- 1Department of Microbiology, Faculty of Medicine, Pamukkale University, Denizli, Turkey
- 2Department of Pharmaceutical Microbiology, Faculty of Pharmacy, University of Mersin, Mersin, Turkey
- 3Department of Molecular Genetics and Microbiology, Duke University Medical Center, Durham, NC, United States
- 4Infectious Disease and Microbiome Program, Broad Institute of MIT and Harvard, Cambridge, MA, United States
- 5Microbiology Service, Department of Laboratory Medicine, Clinical Center, National Institutes of Health, Bethesda, MD, United States
- 6Division of Mycology, Department of Microbiology, Faculty of Medicine, University of Çukurova, Adana, Turkey
Cryptococcus species are life-threatening human fungal pathogens that cause cryptococcal meningoencephalitis in both immunocompromised and healthy hosts. The natural environmental niches of Cryptococcus include pigeon (Columba livia) guano, soil, and a variety of tree species such as Eucalyptus camaldulensis, Ceratonia siliqua, Platanus orientalis, and Pinus spp. Genetic and genomic studies of extensive sample collections have provided insights into the population distribution and composition of different Cryptococcus species in geographic regions around the world. However, few such studies examined Cryptococcus in Turkey. We sampled 388 Olea europaea (olive) and 132 E. camaldulensis trees from seven locations in coastal and inland areas of the Aegean region of Anatolian Turkey in September 2016 to investigate the distribution and genetic diversity present in the natural Cryptococcus population. We isolated 84 Cryptococcus neoformans strains (83 MATα and 1 MATa) and 3 Cryptococcus deneoformans strains (all MATα) from 87 (22.4% of surveyed) O. europaea trees; a total of 32 C. neoformans strains were isolated from 32 (24.2%) of the E. camaldulensis trees, all of which were MATα. A statistically significant difference was observed in the frequency of C. neoformans isolation between coastal and inland areas (P < 0.05). Interestingly, the MATa C. neoformans isolate was fertile in laboratory crosses with VNI and VNB MATα tester strains and produced robust hyphae, basidia, and basidiospores, thus suggesting potential sexual reproduction in the natural population. Sequencing analyses of the URA5 gene identified at least five different genotypes among the isolates. Population genetics and genomic analyses revealed that most of the isolates in Turkey belong to the VNBII lineage of C. neoformans, which is predominantly found in southern Africa; these isolates are part of a distinct minor clade within VNBII that includes several isolates from Zambia and Brazil. Our study provides insights into the geographic distribution of different C. neoformans lineages in the Mediterranean region and highlights the need for wider geographic sampling to gain a better understanding of the natural habitats, migration, epidemiology, and evolution of this important human fungal pathogen.
Introduction
Cryptococcosis is a potentially lethal disease, especially in immunocompromised hosts, around the world. It is caused by environmental encapsulated yeasts belonging to the Cryptococcus genus, including the C. neoformans and C. gattii species complexes (Hagen et al., 2015; Kwon-Chung et al., 2017). C. neoformans has been mainly recovered from pigeon (Columba livia) droppings, urban environments, and soil (Lin and Heitman, 2006; May et al., 2016). In addition, it has been isolated from various tree species (Ellis and Pfeiffer, 1990; Randhawa et al., 2008, 2011; Cogliati et al., 2016a,b). Following the first report of C. gattii isolation from trees in Australia (Ellis and Pfeiffer, 1990), many studies have confirmed the environmental association of Cryptococcus with plants in different climatic zones (Granados and Castañeda, 2005, 2006; Randhawa et al., 2008, 2011; Bedi et al., 2012; Chowdhary et al., 2012). Several studies have characterized the properties of these yeasts that contribute to the colonization of new environmental niches (Granados and Castañeda, 2006; Randhawa et al., 2008; Ergin and Kaleli, 2010; Ergin et al., 2014; Sengul et al., 2019). With the exception of iatrogenic (Baddley et al., 2011) and zoonotic (Nosanchuk et al., 2000; Lagrou et al., 2005; Singh et al., 2018) cases, Cryptococcus infection is caused by the inhalation of airborne basidiospores or desiccated yeast cells from the environment (Hull et al., 2005; Lin and Heitman, 2006; Velagapudi et al., 2009; Springer et al., 2013; May et al., 2016), emphasizing the importance of identifying the natural reservoirs of C. neoformans and the molecular links between environmental and clinical isolates and their association with disease (Litvintseva et al., 2005; Chen et al., 2015; Kangogo et al., 2015; Noguera et al., 2015; Spina-Tensini et al., 2017). In a recent study, MLST analysis revealed that some C. neoformans genotypes (especially ST63) in Mediterranean countries may be genetically linked (Cogliati et al., 2019). In the environment, the most prevalent mating type is MATα (Kwon-Chung and Bennett, 1978).
Most areas colonized by C. neoformans are characterized by the presence of several trees, including 4 dominant species: Eucalyptus camaldulensis (Mahmoud, 1999; Bernardo et al., 2001; Campisi et al., 2003; Ergin et al., 2004; Mseddi et al., 2011; Romeo et al., 2011, 2012; Colom et al., 2012; Gokçen and Ergin, 2014; Cogliati et al., 2016a,b; Elhariri et al., 2016; Ellabib et al., 2016), Ceratonia siliqua (Colom et al., 2012; Romeo et al., 2012; Cogliati et al., 2016a), Olea europaea (Cogliati et al., 2016a; Ellabib et al., 2016), and Pinus spp. (Cogliati et al., 2016a,b). Further, studies have described numerous woody plants colonized by C. neoformans in the Mediterranean region (Mahmoud, 1999; Bernardo et al., 2001; Campisi et al., 2003; Ergin et al., 2004; Mseddi et al., 2011; Romeo et al., 2011, 2012; Colom et al., 2012; Gokçen and Ergin, 2014; Cogliati et al., 2016a,b; Elhariri et al., 2016; Ellabib et al., 2016). Cryptococcus neoformans tree colonization has been observed in northern Mediterranean countries such as Spain (Colom et al., 2012; Cogliati et al., 2016a), Portugal (Bernardo et al., 2001; Ferreira et al., 2014; Cogliati et al., 2016a), France (Cogliati et al., 2016a,b), Italy (Campisi et al., 2003; Romeo et al., 2011, 2012; Cogliati et al., 2016a,b), Greece (Cogliati et al., 2016a,b), and Turkey (Ergin et al., 2004; Ergin, 2010; Ergin and Kaleli, 2010; Gokçen and Ergin, 2014; Cogliati et al., 2016a,b; Sengul et al., 2019), as well as in the northern parts of Cyprus (Cogliati et al., 2016a), Libya (Cogliati et al., 2016a; Ellabib et al., 2016), Tunisia (Mseddi et al., 2011), and Egypt (Mahmoud, 1999; Elhariri et al., 2016). The olive tree is one of the oldest known cultivated trees in the world and is grown in the entire Mediterranean basin mostly for commercial reasons (Uylaşer and Yildiz, 2014). Although the Olea genus is distributed throughout Europe, Asia, Oceania, and Africa, only O. europaea is a cultivated species, and recent studies have reported colonization of O. europaea with C. neoformans in Spain (Cogliati et al., 2016a) and Libya (Ellabib et al., 2016).
In this study, we screened O. europaea and E. camaldulensis trees in southwestern Anatolia for Cryptococcus spp. The isolates we recovered were genotypically diverse, including both mating types. Additionally, whole genome sequencing and phylogenomic analyses showed that most of the isolates in Turkey belong to the VNB lineage of C. neoformans and are closely related to isolates from Zambia and Brazil. Our studies provide insight into the global distribution, epidemiology, and evolution of this important human fungal pathogen.
Materials and Methods
Study Areas
Samples were taken in September 2016 from seven areas along the Aegean coastal line of Anatolia, Turkey to screen O. europaea and E. camaldulensis trees for Cryptococcus spp. (Figure 1). Geospatial characteristics of each sampling area, including climate and geographical coordinates, are presented in Table 1. Sampling of E. camaldulensis (tree symbol in Figure 1) were conducted in southwestern Mediterranean coast of Turkey, near the village of Gokova, from trees which were previously shown to be colonized by C. neoformans (Ergin et al., 2004; Ergin, 2010). This area was sampled again to evaluate the current yeast colonization status and mating type distribution.
