- Department of Immunology, Faculty of Medicine, Kindai University, Osaka, Japan
The interaction between human immunodeficiency virus (HIV) and hematopoietic stem/progenitor cells (HSPCs) has been of great interest. However, it remains unclear whether HSPCs can act as viral reservoirs. Many studies have reported the presence of latently infected HSPCs in the bone marrow of HIV-infected patients, whereas many other investigators have reported negative results. Hence, further evidence is required to elucidate this controversy. The other arm of HSPC investigations of HIV infection involves dynamics analysis in the early and late stages of infection to understand the impact on the pathogenesis of acquired immunodeficiency syndrome. Several recent studies have suggested reduced amounts and/or functional impairment of multipotent, myeloid, and lymphoid progenitors in HIV infection that may contribute to hematological manifestations, including anemia, pancytopenia, and T-cell depletion. In addition, ongoing and future studies on the senescence of HSPCs are expected to further the understanding of HIV pathogenesis. This mini review summarizes reports describing the basic aspects of hematopoiesis in response to HIV infection and offers insights into the association of HIV infection/exposure of the host HSPCs and hematopoietic potential.
Introduction
Human immunodeficiency virus (HIV) infection causes acquired immunodeficiency syndrome (AIDS). The depletion of memory CD4+ T cells preceding the manifestation of AIDS may be mainly due to HIV infection of these cells. However, HIV may also cause reduced production of naïve T cells by infection of CD4+ thymocytes. Although the dynamics of hematopoietic stem/progenitor cells (HSPCs) in response to HIV infection remains unclear, it is well-established that HIV infection is associated with hematological changes, such as anemia and pancytopenia (Parinitha and Kulkarni, 2012; Durandt et al., 2019). Therefore, it is imperative to better elucidate the contribution of altered hematopoietic potential to the disease. The aim of this mini review was to discuss on factors affecting the physiology and pathology of HSPCs by reviewing past publications describing the interactions between HIV and hematopoietic progenitor cells (HPCs) in the bone marrow (BM) and thymus for better understanding the role of hematopoiesis in the pathogenesis.
HSPCs in the BM
Adult hematopoietic differentiation occurs in the BM. Hematopoietic stem cells (HSCs) have long-term self-renewing capacity and can differentiate to any type of blood cell (Rieger and Schroeder, 2012). Although HSC niches have not been fully defined (Morrison and Scadden, 2014), a recent study indicated that HSCs reside in a perivascular niche and are supported by various cytokines secreted by endothelial and stromal cells (Ding et al., 2012). BM HSPCs consist of progenitors for all blood cell lineages including those described in Figure 1 (Rieger and Schroeder, 2012). Proteomic and transcriptomic analyses have reported many potential factors that may work in concert in hematopoiesis, although the significance of individual genes must be further clarified (Liu et al., 2006; Kim et al., 2009; Starnes et al., 2010). Recent evidence indicates that inflammatory signals, such as prostaglandin E2, nitric oxide, granulocyte colony-stimulating factor, interferons, tumor necrosis factor, and Toll-like receptor 4, may be involved in the emergence of HSPCs (He et al., 2015; Luis et al., 2016).
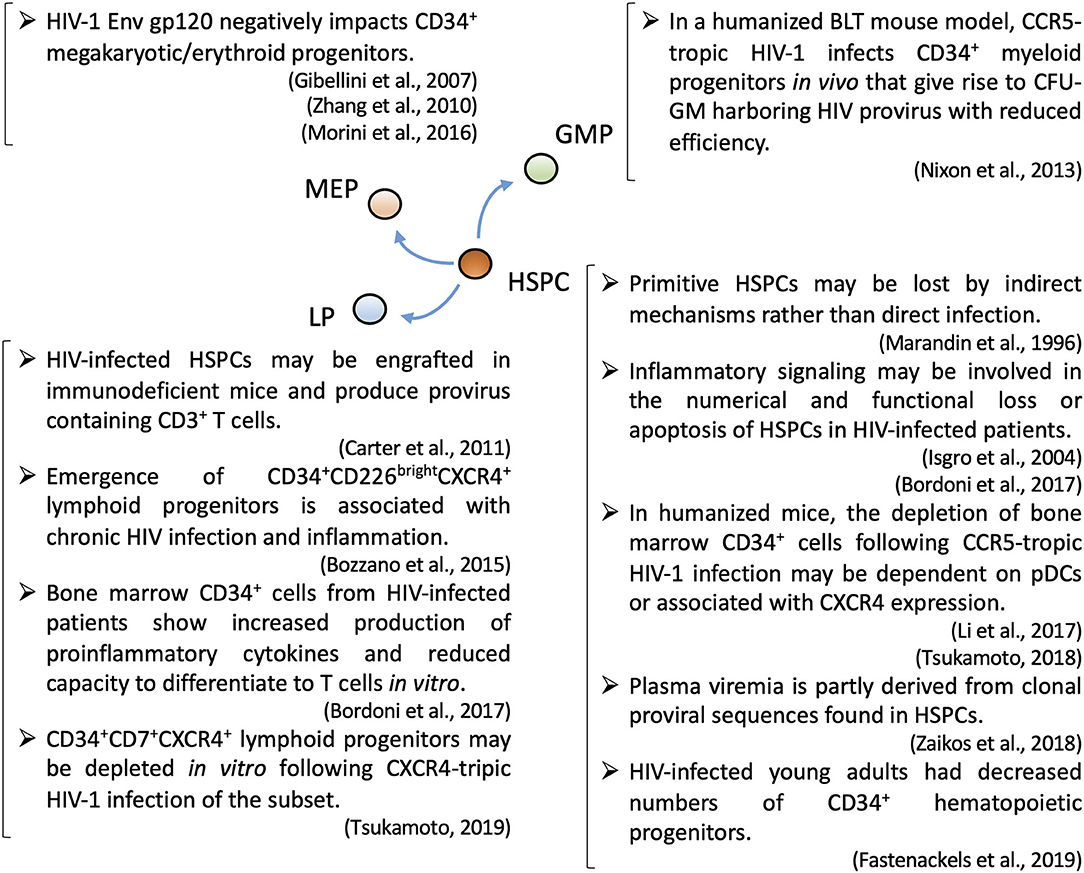
Figure 1. A summary of recent topics regarding HIV-1 pathogenesis associated with subsets of hematopoietic stem/progenitor cells. CFU-GM, granulocyte-macrophage colony-forming unit; GMP, granulocyte-macrophage progenitor; HSPC, hematopoietic stem progenitor cell; LP, lymphoid progenitor; MEP, megakaryocyte–erythrocyte progenitor; pDC, plasmacytoid dendritic cell.
The Roles of HSPCs in T-Lineage Differentiation
T-lineage differentiation in the thymus is dependent on a supply of CD34+ progenitors from the BM (Kondo et al., 1997). Early lymphoid progenitors (LPs) are thought to reside in distinct niches from those of HSCs (Ding and Morrison, 2013). CD34+CD38dim, but not CD34+CD38+, cells can migrate to the thymus and commit to the T cell lineage (Res et al., 1996). Transcriptional regulation of the lymphoid commitment of HSPCs is complex (Laurenti et al., 2013). Notch 1 and its ligands play essential roles in T-lineage commitment (Radtke et al., 2004). For example, Delta-like 1 (DL1) enhances the repopulation capability of human CD34+CD38− cells in the BM and contributes to the generation of thymus-repopulating T-cell precursors (Ohishi et al., 2002). In addition, Delta-like 4 (DL4) induces Notch signaling in the thymus (Hozumi et al., 2008). Accordingly, the stable expression of DL1 or DL4 by OP9 cells allows for the differentiation of human HSPCs to T cells in vitro (La Motte-Mohs et al., 2005; Mohtashami et al., 2010). C-X-C chemokine receptor type 4 (CXCR4) also plays a critical role in the localization and differentiation of T-lineage progenitors in the thymus (Plotkin et al., 2003).
HSPC-Associated Hematological Changes in HIV Infection
Hematological changes in HIV-infected patients may be at least partly associated with abnormalities in the BM (Dhurve and Dhurve, 2013; Durandt et al., 2019). Because HSPCs generally have limited surface expression of CD4, their abnormalities in HIV infection could be largely explained as an indirect effect of HIV infection, rather than the results of direct infection of HSPCs (Louache et al., 1992; De Luca et al., 1993; Maciejewski et al., 1994; Marandin et al., 1996; Koka et al., 1999). Although antiretroviral therapy (ART) generally improves hematopoiesis in HIV-infected patients (Baillou et al., 2003), the immune function in some patients is insufficient despite successful ART; therefore, such patients are referred to as immunological non-responders (Corbeau and Reynes, 2011; Takuva et al., 2014; Rb-Silva et al., 2019). Indeed, the recovery of CD4+ T cell counts after successful ART may depend on the recovery of CD34+ cell counts (Sauce et al., 2011).
