- 1Institute of Microbiology, Infectious Diseases and Immunology, Charité - University Medicine Berlin, Corporate Member of Freie Universität Berlin, Humboldt-Universität zu Berlin, Berlin Institute of Health, Berlin, Germany
- 2Department of Biology, Institute for Microbiology, Friedrich Alexander University Erlangen-Nuremberg, Erlangen, Germany
Even though human Campylobacter jejuni infections are progressively increasing worldwide, the underlying molecular mechanisms of pathogen-host-interactions are still not fully understood. We have recently shown that the secreted serine protease HtrA plays a key role in C. jejuni cellular invasion and transepithelial migration in vitro, and is involved in the onset of intestinal pathology in murine infection models in vivo. In the present study, we investigated whether the protease activity of HtrA had an impact in C. jejuni induced acute enterocolitis. For this purpose, we perorally infected secondary abiotic IL-10−/− mice with wildtype C. jejuni strain NCTC11168 (11168WT) or isogenic bacteria carrying protease-inactive HtrA with a single point mutation at S197A in the active center (11168HtrA−S197A). Irrespective of the applied pathogenic strain, mice harbored similar C. jejuni loads in their feces and exhibited comparably severe macroscopic signs of acute enterocolitis at day 6 postinfection (p.i.). Interestingly, the 11168HtrA−S197A infected mice displayed less pronounced colonic apoptosis and immune cell responses, but enhanced epithelial proliferation as compared to the 11168WT strain infected controls. Furthermore, less distinct microscopic sequelae in 11168HtrA−S197A as compared to parental strain infected mice were accompanied by less distinct colonic secretion of pro-inflammatory cytokines such as MCP-1, IL-6, TNF, and IFN-γ in the former as compared to the latter. Strikingly, the S197A point mutation was additionally associated with less pronounced systemic pro-inflammatory immune responses as assessed in serum samples. In conclusion, HtrA is a remarkable novel virulence determinant of C. jejuni, whose protease activity is not required for intestinal colonization and establishment of disease, but aggravates campylobacteriosis by triggering apoptosis and pro-inflammatory immune responses.
Introduction
Campylobacter jejuni is a Gram-negative, spiral-shaped, flagellated bacterium that grows under microaerobic conditions at a temperature optimum of 37–42°C (Parkhill et al., 2000; Brøndsted et al., 2005; Young et al., 2007). C. jejuni is a commensal in the gastrointestinal tract of various animal hosts such as swine, cattle, and birds (Fouts et al., 2005). Consequently, the consumption of contaminated meat products, raw milk and water are the most frequent sources of C. jejuni infections in humans (Bereswill et al., 2011; Pielsticker et al., 2012). The infection comes along with abdominal pain, fever, myalgia, and watery or bloody diarrhea. In the acute stage of human campylobacteriosis, manifestations like crypt abscesses, ulcerations, and accumulation of immune cells in the colon can be observed (Kist and Bereswill, 2001; Backert et al., 2017). In addition, post-infectious complications such as Guillain–Barré syndrome and Miller Fisher syndrome can emerge in a minority of patients (Masanta et al., 2013; Goodfellow and Willison, 2016; Backert et al., 2017). C. jejuni bacteria enter the host intestine via the oral route and colonize the distal ileum and colon by attaching to epithelial cells (Kist and Bereswill, 2001; Boehm et al., 2018). To reach deeper tissues and cause inflammatory responses during the infection process, C. jejuni need to cross the epithelial barrier, which is accomplished by paracellular transmigration as well as invasion into intestinal epithelial cells (Boehm et al., 2012). After crossing the intestinal epithelial barrier and invasion of the underlying submucosa, C. jejuni can enter the mesenteric lymph nodes (MLN), and may reach different organs such as the spleen or liver via the bloodstream, thereby causing extra-intestinal inflammation (Backert et al., 2013).
The exact mechanisms how C. jejuni induce acute and invasive enterocolitis remain yet unclear. However, it has been observed that C. jejuni interferes with several signal transduction pathways of the innate immune system. We have recently shown that the pattern recognition receptors Toll-like receptor-4 (TLR-4) (Bereswill et al., 2011; Haag et al., 2012a; Otto et al., 2012) and nucleotide-oligomerization-domain-2 (Nod2) (Heimesaat et al., 2017a,b) play a pivotal role in the development of immunopathology during C. jejuni infection. Moreover, C. jejuni rapidly activates MAPK/ERK (mitogen-activated protein kinases/extracellular signal-regulated kinases) as well as the pro-inflammatory transcription factor NF-κB (nuclear factor kappa-light-chain-enhancer of activated B cells) that up-regulates the transcription of a variety of immune response genes (Chen et al., 2006; Hu et al., 2006). It has been shown that C. jejuni can enhance the production of interleukin (IL)−8, a chemoattractant that recruits dendritic cells, macrophages and neutrophils to the site of infection (Jagusztyn-Krynicka et al., 2009). Internalization of C. jejuni by dendritic cells results in their activation and triggers adaptive immune response. This leads to an enhanced expression of a variety of pro-inflammatory cytokines, namely IL-1β, IL-6, IL-8, IL-10, IL-12, Interferon (IFN) -γ and tumor necrosis factor (TNF) in immune cells (Hu et al., 2006; Jagusztyn-Krynicka et al., 2009).
C. jejuni is known to express numerous virulence factors for its adhesion to, invasion into and transmigration across gut epithelial cells (Masanta et al., 2013). Recent studies suggest that high-temperature requirement A (HtrA) has a major impact on these processes and thereby increases virulence of several pathogens (Boehm et al., 2012, 2018). So far, HtrA proteases and their orthologs have been investigated in bacterial pathogens such as Escherichia coli (Jiang et al., 2008; Krojer et al., 2008), Helicobacter pylori (Hoy et al., 2010; Albrecht et al., 2018), Legionella pneumophila (Schubert et al., 2015), Borrelia burgdorferi (Coleman et al., 2013), Bacillus anthracis (Chitlaru et al., 2011, 2017), Streptococcus pneumoniae (Cassone et al., 2012) or defined Chlamydia species (Wu et al., 2011). Various studies revealed distinct bacterial virulence features of HtrA orthologs. For example, inactivation of HtrA in Clostridium difficile resulted in more pronounced virulence as shown in a hamster model (Bakker et al., 2014). In contrast, overexpression of HtrA in Streptococcus pneumoniae was associated with enhanced virulence of the pathogen in vitro, whereas the inactivation of HtrA resulted in attenuated pneumonia in infected mice (de Stoppelaar et al., 2013).
HtrA constitutes a serine protease with an additional chaperone function, which provides a protective effect against temperature- and oxidative stress induced protein degradation in the periplasm (Krojer et al., 2008; Boehm et al., 2018). The protein consists of a signal peptide, a trypsin-like serine protease module and one or two PDZ (post synaptic density protein (PSD59), Drosophila disc large tumor suppressor (Dlg1), and zonula occludens-1 protein (ZO-1)) domains that enable protein-protein interactions (Brøndsted et al., 2005; Backert et al., 2018). Surprisingly, HtrA is not only acting intracellularly within the bacteria, but is also actively secreted into the extracellular environment, where it can degrade extracellular host proteins like the cell surface adhesion protein and tumor-suppressor E-cadherin (Boehm et al., 2012, 2013 Hoy et al., 2012; Backert et al., 2013; Heimesaat et al., 2014a). Cleavage of the extracellular E-cadherin domain leads to the depletion of adherence junctions. The following downregulation of barrier functions enables C. jejuni to transmigrate across the polarized epithelium (Boehm et al., 2012; Hoy et al., 2012). In vitro experiments revealed that deletion of the htrA gene or expression of a HtrA protease activity-deficient point mutant variant (serine residue 197 replaced by alanine) in C. jejuni leads to significantly reduced E-cadherin cleavage and transmigration across polarized epithelial cells, but did not cause loss of bacterial motility (Boehm et al., 2013). The finding that the S197A point mutation abolished protease activity, but did not affect HtrA secretion, led to the assumption that HtrA secretion does not require proteolytic activity (Boehm et al., 2013). Recent studies in the secondary abiotic IL-10−/− mouse model infected with a C. jejuni htrA deletion mutant (11168ΔhtrA) showed notably less severe clinical symptoms and reduced intestinal as well as extra-intestinal immunopathological sequelae of infection as compared to 11168WT strain infected control mice (Heimesaat et al., 2014a). The role of HtrA during C. jejuni induced immunopathology was further confirmed in conventional infant mice which showed reduced intestinal and extra-intestinal (liver, kidney and lung) immune responses after infection with the 11168ΔhtrA mutant in comparison to 11168WT infection (Heimesaat et al., 2014b). However, the role of HtrA's protease activity in C. jejuni infected mice was not yet investigated.
