- 1Departamento de Infectómica y Patogénesis Molecular, Centro de Investigación y de Estudios Avanzados del Instituto Politécnico Nacional, Ciudad de México, Mexico
- 2Sección de Estudios de Posgrado e Investigación, Instituto Politécnico Nacional, Escuela Superior de Medicina, Ciudad de México, Mexico
- 3Coordinación de Morfología, Departamento de Formación Básica Disciplinaria, Instituto Politécnico Nacional, Escuela Superior de Medicina, Ciudad de México, Mexico
Over the past 20 years, gastrointestinal infections in developing countries have been a serious health problem and are the second leading cause of morbidity among all age groups. Among pathogenic protozoans that cause diarrheal disease, the parasite Entamoeba histolytica produces amebic colitis as well as the most frequent extra-intestinal lesion, an amebic liver abscess (ALA). Usually, intestinal amebiasis and ALA are treated with synthetic chemical compounds (iodoquinol, paromomycin, diloxanide furoate, and nitroimidazoles). Metronidazole is the most common treatment for amebiasis. Although the efficacy of nitroimidazoles in killing amebas is known, the potential resistance of E. histolytica to this treatment is a concern. In addition, controversial studies have reported that metronidazole could induce mutagenic effects and cerebral toxicity. Therefore, natural and safe alternative drugs against this parasite are needed. Flavonoids are natural polyphenolic compounds. Flavonoids depend on malonyl-CoA and phenylalanine to be synthesized. Several flavonoids have anti-oxidant and anti-microbial properties. Since the 1990s, several works have focused on the identification and purification of different flavonoids with amebicidal effects, such as, -(-)epicatechin, kaempferol, and quercetin. In this review, we investigated the effects of flavonoids that have potential amebicidal activity and that can be used as complementary and/or specific therapeutic strategies against E. histolytica trophozoites. Interestingly, it was found that these natural compounds can induce morphological changes in the amebas, such as chromatin condensation and cytoskeletal protein re-organization, as well as the upregulation and downregulation of fructose-1,6-bisphosphate aldolase, glyceraldehyde-phosphate dehydrogenase, and pyruvate:ferredoxin oxidoreductase (enzymes of the glycolytic pathway). Although the specific molecular targets, bioavailability, route of administration, and doses of some of these natural compounds need to be determined, flavonoids represent a very promising and innocuous strategy that should be considered for use against E. histolytica in the era of microbial drug resistance.
Introduction
Entamoeba histolytica is a protozoan pathogen in humans and is the causative agent of amebiasis. This disease produces 100,000 deaths each year, particularly in developing countries. E. histolytica can manifest as a commensal or invasive microorganism in the large intestine, producing amebic colitis. The parasite has two cell cycle stages, the cyst and the trophozoite. The cysts are secreted in the stool from individuals who harbor E. histolytica. The trophozoites emerge from the cysts in the intestine, and this vegetative form is able to invade the intestinal mucosa and to disseminate to the liver, producing amebic liver abscess (ALA) (Sehgal et al., 1996; Haque et al., 2003). Few infected persons show symptoms with invasion to the bowel or extraintestinal sites, mainly in the liver (Stanley, 2003).
Diverse drugs have been employed against amebiasis. The treatment depends on the diagnosis and severity of the illness (Table 1). Usually, the drug can attack luminal forms and invasive amebiasis. However, in symptomatic patients and in invasive disease, the most widely used drugs against E. histolytica are the nitroimidazoles (metronidazole and tinidazole) (Marie and Petri, 2013; Ansari et al., 2015). Metronidazole (MTZ) kills amebas but does not cause damage to cysts. Although MTZ is the standard compound for treating amebiasis, it causes adverse effects, such as diarrhea, metallic flavor, loss of appetite, and nausea due to the doses and long-term treatment (1.5 g/day for 10 days) (Haque et al., 1997, 2003; Stanley, 2003). There are in vivo and in vitro studies where the nitroimidazoles induce genotoxic effects, which are related to the ability of cells to reduce these drugs; moreover, the position of the CH3 and NO2 groups of these compounds are involved in DNA damage (Boechat et al., 2015). In the case of MTZ, it is known that its biotransformation produces nitroso intermediates (e.g., hydroxy metabolite and acetic acid), which can form adducts in the DNA or inhibit the thioredoxin reductase-generating reactive oxygen species, causing oxidative cell damage (Mudry et al., 1994; Elizondo et al., 1996; Leitsch et al., 2007). Furthermore, MTZ can cross the blood-brain barrier producing cerebellar toxicity (Agarwal et al., 2016). Thus, it is important to consider the doses of MTZ administration, the individual susceptibility and to evaluate the risk-benefit in relation with the severity of the infection (Mudry et al., 1994; Elizondo et al., 1996).
