- Department of Microbiology and Immunology, Virginia Commonwealth University Medical Center, Richmond, VA, United States
Cyclic-di-GMP (c-di-GMP) contributes to the regulation of processes required by the Lyme disease (LD) spirochetes to complete the tick-mammal enzootic cycle. Our understanding of the effector mechanisms of c-di-GMP in the Borrelia is evolving. While most LD spirochete isolates encode a single PilZ domain containing c-di-GMP receptor designated as PlzA, genome analyses have revealed that a subset encode a second PilZ domain protein (PlzB). The c-di-GMP binding potential of PlzB, and its role in LD spirochete biology, have not been investigated. To determine if PlzB binds c-di-GMP, plzB from B. burgdorferi isolate ZS7 was PCR amplified, cloned, and recombinant protein generated. PlzB bound c-di-GMP but not other nucleotides, indicating a specific binding interaction. To determine if PlzA and PlzB are functionally synonymous, a series of allelic-exchange gene deletion and cis-complemented strains were generated in the B. burgdorferi B31 background. B. burgdorferi B31-ΔplzA was competent to infect Ixodes scapularis larvae but not mice when delivered by either needle or tick feeding. B. burgdorferi B31-ΔplzA also displayed an atypical motility phenotype. Complementation in cis of B. burgdorferi B31-ΔplzA with plzA (B31-plzA KI) restored wild-type (wt) phenotype. However, a strain complemented in cis with plzB (B31-plzB KI) did not. The data presented here are consistent with an earlier study that demonstrated that PlzA plays an essential role in spirochete survival in the mammalian environment. We add to our understanding of the c-di-GMP regulatory network by demonstrating that while PlzB binds c-di-GMP, it is not functionally synonymous with PlzA. The absence of plzB from most strains suggests that it is not required for survival. One possibility is that cells that harbor both PlzA and PlzB might have enhanced biological fitness or increased virulence.
Introduction
Borrelia burgdorferi, B. garinii, B. bavariensis, and B. afzelii are the main causative agents of Lyme disease (LD) in North America and Europe (Burgdorfer et al., 1982). Henceforth, we collectively refer to all Borrelia species that cause LD as the LD spirochetes. Note that the LD spirochetes have recently been assigned to a new genus, Borreliella (Adeolu and Gupta, 2014). The use of this new designation is voluntary. In this report, we continue to use the Borrelia genus designation. The LD spirochetes are host-dependent extracellular pathogens that are maintained in nature by cycling between Ixodes ticks and diverse mammalian reservoirs (Barbour and Hayes, 1986). To successfully complete the enzootic cycle, rapid adaptation to environmental conditions is essential. c-di-GMP is a key contributor to adaptive responses (Ryjenkov et al., 2005; Rogers et al., 2009; Sultan et al., 2010; Kostick et al., 2011; Pitzer et al., 2011). In B. burgdorferi, c-di-GMP levels are regulated by the opposing activities of Rrp1 [a GGDEF domain containing diguanylate cyclase (DGC)] and PdeA and PdeB [EAL and HD-GYP domain containing phosphodiesterases (PDE)] (Galperin et al., 2001; Ryjenkov et al., 2005; Rogers et al., 2009; Sultan et al., 2010). Orthologs of Rrp1, PdeA, and PdeB are encoded by the genomes of all LD and tick-borne relapsing fever spirochete species. The only demonstrated functional activity of Rrp1 is c-di-GMP production. Studies of B. burgdorferi rrp1 deletion mutants have revealed that Rrp1, and by extension c-di-GMP, directly or indirectly regulates a significant proportion of the B. burgdorferi transcriptome (Rogers et al., 2009; Caimano et al., 2015). C-di-GMP levels influence motility, N-acetyl glucosamine directed chemotaxis, glycerol metabolism, chitobiose utilization, and several other metabolic pathways (Rogers et al., 2009; Kostick et al., 2011; Sze et al., 2013; Caimano et al., 2015, 2016). Interestingly, c-di-GMP is not required by the LD spirochetes to infect mammals but it is essential for survival in ticks (Rogers et al., 2009; Kostick et al., 2011; Caimano et al., 2015, 2016).
Proteins harboring C-terminally located PilZ domains are among the primary receptors and effector partners for c-di-GMP (Amikam and Galperin, 2006). B. burgdorferi B31, and most other LD spirochete isolates, encode and produce a single c-di-GMP receptor designated as PlzA (Freedman et al., 2009). PlzA is a 29.6 kDa (261 aa) stand-alone PilZ domain protein (i.e., lacks other identifiable conserved functional domains) that is chromosomally encoded (B. burgdorferi B31 ORF designation, BB0733). The tick-borne relapsing fever spirochetes encode a related c-di-GMP receptor designated as PlzC that is also encoded by a linear chromosome carried gene (Freedman et al., 2009; Mallory et al., 2016). PlzA has 69% amino acid identity to PlzC. Bacterial PilZ domains are identified by two signature motifis; RxxxR and DzSxxG (Amikam and Galperin, 2006). In the Borrelia, these consensus motifs have been further defined as RIHER and DzSYGG (Mallory et al., 2016). The requirement for specific residues within these motifs in c-di-GMP binding has been revealed through site-directed amino acid substitution mutagenesis (Freedman et al., 2009; Mallory et al., 2016). PlzA and PlzC are monomeric in both their apo and holo forms and bind c-di-GMP with a 1:1 stoichiometry (Mallory et al., 2016). C-di-GMP binding to PlzA and PlzC triggers structural rearrangements that may serve as a functional switch (Mallory et al., 2016). While the full range of regulatory activities mediated by PlzA and PlzC are not defined, studies of deletion mutants have demonstrated that PlzA is linked to motility (Pitzer et al., 2011). In addition, PlzA influences the regulatory activity of BosR, a DNA binding protein in the Fur/PerR family that is required for survival in mammals but not ticks (Seshu et al., 2004; Hyde et al., 2006, 2007, 2009; Ouyang et al., 2011; He et al., 2014).
A gene encoding a second PilZ domain-containing protein designated as PlzB has been identified on a linear plasmid of 28 kb in a subset of LD spirochete isolates (Freedman et al., 2009). The function and biological significance of PlzB has not been investigated. plzB encodes a putative 30 kDa protein with ~64 and 53% amino acid identity to PlzA and PlzC, respectively. In this report, the ability of PlzB to bind c-di-GMP and the functional relationship between PlzA and PlzB were assessed. In summary, PlzB binds c-di-GMP in a highly specific manner. Consistent with an earlier report (Sultan et al., 2010), deletion of plzA results in an aberrant motility pattern and eliminates or attenuates infectivity of B. burgdorferi B31 in mice. The ability to infect larval stage Ixodes scapularis ticks was unaffected. While PlzB bound c-di-GMP, cis-complementation of a plzA deficient strain with plzB did not restore wt (wild type) phenotype. The studies presented within indicate that while PlzB is competent to serve as a receptor for c-di-GMP, PlzA, and PlzB are not functionally synonymous.
Materials and Methods
Bacterial Strains and Cultivation Conditions
Borrelia isolates were cultivated in BSK media (33°C; 5% CO2) prepared using bovine serum albumin from Gemini Bio-Products, Inc. (lot #C54) as previously described (Samuels et al., 1994). All genetically manipulated strains were initially cultivated in BSK media or BSK semi-solid media plates containing 75 μg/mL streptomycin or 75 μg/mL kanamycin, as appropriate. BL21 (DE3) and TOP10 E. coli cells were cultivated in LB Broth, Lennox, or LB agar (Fisher BioReagents) containing 100 μg/mL ampicillin at temperatures detailed below.