Lymphopoiesis, myelopoiesis, megakaryopoiesis, and erythropoiesis may be altered during the course of HIV infection (Figure 1). HIV-1 infection may cause defective myelopoiesis/erythropoiesis as well as the accumulation of myeloid/erythroid precursors (Costantini et al., 2009, 2010). Ineffective platelet production noted in HIV-infected patients (Cole et al., 1998) might be due to a negative impact of HIV on the differentiation of megakaryocyte lineages, leading to thrombocytopenia (Costantini et al., 2006; Sundell and Koka, 2006). The V3 loop region of the HIV-1 gp120 envelope protein was described as a potential inhibitor of megakaryocyte differentiation (Zhang et al., 2010). Furthermore, studies have suggested the influence of HIV-1 gp120/CD4 interaction on CD34+ megakaryocytic/erythroid progenitors (Gibellini et al., 2007; Morini et al., 2016).
The Biological Functions of HIV Coreceptors
HIV-1 uses C–C chemokine receptor type 5 (CCR5) and CXCR4 as coreceptors (Weiss, 1996). CCR5 is expressed on the surface of memory CD4+ T cells and causes the massive depletion of this cell type following HIV-1 infection of the host (Mattapallil et al., 2005). Recent evidence suggests that CCR5 is involved in inflammation (Kitade et al., 2012; Barashi et al., 2013; Duan et al., 2014) because the lack of a functional CCR5 allele is associated with the severity of viral infection, possibly due to altered immune responses (Lim et al., 2008). On the other hand, the pathological roles of CCR5 in various infectious and non-infectious diseases, e.g., autoimmune diseases, have been suggested (Vangelista and Vento, 2017). For example, the depletion of CCR5 was associated with attenuation of the adverse effects of inflammation (Muntinghe et al., 2009), and blockade of CCR5 inhibited leukocyte trafficking and reportedly reduced inflammation in a murine model of colitis (Mencarelli et al., 2016). Thus, these findings address the roles of CCR5 in health and disease.
CXCR4 is specific for stromal cell-derived factor 1 (SDF-1, also known as CXCL12). SDF-1 is produced by BM stromal cells, including CXCL12-abundant reticular cells (Nagasawa, 2015), and allows the homing of HSCs to BM. The interaction between SDF-1 and CXCR4 is essential for hematopoiesis (Karpova and Bonig, 2015). In addition, the SDF-1/CXCR4 axis has multiple essential roles in life (Murphy and Heusinkveld, 2018), such as embryonic (Mcgrath et al., 1999) and vascular (Takabatake et al., 2009; Kim et al., 2017) development, while providing support for the survival and migration of neoplastic cells (Chatterjee et al., 2014). The polymorphisms of SDF-1 might affect the ability to prevent HIV-1 infection (Winkler et al., 1998; Kuipers et al., 1999). However, the effect of SDF-1 polymorphisms on the susceptibility of the host to HIV-1 infection might be moderate (Ding et al., 2018). In contrast to the popularity of the topics of CXCR4 as an HIV-1 coreceptor and SDF-1 as an inhibitor of HIV-1 infection (Arenzana-Seisdedos, 2015), relatively few articles have addressed the intrinsic functions of SDF-1 and CXCR4 in the pathogenesis of HIV-1 infection and AIDS (Ikegawa et al., 2001; Tsukamoto, 2018).
Potential Mechanisms Underlying the Loss of or Changes in HSPCs in Response to HIV Infection of the Host
Various potential mechanisms underlying changes in HSPCs during HIV infection have been suggested, such as reduced c-Mpl (thrombopoietin receptor) expression on HSPCs (Koka et al., 2004), elevated plasma SDF-1 levels (Ikegawa et al., 2001), and altered BM niches (Moses et al., 1996). HIV-1 infection results in increased levels of inflammatory cytokines, affecting dynamics and functions (Bordoni et al., 2017) or inducing Fas-mediated apoptosis (Isgro et al., 2004) of HSPCs (Figure 1). Importantly, HSPCs require inflammatory signals in their development (Luis et al., 2016), and therefore may contribute to inflammation (Fischer and Agrawal, 2013). A recent study reported the emergence of a CD34+CD226(DNAM-1)brightCXCR4+ LP subset in association with chronic HIV infection and inflammation, reflecting altered dynamics of natural killer (NK) cells and α/β T cells (Bozzano et al., 2015; Figure 1). Finally, there has recently been an emerging trend to interpret some hematopoietic changes during the course of HIV infection as the accelerated senescence of HSPCs (Appay and Sauce, 2017; Fali et al., 2018; Fastenackels et al., 2019).
Humanized mouse models provide important resources for the analysis of BM HSPCs following HIV-1 infection. For example, in studies with humanized mice challenged with CXCR4-tropic HIV-1NL4−3, CD34+ cells were depleted and/or exhibited impaired ex vivo myeloid and erythroid colony-forming capacities (Jenkins et al., 1998; Koka et al., 1998). Moreover, the reduction in BM HSPC counts in humanized mice was observed even after CCR5-tropic HIV-1 infection (Arainga et al., 2016). Other research groups have reported that the loss of CD34+ cells in CCR5-tropic HIV-1 infection might be dependent on plasmacytoid dendritic cells (pDCs) (Li et al., 2017) or correlated with CXCR4 expression (Tsukamoto, 2018; Figure 1). Therefore, it is important to further investigate changes such as altered expression of cytokines in pDCs and other cells residing in BM in HIV infection. The latter could implicate the involvement of SDF-1/CXCR4 axis in the pathogenesis such as accelerated turnover of HSPCs.
The Impact of HIV on T-Lineage Development
The involvement of the thymus in HIV pathogenesis has been investigated (Ye et al., 2004). HIV-1 may cause thymocyte depletion mediated by an indirect cytopathic effect and infection of CD3−CD4+CD8− progenitor cells (Su et al., 1995). In an in vitro model imitating the thymic environment, thymocyte maturation was inhibited by HIV infection of the CD44+CD25−CD3− cell lineage (Knutsen et al., 1999). Early ART might preserve the lymphopoiesis capability of the host (Bordoni et al., 2015b, 2018; Rb-Silva et al., 2019) and reverse reduced thymic function (Withers-Ward et al., 1997; Levine et al., 2001).
In a BLT (BM, liver, and thymus) mouse model, HIV-1 Nef enhanced HIV-1 replication and caused depletion of CD4+CD8+ thymocytes (Zou et al., 2012). In another humanized mouse model, HIV-1 infection caused perturbation of cytokine mRNA expression in infected thymocytes. For instance, mRNA levels of interleukin (IL)-6, interferon-γ, and IL-2 were increased, whereas macrophage inflammatory protein (MIP)-1β expression was decreased. On the other hand, HIV infection of human stromal cells increased IL-6 levels, whereas SDF-1 expression levels were unaffected (Koka et al., 2003).
There have also been several reports on the T-lineage differentiation of HPCs, although it may be difficult to interpret all the data collectively. T-lineage progenitors express CXCR4 and are susceptible to CXCR4-tropic HIV infection (Berkowitz et al., 1998). In a study, BM cells infected with HIV before ART initiation had reduced amounts of CD34+ cells, but not CD34+CD7+ LPs (Muller et al., 2002). Although the data are intriguing, the study lacked information of absolute cell counts, so their notions were not firmly concluded except for reduced CD34+ frequencies. In another study of BM samples from HIV-infected ART-treated immunological non-responders, clonogenic capability and the sizes of primitive HSPCs were altered, which were associated with reduced production of IL-2, increased production of TNF-α, and increased stromal production of IL-7 (Isgro et al., 2008). Another study using a lentiviral vector expressing HIV-1 Nef showed that Nef may impair the differentiation of HSPCs to CD3ε+CD5+CD1a+ T/NK precursors (Dorival et al., 2008).
