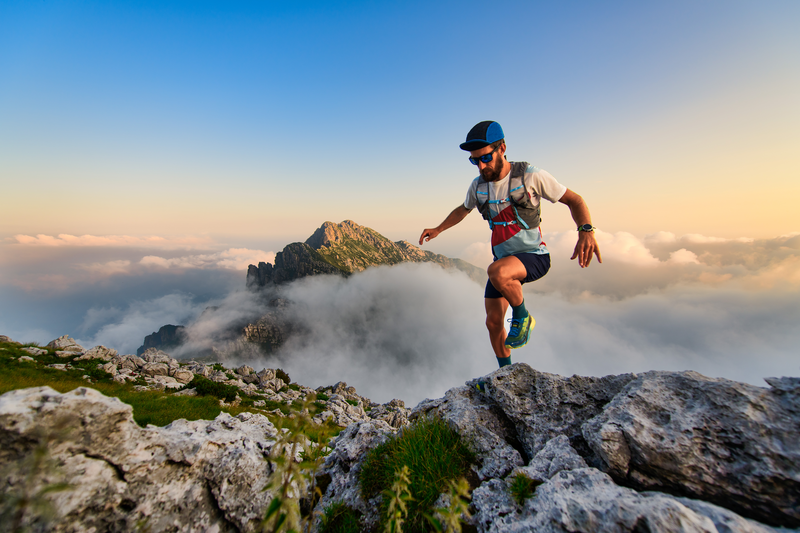
94% of researchers rate our articles as excellent or good
Learn more about the work of our research integrity team to safeguard the quality of each article we publish.
Find out more
ORIGINAL RESEARCH article
Front. Cell. Infect. Microbiol. , 12 March 2019
Sec. Molecular Bacterial Pathogenesis
Volume 9 - 2019 | https://doi.org/10.3389/fcimb.2019.00055
This article is part of the Research Topic Decoding the Streptococcal Language View all 6 articles
In the polymicrobial environment of the human nasopharynx, Streptococcus pneumoniae (pneumococcus) competes with other members of the microbial community for limited nutrients in part by secreting small peptide bacteriocins called pneumocins. Pneumocin production is controlled by a quorum sensing system encoded by the blp locus. Although the locus is found in all pneumococci, there is significant variability in the repertoire of pneumocins and associated immunity proteins encoded in the Bacteriocin Immunity Region (BIR) and in the presence or absence of a functional Blp transporter. Strains without an active Blp transporter are inactive in plate overlay assays and rely on a homologous transporter that is only produced during brief periods of competence to stimulate the blp locus and secrete pneumocins. The variability of the locus suggests that selective pressure is influencing the content to promote the optimal competitive environment. Much of the variability in the blp locus has been described at the genome level; the phenotypic activity attributable to the various BIR genes has not been fully described. To examine the role of the predicted pneumocin peptides in competition, 454 isolates were screened for competence independent blp pheromone secretion using plate assays. Active strains were characterized for inhibition, BIR content, BlpC pherotype and serotype. Deletion analysis on inhibitory strains demonstrated that BlpI and BlpJ peptides function as a two-peptide bacteriocin and that BlpIJ immunity is encoded by the co-transcribed blpU4/5 genes. BlpIJ secretion promotes inhibitory activity against the majority of pneumococcal isolates when expressed in a Blp transporter intact background. Intermediate levels of competition in biofilms were noted when BlpIJ containing strains carried the non-functional Blp transporter. Based on genome data, the combination of BlpIJ in a Blp transporter intact strain is surprisingly rare, despite clear advantages during colonization and biofilm growth. In contrast, we show that the blpK/pncF operon encoding the single-peptide pneumocin BlpK and its immunity protein is found in the majority of isolates. Unlike, BlpIJ and BlpK were shown to promote a limited spectrum of inhibition due in part to immunity that is independent of activation of the blp locus.
Streptococcus pneumoniae (pneumococcus) is a member of the human nasopharyngeal microbial community that can cause a wide spectrum of local and disseminated disease when mucosal barriers are breached. Because colonization precedes infection, survival in the nasopharynx is a crucial step in the pathogenesis of pneumococcal disease. The human nasopharyngeal microbial community is composed of a dynamic array of different bacterial species (Teo et al., 2015; Bosch et al., 2017; Kelly et al., 2017). Pneumococcal colonization is established during infancy and peaks in daycare age children (Teo et al., 2015; Bosch et al., 2017; Kelly et al., 2017). To survive in this polymicrobial environment and to compete for limited nutrients provided by the host, pneumococcus must actively compete with other members of the microbiome. One means of competition is through the secretion of bacteriocins, small antimicrobial peptides that typically target other closely related bacteria by disrupting either cell wall formation or membrane integrity. Bacteriocin secretion can eliminate competitors, freeing up valuable nutrients and space. Bacteriocin production is typically tightly regulated presumably to ensure that the peptides are only made under advantageous conditions (Nes et al., 1996; Wescombe et al., 2006; Cornforth and Foster, 2013; Maricic et al., 2016; Wholey et al., 2016; Shanker and Federle, 2017).
Pneumococci have been shown to encode a wide variety of bacteriocins, however, only a few loci have been shown to be inhibitory (Bogaardt et al., 2015). Inhibitory bacteriocins include the blp encoded pneumocins, the CibAB bacteriocin pair, and the pneumolancidins (Guiral et al., 2005; Dawid et al., 2007; Bogaardt et al., 2015; Maricic et al., 2016). The pneumocins are encoded by the blp locus, which is present in all sequenced pneumococcal isolates (Bogaardt et al., 2015; Miller et al., 2016). The blp locus contains genes that encode a peptide pheromone (BlpC), a two-component regulatory system (BlpRH), a dedicated transporter (BlpAB), a variable array of bacteriocin and immunity proteins within the bacteriocin immunity region (BIR), and some conserved accessory proteins whose functions are unknown but may play a role in immunity (de Saizieu et al., 2000; Dawid et al., 2007; Lux et al., 2007). The operons of the blp locus are upregulated in response to accumulation of the secreted extracellular BlpC pheromone, which activates the two-component system upon binding to the BlpH receptor. BlpC bound BlpH stimulates a phosphor-transfer reaction that results in an activated BlpR regulator. Activated BlpR binds to promoters in the locus and upregulates the operons in the BIR and the blpABC operon resulting in pneumocin secretion and positive feedback activation of the locus via increased pheromone secretion.
Although the blp locus itself is found in all pneumococcal genomes, there is significant variability in the content of the locus. There are four major allelic variants of the gene encoding the BlpC pheromone, designated BlpC164, BlpCR6, BlpC6A, and BlpCT4 in addition to minor variants found in a small number of strains (de Saizieu et al., 2000; Reichmann and Hakenbeck, 2000; Miller et al., 2016). Each pheromone is typically associated with a specific receptor blpH allele which, in general, is restricted to respond maximally to one BlpC type (Pinchas et al., 2015). In addition, the BIR is characterized by significant diversity in bacteriocin content with 16 different putative pneumocin peptides identified to date arranged into one or two operons (Lux et al., 2007; Bogaardt et al., 2015). Many of these bacteriocins are predicted to be classified as type-IIB bacteriocins which are unmodified, two-peptide bacteriocins. Using a bioinformatics approach a recent study analyzed 4,096 distinct pneumococcal genomes categorizing genes in the BIR into a few putative functional groups based on shared bacteriocin content (Miller et al., 2016). The study concluded that the strong association between two bactericin genes could be used to predict the likelihood that they function as a pair although the predicted associations were not tested experimentally. As an example relevant to this study, there was a significant association found between the putative bacteriocin-encoding gene blpK, and the bacteriocin genes blpI, blpM, blpN, and pncW. Based on these associations, the authors speculated that the gene products may function as pairs in a two peptide bacteriocin.
