mortality (OR 6.30; CI 95% 1.78–19.24; P=0.004).
Table 3 Univariate and multivariate analyses of factors associated with for 30-day mortality in 53 patients infected with carbapenem-resistant K. pneumoniae.
Infections caused by CRKP represent a considerable clinical challenge, often burdened by a delay in the introduction of appropriate antimicrobial therapy, prolonged hospitalization, and considerable mortality rates (Gasink et al., 2009; Mouloudi et al., 2010; Freire et al., 2015; David et al., 2019). Therefore, understanding the impact of microbial infection/colonization factors on the outcome of CRKP-induced diseases may help improve patient management and prognosis.
This study analyzed data from 86 oncological patients with an infection or colonization sustained by CRKP. We found that the leading mechanism of carbapenem resistance was due to the expression of the KPC gene, present in 96.5% of the isolates, followed by OXA-48 and VIM, found in 2.3 and 1.2% of cases, respectively. These data, though from a limited group of strains, are consistent with previous epidemiological studies. Indeed, in Italy, approximately 90% of the CRKP isolates carry the KPC gene, followed by VIM (9.2%) and, in a small percentage, by OXA-48 (1.3%) (Giani et al., 2015; Navon-Venezia et al., 2017; Ripabelli et al., 2018; Di Tella et al., 2019). Different classes of carbapenemases exhibit specific functional properties and susceptibilities, which may be clinically relevant (Cassini et al., 2019). Therefore, information regarding the molecular mechanism leading to carbapenem resistance may also provide a guide in antibiotic selection and administration upon suspicion of infection (Giannella et al., 2014).
The antimicrobial susceptibility profile confirmed that almost all the CRKP strains assessed in this study were resistant to three carbapenems with high resistance levels against all the β-lactams tested (Table 2). Novel β-lactam/β-lactamase inhibitor combinations have been recently introduced as new treatment options against infections caused by carbapenem-resistant Enterobacteriaceae (Karaiskos et al., 2019; Sheu et al., 2019). Recent evidence indicates that ceftazidime-avibactam may represent an effective treatment for CRKP infections (Shields et al., 2016; van Duin and Bonomo, 2016; Krapp et al., 2017; Caston et al., 2017; Tumbarello et al., 2019). Indeed, ceftazidime-avibactam inhibits KPC and OXA-48 enzymes, but it is not active against the metallo-β-lactamases (ECDC EARS-NET report, 2017; Shirley, 2018; Sousa et al., 2018; Ambretti et al., 2019). Consistently with these observations, our results show that ceftazidime-avibactam is effective against KPC and OXA-48 but not against CRKP strains harboring the VIM gene (Shirley, 2018; García-Castillo et al., 2018).
Colistin is considered as an antibiotic of last resort for treating severe CRKP infections, because of increasing microbial resistance and associated toxicity (Elnahriry et al., 2016; Schwarz and Johnson, 2016; Rojas et al., 2017; Wang et al., 2018; Ghirga et al., 2020). In this study, we found a 10.5% of CRKP resistant to colistin. This result is consistent with studies performed worldwide, which confirm a colistin-resistant rate not exceeding 8.8%–13% among CRKP isolates, as assessed by broth microdilution (Goel et al., 2014; Olaitan et al., 2014; Rojas et al., 2017; Zafer et al., 2019). Previous colistin therapy was considered an independent risk factor for colistin resistance among CRKP (Giacobbe et al., 2015). In this study, the prevalence of colistin-resistant clinical CRKP isolates was relatively low. Such colistin resistance rates may indicate that infection prevention procedures and antimicrobial stewardship adopted in our institution have reduced the selective pressure, limiting the spread of colistin resistance. In our colistin-resistant group, seven strains were isolated from infected patients and two from a colonized individual. Notably, we did not observe a statistically significant difference in mortality rates between patients infected with colistin-resistant and colistin-susceptible isolates. This observation is consistent with a recent study showing that the patient’s conditions and not the presence of colistin-resistant strains have the most significant impact on the clinical outcome (Brescini et al., 2019). However, other studies pointed to a direct association between colistin-resistant strains and mortality (Giacobbe et al., 2015; Rojas et al., 2017). In particular, results from a multicenter study conducted in Italy, in which a 20% colistin resistance was found, reported a mortality rate significantly higher than that observed in patients infected with colistin-susceptible strains (Giacobbe et al., 2015).
The HMV strains represent a serious health threat, causing severe infections in both immune-compromised and healthy individuals (Shon and Russo, 2012; Shon et al., 2013; Gu et al., 2018; Liu and Guo, 2019). In critically ill patients, such as those from intensive care units, HMV K. pneumoniae can induce invasive infection and syndromes (Lee et al., 2010; Liu and Guo, 2019). Thus, the assessment of the HMV phenotype by the string test has been proposed as a necessary addition into the daily practice of microbiological surveillance in ICU (Hagiya et al., 2014). Globally, the prevalence of HMV strains in K. pneumoniae isolates is reported in the range of 17%–45% (Yu et al., 2006; Liu and Guo, 2019). The HMV strains are usually highly susceptible to antibiotics, and infections can be generally treated with success using carbapenems (Shon and Russo, 2012; Holt et al., 2015). Nevertheless, sporadic reports of isolation of carbapenemase-producing HMV strains are emerging worldwide, mostly occurring in hospitalized patients (Arena et al., 2017; Gu et al., 2018; Simner et al., 2018). In our study, CRKP-HMV strains accounted for 22.1% of the total isolates. This result is in contrast with previously reported epidemiological data showing a prevalence of about 1% (Gu et al., 2018; Simner et al., 2018; Liu and Guo, 2019). An important concern when considering the highly susceptible HMV strains is their ability to became resistant to carbapenems when subjected to a meropenem regimen (Simner et al., 2018). The carbapenem resistance in HMV appears to be maintained only in the presence of meropenem and is lost after antibiotic removal (Huang et al., 2013; Simner et al., 2018). This suggests that the presence of carbapenemase-encoding plasmids in HMV strains may someway harm bacterial fitness and is dispensable in the absence of selective pressure (Huang et al., 2013; Simner et al., 2018). Such instability may recognize several possible causes and associated factors, including the specific K. pneumoniae strains, the type of plasmid incompatibility groups and/or the acquisition of different carbapenemase genes (Simner et al., 2018). The exposure to multiple cycles of prolonged antibiotic treatment in our group of hospitalized patients might have exerted the selective pressure necessary to acquire and preserve carbapenemase genes in such a high number of strains. If true, this further emphasizes the judicious use of antibiotics to limit the development and spread of antibiotic resistance in hypervirulent strains of K. pneumoniae. Of importance, we found that non-HMV strains were associated with a significant increase in infection-related mortality. This is in contrast with a previous study describing high mortality rates caused by HMV K. pneumoniae strains (Shon and Russo, 2012). However, some controversies exist regarding the HMV classification and its putative virulence (Lin et al., 2011; Zhang et al., 2015). In animal models, HMV strains did not show more severe infections or higher mortality rates as compared to non-HMV (Zhang et al., 2016; Catalan-Najera et al., 2017). Besides, CRKP with an HMV phenotype were found to produce a significantly lower amount of biofilm compared to non-HMV isolates, suggesting that exopolysaccharides production has a negative impact on CRKP fitness (Cubero et al., 2019). This further confirms that the presence of the capsular polysaccharides reduces bacterial adhesion probably by the shielding of the fimbrial adhesins (Wang et al., 2015; Wang et al., 2017). However, in this reduced ability of adhesion may reside an advantage of the HMV strains. Indeed, capsule allows tighter bacterial packing, as compared to capsule-deficient cells, promoting an increased ability to disseminate to distant sites, including the lung, eye, soft tissue and central nervous system (Dzul et al., 2011; Choby et al., 2020). On the other hand, biofilm production may explain, at least in part, the association of non-HMV strains with a significant increase in infection-related mortality, since most non-HMV strains (55.8%) were strong biofilm producers, being equally distributed between infected and colonized patients. Notably, in infected patients, the presence of strong biofilm-producing CRKP significantly (P=0.01) correlated with increased mortality. Strong biofilm producers were detected in 80% of pneumonia cases, 63% of urine samples, 63% of RSS, and 43.5% of blood cultures. The fraction of strong biofilm-producing CRKP observed in this study is consistent with previous reports (Di Domenico et al., 2017; Vuotto et al., 2017; Nirwati et al., 2019; Ramos-Vivas et al., 2019). Studies directed at assessing carbapenem-susceptible K. pneumoniae isolated from blood, respiratory specimens, urine, and wounds, found strong biofilm producers in percentages ranging from 65% to 85% (Yang and Zhang, 2008; Hassan et al., 2011; Seifi et al., 2016; Cepas et al., 2019). The analysis of biofilm production in vitro showed a large variation among K. pneumoniae isolates according to the microenvironment, the surface where the biofilm adheres, temperature, pH, and the physicochemical characteristic of the isolate. A number of reports have pointed to an association between higher level of biofilm formation and the acquisition of a multidrug-resistant phenotype in K. pneumoniae (Yang and Zhang, 2008; Subramanian et al., 2012; Sanchez et al., 2013; Vuotto et al., 2017; Bocanegra-Ibarias et al., 2017; Cepas et al., 2019; Nirwati et al., 2019). In particular, an increased rate of horizontal gene transfer among bacteria growing in close contact within the biofilm matrix is deemed responsible for the rapid acquisition of antibiotic resistance, both at the single and multispecies levels (Ghigo, 2001; Madsen et al., 2012; Lebeaux et al., 2014). Despite these findings, the association between antibiotic resistance and biofilm formation is still debated (De Campos et al., 2016; Di Domenico et al., 2017; Cepas et al., 2019).
The overall CRKP infection-related mortality rate observed in the present study was 35.8%. This figure is consistent with recent studies reporting mortality rates of approximately 40% in Italy and other European countries (Hoxha et al., 2016; Xu et al., 2017; Ramos-Castañeda et al., 2018). However, geographic variations, as well as co-morbidities, should be considered. Studies in South America gave figures of 51.0% of CRKP-related mortality while in North America, a 33.2% mortality rate was reported (Rossi Gonçalves et al., 2016; GBD 2015, 2017; Xu et al., 2017). In immune-compromised patients, CRKP infection gave mortality rates higher than those observed in our study, particularly when considering patients undergoing liver transplantation (78%), or patients with hematologic malignancies and solid tumors (56%–73%) (Lübbert et al., 2013; Satlin et al., 2013; Freire et al., 2015; Ramos-Castañeda et al., 2018). We found the highest rate of mortality in patients with pneumoniae and sepsis. Similar results, in association with additional factors, including a high APACHE score, inappropriate initial antimicrobial therapy, advanced age and shock, were previously found among cancer patients infected with multidrug-resistant agents (Gasink et al., 2009; Souli et al., 2010; Zarkotou et al., 2011; Tumbarello et al., 2012; Bodro et al., 2014; Xu et al., 2017).
Assessment of these data by univariate analysis indicated that both a Non-HMV phenotype (P=0.001) and a strong biofilm-producing strain (P=0.01) are predictive of an increased CRKP infection-related mortality. Besides, multivariate analysis indicated that the presence of strong biofilm-producing CRKP strains was the only microbial factor independently associated with death (95% CI, 1.78-19.24; P=0.004) in oncological patients infected with CRKP. This result is also supported by previous study demonstrating that biofilm formation contributes to increased K. pneumoniae pathogenicity (Wu et al., 2011; Ernst et al., 2020). These data further support the notion that biofilm production represents a key CRKP virulence factor, which protects bacteria from physical and chemical insults, including antimicrobials, supporting microbial persistence and dissemination The effective antibiotic concentration required for biofilm eradication in vivo is, in most cases, impossible to reach due to drug toxicity and side effects (Ciofu et al., 2015). Therefore, the diagnosis of a biofilm-associated infection represents an area of serious concern for the clinical management of patients. The timely recognition of a strong biofilm producer, before the development of a mature biofilm matrix, may provide key decision-making elements for most appropriate targeting of either medical or surgical intervention, including type, doses, duration of antimicrobial therapy or removal of medical devices, respectively. However, conventional antimicrobial susceptibility testing performed on planktonic cells does not detect the additional resistance mechanism provided by biofilm. Thus, the introduction of reliable microbiological platforms for the diagnosis of biofilm-associated infections and the determination of biofilm-induced antibiotic tolerance represents a desirable addition in clinical microbiology.
Although bringing relevant information, this study has a few limitations. Being a retrospective study performed in a single oncological Hospital, our epidemiology findings might differ from those emerging from other experiences. Nevertheless, data from this study indicated that the mortality rate among oncological patients infected with CRKP is high (35.8%). The infection-related mortality rate did not correlate with the presence of HMV strains but, conversely, was significantly associated with non-HMV, strong biofilm-producing isolates, the latter representing an independent risk factor of death in oncological patients infected with CRKP. A more in-depth exploration of the mechanisms promoting biofilm formation in K. pneumoniae will help identify specific virulence markers. Nevertheless, the timely recognition of biofilm-associated infections and biofilm-induced drug tolerance still represents an unmet need in clinical microbiology.
The raw data supporting the conclusions of this article will be made available by the authors, without undue reservation.