Because of the undesirable effects of MTZ, it is necessary to develop an alternative treatment from biological or synthetic sources that can eliminate E. histolytica. Specifically, the development or discovery of novel compounds without toxicity and side effects is needed. In many countries, plant extracts have been employed ancestrally and are a good alternative to treat amebiasis. Furthermore, it is necessary to validate, identify and purify the active compounds with anti-amebic properties before employing them as a treatment.
Usually, in plants, polyphenolic metabolites can be present in roots, leaves, flowers, and fruits. Flavonoids are contained within these natural compounds, which have demonstrated promising results mainly in in vitro studies; however, it is necessary to evaluate their potential activity in in vivo models as a complementary and/or specific therapeutic strategy against E. histolytica trophozoites, as we describe below.
Flavonoid Structure and Classification
Flavonoids are natural pigments present in vegetables. These compounds protect organisms against the damage produced by oxidant agents such as UV radiation, pollution, and the chemical substances present in the foods. Humans obtain these protector flavonoids through direct ingestion of aliments and supplements. The chemical structure of flavonoids is characterized by the presence of a variable number of phenolic hydroxyl groups (polyphenols). Flavonoids have low molecular weights (500–4,000 Da) and share a common skeleton of diphenylpyranes (C6-C3-C6) composed of two phenyl rings linked through a ring C of pyran (Figure 1). These compounds present excellent iron-chelating ability that gives them great anti-oxidant capacity (Havsteen, 1983). The anti-oxidant activity of flavonoids depends on the redox properties of their phenolic groups (Bors et al., 1990). According to their structural characteristics (the nature of the C3 element), flavonoids can be classified in Table 2. Different chemical modifications may occur in each group, such as hydroxylation, hydrogenation, sulfuration, methylation, acylation, and glycosylation (Andersen and Markham, 2006; Wang et al., 2017).
Flavones
The flavone family is a subgroup of flavonoids that is synthesized depending on whether they contain C- or O-glycosylation and a hydroxylated B-ring. These compounds have been mainly isolated from leaves, aerial parts, and the exudates of plants. Flavones are characterized by a double bond between C-2 and C-3 and a B ring in C-2 (Jiang et al., 2016). Flavones present anti-oxidant activities due to their ability to scavenge reactive oxygen species (ROS). For example, luteolin inhibits xanthine oxidase, which is an important enzyme that is involved in ROS production (Cos et al., 1998; Spanou et al., 2012). Apigenin reduces the phosphorylation of NF-κB/p65 in mouse macrophages and in human monocytes, inhibiting its transcriptional activity and the expression of pro-inflammatory cytokines (Nicholas et al., 2007). Acacetin has shown important anti-peroxidative and anti-inflammatory activities, and these effects were determined by inhibiting iNOS and COX-2 activity in murine macrophages (Pan et al., 2006) (Table 2).
Flavonols
Flavonols are composed of multiple phenol structural units. Examples of this group are kaempferol, kaempferol-3-methyl ether, quercetin, and quercetin-3-methyl ether among others. The flavonols present important health benefits, such as the anti-oxidant activity, by increasing the activity of catalase and glutathione peroxidase (Bai et al., 2016). They also act as radical scavengers (Tipoe et al., 2007; Bai et al., 2016). In addition, they present anti-inflammatory effects by inhibiting the activity of lipoxygenase and cyclooxygenase (Kim et al., 1998; Marunaka, 2017).