For any given pneumococcus, the blp locus will encode one receptor/pheromone pair, and may contain as many as six bacteriocin genes along with their cognate immunity genes. This degree of diversity in signaling and inhibitory activity suggests that any two pneumococcal isolates might compete in any number of different ways. From a functional standpoint, only the type 6A allelic variants of the bacteriocins BlpM and BlpN have been experimentally shown to have inhibitory activity and to act together as a two-peptide bacteriocin (Dawid et al., 2007). The TIGR4 variants of BlpM and BlpN that differ from the 6A type by only 3 and 2 amino acids, respectively, have no detectable inhibitory activity in overlay assays. Whether all pneumocin peptides form two-peptide bacteriocins or whether peptides can form two peptide pairings with a variety of peptides has not been tested experimentally.
Genome data and functional analysis of strain collections have demonstrated that approximately 25% of the population encode a full length, functional BlpA transporter (BlpA+) (Miller et al., 2016). The majority of remaining BlpA non-functional (BlpANF) strains have a conserved frame shift (BlpAFS) mutation or a large deletion in the blpA transporter gene (Son et al., 2011; Miller et al., 2016). These strains lack evidence of both pheromone and pneumocin secretion in agar overlay assays but retain the capacity to upregulate the locus in response to matched exogenous pheromone secreted by neighboring strains with an intact transporter gene. In addition, BlpANF bacteria are able to secrete and respond to their own BlpC under competence-inducing conditions by utilizing the alternative competence transporter, ComAB (Kjos et al., 2016; Wholey et al., 2016). The ComAB transporter can secrete pneumocins in addition to the BlpC pheromone, however, the period of secretion is limited to times of competence activation (Wang et al., 2018). BlpA+ strains have a competitive advantage during co-colonization of the mouse nasopharynx when they are competed with pneumocin sensitive strains when compared with otherwise matched BlpANF strains (Wang et al., 2018). In addition, competence induced blp activation is not sufficient to protect BlpANF strains from bacteriocin mediated inhibition during mouse colonization (Wang et al., 2018). It is unclear what advantage is gained by inactivation of the BlpAB transporter, however, given the prevalence of this phenotype, it has been hypothesized that BlpANF strains enjoy a fitness advantage over BlpA+ strains under non-competitive conditions.
To further understand the role of the blp locus in the competitive dynamics among pneumococci, we aimed to identify pneumocin genes associated with in vitro evidence of inhibition by screening a large pneumococcal collection for plate overlay evidence of BlpC pheromone secretion which is only seen in BlpA+ isolates under the conditions tested. Pheromone secretors were identified and analyzed for serotype, BIR content, and further screened for evidence of inhibition on agar overlay assays. Bacteriocin genes associated with inhibitory activity included the previously described blpMN6A alleles and the genes encoding the putative bacteriocins BlpI, BlpJ, and BlpK. We show that BlpIJ function as a two-peptide bacteriocin that is associated with potent anti-pneumococcal inhibition. BlpK appears to function alone, however, most pneumococcal strains are immune to this bacteriocin due to BlpRH dependent and independent production of BlpK immunity. Despite the competitive advantage of BlpIJ secretion in a BlpA+ background in mouse colonization and biofilm growth and the ability of such strains to inhibit the majority of pneumococci, this combination is only rarely found in pneumococcal genomes suggesting a fitness defect within the host.
All strains and plasmids used in this study are described in Table 1, all primers listed in Table S1. Pneumococcal strains were grown in Todd-Hewitt broth supplemented with 0.5% yeast extract (THY) or on tryptic soy agar (TSA) plates supplemented with 5 μg/ml of Catalase (Worthington, Lakewood, NJ, USA) or 5% sheep blood agar plates (SBA) and incubated at 37°C in 5% CO2. Starter cultures were prepared for use in broth growth by cultivating in THY medium to an OD620 of 0.5 and then freezing in 20% glycerol. Thawed frozen starter culture were diluted 1/50 into C+Y pH 8.0 (Supplemental Material) for transformation, 1/25 into CDM media (Supplemental Material) for biofilm experiments; or 1/50 into THY for murine colonization studies. For transformation, the pneumococcal culture was allowed to reach OD620 = 0.15 in C+Y and further diluted 1/10 into fresh C+Y containing 1 μg/mL synthetic CSP1+2 (95% purity, Genescript, Piscataway, NJ) for 10 min at 30°C. Approximately 100 pg/ml of DNA was added to the mixture for an additional 40 min at 30°C. The culture was then incubated for 1 h at 37°C before being plated on selective media. Escherichia coli strains were grown in Luria-Bertani (LB) broth or LB agar supplemented with the appropriate antibiotics at 37°C. Antibiotic concentrations used were as follows: for pneumococcus 500 μg/ml kanamycin, 100 μg/ml streptomycin, 2 μg/ml chloramphenicol, 200 μg/ml spectinomycin, and 1 μg/ml erythromycin; and for E. coli, 50 μg/ml kanamycin, 20 μg/ml chloramphenicol, and 100 μg/ml spectinomycin.
We screened a total of 454 pneumococcal isolates from three collections including: 381 clinical specimens collected between the years 2004–2006 from the Microbiology laboratory at the University of Michigan Health Systems located in Ann Arbor, Michigan, 22 pneumococcal isolates from a previous study of colonization patterns from daycare attendees in Ann Arbor, Michigan (St Sauver et al., 2000); and 54 previously described invasive and colonizing isolates from South Africa (Son et al., 2011) (IRB references in Supplemental Material). Both of the daycare and the South Africa collections were collected before PCV7 vaccine was introduced in those locations. The University of Michigan clinical isolates collection consists of samples isolated from blood, CSF, upper and lower respiratory secretions, pleural fluid, synovial fluid, bone, and other tissues and identified by standard culture techniques as Streptococcus pneumoniae. Pneumococci were serotyped by multiplex PCR using oligonucleotide primers for detecting 41 pneumococcal serotypes as per the US isolate scheme (da Gloria Carvalho et al., 2010), Quellung method and latex agglutination were performed for verification using specific antisera (Statens Serum Institut, Copenhagen, Denmark).
The DNA fragments of BIR were PCR amplified using primers 1 and 2, which bind to the highly conserved regions within blpA and blpY genes, respectively. PCR products were purified and the product was digested with restriction enzyme AseI. The size pattern of each isolate was compared to the predicted patterns from the known distinct BIR regions from the sequence database (Table S2). Isolates with identical patterns were assigned to the same group. We confirmed the absence of a blpA repeat or deletion in all strains with pheromone signaling as follows: a 761 bp region of blpA was amplified with primers 3 and 4. The product was digested with enzyme Cac8I, which will only cleave a product containing the common frame shifting 4 bp repeat (AAGC) in the blpA gene. The DNA fragment containing a frame shift repeat would separate into two pieces of fragments while the wild type sequence would remain intact as a single piece fragment.
Construction of deletion mutant strains can be found in the Supplemental Material.
Overlay assays were performed as previously described (Maricic and Dawid, 2014). Briefly, strains to be assayed for inhibitory activity were spiked into TSA plates supplemented with 5 μg/ml of catalase using small sterile pipet tips. Plates were incubated for 5 h at 37°C in 5% CO2 to allow for growth. A volume of 300 μl of the overlay strains grown to an OD620 of 0.3–0.5 was added to a mixture of 100 μl of 1 mg/ml catalase, 5 ml of THY and 3 ml of molten TSA. This soft agar mixture was carefully dripped over the top of the spiked plate, which was then incubated overnight at 37°C in 5% CO2. For inhibitory overlay, the killing activity was indicated by a zone of clearing around the spiked site and the immunity was indicated by the growth surrounding the spiked bacteriocin producer. For BlpC pheromone secretion overlays, different BlpH type BIRlacZ reporters were used as overlay strains (Pinchas et al., 2015). Reporter strain PSD108 for BlpC6A, strain PSD101 for BlpCR6, strain PSD121 for BlpC164, and strain PMP100 for BlpCT4. A volume of 40 μl of X-Gal at 40 mg/ml was added to the reporter-containing soft agar before pouring. Detection of a blue zone indicates BlpC secretion is sensed by the BIRlacZ reporter strain. All overlays were repeated at least three separate times with strains spiked in triplicate to ensure reproducibility. Photos in figures are representative results.