Generation and Expression of Recombinant Proteins
plzA and rrp1 were PCR amplified from B. burgdorferi B31 using standard methods. plzB was amplified from B. burgdorferi strain ZS7. The primers were designed to include 5′ BamHI or 3′ SalI restriction sites as required. The amplicons were digested with the appropriate restriction enzyme, ligated into the multiple cloning site of pCR2.1-TOPO, transformed into TOP10 E. coli cells (Invitrogen), and colonies with the appropriate plasmid inserts identified by PCR. The plasmids were propagated and subcloned into pMAL-c4x (New England BioLabs) via the BamHI and SalI restriction sites to yield pMAL-c4xplzA, pMAL-c4xplzB, and pMAL-c4xrrp1. The plasmids were purified and transformed into BL21 (DE3) E. coli cells (Novagen) for protein expression. Cells were grown at 37°C to an OD600 of 0.6, shifted to 23°C, and protein expression induced with isopropyl-beta-D-1-thiogalactopyranoside (IPTG; 1 mM; 3 h). Cells were harvested by centrifugation, treated with lysozyme (1 mg/mL), sonicated, and soluble protein purified by amylose affinity chromatography as instructed by the supplier (New England BioLabs). It is worth noting that in subsequent work, each protein was successfully produced with N-terminal Hexa-His tags using the pET-45b (+) vector.
Synthesis of Radiolabeled c-di-GMP
C-di-GMP was enzymatically synthesized using recombinant WspR as previously described (Freedman et al., 2009). In brief, the wspR gene sequence from Pseudomonas aeruginosa FRG-1 was PCR amplified and inserted into a cloning vector to produce recombinant protein. To activate the DGC activity of r-WspR, WspR was incubated with acetyl phosphate. Phosphorylated WspR was incubated with GTP [α- 32P] or GTP to generate labeled or cold (unlabeled) c-di-GMP, respectively. C-di-GMP concentrations and purity were assessed using reverse phase FPLC with GTP and unlabeled c-di-GMP served as elution standards. Nucleotides were detected at a wavelength of 254 nm.
PlzA and PlzB c-di-GMP Binding Assays
C-di-GMP binding assays were carried out as previously described (Freedman et al., 2009). In brief, recombinant PlzA and PlzB were spotted onto nitrocellulose membranes and air dried. Rrp1 served as a negative control. The membranes were incubated with [32P]c-di-GMP (2 nM) alone, or in combination with 750 nM of c-di-GMP, GTP, GMP, ATP, cGMP, or cAMP (Sigma; PBS with 1% non-fat dried milk; 2 h; room temperature), washed twice with PBS (10 min) wrapped in cellophane and exposed to film overnight with intensifying screens at −80°C.
Allelic Exchange Mutagenesis
Allelic replacement of plzA was performed using methods developed by Samuels et al. (1994). One kb of DNA upstream and downstream of plzA were PCR amplified in separate reactions and cloned into the pCR2.1-TOPO vector to yield pCR2.1-UP and pCR2.1-DN, respectively. The upstream (UP) and downstream (DN) 1 kb fragments possessed AatII and AgeI restriction sites at the appropriate end to allow for subsequent transfer into other plasmids. The DN fragment was excised from the pCR2.1-DN with AatII and AgeI, ligated into pCR2.1-UP vector to generate pCR2.1-UPDN. A spectinomycin resistance cassette, derived from pKFSS1-AatII (Frank et al., 2003), was inserted into the AatII site at the junction between the UP and DN fragments to yield pCR2.1ΔplzA-specR. The plasmid was linearized with MscI and ScaI and electroporated into infectious B. burgdorferi B31-5A4 to generate the B31-ΔplzA mutant. Electroporation conditions were as previously described (Samuels et al., 1994). Selection was achieved by supplementing BSK with 75 μg ml−1 streptomycin. Clonal populations were obtained by sub-surface plating (Sung et al., 2001). The plasmid content of each clone was determined by PCR utilizing plasmid-specific primers (Labandeira-Rey et al., 2003) with some modifications (Rogers and Marconi, 2007).
Cis-complementation of plzA was obtained by replacement of the spectinomycin cassette with plzA and downstream kanamycin resistance cassette. A PCR product, containing the plzA upstream sequence, plzA ORF, and 3′ flanking AatII and AscI sites, was TOPO cloned into pCR2.1 to generate pCR2.1plzA-UP. A kanamycin resistance cassette was PCR amplified from pBSV2 (Stewart et al., 2001) with a 5′ AatII site and joined to a PCR product derived from the 1,000 bp of native sequence located immediately downstream of B31 plzA with a 3′ AscI site. The kanamycin-DN fusion was TOPO cloned into pCR2.1, digested and ligated into pCR2.1plzA-UP to create pCR2.1 plzA-kan. The plasmid was linearized with ScaI and MscI and electroporated into B31-ΔplzA to yield the cis-complemented strain B31-plzA KI. Selection was achieved with 75 μg ml−1 kanamycin. Clonal populations were isolated from semi-solid BSK media plates and screened for plasmid profile as described above.
plzB Allelic Replacement of plzA
Replacement of plzA with plzB was achieved by allelic exchange mutagenesis. The upstream 1 kb region of plzA was PCR amplified to include the plzA ribosome-binding site (RBS) and cloned into pCR2.1-TOPO to generate plasmid pCR2.1plzA-UP2. The 1kb DN fragment was excised from pCR2.1plzA-DN with AatII and AgeI and ligated into pCR2.1plzA-UP2 to yield pCR2.1plzA-UPDN2. plzB was PCR amplified from B. burgdorferi ZS7 with primers that introduced a 5′ AatII site and a 3′ overlap with the PflgBkanR cassette. The PflgBkanR cassette was amplified from pBSV2 (Stewart et al., 2001) to possess a 5′ overlap with the 3′ plzB ORF and a 3′ AgeI restriction site. A plzB- PflgBkanR fusion was created by overlap extension PCR, then digested with AatII and AgeI, and ligated into pCR2.1plzA-UPDN2 to yield pCR2.1plzB-kan. The plasmid was linearized with ScaI and NcoI, electroporated into B. burgdorferi B31-5A4, and transformants selected by cultivation in media containing 75 μg ml−1 kanamycin.
SDS-PAGE, Immunoblot and ELISA Analyses
Cell lysates were fractionated using 12.5% Criterion Precast Gels (BioRad) and transferred to PVDF membranes by electroblotting. The membranes were incubated with blocking buffer (1x PBS, 0.2% Tween-20, 5% non-fat dry milk) and screened with serum from mice infected or inoculated with each strain at a dilution of 1:1,000. Antibody binding was detected using horseradish peroxidase (HRP)-conjugated secondary antibodies (Pierce; 1:40,000) and SuperSignal West Pico chemiluminescence substrate (Pierce). For ELISAs, 96-well plates (Costar 3590) were coated with B31-5A4 spirochetes (0.1 OD600 of ml−1) in carbonate buffer (100 mM NaHCO3, pH 9.6). Blocking buffer (1% BSA in PBS-T) was added to each well (2 h; room temperature; with shaking). The desired sera were then added in 3-fold serial dilutions (1:50-1:109,350; in triplicate). Plates were washed 3 times with PBS-T and bound IgG was detected with HRP-conjugated goat-anti-mouse IgG antiserum (1:20,000) with ABTS substrate. The plates were read at OD405. The absorbance values obtained from the pre-bleed sera were considered to be baseline and these values were subtracted prior to titer calculation. Titers were calculated as previously described (Earnhart et al., 2010). Statistical analysis was performed using a one-way ANOVA.