For a long time, investigations of distinct C. jejuni pathogenicity factors in vivo have been hampered by the scarcity of appropriate models (Masanta et al., 2013; Heimesaat and Bereswill, 2015). Conventional mice, for instance, are well protected from C. jejuni infection even upon peroral challenge with high pathogenic loads due to the physiological colonization resistance exerted by the murine commensal gut microbiota (Bereswill et al., 2011; Fiebiger et al., 2016). Following depletion of the intestinal microbiota by broad-spectrum antibiotic treatment, however, secondary abitoic mice can be stably infected with C. jejuni following peroral infection, but do not display gross pathogen induced symptoms seen in human campylobacteriosis (Bereswill et al., 2011). Given that mice are approximately 1,000 times more resistant to TLR-4 ligands including lipopolysaccharide and lipooligosacchariade and IL-10 gene deficiency renders mice susceptible to TLR-4 ligands (Warren et al., 2010; Haag et al., 2012a), we could recently show that upon depletion of the gut microbiota by broad-spectrum antibiotic treatment, IL-10−/− mice could not only be stably infected with high C. jejuni loads, but also developed acute, non-self-limiting C. jejuni induced enterocolitis within 1 week postinfection (p.i.), thereby mimicking key features of severe campylobacteriosis in immunocompromized patients (Haag et al., 2012a; Heimesaat et al., 2014c, 2017a).
In order to investigate the role of C. jejuni HtrA protease activity in vivo, we here infected secondary abiotic IL-10−/− mice with the C. jejuni strain 11168HtrA−S197A which expresses the protease activity-deficient point mutant variant and used the corresponding 11168WT as reference. We addressed bacterial colonization efficiencies and translocation rates to extra-intestinal tissue sites and surveyed clinical symptoms as well as intestinal and extra-intestinal including systemic inflammatory immune responses following peroral infection with respective strains.
Materials and Methods
Generation of Secondary Abiotic Mice
Female and male IL-10−/− mice (all in C57BL/6j background) were bred and maintained under specific pathogen free (SPF) conditions in the same unit of the Forschungseinrichtungen für Experimentelle Medizin (FEM, Charité - University Medicine Berlin). To order to assure stable gastrointestinal C. jejuni colonization and override physiological colonization resistance (Bereswill et al., 2011), secondary abiotic (i.e., gnotobiotic) mice virtually lacking an intestinal microbiota were generated (Heimesaat et al., 2006; Bereswill et al., 2011; Haag et al., 2012a). In brief, immediately post weaning 3 weeks old mice were subjected to a 10-week course of broad-spectrum antibiotic treatment by adding ampicillin plus sulbactam (1 g/L; Ratiopharm, Germany), vancomycin (500 mg/L; Cell Pharm, Germany), ciprofloxacin (200 mg/L; Bayer Vital, Germany), imipenem (250 mg/L; MSD, Germany) and metronidazole (1 g/L; Fresenius, Germany) to the autoclaved drinking water (ad libitum). To assure antibiotic washout, the antibiotic cocktail was replaced by autoclaved tap water 2 days prior infection.
C. jejuni Infection
Mice (3 months of age) were then perorally infected with 109 colony forming units (CFU) of the C. jejuni parental strain NCTC11168 (11168WT) or the isogenic mutant strain 11168HtrA−S197A of the serine protease gene htrA (Bæk et al., 2011) in a volume of 0.3 mL phosphate buffered saline (PBS; Gibco, life technologies, UK) on two consecutive days (days 0 and 1) by gavage as reported previously (Bereswill et al., 2011). Animals were continuously maintained in a sterile environment (autoclaved food and drinking water or sterile antibiotic cocktail) and handled under strict aseptic conditions to prevent from contaminations.
Clinical Conditions
Before and after C. jejuni infection the clinical conditions of mice were assessed on a daily basis by using a standardized cumulative clinical score (maximum 12 points), addressing the abundance of blood in feces (0: no blood; 2: microscopic detection of blood by the Guajac method using Haemoccult, Beckman Coulter / PCD, Germany; 4: macroscopic blood visible), diarrhea (0: formed feces; 2: pasty feces; 4: liquid feces), and the clinical aspect (0: normal; 2: ruffled fur, less locomotion; 4: isolation, severely compromised locomotion, pre-final aspect) as described earlier (Heimesaat et al., 2014a).
Sampling Procedures
At day 6 p.i., mice were sacrificed by isoflurane inhalation (Abbott, Germany). Luminal gastrointestinal samples (i.e., from stomach, duodenum, ileum and colon) and ex vivo biopsies from colon, MLN, liver, kidney, and spleen were taken under sterile conditions. Large intestinal samples were collected from each mouse in parallel for microbiological, immunohistopathological and immunological analyses. The absolute colonic lengths were measured by a ruler (in cm).
Immunohistochemistry
In situ immunohistochemical analyses were performed in colonic ex vivo biopsies that had been immediately fixed in 5% formalin and embedded in paraffin as described earlier (Alutis et al., 2015a,b; Heimesaat et al., 2018). In brief, in order to detect apoptotic epithelial cells, proliferating epithelial cells, macrophages/monocytes, T lymphocytes and regulatory T cells, colonic paraffin sections (5 μm) were stained with primary antibodies directed against cleaved caspase 3 (Asp175, Cell Signaling, Beverly, MA, USA, 1:200), Ki67 (TEC3, Dako, Denmark, 1:100), F4/80 (# 14-4801, clone BM8, eBioscience, San Diego, CA, USA, 1:50), CD3 (#N1580, Dako, 1:10), and FOXP3 (FJK-16s, eBioscience, 1:100), respectively. Positively stained cells were then examined by light microscopy (magnification 100 x and 400 x), and for each mouse the average number of respective positively stained cells was determined within at least six high power fields (HPF, 0.287 mm2, 400 × magnification) by a blinded independent investigator.
Bacterial Colonization
C. jejuni were quantitatively assessed in feces over time p.i. and in homogenates of ex vivo biopsies taken from MLN, spleen, liver, and kidney at day 6 p.i. by culture as stated elsewhere (Bereswill et al., 2011; Heimesaat et al., 2013). The detection limit of viable pathogens was ≈100 CFU per g.
Cytokine Detection in Supernatants of Intestinal and Extra-Intestinal ex vivo Biopsies
Colonic ex vivo biopsies were cut longitudinally, washed in PBS, and strips of approximately 1 cm2 tissue were placed in 24-flat-bottom well culture plates (Nunc, Germany) containing 500 μL serum-free RPMI 1640 medium (Gibco, life technologies, UK) supplemented with penicillin (100 U/mL) and streptomycin (100 μg/mL; PAA Laboratories, Germany). After 18 h at 37°C, culture supernatants were tested for MCP-1, IL-6, TNF, and IFN-γ by the Mouse Inflammation Cytometric Bead Assay (CBA; BD Biosciences, Germany) on a BD FACSCanto II flow cytometer (BD Biosciences). Systemic pro-inflammatory cytokine concentrations were measured in serum samples.
Statistical Analysis
Medians and levels of significance were determined using Mann-Whitney test (GraphPad Prism v7, USA) as indicated. Two-sided probability (p) values ≤ 0.05 were considered significant. Experiments were reproduced three times.
Results
Intestinal Colonization Properties of a C. jejuni 11168HtrA-S197A Mutant Strain in Perorally Infected Secondary Abiotic IL-10−/− Mice
To assure stable C. jejuni infection, the gut microbiota and hence physiological colonization resistance of IL-10−/− mice was abrogated by standard procedures using a ten-week course of broad-spectrum antibiotic treatment (Bereswill et al., 2011; Heimesaat et al., 2014a,b). Subsequently, generated secondary abiotic mice were subjected to peroral challenge with 109 CFU of either the parental C. jejuni strain 11168WT or its isogenic mutant 11168HtrA−S197A variant. Our daily survey of fecal pathogen concentrations revealed that the point mutation in the htrA gene did not impact intestinal colonization properties as indicated by comparable median loads of 109 CFU of either C. jejuni strain per g feces until day 6 p.i. (Figure 1). Upon sacrifice, the 11168HtrA−S197A mutant strain infected mice harbored lower C. jejuni numbers in luminal samples taken from the stomach, duodenum and colon as compared to 11168WT infected counterparts (p < 0.05–0.001; Figure 2). Of note, the differences in C. jejuni loads were less pronounced the more distal the gastrointestinal compartment the samples were derived from. Particularly in the colonic lumen the differences in median pathogen loads were approximately 0.5 orders of magnitude and hence rather minor (p < 0.05; Figure 2D).