Establishment of a biofilm was performed as previous described (Marks et al., 2012). Briefly, pneumococcal D39 derivative cultures were diluted 1/25 into chemical defined media (CDM), grown to an OD620 of 0.5, then further diluted 1:500 into pre-warmed 34°C CDM. Pneumocin producer (PSD300 SpR), pneumocin producer with the blpAF.S mutation (PSD309 SpR), pneumocin producer with ΔblpI strain (PSD312 SpR), pneumocin producer with ΔblpSRHC strain (PSD311 SpR), or non-producer (PSD299 SpR) was co-inoculated with D39 sensitive (PSD313 KanR) strains and allowed to form a biofilm on fixed H292 human lung epithelial cells grown on top of glass coverslips at 34°C + 5% CO2 in 24 well plates. Growth media was changed every 12 h for 3 days. For recovery of the biomass, each well was washed with PBS three times and the samples were resuspended in a final volume of 500 μl of PBS. Plates were sealed with parafilm and sonicated for 12 s in a sonication bath to disperse the biofilm. Bacterial composition of the biofilms was determined by CFU counts on single or dual selective plates. Competitive index was calculated by dividing the output ratio to the input ratio. Data from 3 independent experiments were pooled together. Kruskal-Wallis tests for multiple comparisons were performed to determine statistical significance as indicated.
To identify genomes from previously defined collections predicted to encode a full length BlpA, the BlpA protein sequence from strain P1031 was used in the BLAST search engine and the search was limited to the Bioprojects of the well-described Maela collection PRJEB2357, PRJEB2393, PRJEB2395, PRJEB2397, PRJEB2479, or PRJEB2480. This collection consists of the genome sequences of 3,085 colonizing isolates from infants and mothers residing in a refugee camp in Maela near the border of Myanmar and was described in Chewapreecha et al. (2014). All matches of at least 95% identity with the full-length protein were selected, this eliminated ComA sequences, as this homolog shares approximately 66% identity across the length of the protein. Duplicated sequences for an individual strain (due to repeated content in contigs) were eliminated by matching each accession number with its strain designation; any duplicated strains were counted only once. To identify the blpI, pncBC, blpJ region, the DNA encoding the four ORFs, 934nt from the prototypic strain TIGR4 was blasted against the above Bioprojects. Assemblies with 99–100% identity in the entire length of that fragment were used to obtain the matching strain designation. Some strains were not counted because contigs were too short to include the entire cluster. Inclusion was confirmed by protein blast against the BlpI sequence. As above, duplicated strains were counted once. Strains with matches but not appearing in Table S1 from Chewapreecha et al. (2014) were eliminated from consideration. Information of strain serotype was deduced from Table S1 of Chewapreecha et al. (2014). An identical approach was used to screen the Boston isolate collection, a collection of colonizing strains from children residing in Boston just after the release of PCV7 (Croucher et al., 2013, 2015). However, in this case, only full length BlpA and BlpI were individually searched for in the Bioproject containing all of the Boston isolate genome data, PRJEB2632. The BlpK sequence was searched for using an identical strategy in Bioproject PRJEB2632 alone. Using an identical approach, the prevalence of full length BlpA was determined in the NT collection by searching in Bioproject PRJEB2340 (Hilty et al., 2014).
To characterize active pneumocin production locus in the disease-causing and colonizing Streptococcus pneumoniae population, we screened 454 isolates from three collections: (1) 22 distinct isolates isolated from the nasopharynx of daycare attending children in the pre-PCV7 era (St Sauver et al., 2000), (2) 381 clinical isolates that were identified as pneumococcus in the microbiology laboratory at the University of Michigan Health System during a period between 2004 and 2006, (3) 51 previously described colonizing and invasive isolates from South Africa (Son et al., 2011). Using pheromone type specific reporters in overlay assays (Pinchas et al., 2015), we identified 48 strains (11%) with active BlpC pheromone secretion listed in Table 2, consistent with a blp locus that was functional under the in vitro conditions tested. The four reporter strains used in the overlays detect the four major BlpC types: 164, R6, 6A, and T4. Because BlpC6A can cross stimulate BlpHT4 reporters, strains were designated as BlpC6A secretors if they stimulated BlpH6A or BlpHT4; strains that could only stimulate the T4 reporter were designated as BlpCT4 secretors (Pinchas et al., 2015). BlpC secreting strains were further characterized for capsule type, blpA integrity and BIR content using PCR and RFLP analysis which was used to assign bacteriocin content based on AseI digest patterns predicted for known loci.
Table 2. Characteristic of strains with active blp loci identified from clinical and colonizing isolate collections.
The 48 strains with blp loci that were functional for pheromone secretion in plate assays were separated into 9 groups based on RFLP predicted bacteriocin content (Table 2, Table S2). In some cases, whole or portions of the BIR were sequenced for confirmation. We tested the inhibitory activity of all stains using plate overlay assays against complete blp deletion mutants in two distinct genetic backgrounds: 6AΔblpT-X and 19AΔblpT-X. Both carry an identical allelic replacement that removes the entire blp locus, including all of the genes encoding regulatory and secretion proteins in addition to the entire BIR to the start codon of blpY (Maricic and Dawid, 2014).
Five BIR groups had evidence of inhibitory activity on plate overlay assays. For inhibitory groups 1–3, all were predicted to encode the putative pneumocins BlpI and BlpJ in the first operon. Both group 1 isolates had been previously sequenced. This group has two unique putative bacteriocins (here referred to as BlpW1P164 and BlpW2P164) following the blpIJ genes. The only group 2 isolate was also fully sequenced. This isolate only encodes predicted bacteriocins in the first of three BIR operons, this operon contains the blpIJK cluster. The Group 3 BIR has blpIJK followed by a second operon with blpMNO. Group 4 has BlpK alone in the first operon followed by BlpMNO. Group 5 had BlpMNO as its first and only operon and had a mixture of inhibitory and non-inhibitory strains. The blpMN alleles of group 5 were sequenced to determine if allelic differences in the bacteriocins accounted for the activity. Consistent with previous results, inhibitory activity attributable to BlpMN was only noted in strains with the blpMN6A alleles.
For the non-inhibitory groups 6 and 7, both are predicted to encode BlpQ in the first operon followed by either BlpMNO or BlpMNW, respectively, in the second operon. We sequenced a selection of blpMN alleles in these strains and none carried the MN6A alleles. The members of groups 8 and 9 were non-inhibitory and had a BIR RFLP with no match with the known sequence database and were not further analyzed.
We chose strains P164, P140, and A76 for further deletion analysis given their clear, blp dependent inhibitory activity on overlay plates and distinct BIR content. Group 5 isolates were not chosen for further analysis given the previously established association of the blpMN6A alleles with inhibition. The BIR content of strains P164 and P140 was previously confirmed by sequencing (Son et al., 2011). BIR content for strain A76 was confirmed by sequencing in the current study and was found to be identical to the locus found in the sequenced strain Inv200 (GenBank FQ312029). The BIR regions of P140, P164, and A76 were moved into an otherwise isogenic, non-inhibitory, genetically tractable, 19A serotype background to ensure that any inhibitory activity was derived from the blp locus.
The BIRP164 contains some unique genes that may influence either inhibitory activity or immunity (Son et al., 2011). The first operon in the BIR encodes the common putative bacteriocins, BlpI and BlpJ followed by two unique bacteriocin genes, blpW1/2P164 and a predicted protease (here called BlpGP164). The second operon encodes a tdpA-like gene predicted to encode a thioredoxin fold domain containing protein (Figure 1A). Both blpW1/2P164 genes are predicted to encode a peptide with a leader sequence followed by a double glycine motif typical of bacteriocins but no homology was identified in the C-terminal structural peptide. BlpGP164 is predicted to be an Abi type metallo-protease. Abi proteases are found within the BIR in a few sequenced genomes and at the end of the blp locus in nearly all strains (BlpY and PncP) (Bogaardt et al., 2015). These proteins have been proposed to confer bacteriocin immunity by processing or degrading peptides, although functional evidence is lacking (Kjos et al., 2010). The sequence of BlpGP164 is distinct from all other sequenced forms of BlpG. The TdpA-like protein contains an LPXTG motif, which predicts attachment to the cell wall for expression on the outer cell surface. Because the TdpA-like protein in P164 contains a thioredoxin domain and cell membrane anchor, we hypothesized that it may have a role in enhancing the function of secreted bacteriocins by forming intra or inter-molecular disulfide bonds.