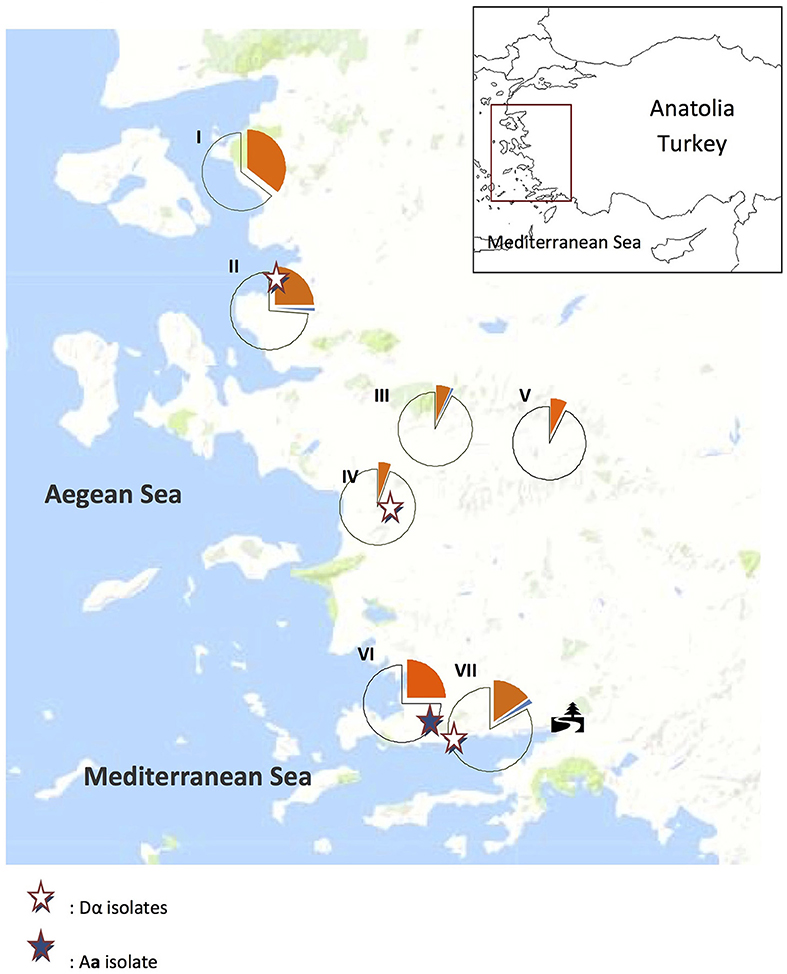
Figure 1. Distribution of trees with Cryptococcus neoformans (orange) and C. deneoformans (blue) colonization and uncolonized (unshaded) trees in Aegean Anatolia, Turkey. The tree symbol designates the region where C. neoformans is recurrently isolated from Eucalyptus camaldulensis.
Sampling, Culture, and Conventional Identification of C. neoformans
In total, 388 typical old O. europaea and 132 old E. camaldulensis trees from the Mediterranean part of Western Turkey were screened in the study. Tree trunks were randomly sampled by rubbing with a sterile cotton-tipped swab, as described by Randhawa et al. (2005). The swabs were soaked in 3 mL sterile saline containing chloramphenicol (10 mg/L) and transferred to the laboratory at 26°C within 48 h. After vortexing, swabs were removed, and samples were left to sediment for 30 min. Then, undiluted sample supernatants (100 μL) were used to inoculate Staib agar plates containing 0.5% (w/v) biphenyl. The plates were incubated at 26°C for 10 days, and moist, characteristically brown-pigmented colonies were analyzed for parameters conventionally used to identify C. neoformans, including urea hydrolysis, nitrate reduction, phenoloxidase production, growth at 37°C, and negative reaction on L-canavanine-glycine-bromothymol blue medium (Table S1).
Genomic DNA Extraction, Polymerase Chain Reaction (PCR) Amplification, and DNA Sequencing
All isolates were collected directly from yeast-peptone-dextrose (YPD; Difco, Detroit, MI) agar plates after the second passage. Genomic DNA was extracted using the MasterPure yeast DNA purification kit (Epicenter Biotechnologies, Madison, WI) according to the manufacturer's instructions.
The species identity and mating type of C. neoformans isolates were analyzed by PCR using primers specific for internal transcribed spacer (ITS) and STE20 genes, respectively (Table S2). PCR assays were conducted in a PTC-200 automated thermal cycler in a total reaction volume of 25 μL containing 300 ng of template DNA, 10 pM of each primer, 2 mM of each dNTP, 2.5 μL of 10× Ex Taq buffer, 0.25 μL of ExTaq polymerase (Takara, Shiga, Japan), and an appropriate volume of distilled water. The following cycling conditions were used for PCR with ITS1 and ITS4 primers: initial denaturation at 94°C for 5 min, followed by 36 cycles of denaturation at 94°C for 1 min, annealing at 57°C for 1 min, and extension at 72°C for 1 min, with a final extension at 72°C for 10 min. For PCR with Aa, Aα, and Dα mating-type primers, cycling conditions were as follows: 95°C for 6 min, followed by 36 cycles at 95°C for 45 s, 60°C for 45 s, and 72°C for 90 s, and a final extension step at 72°C for 6 min. For amplification using Da mating-type primers, the cycling protocol was as follows: 95°C for 6 min, followed by 30 cycles at 95°C for 45 s, 50°C for 45 s, and 72°C for 90 s, and a final extension step at 72°C for 6 min. Sterile water was used instead of DNA in negative control samples. Congenic C. neoformans strains H99 (VNI-Aα) and KN99a (Aa), and C. deneoformans strains JEC20 (VNIV-Da), and JEC21 (VNIV-Dα) were used as positive controls in each assay. PCR products were analyzed on 1% agarose gels.
The ORF of the URA5 gene was amplified using the following primers: 5′-GTGCCCGATAACATCTGCGA-3′ (forward) and 5′-TGGTATGTACTGGGGCAGTGA-3′ (reverse). PCR products were purified and sequenced as previously described (Sun et al., 2012, 2014). Alignment of the URA5 sequences was conducted using program ClustalX (Larkin et al., 2007).
To sequence the ITS region, amplified products were purified using the QIAquick PCR Purification Kit (Qiagen, Germantown, MD) as recommended by the manufacturer. Both DNA strands were sequenced using the BigDye Terminator v. 3.1 cycle sequencing ready reaction mix (Applied Biosystems, Foster City, CA) in an ABI 3130 automated sequencer (Applied Biosystems). Sequences were assembled using the Sequencher 4.8. software (Gene Code Corporation, Ann Arbor, MI).
Mating Assay
Each of the study strains were tested for the ability to mate and compared to the reference C. neoformans strains H99 (VNI-MATα) and KN99a (VNI-MATa) using mating assays as described previously (Sun et al., 2019). Mating abilities for the MATa and MATα strains derived from isolate AD215 (AD215-D1 and AD215-D2) were also tested in crosses between themselves, as well as with the tester strains H99 and Bt63 (VNBI-MATa). Mating assays were carried out at 25°C in the dark for 2 weeks (Idnurm and Heitman, 2005; Li et al., 2012). Hyphae and basidiospore formation were assessed by light microscopy every other day (Figure 2).
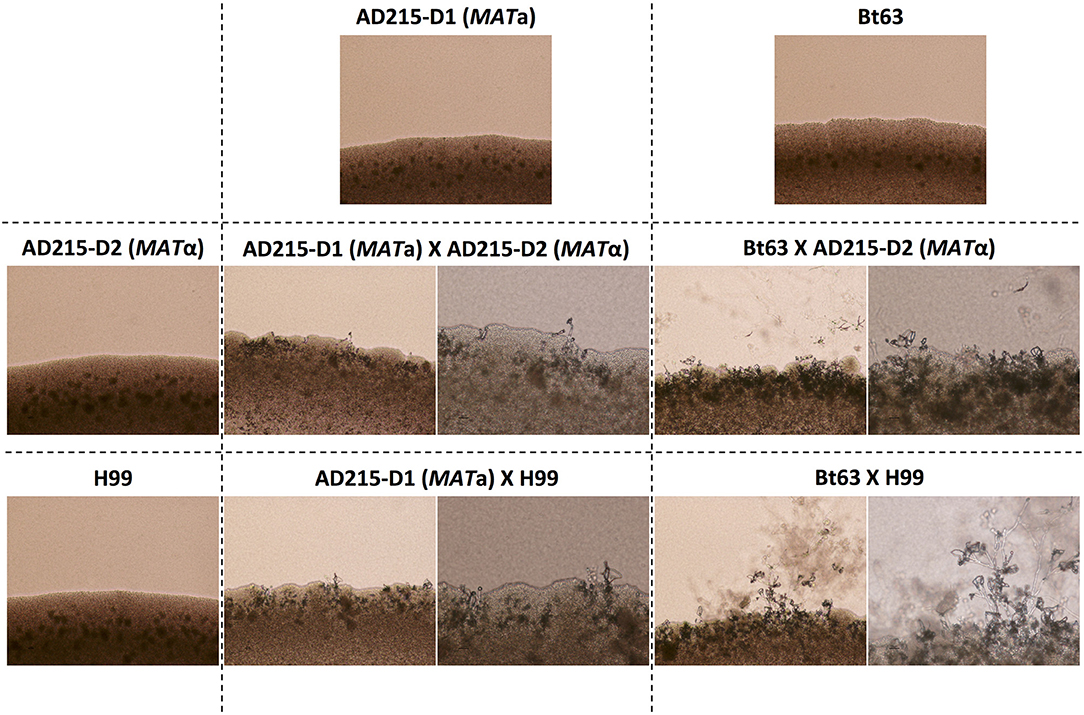
Figure 2. Successful mating between natural MATa and MATα isolates of Cryptococcus neoformans. Top: Images of solo cultures of a MATa colony of strain AD215-D1 and MATa tester strain Bt63; left: images of solo cultures of a MATα colony from strain AD215-D2 and MATα tester strain H99. All images of solo cultures were taken with 10× magnification. Images of pairwise mating between MATa and MATα strains are shown within the 2×2 grid: left, 10× magnification; right, 20× magnification. All crosses were carried out on MS medium.