RNA Isolation and Reverse Transcriptase Polymerase Chain Reaction (RT-PCR)
RNA was isolated from Borrelia cells using the RNeasy Midi kit (Qiagen). Residual DNA was removed by treatment with DNase I (Invitrogen). cDNA was produced using the Superscript III First Strand cDNA Synthesis kit (Invitrogen), 50 ng of random hexamer primers, and 1 μg total RNA. RT-PCR was performed with SYBR green PCR Master Mix (Applied Biosystems) and a CFX96 Real Time System (BioRad) with the following cycle parameters: 1 cycle of 10 min at 95°C followed by 40 cycles of 10 s at 94°C, 30 s at 50°C, and 30 s at 72°C.
Motility Analyses
Rotational (wave propagation) and translational (directional movement) motility of spirochetes in BSK with or without 1% carboxy methylcellulose (CMC) was assessed by dark-field microscopy and differential interference contrast (DIC) microscopy. CMC was added to the culture media to increase viscosity. Motility and flex rates were determined using Slidebook 5 (Intelligent Imaging Innovations) motion-tracking software and represented the average of 20 motility tracking analyses per Borrelia strain.
Swarm Plate and Capillary Tube Chemotaxis Assays
Swarm assays (migration of spirochetes through semi-solid media) were conducted as previously described (Kostick et al., 2011). Cells were introduced into BSK-H semi-solid media plates containing 0.35% (weight/vol) SeaKem GTG agarose by insertion into a depression in the media generated by coring with a 1 ml micropipette tip. 5 × 105 spirochetes (5 μl of a 1:10 BSK-H dilution in dPBS) were introduced into each depression. All assays were performed in triplicate. Plates were incubated at 33°C and the diameter of the visible expanding colony assessed at 2, 4, and 6 days. Capillary chemotaxis assays were performed as previously described (Kostick et al., 2011) using 0.1M N-acetyl-D-glucosamine as the chemoattractant. One-way ANOVA statistical analysis was performed on the means from 3 separate experiments.
Murine Infection, Seroconversion, and Cultivation of Spirochetes
The ability of each strain to infect C3H-HeJ mice (Jackson Labs) was determined using 104 spirochetes (in 100 μl BSK-H complete media) delivered by subcutaneous needle inoculation between the shoulder blades. Four weeks post-infection, mice were sacrificed, blood was obtained by cardiac puncture and tissue and organs collected. Serum was harvested using standard methods and seroconversion of each individual mouse assessed by ELISA and immunoblot as detailed above. To cultivate spirochetes from the mice, sections of tissues and organs were placed in BSK media supplemented with an antimicrobial cocktail consisting of phosphomycin, rifampicin, and amphotericin B (Sigma) and growth monitored using dark-field microscopy. All animal experiments were conducted following the Guide for the Care and Use of Laboratory Animals (8th edition) and in accordance with protocols peer reviewed and approved by VCU Institutional Animal Care and Use Committees.
Infection of Ticks by Immersion in Cultures
Infection free-I. scapularis larvae (Oklahoma State University Tick Rearing Facility) were infected by immersion in B. burgdorferi cultures (108 spirochetes ml−1; 33°C; 2 h) as previously described (Policastro and Schwan, 2003). Ticks were recovered, washed, dried, maintained for 2 days and then fed on naive C3H-HeJ mice. One week after completion of tick feeding, DNA was isolated from the ticks using the DNeasy Blood and Tissue Kit (Qiagen). Randomly selected ticks were analyzed for spirochetes by PCR (flaB). Normalization against tick DNA was achieved using RIB-3 and RIB-4 primers (Zahler et al., 1995) as previously described (Kostick et al., 2011). PCR results were scored as plus or minus.
Results
PlzB Binds Cyclic di-GMP With High Specificity
(32P) c-di-GMP binding to PlzB, PlzA (positive control) and Rrp1 (negative control) was assessed using a membrane overlay assay (Freedman et al., 2009). The purity of the radiolabeled c-di-GMP generated using WspR was verified through reverse phase liquid chromatography (Freedman et al., 2009). C-di-GMP bound to PlzB and PlzA but not to Rrp1. Binding was inhibited by unlabeled c-di-GMP but not by GTP, ATP, cAMP, or cGMP (Figure 1). It can be concluded that recombinant PlzB can serve as a receptor for c-di-GMP.
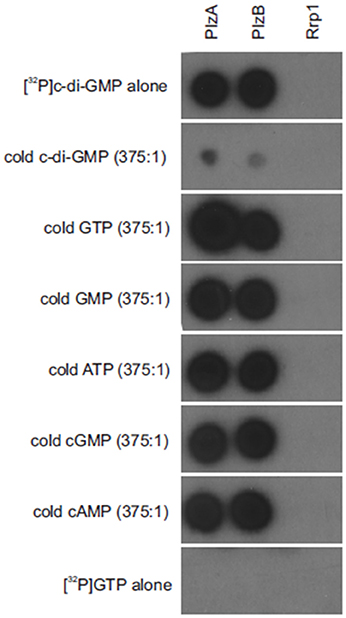
Figure 1. Borrelia burgdorferi PlzB binds to c-di-GMP with high specificity. Equal amounts (0.25 μg) of recombinant PlzB, PlzA (positive control), and Rrp1(negative control) were spotted onto a series of nitrocellulose membrane strips and incubated with [32P]c-di-GMP alone (top; 2 nM) or [32P]c-di-GMP plus a potential competing unlabeled nucleotide (750 nM; 375:1 competitor to [32P]c-di-GMP molar ratio). In the bottom, labeled [32P] GTP alone was incubated with the membrane as a control for possible non-specific radiographic signal. After incubation, all membranes were washed identically and exposed to film. All methods are as detailed in the text.
Characterization of Genetically Modified Strains of B. burgdorferi
plzA was deleted from the B. burgdorferi B31-5A4 infectious clone (B31-wt) and replaced with a spectinomycin/streptomycin resistance cassette (strepR) to yield B31-ΔplzA (Figure 2A). A cis-complemented plzA strain was generated by replacing the strepR cassette of B31-ΔplzA with plzA and a downstream kanamycin resistance cassette (kanR) to yield B31-plzA KI (KI indicating “knock-in”) (Figure 2B). To substitute plzA with plzB, the strepR cassette of B31-ΔplzA was replaced with plzB-kanR to yield B31-plzB KI (Figure 2C). Clonal populations of the mutated strains were obtained by subsurface plating and proper deletion/insertion was verified by PCR (Figure 2E, Table 1). Plasmid specific PCR analyses of the clonal populations identified several that possess the full complement of plasmids carried by the B31-wt parental strain (21 circular and linear plasmids; data not shown). Individual clones of each strain were selected for further analysis.