Figure 1. Functional analysis of the BIR region of P164 strain after moving into a 19A strain background. (A) Schematic representation of BIRP164. Blue arrows indicate predicted pneumocins, and green arrows indicate predicated immunity proteins. Black arrows indicate ORF encoding proteins of unclear significance. Curved arrows represent predicted transcriptional start sites based on the presence of a predicted BlpR binding site. (B) Agar overlay assays showing inhibitory and immune phenotypes. The properties of strains spiked into the plate are shown below the photo, overlay strains designations are shown above the photo.
To determine the role of each of the P164 BIR ORFs, we created a series of deletion mutants of P164 BIR in the 19A serotype background strain and tested these in overlay assays for inhibition and sensitivity. Pheromone secretion was confirmed in all mutants to ensure that the blp locus was active. Unmarked in-frame deletion mutants were created in blpI and blpW1/2P164. Insertion mutants were created in blpGP164 and the tdpA homolog. Strains were spiked into agar plates and overlaid with 6AΔblpT-X and 19AΔblpT-X to evaluate for inhibition while strains were inoculated into the overlay over spikes of strains with the intact locus to evaluate for immunity. The 19A-BIRP164 with an in-frame unmarked deletion of the blpI gene (ΔblpI strain) lost all inhibitory activity, suggesting that BlpI is at least one component of a functional pneumocin and that the BlpW1/2 peptides do not contribute to inhibition in the absence of BlpI (Figure 1B, upper). Strains lacking both blpW1/2P164 genes (ΔblpW strain) showed no change in inhibitory activity, suggesting that the product of these genes do not contribute to inhibitory activity (Figure 1B). Neither disruption in blpG or tdpA altered inhibitory activity or immunity (Figure 1B). These results demonstrate that the accessory proteins do not play an appreciable role in the inhibitory activity or immunity of the P164 locus.
P140 is a colonizing isolate from the South African strain collection that was characterized by inhibition of the majority of pneumococci in the collection (Son et al., 2011). This strain has the bacteriocin cluster blpIJK in the first and only operon in the BIR (Figure 2A). Unmarked in-frame deletions were created in the 19A background to determine which genes in the BIR140 were required for inhibition and immunity. Strains with ΔblpIJK, ΔblpIJ or ΔblpI and ΔblpJ had no inhibitory activity against the 19AΔblpT-X strain. The ΔblpK strain alone showed no defect in inhibitory activity (Figure 2B). When mutants with ΔblpI or ΔblpJ were spiked adjacent to each other on a plate, a zone of inhibition was seen in the region where the diffusion zones would overlap (Figure 2C). These results demonstrate that BlpIJ function as a two peptide bacteriocin where both peptides are required for inhibition. Because we did not perform biochemical analysis of the peptides, their active structure is not known, however, there are no modification enzymes within the locus that would predict post-translational modifications.
Figure 2. Functional analysis of the BIR region of P140 strain after moving into a 19A strain background. (A) Schematic representation of BIRP140. Blue arrows indicate predicted pneumocins and green arrows indicate predicated immunity proteins. Black arrows indicate ORF encoding proteins of unclear significance. Curved arrows represent predicted transcriptional start sites based on the presence of a predicted BlpR binding site. (B–D) Agar overlay assays showing inhibitory and immune phenotypes. The properties of strains spiked into the plate are shown below the photo, overlay strains designations are shown above the photo.
Producer bacteria are protected from the effects of their own bacteriocins via the production of specific immunity proteins that are typically co-transcribed with the genes encoding the bacteriocins (Jack et al., 1995). All strains with a deletion in the blpU4-U5 genes found between the blpI and blpJ ORFs were inhibited by BlpIJ pneumocin producing strains (Figure 2D), indicating that one or both encode immunity peptides that protect against BlpIJ. Consistent with this, the ΔblpJ deletion strain can inhibit the growth of the ΔblpI-U5 strain despite lacking inhibitory activity against any other strain including the ΔblpT-X strain. This finding demonstrates that the complementation between secreted BlpI (from the ΔblpJ spiked strain) and BlpJ (from the ΔblpI-U5 overlay strain) occurs in the overlay and the overlay strain is inhibited because it lacks blpU4/U5 immunity for self-protection (Figure 2D).
A76 is a serotype 14 strain that was isolated from blood. The A76BIR has two bacteriocin operons, one encoding blpK and the second with blpMNO (Figure 3A). Overlay assays on the serotype 19A transformant carrying the A76 blp locus showed that this strain lacked inhibitory activity against the 19AΔblpT-X deletion strain but had clear activity against the 6AΔblpT-X background (Figure 3B). We observed the same pattern of inhibition using the 19ABIRP140 strains lacking blpIJ that would express only the BlpK bacteriocin. This inhibitory activity was not seen in the 19ABIRP140 strain with a deletion in blpIJK. This result suggested that BlpK bacteriocin expression in the 19ABIR140 ΔblpIJ strain and the 19ABIRA76 strain is responsible for inhibition of the 6A serotype ΔblpT-X strain. Of note, the BIRA76 encodes the blpMNT4 allele that lack inhibitory activity in all other backgrounds. In agreement with this, the deletion of the blpK gene in strain 19ABIRA76 resulted in a complete loss of inhibitory activity in plate overlay assay against the 6AΔblpT-X strain (Figure 3C). These results demonstrated that BlpK is a functional pneumocin that is responsible for the inhibitory activity of strain 19ABIRA76 and 19ABIR140ΔblpIJ against the 6AΔblpT-X strain. Given that 19ABIR140 ΔblpIJ only contains the bacteriocin blpK gene in the BIR, we concluded that the BlpK pneumocin peptide does not pair with another bacteriocin and functions alone in providing the observed inhibitory activity.
Figure 3. Functional analysis of the BIR region of A76 strain after moving into a 19A strain background. (A) Schematic representation of BIRA76. Blue arrows indicate predicted pneumocins, and green arrows indicate predicated immunity proteins. Black arrows indicate ORF encoding proteins of unclear significance. Curved arrows represent predicted transcriptional start sites based on the presence of a predicted BlpR binding site. (B,C) Overlay assays showing the inhibitory activities of BlpK-expressing stains. The properties of strains spiked into the plate are shown below the photo, overlay strains designations are shown above the photo.