Genome Sequencing and Phylogenomic Analyses
Whole genome sequences were generated for 9 isolates (AD116, AD119, AD129, AD130, AD131, AD132, AD161, AD215-D1, and AD215-D2). Whole genome sequencing libraries were constructed using the Illumina Nextera XT protocol and sequenced on a HiSeqX, generating 150 base-paired end reads (accessible in the NCBI SRA under BioProject PRJNA533587). For SNP calling, reads were downsampled to ~130X sequence depth using Samtools view. Data from a large population survey of 387 isolates (Desjardins et al., 2017), from a Zambian collection (Vanhove et al., 2017) and of VNB isolates from Brazil (Rhodes et al., 2017) were also included. For this set of 446 isolates, reads for each isolate were aligned to the C. neoformans H99 assembly (GenBank accession GCA_000149245.2) using BWA-MEM version 0.7.12 (Li, 2013). Variants were then identified using GATK version 3.4 (McKenna et al., 2010). Briefly, indels were locally realigned, haplotypeCaller was invoked in GVCF mode with ploidy = 1, and genotypeGVCFs was used to predict variants in each strain. All VCFs were then combined and sites were filtered using variant filtration with QD < 2.0, FS > 60.0, and MQ < 40.0. Individual genotypes were filtered if the minimum genotype quality was <50, percent alternate allele was <0.8, or depth was <10.
For phylogenetic analysis, the 1,269,132 sites with an unambiguous SNP in at least one strain and ambiguity in ≤10% of strains were concatenated, and insertions or deletions at these sites were treated as ambiguous to maintain the alignment. Phylogenetic trees were estimated using FastTreeDP v 2.1.8 with parameters -gtr and -nt.
Statistical Analysis
Data were analyzed by Chi-square test using the Epi InfoTM Stat Calc software (v. 7.2.1.0; Centers for Disease Control and Prevention, Atlanta, GA). A 2-tailed P ≤ 0.05 was considered to indicate statistical significance.
Results
Environmental Parameters Characterizing the Sampling Regions
In the current study, samples were collected from seven geographic locations in Anatolia, including four coastal regions (I, II, VI, and VII in Figure 1), and three inland areas (III, IV, and V in Figure 1). During the sampling period (September 2016), the mean temperature and humidity were higher in the coastal than in the inland regions (25.3°C vs. 23.5°C and 5.5 vs. 5.0 mm, respectively).
All seven sampling regions are located within the natural propagation area of O. europea (Uylaşer and Yildiz, 2014) with the typical “macchia” vegetation within the Mediterranean climate (Colom et al., 2012).
Both Mating Types Are Present in Natural C. neoformans Isolates From Turkey
In the Aegean region of Anatolia, C. neoformans (n = 84) and C. deneoformans (n = 3) were isolated from 22.4% (87/388) of sampled O. europae trees. Among them, 95.4% (83/87), 1.1% (1/87), and 3.5% (3/87), were identified as serotypes A MATα, A MATa, and D MATα, respectively (Figure 3 and Table 2), whereas strains of serotype D MATa were not identified. This corresponded to a significantly higher frequency of C. neoformans strains isolated from the beach/coastal regions (75/221) compared to inland areas located ≥10 km from the sea (12/167) (P < 0.001; Table 3).
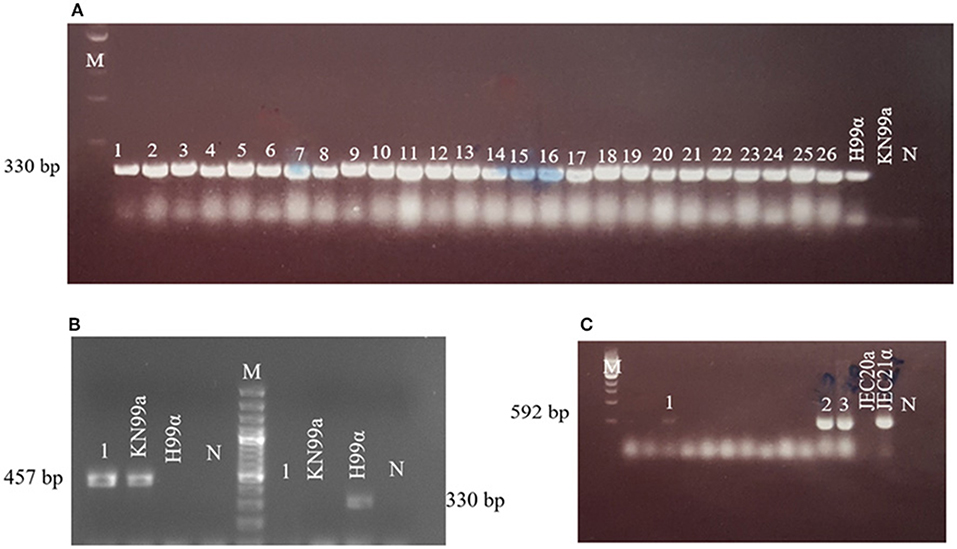
Figure 3. Mating type profiles obtained by PCR using STE20 gene-specific primers. (A) 1–26: serotype Aα, H99α, KN99a; (B) 1: serotype Aa (strain AD215), KN99a, H99α; (C) 1–3: serotype Dα, JEC20a, JEC21α. Congenic C. neoformans strains H99 (VNI-αA) and KN99a (aA), and C. deneoformans JEC20 (VNIV-aD) and JEC21 (VNIV-αD) were used as positive controls. M, molecular weight markers; N, negative control.
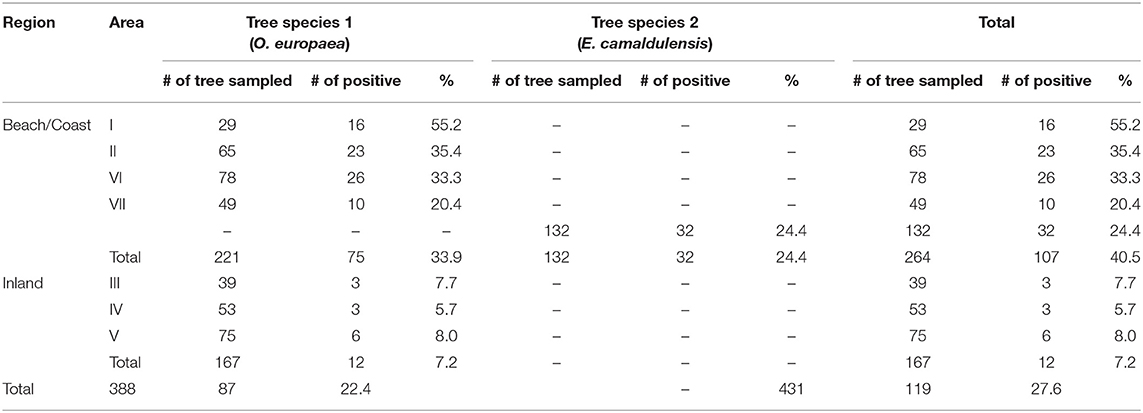
Table 3. Geographical distribution of Olea europaea and Eucalyptus camaldulensis colonized with Cryptococcus neoformans.
The single MATa type C. neoformans strain (AD215) isolated from area VII showed no sign of mating when crossed with a MATa tester strain (KN99a), but did show signs of robust sexual reproduction when crossed with the MATα tester strain (H99) (Figure 2), phenotypically confirming that this isolate is a true MATa C. neoformans isolate.
