- 1Department of Periodontology and Operative Dentistry, Münster University Hospital, Münster, Germany
- 2Department of Periodontology, Johann Wolfgang Goethe-University Frankfurt, Frankfurt, Germany
- 3Department of Periodontology, TU Dresden, Dresden, Germany
- 4Section of Periodontology, Department of Conservative Dentistry, University Hospital Heidelberg, Heidelberg, Germany
- 5Unit of Periodontology, University Medicine Greifswald, Greifswald, Germany
- 6Department of Periodontology, University of Giessen, Giessen, Germany
- 7Department of Periodontology, Dental School, Faculty of Health, University of Witten/Herdecke, Witten, Germany
- 8Departments of Periodontology and Synoptic Dentistry, Charité University Medicine Berlin, Berlin, Germany
- 9Department of Periodontology, University Hospital Würzburg, Würzburg, Germany
The aim of this follow-up study was, to compare the effects of mechanical periodontal therapy with or without adjunctive amoxicillin and metronidazole on the subgingival microbiome of smokers with periodontitis using 16S rDNA amplicon next generation sequencing. Fifty-four periodontitis patients that smoke received either non-surgical periodontal therapy with adjunctive amoxicillin and metronidazole (n = 27) or with placebos (n = 27). Subgingival plaque samples were taken before and two months after therapy. Bacterial genomic DNA was isolated and the V4 hypervariable region of the bacterial 16S rRNA genes was amplified. Up to 96 libraries were normalized and pooled for Illumina MiSeq paired-end sequencing with almost fully overlapping 250 base pairs reads. Exact ribosomal sequence variants (RSVs) were inferred with DADA2. Microbial diversity and changes on the genus and RSV level were analyzed with non-parametric tests and a negative binomial regression model, respectively. Before therapy, the demographic, clinical, and microbial parameters were not significantly different between the placebo and antibiotic groups. Two months after the therapy, clinical parameters improved and there was a significantly increased dissimilarity of microbiomes between the two groups. In the antibiotic group, there was a significant reduction of genera classified as Porphyromonas, Tannerella, and Treponema, and 22 other genera also decreased significantly, while Selenomonas, Capnocytophaga, Actinomycetes, and five other genera significantly increased. In the placebo group, however, there was not a significant decrease in periodontal pathogens after therapy and only five other genera decreased, while Veillonella and nine other genera increased. We conclude that in periodontitis patients who smoke, microbial shifts occurred two months after periodontal therapy with either antibiotics or placebo, but genera including periodontal pathogens decreased significantly only with adjunctive antibiotics.
Introduction
Periodontitis is a highly prevalent, biofilm-associated disease and the main reason for tooth loss in the elderly population (Frencken et al., 2017). In a large multi-center study (ABPARO) a statistically significant benefit of amoxicillin (500 mg) and metronidazole (400 mg) as an adjunct to periodontal therapy was investigated and clinical results were published (Harks et al., 2015). We recently performed a microbial sub-group analysis of non-smoking periodontitis patients from the ABPARO study (Hagenfeld et al., 2018). We showed that the used antibiotics caused a microbiome shift characterized by the reduction of the amounts of periodontal pathogens and increase of commensal bacteria in the subgingival biofilm, 2 months after periodontal therapy. Cigarette use is a major risk factor for destructive forms of periodontal disease and profoundly influences the subgingival microbiome, which means that it is more diverse, pathogen rich, and commensal poor (Winkelhoff et al., 2001; Shchipkova et al., 2010; Bizzarro et al., 2013; Moon et al., 2015), even in shallow periodontal pockets ≤4 mm (Haffajee and Socransky, 2001). Re-colonization with commensals after periodontal therapy is compromised in smokers, because they maintain a pro-inflammatory host phenotype (Joshi et al., 2014).
Metronidazole interferes with the nucleic acid synthesis of bacteria. It has an antimicrobial spectrum against anaerobic bacteria including the periodontal pathogens Porphyromonas ginigvalis, Tannerella forsythia, and Treponema denticola (Loesche et al., 1984). Amoxicillin inhibits the synthesis of bacterial cell walls and has a synergistic effect together with metronidazole on the reduction of Aggregatibacter actinomycetemcomitans that is associated with aggressive forms of periodontitis (van Winkelhoff et al., 1989). Currently, there are only a few studies that have examined the microbial community effects of adjunctive amoxicillin and metronidazole in the periodontal treatment of smokers (Matarazzo et al., 2008; Faveri et al., 2014). Those studies, however, detected only a pre-defined and limited number of bacteria with the DNA-DNA checkerboard method. So-called “next-generation sequencing” allows for a hypothesis-free and unrestricted view on the microbial dynamics, because no pre-selection of bacteria is needed.
To date, there has been no next generation sequencing study that has investigated the microbial dynamics of smokers after therapy with or without antibiotics. Therefore, the aim of this study was to compare the effects of mechanical periodontal therapy with or without adjunctive amoxicillin and metronidazole on the subgingival microbiome of patients that smoke and have periodontitis using 16S rDNA amplicon next generation sequencing.