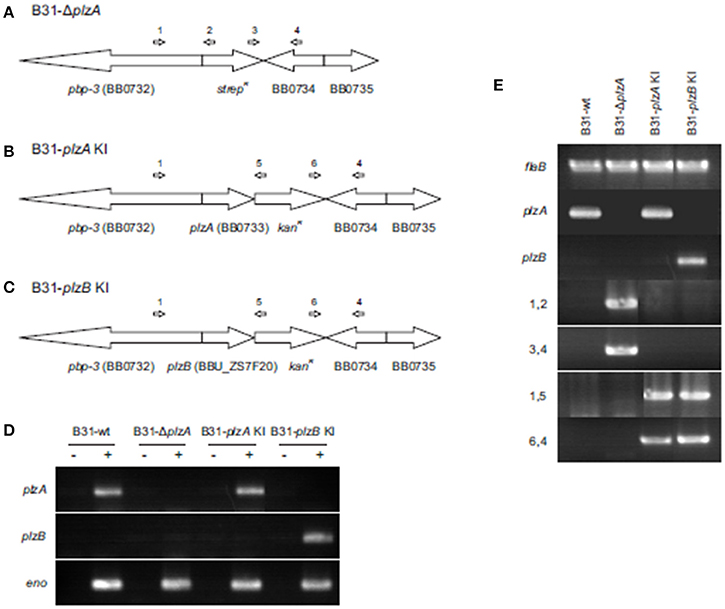
Figure 2. Generation and confirmation of genetic constructs. (A) Depicts in schematic form the organization of the plzA locus of the B. burgdorferi B31 linear chromosome after replacement of plzA with a strepR cassette. (B,C) Depict the gene organization of the same region of the chromosome after cis-complementation or “knock in” (KI) with plzA or plzB, respectively. Note that the intergenic regions between are not indicated in the schematics. The ORF designations originally assigned to the B. burgdorferi B31 genome are indicated. The general location of hybridization sites for primers used to verify the intended allelic exchange events are indicated above each schematic with the PCR results presented in (E). Amplification of the flaB gene served as a positive control for PCR. (D) Shows the results of RT-PCR analyses conducted to assess transcription of each gene. Amplification of the enolase gene served as a positive control for RT-PCR. The plus and minus signs indicate the inclusion or absence of reverse transcriptase in the reactions. All primers used in this study are listed in Table 1. Note that the PCR gel images are composites of images from independent gels that were cropped and aligned for reader convenience.
Transcription of plzA or plzB was assessed by RT-PCR and as expected, plzA mRNA was detected in B31-wt and B31-plzA KI but not in B31-ΔplzA and B31 plzB-KI. plzB transcript was detected in B31 plzB KI but not in the other strains (Figure 2D). To determine if the process of genetic manipulation had an impact on in vitro growth, standard growth curves were generated at 27°, 33°, and 37°C (data not shown) using previously established methods (Earnhart et al., 2010). Deletion of plzA, complementation with plzA or plzB, or the introduction of kanR, specR, or ampR antibiotic resistance cassettes had no effect on growth rate at any temperature.
The Motility Defects in a plzA Deletion Mutant can be Restored by Complementation With plzA but not plzB
Motility patterns of cells growing in BSK with 1% CMC were assessed using dark-field and DIC microscopy. While all strains displayed similar rotational motility (wave propagation) as assessed by dark-field microscopy, the rate of translational motility (directional movement) and flexing differed (as determined using DIC microscopy). B31-ΔplzA and B31-plzB KI had reduced translational motility and flex frequency, whereas the B31-plzA KI complemented strain displayed wt motility patterns (data are summarized in Table 2). Motility was assessed using swarm assays (Kostick et al., 2011). Colony diameters for the B31-ΔplzA and B31-plzB KI strains were reduced by ~60% in comparison to B31-wt (p < 0.05) (Figures 3A,B). Complementation with plzA, but not plzB, restored normal colony size (swarm diameter). The chemotactic responses of each strain to N-acetyl-D-glucosamine (NAG) were also assessed. Deletion of plzA had no effect on chemotactic responses to NAG (Figure 3C). Note that other potential chemoattractants were not tested. The data indicate that PlzA plays a direct or indirect role in translational motility, but not rotational motility, and that this specific PlzA function is not complemented by PlzB.
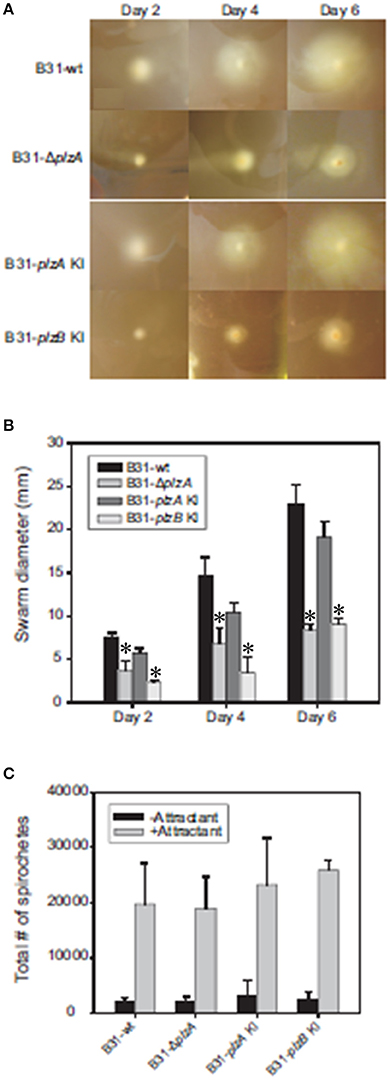
Figure 3. Motility and chemotaxis analyses. B. burgdorferi B31-wt, B31-ΔplzA, B31-plzA KI, and B31-plzB KI were evaluated for motility using a “plate swarming” assay as detailed in the text. Photographs of representative plates taken 2, 4, and 6 days after inoculation of the plates are shown in (A). The diameters of each expanding zone of growth was determined and are presented as bar graphs in (B). In (C), the results of capillary tube-based chemotaxis assays using N-acetyl glutamic acid as the chemoattractant are shown. The assays were conducted as detailed in the text and statistical significance was assessed using the One-Way ANOVA. *Statistical significance was assessed relative to wild type (p < 0.05).
Assessment of the Ability of B. burgdorferi B31 Wild Type, plzA Gene Deletion and plzA/B cis-Complemented Strains to Infect Mice
Each strain was delivered to five C3H-HeJ mice by subcutaneous needle inoculation. Tissue and organ biopsies (bladder, heart, and skin) were harvested after 4 weeks, sections were placed in BSK and spirochete growth monitored. All biopsy samples (n = 15; one sample from heart, bladder and tissue from each mouse) yielded positive cultures except those obtained from mice that had been inoculated with B31-ΔplzA and B31-plzB KI (Table 3). All mice that yielded positive cultures were also seropositive as assessed by ELISA (Figure 4A). The IgG titers in mice infected with B31-wt and B31-plzA KI were similar while significantly lower titers were measured in B31-ΔplzA and B31plzB KI (p < 0.01). Immunoblot analyses using B. burgdorferi B31whole cell lysate as the detecting antigen yielded results that were consistent with the ELISA and cultivation data (Figure 4B). As expected, the immunoblot analyses revealed that a wide range of proteins were recognized by antibody elicited by infection.
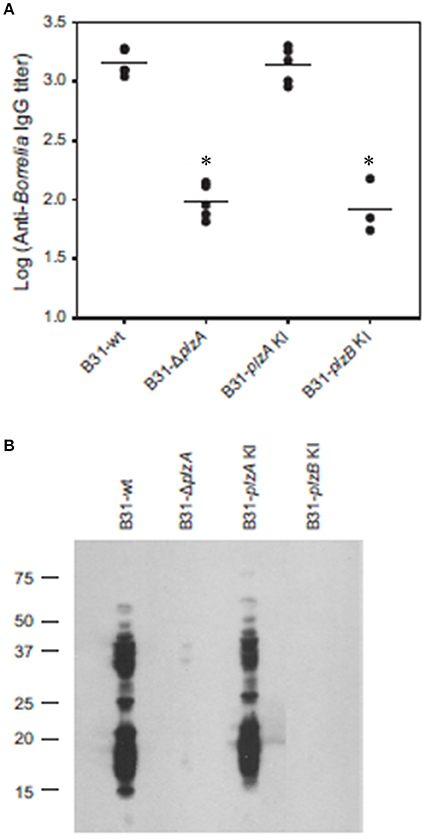
Figure 4. PlzB does not restore infectivity to plzA gene deletion mutant delivered by needle inoculation. C3H-HeJ mice were inoculated by subcutaneous needle injection with 104 spirochetes of each strain investigated. Serum was collected from each mouse 4 weeks post-inoculation and anti-B. burgdorferi IgG titer was determined by ELISA (endpoint dilution) using whole cell lysate as the detecting antigen (A). (B) Shows the results of immunoblot analyses. B. burgdorferi B31 served as the immobilized antigen. For the immunoblot analyses, serum samples from each experimental group were pooled. All methods were conducted as detailed in the text and statistical significance was assessed using the One-Way ANOVA. *Statistical significance was assessed relative to wild type (p < 0.05).