In a recent study, BM-derived HSPCs from HIV-infected patients exhibited reduced T-cell differentiation potential and increased production of pro-inflammatory cytokines, indicating that they are also produced by non-LPs. However, it remains unclear whether pro-inflammatory cytokine secretion is the cause or consequence of impaired T cell differentiation potential (Bordoni et al., 2017; Figure 1). Also, in a macaque model, following challenge with simian immunodeficiency virus, BM-derived CD34+ cells exhibited reduced T-lineage differentiation potential in vitro without significant changes in phenotypic analysis of CD34+ subsets (Thiebot et al., 2005). Another recent study suggested that CD34+CD7+CXCR4+ cells may be depleted in response to CXCR4-tropic HIV-1 infection in a coculture of HIV-infected umbilical cord-derived CD34+ and OP9-DL1 cells (Tsukamoto, 2019b; Figure 1). Despite the evidence of LPs during HIV-1 infection, our understanding of the impact of HIV-1 on LPs remains limited.
Direct HIV Infection of HSPCs
HSPCs have limited surface levels of HIV receptors and coreceptors compared with differentiated CD4+ cells. CD34+CD133+ umbilical cord-derived HSCs may have further limited expression levels of CD4, CXCR4, and CCR5 (Hariharan et al., 1999). In an in vitro culture study, BM-derived CD34+CD38− primitive HPCs were exposed to HIV-1 or HIV-2, but infection was not observed (Weichold et al., 1998). In another study, HIV-1 exposure had no effect on the in vitro expansion/proliferation dynamics of HSPCs (Kaushal et al., 1996). However, accumulating evidence has implicated HIV-susceptible subsets of HSPCs in patients (Louache et al., 1994; Zauli et al., 1994; Chelucci et al., 1995, 1999). In addition, peripheral blood CD34+ cells expressing CXCR4/CCR5 are susceptible to diverse strains of HIV-1 (Ruiz et al., 1998). Another study found that BM CD34+CD4+ cells are depleted in HIV-infected patients (Banda et al., 1999). Moreover, the HIV-1 Gag protein was expressed by BM HSPCs isolated from HIV-infected patients (Carter et al., 2010). A recent study of patient samples revealed that some HSPC subsets express high levels of CD4 and may harbor both CCR5-tropic and CXCR4-tropic HIV genomes (Sebastian et al., 2017). Furthermore, HSPCs latently infected with cytomegalovirus may have enhanced susceptibility to HIV-1 infection (Cheung et al., 2017). To confirm this evidence, another study using humanized BLT mice demonstrated HIV-1 infection of HPCs in vivo. These infected HPCs remained capable of differentiating to myeloid cells in vitro, albeit with reduced efficacy (Nixon et al., 2013; Figure 1).
Regarding molecular mechanisms preventing HIV infection except limited (co) receptor expression, a recent study suggested a post-entry mechanism to allow HSPCs to restrict HIV-1 replication prior to conversion of viral RNA into DNA and integration into the host genome (Griffin and Goff, 2015). Variations of tripartite motif-containing protein 5 may also influence the infection efficiency of lentiviruses in human and rhesus HSPCs (Evans et al., 2014). CCR5-ligand β-chemokines, including RANTES and MIP-1β, produced by HSPCs may modify the susceptibility of these cells to CCR5-tropic HIV-1 Env (Majka et al., 1999, 2000).
Some studies on HIV infection of HSPCs have relied on in vitro stimulation of cells with 50–100 ng/mL of individual stem cell factors, thrombopoietin, or FMS-like tyrosine kinase 3 ligand to overcome the low permissiveness of these cells to retrovirus/lentivirus infection (Santoni De Sio and Naldini, 2009). Such stimulation may enhance gene expression of the HIV-1 receptor and coreceptors, leading to overestimation of HIV infection/replication levels in HSPCs (Zhang et al., 2009). A method to achieve reproducible in vitro infection of HSPCs with CXCR4-tropic HIV-1 with RetroNectin-coated plate, but without strong cytokine stimulation, has been proposed (Tsukamoto and Okada, 2017).
HSPCs as Viral Reservoirs
There is no consensus on whether HSPCs are a major HIV reservoir (Von Laer et al., 1990; Stanley et al., 1992; Neal et al., 1995; Kandathil et al., 2016). A relatively recent study of BM HSPCs from eight patients following long-term effective ART found no HIV DNA in the collected cells (Josefsson et al., 2012), suggesting that HIV reservoir surveys of purified CD34+ cells may fail to exclude HIV-contaminated CD4+ T cells (Durand et al., 2012). In contrast, accumulating data support latent HIV infection of HSPCs. Moreover, some BM HSPCs may remain latently infected after successful treatment (Bordoni et al., 2015a). Another study suggested that multiple subsets of HSPCs may be latently infected with HIV-1, including immature (CD34+CD38−CD45RA−) progenitors, which are more likely to persist and serve as latent reservoirs following ART (Mcnamara et al., 2012). Humanized mouse models have also been utilized to investigate CD34+ HIV reservoirs. A previous study revealed that the HIV-infected HSPCs may serve as long-term HIV reservoirs in the BM of humanized mice, leading to production of HIV-integrated CD3+ T cells (Carter et al., 2011). Taken together, HSPCs might constitute significant HIV reservoirs, which should be further investigated.
While it remains unclear whether infected HSPCs contribute to residual viremia after ART (Onafuwa-Nuga et al., 2010; Mcnamara and Collins, 2011), a recent article reported that HSPCs in suppressed patients harbor functional HIV proviral genomes that often match residual peripheral viral RNA (Zaikos et al., 2018). If these findings are confirmed, HSPCs might be finally regarded as long-term viral reservoirs, because they are long-lived cells with regulated susceptibility to apoptosis (Durdik et al., 2017). Thus, precise identification of HSPC subsets harboring functional HIV proviral copies could further facilitate these findings and clarify the role of HSPCs in HIV persistence even after successful ART. Furthermore, it is interesting to assess whether early initiation of ART could prevent the establishment of viral reservoirs in HSPCs.
Protection of HSPCs Against HIV Infection
Presently, the best method for treating HIV-infected individuals in terms of protection of HSPCs is to initiate ART as early as possible regardless of the disease stage (World Health Organization, 2015). By interrupting HIV pathogenesis early during infection, it is expected that existing CD4+ T cells and HSPCs as well as the host's hematopoietic capacity will be preserved for long (Bordoni et al., 2015b). However, more treatment options might be helpful for patients who are diagnosed in the chronic phase and/or those who manifest the characteristics of immunological non-responders against the current ART regimens (Rb-Silva et al., 2019).
CXCR4 may be targeted to protect HSPCs against CXCR4-tropic HIV-1 infection, because they express CXCR4 and are considered susceptible to CXCR4-tropic HIV-1 infection. For example, the μ-opioid agonist DAMGO (C26H35N5O6) was found to downregulate CXCR4 expression and prevent HIV-1 infection of BM HSPCs (Strazza et al., 2014). On the other hand, a clinical study reported that the CXCR4 antagonist plerixafor was not successful for the treatment of HIV-infected patients (Hendrix et al., 2004). Because systemic administration of plerixafor is associated with adverse effects, especially to patients with cardiovascular diseases, further development of CXCR4-tropic HIV-1 entry inhibitors with weaker affinity to CXCR4 than plerixafor is needed (Berg et al., 2018). Other entry inhibitors such as ibalizumab, a humanized monoclonal anti-CD4 antibody that inhibits the binding of HIV gp120, might also be highly effective in preventing HSPCs from infection (Emu et al., 2018). It is unclear whether CCR5-tropic HIV-1 entry inhibitors such as maraviroc are effective in protecting HSPCs because HIV-1 is considered to use CXCR4 to enter those cells (Carter et al., 2011). However, those entry inhibitors can lower viral burden by protecting CCR5+ memory CD4+ T cells and lead to lower risks for indirect damages to HSPCs.
The significance of CXCR4 in HIV-1 infection is not necessarily limited to its function as an HIV-1 coreceptor. For example, it is unclear how the biological roles of CXCR4, including the SDF-1/CXCR4 signaling pathway in the BM and thymus, affect hematopoiesis in response to HIV infection. It has been indicated that elevation of plasma SDF-1 levels may be associated with disease progression (Ikegawa et al., 2001). Another study suggested the use of granulocyte colony-stimulating factor to increase CD34+ and CD4+ cell counts in HIV-infected patients (Nielsen et al., 1998). In addition, a recent humanized mouse study indicated the involvement of CXCR4 in the loss of BM HSPCs in CCR5-tropic HIV-1 infection (Tsukamoto, 2018; Figure 1). These results must be further investigated to elucidate whether the loss of HSPCs following HIV-1 infection can be alleviated by interrupting the SDF-1/CXCR4 signaling pathway.