Materials and Methods
Patient Characteristics
Specimens from the ABPARO study—a multicenter randomized, double-blinded, parallel group, placebo-controlled study on the effects of adjunctive antibiotics during periodontal treatment—were used (ISRCTN: 64254080, Clinical Trials.gov NCT00707369) (Harks et al., 2015). Smokers were defined as persons self-declared as smokers and having a concentration of carbon-monoxide (CO) ≥ 7 ppm by using a Smokerlyzer (Bedfont-Smokerlyzer®, Bedfont, UK) (Harks et al., 2015). All smokers received the same mechanical periodontal treatment. In total, 71 of the 345 per-protocol patients were smokers. Of those, nine were diagnosed with severe (Stage III) and 62 with moderate periodontitis (Stage II) (Tonetti et al., 2018). Seven severely and 27 moderately diseased smokers received an adjunctive placebo, and two severely and 35 moderately diseased smokers received adjunctive amoxicillin and metronidazole (500 mg/400 mg thrice daily for 7 days). Because of the small and unbalanced treatment groups of severely diseased smokers, only the data from the smokers with moderate periodontitis were included in this study and reported here. To ensure the best clinically comparable groups at baseline, the 27 smokers with moderate periodontitis from the placebo group were matched to 27 of the 35 moderately diseased smokers from the antibiotic group (Ho et al., 2011). Samples of those 54 smokers were analyzed for the examination time-points before and 2 months after therapy. Subgingival specimens were taken from four teeth with periodontitis and a pocket probing depth of ≥6 mm, one in each quadrant, as described previously (Hagenfeld et al., 2018, 2019). One sterile paper point (ISO45, Roeko Dental, Langenau, Germany) was inserted for 10 s in each site and all paper points were removed and pooled in one sterile collection tube. The sampling sites remained the same for both sampling timepoints. This study was approved by the Medical Ethics Committee of the University of Muenster (ref: 2016-505-f-S).
Library Preparation
Bacterial genomic DNA from the samples of smokers and non-smokers was isolated and purified using the QiaAmp DNA- Mini Kit (Qiagen, Hilden, Germany). The integrity and purity of the DNA was checked by agarose gel electrophoresis and a Nanodrop (Thermo Scientific, Silverside, USA) measurement, respectively. DNA quantification was performed with a Qubit 2.0 fluorometer together with the Qubit dsDNA BR Assay Kit (Thermo Fisher, Waltham, MA, USA). Two PCRs were done with KAPA HiFi Hot Start DNA Polymerase (Ready Mix, KAPA-Biosystems, Boston, MA, USA). In the first PCR, universal eubacterial tailed tag dual index amplification primers with an amplicon size of 291 bp (E. coli-position 515 to 806) were utilized for amplifying the V4 hypervariable regions of the bacterial 16S rRNA gene (Klindworth et al., 2013): EMP515f TCGTCGGCAGCGTCAGATGTGTATAAGAGACAGGTGYCAGCMGCCGCGGTAA; 806_R GTCTCGTGGGCTCGGAGATGTGTATAAGAGACAGGACTACHVGGGTATCTAATCC. The 16S rDNA specific regions were marked as bold. In the second PCR, sample-specific “barcode”-primers and adapter sequences were attached using the Nextera XT Index Kit Version 2 (Illumina, San Diego, CA, USA) with 96 barcode combinations. Up to 96 libraries were normalized and pooled for an Illumina MiSeq sequencing run with 250 base-pair paired-end reads using MiSeq Reagent Kit Version 2 (Illumina). Five negative control samples contained molecular biology grade water which allowed for the identification of potential external contamination in each sequencing run. Additionally, one evenly balanced mock sample supplemented each run with 23 known species with 24 RSVs to allow for inter-run quality control. The composition of the mock sample can be found in Supplementary File 1. The DNA extraction steps for the mock sample were performed as described earlier with one modification: the DNA isolation for the mock species was performed with Qiagen Genomic Tips 20/G (Qiagen, Hilden, Germany). All 23 single-species samples were adjusted to 107 copies of the 16S rRNA operon per organism per microliter. Afterwards, an even mock sample was created by combining equal amounts of these dilutions with a total copy number of 107 16S rRNA operons per microliter resulting in 4.3 × 105 16S rRNA operons per species per microliter.
Sequence Generation, Primer, and Adapter Removal
lllumina's MiSeq Control Software v.2.6.2.1 was used to operate the MiSeq machine. The Real-time Analysis Software v.1.18.54 performed image analysis, base calling, and quality score assignment to each base for each cycle. The MiSeq Reporter Software v.2.6.3 was used for demultiplexing, FastQ file generation, and adapter removal. Sequencing primers were removed with Cutadapt v.1.8.1 (Martin, 2011) using the default setting, that is, a maximum error rate in the adapter region of 10%. Those trimmed reads were submitted to the European Nucleotide Archive (http://www.ebi.ac.uk/ena/) of EMBL European Bioinformatics Institute under the study accession number PRJEB35812.
Denoising and Merging
The adapter and primer-free FastQ files were further processed using R v.3.6.1 and RStudio v.1.1.463 (R Development Core Team, 2017) with DADA2 v.1.12.1 (Callahan et al., 2016). If not mentioned explicitly below, the default settings (most noticeably, a zero-mismatch allowed in the overlap region while merging the forward and reverse reads) were used. First, the forward and reverse reads were trimmed one base at the 3′-end to remove the terminal base without the quality score that was attached during sequencing. Second, the reads were trimmed at the 5′-end to allow for merging with minimal unpaired bases at both ends calculated for E. coli (forward reads were trimmed 22 bases, reverse reads 20 bases). Third, a maximum of two expected errors per read was allowed to filter out low-quality reads. Finally, the minimal abundance was set to 10 reads per run for forming the ribosomal sequence variant (RSV) partitions during denoising.