Thirty-two of 132 (24.2%) isolates have been isolated from E. camaldulensis trees, all of which were identified as MATα C. neoformans.
Natural C. neoformans Isolates in Turkey Are Genetically Diverse (URA5)
Because the genotyping of the MAT locus showed that the vast majority of the isolates in this study are MATα, we further investigated how much genetic diversity is present within the Turkish Cryptococcus isolates. We randomly picked 41 strains and PCR amplified and Sanger sequenced the URA5 locus, which in previous studies has been suggested to be more genetically diverse among natural Cryptococcus strains and has power to differentiate among different lineages of C. neoformans. Our sequencing analyses identified 5 URA5 alleles in the 41 isolates (Figure S1), including 2 major genotypes represented by 14 and 18 isolates, respectively, 1 genotype represent by 7 isolates, and 2 unique genotypes each represented by 1 isolate. Thus, ample genetic diversity is present among the natural isolates in Turkey.
Natural C. neoformans Isolates in Turkey Are Closely Related to Those From Brazil and Zambia
To investigate how isolates from Turkey compare to other global C. neoformans isolates, we carried out whole genome sequencing for 7 isolates that represent the 5 URA5 genotypes (Figure S1), as well as the MATa (AD215-D1) and MATα (AD215-D2) strains derived from isolate AD-215, and then compared their genome sequences with those of the global C. neoformans sequences that have been recently published (Desjardins et al., 2017; Vanhove et al., 2017) based on variants identified compared to the H99 reference genome.
URA5 sequences extracted from the variant calls of these isolates are in overall agreement with the URA5 genotyping results described above. Phylogenetic analysis suggested that three isolates are VNI (AD119, AD129, and AD130), while four isolates (AD116, AD131, AD132, and AD161) and the two strains derived from AD215 (AD215-D1 and AD215-D2) are VNB. The three VNI isolates (AD119, AD129, and AD130) were placed within the VNIb subclade of global isolates (Figure S2), and all VNB isolates were placed within the VNBII clade, which primarily includes South African isolates (Figure 4). Of the VNBII isolates from Turkey, AD116 belongs to a clade that contains mostly isolates from Botswana, South Africa, and Zambia, while the others are more divergent from most VNBII isolates and are closely related to two isolates from Brazil and three isolates from Zambia (Figure 4).
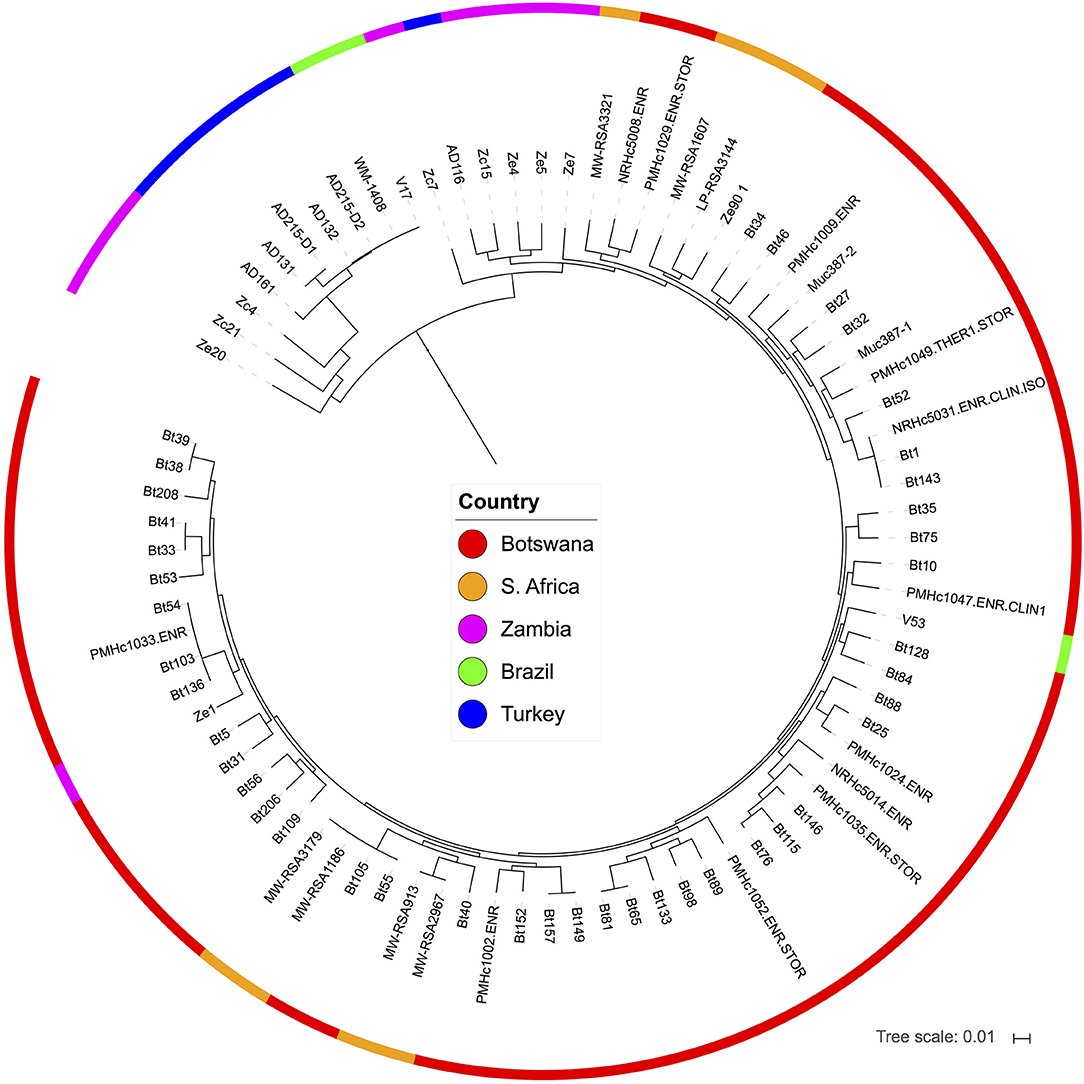
Figure 4. Phylogenic analyses places isolates from Turkey in the VNBII lineage of C. neoformans. Isolates from Turkey are part of a divergent subclade that included isolates from Brazil and Zambia. The phylogeny was estimated from 1,269,132 segregating sites using FastTree (Price et al., 2009), and the tree was rooted with VNII as the out-group, see Figure S2 for full phylogeny.
Interestingly, two isolates from Turkey (AD132 and AD215-D2) are separated by only an average of 590 SNPs from two isolates from Brazil (an environmental isolate, WM-1408, and a clinical isolate, V17; Figure 4). This number of SNPs does not suggest a recent transmission event between these locations, but rather a closer than expected relationship across continents in a lineage formerly thought to be confined to southern Africa.
Discussion
The present study revealed that olive trees are a major reservoir of environmental C. neoformans strains in the Aegean part of the Mediterranean region, where cultivation of olive trees is a tradition (Uylaşer and Yildiz, 2014). This is consistent with previous studies suggesting that olive trees are one of the major reservoirs of Cryptococcus species (Cogliati et al., 2016a; Ellabib et al., 2016, 2017; Montagna et al., 2018). We sampled only old tree trunks with hollows that constitute an appropriate yeast habitat, providing stable humidity and temperature and protection from solar radiation (Lin and Heitman, 2006; Velagapudi et al., 2009; May et al., 2016). Compared to inland regions, the colonization of olive trees by C. neoformans is more prominent in the coastal areas, which are less arid, are at a lower altitude, and have milder winters. Throughout the study period (September 2016), the mean temperatures and precipitation in the coastal areas were higher than those in the inland areas, and accordingly, the largest number of C. neoformans isolates was obtained from area I, which had the highest temperature and precipitation (Tables 1, 3; P < 0.01). Previous reports from the Mediterranean area (Cogliati et al., 2016a) and Libya (Ellabib et al., 2016) showed O. europea colonization rates of 9.1 and 1.4%, respectively. The results of this study were higher at 22.4%.