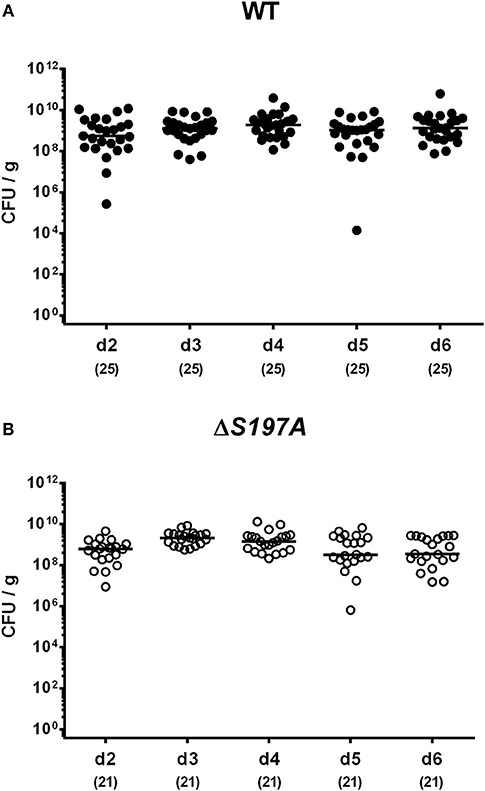
Figure 1. Time course of fecal C. jejuni loads following peroral infection of secondary abiotic IL-10−/− mice. Secondary abiotic IL-10−/− mice were perorally infected either with the C. jejuni 11168WT strain [(A), closed circles] or the isogenic htrA mutant 11168HtrA−S197A [(B), open circles] by gavage on two consecutive days (d) 0 and 1. Pathogenic loads were quantitated in fecal samples derived from each mouse on a daily basis post-infection by culture and expressed in colony forming units per g (CFU/g). Medians (black bars) and numbers of analyzed animals (in parentheses) are indicated. Data were pooled from four independent experiments.
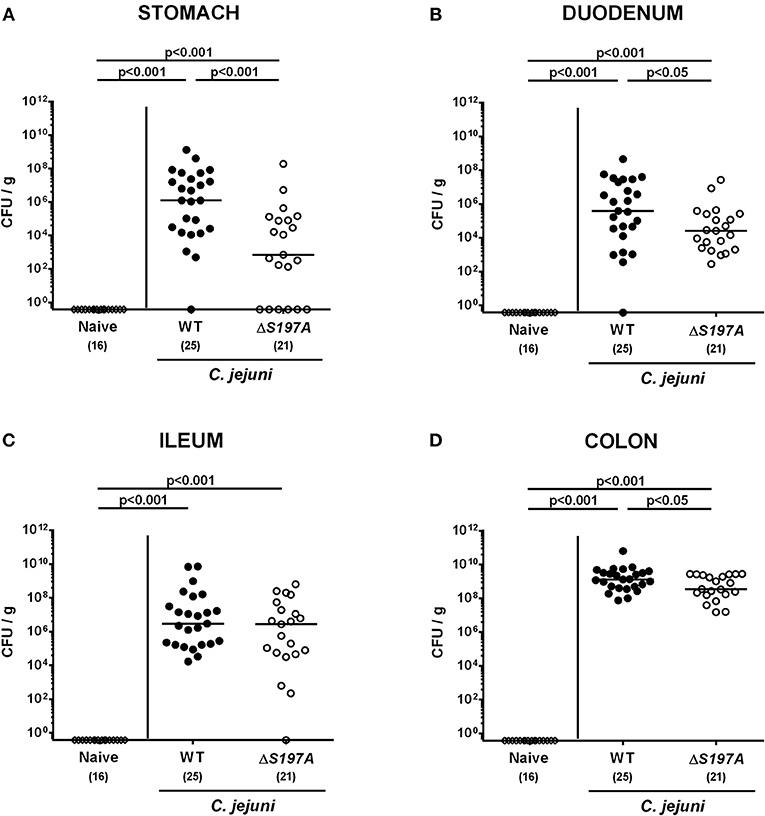
Figure 2. C. jejuni loads in the gastrointestinal tract of secondary abiotic IL-10−/− mice six days following peroral infection. Secondary abiotic IL-10−/− mice were perorally infected either with the C. jejuni 11168WT strain (closed circles) or the isogenic htrA mutant 11168HtrA−S197A (open circles) by gavage on days 0 and 1. Pathogenic loads (CFU, colony forming units per gram) were assessed in luminal samples derived from distinct parts of the gastrointestinal tract (A–D) at day 6 postinfection as indicated by culture. Naive mice (open diamonds) served as uninfected controls. Medians (black bars), levels of significance (p-values) determined by the Mann-Whitney U-test and numbers of analyzed animals (in parentheses) are indicated. Data were pooled from four independent experiments.
Macroscopic Sequelae in C. jejuni 11168HtrA-S197A Infected Secondary Abiotic IL-10−/− Mice
We further assessed whether the disease development upon peroral infection of secondary abiotic IL-10−/− mice differed between 11168HtrA−S197A mutant and 11168WT strains. Until day 6 following infection with either strain, mice were suffering from comparably severe C. jejuni induced disease as indicated by similar clinical scores that had been assessed during our kinetic survey (Figure 3A and Figure S1). We further stratified clinical conditions of C. jejuni infected mice to fecal blood positivity rates and determined virtually comparably frequent abundances of fecal blood following infection with either C. jejuni strain (Figure S2). Given that intestinal inflammation is accompanied by significant shortening of the inflamed intestine (Heimesaat et al., 2006, 2007; Haag et al., 2012a,b), we measured the large intestinal lengths upon necropsy. At day 6 p.i., colonic lengths of mice infected with either strain were comparable (n.s. Figure 3B), but lower as compared to uninfected naive control animals as expected (p < 0.001; Figure 3B). Hence, the loss of proteolytic activity of HtrA caused by the S197A point mutation in the htrA gene did neither impact pathogenic intestinal colonization properties nor gross disease following peroral C. jejuni infection of secondary abiotic IL-10−/− mice.
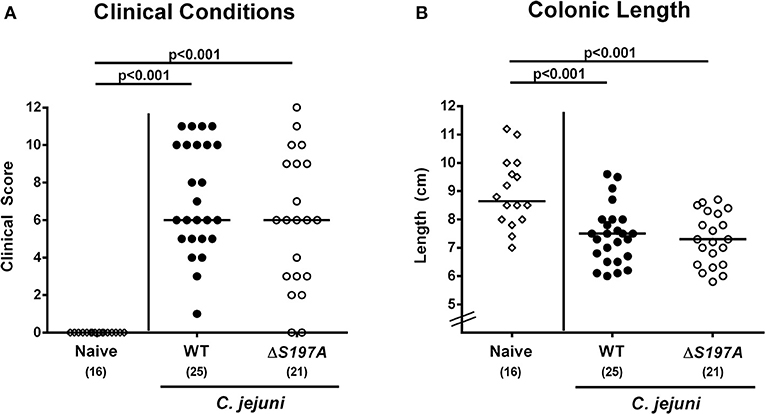
Figure 3. Macroscopic sequelae in C. jejuni infected secondary abiotic IL-10−/− mice. Secondary abiotic IL-10−/− mice were perorally infected either with the C. jejuni 11168WT strain (closed circles) or the isogenic htrA mutant 11168HtrA−S197A (open circles) by gavage on days 0 and 1. (A) Clinical symptoms were quantitatively assessed applying a standardized clinical scoring system on day 6 postinfection (see methods) and (B) colonic lengths were measured by a ruler (in cm). Naive mice (open diamonds) served as uninfected controls. Medians (black bars), level of significance (p-values) as determined by Mann-Whitney U-test and numbers of analyzed animals (in parentheses) are indicated. Data were pooled from four independent experiments.