The studies involving human participants were reviewed and approved by The Central Ethics Committee I.R.C.C.S. Lazio, approved the study (Prot. CE/1016/15—15 December 2015, trials registry N. 730/15). Written informed consent for participation was not required for this study in accordance with the national legislation and the institutional requirements.
ED designed the study and wrote the paper. FM, GP, FP, IS, LP, FA, LT, AL, AM, and FE, discussed the results and implications and wrote the manuscript. ICa, FS, and TK performed the phenotypic and genomics experiments. FDS, ICe, and SP analyzed the presence of the carbapenemase genes. TK and CP collected and interpreted the clinical data. All authors contributed to the article and approved the submitted version.
The authors declare that the research was conducted in the absence of any commercial or financial relationships that could be construed as a potential conflict of interest.
The reviewer AA declared a shared affiliation with the authors to the handling editor at time of review.
Alcantar-Curiel M. D., Giron J. A. (2015). Klebsiella pneumoniae and the pyogenic liver abscess: implications and association of the presence of rpmA genes and expression of hypermucoviscosity. Virulence 6, 407–409. doi: 10.1080/21505594.2015.1030101
Ambretti S., Bassetti M., Clerici P., Petrosillo N., Tumietto F., Viale P., et al. (2019). Screening for carriage of carbapenem-resistant Enterobacteriaceae in settings of high endemicity: a position paper from an Italian working group on CRE infections. Antimicrob. Resist. Infect. Control 8, 136. doi: 10.1186/s13756-019-0591-6
Arena F., Henrici De Angelis L., D’Andrea M. M., Cannatelli A., Fossati L., Di Pilato V., et al. (2017). Infections caused by carbapenem-resistant Klebsiella pneumoniae with hypermucoviscous phenotype: a case report and literature review. Virulence 8, 1900–1908. doi: 10.1080/21505594.2017.1286439
Bachman M. A., Breen P., Deornellas V., Mu Q., Zhao L., Wu W., et al. (2015). Genome-Wide Identification of Klebsiella pneumoniae Fitness Genes during Lung Infection. mBio 6, e00775. doi: 10.1128/mBio.00775-15
Bassetti M., Righi E., Carnelutti A., Graziano E., Russo A. (2018). Multidrug-resistant Klebsiella pneumoniae? Challenges for treatment, prevention and infection control. Expert Rev. Anti Infect. Ther. 16, 749–761. doi: 10.1080/14787210.2018.1522249
Bocanegra-Ibarias P., Garza-Gonzalez E., Morfin-Otero R., Barrios H., Villarreal-Trevino L., Rodriguez-Noriega E., et al. (2017). Molecular and microbiological report of a hospital outbreak of NDM-1-carrying Enterobacteriaceae in Mexico. PLoS One 12, e0179651. doi: 10.1371/journal.pone.0179651
Bodro M., Gudiol C., Garcia-Vidal C., Tubau F., Contra A., Boix L., et al. (2014). Epidemiology, antibiotic therapy and outcomes of bacteremia caused by drugresistant ESKAPE pathogens in cancer patients. Support Care Cancer 22, 603–610. doi: 10.1007/s00520-013-2012-3
Brescini L., Morroni G., Valeriani C., Castelletti S., Mingoia M., Simoni S., et al. (2019). Clinical and epidemiological characteristics of KPC-producing Klebsiella pneumoniae from bloodstream infections in a tertiary referral center in Italy. BMC Infect. Dis. 19, 611. doi: 10.1186/s12879-019-4268-9
Casanova C., Lorente J. A., Carrillo F., Perez-Rodriguez E., Nunez N. (1989). Klebsiella pneumoniae liver abscess associated with septic endophthalmitis. Arch. Intern. Med. 149, 1467. doi: 10.1001/archinte.1989.00390060171048
Cassini A., Högberg L. D., Plachouras D., Quattrocchi A., Hoxha A., Simonsen G. S., et al. (2019). Attributable deaths and disability-adjusted life-years caused by infections with antibiotic-resistant bacteria in the EU and the European Economic Area in 2015: a population-level modelling analysis. Lancet Infect. Dis. 19, 56–66. doi: 10.1016/S1473-3099(18)30605-4
Caston J. J., Lacort-Peralta I., Martin-Davila P., Loeches B., Tabares S., Temkin L., et al. (2017). Clinical efficacy of ceftazidime/avibactam versus other active agents for the treatment of bacteremia due to carbapenemase-producing Enterobacteriaceae in hematologic patients. Int. J. Infect. Dis. 59, 118–123. doi: 10.1016/j.ijid.2017.03.021
Catalan-Najera J. C., Garza-Ramos U., Barrios-Camacho H. (2017). Hypervirulence and hypermucoviscosity: two different but complementary Klebsiella spp. phenotypes? Virulence 8, 1–13. doi: 10.1080/21505594.2017.1317412
Cepas V., Lopez Y., Munoz E., Rolo D., Ardanuy C., Marti S., et al. (2019). Relationship between biofilm formation and antimicrobial resistance in gram-negative Bacteria. Microb. Drug Resist. 25, 72–79. doi: 10.1089/mdr.2018.0027
Choby J. E., Howard-Anderson J., Weiss D. S. (2020). Hypervirulent Klebsiella pneumoniae - clinical and molecular perspectives. J. Intern. Med. 287, 283–300. doi: 10.1111/joim.13007
Ciofu O., Tolker-Nielsen T., Jensen P. Ø., Wang H., Høiby N. (2015). Antimicrobial resistance, respiratory tract infections and role of biofilms in lung infections in cystic fibrosis patients. Adv. Drug Delivery Rev. 85, 7–23. doi: 10.1016/j.addr.2014.11.017
Clegg S., Murphy C. N. (2016). Epidemiology and virulence of Klebsiella pneumoniae. Microbiol. Spectr. 4, UTI-0005-2012. doi: 10.1128/microbiolspec.UTI-0005-2012
Compain F., Babosan A., Brisse S., Genel N., Audo J., Ailloud F., et al. (2014). Multiplex PCR for detection of seven virulence factors and K1/K2 capsular serotypes of Klebsiella pneumoniae. J. Clin. Microbiol. 52, 4377–4380. doi: 10.1128/JCM.02316-14