In the current study, C. deneoformans was obtained from areas II, IV, and VII. In 2008, C. deneoformans was isolated mainly from pigeon droppings in the Aegean region (Karaca Derici and Tumbay, 2008), which corresponds to area II in the current study. However, clinical cases of C. deneoformans were documented in the Black Sea coastal area (Kaya et al., 2012; Birinci et al., 2016), which is distant from the Aegean coast and where the climate is more humid and the temperature lower. This finding is consistent with reports that C. deneoformans is more sensitive to heat than C. neoformans (Martinez et al., 2001; Lin and Heitman, 2006; Bedi et al., 2012). Molecular type VNIV (serotype AD diploid hybrid) has been reported in the northern part of the Aegean region, where temperatures are lower (Cogliati et al., 2016a,b). Three reports using a MaxEnt model for the prediction of the Cryptococcus species showed that C. neoformans strains may have spread mostly in the southern and western part of Turkey (Cogliati et al., 2017; Acheson et al., 2018; Ergin et al., 2019). The discrepancy between the environmental presence of C. neoformans and related clinical cases should be addressed by further studies screening different areas in Turkey. The northern and southern areas of the Turkish Mediterranean coast have different climatic conditions and, consequently, distinct tree populations, which may potentially influence the rate of cryptococcal colonization.
The first isolation of C. neoformans from E. camaldulensis in Anatolia was reported in 2004 (Ergin et al., 2004). A previous study investigated the presence of C. neoformans in the flowers of E. camaldulensis trees from the Akyaka/Gökova (tree symbol, Figure 1) districts in southwestern Turkey and identified only 1 C. neoformans isolate (0.09%) (Ergin et al., 2004). In 2010, repeated screening of 17 E. camaldulensis trees with large trunks was performed in the same region, and a colonization rate of 64% was reported (Ergin, 2010). In this study, we had only found 24.2% colonization rate from 132 sampled E. camaldulensis trees in the same region which is probably more representative due to the larger screening area. Compared with previous reports form Mediterranean area (Cogliati et al., 2016a) and Libya (Ellabib et al., 2016) about colonization rate (48/527; 9.1% and 3/210; 1.4%, respectively) on O. europaea, our result is higher (87/388; 22.4%, Table 3).
Trees play an essential role as a reservoir and breeding ground for the propagation of C. neoformans. A recent study showed that Cryptococcus has the ability to colonize some plant such as E. camaldulensis, Terminalia catappa, Arabidopsis thaliana, Colophospermum mopane, Tsuga heterophylla, and Pseudotsuga menziesii, as well as their debris, which constitutes the ecological niche and reservoirs of infectious propagules of Cryptococcus in the environment (Springer et al., 2017). Climate conditions, including humidity, temperature, evaporation, and solar radiation, play significant roles in the environmental distribution of C. neoformans (Lin and Heitman, 2006; Velagapudi et al., 2009; Springer et al., 2013; May et al., 2016). Our findings indicate that C. neoformans colonization of olive trees reflects the Mediterranean ecological model influenced by climate changes and urbanization (García-Mozo et al., 2016). Several studies reported the presence of environmental C. neoformans in Mediterranean countries, including Spain (Colom et al., 2012; Cogliati et al., 2016a) and Libya (Ellabib et al., 2016), based on the association between the climate and yeast distribution. Warmer temperatures can affect Cryptococcus spp. spread, especially that of C. gattii, a sibling species of C. neoformans (Granados and Castañeda, 2006; Randhawa et al., 2011; Bedi et al., 2012; Chowdhary et al., 2012; Uejio et al., 2015; Cogliati et al., 2016b). Although it is seen that the olive trees practiced in the Mediterranean “macchia” are more interrelated with the C. gattii, C. neoformans colonization is not far from the ecosystem (Cogliati et al., 2017). The duration of the dry season, natural degradation of woods, and drier habitats account for lower bacterial presence and less competition for nutrients, thus constituting favorable conditions for C. neoformans colonization, especially of old tree trunks (Ruiz et al., 1981; Granados and Castañeda, 2006; Cogliati et al., 2017).
Worldwide screening for the presence of C. neoformans in the environment has been performed since the mid−1990s, and E. camaldulensis was established as the major source of tree-associated cryptococcosis in the early 2000s (Ellis and Pfeiffer, 1990; Campisi et al., 2003; Ergin et al., 2004; Lin and Heitman, 2006; Randhawa et al., 2008; Noguera et al., 2015). Several studies reported that the majority of C. neoformans environmental isolates contain MATα mating-type alleles (Litvintseva et al., 2005; Nielsen et al., 2005; Lin and Heitman, 2006; Chen et al., 2015; Kangogo et al., 2015), which is consistent with our findings that Cryptococcus isolates from E. camaldulensis have the MATα phenotype. However, in O. europaea, we isolated C. neoformans harboring Dα and even Aa alleles. While MATa Cryptococcus strains have been previously isolated from trees in the Mediterranean regions such as the Mediterranean coast of Spain and Southern Italy (Viviani et al., 2001; Cogliati et al., 2016b), to the best of our knowledge, it is the first MATa-containing serotype A strain isolated from the environment in Anatolia. A previous study performed by Saracli et al. (2006) in Anatolia identified C. neoformans with mating types Aα (65.4%), Da (15.4%), and Dα (11.5%), but not Aa, in pigeon droppings. Although MATα predominates in clinical and environmental populations, MATa C. neoformans isolates have also been isolated in a number of studies from a variety of geographic areas (Lengeler et al., 2000; Viviani et al., 2001, 2003; Keller et al., 2003; Nielsen et al., 2003; Cogliati et al., 2006; Litvintseva et al., 2007, 2011). Population studies suggest sexual reproduction, including inter-lineage introgression is ongoing in natural Cryptococcus populations (Desjardins et al., 2017; Rhodes et al., 2017). In the present study, isolation of a novel serotype A MATa strain from the environment suggests that a-α sexual reproduction might occur in the serotype A population. However, whole genome analyses of additional isolates is required to detect recombination signatures in the Turkish Cryptococcus population.
Cryptococcus isolates in Turkey are genetically diverse. Based on our URA5 genotyping and whole genome sequencing analyses, most isolates from Turkey belong to the VNI and VNBII groups. Specifically, of the 41 isolates that were genotyped for the URA5 locus, 21 likely belong to the VNI group that includes isolates AD119, AD129, and AD130, while the other 20 belong to the VNBII group that includes isolates AD116, AD131, AD132, and AD161. Interestingly, of the VNBII isolates, only 1 (AD116) belongs to the larger sublineage that contains most VNBII isolates from Botswana, South Africa, and Zambia, while the other 19 have URA5 alleles identical to isolates AD131, AD132, and AD161, which, based on whole genome sequence analyses, belong to within the smaller VNBII sublineage that includes strains from Botswana and Brazil. Two strains from Brazil (V17 and WM-1408) may have contributed significant genetic material to the other lineages (VNI, VNII, and VNB) through recombination, with V17 donating the most genetic material to VNI isolates in Africa, India, and Thailand (Rhodes et al., 2017). The isolation of Turkish isolates, including the MATa and MATα fertile strains derived from isolate AD215, that are almost genetically identical to V17 and WM-1408, suggests that it is possible that the Mediterranean region could be a fertile ground for genetically diverse Cryptococcus isolates and could serve as an important center for the global migration and distribution of Cryptococcus isolates. Further WGS analyses of additional natural isolates will provide a more complete picture of the genetic diversity and phylogeny of natural isolates in Turkey and its surrounding Mediterranean area.
In conclusion, our results indicate that compared to C. deneoformans, C. neoformans is more common on olive trees and E. camaldulensis in the Aegean region of Anatolia. While the vast majority of the natural isolates in Turkey are mating type α, the presence of a fertile MATa isolate suggests that sexual reproduction could be ongoing in natural C. neoformans populations. Our finding that C. neoformans isolates from Turkey belong to VNBII and are more closely related to strains from Zambia and Brazil provides insights into the global distribution of C. neoformans and emphasizes the need for more extensive environmental screening to reveal new reservoirs for C. neoformans, which would promote our understanding of the natural distribution, epidemiology, and evolution of this important human fungal pathogen.
Data Availability Statement
The data analyzed in this study are accessible at NCBI SRA under BioProject PRJNA533587.
Author Contributions
ÇE, AD, SSu, CC, JH, and MI conceived and designed the experiments and analyzed the data. ÇE, MŞ, LA, AD, SSu, AA, and CC performed the experiments. ÇE, AD, SSu, CC, and JH contributed reagents, materials, and analysis tools. ÇE, MŞ, LA, AD, SSu, AA, CC, SSe, JH, and MI drafted and revised the manuscript. All authors have read, revised, and approved the final manuscript.
Funding
This work was supported by NIH/NIAID R37 MERIT Award AI39115-21 and NIH/NIAID R01 AI50113-15 to JH. CC was supported by NIH/NIAID grant number U19AI110818. SSe was supported by the Intramural Research Program of the National Institutes of Health, Clinical Center, Department of Laboratory Medicine.
Conflict of Interest
The authors declare that the research was conducted in the absence of any commercial or financial relationships that could be construed as a potential conflict of interest.
Acknowledgments
The authors are grateful to the members of the Heitman laboratory at the Duke University Department of Molecular Genetics and Microbiology (Durham, NC, USA) for their valuable assistance with laboratory analyses.