Recent studies indicate HSPCs as an ideal target for anti-HIV gene therapy aimed to protect hosts' hematopoietic potential (Kitchen et al., 2011; Savkovic et al., 2014). For detailed discussions on recent advances in the field, see a recently published review by this author Tsukamoto (2019a).
Concluding Remarks
Despite previous efforts and accumulating data to better clarify the interactions between HIV-1 and HSPCs, studies on their involvement in HIV pathogenesis are ongoing. The contribution of latently infected HSPCs to viral persistence should be better described. Regarding HSPC subsets, recent evidence supports the influence of HIV-1 on myeloid progenitor cells. On the other hand, among various steps in T-lineage development, the functional and numerical alteration of CD34+ LPs in HIV-1 infection needs to be further elucidated to improve the current understanding of the degree of impaired CD4+ T-cell generation on peripheral CD4+ T-cell loss and AIDS onset. Humanized mouse models and in vitro models including OP9-DL1/OP9-DL4 coculture systems could be used for further analysis of HSPCs in HIV infection in this context. Further investigations in these fields will collectively enhance our understanding on the significance of protecting HSPCs in HIV infection.
Author Contributions
The author confirms being the sole contributor of this work and has approved it for publication.
Funding
This study was partly supported by a grant from The Imai Memorial Trust for AIDS Research (Tokyo, Japan).
Conflict of Interest
The author declares that the research was conducted in the absence of any commercial or financial relationships that could be construed as a potential conflict of interest.
Acknowledgments
The author thanks Enago (http://www.enago.jp) for the English language review.
References
Appay, V., and Sauce, D. (2017). Assessing immune aging in HIV-infected patients. Virulence 8, 529–538. doi: 10.1080/21505594.2016.1195536
Arainga, M., Su, H., Poluektova, L. Y., Gorantla, S., and Gendelman, H. E. (2016). HIV-1 cellular and tissue replication patterns in infected humanized mice. Sci. Rep. 6:23513. doi: 10.1038/srep23513
Arenzana-Seisdedos, F. (2015). SDF-1/CXCL12: a chemokine in the life cycle of HIV. Front. Immunol. 6:256. doi: 10.3389/fimmu.2015.00256
Baillou, C., Simon, A., Leclercq, V., Azar, N., Rosenzwajg, M., Herson, S., et al. (2003). Highly active antiretroviral therapy corrects hematopoiesis in HIV-1 infected patients: interest for peripheral blood stem cell-based gene therapy. AIDS 17, 563–574. doi: 10.1097/00002030-200303070-00012
Banda, N. K., Simon, G. R., Sipple, J. D., Terrell, K. L., Archer, P., Shpall, E. J., et al. (1999). Depletion of CD34+ CD4+ cells in bone marrow from HIV-1-infected individuals. Biol. Blood Marrow Transplant. 5, 162–172. doi: 10.1053/bbmt.1999.v5.pm10392962
Barashi, N., Weiss, I. D., Wald, O., Wald, H., Beider, K., Abraham, M., et al. (2013). Inflammation-induced hepatocellular carcinoma is dependent on CCR5 in mice. Hepatology 58, 1021–1030. doi: 10.1002/hep.26403
Berg, C., Daugvilaite, V., Steen, A., Jorgensen, A. S., Vabeno, J., and Rosenkilde, M. M. (2018). Inhibition of HIV fusion by small molecule agonists through efficacy-engineering of CXCR4. ACS Chem. Biol. 13, 881–886. doi: 10.1021/acschembio.8b00061
Berkowitz, R. D., Beckerman, K. P., Schall, T. J., and Mccune, J. M. (1998). CXCR4 and CCR5 expression delineates targets for HIV-1 disruption of T cell differentiation. J. Immunol. 161, 3702–3710.
Bordoni, V., Bibas, M., Abbate, I., Viola, D., Rozera, G., Agrati, C., et al. (2015a). Bone marrow CD34+ progenitor cells may harbour HIV-DNA even in successfully treated patients. Clin. Microbiol. Infect 21, 290.e295–298. doi: 10.1016/j.cmi.2014.11.003
Bordoni, V., Bibas, M., Viola, D., Sacchi, A., Cimini, E., Tumino, N., et al. (2017). Bone marrow CD34(+) progenitor cells from HIV-infected patients show an impaired T cell differentiation potential related to proinflammatory cytokines. AIDS Res. Hum. Retroviruses 33, 590–596. doi: 10.1089/AID.2016.0195
Bordoni, V., Casetti, R., Viola, D., Abbate, I., Rozera, G., Sacchi, A., et al. (2015b). Early ART in primary HIV infection may also preserve lymphopoiesis capability in circulating haematopoietic progenitor cells: a case report. J. Antimicrob. Chemother. 70, 1598–1600. doi: 10.1093/jac/dku559
Bordoni, V., Viola, D., Sacchi, A., Pinnetti, C., Casetti, R., Cimini, E., et al. (2018). IL-18 and stem cell factor affect hematopoietic progenitor cells in HIV-infected patients treated during primary HIV infection. Cytokine 103, 34–37. doi: 10.1016/j.cyto.2017.12.033
Bozzano, F., Marras, F., Ascierto, M. L., Cantoni, C., Cenderello, G., Dentone, C., et al. (2015). 'Emergency exit' of bone-marrow-resident CD34(+)DNAM-1(bright)CXCR4(+)-committed lymphoid precursors during chronic infection and inflammation. Nat. Commun. 6:8109. doi: 10.1038/ncomms9109
Carter, C. C., Mcnamara, L. A., Onafuwa-Nuga, A., Shackleton, M., Riddell, J. T., Bixby, D., et al. (2011). HIV-1 utilizes the CXCR4 chemokine receptor to infect multipotent hematopoietic stem and progenitor cells. Cell Host Microbe 9, 223–234. doi: 10.1016/j.chom.2011.02.005
Carter, C. C., Onafuwa-Nuga, A., Mcnamara, L. A., Riddell, J. T., Bixby, D., Savona, M. R., et al. (2010). HIV-1 infects multipotent progenitor cells causing cell death and establishing latent cellular reservoirs. Nat. Med. 16, 446–451. doi: 10.1038/nm.2109
Chatterjee, S., Behnam Azad, B., and Nimmagadda, S. (2014). The intricate role of CXCR4 in cancer. Adv. Cancer Res. 124, 31–82. doi: 10.1016/B978-0-12-411638-2.00002-1
Chelucci, C., Casella, I., Federico, M., Testa, U., Macioce, G., Pelosi, E., et al. (1999). Lineage-specific expression of human immunodeficiency virus (HIV) receptor/coreceptors in differentiating hematopoietic precursors: correlation with susceptibility to T- and M-tropic HIV and chemokine-mediated HIV resistance. Blood 94, 1590–1600. doi: 10.1182/blood.V94.5.1590
Chelucci, C., Hassan, H. J., Locardi, C., Bulgarini, D., Pelosi, E., Mariani, G., et al. (1995). In vitro human immunodeficiency virus-1 infection of purified hematopoietic progenitors in single-cell culture. Blood 85, 1181–1187. doi: 10.1182/blood.V85.5.1181.bloodjournal8551181
Cheung, A. K. L., Huang, Y., Kwok, H. Y., Chen, M., and Chen, Z. (2017). Latent human cytomegalovirus enhances HIV-1 infection in CD34(+) progenitor cells. Blood Adv. 1, 306–318. doi: 10.1182/bloodadvances.2016000638