Cubero M., Marti S., Domínguez M.Á., González-Díaz A., Berbel D., Ardanuy C. (2019). Hypervirulent Klebsiella pneumoniae serotype K1 clinical isolates form robust biofilms at the air-liquid interface. PLoS One 14, e0222628. doi: 10.1371/journal.pone.0222628
David S., Reuter S., Harris S. R., Glasner C., Feltwell T., Argimon S., et al. (2019). Epidemic of carbapenem-resistant Klebsiella pneumoniae in Europe is driven by nosocomial spread. Nat. Microbiol. 4, 1919–1929. doi: 10.1038/s41564-019-0492-8
De Campos P. A., Royer S., Batistao D. W., Araujo B. F., Queiroz L. L., de Brito C. S., et al. (2016). Multidrug resistance related to biofilm formationin Acinetobacter baumannii and Klebsiella pneumoniae clinical strains fromdifferent Pulsotypes. Curr. Microbiol. 72, 617–627. doi: 10.1007/s00284-016-0996-x
Decre D., Verdet C., Emirian A., Le Gourrierec T., Petit J. C., Offenstadt G., et al. (2011). Emerging severe and fatal infections due to Klebsiella pneumoniae in two university hospitals in France. J. Clin. Microbiol. 49, 3012–3014. doi: 10.1128/JCM.00676-11
Di Domenico E. G., Toma L., Provot C., Ascenzioni F., Sperduti I., Prignano G., et al. (2016). Development of an in vitro Assay, Based on the BioFilm Ring Test®, for Rapid Profiling of Biofilm-Growing Bacteria. Front. Microbiol. 7, 1429. eCollection 2016. doi: 10.3389/fmicb.2016.01429
Di Domenico E. G., Farulla I., Prignano G., Gallo M. T., Vespaziani M., Cavallo I., et al. (2017). Biofilm is a Major Virulence Determinant in Bacterial Colonization of Chronic Skin Ulcers Independently from the Multidrug Resistant Phenotype. Int. J. Mol. Sci. 18, E1077. doi: 10.3390/ijms18051077
Di Domenico E. G., Rimoldi S. G., Cavallo I., D’Agosto G., Trento E., Cagnoni G., et al. (2019). Microbial biofilm correlates with an increased antibiotic tolerance and poor therapeutic outcome in infective endocarditis. BMC Microbiol. 19, 228. doi: 10.1186/s12866-019-1596-2
Di Tella D., Tamburro M., Guerrizio G., Fanelli I., Sammarco M. L., Ripabelli G. (2019). Molecular Epidemiological Insights into Colistin-Resistant and Carbapenemases-Producing Clinical Klebsiella pneumoniae Isolates. Infect. Drug Resist. 12, 3783–3795. doi: 10.2147/IDR.S226416
Dzul S. P., Thornton M. M., Hohne D. N., Stewart E. J., Shah A. A., Bortz D. M., et al. (2011). Contribution of the Klebsiella pneumoniae capsule to bacterial aggregate and biofilm microstructures. Appl. Environ. Microbiol. 77, 1777–1782. doi: 10.1128/AEM.01752-10
Elnahriry S. S., Khalifa H. O., Soliman A. M., Ahmed A. M., Hussein A. M., Shimamoto T., et al. (2016). Emergence of plasmid-mediated colistin resistance gene mcr-1 in a clinical Escherichia coli isolate from Egypt. Antimicrob. Agents Chemother. 60, 3249–3250. doi: 10.1128/AAC.00269-16
Ernst C. M., Braxton J. R., Rodriguez-Osorio C. A., Zagieboylo A. P., Li L., Pironti A., et al. (2020). Adaptive evolution of virulence and persistence in carbapenem-resistant Klebsiella pneumoniae. Nat. Med. 26, 705–711. doi: 10.1038/s41591-020-0825-4
European Centre for Disease Prevention and Control (ECDC) (2018). Surveillance of antimicrobial resistance in Europe 2017. Annual Report of the European Antimicrobial Resistance Surveillance Network (EARS-Net). Stockholm: ECDC. Available from: https://ecdc.europa.eu/sites/portal/files documents/EARSNet- report-2017-update-jan-2019.pdf.
Fang C. T., Lai S. Y., Yi W. C., Hsueh P. R., Liu K. L., Chang S. C. (2007). Klebsiella pneumoniae genotype K1: an emerging pathogen that causes septic ocular or central nervous system complications from pyogenic liver abscess. Clin. Infect. Dis. 45, 284–293. doi: 10.1086/519262
Ferreira R. L., da Silva B. C. M., Rezende G. S., Nakamura-Silva R., Pitondo-Silva A., Campanini E. B., et al. (2019). High Prevalence of Multidrug-Resistant Klebsiella pneumoniae Harboring Several Virulence and β-Lactamase Encoding Genes in a Brazilian Intensive Care Unit. Front. Microbiol. 9:3198:3198. doi: 10.3389/fmicb.2018.03198
Follador R., Heinz E., Wyres K. L., Ellington M. J., Kowarik M., Holt K. E., et al. (2016). The diversity of Klebsiella pneumoniae surface polysaccharides. Microb. Genomics 2, e000073. doi: 10.1099/mgen.0.000073
Freire M. P., Pierrotti L. C., Filho H. H., Ibrahim K. Y., Magri A. S., Bonazzi P. R., et al. (2015). Infection with Klebsiella pneumoniae carbapenemase (KPC)-producing Klebsiella pneumoniae in cancer patients. Eur. J. Clin. Microbiol. Infect. Dis. 34, 277–286. doi: 10.1007/s10096-014-2233-5
García-Castillo M., García-Fernández S., Gómez-Gil R., Pitart C., Oviaño M., Gracia-Ahufinger I., et al. (2018). Activity of ceftazidime-avibactam against carbapenemase-producing Enterobacteriaceae from urine specimens obtained during the infection-carbapenem resistance evaluation surveillance trial (iCREST) in Spain. Int. J. Antimicrob. Agents 51, 511–515. doi: 10.1016/j.ijantimicag.2018.01.011
Gasink L. B., Edelstein P. H., Lautenbach E., Synnestvedt M., Fishman N. O. (2009). Risk factors and clinical impact of Klebsiella pneumoniae carbapenemase-producing K. pneumoniae. Infect. Control Hosp. Epidemiol. 30, 1180–1185. doi: 10.1086/648451
GBD 2015 Healthcare Access and Quality Collaborators (2017). Healthcare Access and Quality Index based on mortality from causes amenable to personal health care in 195 countries and territories 1990–2015: a novel analysis from the Global Burden of Disease Study 2015. Lancet 390, 231–266. doi: 10.1016/S0140-6736(17)30818-8
Ghigo J. M. (2001). Natural conjugative plasmids induce bacterial biofilm development. Nature 412, 442–445. doi: 10.1038/35086581
Ghirga F., Stefanelli R., Cavinato L., Lo Sciuto A., Corradi S., Quaglio D., et al. (2020). A novel colistin adjuvant identified by virtual screening for ArnT inhibitors. J. Antimicrob. Chemother. 75 (9), 2564–2572. doi: 10.1093/jac/dkaa200