Flavanones
This group is characterized by the lack of a double bond between C-2 and C-3 in the C ring of the flavonoid skeleton. Thus, in these compounds, C-2 bears one hydrogen atom in addition to the phenolic B-ring, and C-3 has two hydrogen atoms (Grayer and Veitch, 2006). Flavanones have anti-oxidant, anti-inflammatory, and neuroprotective activity (Hernández-Aquino et al., 2017).
Flavan-3-ols (Flavanols)
The flavanols or catechins, also referred to as flavan-3-ols, are non-glycosylated compounds that are present in plants in the form of monomers (catechins). The hydroxyl group is bound to position 3 of the C ring, and has no double bonds between positions 2 and 3. Another important characteristic is the high nucleophilicity of their A-rings to HO− and RO− (Andersen and Markham, 2006; Panche et al., 2016). These compounds have anti-oxidant, anti-inflammatory and anti-microbial properties. The most studied flavan-3-ol monomers are (+)-catechins, (–)-epicatechin, (–)-epigallocatechin, (–)-epicatechin gallate, (–)-epigallocatechin gallate, and (+)-gallocatechin (Panche et al., 2016; Borges et al., 2017; Kuhnle, 2017).
Anthocyanidins
Anthocyanidins have an -OH group in position 3 but also have a double bound between carbons 3 and 4 of ring C. This group is the main flavonoid responsible for cyanic colors (red, purple, and blue) in vegetables, flowers and fruits. The most common examples are cyanidin, delphinidin, pelargonidin, malvidin, and peonidin (Andersen and Markham, 2006; Azzini et al., 2017). They also have anti-oxidant and anti-microbial effects (Middleton et al., 2000; Pietta, 2000; Azzini et al., 2017; Khoo et al., 2017).
Chalcones
The chalcones are referred to as open-chain flavonoids. Chalcones are the yellow to orange flower pigments of some plants (Andersen and Markham, 2006; Panche et al., 2016). The A and B rings of chalcones are linked by a three-carbon chain instead of a C ring, which is absent (Veitch and Grayer, 2006). Chalcones have nutritional and biological benefits owing to their anti-bacterial and anti-parasitic activities (Nowakowska, 2007; Costa et al., 2016).
Isoflavonoids
The isoflavones are commonly known as ß-glucosides and have a B ring in position 3. These compounds have potent anti-oxidant activity. Genistein and daidzein are the main two soy isoflavones, whose main effects are the inhibition of lipid peroxidation (Lapcík et al., 1998; Yu et al., 2016). Genistein and daidzein present anti-oxidant activity in peripheral blood lymphocytes, increasing DNA protection against oxidative damage, which contributes to homeostasis in humans (Takahashi et al., 2009).
Flavonoids and Anti-Oxidant Properties
The imbalance between the generation of ROS and reactive nitrogen species (RNS) and their elimination is classically described as “oxidative stress,” which plays an important role in the pathophysiology of many diseases because ROS and RNS can react with lipids, proteins and DNA, inducing their oxidation and causing cell damage. The human body has several anti-oxidant systems, where anti-oxidants are understood as “any substance that retards, prevents or removes oxidative damage to target molecules” (Halliwell and Gutteridge, 2015). The most important anti-oxidant enzymes in mammalian cells are superoxide dismutase (SOD), catalase (CAT), and glutathione peroxidase (GPx) (Valko et al., 2007; Deponte, 2013; Losada-Barreiro and Bravo-Díaz, 2017). Non-enzymatic mechanisms include iron-binding proteins such as transferrin and ferritin, melatonin, and uric acid (Othman et al., 2008; Pizzino et al., 2017). These endogenous anti-oxidant systems are complementary and usually sufficient to prevent oxidative damage to the cells, but in certain conditions, such as when ROS production is excessive, the intake of exogenous anti-oxidants is convenient to reduce damage. These anti-oxidants derived from the diet are found in all vegetables and include phenols, phenolic acids, tannins, lignans, and flavonoids (Pizzino et al., 2017). The human diet contains between 50 mg and 800 mg of flavonoids per day, depending on the consumption of fruits and vegetables.