Supplementary Material
The Supplementary Material for this article can be found online at: https://www.frontiersin.org/articles/10.3389/fcimb.2019.00384/full#supplementary-material
Figure S1. Genotyping of the URA5 locus revealed that the isolates from Turkey are genetically diverse.
Figure S2. Phylogenetic analysis of isolates from Turkey with a diverse set of other global isolates. The full phylogeny estimated for Figure 4 is shown, including VNI, VNII, VNBI, and VNBII lineages. The phylogeny was estimated from 1,269,132 segregating sites using FastTree (Price et al., 2009), and the tree was rooted with VNII as the out-group. Isolates from Turkey were placed with the VNIb sublineage of VNI and in VNBII.
Table S1. Cryptococcus isolates recovered and analyzed in this study.
Table S2. Primers used in this study.
References
Acheson, E. S., Galanis, E., Bartlett, K., and Klinkenberg, B. (2018). Searching for clues for eighteen years: deciphering the ecological determinants of Cryptococcus gattii on Vancouver Island, British Columbia. Med. Mycol. 56, 129–144. doi: 10.1093/mmy/myx037
Baddley, J. W., Schain, D. C., Gupte, A. A., Lodhi, S. A., Kayler, L. K., Frade, J. P., et al. (2011). Transmission of Cryptococcus neoformans by organ transplantation. Clin. Infect. Dis. 52, e94–98. doi: 10.1093/cid/ciq216
Bedi, N. G., Nawange, S. R., Singh, S. M., Naidu, J., and Kavishwar, A. (2012). Seasonal prevalence of Cryptococcus neoformans var. grubii and Cryptococcus gattii inhabiting Eucalyptus terreticornis and Eucalyptus camaldulensis trees in Jabalpur City of Madhya Pradesh, Central India. J. Mycol. Med. 22, 341–347. doi: 10.1016/j.mycmed.2012.09.001
Bernardo, F. M., Martins, H. M., and Martins, M. L. (2001). Urban sources of Cryptococcus spp. – Lisbon (Portugal). Rev. Port. Cién. Vet. 96, 157–160.
Birinci, A., Tanriverdi-Cayci, Y., and Alakbarova, G. (2016). Cryptococcosis cases in a university hospital. Turk. Mikrobiyol. Cem. Derg. 46, 188–190. doi: 10.5222/TMCD.2016.188
Campisi, E., Mancianti, F., Pini, G., Faggi, E., and Gargani, G. (2003). Investigation in Central Italy of the possible association between Cryptococcus neoformans var. gattii and Eucalyptus camaldulensis. Eur. J. Epidemiol. 18, 357–362. doi: 10.1023/A:1023652920595
Chen, Y., Litvintseva, A. P., Frazzitta, A. E., Haverkamp, M. R., Wang, L., Fang, C., et al. (2015). Comparative analyses of clinical and environmental populations of Cryptococcus neoformans in Botswana. Mol. Ecol. 24, 3559–3571. doi: 10.1111/mec.13260
Chowdhary, A., Randhawa, H. S., Boekhout, T., Hagen, F., Klaassen, C. H., and Meis, J. F. (2012). Temperate climate niche for Cryptococcus gattii in Northern Europe. Emerg. Infect. Dis. 18, 172–174. doi: 10.3201/eid1801.111190
Cogliati, M., D'Amicis, R., Zani, A., Montagna, M. T., Caggiano, G., De Giglio, O., et al. (2016a). Environmental distribution of Cryptococcus neoformans and C. gattii around the Mediterranean basin. FEMS. Yeast. Res. 16:fow045. doi: 10.1093/femsyr/fow045
Cogliati, M., Desnos-Ollivier, M., McCormick-Smith, I., Rickerts, V., Ferreira-Paim, K., Meyer, W., et al. (2019). Genotypes and population genetics of Cryptococcus neoformans and Cryptococcus gattii species complexes in Europe and the mediterranean area. Fungal. Genet. Biol. 129, 16–29. doi: 10.1016/j.fgb.2019.04.001
Cogliati, M., Esposto, M. C., Tortorano, A. M., and Viviani, M. A. (2006). Cryptococcus neoformans population includes hybrid strains homozygous at mating-type locus. FEMS. Yeast. Res. 6, 608–613. doi: 10.1111/j.1567-1364.2006.00085.x
Cogliati, M., Puccianti, E., Montagna, M. T., De Donno, A., Susever, S., Ergin, C., et al. (2017). Fundamental niche prediction of the pathogenic yeasts Cryptococcus neoformans and Cryptococcus gattii in Europe. Environ. Microbiol. 19, 4318–4325. doi: 10.1111/1462-2920.13915
Cogliati, M., Zani, A., Rickerts, V., McCormick, I., Desnos-Ollivier, M., Velegraki, A., et al. (2016b). Multilocus sequence typing analysis reveals that Cryptococcus neoformans var. neoformans is a recombinant population. Fungal. Genet. Biol. 87, 22–29. doi: 10.1016/j.fgb.2016.01.003
Colom, M. F., Hagen, F., Gonzalez, A., Mellado, A., Morera, N., Linares, C., et al. (2012). Ceratonia siliqua (carob) trees as natural habitat and source of infection by Cryptococcus gattii in the Mediterranean environment. Med. Mycol. 50, 67–73. doi: 10.3109/13693786.2011.574239
Desjardins, C. A., Giamberardino, C., Sykes, S. M., Yu, C. H., Tenor, J. L., Chen, Y., et al. (2017). Population genomics and the evolution of virulence in the fungal pathogen Cryptococcus neoformans. Genome. Res. 27, 1207–1219. doi: 10.1101/gr.218727.116
Elhariri, M., Hamza, D., Elhelw, R., and Refai, M. (2016). Eucalyptus tree: a potential source of Cryptococcus neoformans in Egyptian environment. Int. J. Microbiol. 2016:4080725. doi: 10.1155/2016/4080725
Ellabib, M. S., Aboshkiwa, M. A., Husien, W. M., D'Amicis, R., and Cogliati, M. (2016). Isolation, identification and molecular typing of Cryptococcus neoformans from pigeon droppings and other environmental sources in Tripoli, Libya. Mycopathologia 181, 603–608. doi: 10.1007/s11046-016-9996-4
Ellabib, M. S., Krema, Z. A., Allafi, A. A., and Cogliati, M. (2017). First report of two cases of cryptococcosis in Tripoli, Libya, infected with Cryptococcus neoformans isolates present in the urban area. J. Mycol. Med. 27, 421–424. doi: 10.1016/j.mycmed.2017.04.104
Ellis, D. H., and Pfeiffer, T. J. (1990). Natural habitat of Cryptococcus neoformans var. gattii. J. Clin. Microbiol. 28, 1642–1644.
Ergin, C. (2010). High environmental isolation rate of Cryptococcus neoformans by swabbing technique in Gökova region. Turk. Mikrobiyol. Cem. Derg. 40, 163–168. doi: 10.1002/yea.1112
Ergin, C., Ilkit, M., Hilmioglu, S., Kaleli, I., Gulbaba, A. G., Demirci, M., et al. (2004). The first isolation of Cryptococcus neoformans from Eucalyptus trees in south aegean and mediterranean regions of anatolia in turkey despite taurus mountains alkalinity. Mycopathologia 158, 43–47. doi: 10.1023/B:MYCO.0000038431.72591.7e
Ergin, C., and Kaleli, I. (2010). Isolation of Cryptococcus neoformans from living tree trunk hollows in Denizli city center, Turkey. Mikrobiyol. Bul. 44, 79–85.