Cole, J. L., Marzec, U. M., Gunthel, C. J., Karpatkin, S., Worford, L., Sundell, I. B., et al. (1998). Ineffective platelet production in thrombocytopenic human immunodeficiency virus-infected patients. Blood 91, 3239–3246. doi: 10.1182/blood.V91.9.3239
Corbeau, P., and Reynes, J. (2011). Immune reconstitution under antiretroviral therapy: the new challenge in HIV-1 infection. Blood 117, 5582–5590. doi: 10.1182/blood-2010-12-322453
Costantini, A., Giuliodoro, S., Butini, L., Silvestri, G., Leoni, P., and Montroni, M. (2009). Abnormalities of erythropoiesis during HIV-1 disease: a longitudinal analysis. J. Acquir. Immune Defic. Syndr. 52, 70–74. doi: 10.1097/QAI.0b013e3181af65c5
Costantini, A., Giuliodoro, S., Butini, L., Silvestri, G., Leoni, P., and Montroni, M. (2010). HIV-induced abnormalities in myelopoiesis and their recovery following antiretroviral therapy. Curr. HIV Res. 8, 336–339. doi: 10.2174/157016210791208604
Costantini, A., Giuliodoro, S., Mancini, S., Butini, L., Regnery, C. M., Silvestri, G., et al. (2006). Impaired in-vitro growth of megakaryocytic colonies derived from CD34 cells of HIV-1-infected patients with active viral replication. AIDS 20, 1713–1720. doi: 10.1097/01.aids.0000242817.88086.8c
De Luca, A., Teofili, L., Antinori, A., Iovino, M. S., Mencarini, P., Visconti, E., et al. (1993). Haemopoietic CD34+ progenitor cells are not infected by HIV-1 in vivo but show impaired clonogenesis. Br. J. Haematol. 85, 20–24. doi: 10.1111/j.1365-2141.1993.tb08640.x
Dhurve, S. A., and Dhurve, A. S. (2013). Bone Marrow abnormalities in HIV disease. Mediterr. J. Hematol. Infect. Dis. 5:e2013033. doi: 10.4084/MJHID.2013.033
Ding, J., Zhao, J., Zhou, J., Li, X., Wu, Y., Ge, M., et al. (2018). Association of gene polymorphism of SDF1(CXCR12) with susceptibility to HIV-1 infection and AIDS disease progression: a meta-analysis. PLoS ONE 13:e0191930. doi: 10.1371/journal.pone.0191930
Ding, L., and Morrison, S. J. (2013). Haematopoietic stem cells and early lymphoid progenitors occupy distinct bone marrow niches. Nature 495, 231–235. doi: 10.1038/nature11885
Ding, L., Saunders, T. L., Enikolopov, G., and Morrison, S. J. (2012). Endothelial and perivascular cells maintain haematopoietic stem cells. Nature 481, 457–462. doi: 10.1038/nature10783
Dorival, C., Brizzi, F., Lelievre, J. D., Sol-Foulon, N., Six, E., Henry, A., et al. (2008). HIV-1 Nef protein expression in human CD34+ progenitors impairs the differentiation of an early T/NK cell precursor. Virology 377, 207–215. doi: 10.1016/j.virol.2008.04.009
Duan, H., Yang, P., Fang, F., Ding, S., and Xiao, W. (2014). CCR5 small interfering RNA ameliorated joint inflammation in rats with adjuvant-induced arthritis. Immunol. Lett. 162, 258–263. doi: 10.1016/j.imlet.2014.09.018
Durand, C. M., Ghiaur, G., Siliciano, J. D., Rabi, S. A., Eisele, E. E., Salgado, M., et al. (2012). HIV-1 DNA is detected in bone marrow populations containing CD4+ T cells but is not found in purified CD34+ hematopoietic progenitor cells in most patients on antiretroviral therapy. J. Infect. Dis. 205, 1014–1018. doi: 10.1093/infdis/jir884
Durandt, C., Potgieter, J., Mellet, J., Herd, C., Khoosal, R., Nel, J. G., et al. (2019). HIV and haematopoiesis. S. Afr. Med. J. 109, 40–45. doi: 10.7196/SAMJ.2019.v109i8b.13829
Durdik, M., Kosik, P., Kruzliakova, J., Jakl, L., Markova, E., and Belyaev, I. (2017). Hematopoietic stem/progenitor cells are less prone to undergo apoptosis than lymphocytes despite similar DNA damage response. Oncotarget 8, 48846–48853. doi: 10.18632/oncotarget.16455
Emu, B., Fessel, J., Schrader, S., Kumar, P., Richmond, G., Win, S., et al. (2018). Phase 3 study of ibalizumab for multidrug-resistant HIV-1. N. Engl. J. Med. 379, 645–654. doi: 10.1056/NEJMoa1711460
Evans, M. E., Kumkhaek, C., Hsieh, M. M., Donahue, R. E., Tisdale, J. F., and Uchida, N. (2014). TRIM5α variations influence transduction efficiency with lentiviral vectors in both human and rhesus CD34(+) cells in vitro and in vivo. Mol. Ther. 22, 348–358. doi: 10.1038/mt.2013.256
Fali, T., Fabre-Mersseman, V., Yamamoto, T., Bayard, C., Papagno, L., Fastenackels, S., et al. (2018). Elderly human hematopoietic progenitor cells express cellular senescence markers and are more susceptible to pyroptosis. JCI Insight 3:e95319. doi: 10.1172/jci.insight.95319
Fastenackels, S., Sauce, D., Vigouroux, C., Avettand-Fenoel, V., Bastard, J. P., Fellahi, S., et al. (2019). HIV-mediated immune aging in young adults infected perinatally or during childhood. AIDS 33, 1705–1710. doi: 10.1097/QAD.0000000000002275
Fischer, K. D., and Agrawal, D. K. (2013). Hematopoietic stem and progenitor cells in inflammation and allergy. Front. Immunol. 4:428. doi: 10.3389/fimmu.2013.00428
Gibellini, D., Vitone, F., Buzzi, M., Schiavone, P., De Crignis, E., Cicola, R., et al. (2007). HIV-1 negatively affects the survival/maturation of cord blood CD34(+) hematopoietic progenitor cells differentiated towards megakaryocytic lineage by HIV-1 gp120/CD4 membrane interaction. J. Cell. Physiol. 210, 315–324. doi: 10.1002/jcp.20815
Griffin, D. O., and Goff, S. P. (2015). HIV-1 is restricted prior to integration of viral DNA in primary cord-derived human CD34+ cells. J. Virol. 89, 8096–8100. doi: 10.1128/JVI.01044-15
Hariharan, D., Li, Y., Campbell, D. E., Douglas, S. D., Starr, S. E., and Ho, W. (1999). Human immunodeficiency virus infection of human placental cord blood CD34+AC133+ stem cells and their progeny. AIDS Res. Hum. Retroviruses 15, 1545–1552. doi: 10.1089/088922299309838
He, Q., Zhang, C., Wang, L., Zhang, P., Ma, D., Lv, J., et al. (2015). Inflammatory signaling regulates hematopoietic stem and progenitor cell emergence in vertebrates. Blood 125, 1098–1106. doi: 10.1182/blood-2014-09-601542
Hendrix, C. W., Collier, A. C., Lederman, M. M., Schols, D., Pollard, R. B., Brown, S., et al. (2004). Safety, pharmacokinetics, and antiviral activity of AMD3100, a selective CXCR4 receptor inhibitor, in HIV-1 infection. J. Acquir. Immune Defic. Syndr. 37, 1253–1262. doi: 10.1097/01.qai.0000137371.80695.ef
Hozumi, K., Mailhos, C., Negishi, N., Hirano, K., Yahata, T., Ando, K., et al. (2008). Delta-like 4 is indispensable in thymic environment specific for T cell development. J. Exp. Med. 205, 2507–2513. doi: 10.1084/jem.20080134
Ikegawa, M., Yuan, J., Matsumoto, K., Herrmann, S., Iwamoto, A., Nakamura, T., et al. (2001). Elevated plasma stromal cell-derived factor 1 protein level in the progression of HIV type 1 infection/AIDS. AIDS Res. Hum. Retroviruses 17, 587–595. doi: 10.1089/088922201300119680
Isgro, A., Leti, W., De Santis, W., Marziali, M., Esposito, A., Fimiani, C., et al. (2008). Altered clonogenic capability and stromal cell function characterize bone marrow of HIV-infected subjects with low CD4+ T cell counts despite viral suppression during HAART. Clin. Infect. Dis. 46, 1902–1910. doi: 10.1086/588480