The ability of each strain to infect ticks was assessed using laboratory-reared Ixodes scapularis larvae. The larvae were infected by immersion in high density cultures of each strain, washed and then tested for spirochetes using PCR. All strains were able to cause at least a transient infection in ticks, indicating that PlzA and PlzB are not strictly required for early survival in the larval ticks (Table 4). Note that long term survival in ticks was not assessed in this study. Infected larval ticks were then fed on näive mice to determine if PlzA is required for spirochete transmission from ticks to mice. Infection status of the mice was assessed by serological analyses (ELISA and immunoblot) (Figure 5). All strains except B31-ΔplzA and B31-plzB KI were successfully transmitted from ticks to mammals as evidence by an antibody response. It should be noted, however, that only 1 of 3 mice that were exposed to ticks carrying B31-plzA KI developed antibody titers equivalent to that of the parental strain. The basis for the decreased efficiency of transmission of this strain is unknown. Immunoblot analyses using the pooled serum demonstrated that an antibody response to numerous proteins developed. Interestingly, there were significant differences in the array of proteins that were detected by infection-induced antibody in mice that were needle inoculated versus those that were infected by transmission from ticks (compare Figures 4B, 5B). Most notably, the mice infected by needle inoculation developed antibody against proteins in the 30–35 kDa range whereas the mice infected by tick feeding did not. Although not directly assessed, this difference most likely reflects differing antibody responses to OspA and OspB. It is firmly established in the literature that OspA and B are expressed at high levels in spirochetes cultured in vitro but not by spirochetes that transit into mammals from ticks. It can be concluded that PlzA is not strictly required to establish infection in ticks but is required to either allow spirochetes to transit from ticks to mice or to survive upon entering the mammalian environment. Importantly, these analyses also suggest that PlzB cannot substitute for or complement functions lost as a result of deletion of plzA.
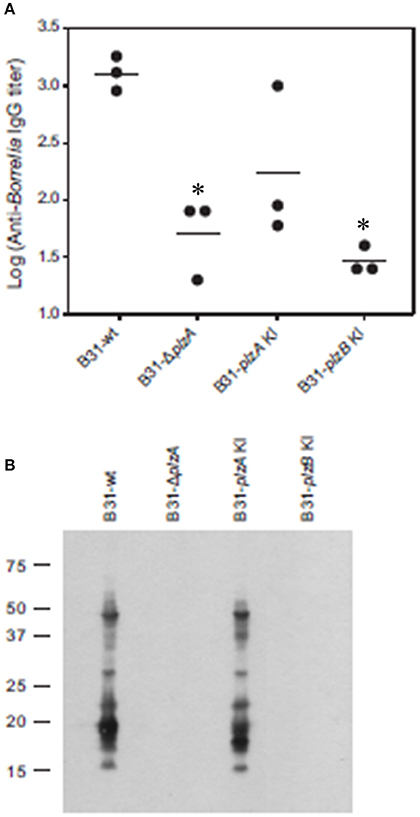
Figure 5. Tick to mouse spirochete transmission analyses. I. scapularis larvae were infected with each strain by immersion in actively growing cultures. After verifying infection, the infected larvae were fed on naive C3H-HeJ mice. Serum was collected from each mouse 4 weeks post-inoculation and anti-B. burgdorferi IgG titer was determined by ELISA (endpoint dilution) using whole cell lysate as the detecting antigen (A). (B) Shows the results of immunoblot analyses. B. burgdorferi B31 served as the immobilized antigen. For the immunoblot analyses, serum samples from each experimental group were pooled. All methods were conducted as detailed in the text and statistical significance was assessed using the One-Way ANOVA. *Statistical significance was assessed relative to wild type (p < 0.05).
Discussion
Cyclic di-nucleotides (CDN) are secondary messenger molecules that contribute to the regulation of a wide range of biological processes in bacteria (Jenal et al., 2018). Of these, c-di-GMP, which was discovered nearly 40 years ago (Ross et al., 1987), has been the most intensively studied. While there is considerable information about the function, domain organization and structure of DGCs and PDEs that produce and degrade c-di-GMP, respectively (Galperin et al., 2001; Jenal and Malone, 2006; Stock, 2007; Sondermann et al., 2012), the effector mechanisms of c-di-GMP in Borrelia are only partially understood (reviewed in Novak et al., 2014). In other bacteria, c-di-GMP exerts its regulatory activities through multiple mechanisms including direct binding to RNA riboswitches, transcriptional regulators, and a functionally and architecturally diverse group of proteins that harbor PilZ domains (Amikam and Galperin, 2006; Jenal and Malone, 2006; Römling and Amikam, 2006; Benach et al., 2007; Hengge, 2009; Jenal et al., 2018). The LD and relapsing fever spirochetes encode only a single known c-di-GMP receptor; PlzA and PlzC, respectively (Mallory et al., 2016). The dearth of c-di-GMP receptors in Borrelia is atypical, as most bacteria produce multiple c-di-GMP receptors. Earlier studies revealed that a subset of LD spirochete isolates carry a gene encoding a second PilZ domain protein designated as PlzB (Freedman et al., 2009). BLAST analyses of available genome sequences revealed limited distribution of plzB among Lyme disease isolates, which may reflect its location on a linear plasmid that is not essential for survival in ticks or mammals.
The ability of PlzB to bind c-di-GMP was assessed through nucleotide binding assays. Competitive binding experiments revealed that PlzB binds c-di-GMP in a specific manner (Figure 1). C-di-GMP binding was not inhibited by GTP, GMP, ATP, cGMP, or cAMP. PlzA and PlzC have been demonstrated to undergo significant structural rearrangement upon binding c-di-GMP (Mallory et al., 2016). It remains to be determined if a similar ligand-induced structural rearrangement occurs with PlzB. It can be concluded that PlzB binds c-di-GMP with high specificity and has the potential to serve as a c-di-GMP receptor in vivo.
To determine if PlzA and PlzB have distinct or synonymous functions, gene deletion and allelic exchange replacement studies were conducted in the B. burgdorferi strain B31 genetic background (see Figure 2). A plzA gene deletion mutant was generated by replacing the chromosomally encoded gene with a strepR cassette (B31-ΔplzA). This strain served as the parental strain for subsequent genetic manipulations. plzA-kanR and plzB-kanR cassettes were used to replace the strepR cassette of B31-ΔplzA to yield B31-plzA KI and B31-plzB KI. The plzB gene originated from B. burgdorferi ZS7. RT-PCR analyses confirmed that plzB is transcribed in the B31-plzB KI strain. As expected, plzB transcript was not detected in strains that lack plzB. Efforts to demonstrate PlzB protein production using immunoblot or IFA were inconclusive. This may be due to low level plzB expression. Consistent with this, earlier studies were unable to detect PlzA during in vitro cultivation (Freedman et al., 2009; Pitzer et al., 2011; He et al., 2014). In addition to low level expression, PlzA may also be compartmentalized in cells, potentially near the flagellar motors.