Ergin, C., Sengul, M., Dogen, A., and Ilkit, M. (2019). Prediction of the species distribution of Cryptococcus neoformans throughout Turkey. Mikrobiyol. Bul. 53, 233–238. doi: 10.5578/mb.67999
Ergin, C., Sengul, M., and Kiris-Satilmis, O. (2014). Colonization survey of Cryptococcus neoformans in Tamarix tree (Genus: Tamarix L.) in South-Western Anatolia, Turkey. Turk. Mikrobiyol. Cem. Derg. 44, 158–162. doi: 10.5222/TMCD.2014.158
Ferreira, A. S., Sampaio, A., Maduro, A. P., Silva, I., Teles, F., Martins Mda, L., et al. (2014). Genotypic diversity of environmental Cryptococcus neoformans isolates from Northern Portugal. Mycoses 57, 98–104. doi: 10.1111/myc.12106
García-Mozo, H., Oteros, J. A., and Galán, C. (2016). Impact of land cover changes and climate on the main airborne pollen types in Southern Spain. Sci. Total. Environ. 548–549, 221–228. doi: 10.1016/j.scitotenv.2016.01.005
Gokçen, H., and Ergin, C. (2014). The isolation of Cryptococcus neoformans from Eucalyptus camaldulensis trees in Mugla-Milas city center. Pam. Tip. Derg. 7, 109–112. doi: 10.5505/ptd.2014.71676
Granados, D. P., and Castañeda, E. (2005). Isolation and characterization of Cryptococcus neoformans varieties recovered from natural sources in Bogotá, Colombia, and study of ecological conditions in the area. Microb. Ecol. 49, 282–290. doi: 10.1007/s00248-004-0236-y
Granados, D. P., and Castañeda, E. (2006). Influence of climatic conditions on the isolation of members of the Cryptococcus neoformans species complex from trees in Colombia from 1992–2004. FEMS. Yeast. Res. 6, 636–644. doi: 10.1111/j.1567-1364.2006.00090.x
Hagen, F., Khayhan, K., Theelen, B., Kolecka, A., Polacheck, I., Sionov, E., et al. (2015). Recognition of seven species in the Cryptococcus gattii/Cryptococcus neoformans species complex. Fungal. Genet. Biol. 78, 16–48. doi: 10.1016/j.fgb.2015.02.009
Hull, C. M., Boily, M. J., and Heitman, J. (2005). Sex-specific homeodomain proteins SXI1α and SXI2a coordinately regulate sexual development in Cryptococcus neoformans. Eukaryot. Cell. 4, 526–535. doi: 10.1128/EC.4.3.526-535.2005
Idnurm, A., and Heitman, J. (2005). Light controls growth and development via a conserved pathway in the fungal kingdom. PLoS Biol. 3:e95. doi: 10.1371/journal.pbio.0030095
Kangogo, M., Bader, O., Boga, H., Wanyoike, W., Folba, C., Worasilchai, N., et al. (2015). Molecular types of Cryptococcus gattii/Cryptococcus neoformans species complex from clinical and environmental sources in Nairobi, Kenya. Mycoses 58, 665–670. doi: 10.1111/myc.12411
Karaca Derici, Y., and Tumbay, E. (2008). Variety and serotypes of Cryptococcus neoformans strains isolated from clinical specimens and environmental sources in Izmir, Turkey. Turk. J. Infect. 22, 53–58.
Kaya, S., Koksal, I., Tosun, I., Sari, A., and Alioglu, Z. (2012). Cryptococcal meningitis with accompanying recurrent cerebellitis in an immunocompetent patient. Med. Mycol. Case. Rep. 1, 127–129. doi: 10.1016/j.mmcr.2012.10.006
Keller, S. M., Viviani, M. A., Esposto, M. C., Cogliati, M., and Wickes, B. L. (2003). Molecular and genetic characterization of a serotype A MATa Cryptococcus neoformans isolate. Microbiology 149, 131–142. doi: 10.1099/mic.0.25921-0
Kwon-Chung, K. J., and Bennett, J. E. (1978). Distribution of α and α mating types of Cryptococcus neoformans among natural and clinical isolates. Am. J. Epidemiol. 108, 337–340. doi: 10.1093/oxfordjournals.aje.a112628
Kwon-Chung, K. J., Bennett, J. E., Wickes, B. L., Meyer, W., Cuomo, C. A., Wollenburg, K. R., et al. (2017). The case for adopting the “species complex” nomenclature for the etiologic agents of cryptococcosis. mSphere 2, e00357–e00316. doi: 10.1128/mSphere.00357-16
Lagrou, K., van Eldere, J., Keuleers, S., Hagen, F., Merckx, R., Verhaegen, J., et al. (2005). Zoonotic transmission of Cryptococcus neoformans from a magpie to an immunocompetent patient. J. Intern. Med. 257, 385–388. doi: 10.1111/j.1365-2796.2005.01466.x
Larkin, M. A., Blackshields, G., Brown, N. P., Chenna, R., McGettigan, P. A., McWilliam, H., et al. (2007). Clustal W and Clustal X version 2.0. Bioinformatics. 23, 2947–2948. doi: 10.1093/bioinformatics/btm404
Lengeler, K. B., Wang, P., Cox, G. M., Perfect, J. R., and Heitman, J. (2000). Identification of the MATa mating-type locus of Cryptococcus neoformans reveals a serotype A MATa strain thought to have been extinct. Proc. Natl. Acad. Sci. U.S.A. 97, 14455–14460. doi: 10.1073/pnas.97.26.14455
Li, H. (2013). Aligning sequence reads, clone sequences and assembly contigs with BWA-MEM. arXiv:1303.3997.
Li, W., Averette, A. F., Desnos-Ollivier, M., Ni, M., Dromer, F., and Heitman, J. (2012). Genetic diversity and genomic plasticity of Cryptococcus neoformans AD hybrid strains. Gene Genome Genet. 2, 83–97. doi: 10.1534/g3.111.001255
Lin, X., and Heitman, J. (2006). The biology of the Cryptococcus neoformans species complex. Annu. Rev. Microbiol. 60, 69–105. doi: 10.1146/annurev.micro.60.080805.142102
Litvintseva, A. P., Carbone, I., Rossouw, J., Thakur, R., Govender, N. P., and Mitchell, T. G. (2011). Evidence that the human pathogenic fungus Cryptococcus neoformans var. grubii may have evolved in Africa. PLoS ONE 6:e19688. doi: 10.1371/journal.pone.0019688
Litvintseva, A. P., Kestenbaum, L., Vilgalys, R., and Mitchell, T. G. (2005). Comparative analysis of environmental and clinical populations of Cryptococcus neoformans. J. Clin. Microbiol. 43, 556–564. doi: 10.1128/JCM.43.2.556-564.2005
Litvintseva, A. P., Lin, X., Templeton, I., Heitman, J., and Mitchell, T. G. (2007). Many globally isolated AD hybrid strains of Cryptococcus neoformans originated in Africa. PLoS Pathog. 3:e114. doi: 10.1371/journal.ppat.0030114
Mahmoud, Y. A. (1999). First environmental isolation of Cryptococcus neoformans var. neoformans and var. gattii from the Gharbia Governorate, Egypt. Mycopathologia 148, 83–86. doi: 10.1023/A:1007166818993
Martinez, L. R., Garcia-Rivera, J., and Casadevall, A. (2001). Cryptococcus neoformans var. neoformans (serotype D) strains are more susceptible to heat than C. neoformans var. grubii (serotype A) strains. J. Clin. Microbiol. 39, 3365–3367. doi: 10.1128/JCM.39.9.3365-3367.2001
May, R. C., Stone, N. R., Wiesner, D. L., Bicanic, T., and Nielsen, K. (2016). Cryptococcus: from environmental saprophyte to global pathogen. Nat. Rev. Microbiol. 14, 106–117. doi: 10.1038/nrmicro.2015.6
McKenna, A., Hanna, M., Banks, E., Sivachenko, A., Cibulskis, K., Kernytsky, A., et al. (2010). The genome analysis toolkit: a MapReduce framework for analyzing next-generation DNA sequencing data. Genome. Res. 20, 1297–1303. doi: 10.1101/gr.107524.110
Montagna, M. T., De Donno, A., Caggiano, G., Serio, F., De Giglio, O., Bagordo, F., et al. (2018). Molecular characterization of Cryptococcus neoformans and Cryptococcus gattii from environmental sources and genetic comparison with clinical isolates in Apulia, Italy. Environ. Res. 160, 347–352. doi: 10.1016/j.envres.2017.09.032
Mseddi, F., Sellami, A., Jarboui, M. A., Sellami, H., Makni, F., and Ayadi, A. (2011). First environmental isolations of Cryptococcus neoformans and Cryptococcus gattii in Tunisia and review of published studies on environmental isolations in Africa. Mycopathologia 171, 355–360. doi: 10.1007/s11046-010-9381-7
Nielsen, K., Cox, G. M., Wang, P., Toffaletti, D. L., Perfect, J. R., and Heitman, J. (2003). Sexual cycle of Cryptococcus neoformans var. grubii and virulence of congenic a and alpha isolates. Infect. Immun. 71, 4831–4841. doi: 10.1128/IAI.71.9.4831-4841.2003