Isgro, A., Mezzaroma, I., Aiuti, A., Fantauzzi, A., Pinti, M., Cossarizza, A., et al. (2004). Decreased apoptosis of bone marrow progenitor cells in HIV-1-infected patients during highly active antiretroviral therapy. AIDS 18, 1335–1337. doi: 10.1097/00002030-200406180-00013
Jenkins, M., Hanley, M. B., Moreno, M. B., Wieder, E., and Mccune, J. M. (1998). Human immunodeficiency virus-1 infection interrupts thymopoiesis and multilineage hematopoiesis in vivo. Blood 91, 2672–2678. doi: 10.1182/blood.V91.8.2672.2672_2672_2678
Josefsson, L., Eriksson, S., Sinclair, E., Ho, T., Killian, M., Epling, L., et al. (2012). Hematopoietic precursor cells isolated from patients on long-term suppressive HIV therapy did not contain HIV-1 DNA. J. Infect. Dis. 206, 28–34. doi: 10.1093/infdis/jis301
Kandathil, A. J., Sugawara, S., and Balagopal, A. (2016). Are T cells the only HIV-1 reservoir? Retrovirology 13:86. doi: 10.1186/s12977-016-0323-4
Karpova, D., and Bonig, H. (2015). Concise review: CXCR4/CXCL12 signaling in immature hematopoiesis–lessons from pharmacological and genetic models. Stem Cells 33, 2391–2399. doi: 10.1002/stem.2054
Kaushal, S., La Russa, V. F., Gartner, S., Kessler, S., Perfetto, S., Yu, Z., et al. (1996). Exposure of human CD34+ cells to human immunodeficiency virus type 1 does not influence their expansion and proliferation of hematopoietic progenitors in vitro. Blood 88, 130–137. doi: 10.1182/blood.V88.1.130.130
Kim, B. G., Kim, Y. H., Stanley, E. L., Garrido-Martin, E. M., Lee, Y. J., and Oh, S. P. (2017). CXCL12-CXCR4 signalling plays an essential role in proper patterning of aortic arch and pulmonary arteries. Cardiovasc. Res. 113, 1677–1687. doi: 10.1093/cvr/cvx188
Kim, Y. C., Wu, Q., Chen, J., Xuan, Z., Jung, Y. C., Zhang, M. Q., et al. (2009). The transcriptome of human CD34+ hematopoietic stem-progenitor cells. Proc. Natl. Acad. Sci. U.S.A. 106, 8278–8283. doi: 10.1073/pnas.0903390106
Kitade, H., Sawamoto, K., Nagashimada, M., Inoue, H., Yamamoto, Y., Sai, Y., et al. (2012). CCR5 plays a critical role in obesity-induced adipose tissue inflammation and insulin resistance by regulating both macrophage recruitment and M1/M2 status. Diabetes 61, 1680–1690. doi: 10.2337/db11-1506
Kitchen, S. G., Shimizu, S., and An, D. S. (2011). Stem cell-based anti-HIV gene therapy. Virology 411, 260–272. doi: 10.1016/j.virol.2010.12.039
Knutsen, A. P., Roodman, S. T., Freeman, J. J., Mueller, K. R., and Bouhasin, J. D. (1999). Inhibition of thymopoiesis of CD34+ cell maturation by HIV-1 in an in vitro CD34+ cell and thymic epithelial organ culture model. Stem Cells 17, 327–338. doi: 10.1002/stem.170327
Koka, P. S., Brooks, D. G., Razai, A., Kitchen, C. M., and Zack, J. A. (2003). HIV type 1 infection alters cytokine mRNA expression in thymus. AIDS Res. Hum. Retroviruses 19, 1–12. doi: 10.1089/08892220360473916
Koka, P. S., Fraser, J. K., Bryson, Y., Bristol, G. C., Aldrovandi, G. M., Daar, E. S., et al. (1998). Human immunodeficiency virus inhibits multilineage hematopoiesis in vivo. J. Virol. 72, 5121–5127. doi: 10.1128/JVI.72.6.5121-5127.1998
Koka, P. S., Jamieson, B. D., Brooks, D. G., and Zack, J. A. (1999). Human immunodeficiency virus type 1-induced hematopoietic inhibition is independent of productive infection of progenitor cells in vivo. J. Virol. 73, 9089–9097. doi: 10.1128/JVI.73.11.9089-9097.1999
Koka, P. S., Kitchen, C. M., and Reddy, S. T. (2004). Targeting c-Mpl for revival of human immunodeficiency virus type 1-induced hematopoietic inhibition when CD34+ progenitor cells are re-engrafted into a fresh stromal microenvironment in vivo. J. Virol. 78, 11385–11392. doi: 10.1128/JVI.78.20.11385-11392.2004
Kondo, M., Weissman, I. L., and Akashi, K. (1997). Identification of clonogenic common lymphoid progenitors in mouse bone marrow. Cell 91, 661–672. doi: 10.1016/s0092-8674(00)80453-5
Kuipers, H., Workman, C., Dyer, W., Geczy, A., Sullivan, J., and Oelrichs, R. (1999). An HIV-1-infected individual homozygous for the CCR-5 delta32 allele and the SDF-1 3'A allele. AIDS 13, 433–434. doi: 10.1097/00002030-199902250-00025
La Motte-Mohs, R. N., Herer, E., and Zuniga-Pflucker, J. C. (2005). Induction of T-cell development from human cord blood hematopoietic stem cells by Delta-like 1 in vitro. Blood 105, 1431–1439. doi: 10.1182/blood-2004-04-1293
Laurenti, E., Doulatov, S., Zandi, S., Plumb, I., Chen, J., April, C., et al. (2013). The transcriptional architecture of early human hematopoiesis identifies multilevel control of lymphoid commitment. Nat. Immunol. 14, 756–763. doi: 10.1038/ni.2615
Levine, A. M., Scadden, D. T., Zaia, J. A., and Krishnan, A. (2001). Hematologic Aspects of HIV/AIDS. Hematol. Am. Soc. Hematol. Educ. Program 2001, 463–478. doi: 10.1182/asheducation-2001.1.463
Li, G., Zhao, J., Cheng, L., Jiang, Q., Kan, S., Qin, E., et al. (2017). HIV-1 infection depletes human CD34+CD38- hematopoietic progenitor cells via pDC-dependent mechanisms. PLoS Pathog. 13:e1006505. doi: 10.1371/journal.ppat.1006505
Lim, J. K., Louie, C. Y., Glaser, C., Jean, C., Johnson, B., Johnson, H., et al. (2008). Genetic deficiency of chemokine receptor CCR5 is a strong risk factor for symptomatic West Nile virus infection: a meta-analysis of 4 cohorts in the US epidemic. J. Infect. Dis. 197, 262–265. doi: 10.1086/524691
Liu, F., Lu, J., Fan, H. H., Wang, Z. Q., Cui, S. J., Zhang, G. A., et al. (2006). Insights into human CD34+ hematopoietic stem/progenitor cells through a systematically proteomic survey coupled with transcriptome. Proteomics 6, 2673–2692. doi: 10.1002/pmic.200500032
Louache, F., Debili, N., Marandin, A., Coulombel, L., and Vainchenker, W. (1994). Expression of CD4 by human hematopoietic progenitors. Blood 84, 3344–3355. doi: 10.1182/blood.V84.10.3344.3344
Louache, F., Henri, A., Bettaieb, A., Oksenhendler, E., Raguin, G., Tulliez, M., et al. (1992). Role of human immunodeficiency virus replication in defective in vitro growth of hematopoietic progenitors. Blood 80, 2991–2999. doi: 10.1182/blood.V80.12.2991.2991
Luis, T. C., Tremblay, C. S., Manz, M. G., North, T. E., King, K. Y., and Challen, G. A. (2016). Inflammatory signals in HSPC development and homeostasis: too much of a good thing? Exp. Hematol. 44, 908–912. doi: 10.1016/j.exphem.2016.06.254
Maciejewski, J. P., Weichold, F. F., and Young, N. S. (1994). HIV-1 suppression of hematopoiesis in vitro mediated by envelope glycoprotein and TNF-alpha. J. Immunol. 153, 4303–4310.