Our inhibitory overlay analysis showed that the 19AΔblpT-X strain is immune to BlpK expressing strains while the 6AΔblpT-X strain is inhibited (Figure 3B). Because both pneumocin sensitive strains carry identical deletions of the entire blp locus spanning from upstream of blpT to just 5' of blpY including all regulatory, secretion and BIR genes; the immunity to the BlpK bacteriocin in the 19A background must be independent of the blp locus and BlpC/H signaling. Many pneumococcal genomes have two nearly identical copies of blpK and its co-transcribed gene pncF (Lux et al., 2007). One copy is often found in the first operon of the BIR as in A76 and P140 (here called blpKin), and the other copy is found distant from the blp locus (here called blpKout). In many studied genomes, blpKout/pncFout is found adjacent to the genes encoding the competence transporter comAB. We sequenced the blpKout/pncFout region of each deletion strain and found that the 6AΔ blpT-X strain that is inhibited by the BlpK expressing strains would be predicted to encode a truncated BlpKout due to a premature stop codon within the coding sequence. The 19AΔ blpT-X deletion strain that is not inhibited by BlpK expressing strains carried an intact blpKout ORF. Although all blp genes were originally identified by their activation by the BlpR regulator, previous work suggested that some transcription of blpKout/pncFout might be independent of the activity of the blp locus (Lux et al., 2007). This group showed that transcription of the blpKout gene could be appreciated in strains carrying a disruption of the BlpR regulator (Lux et al., 2007). Given the sequence identity between pncFout and pncFin and their presumed role encoding BlpK specific immunity proteins, we hypothesized that in the 19AΔblpT-X strain, PncFout can serve as an immunity protein against the inhibitory activity of the BlpKin bacteriocin. The disruption in transcription/translation of the mutated blpKout gene in the 6AΔblpT-X strain might result in a decrease or lack of expression of pncFout, explaining its sensitivity to BlpK. To test this, we created a deletion of both blpKout and pncFout genes in the 19AΔblpT-X strain (19AΔblpT-X,Kout) and tested this strain's immunity activity against BlpK expressing strains. Strain 19AΔblpT-X,Kout was found to be susceptible to inhibition by the BlpK expressing 19ABIRA76 and 19ABIRP140 ΔblpIJ strains (Figure 3B). This result demonstrates that PncFout can serve as the immunity protein for the BlpKin pneumocin and that pncFout derived immunity is effective even when the regulatory genes controlling the blp locus are inactive. The 6A wildtype (blp+) strain does not encode BlpKin but showed immunity against both 19ABIRA76 and 19ABIR140ΔIJ strains (Figure 3B), suggesting that the immunity protein encoded in pncFout downstream of the truncated blpKout gene might only be produced in sufficient quantities to provide protection when the locus is upregulated by BlpR. It is also possible that there is another general immunity protein encoded in the blp locus.
To test the possibility that inhibitory BlpKin activity is augmented by BlpKout expression, we removed the blpKout/pncFout genes from 19ABIRP140 ΔblpIJ strain that contained only blpKin in the BIR. This mutant showed no change in inhibition against 6AΔblpT-X sensitive strain compared with its parent 19ABIRP140ΔblpIJ strain (Figure 3D). This result confirmed that BlpKin mediated inhibition is independent of BlpKout expression. In addition, because the 19ABIR164ΔblpI strain and the 19ABIR140ΔblpIJK strains lack evidence of inhibition on plate overlay assays, there is no evidence that BlpKout expression alone is sufficient for detectable in vitro inhibition.
Our results so far have identified two functional pneumocins that are responsible for the inhibitory activity of three pneumococcal isolates. Because the majority of the pneumococcal population encodes the blpKout/pncFout genes (Miller et al., 2016), BlpK pneumocin would be predicted to play less of a role in pneumococcal/pneumococcal competition. BlpIJ producing strains would be expected to inhibit any strain that lacks blpU4/5 genes or contains these genes but fails to express them. To determine the spectrum of BlpIJ mediated inhibition, we tested the 19ABIRP164 strain against 368 of the clinical isolates from University of Michigan in overlay assays. Strains that did not grow well in overlays were excluded from the analysis (n = 13 isolates). The majority of pneumococcal isolates were inhibited by BlpIJ pneumocin produced by 19ABIRP164 strain. Only a small subpopulation of 46 isolates (12.5%) were found to be immune. We examined these isolates for the presence of the blpU4/5 genes using restriction digestion of PCR amplified DNA region from blpA to start of blpJ gene. Forty-three immune isolates (11.7% of total) were predicted to encode blpU4/5 genes. Only three strains had undefined immunity against the BlpIJ pneumocin.
To determine the spectrum of inhibition against other gram positive organisms, BlpIJ producing strains were tested in overlay assay against representatives of several Streptococcal species and other gram positive organisms (Table 3). D39BIRP140, expressing BlpIJ and BlpK was able to inhibit all tested S. pyogenes strains, S. mitis, S. oralis and Lactococcus lactis but not S. gordonii, S. mutans, S. sobrinus, S. agalactiae, Enterococcus faecalis, or Listeria monocytogenes. Inhibition of S. pyogenes and mitis was dependent on the expression of the BlpIJ bacteriocins as strains with individual blpI or blpJ deletions lost inhibition in overlay assays. The inhibition of L. lactis and S. oralis is dependent on a functional blp locus as no inhibition was noted in the 19AΔblpT-X background or a background in which 19ABIRP140 contained a BlpA frameshift mutation. This inhibition, however, did not require any of the known blp bacteriocins as strain 19ABIRP140ΔblpI-kin kout lacking all known blp bacteriocins retained inhibition of both strains. This suggests that bacteriocins encoded outside of the blp locus can be secreted by the BlpAB transporter in this strain background or that the inhibition is not mediated by bacteriocins but by some other, unidentified factor produced during activation of the locus. These possibilities are currently being explored.
Pneumococcal strains are divided into BlpA-intact (BlpA+) and BlpA non-functional (BlpANF) backgrounds with an approximate distribution of 25 and 75%, respectively. In plate assays, only BlpA+ strains demonstrate evidence of activation of the locus either via pheromone secretion or inhibition. Because BlpANF strains require competence induction for activation of the blp locus through their requirement for pheromone and bacteriocin secretion through the ComAB transporter (Kjos et al., 2016; Wholey et al., 2016), we hypothesized that plate inhibition assays might be unable to assess any bacteriocin mediated competition in these strains because the plate assays are not conducive to competence development. To determine the role of the BlpIJ pneumocin in competition in both BlpA+ and BlpANF backgrounds we performed competitive colonization studies using a previously described biofilm assay that was shown to support high levels of DNA exchange through natural spontaneous competence induction (Marks et al., 2012; Wholey et al., 2016). Because the 19A strain background did not form robust biofilms, all modified and unmodified blp loci were moved into the non-inhibitory D39 background, replacing the original blp locus. D39BIRP164 BlpIJ pneumocin producer strain carrying spectinomycin resistance was co-inoculated with the wildtype D39 sensitive strain carrying a kanamycin cassette (D39kan). The native D39 blp locus is non-inhibitory because it has both a blpAFS (frame shift mutation in blpA gene), and a truncated BIR that lacks functional pneumocins. To ensure that any competition noted is mediated by the blp locus, we included as controls D39spec and versions of D39BIRP164 lacking either the regulatory genes (ΔblpSRHC) or the pneumocin gene (ΔblpI). Biofilms were harvested and plated on selective media after 3 days of incubation. Individually inoculated strains had similar recovered biomass from the biofilm indicating that these mutations did not alter the bacteria's ability to colonize and form a biofilm (Figure 4A). We had previously shown in plate inhibitory overlay assays that D39BIRP164 inhibited the growth of the parent D39 strain (Wholey et al., 2016). When inoculated into the biofilm, D39BIRP164 similarly out-competed D39kan (Figure 4B). Significant differences in competitive index compared with the D39spec/D39kan pairing were not observed when D39BIRP164ΔblpI or D39BIRP164ΔblpSRH were paired with D39kan. When the D39BIRP164blpANF strain was competed with D39kan, the competitive index was statistically higher than that noted with the D39spec or ΔblpSRHC strain, however, the median competitive index value of this blpANFstrain was significantly less than that noted with the blpA+ strain (Figure 4B). Transformants with dual spectinomycin and kanamycin resistance were recovered in all co-inoculated pairs confirming that the competence system was induced during biofilm formation (Figure 4C). These data demonstrate that even under competence permissive conditions, BlpIJ expressing strains with an intact BlpAB transporter have a competitive advantage over BlpANF strains that rely on the ComAB transporter for activation.
Figure 4. Pneumocin BlpIJ production in a BlpA+ intact strain promotes competition in dual-colonized biofilm. (A) Biomass of biofilm when strains were inoculated alone. (B) Competitive index when competitors were co-inoculated with a sensitive strain (D39kanR). (C) Dual Kan/Spect resistant transformants per well. LOD was 2.5 CFU/mL. There were no statistical differences between groups in A and C. Median values of 3 independent experiments are shown for each graph. Kruskal-Wallis test for multiple comparisons was used to determine statistical significance. P-values: **0.0015, ***0.0004, **** < 0.0001.