Interruption of c-di-GMP pathways, or an imbalance in its synthesis and degradation, has been demonstrated to result in aberrant motility phenotypes of B. burgdorferi (Freedman et al., 2009; Rogers et al., 2009; Caimano et al., 2011; Kostick et al., 2011; Pitzer et al., 2011; Sultan et al., 2011). Here the impact of genetic manipulation on both rotational and translational motility was assessed (results are summarized in Table 2). Strains were grown in BSK with or without 1% CMC. CMC increases the viscosity of the media and thus simulates the dense matrices spirochetes encounter in ticks and mammals. Rotational motility in BSK with or without CMC was not affected by deletion of plzA, indicating that PlzA is not required for the flagellar motors to function. Interestingly, relative to B31-wt and B31-plzA KI; B31ΔplzA and B31-plzB KI had significantly reduced translational movement with a significant decrease in flex frequency in BSK with CMC. Flexing is an inherent aspect of spirochetal motility that occurs when the flagellar motors located at opposite ends of the cell transiently rotate in the same direction. DIC microscopy revealed that the translational rate of B31-ΔplzA and B31-plzB KI was only 40% of that of the wt and B31-plzA KI complemented strains.
B31-ΔplzA and B31-plzB KI also displayed significantly reduced motility in swarm plate assays (Figure 3). Note that in the Borrelia field, the term “swarm assay” has been widely used to describe an assay that measures migration distance from a point of inoculation in a semi-solid media plate (i.e., colony size). To be clear, this is distinctly different from the phenomenon of “swarm motility” that has been described for some other bacteria including Caulobacter (Aldridge et al., 2003). We observed a significant decrease in colony size for the plzA deletion mutant (relative to wild type). Normal colony size was restored by complementation with plzA but not plzB. Pitzer et al also reported a similar level of reduced swarming. It is important to note that they also reported a change in colony morphology with the mutant and parental strains forming opaque and translucent colonies, respectively. The variable appearance of the colonies was concluded to represent phase variation. However, we saw no evidence of a distinct colony morphology for the B31-ΔplzA or B31-plzB KI strains. This discrepancy may reflect a simple difference in interpretation. While we observed denser “colonies” at the plate inoculation site, we suggest that this simply reflects a concentration of cells as would be expected based on their attenuated motility. Furthermore, Pitzer et al. did not demonstrate changes in protein expression that might be indicative of phase variation. While the overall findings of this report and the Pitzer study are in good agreement, there are subtle differences (Pitzer et al., 2011). It is possible that this is due to differences in the wt and parental strains used in each study. Pitzer used a clone derived from B31, designated as B31-A3-68, that has been engineered to have increased transformation efficiency. In this clone, ORF bbe02 was replaced with a PflgB-aadA cassette. This strain also lacks linear plasmid 56 and circular plasmid 9. The B31 derived clone (5A4) used here has not been subject to previous genetic manipulation and thus harbors bbe02. It is possible parental strain differences could be a contributing factor to the differences noted in ΔplzA mutant phenotypes between these two studies. We conclude that yet to be identified aspects of flagellar motor function are controlled directly or indirectly, at least in part, by PlzA and that PlzB cannot complement these functions.
Here we also investigated chemotaxis, as this represents another cellular activity that is dependent on controlled and directed motility (Figure 3C). Earlier studies demonstrated that c-di-GMP positively regulates the transcription of several genes involved in chemotaxis and NAG metabolism (Rogers et al., 2009; Kostick et al., 2011). Consistent with this, strains deficient in c-di-GMP production displayed reduced chemotaxis toward N-acetyl glucosamine (NAG). However, deletion of plzA did not result in a statistically significant difference in chemotaxis. While it is possible that chemotaxis to other chemoattractants could be affected, in this study we focused solely on NAG, a chemoattractant that is abundant in ticks.
Infectivity studies in C3H-HeJ mice using needle delivered spirochetes revealed that B31ΔplzA was non-infectious at a dose of 104 spirochetes (Figure 4). This observation differs slightly from an earlier report that demonstrated that the infectivity of plzA deletion mutant was attenuated with an ID50 of 104 (Pitzer et al., 2011). Cis-complementation of the B31ΔplzA strain in both this and the Pitzer study restored infectivity. We also sought to determine if the loss of PlzA function could be restored by cis-complementation of B31ΔplzA with plzB. As discussed above, while plzB transcription was demonstrated in the complemented strain, the ability to infect mice was not. All other strains infected mice and efficiently disseminated to distal tissues and organs. It can be concluded that PlzA is an important virulence factor required for B. burgdorferi to efficiently infect mice when delivered by needle inoculation.
The ability to infect mice when delivered by tick bite was also assessed (Figure 5). Since B31ΔplzA and B31-plzB KI were not able to infect mice when delivered by needle inoculation, ticks could not be infected by feeding larvae on mice. Hence, an “immersion feeding” approach was applied (Policastro and Schwan, 2003). This approach has proven effective and serves as a surrogate approach for studying the transmission process of strains that are not-infectious in mice (Kostick et al., 2011; Pitzer et al., 2011). The efficiency of infecting ticks by this approach was determined by PCR analysis of a random sampling of ticks to be >80%. All strains, including the mutant and complemented strains, infected ticks with similar efficiency. Pitzer also reported that a plzA gene deletion mutant could infect ticks. They also noted that the spirochete burden was significant reduced by day 7 post-immersion (Pitzer et al., 2011). In this report, we did not specifically assess the long-term survival of each strain in ticks. To assess transmission from ticks, 10 larval ticks were allowed to feed to repletion on mice. It is important to note in this report only 1 of 3 mice became infected with the complemented strain. It is unclear if this represents reduced infectivity or simply is a consequence of the small group size used in this experiment. In any event, the successful complementation of the non-infectious plzA mutant suggests that the non-infectious phenotype of B31ΔplzA was not the result of an unrelated secondary event. Cultivation and serological assays revealed B31-ΔplzA to be non-infectious. The B31-plzB KI strain was also determined to be compromised in its ability to transit from ticks to mice and or establish infection in mice. This observation, coupled with the needle inoculation analyses detailed above, suggests that the functions of PlzA and PlzB are not synonymous.
PlzA has been demonstrated to have global regulatory activity through its interactions with BosR and RpoS (He et al., 2014). It has been generally assumed that c-di-GMP binding serves to activate PlzA and consistent with this, FRET based analyses have revealed that PlzA undergoes significant structural rearrangements upon binding c-di-GMP (Mallory et al., 2016). While c-di-GMP binding could potentially serve as an on-off switch for PlzA function, studies presented to date suggest that the story is more complex. First, it has been firmly established that B. burgdorferi Δrrp1 mutants, which lack the ability to produce c-di-GMP, are infectious in mice but not in ticks. Second, as demonstrated here and in earlier reports, PlzA is required to infect mammals but is not essential for infection of ticks. plzA transcription, like that of rrp1, is upregulated in fed ticks (Freedman et al., 2009; Rogers et al., 2009). Since PlzA is the only known c-di-GMP receptor of most B. burgdorferi isolates, the concurrent upregulation of rrp1 and plzA in ticks is not unexpected and could point to PlzA having a c-di-GMP dependent function in ticks. However, the fact that an rrp1−/plzA+ strain can infect mice while an rrp1+/plzA− strain cannot, suggests that the essential functions mediated by PlzA in mammals are not dependent on c-di-GMP. It is our hypothesis that PlzA is a multi-functional protein that can carry out c-di-GMP dependent and c-di-GMP independent functions in different environments (i.e., tick and mammal).