Giacobbe D. R., Del Bono V., Trecarichi E. M., De Rosa F. G., Giannella M., Bassetti M., et al. (2015). Risk factors for bloodstream infections due to colistin-resistant KPC-producing Klebsiella pneumoniae: results from a multicenter case–control–control study. Clin. Microbiol. Infect. 21, 1106 e1–8. doi: 10.1016/j.cmi.2015.08.001
Giani T., Arena F., Vaggelli G., Conte V., Chiarelli A., Henrici De Angelis L., et al. (2015). Large nosocomial outbreak of colistin-resistant, carbapenemase-producing Klebsiella pneumoniae traced to clonal expansion of an mgrB deletion mutant. J. Clin. Microbiol. 53, 3341–3344. doi: 10.1128/JCM.01017-15
Giannella M., Trecarichi E. M., De Rosa F. G., Del Bono V., Bassetti M., Lewis R. E., et al. (2014). Risk factors for carbapenem-resistant Klebsiella pneumoniae bloodstream infection among rectal carriers: a prospective observational multicentre study. Clin. Microbiol. Infect. 20, 1357–1362. doi: 10.1111/1469-0691.12747
Goel G., Hmar L., De Sarkar M., Bhattacharya S., Chandy M. (2014). Colistin resistant Klebsiella pneumoniae: report of a cluster of 24 cases from a new oncology center in eastern India. Infect. Control Hosp. Epidemiol. 35, 1076–1077. doi: 10.1086/677170
Gu D., Dong N., Zheng Z., Lin D., Huang M., Wang L., et al. (2018). A fatal outbreak of ST11 carbapenem-resistant hypervirulent Klebsiella pneumoniae in a Chinese hospital: a molecular epidemiological study. Lancet Infect. Dis. 18, 37–46. doi: 10.1016/S1473-3099(17)30489-9
Hagiya H., Watanabe N., Maki M., Murase T., Otsuka F. (2014). Clinical utility of string test as a screening method for hypermucoviscosity-phenotype Klebsiella pneumoniae. Acute Med. Surg. 1, 245–246. doi: 10.1002/ams2.40
Hassan A., Usman J., Kaleem F., Omair M., Khalid A., Iqbal M. (2011). Evaluation of different detection methods of biofilm formation in the clinical isolates. Braz. J. Infect. Dis. 15, 305–311. doi: 10.1016/S1413-8670(11)70197-0
Holt K. E., Wertheim H., Zadoks R. N., Baker S., Whitehouse C. A., Dance D., et al. (2015). Genomic analysis of diversity, population structure, virulence, and antimicrobial resistance in Klebsiella pneumoniae, an urgent threat to public health. Proc. Natl. Acad. Sci. U.S.A. 112, E3574–EE3581. doi: 10.1073/pnas.1501049112
Hoxha A., Kärki T., Giambi C., Montano C., Sisto A., Bella A., et al. (2016). Attributable mortality of carbapenem-resistant Klebsiella pneumoniae infections in a prospective matched cohort study in Italy 2012-2013. J. Hosp. Infect. 92, 61–66. doi: 10.1016/j.jhin.2015.06.018
Huang T. W., Chen T. L., Chen Y. T., Lauderdale T. L., Liao T. L., Lee Y. T., et al. (2013). Copy number change of theNDM-1 sequence in a multidrug-resistant Klebsiella pneumoniae clinical isolate. PLoS One 8, e62774. doi: 10.1371/journal.pone.0062774
Huang Y. H., Chou S. H., Liang S. W., Ni C. E., Lin Y. T., Huang Y. W., et al. (2018). Emergence of an XDR and carbapenemaseproducing hypervirulent Klebsiella pneumoniae strain in Taiwan. J. Antimicrob. Chemother. 73, 2039–2046. doi: 10.1093/jac/dky164
Kanj S., Kanafani Z. (2011). Current Concepts in Antimicrobial Therapy Against Resistant Gram-Negative Organisms: Extended-Spectrum β-Lactamase–Producing Enterobacteriaceae, Carbapenem-Resistant Enterobacteriaceae, and Multidrug-Resistant Pseudomonas aeruginosa. Mayo Clin. Proc. 86, 250–259. doi: 10.4065/mcp.2010.0674
Karaiskos I., Souli M., Galani I., Giamarellou H. (2017). Colistin: still a lifesaver for the 21st century? Expert Opin. Drug Metab. Toxicol. 13, 59–71. doi: 10.1080/17425255.2017.1230200
Karaiskos I., Galani I., Souli M., Giamarellou H. (2019). Novel beta-lactam-betalactamase inhibitor combinations: expectations for the treatment of carbapenem-resistant gram-negative pathogens. Expert Opin. Drug Metab. Toxicol. 15, 133–149. doi: 10.1080/17425255.2019.1563071
Krapp F., Grant J. L., Sutton S. H., Ozer E. A., Barr V. O. (2017). Treating complicated carbapenem-resistant enterobacteriaceae infections with ceftazidime/avibactam: a retrospective study with molecular strain characterisation. Int. J. Antimicrob. Agents 49, 770–773. doi: 10.1016/j.ijantimicag.2017.01.018
Lübbert C., Becker-Rux D., Rodloff A. C., Laudi S., Busch T., Bartels M., et al. (2013). Colonization of liver transplant recipients with KPC-producing Klebsiella pneumoniae is associated with high infection rates and excess mortality: a case–control analysis. Infection 42, 309–316. doi: 10.1007/s15010-013-0547-3
Lebeaux D., Ghigo J. M., Beloin C. (2014). Biofilm-related infections: Bridging the gap between clinical management and fundamental aspects of recalcitrance toward antibiotics. Microbiol. Mol. Biol. Rev. 78, 510–543. doi: 10.1128/MMBR.00013-14
Lee C. H., Liu J. W., Su L. H., Chien C. C., Li C. C., Yang K. D. (2010). Hypermucoviscosity associated with Klebsiella pneumoniaemediated invasive syndrome: a prospective cross-sectional study in Taiwan. Int. J. Infect. Dis. 14, e688–e692. doi: 10.1016/j.ijid.2010.01.007
Lev A. I., Astashkin E. I., Kislichkina A. A., Solovieva E. V., Kombarova T. I., Korobova O. V., et al. (2018). Comparative analysis of Klebsiella pneumoniae strains isolated in 2012-2016 that differ by antibiotic resistance genes and virulence genes profiles. Pathog. Glob. Health 112, 142–151. doi: 10.1080/20477724.2018.1460949
Lin Y. C., Lu M. C., Tang H. L., Liu H. C., Chen C. H., Liu K. S., et al. (2011). Assessment of hypermucoviscosity as a virulence factor for experimental Klebsiella pneumoniae infections: comparative virulence analysis with hypermucoviscosity-negative strain. BMC Microbiol. 11, 50. doi: 10.1186/1471-2180-11-50