Nielsen, K., Marra, R. E., Hagen, F., Boekhout, T., Mitchell, T. G., Cox, G. M., et al. (2005). Interaction between genetic background and the mating-type locus in Cryptococcus neoformans virulence potential. Genetics 171, 975–983. doi: 10.1534/genetics.105.045039
Noguera, M. C., Escandón, P., and Castañeda, E. (2015). Cryptococcosis in Atlántico, Colombia: an approximation of the prevalence of this mycosis and the distribution of the etiological agent in the environment. Rev. Soc. Bras. Med. Trop. 48, 580–586. doi: 10.1590/0037-8682-0178-2015
Nosanchuk, J. D., Shoham, S., Fries, B. C., Shapiro, D. S., Levitz, S. M., and Casadevall, A. (2000). Evidence of zoonotic transmission of Cryptococcus neoformans from a pet cockatoo to an immunocompromised patient. Ann. Intern. Med. 132, 205–208. doi: 10.7326/0003-4819-132-3-200002010-00006
Price, M. N., Dehal, P. S., and Arkin, A. P. (2009). FastTree: computing large minimum evolution trees with profiles instead of a distance matrix. Mol. Biol. Evol. 26, 1641–1650. doi: 10.1093/molbev/msp077
Randhawa, H. S., Kowshik, T., Chowdhary, A., Prakash, A., Khan, Z. U., and Xu, J. (2011). Seasonal variations in the prevalence of Cryptococcus neoformans var. grubii and Cryptococcus gattii in decayed wood inside trunk hollows of diverse tree species in north-western India: a retrospective study. Med. Mycol. 49, 320–323. doi: 10.3109/13693786.2010.516457
Randhawa, H. S., Kowshik, T., Chowdhary, A., Preeti Sinha, K., Khan, Z. U., Sun, S., et al. (2008). The expanding host tree species spectrum of Cryptococcus gattii and Cryptococcus neoformans and their isolations from surrounding soil in India. Med. Mycol. 46, 823–833. doi: 10.1080/13693780802124026
Randhawa, H. S., Kowshik, T., and Khan, Z. U. (2005). Efficacy of swabbing versus a conventional technique for isolation of Cryptococcus neoformans from decayed wood in tree trunk hollows. Med. Mycol. 43, 67–71. doi: 10.1080/13693780410001712025
Rhodes, J., Desjardins, C. A., Sykes, S. M., Beale, M. A., Vanhove, M., Sakthikumar, S., et al. (2017). Tracing genetic exchange and biogeography of Cryptococcus neoformans var. grubii at the global population level. Genetics 207, 327–346. doi: 10.1534/genetics.117.203836
Romeo, O., Scordino, F., Chillemi, V., and Criseo, G. (2012). Cryptococcus neoformans/Cryptococcus gattii species complex in southern Italy: an overview on the environmental diffusion of serotypes, genotypes and mating-types. Mycopathologia 174, 283–291. doi: 10.1007/s11046-012-9547-6
Romeo, O., Scordino, F., and Criseo, G. (2011). Environmental isolation of Cryptococcus gattii serotype B, VGI/MATα strains in southern Italy. Mycopathologia 171, 423–430. doi: 10.1007/s11046-010-9389-z
Ruiz, A., Fromtling, R. A., and Bulmer, G. S. (1981). Distribution of Cryptococcus neoformans in a natural site. Infect. Immun. 31, 560–563.
Saracli, M. A., Yildiran, S. T., Sener, K., Gonlum, A., Doganci, L., Keller, S. M., et al. (2006). Genotyping of Turkish environmental Cryptococcus neoformans var. neoformans isolates by pulsed field gel electrophoresis and mating type. Mycoses 49, 124–129. doi: 10.1111/j.1439-0507.2006.01203.x
Sengul, M., Kutlu, M., Dogen, A., Aksoy, L., Gonca, S., Ilkit, M., et al. (2019). Isolation of Cryptococcus neoformans from a chestnut tree (Castanea sativa), Denizli, Turkey. Mikrobiyol. Bul. 53, 61–69. doi: 10.5578/mb.67583
Singh, K., Ilkit, M., Shokohi, T., Tolooe, A., Malik, R., and Seyedmousavi, S. (2018). “Cryptococcosis: emergence of Cryptococcus gattii in animals and zoonotic potential,” in Emerging and Epizootic Fungal Infections in Animals, eds S. Seyedmousavi, G. S. de Hoog, J. Guillot, and P. Verweij (Cham: Springer), 249–287. doi: 10.1007/978-3-319-72093-7_12
Spina-Tensini, T., Muro, M. D., Queiroz-Telles, F., Strozzi, I., Moraes, S. T., Petterle, R. R., et al. (2017). Geographic distribution of patients affected by Cryptococcus neoformans/Cryptococcus gattii species complexes meningitis, pigeon and tree populations in Southern Brazil. Mycoses 60, 51–58. doi: 10.1111/myc.12550
Springer, D. J., Mohan, R., and Heitman, J. (2017). Plants promote mating and dispersal of the human pathogenic fungus Cryptococcus. PLoS ONE 12:e0171695. doi: 10.1371/journal.pone.0171695
Springer, D. J., Saini, D., Byrnes, E. J., Heitman, J., and Frothingham, R. (2013). Development of an aerosol model of Cryptococcus reveals humidity as an important factor affecting the viability of Cryptococcus during aerosolization. PLoS ONE 8:e69804. doi: 10.1371/journal.pone.0069804
Sun, S., Billmyre, R. B., Mieczkowski, P. A., and Heitman, J. (2014). Unisexual reproduction drives meiotic recombination and phenotypic and karyotypic plasticity in Cryptococcus neoformans. PLoS Genet. 10:e1004849. doi: 10.1371/journal.pgen.1004849
Sun, S., Hsueh, Y. P., and Heitman, J. (2012). Gene conversion occurs within the mating-type locus of Cryptococcus neoformans during sexual reproduction. PLoS Genet. 8:e1002810. doi: 10.1371/journal.pgen.1002810
Sun, S., Priest, S. J., and Heitman, J. (2019). Cryptococcus neoformans mating and genetic crosses. Curr. Protoc. Microbiol. 53:e.75. doi: 10.1002/cpmc.75
Uejio, C. K., Mak, S., Manangan, A., Luber, G., and Bartlett, K. H. (2015). Climatic influences on Cryptococcus gattii populations, Vancouver Island, Canada, 2002–2004. Emerg. Infect. Dis. 21, 1989–1996. doi: 10.3201/eid2111.141161
Uylaşer, V., and Yildiz, G. (2014). The historical development and nutritional importance of olive and olive oil constituted an important part of the Mediterranean diet. Crit. Rev. Food Sci. Nutr. 54, 1092–1101. doi: 10.1080/10408398.2011.626874
Vanhove, M., Beale, M. A., Rhodes, J., Chanda, D., Lakhi, S., Kwenda, G., et al. (2017). Genomic epidemiology of Cryptococcus yeasts identifies adaptation to environmental niches underpinning infection across an African HIV/AIDS cohort. Mol. Ecol. 26, 1991–2005. doi: 10.1111/mec.13891
Velagapudi, R., Hsueh, Y. P., Geunes-Boyer, S., Wright, J. R., and Heitman, J. (2009). Spores as infectious propagules of Cryptococcus neoformans. Infect. Immun. 77, 4345–4355. doi: 10.1128/IAI.00542-09
Viviani, M. A., Esposto, M. C., Cogliati, M., Montagna, M. T., and Wickes, B. L. (2001). Isolation of a Cryptococcus neoformans serotype A MATa strain from the Italian environment. Med. Mycol. 39, 383–386. doi: 10.1080/mmy.39.5.383.386
Keywords: Cryptococcus neoformans, natural isolates, Turkey, genetic diversity, population, evolution
Citation: Ergin Ç, Şengül M, Aksoy L, Döğen A, Sun S, Averette AF, Cuomo CA, Seyedmousavi S, Heitman J and Ilkit M (2019) Cryptococcus neoformans Recovered From Olive Trees (Olea europaea) in Turkey Reveal Allopatry With African and South American Lineages. Front. Cell. Infect. Microbiol. 9:384. doi: 10.3389/fcimb.2019.00384
Received: 07 August 2019; Accepted: 25 October 2019;
Published: 08 November 2019.
Edited by:
Brian Wickes, The University of Texas Health Science Center at San Antonio, United StatesReviewed by:
Massimo Cogliati, University of Milan, ItalyVishukumar Aimanianda, Institut Pasteur, France
Copyright © 2019 Ergin, Şengül, Aksoy, Döğen, Sun, Averette, Cuomo, Seyedmousavi, Heitman and Ilkit. This is an open-access article distributed under the terms of the Creative Commons Attribution License (CC BY). The use, distribution or reproduction in other forums is permitted, provided the original author(s) and the copyright owner(s) are credited and that the original publication in this journal is cited, in accordance with accepted academic practice. No use, distribution or reproduction is permitted which does not comply with these terms.
*Correspondence: Macit Ilkit, bWFjaXRpbGtpdEBnbWFpbC5jb20=