Majka, M., Rozmyslowicz, T., Lee, B., Murphy, S. L., Pietrzkowski, Z., Gaulton, G. N., et al. (1999). Bone marrow CD34(+) cells and megakaryoblasts secrete beta-chemokines that block infection of hematopoietic cells by M-tropic R5 HIV. J. Clin. Invest. 104, 1739–1749. doi: 10.1172/JCI7779
Majka, M., Rozmyslowicz, T., Ratajczak, J., Dobrowsky, A., Pietrzkowski, Z., Gaulton, G. N., et al. (2000). The limited infectability by R5 HIV of CD34(+) cells from thymus, cord, and peripheral blood and bone marrow is explained by their ability to produce beta-chemokines. Exp. Hematol. 28, 1334–1342. doi: 10.1016/s0301-472x(00)00541-5
Marandin, A., Katz, A., Oksenhendler, E., Tulliez, M., Picard, F., Vainchenker, W., et al. (1996). Loss of primitive hematopoietic progenitors in patients with human immunodeficiency virus infection. Blood 88, 4568–4578. doi: 10.1182/blood.V88.12.4568.bloodjournal88124568
Mattapallil, J. J., Douek, D. C., Hill, B., Nishimura, Y., Martin, M., and Roederer, M. (2005). Massive infection and loss of memory CD4+ T cells in multiple tissues during acute SIV infection. Nature 434, 1093–1097. doi: 10.1038/nature03501
Mcgrath, K. E., Koniski, A. D., Maltby, K. M., Mcgann, J. K., and Palis, J. (1999). Embryonic expression and function of the chemokine SDF-1 and its receptor, CXCR4. Dev. Biol. 213, 442–456. doi: 10.1006/dbio.1999.9405
Mcnamara, L. A., and Collins, K. L. (2011). Hematopoietic stem/precursor cells as HIV reservoirs. Curr. Opin. HIV AIDS 6, 43–48. doi: 10.1097/COH.0b013e32834086b3
Mcnamara, L. A., Ganesh, J. A., and Collins, K. L. (2012). Latent HIV-1 infection occurs in multiple subsets of hematopoietic progenitor cells and is reversed by NF- κB activation. J. Virol. 86, 9337–9350. doi: 10.1128/JVI.00895-12
Mencarelli, A., Cipriani, S., Francisci, D., Santucci, L., Baldelli, F., Distrutti, E., et al. (2016). Highly specific blockade of CCR5 inhibits leukocyte trafficking and reduces mucosal inflammation in murine colitis. Sci. Rep. 6:30802. doi: 10.1038/srep30802
Mohtashami, M., Shah, D. K., Nakase, H., Kianizad, K., Petrie, H. T., and Zuniga-Pflucker, J. C. (2010). Direct comparison of Dll1- and Dll4-mediated Notch activation levels shows differential lymphomyeloid lineage commitment outcomes. J. Immunol. 185, 867–876. doi: 10.4049/jimmunol.1000782
Morini, S., Musumeci, G., Bon, I., Miserocchi, A., Alviano, F., Longo, S., et al. (2016). HIV-1 gp120 impairs the differentiation and survival of cord blood CD34+ HPCs induced to the erythroid lineage. New Microbiol. 39, 13–23.
Morrison, S. J., and Scadden, D. T. (2014). The bone marrow niche for haematopoietic stem cells. Nature 505, 327–334. doi: 10.1038/nature12984
Moses, A. V., Williams, S., Heneveld, M. L., Strussenberg, J., Rarick, M., Loveless, M., et al. (1996). Human immunodeficiency virus infection of bone marrow endothelium reduces induction of stromal hematopoietic growth factors. Blood 87, 919–925. doi: 10.1182/blood.V87.3.919.bloodjournal873919
Muller, F., Tjonnfjord, G. E., Nordoy, I., Kvale, D., Mellbye, O. J., Aukrust, P., et al. (2002). Immunophenotypic analyses of CD34(+) cell subsets in bone marrow from HIV-infected patients during highly- active antiretroviral therapy. Eur. J. Clin. Invest. 32, 535–540. doi: 10.1046/j.1365-2362.2002.00995.x
Muntinghe, F. L., Verduijn, M., Zuurman, M. W., Grootendorst, D. C., Carrero, J. J., Qureshi, A. R., et al. (2009). CCR5 deletion protects against inflammation-associated mortality in dialysis patients. J. Am. Soc. Nephrol. 20, 1641–1649. doi: 10.1681/ASN.2008040432
Murphy, P. M., and Heusinkveld, L. (2018). Multisystem multitasking by CXCL12 and its receptors CXCR4 and ACKR3. Cytokine 109, 2–10. doi: 10.1016/j.cyto.2017.12.022
Neal, T. F., Holland, H. K., Baum, C. M., Villinger, F., Ansari, A. A., Saral, R., et al. (1995). CD34+ progenitor cells from asymptomatic patients are not a major reservoir for human immunodeficiency virus-1. Blood 86, 1749–1756. doi: 10.1182/blood.V86.5.1749.bloodjournal8651749
Nielsen, S. D., Afzelius, P., Dam-Larsen, S., Nielsen, C., Nielsen, J. O., Mathiesen, L., et al. (1998). Effect of granulocyte colony-stimulating factor (G-CSF) in human immunodeficiency virus-infected patients: increase in numbers of naive CD4 cells and CD34 cells makes G-CSF a candidate for use in gene therapy or to support antiretroviral therapy. J. Infect. Dis. 177, 1733–1736. doi: 10.1086/517434
Nixon, C. C., Vatakis, D. N., Reichelderfer, S. N., Dixit, D., Kim, S. G., Uittenbogaart, C. H., et al. (2013). HIV-1 infection of hematopoietic progenitor cells in vivo in humanized mice. Blood 122, 2195–2204. doi: 10.1182/blood-2013-04-496950
Ohishi, K., Varnum-Finney, B., and Bernstein, I. D. (2002). Delta-1 enhances marrow and thymus repopulating ability of human CD34(+)CD38(−) cord blood cells. J. Clin. Invest. 110, 1165–1174. doi: 10.1172/JCI16167
Onafuwa-Nuga, A., Mcnamara, L. A., and Collins, K. L. (2010). Towards a cure for HIV: the identification and characterization of HIV reservoirs in optimally treated people. Cell Res. 20, 1185–1187. doi: 10.1038/cr.2010.140
Parinitha, S., and Kulkarni, M. (2012). Haematological changes in HIV infection with correlation to CD4 cell count. Australas. Med. J. 5, 157–162. doi: 10.4066/AMJ.20121008
Plotkin, J., Prockop, S. E., Lepique, A., and Petrie, H. T. (2003). Critical role for CXCR4 signaling in progenitor localization and T cell differentiation in the postnatal thymus. J. Immunol. 171, 4521–4527. doi: 10.4049/jimmunol.171.9.4521
Radtke, F., Wilson, A., Mancini, S. J., and Macdonald, H. R. (2004). Notch regulation of lymphocyte development and function. Nat. Immunol. 5, 247–253. doi: 10.1038/ni1045
Rb-Silva, R., Nobrega, C., Azevedo, C., Athayde, E., Canto-Gomes, J., Ferreira, I., et al. (2019). Thymic function as a predictor of immune recovery in chronically HIV-infected patients initiating antiretroviral therapy. Front. Immunol. 10:25. doi: 10.3389/fimmu.2019.00025
Res, P., Martinez-Caceres, E., Cristina Jaleco, A., Staal, F., Noteboom, E., Weijer, K., et al. (1996). CD34+CD38dim cells in the human thymus can differentiate into T, natural killer, and dendritic cells but are distinct from pluripotent stem cells. Blood 87, 5196–5206. doi: 10.1182/blood.V87.12.5196.bloodjournal87125196
Rieger, M. A., and Schroeder, T. (2012). Hematopoiesis. Cold Spring Harb. Perspect. Biol. 4:a008250. doi: 10.1101/cshperspect.a008250
Ruiz, M. E., Cicala, C., Arthos, J., Kinter, A., Catanzaro, A. T., Adelsberger, J., et al. (1998). Peripheral blood-derived CD34+ progenitor cells: CXC chemokine receptor 4 and CC chemokine receptor 5 expression and infection by HIV. J. Immunol. 161, 4169–4176.
Santoni De Sio, F., and Naldini, L. (2009). Short-term culture of human CD34+ cells for lentiviral gene transfer. Methods Mol. Biol. 506, 59–70. doi: 10.1007/978-1-59745-409-4_5
Sauce, D., Larsen, M., Fastenackels, S., Pauchard, M., Ait-Mohand, H., Schneider, L., et al. (2011). HIV disease progression despite suppression of viral replication is associated with exhaustion of lymphopoiesis. Blood 117, 5142–5151. doi: 10.1182/blood-2011-01-331306
Savkovic, B., Nichols, J., Birkett, D., Applegate, T., Ledger, S., Symonds, G., et al. (2014). A quantitative comparison of anti-HIV gene therapy delivered to hematopoietic stem cells versus CD4+ T cells. PLoS Comput. Biol. 10:e1003681. doi: 10.1371/journal.pcbi.1003681
Sebastian, N. T., Zaikos, T. D., Terry, V., Taschuk, F., Mcnamara, L. A., Onafuwa-Nuga, A., et al. (2017). CD4 is expressed on a heterogeneous subset of hematopoietic progenitors, which persistently harbor CXCR4 and CCR5-tropic HIV proviral genomes in vivo. PLoS Pathog. 13:e1006509. doi: 10.1371/journal.ppat.1006509
Stanley, S. K., Kessler, S. W., Justement, J. S., Schnittman, S. M., Greenhouse, J. J., Brown, C. C., et al. (1992). CD34+ bone marrow cells are infected with HIV in a subset of seropositive individuals. J. Immunol. 149, 689–697.