Taxonomic Labeling and Decontamination
Taxonomic labeling of RSVs was done with a naïve Bayesian classifier and a SILVA v.128 training set (Pruesse et al., 2007). Each run was stored using phyloseq v.1.19.1 (McMurdie and Holmes, 2013) into a single file containing taxonomic labels and reads for each RSV. The QC-checked sequencing runs were individually screened for potential external contaminants, using the decontam package v.1.4.0 (Davis et al., 2018). The combined prevalence and frequency methods were used with the default threshold of 0.1 for identifying contaminants. Afterwards, all single-run phyloseq files were merged and combined with clinical and demographic variables into the final phyloseq file. Finally, prevalence filtering over all the runs was done of RSVs occurring in only two or less samples to remove spurious ones.
Run and Sample Quality Control
Run quality control was performed after each sequencing run in two consecutive steps. First, the run-Q30 scores were checked with the MiSeq Reporter software. Here, the values had to be above 75% as recommended by the manufacturer (Illumina, 2012). Second, the mock sample was denoised using the DADA2 pipeline and checked with phyloseq. A run was judged of sufficient quality when 24 found RSVs completely matched the reference 16S RNA genes of each 23 species from the mock sample and ≤ 0.5% reads per sample were found without matching references. Sample quality control was first performed for all samples after each run and again a second time after the final phyloseq file with all included runs was created. Samples had insufficient quality when the rarefaction quality control did not show saturation or the samples had ≤5,000 reads.
Statistical Analysis
For the community level analysis, phyloseq was used to analyze the bacterial diversity. For alpha diversity, the richness, that is, the randomly sub-sampled number of observed reads per RSVs in each sample, was determined. In addition, the Shannon index for diversity was provided. For beta diversity, a Bray-Curtis distance matrix was created, and the mean dissimilarity for each patient was calculated. Fisher's exact test was used for the intergroup differences of the categorical variables. For all other continuous variables, the two-sided Mann–Whitney U-test for intergroup differences was used. For the changes of the continuous variables in both groups before and after the treatment, the Wilcoxon signed-rank test was applied.
The differences in the mean number of randomly subsampled RSV reads per genus between the antibiotic and placebo before and after therapy were displayed in a heatmap. For the statistical analysis of the differential abundance of single RSVs classified on genus level, the R package DESeq2 v.1.18.1 (Love et al., 2014) was used. The model formula contained the treatment-group (AB, placebo), the timepoint (before, after), and the interaction term between both groups as fixed effects. The RSVs grouped per genus that changed significantly in the antibiotic and placebo groups were displayed in a bubble chart. If one genus included species associated with periodontal disease or health by the Socransky group (Socransky et al., 1998), this genus was allocated to the given complex as described earlier (Hagenfeld et al., 2018).
Results
Demographic, Clinical Parameters Before and After Therapy and Preprocessing the Sequencing Output
Before therapy, there were no statistically significant differences between the placebo and antibiotic groups in all the demographic and clinical parameters examined (Table 1). After therapy, clinical parameters improved significantly in both groups (Supplementary Table 1, Supplementary File 2). All sequencing runs passed the run quality control as described previously (Supplementary Table 3). A total of five RSVs were found to be suspicious for external contaminants and removed. In addition, 645 RSVs had a prevalence in ≤2 samples and were also removed, together with two RSVs not classified as from the bacterial kingdom. All samples showed a saturation during rarefaction and had more than 5,000 reads per sample (data not shown).
Microbial Diversity Parameters Before and After Therapy
Before therapy, there were no statistically significant differences between the placebo and antibiotic groups in any of the microbial diversity parameters tested: mean richness (placebo 161.81 ± 53.27 sd vs. antibiotic 169.93 ± 57.32 sd, p = 0.595), mean diversity (placebo 3.84 ± 0.39 vs. antibiotic 3.86 ± 0.41, p = 0.797), and mean dissimilarity (placebo 3.84 ± 0.39 vs. antibiotic 3.86 ± 0.41, p = 0.757). After therapy, the dissimilarity increased significantly in both the placebo (p = 0.034) and the antibiotic group (p < 0.001). Richness (p = 0.768) and diversity (p = 0.090) were not significantly altered in the placebo group. In the antibiotic group, however, richness (p = 0.002) and diversity (p = 0.016) significantly decreased. A detailed table of diversity parameters can be found in Supplementary Table 2.
Genus Level Abundances, Before, and After Therapy
We found in total 1060 RSVs that can be assigned to 143 different genera (Supplementary File 3). Before therapy, the relative number of sub-sampled reads summarized by per genus was highly similar between the placebo and the antibiotic groups (Figure 1).