Intestinal Histopathology and Inflammatory Responses in C. jejuni 11168HtrA-S197A Infected Secondary Abiotic IL-10−/− Mice
We next surveyed inflammatory sequelae of C. jejuni infection histologically. At day 6 p.i., mice infected with either strain exhibited multi-fold increased numbers of apoptotic epithelial cells as compared to uninfected counterparts (p < 0.001 vs. naive; Figure 4A and Figure S3A). This increase was, however, less pronounced in mice infected with the 11168HtrA−S197A mutant as compared to the 11168WT infected animals (p < 0.01; Figure 4A and Figure S3A), given that the former displayed approximately 50% of caspase3 positive colonic epithelial cells as compared to the latter (Figure 4A and Figure S3A). Furthermore, C. jejuni infection resulted in increased numbers of Ki67 positive colonic epithelial cells indicative for enhanced cell proliferation and regeneration (p < 0.001 vs. naive; Figure 4B and Figure S3B). Conversely to the caspase3 positive cells, 11168HtrA−S197A infected mice displayed slightly higher Ki67 positive cells in the large intestinal epithelium as compared to 11168WT strain infected counterparts at day 6 p.i. (Figure 4B and Figure S3B).
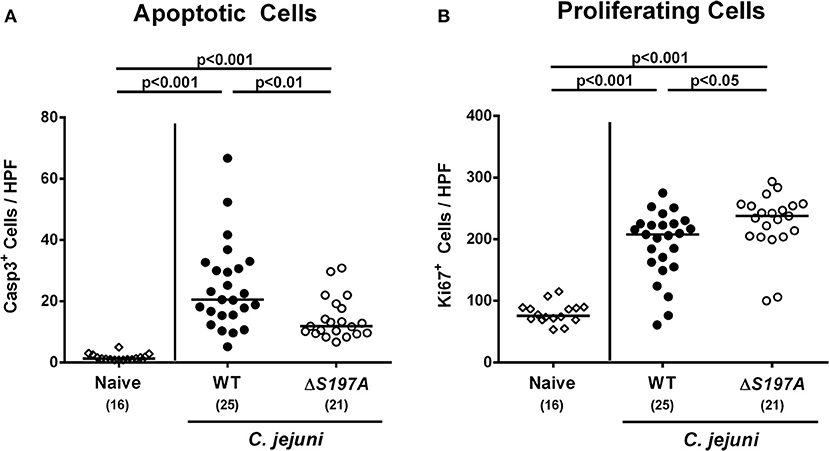
Figure 4. Microscopic colonic sequelae in C. jejuni infected secondary abiotic IL-10−/− mice. Secondary abiotic IL-10−/− mice were perorally infected either with the C. jejuni 11168WT strain (closed circles) or the isogenic htrA mutant 11168HtrA−S197A (open circles) by gavage on days 0 and 1. The average numbers of colonic epithelial (A) apoptotic cells (positive for caspase-3, Casp3) and (B) proliferating/regenerating cells (positive for Ki67) from six high power fields (HPF, 400x magnification) per animal were assessed microscopically in immunohistochemically stained large intestinal paraffin sections at day 6 postinfection. Naive mice (open diamonds) served as uninfected controls. Medians (black bars), levels of significance (p-values) determined by the Mann-Whitney U-test and numbers of analyzed animals (in parentheses) are indicated. Data were pooled from four independent experiments.
We further assessed innate (such as F4/80 positive macrophages and monocytes) and adaptive (including CD3 positive T lymphocytes and FOXP3 positive regulatory T cells) immune responses upon C. jejuni infection. At day 6 p.i., increased cell numbers of both adaptive and innate immunity parameters could be detected in the mucosa and lamina propria of infected mice irrespective of the applied C. jejuni strain (Figure 5 and Figure S3). In the colon of 11168HtrA−S197A infected mice, however, lower numbers of F4/80 positive and FOXP3 positive cells could be determined as compared to 11168WT infected control mice (p < 0.05; Figures 5A,C and Figures S3C,E). Hence, the protease-inactive htrA point mutant C. jejuni strain caused less pronounced colonic apoptosis and immune cell responses, but enhanced epithelial proliferation upon peroral infection of secondary abiotic IL-10−/− mice.
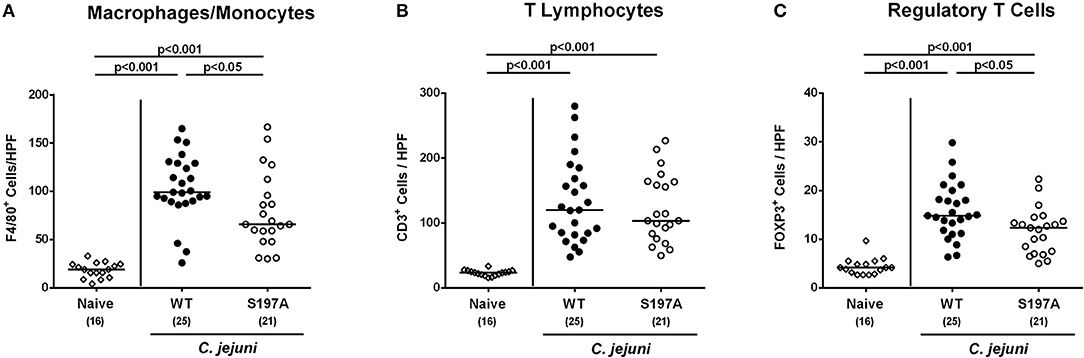
Figure 5. Colonic immune cell responses in C. jejuni infected secondary abiotic IL-10−/− mice. Secondary abiotic IL-10−/− mice were perorally infected either with the C. jejuni 11168HtrA−S197A strain (closed circles) or the isogenic htrA mutant 11168HtrA−S197A (open circles) by gavage on days 0 and 1. At day 6 postinfection, the average numbers of colonic epithelial of colonic (A) macrophages and monocytes (positive for F4/80), (B) T lymphocytes (positive for CD3), and (C) regulatory T cells (positive for FOXP3) were assessed microscopically from six high power fields (HPF, 400x magnification) per animal in immunohistochemically stained large intestinal paraffin sections. Naive mice (open diamonds) served as uninfected controls. Medians (black bars), levels of significance (p-values) determined by the Mann-Whitney U-test and numbers of analyzed animals (in parentheses) are indicated. Data were pooled from four independent experiments.
Colonic and Systemic Pro-Inflammatory Cytokine Production in C. jejuni 11168HtrA-S197A Infected Secondary Abiotic IL-10−/− Mice
We next surveyed pro-inflammatory cytokine concentrations upon infection with respective C. jejuni strains in colonic ex vivo biopsies (Figure 6). Six days following peroral challenge, large intestinal MCP-1 concentrations induced by the 11168HtrA−S197A strain were lower as compared to those in mice infected with C. jejuni 11168WT(p < 0.05 vs. naive; Figure 6A). In addition, C. jejuni infection with either strain resulted in elevated colonic IL-6, TNF and IFN-γ secretion, whereas a trend toward lower IL-6 concentrations could be assessed in the large intestines of 11168HtrA−S197A as compared to 11168WT strain infected mice at day 6 p.i. (n.s.; Figure 6B). Strikingly, strain-dependent pro-inflammatory cytokine secretion was not restricted to the gut, given that pro-inflammatory cytokines such as MCP-1, IL-6, TNF, and IFN-γ in serum samples taken at day 6 p.i. were lower in mice infected with the 11168HtrA−S197A strain as compared to mice challenged with C. jejuni 11168WT (p < 0.05–0.01; Figure 7).