Liu C., Guo J. (2019). Hypervirulent Klebsiella pneumoniae (hypermucoviscous and aerobactin positive) infection over 6 years in the elderly in China: antimicrobial resistance patterns, molecular epidemiology and risk factor. Ann. Clin. Microbiol. Antimicrob. 18, 4. doi: 10.1186/s12941-018-0302-9
Liu Z., Gu Y., Li X., Liu Y., Ye Y., Guan S., et al. (2019). Identification and Characterization of NDM-1-producing Hypervirulent (Hypermucoviscous) Klebsiella pneumoniae in China. Ann. Lab. Med. 39, 167–175. doi: 10.3343/alm.2019.39.2.167
Lucarelli C., Di Domenico E. G., Toma L., Bracco D., Prignano G., Fortunati M., et al. (2017). Ralstonia mannitolilytica infections in an oncologic day ward: description of a cluster among high-risk patients. Antimicrob. Resist. Infect. Control 6, 20. doi: 10.1186/s13756-017-0178-z
Madsen J. S., Burmølle M., Hansen L. H., Sørensen S. J. (2012). The interconnection between biofilm formation and horizontal gene transfer. FEMS Immunol. Med. Microbiol. 65, 183–195. doi: 10.1111/j.1574-695X.2012.00960.x
Martin J., Phan H. T. T., Findlay J., Stoesser N., Pankhurst L., Navickaite I., et al. (2017). Covert dissemination of carbapenemase-producing Klebsiella pneumoniae (KPC) in a successfully controlled outbreak: long- and short-read whole-genome sequencing demonstrate multiple genetic modes of transmission. J. Antimicrob. Chemother. 72, 3025–3034. doi: 10.1093/jac/dkx264
Mathers A. J., Cox H. L., Kitchel B., Bonatti H., Brassinga A. K., Carroll J., et al. (2011). Molecular dissection of an outbreak of carbapenem resistant Enterobacteriaceae reveals intergenus KPC carbapenemase transmission through a promiscuous plasmid. mBio 2, e00204–e00211. doi: 10.1128/mBio.00204-11
Meletis G. (2016). Carbapenem resistance: overview of the problem and future perspectives. Ther. Adv. Infect. Dis. 3, 15–21. doi: 10.1177/2049936115621709
Melot B., Colot J., Guerrier G. (2015). Bacteremic community-acquired infections due to Klebsiella pneumoniae: clinical and microbiological presentation in New Caledonia 2008–2013. Int. J. Infect. Diseases: IJID: Off. Publ. Int. Soc. Infect. Dis. 41, 29–31. doi: 10.1016/j.ijid.2015.10.013
Molton J. S., Tambyah P. A., Ang B. S., Ling M. L., Fisher D. A. (2013). The global spread of healthcare-associated multidrug-resistant bacteria: a perspective from Asia. Clin. Infect. Dis. 56, 1310–1318. doi: 10.1093/cid/cit020
Morrissey I., Hackel M., Badal R., Bouchillon S., Hawser S., Biedenbach D. (2013). A review of ten years of the Study for Monitoring Antimicrobial Resistance Trends (SMART) from 2002 to 2011. Pharmaceuticals 6, 1335–1346. doi: 10.3390/ph6111335
Mouloudi E., Protonotariou E., Zagorianou A., Iosifidis E., Karapanagiotou A., Giasnetsova T., et al. (2010). Bloodstream infections caused by metallo-β-lactamase/Klebsiella pneumoniae carbapenemase producing K. pneumoniae among intensive care unit patients in Greece: risk factors for infection and impact of type of resistance on outcomes. Infect. Control Hosp. Epidemiol. 31, 1250–1256. doi: 10.1086/657135
Munoz-Price L. S., Poirel L., Bonomo R. A., Schwaber M. J., Daikos G. L., Cormican M., et al. (2013). Clinical epidemiology of the global expansion of Klebsiella pneumoniae carbapenemases. Lancet Infect. Dis. 13, 785–796. doi: 10.1016/S1473-3099(13)70190-7
Navon-Venezia S., Kondratyeva K., Carattoli A. (2017). Klebsiella pneumoniae: a major worldwide source and shuttle for antibiotic resistance. FEMS Microbiol. Rev. 41, 252–275. doi: 10.1093/femsre/fux013
Nirwati H., Sinanjung K., Fahrunissa F., Wijaya F., Napitupulu S., Hati V. P., et al. (2019). Biofilm formation and antibiotic resistance of Klebsiella pneumoniae isolated from clinical samples in a tertiary care hospital, Klaten, Indonesia. BMC Proc. 13, 20. doi: 10.1186/s12919-019-0176-7
Olaitan A. O., Diene S. M., Kempf M., Berrazeg M., Bakour S., Gupta S. K., et al. (2014). Worldwide emergence of colistin resistance in Klebsiella pneumoniae from healthy humans and patients in Lao PDR, Thailand, Israel, Nigeria and France owing to inactivation of the PhoP/PhoQ regulator mgrB: an epidemiological and molecular study. Int. J. Antimicrob. Agents 44, 500–507. doi: 10.1016/j.ijantimicag.2014.07.020
Paczosa M. K., Mecsas J. (2016). Klebsiella pneumoniae: going on the offense with a strong defense. Microbiol. Mol. Biol. Rev. 80, 629–661. doi: 10.1128/MMBR.00078-15
Partridge S. R., Kwong S. M., Firth N., Jensen S. O. (2018). Mobile Genetic Elements Associated with Antimicrobial Resistance. Clin. Microbiol. Rev. 31, e00088–e00017. doi: 10.1128/CMR.00088-17
Pitout J. D., Nordmann P., Poirel L. (2015). Carbapenemase-Producing Klebsiella pneumoniae, a Key Pathogen Set for Global Nosocomial Dominance. Antimicrob. Agents Chemother. 59, 5873–5884. doi: 10.1128/AAC.01019-15
Ramos-Castañeda J. A., Ruano-Ravina A., Barbosa-Lorenzo R., Paillier-Gonzalez J. E., Saldaña-Campos J. C., Salinas D. F., et al. (2018). Mortality due to KPC carbapenemase-producing Klebsiella pneumoniae infections: Systematic review and meta-analysis: Mortality due to KPC Klebsiella pneumoniae infections. J. Infect. 76, 438–448. doi: 10.1016/j.jinf.2018.02.007
Ramos-Vivas J., Chapartegui-González I., Fernández-Martínez M., González-Rico C., Fortún J., Escudero R., et al. (2019). Biofilm formation by multidrug resistant Enterobacteriaceae strains isolated from solid organ transplant recipients. Sci. Rep. 9, 8928. doi: 10.1038/s41598-019-45060-y