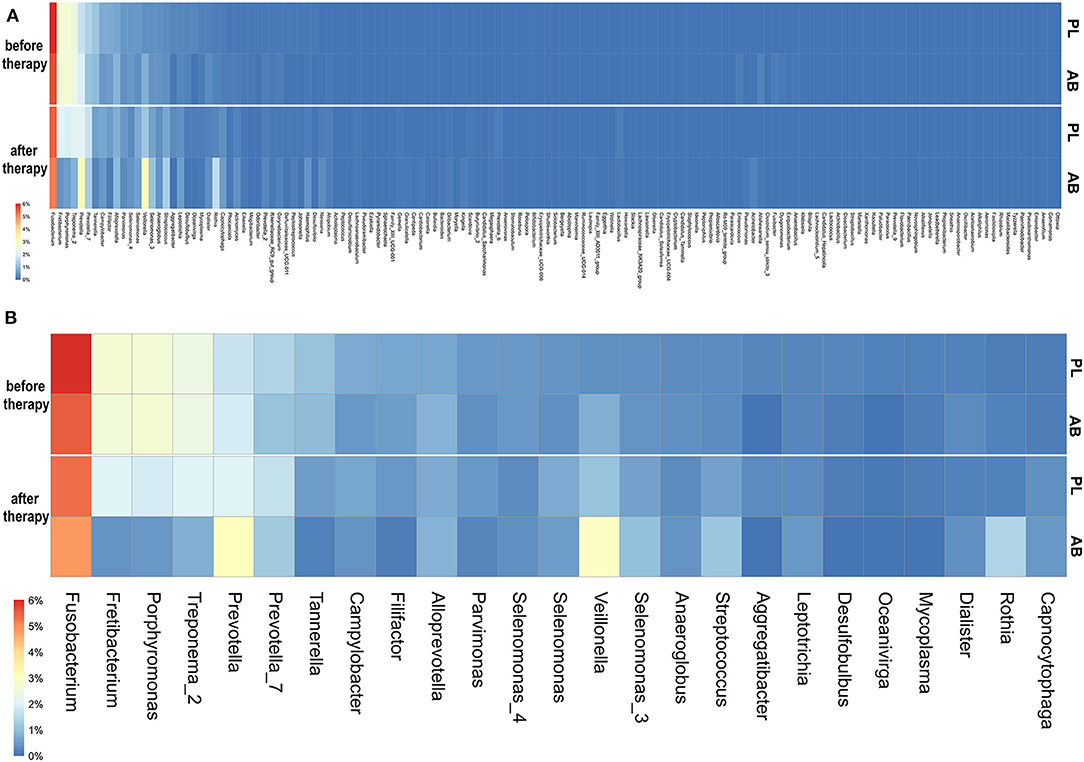
Figure 1. (A) Heatmap showing the randomly sub-sampled reads summarized per genus in the placebo and antibiotic groups before and after therapy. The color codes ranged from 6% to 0% of the summarized reads for each genus. Higher relative numbers of genus reads are colored red, lower numbers are yellow to white, and dark-blue represents zero values. PL, placebo group; AB, antibiotic group. (B) Heatmap showing randomly sub-sampled reads of the 25 most abundant genera in the placebo and antibiotic groups before and after therapy. Here, only the 25 most abundant genera before therapy are shown. The color codes ranged from 6 to 0% of the summarized reads for each genus. Higher relative numbers of genus reads are colored red, lower numbers are yellow to white, and dark-blue represents zero values. PL, placebo group; AB, antibiotic group.
Before therapy, no genus was more dissimilar than 1.0 percent between antibiotic and placebo. Those RSVs with the highest differences before therapy were Fusobacterium, Veillonella, and Prevotella, all below a 0.5 percent difference. The three genera with the highest relative abundance before therapy were: Fusobacterium (placebo: 6.0% vs. antibiotic: 5.5%), Veillonella (placebo: 0.4% vs. antibiotic: 0.8%), and Prevotella (placebo: 1.4% vs. antibiotic: 1.1%).
After therapy, the microbiomes became more dissimilar between the placebo and antibiotic groups. The genus with the highest difference after therapy was Veillonella, which increased after therapy in both groups and had a relative abundance of 1.1% in the placebo group, compared to 1.9% in the antibiotic group. Fretibacterium and Porphyromonas decreased less in the placebo group; they had a relative abundance of 2.0 and 1.9% in the placebo vs. 0.4 and 0.5% in the antibiotic group, respectively. In the placebo group, no genus changed more strongly than one percent of relative abundance. In the antibiotic group, six genera changed more than one percent. Of those, three genera (Fretibacterium, Porphyromonas, and Treponema) reduced and three genera increased more than 1.0% (Veillonella, Rothia, and Prevotella). In the placebo group, also mainly periodontal pathogens were reduced and commensals increased after therapy. However, those changes were in general roughly 0.5–1.5% smaller than in the antibiotic group.
RSVs Grouped at Genus Level That Significantly Changed After Therapy
The vast majority (98.8%) of all RSVs were not statistically different in abundance between the antibiotic and placebo group before therapy. Those 21 RSVs that were found different in abundance before therapy belonged to exclusively low-abundant RSVs with a mean baseline abundance below 1% of each sample (Supplementary File 4). The abundances of RSVs from genera including periodontal pathogens like Porphyromonas, Tannerella, and Treponema decreased significantly only in the antibiotic group, together with several other species (Figure 2). RSVs belonging to genera from the commensal bacteria Selenomonas, Capnocytophaga, and Actinomyces significantly increased after therapy only in the antibiotic group. Two RSVs belonging to the genus Veillonella increased only in the placebo group, together with several other RSVs not belonging to formerly identified commensal species.