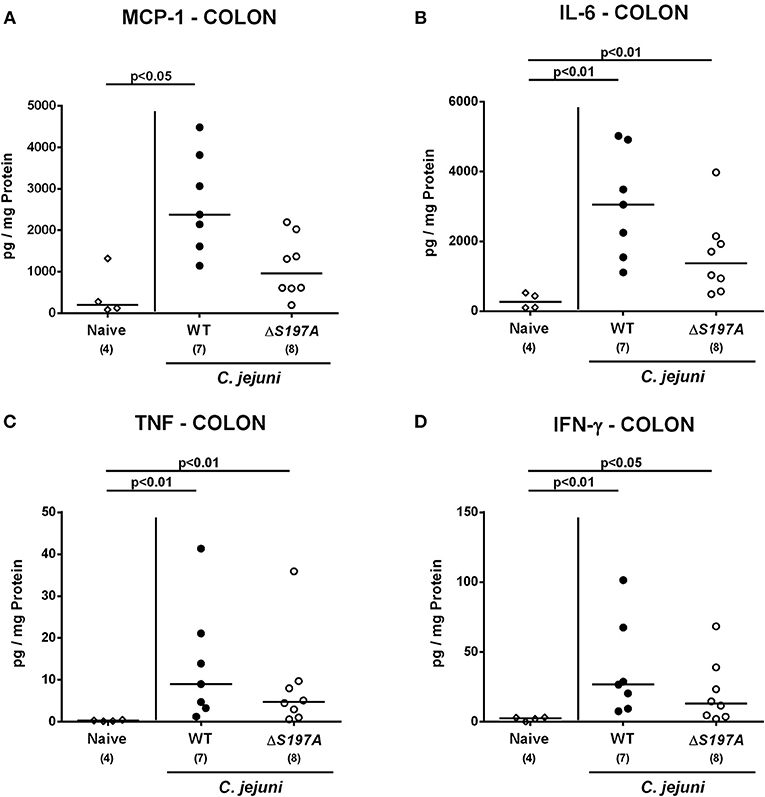
Figure 6. Colonic secretion of pro-inflammatory cytokines in C. jejuni infected secondary abiotic IL-10−/− mice. Secondary abiotic IL-10−/− mice were perorally infected either with the C. jejuni 11168HtrA−S197A strain (closed circles) or the isogenic htrA mutant 11168HtrA−S197A (open circles) by gavage on days 0 and 1. (A) MCP-1, (B) IL-6, (C) TNF, and (D) IFN-γ concentrations were measured in supernatants derived from colonic ex vivo biopsies at day 6 postinfection. Naive mice (open diamonds) served as uninfected controls. Medians (black bars), levels of significance (p-value) determined by the Mann-Whitney U-test and numbers of analyzed animals (in parentheses) are indicated. Data representative for four independent experiments are shown.
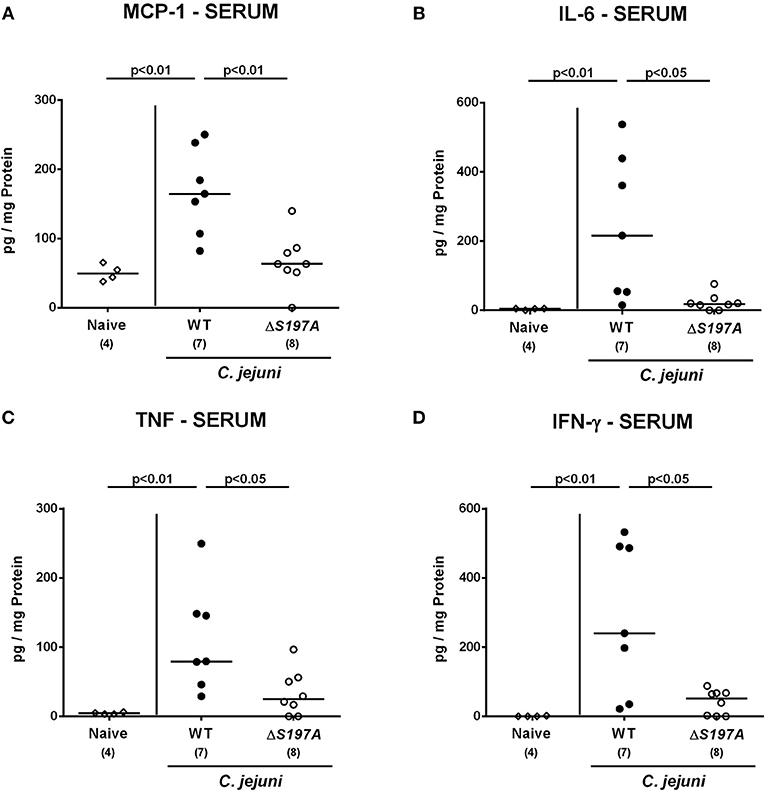
Figure 7. Systemic secretion of pro-inflammatory cytokines in C. jejuni infected secondary abiotic IL-10−/− mice. Secondary abiotic IL-10−/− mice were perorally infected either with the C. jejuni 11168HtrA−S197A strain (closed circles) or the isogenic htrA mutant 11168HtrA−S197A (open circles) by gavage on days 0 and 1. (A) MCP-1, (B) IL-6, (C) TNF, and (D) IFN-γ concentrations were measured in serum samples taken at day 6 postinfection. Naive mice (open diamonds) served as uninfected controls. Medians (black bars), levels of significance (p-value) determined by the Mann-Whitney U-test and numbers of analyzed animals (in parentheses) are indicated. Data representative for four independent experiments are shown.
We further addressed whether the lowered immune responses observed in mice challenged with the 11168HtrA−S197A mutant had a consequence for transepithelial migration and subsequent spread of viable C. jejuni from the intestinal tract to extra-intestinal including systemic compartments. Notably, at day 6 p.i., C. jejuni could be cultured from 40% and 75% of MLN taken from 11168WT and 11168HtrA−S197A strain infected mice, respectively (Figure S4). Furthermore, viable C. jejuni could be isolated from extra-intestinal and systemic compartments such as liver, kidney, spleen and cardiac blood in single cases only (Figure S4). Interestingly, in 14.3% of 11168HtrA−S197A strain infected mice, but none of 11168WT samples, viable pathogens were detectable in cardiac blood (Figure S4). Hence, the C. jejuni 11168HtrA−S197A strain translocated with higher efficiency possibly as a result of less distinct intestinal, but also less pronounced systemic pro-inflammatory cytokine secretion as compared to the 11168WT strain.
Taken together, investigation of the 11168HtrA−S197A mutant strain in the secondary abiotic IL-10−/− mice infection model revealed that the protease activity of C. jejuni HtrA is not required for intestinal colonization and establishment of disease, but triggers apoptosis and pro-inflammatory immune responses in the intestines as well as in systemic compartments. It is of note that the proteolytic activity of HtrA limits bacterial translocation to MLN and thus protects the host against systemic spread of C. jejuni.
Discussion
The serine protease and chaperone HtrA constitutes a remarkable virulence factor that enables C. jejuni to transmigrate across polarized epithelial cells by cleavage of E-cadherin and probably other junctional proteins (Boehm et al., 2012, 2018; Hoy et al., 2012). HtrA chaperone activity seems to be crucial for C. jejuni adherence to epithelial host cells and subsequently invasion, whereby HtrA protease activity appears to be not essential for these processes (Bæk et al., 2011). We have recently shown in infected mice, that the serine protease HtrA is not required for induction of enterocolitis by C. jejuni per se and plays a role in the modulation of apoptosis and immunopathology during campylobacteriosis (Heimesaat et al., 2014a,b). Six days after infection with the C. jejuni ΔhtrA deletion mutant, secondary abiotic IL-10−/− mice were suffering from less severe C. jejuni induced symptoms and developed less pronounced intestinal as well as extra-intestinal including systemic pro-inflammatory immune responses upon infection as compared to 11168WT strain infected control mice suffering from acute enterocolitis (Heimesaat et al., 2014a,b). In order to unravel in particular the role of the protease activity of HtrA in colonization efficiency, clinical symptoms as well as intestinal and extra-intestinal inflammation in more detail, we analyzed the C. jejuni 11168HtrA−S197A point mutant variant in secondary abiotic IL-10−/− mice in the present study. This mutant lacks protease activity, but still retains the chaprone function (Bæk et al., 2011; Boehm et al., 2012). Six days following peroral infection, mice harbored comparable pathogen loads of the mutant strain 11168HtrA−S197A or the parental 11168WT strain. Hence, inactivation of the serine protease active site did not alter colonic colonization capacity of C. jejuni. This supports the findings of Bæk and co-workers that the periplasmic chaperone activity of HtrA is more important for efficient binding to epithelial host cells than its protease activity (Bæk et al., 2011). More importantly, in vitro studies revealed that the protease-deficient S197A point mutant is incapable of transmigrating across polarized epithelial cells (Boehm et al., 2012). These findings led to the hypothesis, that the mutant expressing the 11168HtrA−S197A variant would rather not induce intestinal immunopathology of campylobacteriosis. However, our present in vivo study revealed that secondary abiotic IL-10−/− mice infected with the 11168HtrA−S197A strain did in fact develop ulcerative enterocolitis, which was characterized by bloody diarrhea and wasting symptoms and were comparable to the symptoms observed following 11168WT strain infection. This observation is surprising and suggests that in addition to HtrA other yet unknown bacterial factors contribute to transepithelial migration of C. jejuni and disease development in mice.