In conclusion, this study provides further insight into the contribution of c-di-GMP receptors in Borrelia biology in both the tick and mammalian environment. It is striking that so few receptors have been identified in the Borrelia. It is probable that additional receptors or c-di-GMP binding partners (riboswitches, transcription factors etc.) do in fact exist but have not been identified by the methods that have been employed thus far. One of the confounding factors that could complicate the identification of additional c-di-GMP receptors is the environmentally regulated differential expression of a large number of Borrelia genes. There may be potential receptors that are produced during infection but not produced during cultivation. Regarding the biological significance of PlzB, it is clear that PlzB is not strictly required for Borrelia survival in ticks, in mammals or in culture. The potential in vivo functions of PlzB and its biological significance remains to be determined. As suggested in an earlier study, it is possible that the presence of both genes in a cell might enhance fitness and adaptive responses (Kostick et al., 2011). Studies that directly address this hypothesis are currently underway.
Author Contributions
JK-D conducted most of the experiments described within. JI, JF, LS, and LO all conducted experiments that were essential for confirmation of data presented within. JD and RM conceptualized the study framework and wrote the manuscript. JI and LO critiqued the manuscript and provided edits.
Funding
This study was supported by internal funds from Virginia Commonwealth University. There was no external funding.
Conflict of Interest Statement
The handling Editor declared a past co-authorship with one of the authors RM.
The remaining authors declare that the research was conducted in the absence of any commercial or financial relationships that could be construed as a potential conflict of interest.
Acknowledgments
Microscopy was performed at the VCU Department of Anatomy and Neurobiology Microscopy Facility, supported, in part, with funding from NIH-NINDS Center core grant (5P30NS047463–02).
References
Adeolu, M., and Gupta, R. S. (2014). A phylogenomic and molecular marker based proposal for the division of the genus Borrelia into two genera: the emended genus Borrelia containing only the members of the relapsing fever Borrelia, and the genus Borreliella gen. nov. containing the members of the Lyme disease Borrelia (Borrelia burgdorferi sensu lato complex). Ant. Van Leeuwenhoek 105, 1049–1072. doi: 10.1007/s10482-014-0164-x
Aldridge, P., Paul, R., Goymer, P., Rainey, P., and Jenal, U. (2003). Role of the GGDEF regulator PleD in polar development of Caulobacter crescentus. Mol. Microbiol. 47, 1695–1708. doi: 10.1046/j.1365-2958.2003.03401.x
Amikam, D., and Galperin, M. Y. (2006). PilZ domain is part of the bacterial c-di-GMP binding protein. Bioinformatics 22, 3–6. doi: 10.1093/bioinformatics/bti739
Benach, J., Swaminathan, S. S., Tamayo, R., Handelman, S. K., Folta-Stogniew, E., Ramos, J. E., et al. (2007). The structural basis of cyclic diguanylate signal transduction by PilZ domains. EMBO J. 26, 5153–5166. doi: 10.1038/sj.emboj.7601918
Burgdorfer, W., Barbour, A. G., Hayes, S. F., Benach, J. L., Grunwaldt, E., and Davis, J. P. (1982). Lyme disease–a tick-borne spirochetosis? Science 216:1317–1319. doi: 10.1126/science.7043737
Caimano, M. J., Drecktrah, D., Kung, F., and Samuels, D. S. (2016). Interaction of the Lyme disease spirochete with its tick vector. Cell Microbiol. 18, 919–927. doi: 10.1111/cmi.12609
Caimano, M. J., Dunham-Ems, S., Allard, A. M., Cassera, M. B., Kenedy, M., and Radolf, J. D. (2015). Cyclic di-GMP modulates gene expression in lyme disease spirochetes at the tick-mammal interface to promote spirochete survival during the blood meal and tick-to-mammal transmission. Infect. Immun. 83, 3043–3060. doi: 10.1128/IAI.00315-15
Caimano, M. J., Kenedy, M. R., Kairu, T., Desrosiers, D. C., Harman, M., Dunham-Ems, S., et al. (2011). The hybrid histidine kinase Hk1 is part of a two-component system that is essential for survival of Borrelia burgdorferi in feeding Ixodes scapularis ticks. Infect. Immun. 79, 3117–3130. doi: 10.1128/IAI.05136-11
Earnhart, C. G., Leblanc, D. V., Alix, K. E., Desrosiers, D. C., Radolf, J. D., and Marconi, R. T. (2010). Identification of residues within ligand-binding domain 1 (LBD1) of the Borrelia burgdorferi OspC protein required for function in the mammalian environment. Mol. Microbiol. 76, 393–408. doi: 10.1111/j.1365-2958.2010.07103.x
Frank, K. L., Bundle, S. F., Kresge, M. E., Eggers, C. H., and Samuels, D. S. (2003). aadA confers streptomycin resistance in Borrelia burgdorferi. J. Bacteriol. 185, 6723–6727. doi: 10.1128/JB.185.22.6723-6727.2003
Freedman, J. C., Rogers, E. A., Kostick, J. L., Zhang, H., Iyer, R., Schwartz, I., et al. (2009). Identification and molecular characterization of a cyclic-di-GMP effector protein, PlzA (BB0733): additional evidence for the existence of a functional cyclic-di-GMP regulatory network in the Lyme disease spirochete, Borrelia burgdorferi. FEMS Immunol. Med. Microbiol. 58, 285–294. doi: 10.1111/j.1574-695X.2009.00635.x
Galperin, M. Y., Nikolskaya, A. N., and Koonin, E. V. (2001). Novel domains of the prokaryotic two-component signal transduction systems. FEMS Microbiol. Lett. 203, 11–21. doi: 10.1111/j.1574-6968.2001.tb10814.x
He, M., Zhang, J. J., Ye, M., Lou, Y., and Yang, X. F. (2014). Cyclic Di-GMP receptor PlzA controls virulence gene expression through RpoS in Borrelia burgdorferi. Infect. Immun. 82, 445–452. doi: 10.1128/IAI.01238-13
Hengge, R. (2009). Principles of c-di-GMP signalling in bacteria. Nat. Rev. Microbiol. 7, 263–273. doi: 10.1038/nrmicro2109
Hyde, J. A., Seshu, J., and Skare, J. T. (2006). Transcriptional profiling of Borrelia burgdorferi containing a unique bosR allele identifies a putative oxidative stress regulon. Microbiology 152(Pt 9), 2599–2609. doi: 10.1099/mic.0.28996-0
Hyde, J. A., Shaw, D. K., Smith Iii, R., Trzeciakowski, J. P., and Skare, J. T. (2009). The BosR regulatory protein of Borrelia burgdorferi interfaces with the RpoS regulatory pathway and modulates both the oxidative stress response and pathogenic properties of the Lyme disease spirochete. Mol. Microbiol. 74, 1344–1355. doi: 10.1111/j.1365-2958.2009.06951.x
Hyde, J. A., Trzeciakowski, J. P., and Skare, J. T. (2007). Borrelia burgdorferi alters its gene expression and antigenic profile in response to CO2 levels. J. Bacteriol. 189, 437–445. doi: 10.1128/JB.01109-06
Jenal, U., and Malone, J. (2006). Mechanisms of cyclic-di-GMP signaling in bacteria. Annu. Rev. Genet. 40:385–407. doi: 10.1146/annurev.genet.40.110405.090423