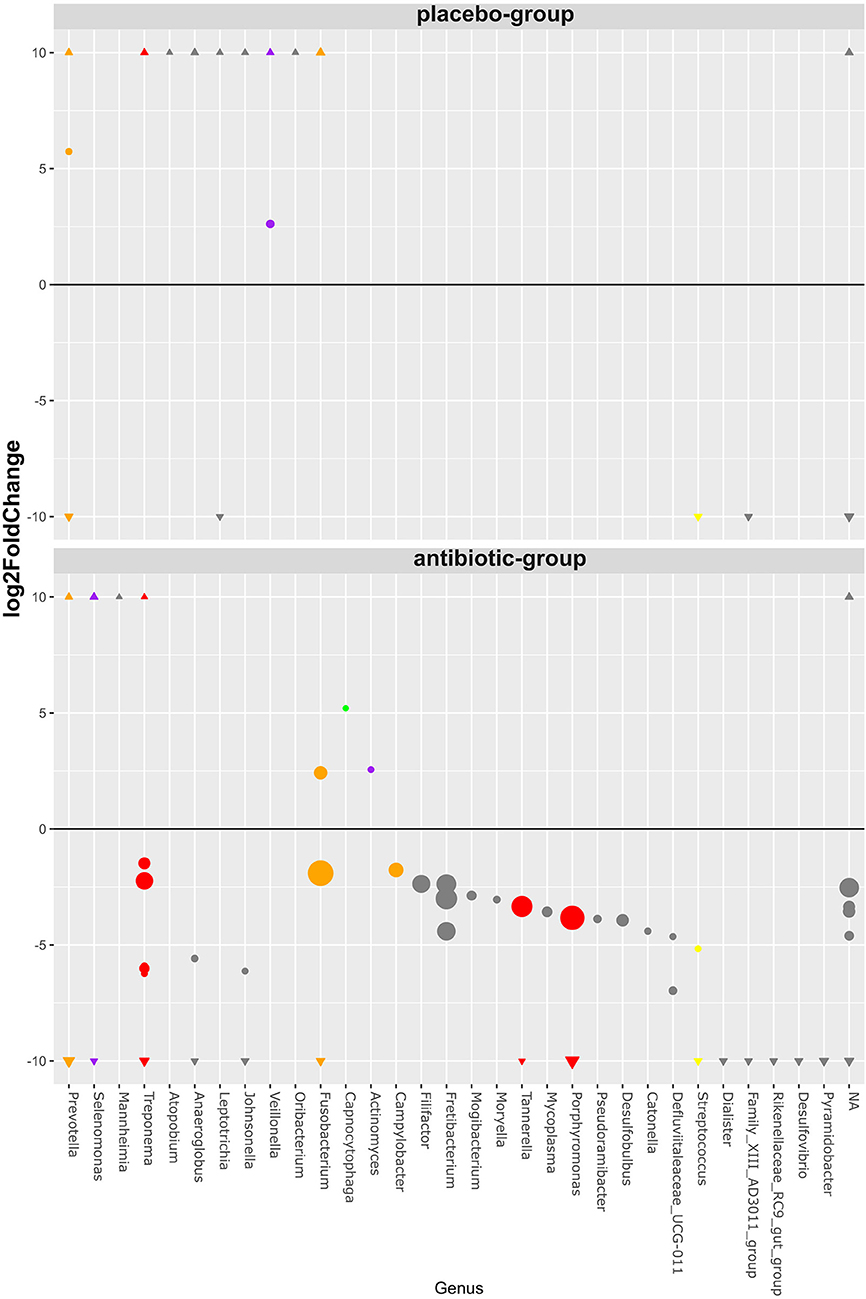
Figure 2. Bubble chart of significant RSV abundance changes after periodontal therapy classified on the genus level. The bubbles represent 98 RSVs, belonging to 31 uniquely named genera (x-axis), that showed statistically significant changes (y-axis) after therapy on a log2 scale. All RSVs unclassified on the genus level are grouped together (NA). The sizes of the bubbles represent the mean relative RSV abundance over all samples before therapy. Those RSVs with ≥10 log2fold and ≤ −10 log2fold changes were marked as triangles on their respective y-axis section. All RSVs belonging to a genus that includes species previously described by Socransky are colored according to their complex affiliation.
Discussion
By using our hypothesis-free next-generation-sequencing approach, we have provided the first study that examined in-depth microbial changes in smokers after periodontal therapy with or without antibiotics. We found a short-term microbiome shift in the form of a significantly increased beta-diversity after therapy in both the placebo and antibiotic group. Those microbial shifts differed markedly between the placebo and antibiotic group. In the antibiotic group, the microbial shift was characterized by a strong reduction of alpha-diversity in general, and especially, periodontal pathogens decreased significantly while commensals increased. In the placebo group, however, there was not a significant decrease in alpha-diversity and also not a significant reduction of periodontal pathogens 2 months after therapy.