Starnes, L. M., Sorrentino, A., Ferracin, M., Negrini, M., Pelosi, E., Nervi, C., et al. (2010). A transcriptome-wide approach reveals the key contribution of NFI-A in promoting erythroid differentiation of human CD34(+) progenitors and CML cells. Leukemia 24, 1220–1223. doi: 10.1038/leu.2010.78
Strazza, M., Banerjee, A., Alexaki, A., Passic, S. R., Meucci, O., Pirrone, V., et al. (2014). Effect of mu-opioid agonist DAMGO on surface CXCR4 and HIV-1 replication in TF-1 human bone marrow progenitor cells. BMC Res. Notes 7:752. doi: 10.1186/1756-0500-7-752
Su, L., Kaneshima, H., Bonyhadi, M., Salimi, S., Kraft, D., Rabin, L., et al. (1995). HIV-1-induced thymocyte depletion is associated with indirect cytopathicity and infection of progenitor cells in vivo. Immunity 2, 25–36. doi: 10.1016/1074-7613(95)90076-4
Sundell, I. B., and Koka, P. S. (2006). Thrombocytopenia in HIV infection: impairment of platelet formation and loss correlates with increased c-Mpl and ligand thrombopoietin expression. Curr. HIV Res. 4, 107–116. doi: 10.2174/157016206775197646
Takabatake, Y., Sugiyama, T., Kohara, H., Matsusaka, T., Kurihara, H., Koni, P. A., et al. (2009). The CXCL12 (SDF-1)/CXCR4 axis is essential for the development of renal vasculature. J. Am. Soc. Nephrol. 20, 1714–1723. doi: 10.1681/ASN.2008060640
Takuva, S., Maskew, M., Brennan, A. T., Long, L., Sanne, I., and Fox, M. P. (2014). Poor CD4 recovery and risk of subsequent progression to AIDS or death despite viral suppression in a South African cohort. J. Int. AIDS Soc. 17:18651. doi: 10.7448/IAS.17.1.18651
Thiebot, H., Vaslin, B., Derdouch, S., Bertho, J. M., Mouthon, F., Prost, S., et al. (2005). Impact of bone marrow hematopoiesis failure on T-cell generation during pathogenic simian immunodeficiency virus infection in macaques. Blood 105, 2403–2409. doi: 10.1182/blood-2004-01-0025
Tsukamoto, T. (2018). Transcriptional gene silencing limits CXCR4-associated depletion of bone marrow CD34+ cells in HIV-1 infection. AIDS 32, 1737–1747. doi: 10.1097/QAD.0000000000001882
Tsukamoto, T. (2019a). Gene therapy approaches to functional cure and protection of hematopoietic potential in HIV infection. Pharmaceutics 11:114. doi: 10.3390/pharmaceutics11030114
Tsukamoto, T. (2019b). HIV Impacts CD34+ Progenitors involved in T-Cell differentiation during coculture with mouse stromal OP9-DL1 cells. Front. Immunol. 10:81. doi: 10.3389/fimmu.2019.00081
Tsukamoto, T., and Okada, S. (2017). The use of RetroNectin in studies requiring in vitro HIV-1 infection of human hematopoietic stem/progenitor cells. J. Virol. Methods 248, 234–237. doi: 10.1016/j.jviromet.2017.08.003
Vangelista, L., and Vento, S. (2017). The expanding therapeutic perspective of CCR5 blockade. Front. Immunol. 8:1981. doi: 10.3389/fimmu.2017.01981
Von Laer, D., Hufert, F. T., Fenner, T. E., Schwander, S., Dietrich, M., Schmitz, H., et al. (1990). CD34+ hematopoietic progenitor cells are not a major reservoir of the human immunodeficiency virus. Blood 76, 1281–1286. doi: 10.1182/blood.V76.7.1281.1281
Weichold, F. F., Zella, D., Barabitskaja, O., Maciejewski, J. P., Dunn, D. E., Sloand, E. M., et al. (1998). Neither human immunodeficiency virus-1 (HIV-1) nor HIV-2 infects most-primitive human hematopoietic stem cells as assessed in long-term bone marrow cultures. Blood 91, 907–915. doi: 10.1182/blood.V91.3.907
Weiss, R. A. (1996). HIV receptors and the pathogenesis of AIDS. Science 272, 1885–1886. doi: 10.1126/science.272.5270.1885
Winkler, C., Modi, W., Smith, M. W., Nelson, G. W., Wu, X., Carrington, M., et al. (1998). Genetic restriction of AIDS pathogenesis by an SDF-1 chemokine gene variant. ALIVE study, hemophilia growth and development study (HGDS), multicenter AIDS cohort study (MACS), multicenter hemophilia cohort study (MHCS), San Francisco City cohort (SFCC). Science 279, 389–393. doi: 10.1126/science.279.5349.389
Withers-Ward, E. S., Amado, R. G., Koka, P. S., Jamieson, B. D., Kaplan, A. H., Chen, I. S., et al. (1997). Transient renewal of thymopoiesis in HIV-infected human thymic implants following antiviral therapy. Nat. Med. 3, 1102–1109. doi: 10.1038/nm1097-1102
World Health Organization (2015). Guideline on When to Start Antiretroviral Therapy and on Pre-Exposure Prophylaxis for HIV. Geneva: WHO.
Ye, P., Kirschner, D. E., and Kourtis, A. P. (2004). The thymus during HIV disease: role in pathogenesis and in immune recovery. Curr. HIV Res. 2, 177–183. doi: 10.2174/1570162043484898
Zaikos, T. D., Terry, V. H., Sebastian Kettinger, N. T., Lubow, J., Painter, M. M., Virgilio, M. C., et al. (2018). Hematopoietic stem and progenitor cells are a distinct HIV reservoir that contributes to persistent viremia in suppressed patients. Cell Rep. 25, 3759–3773.e3759. doi: 10.1016/j.celrep.2018.11.104
Zauli, G., Furlini, G., Vitale, M., Re, M. C., Gibellini, D., Zamai, L., et al. (1994). A subset of human CD34+ hematopoietic progenitors express low levels of CD4, the high-affinity receptor for human immunodeficiency virus-type 1. Blood 84, 1896–1905. doi: 10.1182/blood.V84.6.1896.1896
Zhang, J. C., Sun, L., Nie, Q. H., Huang, C. X., Jia, Z. S., Wang, J. P., et al. (2009). Down-regulation of CXCR4 expression by SDF-KDEL in CD34(+) hematopoietic stem cells: an anti-human immunodeficiency virus strategy. J. Virol. Methods 161, 30–37. doi: 10.1016/j.jviromet.2009.05.009
Zhang, M., Evans, S., Yuan, J., Ratner, L., and Koka, P. S. (2010). HIV-1 determinants of thrombocytopenia at the stage of CD34+ progenitor cell differentiation in vivo lie in the viral envelope gp120 V3 loop region. Virology 401, 131–136. doi: 10.1016/j.virol.2010.03.005
Keywords: human immunodeficiency virus, acquired immunodeficiency syndrome, hematopoietic stem/progenitor cells, hematopoiesis, senescence
Citation: Tsukamoto T (2020) Hematopoietic Stem/Progenitor Cells and the Pathogenesis of HIV/AIDS. Front. Cell. Infect. Microbiol. 10:60. doi: 10.3389/fcimb.2020.00060
Received: 19 November 2019; Accepted: 06 February 2020;
Published: 21 February 2020.
Edited by:
Samuel K. Campos, The University of Arizona, United StatesReviewed by:
Julià Blanco, IrsiCaixa, SpainLarisa Y. Poluektova, University of Nebraska Medical Center, United States
Copyright © 2020 Tsukamoto. This is an open-access article distributed under the terms of the Creative Commons Attribution License (CC BY). The use, distribution or reproduction in other forums is permitted, provided the original author(s) and the copyright owner(s) are credited and that the original publication in this journal is cited, in accordance with accepted academic practice. No use, distribution or reproduction is permitted which does not comply with these terms.
*Correspondence: Tetsuo Tsukamoto, dHRzdWthbW90byYjeDAwMDQwO21lZC5raW5kYWkuYWMuanA=