Ribeiro S. M., Cardoso M. H., Candido Ede S., Franco O. L. (2016). Understanding, preventing and eradicating Klebsiella pneumoniae biofilms. Future Microbiol. 11, 527–538. doi: 10.2217/fmb.16.7
Ripabelli G., Tamburro M., Guerrizio G., Fanelli I., Flocco R., Scutellà M., et al. (2018). Tracking multidrug-resistant Klebsiella pneumoniae from an Italian hospital: molecular epidemiology and surveillance by PFGE, RAPD and PCR-based resistance genes prevalence. Curr. Microbiol. 75, 977–987. doi: 10.1007/s00284-018-1475-3
Rojas L. J., Salim M., Cober E., Richter S. S., Perez F., Salata R. A., et al. (2017). Colistin resistance in Carbapenem-resistant Klebsiella pneumoniae: laboratory detection and impact on mortality. Clin. Infect. Dis. 64, 711–718. doi: 10.1093/cid/ciw805
Rossi Gonçalves I., Ferreira M. L., Araujo B. F., Campos P. A., Royer S., Batistão D. W., et al. (2016). Outbreaks of colistin-resistant and colistin-susceptible KPC-producing Klebsiella pneumoniae in a Brazilian intensive care unit. J. Hosp. Infect. 94, 322–329. doi: 10.1016/j.jhin.2016.08.019
Sanchez Jr C. J., Mende K., Beckius M. L., Akers K. S., Romano D. R., Wenke J. C., et al. (2013). Biofilm formation by clinical isolates and the implications in chronic infections. BMC Infect. Dis. 13, 47. doi: 10.1186/1471-2334-13-47
Satlin M. J., Calfee D. P., Chen L., Fauntleroy K. A., Wilson S. J., Jenkins S. G., et al. (2013). Emergence of carbapenem-resistant Enterobacteriaceae as causes of bloodstream infections in patients with hematologic malignancies. Leuk. Lymphoma 54, 799–806. doi: 10.3109/10428194.2012.723210
Schwarz S., Johnson A. P. (2016). Transferable resistance to colistin: a new but old threat. J. Antimicrob. Chemother. 71, 2066–2070. doi: 10.1093/jac/dkw274
Seifi K., Kazemian H., Heidari H., Rezagholizadeh F., Saee Y., Shirvani F., et al. (2016). Evaluation of biofilm formation among Klebsiella pneumoniae isolates and molecular characterization by ERIC-PCR. Jundishapur J. Microbiol. 9, e30682. doi: 10.5812/jjm.30682
Sheu C. C., Chang Y. T., Lin S. Y., Chen Y. H., Hsueh P. R. (2019). Infections caused by Carbapenem-resistant Enterobacteriaceae: an update on therapeutic options. Front. Microbiol. 10, 80. doi: 10.3389/fmicb.2019.00080
Shields R. K., Potoski B. A., Haidar G., Hao B., Doi Y., Chen L., et al. (2016). Clinical outcomes, drug toxicity, and emergence of ceftazidime-avibactam resistance among patients treated for Carbapenem-resistant Enterobacteriaceae infections. Clin. Infect. Dis. 63, 1615–1618. doi: 10.1093/cid/ciw636
Shirley M. (2018). Ceftazidime-avibactam: a review in the treatment of serious gram-negative bacterial infections. Drugs 78, 675–692. doi: 10.1007/s40265-018-0902-x
Shon A. S., Russo T. A. (2012). Hypervirulent Klebsiella pneumoniae: the next superbug? Future Microbiol. 7, 669 – 671. doi: 10.2217/fmb.12.43
Shon A. S., Bajwa R. P., Russo T. A. (2013). Hypervirulent (hypermucoviscous) Klebsiella pneumoniae: a new and dangerous breed. Virulence 4, 107–118. doi: 10.4161/viru.22718
Simner P. J., Antar A. A. R., Hao S., Gurtowski J., Tamma P. D., Rock C., et al. (2018). Antibiotic pressure on the acquisition and loss of antibiotic resistance genes in Klebsiella pneumoniae. J. Antimicrob. Chemother. 73, 1796–1803. doi: 10.1093/jac/dky121
Souli M., Galani I., Antoniadou A., Papadomichelakis E., Poulakou G., Panagea T., et al. (2010). An outbreak of infection due to beta-lactamase Klebsiella pneumoniae carbapenemase 2-producing K. Pneumoniae in a Greek university hospital: molecular characterization, epidemiology, and outcomes. Clin. Infect. Dis. 50, 364–373. doi: 10.1086/649865
Sousa A., Perez-Rodriguez M. T., Soto A., Rodriguez L., Perez-Landeiro A., Martinez-Lamas L., et al. (2018). Effectiveness of ceftazidime/avibactam as salvage therapy for treatment of infections due to OXA-48 carbapenemase-producing Enterobacteriaceae. J. Antimicrob. Chemother. 73, 3170–3175. doi: 10.1093/jac/dky295
Subramanian P., Shanmugam N., Sivaraman U., Kumar S., Selvaraj S. (2012). Antiobiotic resistance pattern of biofilm-forming uropathogens isolated from catheterised patients in Pondicherry. India Australas. Med. J. 5, 344–348. doi: 10.4066/AMJ.2012.1193
Tumbarello M., Viale P., Viscoli C., Trecarichi E. M., Tumietto F., Marchese A., et al. (2012). Predictors of mortality in bloodstream infections caused by Klebsiella pneumoniae carbapenemase-producing K. pneumoniae: importance of combination therapy. Clin. Infect. Dis. 55, 943–950. doi: 10.1093/cid/cis588
Tumbarello M., Trecarichi E. M., Corona A., De Rosa F. G., Bassetti M., Mussini C., et al. (2019). Efficacy of ceftazidimeavibactam salvage therapy in patients with infections caused by Klebsiella pneumoniae Carbapenemase-producing K. pneumoniae. Clin. Infect. Dis. 68, 355–364. doi: 10.1093/cid/ciy492
Turton J. F., Perry C., Elgohari S., Hampton C. V. (2010). PCR characterization and typing of Klebsiella pneumoniae using capsular type-specific, variable number tandem repeat and virulence gene targets. J. Med. Microbiol. 59, 541–547. doi: 10.1099/jmm.0.015198-0
Tzouvelekis L. S., Markogiannakis A., Psichogiou M., Tassios P. T., Daikos G. L. (2012). Carbapenemases in Klebsiella pneumoniae and other Enterobacteriaceae: an evolving crisis of global dimensions. Clin. Microbiol. Rev. 25, 682–707. doi: 10.1128/CMR.05035-11
van Duin D., Bonomo R. A. (2016). Ceftazidime/avibactam and Ceftolozane/Tazobactam: second-generation beta-lactam/beta-lactamase inhibitor combinations. Clin. Infect. Dis. 63, 234–241. doi: 10.1093/cid/ciw243