Compared to our previous study with non-smokers (Hagenfeld et al., 2018), the composition of highly abundant genera at baseline had a strong resemblance with the smokers in this study. In both studies, Fusobacterium, Fretibacterium, and Porphyromonas had the highest baseline abundance. Regarding RSV changes after therapy, we also had very similar results on the genus level compared to our study conducted with non-smokers; RSVs belonging to genera containing periodontal pathogens like Treponema, Porphyromonas, Tannerella, and Fusobacterium decreased as in our previous study with non-smokers. Additionally, in both studies, Fretibacterium and Fillifactor decreased among several other RSVs classified as genera not regarded as periodontal pathogens by the fundamental study of Socransky et al. (1998). Also, in both studies, RSVs that were classified as genera containing commensal species (Selenomonas, Capnocytophaga, and Actinomyces) increased in the antibiotic group. In the placebo group of non-smokers, however, we did not find dissimilarity changes, and secondly, only three RSVs changed significantly in the placebo group of our previous study with non-smokers.
One previous study (Matarazzo et al., 2008) that used the DNA-DNA checkerboard method is very comparable to our study, because they made a direct comparison of two groups of periodontitis patients who smoked that received either periodontal therapy with two different kinds of antibiotics or periodontal therapy without antibiotics. In that study, patients from the amoxicillin and metronidazole group underwent similar microbial shifts as in our study. Here, also periodontal pathogenic species with the genera Porphyromonas, Tannerella, and Treponema were significantly reduced only in the antibiotic group, accompanied by an increase of the species from commensals, that are, Actinomyces, Selenomonas, and Capnocytophaga.
Another study using the DNA-DNA checkerboard method compared the use of amoxicillin and metronidazole between smokers and non-smokers. Here, the smokers treated with adjunctive antibiotics also had a significant reduction of those three genera containing periodontal pathogens (Faveri et al., 2014).
Other groups that examined periodontal therapy without antibiotics in smokers and non-smokers found a lower reduction of periodontal pathogens in smokers compared to non-smokers by using the DNA-DNA checkerboard (Darby et al., 2005; Feres et al., 2015). These findings were confirmed by our results from the smokers in the placebo group where the relative numbers of high-abundant bacteria in general were slightly reduced, but no RSVs from periodontal pathogens were significantly changed after therapy.
In contrast to those previous hypothesis-driven studies, the majority of genera (55%) that were reduced significantly in our study with patients that smoked and received adjunctive antibiotics were not regarded as periodontal pathogens (Socransky et al., 1998). For example, uncultivated Fretibacterium sp. human oral taxon no. 360 was recently found as a novel biomarker for periodontitis in a Japanese population (Khemwong et al., 2019). RSVs from the genus Fretibacterium were also highly abundant in our population of German smokers and decreased significantly after periodontal therapy with antibiotics. Another example is Filifactor alocis that is regarded as a new emerging periodontal pathogen (Aruni et al., 2014), or Mogibacterium as a new genus isolated from periodontal pockets of adult human patients with periodontal disease and infected root canals (Nakazawa et al., 2000). Both of those genera were reduced significantly in our study using antibiotics. These findings point out the importance of those and other previously unidentified species in the treatment of periodontal disease, as recently reviewed (Perez-Chaparro et al., 2014).
Our study has also some limitations. A higher taxonomic resolution down to the species level was not possible with our 250-bp read length. We could not access functional capacities of the microbiomes as would be possible with a meta-genomic approach. In addition, the negative binomial regression model used for the RSV-level changes cannot account for random patient effects. By using a pooled microbial sample, we cannot asses site specific differences. We only included moderately diseased periodontitis patients. A longer follow-up would also be needed to assess the stability of changes and patterns of re-colonization after therapy.
Conclusions
With our hypothesis-free 16S rDNA amplicon next generation sequencing study with periodontitis patients that smoke, we found RSVs from genera including periodontal pathogens exclusively decreased with adjunctive amoxicillin and metronidazole. Additionally, we expanded the spectrum of bacteria that changed in short-term after periodontal therapy in smokers beyond the well-studied periodontal pathogens and commensals.
Data Availability Statement
The datasets generated for this study can be found in the European Nucleotide Archive (http://www.ebi.ac.uk/ena/) of EMBL European Bioinformatics Institute PRJEB35812.
Ethics Statement
The studies involving human participants were reviewed and approved by Medical Ethics Committee of the University of Muenster (ref: 2016-505-f-S). The patients/participants provided their written informed consent to participate in this study.
Author Contributions
DHag and JMa contributed to formal analysis, validation, software, and methodology. DHag and KP contributed to investigation and data curation. BE and DHar contributed to project administration, conceptualization, and supervision. BE, IH, and DHar contributed to funding acquisition. PE, KL, T-SK, TK, JMe, DK, US, and BE contributed to resources. DHag contributed to Writing - original preparation. JMa, JMe, KP, IH, PE, KL, T-SK, TK, DK, US, BE, and DHar contributed to Writing - Review and Editing.
Conflict of Interest
The authors declare that the research was conducted in the absence of any commercial or financial relationships that could be construed as a potential conflict of interest.
Acknowledgments
The authors would like to thank Anja Schultes, Margard Karpisch-Tölke, and Beate Walters for excellent technical assistance. The authors are greatly indebted to collaborators and staff members representing the ABPARO Group for their successful work on this project.