In general, the anti-oxidant activity of a flavonoid depends on three of its structural characteristics: (a) the presence of the catechol group in the B ring, (b) a Δ2 double bond and a 4-oxo group in the C ring, and (c) the hydroxyl groups on positions C-3 and C-5 (Wolfe and Liu, 2008; Kumar et al., 2013). These activities are modulated by three general mechanisms: (a) scavenging the ROS, (b) suppressing the formation of free radicals by enzymatic inhibition or chelating elements involved in the formation of them, and (c) protecting or upregulating the anti-oxidants. The ROS-scavenging activity is carried out by direct donation of hydrogen atoms, resulting in more stable and less reactive radicals.
Furthermore, some flavonoids can scavenge and others scavenge NO (Hanasaki et al., 1994; Vanacker et al., 1995; Prior and Cao, 2000). Anthocyanidins present the most effective scavenger function, with an activity 10–1,000 times greater than glutathione (GSH) (Cao et al., 1997). The activity of scavenging and NO prevents the formation of ONOO−, which is highly oxidizing. Moreover, flavonoids are direct chelators of ONOO−, as was reported for quercetin (Haenen et al., 1997; Heijnen et al., 2001; Spencer et al., 2003).
However, flavonoids that chelate Fe2+ or Cu+ can remove a causal factor in the formation of free radicals (e.g., quercetin) (de Groot and Rauen, 1998). These natural compounds stimulate the induction of anti-oxidant enzymes, such as glutathione-S transferase, UDP-glucuronosyl transferase, and NADH-quinone oxidoreductase, which are the main defense against toxic electrophilic and oxidant stress (Procházková et al., 2011). Other enzymes inhibited by flavonoids are those involved in the metabolism of arachidonic acid, such as lipoxygenase, cyclooxygenase, microsomal succinoxidase, and NADH oxidase (de Groot and Rauen, 1998). The anti-oxidant function of flavonoids includes the reduction of α-tocopherol, which represents the main anti-oxidant of membranes and low-density lipoproteins (LDL). Flavonoids can donate a hydrogen to α-tocopherol radicals and thus protect from oxidation to LDL (Hirano et al., 2001), as observed for quercetin and catechins (Zhu et al., 2000).
Although the anti-oxidant role of flavonoids is well-documented, many studies have reported their pro-oxidant activity, which seems to depend on their concentration and is directly proportional to the total number of OH groups in the molecule, especially those located in the B ring (Perron et al., 2011).
Oxidative and Anti-Oxidative Microenvironment in Amebiasis
During ALA, E. histolytica is capable of inducing an important inflammatory response, which is mainly composed of neutrophils (PMNs) and macrophages. These cells create an oxidative stress environment. In the initial stages of ALA, the amebas are surrounded by neutrophils and posteriorly by macrophages. Chronic inflammation allows the formation of a granulomatous reaction. This exacerbated response can lead to extensive areas of necrosis (Tsutsumi et al., 1984). In this milieu, E. histolytica interacts with oxidative and non-oxidative metabolites produced by the inflammatory cells. In experimental ALA, NO concentration increases in a time dependent manner, which was found in serum samples from hamsters at different stages of the infection. In this study, the authors used histochemistry to determine the presence of the NADPH enzyme that correlated with the size and severity of the lesion. With these findings, they concluded that during the establishment of ALA, E. histolytica trophozoites resisted the increment of NO produced by inflammatory cells; therefore, this molecule is not sufficient to eliminate the parasite in in vivo studies (Pacheco-Yépez et al., 2001). The presence of NO was also evaluated in intestinal amebiasis; in these reports, a significant increase in the NO levels in patients with diarrhea was found compared with the control group. These results support that this oxidative molecule possesses a central role in the pathophysiological mechanisms underlying amebiasis (Pérez-Fuentes et al., 2000; Namiduru et al., 2011).