Our results suggest that the BlpIJ bacteriocins are associated with potent anti-pneumococcal activity when expressed in strains that carry an intact blpA transporter gene. To determine the distribution of such strains in the overall pneumococcal population, we took advantage of and examined the genome data from the collection of 3,085 genomes that represent the pneumococcal carriage population of an isolated refugee camp in Maela and a collection of 616 strains isolated from children living in Boston for strains that had both an intact blpA gene and the genes encoding the BlpI and immunity proteins blpU4/5 in the BIR (Chewapreecha et al., 2014). Out of 3,085 Maela isolates, 40% (1,240) have the genes blpI through blpJ (including blpU4/5) and 23% (702) are predicted to encode a full length BlpA. Only 116 isolates (4%) were predicted to encode both a full length BlpA and the genes for blpI through blpJ together (P < 0.0001 difference between observed and expected by chi square). Nearly identical numbers were obtained for the Boston isolates, 289/616 (47%) are predicted to encode BlpI, 152/616 (25%) are predicted to encode a full length BlpA and 27/616 (4%) are predicted to encode both (P < 0.0001). Consistent with our findings using the strain collection tested in this work, these data suggest that highly competitive BlpIJ producer strains are quite rare despite the competitive advantage in co-colonization.
In looking at BlpKin/out distribution in the Boston collection, we found that 509/618 (82%) of genomes encoded either BlpKin or BlpKout or both. Sixty three percent have BlpKout alone, 10% have BlpKin alone and the remainder have both. The small fraction of genomes that were not found to contain any blpK gene might have been missed due to incomplete sequencing given the small size of the blpKout/pncFout operon.
Pneumocin production by the blp locus is controlled by a quorum sensing regulon characterized by significant diversity in the BIR region encoding bacteriocins and immunity proteins in addition to variability in the expression of signaling pheromones and transporters. This diversity has been proposed to be the outcome of intense selective pressure induced by bacterial competition. The locus and its encoded pneumocins have been shown to provide a competitive advantage in murine models (Throup et al., 2000; Dawid et al., 2007). Through screening a large collection of isolates, here we defined the activity of two blp encoded pneumocins with activity in vitro: the two-peptide bacteriocin BlpIJ and a single-peptide bacteriocin BlpK. We had previously shown that only one of the three major allelic forms of BlpMN6A is associated with inhibitory activity in overlay assays (Dawid et al., 2007). This observation was confirmed by our isolate screening; no inhibitory activity could be seen in isolates encoding other allelic forms of BlpMN. This study brings the number of blp encoded bacteriocins with in vitro evidence in inhibition to three.
BlpIJ has potent inhibitory activity against other pneumococcal isolates when expressed in a strain with an intact BlpAB+ transporter and promotes inhibition against some other Streptococcal species including S. pyogenes and mitis. Overlay assays have shown that immune pneumococcal isolates must encode the BlpU4/5 immunity proteins and have either an intact BlpAB+ system or a responsive BlpH type to that secreted by the producing strain allowing the strain to bypass the need for BlpAB mediated BlpC secretion (Son et al., 2011). Our biofilm experiments confirm that a strain with a BlpAB+ system is more competitive than an otherwise identical strain with a non-functional BlpAFS. The biofilm conditions allow for activation of competence and the presence of transformants confirms that the competence occurred during biofilm growth. This suggests that com-mediated secretion of blp peptides is unable to fully reproduce the blp-mediated competition seen in a strain that can produce both BlpAB and ComAB transporters. We recently made similar observations using a mouse model of competitive colonization (Wang et al., 2018).
Several studies of pneumococcal genomes and strain collections have demonstrated that approximately 25% of strains carry an intact blpA gene. Our analysis of the Maela and Boston collection of genomes demonstrated that only 4% of strains contain the blpIU4/5J operon together with an intact blpA gene. The spectrum of inhibition among pneumococci promoted by BlpIJ producing strains would be expected to be broad. Given that there are four major pherotypes that are relatively evenly distributed and that between 40 and 45% of isolates encode blpIU4/5 (Pinchas et al., 2015; Miller et al., 2016), an additional 10% of strains would be predicted to be immune to a BlpIJ producer secreting one of the four common BlpC types. These numbers (13.8%) are consistent with the observed number of BlpIJ immune strains in the clinical isolate collection (12.5%). Only three isolates were BlpIJ immune despite lacking the linked immunity genes, blpU4/5 by PCR screening. It is possible that these isolates do encode the immunity genes, but sequence differences resulted in the failure of PCR primers to anneal, or there may be additional mechanisms of either immunity or resistance that remain to be defined. Why blpIJ genes are rarely found in BlpA intact strains is not clear given a clear competitive advantage of these strains during colonization. We speculate that the large scale secretion of BlpIJ may have some inhibitory properties even on the BlpU4/5-immune producing strains, resulting in some degree of self-inhibition and a resultant fitness cost to BlpA intact strains exclusively. We have not identified experimental conditions that can demonstrate a clear fitness defect in this subset of strains to verify this hypothesis. Of note, blpI and blpJ encoding genes can be found in the genomes of closely related Streptococcal species including S. mitis (1 genome), oralis (3 genomes) and pseudopneumoniae (1 genome). These genes are associated with a region containing a highly homologous blp locus encoding a peptide pheromone, transporter and two-component regulatory system, consistent with either their common ancestry or horizontal gene transfer. To our knowledge, no inhibitory activity has been attributed to these loci in non-pneumococcal species although given the fact that all five have apparently intact BlpA homologs, we would expect these strains to have similar activity to the BlpIJ secreting pneumococci.
In our survey of sequenced strains we found that 71% of all BlpA+, BlpIJ predicted producing strains in the Maela collection are non-typeable (NT) although un-encapsulated isolates make up only 17% of the whole collection. Overall, 45% of NT isolates in this collection are predicted to make a full length BlpA compared with 18% of the encapsulated strains. The Boston collection contains only 14 NT isolates, but 9/14 encode a full length BlpA. In the global array of 131 NT strains analyzed by Hilty et al. (2014), 80% of sequenced isolates are predicted to encode a full length BlpA; this includes all the members of the two major NT lineages, ST344 and ST448. In the remaining “sporadic” NT isolates that were analyzed, 47% are predicted to encode a full length BlpA. It is possible that NT strains may require the competitive advantage of a fully functional blp locus to remain competitive in the nasopharynx in the absence of capsule. In addition, NT strains may act as DNA donors, providing genome content to virulent strains. The prevalence of the intact blpA gene in this background may serve as a reservoir for this allele for the entire population, explaining its persistence.
We demonstrated that BlpK secretion is associated with narrow spectrum inhibition. Bacteria producing this pneumocin are only able to inhibit pneumococcal strains with both a deletion in the blp locus removing all regulatory and bacteriocin genes plus a disruption in the unlinked blpKout/pncFout gene cluster and do not promote inhibition of other tested species. No inhibition is noted in BlpK secreting strains when tested against any strain with an active blp locus, even those that lack pncFin. This finding suggests that some other component of the blp regulon (likely pncFout) protects cells against the inhibitory activity of BlpK.
The disruption in the blpKout/pncFout operon found in our prototypic 6A strain may not be that unusual, a different amber mutation that would result in a non-functional pncFout gene product was identified in 9 of 39 completed pneumococcal genomes; the remaining 30 had an intact cluster. Our data supports the findings of Lux et al. (2007) that the blpKout/pncFout operon has blpRH independent expression which differentiates it from the pneumocin encoding operons in the BIR. The blpKout/pncFout operon is preceded by a typical BlpR binding site and has been shown to be upregulated with the remainder of the blp locus by the addition of BlpC (de Saizieu et al., 2000), but appears to also have some degree of blp independent transcription. We have shown that this blp independent transcription is functionally relevant by demonstrating that PncFout provides BlpK specific immunity even when all the regulatory blp genes are deleted. The blpKout/pncFout operon is found adjacent to the comAB genes. We have shown that ComAB can secrete the pneumocins, but the effect of this is limited by the short duration of competence (Wang et al., 2018). It is possible that during competence induction, preformed BlpK is secreted by the newly made ComAB transporter allowing for some BlpK-mediated inhibition that cannot be seen on plates. However, given the degree of in vitro observed BlpK immunity in the pneumococcal population and the distribution of blpK/pncF genes, it seems likely that the in vivo target of BlpK-mediated killing is a member of the non-pneumococcal population.