Interestingly, mice infected with the C. jejuni 11168HtrA−S197A strain displayed less immunopathological sequelae within the intestinal tract including colonic epithelial apoptosis when compared to the 11168WT strain infected mice, which is in contrast to the macroscopic phenotype that was similar in 11168WT strain infection. One needs to take into consideration, however, that the macroscopic aspect (i.e., the clinical symptoms) of the mice are rather the sum of many different pathophysiological sequelae from C. jejuni infection and cannot only be attributed to the immune responses and immunopathological features observed microscopically in the intestinal mucosa. In fact, cell proliferative and hence regenerative measures observed in colonic epithelia, thus counteracting C. jejuni induced cell damage including colonic epithelial apoptosis, were more pronounced in C. jejuni 11168HtrA−S197A strain as compared to parental strain infected mice. Thus, protease activity of HtrA triggers colonic apoptosis, but dampens cell proliferation required for tissue regeneration. Similar results were obtained in our previous analysis of the htrA deletion mutant ΔhtrA (Heimesaat et al., 2014a,b), which further supports that the protease activity of HtrA rather inhibits regeneration of damaged tissue and thereby further aggravates intestinal inflammation. This let us to propose that secreted HtrA might target immunoregulatory factor(s) directly, and future experiments should address this hypothesis. The idea that the protease activity of HtrA triggers inflammatory processes in host cells is further underlined by lower numbers of immune cells at the site of infection and less secretion of pro-inflammatory cytokines in intestinal, but also extra-intestinal and, remarkably, even systemic compartments following C. jejuni 11168HtrA−S197A as compared to 11168WT strain infection as shown here and in our previous studies with the C. jejuni ΔhtrA mutant vs. parental strain (Heimesaat et al., 2014a,b). Of note, immune responses triggered by the protease activity of HtrA prevented translocation of viable pathogens from the intestinal lumen to MLN and possibly to extra-intestinal including systemic compartments. However, on the systemic level live bacteria could be isolated, if at all, in single case only, irrespective of the applied strain.
Taken together, our data demonstrate that the proteolytic activity of HtrA results in the amplification of host immune responses upon C. jejuni infection of mice, but is not required for the onset and establishment of intestinal disease. Future studies will unravel if further distinct domains within the HtrA protein might have functional immunopathological implications during campylobacteriosis.
Data Availability
All datasets generated for this study are included in the manuscript and/or the Supplementary Files.
Ethics Statement
All animal experiments were conducted in accordance with the European Guidelines for animal welfare (2010/63/EU) following approval of the protocol by the commission for animal experiments headed by the “Landesamt für Gesundheit und Soziales” (LaGeSo, Berlin, registration number G0172/16). Clinical conditions of mice were assessed twice a day.
Author Contributions
A-MS: designed and performed experiments, analyzed data, co-wrote paper; SM: performed experiments, analyzed data; UE: performed experiments; MB: provided bacterial strains, co-edited paper; SBa: provided advice in experimental design, critically discussed results, co-edited paper; SBe: provided advice in experimental design, critically discussed results, co-edited paper; MH: designed and performed experiments, analyzed data, wrote paper.
Funding
This work was supported by the German Research Foundation (DFG) to MB (project BO4724/1-1), MH and UE (HE3040/3-1), as well as from the German Federal Ministries of Education and Research (BMBF) in frame of the zoonoses research consortium PAC-Campylobacter to SBe, MH, and SM (IP7/ 01KI1725D) and to SBa (IP9/ 01KI1725E). The funders had no role in study design, data collection and analysis, decision to publish or preparation of the manuscript.
Conflict of Interest Statement
The authors declare that the research was conducted in the absence of any commercial or financial relationships that could be construed as a potential conflict of interest.
Acknowledgments
We thank Alexandra Bittroff-Leben, Ines Puschendorf, Ulrike Fiebiger, Gernot Reifenberger, and the staff of the animal research facility at Charité – University Medicine Berlin for excellent technical assistance and animal breeding. We further thank Dr. Anja A. Kühl (Department of Medicine I for Gastroenterology, Infectious Diseases and Rheumatology/Research Center ImmunoSciences (RCIS), Charité – Universitätsmedizin Berlin) for taking representative photomicrographs of immunohistochemically stained paraffin sections. We acknowledge support from the German Research Foundation (DFG) and the Open Access Publication Fund of Charité – Universitätsmedizin Berlin.
Supplementary Material
The Supplementary Material for this article can be found online at: https://www.frontiersin.org/articles/10.3389/fcimb.2019.00079/full#supplementary-material
Figure S1. Kinetic survey of clinical conditions in C. jejuni infected secondary abiotic IL-10−/− mice. Secondary abiotic IL-10−/− mice were perorally infected either with the C. jejuni 11168WT strain (A, closed circles) or the isogenic htrA mutant 11168HtrA−S197A (B, open circles) by gavage on day (d) 0 and d1. Clinical symptoms were quantitatively assessed applying a standardized clinical scoring system from d0 until d6 postinfection (see methods). Medians (black bars) and numbers of analyzed mice (in parentheses) are indicated. Data were pooled from four independent experiments.
Figure S2. Kinetic survey of fecal blood in C. jejuni infected secondary abiotic IL-10−/− mice. Secondary abiotic IL-10−/− mice were perorally infected either with the C. jejuni 11168WT strain (A, black bars) or the isogenic htrA mutant 11168HtrA−S197A (B, white bars) by gavage on days (d) 0 and 1. The cumulative relative abundance of fecal blood (fecal blood+) out of four independent experiments was assessed macroscopically and microscopically (in %). The numbers of fecal blood positive mice out of the total numbers of analyzed animals are given in parentheses at defined time points.
Figure S3. Representative photomicrographs illustrating apoptotic and proliferating epithelial as well as immune cells responses in large intestines of C. jejuni infected secondary abiotic IL-10−/− mice. Secondary abiotic IL-10−/− mice were perorally infected either with the strain C. jejuni 11168WT or the isogenic htrA mutant 11168HtrA−S197A by gavage on days 0 and 1. Naive mice served as uninfected controls. Photomicrographs representative for four independent experiments illustrate the average numbers of (A) apoptotic epithelial cells (Casp3+), (B) proliferating epithelial cells, (C) macrophages and monocytes (F4/80+), (D) T lymphocytes (CD3+), and (E) regulatory T cells (FOXP3+) in at least six high power fields (HPF) as quantitatively assessed in colonic paraffin sections applying in situ immunohistochemistry at day 6 postinfection (100 × magnification, scale bar 100 μm).
Figure S4. Bacterial translocation in C. jejuni infected secondary abiotic IL-10−/− mice. Secondary abiotic IL-10−/− mice were perorally infected either with the C. jejuni strain 11168WT (black bars) or the isogenic htrA mutant 11168HtrA−S197A (open bars) by gavage on days 0 and 1. At day 6 postinfection, pathogenic loads were quantitatively assessed in ex vivo biopsies derived from (A) MLN, (B) liver, (C) kidney, (D) spleen and (E) cardiac blood by culture. The cumulative relative translocation rate of viable pathogens into the respective compartment out of four independent experiments is indicated (in %).
Abbreviations
CBA, Cytometric Bead Array; CFU, colony-forming units; HtrA, high-temperature requirement A; i.e., id est; IFN, interferon; IL, interleukin; MAPK/ERK, mitogen-activated protein kinases/extracellular signal-regulated kinases; MCP, Monocyte chemoattractant protein; MLN, mesenteric lymph nodes; n.s., not significant; NF-κB, nuclear factor kappa-light-chain-enhancer of activated B cells; Nod, nucleotide-oligomerization-domain; PBS, phosphate-buffered saline; PDZ, post synaptic density protein (PSD59), Drosophila disc large tumor suppressor (Dlg1), zonula occludens-1 protein (ZO-1); p, probability; p.i., postinfection; SPF, specific pathogen free; TLR, toll-like receptor; TNF, tumor necrosis factor; WT, wildtype.