Supplementary Material
The Supplementary Material for this article can be found online at: https://www.frontiersin.org/articles/10.3389/fcimb.2020.00167/full#supplementary-material
References
Aruni, W., Chioma, O., and Fletcher, H. M. (2014). Filifactor alocis: the newly discovered kid on the block with special talents. J. Dent. Res. 93, 725–732. doi: 10.1177/0022034514538283
Bizzarro, S., Loos, B. G., Laine, M. L., Crielaard, W., and Zaura, E. (2013). Subgingival microbiome in smokers and non-smokers in periodontitis: an exploratory study using traditional targeted techniques and a next-generation sequencing. J. Clin. Periodontol. 40, 483–492. doi: 10.1111/jcpe.12087
Callahan, B. J., McMurdie, P. J., Rosen, M. J., Han, A. W., Johnson, A. J. A., and Holmes, S. P. (2016). DADA2: high-resolution sample inference from Illumina amplicon data. Nat. Methods 13, 581–583. doi: 10.1038/nmeth.3869
Darby, I. B., Hodge, P. J., Riggio, M. P., and Kinane, D. F. (2005). Clinical and microbiological effect of scaling and root planing in smoker and non-smoker chronic and aggressive periodontitis patients. J. Clin. Periodontol. 32, 200–206. doi: 10.1111/j.1600-051X.2005.00644.x
Davis, N. M., Proctor, D. M., Holmes, S. P., Relman, D. A., and Callahan, B. J. (2018). Simple statistical identification and removal of contaminant sequences in marker-gene and metagenomics data. Microbiome 6:226. doi: 10.1186/s40168-018-0605-2
Faveri, M., Rebello, A., de Oliveira Dias, R., Borges-Junior, I., Duarte, P. M., Figueiredo, L. C., et al. (2014). Clinical and microbiologic effects of adjunctive metronidazole plus amoxicillin in the treatment of generalized chronic periodontitis: smokers versus non-smokers. J. Periodontol. 85, 581–591. doi: 10.1902/jop.2013.130278
Feres, M., Bernal, M., Matarazzo, F., Faveri, M., Duarte, P. M., and Figueiredo, L. C. (2015). Subgingival bacterial recolonization after scaling and root planing in smokers with chronic periodontitis. Aust. Dent. J. 60, 225–232. doi: 10.1111/adj.12225
Frencken, J. E., Sharma, P., Stenhouse, L., Green, D., Laverty, D., and Dietrich, T. (2017). Global epidemiology of dental caries and severe periodontitis - a comprehensive review. J. Clin. Periodontol. 44, S94–S105. doi: 10.1111/jcpe.12677
Haffajee, A. D., and Socransky, S. S. (2001). Relationship of cigarette smoking to the subgingival microbiota. J. Clin. Periodontol. 28, 377–388. doi: 10.1034/j.1600-051x.2001.028005377.x
Hagenfeld, D., Koch, R., Jünemann, S., Prior, K., Harks, I., Eickholz, P., et al. (2018). Do we treat our patients or rather periodontal microbes with adjunctive antibiotics in periodontal therapy? A 16S rDNA microbial community analysis. PLoS ONE 13:e0195534. doi: 10.1371/journal.pone.0195534
Hagenfeld, D., Prior, K., Harks, I., Jockel-Schneider, Y., May, T. W., Harmsen, D., et al. (2019). No differences in microbiome changes between anti-adhesive and antibacterial ingredients in toothpastes during periodontal therapy. J. Periodontal Res. 54, 435–443. doi: 10.1111/jre.12645
Harks, I., Koch, R., Eickholz, P., Hoffmann, T., Kim, T.-S., Kocher, T., et al. (2015). Is progression of periodontitis relevantly influenced by systemic antibiotics? A clinical randomized trial. J. Clin. Periodontol. 42, 832–842. doi: 10.1111/jcpe.12441
Ho, D. E., Imai, K., King, G., and Stuart, E. A. (2011). MatchIt: nonparametric preprocessing for parametric causal inference. J. Stat. Softw. 42, 1–28. doi: 10.18637/jss.v042.i08
Illumina (2012). Understanding Illumina Quality Scores. Available online at: https://emea.illumina.com/content/dam/illumina-marketing/documents/products/technotes/technote_understanding_quality_scores.pdf (accessed April 13, 2020).