The relevance of NO is also related with its capacity to react with other molecules that are present in this oxidative environment, such as the superoxide anion (), which leads to the production of peroxynitrite (ONOO−), which has been described as one of the most potent oxidants produced in a biological system. To date, there are no reports related with peroxynitrites in amebiasis. It is necessary to evaluate the role of this molecule in the pathogenesis of amebiasis (Pacheco-Yepez et al., 2014).
In this oxidative milieu, the production of depends of the activity of the NADPH-oxidase, besides the SOD enzyme converts the superoxide anion to H2O2, which activates the myeloperoxidase system (MPO). This enzyme catalyzed the production of hypochlorous acid (HOCl), a highly oxidant molecule, as cytotoxic effector synthetized mainly by neutrophils. The anti-amebic activity of the MPO was described in in vivo experiments. The MPO caused important damage in trophozoites. These results showed that this enzyme, which was produced by inflammatory cells, is capable of protecting host tissue against E. histolytica (Pacheco-Yépez et al., 2011).
As was previously mentioned, trophozoites are exposed to oxidative stress (ROS and RNS such as , H2O2, ONOO−, and NO). In these conditions, the ameba displays endogenous anti-oxidant enzymes to avoid the effect of these toxic molecules; therefore, E. histolytica can survival in this environment. A trypanothione reductase in E. histolytica was identified (Ondarza et al., 2005; Tamayo et al., 2005). This enzyme possesses anti-oxidant properties similar to glutathione and thioredoxin enzymatic systems (Krauth-Siegel and Comini, 2008; Krauth-Siegel and Leroux, 2012).
A 29 kDa thiol-dependent peroxiredoxin protein (Eh2CysPrx or Eh29) has been reported. The authors demonstrated that the recombinant protein presented thiol peroxidase activity due to its ability to remove H2O2. In addition, they identified the protein in the ameba cytoplasm with a molecular weight of 29 kDa (Bruchhaus et al., 1997). In contrast, other research groups reported that peroxiredoxin was localized in the membrane, and the authors demonstrated that E. histolytica presented a larger amount of this enzyme compared with E. dispar. These results showed that peroxiredoxin can be a virulence factor of E. histolytica, protecting trophozoites from oxidative stress (Choi et al., 2005). When the amebas were exposed for 1 h to high oxygen concentrations, the expression of eh29 was increased 2.1-fold. The authors concluded that the enzyme is involved in the detoxification of peroxides and peroxynitrites (Akbar et al., 2004; Sen et al., 2007).
More recently, the thioredoxin system (EhTRXR/TRX), a group of anti-oxidant enzymes, has been described in E. histolytica. The recombinant protein is able to catalyze the reduction of NADPH or NADH and S-nitrosothiols. This enzyme exhibited NADPH dependent oxidase activity, which generates H2O2 from O2. This protein represents an important mechanism to regulate intracellular and extracellular levels of oxidative molecules (Arias et al., 2012). It is important to mention that these anti-oxidant systems maintain a redox balance in the parasite, allowing their survival in adverse oxidative conditions (Arias et al., 2007, 2008).
Based on these findings, we strongly suggest that flavonoids could participate as natural anti-oxidant compounds in the highly oxidative environment during ALA, favoring the resolution of liver tissue damage caused not only by the presence of the ameba and its secretion products but also by the inflammatory response characteristic of this pathology. In addition, it is important to consider the role of these natural molecules that could act directly on trophozoites. Further studies are necessary to determine the effect of flavonoids in in vivo models to identify their possible molecular targets in amebiasis (Figure 5).
Flavonoids and Their Molecular Targets in Protozoa
Flavonoids and their therapeutic applications in human health have been extensively investigated in recent years due to their use in traditional medicine. Interestingly, these studies demonstrated a possible correlation between the chemical structure of the flavonoid and the molecular target in different cell lines (Panche et al., 2016). However, the mechanisms of action and the multiple effects of these natural compounds on the cells are not fully understood.