Jenal, U., Reinders, A., and Lori, C. (2018). Cyclic di-GMP: second messenger extraordinaire. Nat. Rev. Microbiol. 15, 271–284. doi: 10.1038/nrmicro.2016.190
Kostick, J. L., Szkotnicki, L. T., Rogers, E. A., Bocci, P., Raffaelli, N., and Marconi, R. T. (2011). The diguanylate cyclase, Rrp1, regulates critical steps in the enzootic cycle of the Lyme disease spirochetes. Mol. Microbiol. 81, 219–231. doi: 10.1111/j.1365-2958.2011.07687.x
Labandeira-Rey, M., Seshu, J., and Skare, J. T. (2003). The absence of linear plasmid 25 or 28-1 of Borrelia burgdorferi dramatically alters the kinetics of experimental infection via distinct mechanisms. Infect. Immun.. 71, 4608–4613 doi: 10.1128/IAI.71.8.4608-4613.2003
Mallory, K. L., Miller, D. P., Oliver, L. D. Jr., Freedman, J. C., Kostick-Dunn, J. L., Carlyon, J. A., et al. (2016). Cyclic-di-GMP binding induces structural rearrangements in the PlzA and PzC proteins of the Lyme disease and relapsing fever functions. Pathog. Dis. 74:pii: ftw105. doi: 10.1093/femspd/ftw105
Novak, E. A., Sultan, S. Z., and Motaleb, M. A. (2014). The cyclic-di-GMP signaling pathway in the Lyme disease spirochete, Borrelia burgdorferi. Front. Cell. Infect. Microbiol. 4:56. doi: 10.3389/fcimb.2014.00056
Ouyang, Z., Deka, R. K., and Norgard, M. V. (2011). BosR (BB0647) controls the RpoN-RpoS regulatory pathway and virulence expression in Borrelia burgdorferi by a novel DNA-binding mechanism. PLoS Pathog. 7:e1001272. doi: 10.1371/journal.ppat.1001272
Pitzer, J. E., Sultan, S. Z., Hayakawa, Y., Hobbs, G., Miller, M. R., and Motaleb, M. A. (2011). Analysis of the Borrelia burgdorferi cyclic-di-GMP binding protein PlzA reveals a role in motility and virulence. Infect. Immun. 79:1815–1825. doi: 10.1128/IAI.00075-11
Policastro, P. F., and Schwan, T. G. (2003). Experimental infection of Ixodes scapularis larvae (Acari: Ixodidae) by immersion in low passage cultures of Borrelia burgdorferi. J. Med. Entomol. 40, 364–370. doi: 10.1603/0022-2585-40.3.364
Rogers, E. A., and Marconi, R. T. (2007). Delineation of species-specific binding properties of the CspZ protein (BBH06) of Lyme disease spirochetes: evidence for new contributions to the pathogenesis of Borrelia spp. Infect. Immun. 75, 5272–5281. doi: 10.1128/IAI.00850-07
Rogers, E. A., Terekhova, D., Zhang, H. M., Hovis, K. M., Schwartz, I., and Marconi, R. T. (2009). Rrp1, a cyclic-di-GMP-producing response regulator, is an important regulator of Borrelia burgdorferi core cellular functions. Mol. Microbiol. 71, 1551–1573. doi: 10.1111/j.1365-2958.2009.06621.x
Römling, U., and Amikam, D. (2006). Cyclic di-GMP as a second messenger. Curr. Opin. Microbiol. 9, 218–228. doi: 10.1016/j.mib.2006.02.010
Ross, P., Weinhouse, H., Aloni, Y., Michaeli, D., Weinberger-Ohana, P., Mayer, R., et al. (1987). Regulation of cellulose synthesis in Acetobacter xylinum by cyclic diguanylic acid. Nature 325, 279–281. doi: 10.1038/325279a0
Ryjenkov, D. A., Tarutina, M., Moskvin, O. V., and Gomelsky, M. (2005). Cyclic diguanylate is a ubiquitous signaling molecule in bacteria: insights into biochemistry of the GGDEF protein domain. J. Bacteriol. 187, 1792–1798. doi: 10.1128/JB.187.5.1792-1798.2005
Samuels, D. S., Mach, K., and Garon, C. F. (1994). Genetic transformation of the Lyme disease agent Borrelia burgdorferi with coumarin-resistant gyrB. J. Bacteriol. 176: 6045–6049. doi: 10.1128/jb.176.19.6045-6049.1994
Seshu, J., Boylan, J. A., Hyde, J. A., Swingle, K. L., Gherardini, F. C., and Skare, J. T. (2004). A conservative amino acid change alters the function of BosR, the redox regulator of Borrelia burgdorferi. Mol. Microbiol. 54, 1352–1363. doi: 10.1111/j.1365-2958.2004.04352.x
Sondermann, H., Shikuma, N. J., and Yildiz, F. H. (2012). You've come a long way: c-di-GMP signaling. Curr. Opin. Microbiol. 15, 140–146. doi: 10.1016/j.mib.2011.12.008
Stewart, P. E., Thalken, R., Bono, J. L., and Rosa, P. (2001). Isolation of a circular plasmid region sufficient for autonomous replication and transformation of infectious Borrelia burgdorferi. Mol. Microbiol. 39:714–721. doi: 10.1046/j.1365-2958.2001.02256.x
Stock, A. M. (2007). Diguanylate cyclase activation: it takes two. Structure 15, 887–888. doi: 10.1016/j.str.2007.07.003
Sultan, S. Z., Pitzer, J. E., Boquoi, T., Hobbs, G., Miller, M. R., and Motaleb, M. A. (2011). Analysis of the HD-GYP domain cyclic dimeric GMP phosphodiesterase reveals a role in motility and the enzootic life cycle of Borrelia burgdorferi. Infect. Immun. 79, 3273–3283. doi: 10.1128/IAI.05153-11
Sultan, S. Z., Pitzer, J. E., Miller, M. R., and Motaleb, M. A. (2010). Analysis of a Borrelia burgdorferi phosphodiesterase demonstrates a role for cyclic-di-guanosine monophosphate in motility and virulence. Mol. Microbiol. 77, 128–142. doi: 10.1111/j.1365-2958.2010.07191.x
Sung, S. Y., McDowell, J. V., and Marconi, R. T. (2001). Evidence for the contribution of point mutations to vlsE variation and for apparent constraints on the net accumulation of sequence changes in vlsE during infection with Lyme disease spirochetes. J. Bacteriol. 183, 5855–5861. doi: 10.1128/JB.183.20.5855-5861.2001
Sze, C. W., Smith, A., Choi, Y. H., Yang, X., Pal, U., Yu, A., et al. (2013). Study of the response regulator Rrp1 reveals its regulatory role on chitobiose utilization and virulence of Borrelia burgdorferi. Infect. Immun. 81, 1775–1787. doi: 10.1128/IAI.00050-13
Keywords: Borrelia, Lyme disease, PlzB, cyclic-di-GMP, PlzA, PilZ
Citation: Kostick-Dunn JL, Izac JR, Freedman JC, Szkotnicki LT, Oliver LD Jr and Marconi RT (2018) The Borrelia burgdorferi c-di-GMP Binding Receptors, PlzA and PlzB, Are Functionally Distinct. Front. Cell. Infect. Microbiol. 8:213. doi: 10.3389/fcimb.2018.00213
Received: 20 March 2018; Accepted: 07 June 2018;
Published: 11 July 2018.
Edited by:
Melissa Jo Caimano, University of Connecticut Health Center, United StatesReviewed by:
Jon Blevins, University of Arkansas for Medical Sciences, United StatesJenny A. Hyde, Texas A&M Health Science Center, United States
Copyright © 2018 Kostick-Dunn, Izac, Freedman, Szkotnicki, Oliver and Marconi. This is an open-access article distributed under the terms of the Creative Commons Attribution License (CC BY). The use, distribution or reproduction in other forums is permitted, provided the original author(s) and the copyright owner(s) are credited and that the original publication in this journal is cited, in accordance with accepted academic practice. No use, distribution or reproduction is permitted which does not comply with these terms.
*Correspondence: Richard T. Marconi, cmljaGFyZC5tYXJjb25pQHZjdWhlYWx0aC5vcmc=