Joshi, V., Matthews, C., Aspiras, M., de Jager, M., Ward, M., and Kumar, P. (2014). Smoking decreases structural and functional resilience in the subgingival ecosystem. J. Clin. Periodontol. 41, 1037–1047. doi: 10.1111/jcpe.12300
Khemwong, T., Kobayashi, H., Ikeda, Y., Matsuura, T., Sudo, T., Kano, C., et al. (2019). Fretibacterium sp. human oral taxon 360 is a novel biomarker for periodontitis screening in the Japanese population. PLoS ONE 14:e0218266. doi: 10.1371/journal.pone.0218266
Klindworth, A., Pruesse, E., Schweer, T., Peplies, J., Quast, C., Horn, M., et al. (2013). Evaluation of general 16S ribosomal RNA gene PCR primers for classical and next-generation sequencing-based diversity studies. Nucleic Acids Res. 41:e1. doi: 10.1093/nar/gks808
Loesche, W. J., Syed, S. A., Morrison, E. C., Kerry, G. A., Higgins, T., and Stoll, J. (1984). Metronidazole in periodontitis: I. clinical and bacteriological results after 15 to 30 Weeks. J. Periodontol. 55, 325–335. doi: 10.1902/jop.1984.55.6.325
Love, M. I., Huber, W., and Anders, S. (2014). Moderated estimation of fold change and dispersion for RNA-seq data with DESeq2. Genome Biol. 15:550. doi: 10.1186/s13059-014-0550-8
Martin, M. (2011). Cutadapt removes adapter sequences from high-throughput sequencing reads. EMBnet. J. 17:10. doi: 10.14806/ej.17.1.200
Matarazzo, F., Figueiredo, L. C., Cruz, S. E. B., Faveri, M., and Feres, M. (2008). Clinical and microbiological benefits of systemic metronidazole and amoxicillin in the treatment of smokers with chronic periodontitis: a randomized placebo-controlled study. J. Clin. Periodontol. 35, 885–896. doi: 10.1111/j.1600-051X.2008.01304.x
McMurdie, P. J., and Holmes, S. (2013). phyloseq: an R package for reproducible interactive analysis and graphics of microbiome census data. PLoS ONE 8:e61217. doi: 10.1371/journal.pone.0061217
Moon, J.-H., Lee, J.-H., and Lee, J.-Y. (2015). Subgingival microbiome in smokers and non-smokers in Korean chronic periodontitis patients. Mol. Oral Microbiol. 30, 227–241. doi: 10.1111/omi.12086
Nakazawa, F., Sato, M., Poco, S. E., Hashimura, T., Ikeda, T., Kalfas, S., et al. (2000). Description of Mogibacterium pumilum gen. nov., sp. nov. and Mogibacterium vescum gen. nov., sp. nov., and reclassification of Eubacterium timidum (Holdeman et al. 1980) as Mogibacterium timidum gen. nov., comb. nov. Int. J. Syst. Evol. Microbiol. 50, 679–688. doi: 10.1099/00207713-50-2-679
Perez-Chaparro, P. J., Goncalves, C., Figueiredo, L. C., Faveri, M., Lobao, E., Tamashiro, N., et al. (2014). Newly identified pathogens associated with periodontitis: a systematic review. J. Dent. Res. 93, 846–858. doi: 10.1177/0022034514542468
Pruesse, E., Quast, C., Knittel, K., Fuchs, B. M., Ludwig, W., Peplies, J., et al. (2007). SILVA: a comprehensive online resource for quality checked and aligned ribosomal RNA sequence data compatible with ARB. Nucleic Acids Res. 35, 7188–7196. doi: 10.1093/nar/gkm864
R Development Core Team (2017). R: A Language and Environment for Statistical Computing. Available online at: https://www.r-project.org (accessed September 7, 2015).
Shchipkova, A. Y., Nagaraja, H. N., and Kumar, P. S. (2010). Subgingival microbial profiles of smokers with periodontitis. J. Dent. Res. 89, 1247–1253. doi: 10.1177/0022034510377203
Socransky, S. S., Haffajee, A. D., Cugini, M. A., Smith, C., and Kent, R. L. (1998). Microbial complexes in subgingival plaque. J. Clin. Periodontol. 25, 134–144. doi: 10.1111/j.1600-051X.1998.tb02419.x
Tonetti, M. S., Greenwell, H., and Kornman, K. S. (2018). Staging and grading of periodontitis: framework and proposal of a new classification and case definition. J. Clin. Periodontol. 45, S149–S161. doi: 10.1111/jcpe.12945
van Winkelhoff, A. J., Rodenburg, J. P., Goené, R. J., Abbas, F., Winkel, E. G., and de Graaff, J. (1989). Metronidazole plus amoxycillin in the treatment of Actinobacillus actinomycetemcomitans associated periodontitis. J. Clin. Periodontol. 16, 128–131. doi: 10.1111/j.1600-051X.1989.tb01626.x
Keywords: microbiota, smoking, periodontal therapy, systemic antibiotics, 16S rDNA amplicon sequencing
Citation: Hagenfeld D, Matern J, Prior K, Harks I, Eickholz P, Lorenz K, Kim T-S, Kocher T, Meyle J, Kaner D, Schlagenhauf U, Harmsen D and Ehmke B (2020) Significant Short-Term Shifts in the Microbiomes of Smokers With Periodontitis After Periodontal Therapy With Amoxicillin & Metronidazole as Revealed by 16S rDNA Amplicon Next Generation Sequencing. Front. Cell. Infect. Microbiol. 10:167. doi: 10.3389/fcimb.2020.00167
Received: 16 January 2020; Accepted: 30 March 2020;
Published: 05 May 2020.
Edited by:
Georgios N. Belibasakis, Karolinska Institutet, SwedenReviewed by:
Marcello Riggio, University of Glasgow, United KingdomJanina P. Lewis, Virginia Commonwealth University, United States
Copyright © 2020 Hagenfeld, Matern, Prior, Harks, Eickholz, Lorenz, Kim, Kocher, Meyle, Kaner, Schlagenhauf, Harmsen and Ehmke. This is an open-access article distributed under the terms of the Creative Commons Attribution License (CC BY). The use, distribution or reproduction in other forums is permitted, provided the original author(s) and the copyright owner(s) are credited and that the original publication in this journal is cited, in accordance with accepted academic practice. No use, distribution or reproduction is permitted which does not comply with these terms.
*Correspondence: Daniel Hagenfeld, daniel.hagenfeld@ukmuenster.de
†These authors have contributed equally to this work