We have found that strains expressing many blp encoded bacteriocins lack in vitro evidence of inhibition. In our screen the prevalent blpQ, blpO, pncW, and non-6A blpMN alleles all were not associated with an inhibitory phenotype. It is possible that the degree of inhibition promoted by these bacteriocins is too small to be observed using the plate assay. Alternatively, it is possible that we have not yet identified the target organism or the true function of the peptides.
The highly adaptable pathogen, pneumococcus, competes with neighboring pneumococci and some other Streptococcal species by secreting pneumocins. We demonstrated that BlpIJ and BlpK are two functional pneumocins that demonstrate inhibitory activity when expressed in strains with intact BlpA transporter, although some competition in BlpANF backgrounds can be seen during biofilm growth. The combination of the BlpIJ pneumocin and a functional BlpA transporter gives pneumococcus a competitive advantage during colonization of a biofilm and presumably in the human nasopharynx. Despite these advantages, encapsulated strains with this combination are rare in the pneumococcal population, suggesting that the competitive advantage might be offset by energetic cost or some other effect on fitness.
All genome references in the paper have already been deposited and are included in the manuscript tables.
W-YW, MA-K, and SD designed and performed the experiments and wrote the manuscript. EY, OE, and SS performed the experiments.
R01 AI101285 and R01AI078538 supported SD and supplied research funds for the work performed. Some supplies were provided by the Janette Ferrantino award and the Amendt-Heler Endowment for Newborn Research. The Hartwell Foundation Research Fellowship supported W-YW salary.
The authors declare that the research was conducted in the absence of any commercial or financial relationships that could be construed as a potential conflict of interest.
We thank National Institute of Health, USA (Grant R01 AI101285) and The Hartwell Foundation Research Fellowship for funding support. We thank Michael Watson for supplying S. pyogenes isolates.
The Supplementary Material for this article can be found online at: https://www.frontiersin.org/articles/10.3389/fcimb.2019.00055/full#supplementary-material
Ajdic, D., McShan, W. M., McLaughlin, R. E., Savic, G., Chang, J., Carson, M. B., et al. (2002). Genome sequence of Streptococcus mutans UA159, a cariogenic dental pathogen. Proc. Natl. Acad. Sci. U.S.A. 99, 14434–14439. doi: 10.1073/pnas.172501299
Beres, S. B., Sylva, G. L., Barbian, K. D., Lei, B., Hoff, J. S., Mammarella, N. D., et al. (2002). Genome sequence of a serotype M3 strain of group A Streptococcus: phage-encoded toxins, the high-virulence phenotype, and clone emergence. Proc. Natl. Acad. Sci. U.S.A. 99, 10078–10083. doi: 10.1073/pnas.152298499
Bogaardt, C., van Tonder, A. J., and Brueggemann, A. B. (2015). Genomic analyses of pneumococci reveal a wide diversity of bacteriocins—including pneumocyclicin, a novel circular bacteriocin. BMC Genomics 16:554. doi: 10.1186/s12864-015-1729-4
Bosch, A. A., de Steenhuijsen Piters, W. A., van Houten, M. A., Chu, M., Biesbroek, G., Kool, J., et al. (2017). Maturation of the infant respiratory microbiota, environmental drivers and health consequences: a prospective cohort study. Am. J. Respir. Crit. Care Med. 196, 1582–1590. doi: 10.1164/rccm.201703-0554OC
Chewapreecha, C., Harris, S. R., Croucher, N. J., Turner, C., Marttinen, P., Cheng, L., et al. (2014). Dense genomic sampling identifies highways of pneumococcal recombination. Nat. Genet. 46, 305–309. doi: 10.1038/ng.2895
Cisar, J. O., Kolenbrander, P. E., and McIntire, F. C. (1979). Specificity of coaggregation reactions between human oral streptococci and strains of Actinomyces viscosus or Actinomyces naeslundii. Infect. Immun. 24, 742–752.
Claverys, J. P., Dintilhac, A., Pestova, E. V., Martin, B., and Morrison, D. A. (1995). Construction and evaluation of new drug-resistance cassettes for gene disruption mutagenesis in Streptococcus pneumoniae, using an ami test platform. Gene. 164, 123–128.
Cornforth, D. M., and Foster, K. R. (2013). Competition sensing: the social side of bacterial stress responses. Nat. Rev. Microbiol. 11, 285–293. doi: 10.1038/nrmicro2977
Croucher, N. J., Finkelstein, J. A., Pelton, S. I., Mitchell, P. K., Lee, G. M., Parkhill, J., et al. (2013). Population genomics of post-vaccine changes in pneumococcal epidemiology. Nat. Genet. 45, 656–663. doi: 10.1038/ng.2625
Croucher, N. J., Kagedan, L., Thompson, C. M., Parkhill, J., Bentley, S. D., Finkelstein, J. A., et al. (2015). Selective and genetic constraints on pneumococcal serotype switching. PLoS Genet. 11:e1005095. doi: 10.1371/journal.pgen.1005095
da Gloria Carvalho, M., Pimenta, F. C., Jackson, D., Roundtree, A., Ahmad, Y., Millar, E. V., et al. (2010). Revisiting pneumococcal carriage by use of broth enrichment and PCR techniques for enhanced detection of carriage and serotypes. J. Clin. Microbiol. 48, 1611–1618. doi: 10.1128/JCM.02243-09
Dawid, S., Roche, A. M., and Weiser, J. N. (2007). The blp bacteriocins of Streptococcus pneumoniae mediate intraspecies competition both in vitro and in vivo. Infect. Immun. 75, 443–451. doi: 10.1128/IAI.01775-05
de Saizieu, A., Gardes, C., Flint, N., Wagner, C., Kamber, M., Mitchell, T. J., et al. (2000). Microarray-based identification of a novel Streptococcus pneumoniae regulon controlled by an autoinduced peptide. J. Bacteriol. 182, 4696–4703. doi: 10.1128/JB.182.17.4696-4703.2000
Ferretti, J. J., McShan, W. M., Ajdic, D., Savic, D. J., Savic, G., Lyon, K., et al. (2001). Complete genome sequence of an M1 strain of Streptococcus pyogenes. Proc. Natl. Acad. Sci. U.S.A. 98, 4658–4663. doi: 10.1073/pnas.071559398
Guiral, S., Mitchell, T. J., Martin, B., and Claverys, J. P. (2005). Competence-programmed predation of noncompetent cells in the human pathogen Streptococcus pneumoniae: genetic requirements. Proc. Natl. Acad. Sci. U.S.A. 102, 8710–8715. doi: 10.1073/pnas.0500879102
Hilty, M., Wuthrich, D., Salter, S. J., Engel, H., Campbell, S., Sa-Leao, R., et al. (2014). Global phylogenomic analysis of nonencapsulated Streptococcus pneumoniae reveals a deep-branching classic lineage that is distinct from multiple sporadic lineages. Genome Biol. Evol. 6, 3281–3294. doi: 10.1093/gbe/evu263
Jack, R. W., Tagg, J. R., and Ray, B. (1995). Bacteriocins of gram-positive bacteria. Microbiol. Rev. 59, 171–200.