References
Albrecht, N., Tegtmeyer, N., Sticht, H., Skórko-Glonek, J., and Backert, S. (2018). Amino-terminal processing of Helicobacter pylori serine protease HtrA: role in oligomerization and activity regulation. Front. Microbiol. 9:642. doi: 10.3389/fmicb.2018.00642
Alutis, M. E., Grundmann, U., Fischer, A., Hagen, U., Küehl, A. A., Göebel, U. B., et al. (2015a). The role of gelatinases in Campylobacter Jejuni infection of gnotobiotic mice. Eur. J. Microbiol. Immunol. 5, 256–267. doi: 10.1556/1886.2015.00033
Alutis, M. E., Grundmann, U., Hagen, U., Fischer, A., Küehl, A. A., Göebel, U. B., et al. (2015b). Matrix metalloproteinase-2 mediates intestinal immunopathogenesis in Campylobacter Jejuni-infected infant mice. Eur. J. Microbiol. Immunol. 5, 188–198. doi: 10.1556/1886.2015.00020
Bæk, K. T., Vegge, C. S., and Brøndsted, L. (2011). HtrA chaperone activity contributes to host cell binding in Campylobacter jejuni. Gut Pathog. 3:13. doi: 10.1186/1757-4749-3-13
Backert, S., Bernegger, S., Skórko-Glonek, J., and Wessler, S. (2018). Extracellular HtrA serine proteases: an emerging new strategy in bacterial pathogenesis. Cell. Microbiol. 20:e12845. doi: 10.1111/cmi.12845
Backert, S., Boehm, M., Wessler, S., and Tegtmeyer, N. (2013). Transmigration route of Campylobacter jejuni across polarized intestinal epithelial cells: paracellular, transcellular or both? Cell Comm. Signal. 11:72. doi: 10.1186/1478-811X-11-72
Backert, S., Tegtmeyer, N., Crónin, T., Boehm, M., and Heimesaat, M. M. (2017). “Human campylobacteriosis,” in Campylobacter - Feature, Detection, and Prevention of Foodborne Disease, ed G. Klein (London: Elsevier), 1–16. doi: 10.1016/B978-0-12-803623-5.00001-0
Bakker, D., Buckley, A. M., de Jong, A., van Winden, V. J., Verhoeks, J. P., Kuipers, O. P., et al. (2014). The HtrA-like protease CD3284 modulates virulence of Clostridium difficile. Infect. Immun. 82, 4222–4232. doi: 10.1128/IAI.02336-14
Bereswill, S., Fischer, A., Plickert, R., Haag, L. M., Otto, B., Küehl, A. A., et al. (2011). Novel murine infection models provide deep insights into the “Ménage à Trois” of Campylobacter jejuni, microbiota and host innate immunity. PLoS ONE 6:e20953. doi: 10.1371/journal.pone.0020953
Boehm, M., Haenel, I., Hoy, B., Brøndsted, L., Smith, T. G., Hoover, T., et al. (2013). Extracellular secretion of protease HtrA from Campylobacter jejuni is highly efficient and independent of its protease activity and flagellum. Eur. J. Microbiol. Immunol. 3, 163–173. doi: 10.1556/EuJMI.3.2013.3.3
Boehm, M., Hoy, B., Rohde, M., Tegtmeyer, N., Bæk, K. T., Oyarzabal, O. A., et al. (2012). Rapid paracellular transmigration of Campylobacter jejuni across polarized epithelial cells without affecting TER: role of proteolytic-active HtrA cleaving E-cadherin but not fibronectin. Gut Pathog. 4:3. doi: 10.1186/1757-4749-4-3
Boehm, M., Simson, D., Escher, U., Schmidt, A.-M., Bereswill, S., Tegtmeyer, N., et al. (2018). Function of serine protease HtrA in the lifecycle of the foodborne pathogen Campylobacter jejuni. Eur. J. Microbiol. Immunol. 8, 70–77. doi: 10.1556/1886.2018.00011
Brøndsted, L., Andersen, M. T., Parker, M., Jørgensen, K., and Ingmer, H. (2005). The HtrA protease of Campylobacter jejuni is Required for heat and oxygen tolerance and for optimal interaction with human epithelial cells. Appl. Environ. Microbiol. 71, 3205–3212. doi: 10.1128/AEM.71.6.3205-3212.2005
Cassone, M., Gagne, A. L., Spruce, L. A., Seeholzer, S. H., and Sebert, M. E. (2012). The HtrA protease from Streptococcus pneumoniae digests both denatured proteins and the competence-stimulating peptide. J. Biol. Chem. 287, 38449–38459. doi: 10.1074/jbc.M112.391482
Chen, M. L., Ge, Z., Fox, J. G., and Schauer, D. B. (2006). Disruption of tight junctions and induction of proinflammatory cytokine responses in colonic epithelial cells by Campylobacter jejuni. Infect. Immun. 74, 6581–6589. doi: 10.1128/IAI.00958-06
Chitlaru, T., Israeli, M., Rotem, S., Elia, U., Bar-Haim, E., Ehrlich, S., et al. (2017). A novel live attenuated anthrax spore vaccine based on an acapsular Bacillus anthracis sterne strain with mutations in the htrA, lef and cya genes. Vaccine 35, 6030–6040. doi: 10.1016/j.vaccine.2017.03.033
Chitlaru, T., Zaide, G., Ehrlich, S., Inbar, I., Cohen, O., and Shafferman, A. (2011). HtrA is a major virulence determinant of Bacillus anthracis. Mol. Microbiol. 81, 1542–1559. doi: 10.1111/j.1365-2958.2011.07790.x
Coleman, J. L., Crowley, J. T., Toledo, A. M., and Benach, J. L. (2013). The HtrA protease of Borrelia burgdorferi degrades outer membrane protein BmpD and chemotaxis phosphatase CheX. Mol. Microbiol. 88, 619–633. doi: 10.1111/mmi.12213
de Stoppelaar, S. F., Bootsma, H. J., Zomer, A., Roelofs, J. J., Hermans, P. W., van 't Veer, C., et al. (2013). Streptococcus pneumoniae serine protease HtrA, but not SFP or PrtA, is a major virulence factor in pneumonia. PLoS ONE. 8:e80062. doi: 10.1371/journal.pone.0080062
Fiebiger, U., Bereswill, S., and Heimesaat, M. M. (2016). Dissecting the interplay between intestinal microbiota and host immunity in health and disease: lessons learned from germfree and gnotobiotic animal models. Eur. J. Microbiol. Immunol. 6, 253–271. doi: 10.1556/1886.2016.00036
Fouts, D. E., Mongodin, E. F., Mandrell, R. E., Miller, W. G., Rasko, D. A., Ravel, J., et al. (2005). Major structural differences and novel potential virulence mechanisms from the genomes of multiple Campylobacter species. PLoS Biol. 3:e15. doi: 10.1371/journal.pbio.0030015
Goodfellow, J. A., and Willison, H. J. (2016). Guillain-Barré syndrome: a century of progress. Nat. Rev. Neur. 12, 723–731. doi: 10.1038/nrneurol.2016.172
Haag, L.-M., Fischer, A., Otto, B., Plickert, R., Kuehl, A. A., Goebel, U. B., et al. (2012a). Campylobacter jejuni induces acute enterocolitis in gnotobiotic IL-10−/− mice via toll-like-receptor-2 and−4 signaling. PLoS ONE 7, 1–11. doi: 10.1371/journal.pone.0040761
Haag, L.-M., Fischer, A., Otto, B., Plickert, R., Küehl, A. A., Göebel, U. B., et al. (2012b). Intestinal microbiota shifts towards elevated commensal Escherichia coli loads abrogate colonization resistance against Campylobacter jejuni in mice. PLoS ONE 7:e35988. doi: 10.1371/journal.pone.0035988
Heimesaat, M. M., Alutis, M., Grundmann, U., Fischer, A., Tegtmeyer, N., Böehm, M., et al. (2014a). The role of serine protease HtrA in acute ulcerative enterocolitis and extra-intestinal immune responses during Campylobacter jejuni infection of gnotobiotic IL-10 deficient mice. Front. Cell. Infect. Microbiol. 4:77. doi: 10.3389/fcimb.2014.00077
Heimesaat, M. M., and Bereswill, S. (2015). Murine infection models for the investigation of Campylobacter jejuni–host interactions and pathogenicity. Berl. Münch. Tierärztl. Wochenschr. 128, 98–103.