Vuotto C., Longo F., Pascolini C., Donelli G., Balice M. P., Libori M. F., et al. (2017). Biofilm formation and antibiotic resistance in Klebsiella pneumoniae urinary strains. J. Appl. Microbiol. 123, 1003–1018. doi: 10.1111/jam.13533
Walker K. A., Miller V. L. (2020). The intersection of capsule gene expression, hypermucoviscosity and hypervirulence in Klebsiella pneumoniae. Curr. Opin. Microbiol. 54, 95–102. doi: 10.1016/j.mib.2020.01.006
Walker K. A., Miner T. A., Palacios M., Trzilova D., Frederick D. R., Broberg C. A., et al. (2019). A Klebsiella pneumoniae Regulatory Mutant Has Reduced Capsule Expression but Retains Hypermucoviscosity. mBio 10, e00089–e00019. doi: 10.1128/mBio.00089-19
Wang H., Wilksch J. J., Strugnell R. A., Gee M. L. (2015). Role of Capsular Polysaccharides in Biofilm Formation: An AFM Nanomechanics Study. ACS Appl. Mater. Interfaces 7, 13007–13013. doi: 10.1021/acsami.5b03041
Wang H., Wilksch J. J., Chen L., Tan J. W., Strugnell R. A., Gee M. L. (2017). Influence of Fimbriae on Bacterial Adhesion and Viscoelasticity and Correlations of the Two Properties with Biofilm Formation. Langmuir 33, 100–106. doi: 10.1021/acs.langmuir.6b03764
Wang X., Wang Y., Zhou Y., Li J., Yin W., Wang S., et al. (2018). Emergence of a novel mobile colistin resistance gene, mcr-8, in NDM-producing Klebsiella pneumonia. Emerg. Microbes Infect. 7, 122. doi: 10.1038/s41426-018-0124-z
Wu M. C., Lin T. L., Hsieh P. F., Yang H. C., Wang J. T. (2011). Isolation of genes involved in biofilm formation of a Klebsiella pneumoniae strain causing pyogenic liver abscess. PLoS One 6, e23500. doi: 10.1371/journal.pone.0023500
Xu L., Sun X., Ma X. (2017). Systematic review and meta-analysis of mortality of patients infected with carbapenem-resistant Klebsiella pneumoniae. Ann. Clin. Microbiol. Antimicrob. 16, 18. doi: 10.1186/s12941-017-0191-3
Yang D., Zhang Z. (2008). Biofilm-forming Klebsiella pneumoniae strains have greater likelihood of producing extended-spectrum beta-lactamases. J. Hosp. Infect. 68, 369–371. doi: 10.1016/j.jhin.2008.02.001
Yeh K. M., Kurup A., Siu L. K., Koh Y. L., Fung C. P., Lin J. C., et al. (2007). Capsular serotype K1 or K2, rather than magA and rmpA, is a major virulence determinant for Klebsiella pneumoniae liver abscess in Singapore and Taiwan. J. Clin. Microbiol. 45, 466–471. doi: 10.1128/JCM.01150-06
Yu W. L., Ko W. C., Cheng K. C., Lee H. C., Ke D. S., Lee C. C., et al. (2006). Association between rmpA and magA genes and clinical syndromes caused by Klebsiella pneumoniae in Taiwan. Clin. Infect. Dis. 42, 1351–1358. doi: 10.1086/503420
Zafer M. M., El-Mahallawy H. A., Abdulhak A., Amin M. A., Al-Agamy M. H., Radwan H. H. (2019). Emergence of colistin resistance in multidrug-resistant Klebsiella pneumoniae and Escherichia coli strains isolated from cancer patients. Ann. Clin. Microbiol. Antimicrob. 18, 40. doi: 10.1186/s12941-019-0339-4
Zarkotou O., Pournaras S., Tselioti P., Dragoumanos V., Pitiriga V., Ranellou K., et al. (2011). Predictors of mortality in patients with bloodstream infections caused by KPC-producing Klebsiella pneumoniae and impact of appropriate antimicrobial treatment. Clin. Microbiol. Infect. 17, 1798–1803. doi: 10.1111/j.1469-0691.2011.03514.x
Zhan L., Wang S., Guo Y., Jin Y., Duan J., Hao Z., et al. (2017). Outbreak by hypermucoviscous Klebsiella pneumoniae ST11 isolates with carbapenem resistance in a tertiary hospital in China. Front. Cell Infect. Microbiol. 7, 182. doi: 10.3389/fcimb.2017.00182
Zhang Y., Zeng J., Liu W., Zhao F., Hu Z., Zhao C., et al. (2015). Emergence of a hypervirulent carbapenem-resistant Klebsiella pneumoniae isolate from clinical infections in China. J. Infect. 71, 553–560. doi: 10.1016/j.jinf.2015.07.010
Keywords: biofilm, Klebsiella, carbapenem, skin colonization, cancer
Citation: Di Domenico EG, Cavallo I, Sivori F, Marchesi F, Prignano G, Pimpinelli F, Sperduti I, Pelagalli L, Di Salvo F, Celesti I, Paluzzi S, Pronesti C, Koudriavtseva T, Ascenzioni F, Toma L, De Luca A, Mengarelli A and Ensoli F (2020) Biofilm Production by Carbapenem-Resistant Klebsiella pneumoniae Significantly Increases the Risk of Death in Oncological Patients. Front. Cell. Infect. Microbiol. 10:561741. doi: 10.3389/fcimb.2020.561741
Received: 13 May 2020; Accepted: 10 November 2020;
Published: 10 December 2020.
Edited by:
Alessandra Oliva, Sapienza University of Rome, ItalyReviewed by:
Muhammad Ammar Zafar, Wake Forest School of Medicine, United StatesCopyright © 2020 Di Domenico, Cavallo, Sivori, Marchesi, Prignano, Pimpinelli, Sperduti, Pelagalli, Di Salvo, Celesti, Paluzzi, Pronesti, Koudriavtseva, Ascenzioni, Toma, De Luca, Mengarelli and Ensoli. This is an open-access article distributed under the terms of the Creative Commons Attribution License (CC BY). The use, distribution or reproduction in other forums is permitted, provided the original author(s) and the copyright owner(s) are credited and that the original publication in this journal is cited, in accordance with accepted academic practice. No use, distribution or reproduction is permitted which does not comply with these terms.
*Correspondence: Enea Gino Di Domenico, ZW5lYS5kaWRvbWVuaWNvQGlmby5nb3YuaXQ=
Disclaimer: All claims expressed in this article are solely those of the authors and do not necessarily represent those of their affiliated organizations, or those of the publisher, the editors and the reviewers. Any product that may be evaluated in this article or claim that may be made by its manufacturer is not guaranteed or endorsed by the publisher.
Research integrity at Frontiers
Learn more about the work of our research integrity team to safeguard the quality of each article we publish.