Jacob, K. M., Spilker, T., LiPuma, J. J., Dawid, S. R., and Watson, M. E. Jr. (2016a). Complete genome sequence of emm28 type Streptococcus pyogenes MEW123, a streptomycin-resistant derivative of a clinical throat isolate suitable for investigation of pathogenesis. Genome Announc. 4, e00136–e00116. doi: 10.1128/genomeA.00136-16
Jacob, K. M., Spilker, T., LiPuma, J. J., Dawid, S. R., and Watson, M. E. Jr. (2016b). Complete genome sequence of emm4 Streptococcus pyogenes MEW427, a throat isolate from a child meeting clinical criteria for pediatric autoimmune neuropsychiatric disorders associated with Streptococcus (PANDAS). Genome Announc. 4, e00127–e00116. doi: 10.1128/genomeA.00127-16
Kelly, M. S., Surette, M. G., Smieja, M., Pernica, J. M., Rossi, L., Luinstra, K., et al. (2017). The nasopharyngeal microbiota of children with respiratory infections in botswana. Pediatr. Infect. Dis. J. 36, e211–e218. doi: 10.1097/INF.0000000000001607
Kjos, M., Miller, E., Slager, J., Lake, F. B., Gericke, O., Roberts, I. S., et al. (2016). Expression of Streptococcus pneumoniae bacteriocins is induced by antibiotics via regulatory interplay with the competence system. PLoS Pathog. 12:e1005422. doi: 10.1371/journal.ppat.1005422
Kjos, M., Snipen, L., Salehian, Z., Nes, I. F., and Diep, D. B. (2010). The abi proteins and their involvement in bacteriocin self-immunity. J. Bacteriol. 192, 2068–2076. doi: 10.1128/JB.01553-09
Lux, T., Nuhn, M., Hakenbeck, R., and Reichmann, P. (2007). Diversity of bacteriocins and activity spectrum in Streptococcus pneumoniae. J. Bacteriol. 189, 7741–7751. doi: 10.1128/JB.00474-07
Maricic, N., Anderson, E. S., Opipari, A. E., Yu, E. A., and Dawid, S. (2016). Characterization of a multipeptide lantibiotic locus in Streptococcus pneumoniae. mBio 7, e01656–e01615. doi: 10.1128/mBio.01656-15
Maricic, N., and Dawid, S. (2014). Using the overlay assay to qualitatively measure bacterial production of and sensitivity to pneumococcal bacteriocins. J. Vis. Exp. 90, e51876. doi: 10.3791/51876
Marks, L. R., Reddinger, R. M., and Hakansson, A. P. (2012). High levels of genetic recombination during nasopharyngeal carriage and biofilm formation in Streptococcus pneumoniae. mBio 3, e00200–e00212. doi: 10.1128/mBio.00200-12
Miller, E. L., Abrudan, M. I., Roberts, I. S., and Rozen, D. E. (2016). Diverse ecological strategies are encoded by Streptococcus pneumoniae bacteriocin-like peptides. Genome Biol. Evol. 8, 1072–1090. doi: 10.1093/gbe/evw055
Nes, I. F., Diep, D. B., Havarstein, L. S., Brurberg, M. B., Eijsink, V., and Holo, H. (1996). Biosynthesis of bacteriocins in lactic acid bacteria. Antonie Van Leeuwenhoek 70, 113–128. doi: 10.1007/BF00395929
Orsi, R. H., Bergholz, T. M., Wiedmann, M., and Boor, K. J. (2015). The Listeria monocytogenes strain 10403S BioCyc database. Database 2015:bav027. doi: 10.1093/database/bav027
Pinchas, M. D., LaCross, N. C., and Dawid, S. (2015). An electrostatic interaction between BlpC and BlpH dictates pheromone specificity in the control of bacteriocin production and immunity in Streptococcus pneumoniae. J. Bacteriol. 197, 1236–1248. doi: 10.1128/JB.02432-14
Port, G. C., Paluscio, E., and Caparon, M. G. (2013). Complete genome sequence of emm type 14 Streptococcus pyogenes strain HSC5. Genome Announc. 1, e00612–e00613. doi: 10.1128/genomeA.00612-13
Port, G. C., Paluscio, E., and Caparon, M. G. (2015). Complete genome sequences of emm6 Streptococcus pyogenes JRS4 and parental strain D471. Genome Announc. 3, e00725–e00715. doi: 10.1128/genomeA.00725-15
Reichmann, P., and Hakenbeck, R. (2000). Allelic variation in a peptide-inducible two-component system of Streptococcus pneumoniae. FEMS Microbiol. Lett. 190, 231–236. doi: 10.1111/j.1574-6968.2000.tb09291.x
Shanker, E., and Federle, M. J. (2017). Quorum sensing regulation of competence and bacteriocins in Streptococcus pneumoniae and mutans. Genes 8:15. doi: 10.3390/genes8010015
Son, M. R., Shchepetov, M., Adrian, P. V., Madhi, S. A., de Gouveia, L., von Gottberg, A., et al. (2011). Conserved mutations in the pneumococcal bacteriocin transporter gene, blpA, result in a complex population consisting of producers and cheaters. mBio 2, e00179–e00111. doi: 10.1128/mBio.00179-11
St Sauver, J., Marrs, C. F., Foxman, B., Somsel, P., Madera, R., and Gilsdorf, J. R. (2000). Risk factors for otitis media and carriage of multiple strains of Haemophilus influenzae and Streptococcus pneumoniae. Emerg. Infect. Dis. 6, 622–630. doi: 10.3201/eid0606.000611
Teo, S. M., Mok, D., Pham, K., Kusel, M., Serralha, M., Troy, N., et al. (2015). The infant nasopharyngeal microbiome impacts severity of lower respiratory infection and risk of asthma development. Cell Host Microbe 17, 704–715. doi: 10.1016/j.chom.2015.03.008
Throup, J. P., Koretke, K. K., Bryant, A. P., Ingraham, K. A., Chalker, A. F., Ge, Y., et al. (2000). A genomic analysis of two-component signal transduction in Streptococcus pneumoniae. Mol. Microbiol. 35, 566–576. doi: 10.1046/j.1365-2958.2000.01725.x
Wang, C. Y., Patel, N., Wholey, W. Y., and Dawid, S. (2018). ABC transporter content diversity in Streptococcus pneumoniae impacts competence regulation and bacteriocin production. Proc. Natl. Acad. Sci. U.S.A. 115, E5776–E5785. doi: 10.1073/pnas.1804668115
Wescombe, P. A., Upton, M., Dierksen, K. P., Ragland, N. L., Sivabalan, S., Wirawan, R. E., et al. (2006). Production of the lantibiotic salivaricin A and its variants by oral streptococci and use of a specific induction assay to detect their presence in human saliva. Appl. Environ. Microbiol. 72, 1459–1466. doi: 10.1128/AEM.72.2.1459-1466.2006
Keywords: Streptococcus pneumoniae, bacteriocin, colonization, competition, quorum sensing
Citation: Wholey W-Y, Abu-Khdeir M, Yu EA, Siddiqui S, Esimai O and Dawid S (2019) Characterization of the Competitive Pneumocin Peptides of Streptococcus pneumoniae. Front. Cell. Infect. Microbiol. 9:55. doi: 10.3389/fcimb.2019.00055
Received: 12 December 2018; Accepted: 20 February 2019;
Published: 12 March 2019.
Edited by:
Michael J. Federle, University of Illinois at Chicago, United StatesReviewed by:
Gee W. Lau, University of Illinois at Urbana-Champaign, United StatesCopyright © 2019 Wholey, Abu-Khdeir, Yu, Siddiqui, Esimai and Dawid. This is an open-access article distributed under the terms of the Creative Commons Attribution License (CC BY). The use, distribution or reproduction in other forums is permitted, provided the original author(s) and the copyright owner(s) are credited and that the original publication in this journal is cited, in accordance with accepted academic practice. No use, distribution or reproduction is permitted which does not comply with these terms.
*Correspondence: Suzanne Dawid, c2Rhd2lkQHVtaWNoLmVkdQ==
Disclaimer: All claims expressed in this article are solely those of the authors and do not necessarily represent those of their affiliated organizations, or those of the publisher, the editors and the reviewers. Any product that may be evaluated in this article or claim that may be made by its manufacturer is not guaranteed or endorsed by the publisher.
Research integrity at Frontiers
Learn more about the work of our research integrity team to safeguard the quality of each article we publish.