Heimesaat, M. M., Bereswill, S., Fischer, A., Fuchs, D., Struck, D., Niebergall, J., et al. (2006). Gram-negative bacteria aggravate murine small intestinal Th1-type immunopathology following oral infection with Toxoplasma gondii. J. Immunol. 177, 8785–8795. doi: 10.4049/jimmunol.177.12.8785
Heimesaat, M. M., Fischer, A., Alutis, M., Grundmann, U., Boehm, M., Tegtmeyer, N., et al. (2014b). The impact of serine protease HtrA in apoptosis, intestinal immune responses and extra-intestinal histopathology during Campylobacter jejuni infection of infant mice. Gut Pathog. 6:16. doi: 10.1186/1757-4749-6-16
Heimesaat, M. M., Fischer, A., Jahn, H. K., Niebergall, J., Freudenberg, M., Blaut, M., et al. (2007). Exacerbation of murine ileitis by toll-like receptor 4 mediated sensing of lipopolysaccharide from commensal Escherichia coli. Gut 56, 941–948. doi: 10.1136/gut.2006.104497
Heimesaat, M. M., Giladi, E., Kühl, A. A., Bereswill, S., and Gozes, I. (2018). The octapeptide NAP alleviates intestinal and extra-intestinal anti-inflammatory sequelae of acute experimental colitis. Peptides 101, 1–9. doi: 10.1016/j.peptides.2017.12.023
Heimesaat, M. M., Grundmann, U., Alutis, M. E., Fischer, A., and Bereswill, S. (2017a). Absence of nucleotide-oligomerization-domain-2 is associated with less distinct disease in Campylobacter jejuni infected secondary abiotic IL-10 deficient mice. Front. Cell. Infect. Microbiol. 7:322. doi: 10.3389/fcimb.2017.00322
Heimesaat, M. M., Grundmann, U., Alutis, M. E., Fischer, A., and Bereswill, S. (2017b). Small intestinal pro-inflammatory immune responses following Campylobacter jejuni infection of secondary abiotic IL-10−/− mice lacking nucleotide-oligomerization-domain-2. Eur. J. Microbiol. Immunol. 7, 138–145. doi: 10.1556/1886.2017.00005
Heimesaat, M. M., Haag, L.-M., Fischer, A., Otto, B., Küehl, A. A., and Göbel, U. B. (2013). Survey of extra-intestinal immune responses in asymptomatic long-term Campylobacter jejuni-infected mice. Eur. J. Microbiol. Immunol. 3, 174–182. doi: 10.1556/EuJMI.3.2013.3.4
Heimesaat, M. M., Lugert, R., Fischer, A., Alutis, M., Küehl, A. A., Zautner, A. E., et al. (2014c). Impact of Campylobacter jejuni cj0268c knockout mutation on intestinal colonization, translocation, and induction of immunopathology in gnotobiotic IL-10 deficient mice. PLoS ONE 9:e90148. doi: 10.1371/journal.pone.0090148
Hoy, B., Geppert, T., Boehm, M., Reisen, F., Plattner, P., Gadermaier, G., et al. (2012). Distinct roles of secreted HtrA proteases from gram-negative pathogens in cleaving the junctional protein and tumor suppressor E-cadherin. J. Biol. Chem. 287, 10115–10120. doi: 10.1074/jbc.C111.333419
Hoy, B., Löewer, M., Weydig, C., Carra, G., Tegtmeyer, N., Geppert, T., et al. (2010). Helicobacter pylori HtrA is a new secreted virulence factor that cleaves E-cadherin to disrupt intercellular adhesion. EMBO Rep. 11, 798–804. doi: 10.1038/embor.2010.114
Hu, L., Bray, M. D., Osorio, M., and Kopecko, D. J. (2006). Campylobacter jejuni induces maturation and cytokine production in human dendritic cells. Infect. Immun. 74, 2697–2705. doi: 10.1128/IAI.74.5.2697-2705.2006
Jagusztyn-Krynicka, E. K., Łaniewski, P., and Wyszynska, A. (2009). Update on Campylobacter jejuni vaccine development for preventing human campylobacteriosis. Expert Rev. Vaccines 8, 625–645. doi: 10.1586/erv.09.21
Jiang, J., Zhang, X., Chen, Y., Wu, Y., Zhou, Z. H., Chang, Z., et al. (2008). Activation of DegP chaperone-protease via formation of large cage-like oligomers upon binding to substrate proteins. Proc. Natl. Acad. Sci. U.S.A. 105, 11939–11944. doi: 10.1073/pnas.0805464105
Kist, M., and Bereswill, S. (2001). Campylobacter jejuni. Contrib. Microbiol. Immunol. 8, 150–165. doi: 10.1159/000060405
Krojer, T., Sawa, J., Schäefer, E., Saibil, H. R., Ehrmann, M., and Clausen, T. (2008). Structural basis for the regulated protease and chaperone function of DegP. Nature 453, 885–890. doi: 10.1038/nature07004
Masanta, W. O., Heimesaat, M. M., Bereswill, S., Tareen, A. M., Lugert, R., Gro,ß, U., et al. (2013). Modification of intestinal microbiota and its consequences for innate immune response in the pathogenesis of campylobacteriosis. Clin. Dev. Immunol. 2013:526860. doi: 10.1155/2013/526860
Otto, B., Haag, L.-M., Fischer, A., Plickert, R., Küehl, A. A., Göebel, U. B., et al. (2012). Campylobacter jejuni induces extra-intestinal immune responses via Toll-like-receptor-4 signaling in conventional IL-10 deficient mice with chronic colitis. Eur. J. Microbiol. Immunol. 2, 210–219. doi: 10.1556/EuJMI.2.2012.3.7
Parkhill, J., Wren, B. W., Mungall, K., Ketley, J. M., Churcher, C., Basham, D., et al. (2000). The genome sequence of the food-borne pathogen Campylobacter jejuni reveals hypervariable sequences. Nature 403, 665–668. doi: 10.1038/35001088
Pielsticker, C., Glüender, G., and Rautenschlein, S. (2012). Colonization properties of Campylobacter jejuni in chickens. Eur. J. Microbiol. Immunol. 2, 61–65. doi: 10.1556/EuJMI.2.2012.1.9
Schubert, A., Wrase, R., Hilgenfeld, R., and Hansen, G. (2015). Structures of DegQ from Legionella pneumophila define distinct ON and OFF states. J. Mol. Biol. 427, 2840–2851. doi: 10.1016/j.jmb.2015.06.023
Warren, H. S., Fitting, C., Hoff, E., Adib-Conquy, M., Beasley-Topliffe, L., Tesini, B., et al. (2010). Resilience to bacterial infection: difference between species could be due to proteins in serum. J. Infect. Dis. 201, 223–232. doi: 10.1086/649557
Wu, X., Lei, L., Gong, S., Chen, D., Flores, R., and Zhong, G. (2011). The chlamydial periplasmic stress response serine protease cHtrA is secreted into host cell cytosol. BMC Microbiol. 11:87. doi: 10.1186/1471-2180-11-87
Keywords: Campylobacter jejuni, secondary abiotic IL-10−/− mice, serine protease activity, high-temperature requirement A (HtrA), host-pathogen-interaction, intestinal immunopathology, extra-intestinal and systemic immune responses, bacterial translocation
Citation: Schmidt A-M, Escher U, Mousavi S, Boehm M, Backert S, Bereswill S and Heimesaat MM (2019) Protease Activity of Campylobacter jejuni HtrA Modulates Distinct Intestinal and Systemic Immune Responses in Infected Secondary Abiotic IL-10 Deficient Mice. Front. Cell. Infect. Microbiol. 9:79. doi: 10.3389/fcimb.2019.00079
Received: 10 January 2019; Accepted: 08 March 2019;
Published: 29 March 2019.
Edited by:
Margaret E. Bauer, Indiana University Bloomington, United StatesReviewed by:
Arun K. Bhunia, Purdue University, United StatesSarah Maddocks, Cardiff Metropolitan University, United Kingdom
Copyright © 2019 Schmidt, Escher, Mousavi, Boehm, Backert, Bereswill and Heimesaat. This is an open-access article distributed under the terms of the Creative Commons Attribution License (CC BY). The use, distribution or reproduction in other forums is permitted, provided the original author(s) and the copyright owner(s) are credited and that the original publication in this journal is cited, in accordance with accepted academic practice. No use, distribution or reproduction is permitted which does not comply with these terms.
*Correspondence: Markus M. Heimesaat, bWFya3VzLmhlaW1lc2FhdEBjaGFyaXRlLmRl