The anti-protozoa effect of flavonoids and some of their molecular targets have been demonstrated in Plasmodium falciparum, Trypanosoma brucei brucei, T. brucei gambiense, T. cruzi, Leishmania donovani, Cryptosporidium parvum, Toxoplasma gondii, and Giardia lamblia. In P. falciparum the catechins acts against some identified molecular targets that include enzymes involved in the biosynthesis of fatty acids (FabG, FabZ, FabI, and enoyl-ACP reductase) (Sharma et al., 2007). In Trypanosoma cruzi, (–)-epicatechin has been described to affect the arginine kinase activity and NADH-oxidase activity (Paveto et al., 2004; Maya et al., 2007; Scotti et al., 2010; Dodson et al., 2011). In Leishmania donovani, it was reported that kaempferol promotes the inhibition of the activity of pyruvate kinase, the dihydroorotase enzyme (LdDHOase) and the cytidine deaminase, which impact the pyrimidine biosynthesis pathway, causing the death of the parasites (Scotti et al., 2015; Tiwari et al., 2016). In the case of Toxoplasma gondii, quercetin inhibits the synthesis of HSP90, HSP70, and HSP27, that have been described as virulence factors (Dobbin et al., 2002; Kerboeuf et al., 2008). These alterations promote reductions in the invasion to the host tissues, adhesion, proliferation and cell differentiation (Calzada et al., 1998; Mamani-Matsuda et al., 2004; Mead and McNair, 2006; Kerboeuf et al., 2008; Sen et al., 2008; Dodson et al., 2011; Jin et al., 2014; Cornelio et al., 2017) (Figure 2).
Flavonoids With Anti-Amebic Activity
The diarrheal infections caused by E. histolytica represent a great problem in developing countries. They are responsible for a considerable number of morbidities and mortalities in these populations. The development of novel and effective anti-amebic compounds without side effects is necessary. Since the 1990s, medicinal plants have gained popularity as potential therapeutic alternatives. Because of this, in the last three decades, natural drugs and their products have represented approximately 50% of all treatments that have come to market (Newman and Cragg, 2016), and flavonoids display significant anti-amebic activity in in vitro studies (Figure 3 and Table 3).
In recent years, flavonoids have generated great interest in the scientific community. However, there are few studies concerning their molecular mechanisms against E. histolytica. The most studied are kaempferol, (–)-epicatechin and tiliroside. In these studies, it has been observed that the main molecular targets correspond to cytoskeleton related proteins (myosin II heavy chain, α-actinin, and actin). The authors also demonstrated a dysregulation of glycolytic enzymes and stress oxidative proteins. They concluded that all these changes modify the pathogenic mechanism, such as adhesion, cytolysis, phagocytosis, and migration (Bolaños et al., 2014, 2015; Calzada et al., 2017a) (Figure 4 and Table 4). Below, we highlight the therapeutic effectiveness of the main flavonoids with anti-amebic activity and explain in more detail the molecular targets that could probably be affected in the ameba.
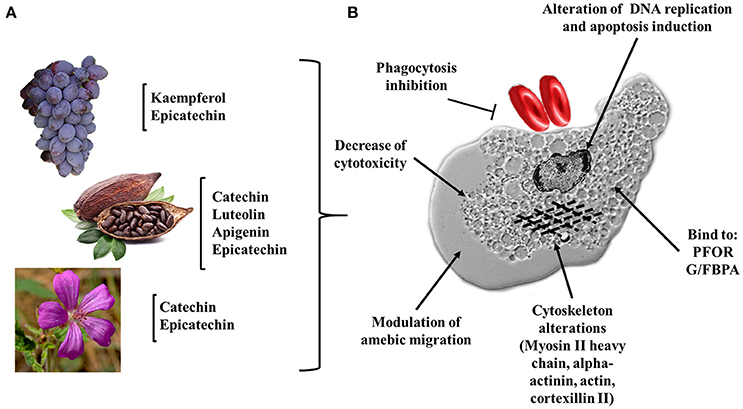
Figure 4. Flavonoids and their possible targets on E. histolytica. (A) Natural source of flavonoids with anti-amebic activity, fruits (grapes), seeds (cacao), and flowers (Geranium mexicanum). (B) Potential cellular targets in the ameba. Alteration of DNA replication and apoptosis induction; dysregulation of cytoplasmic proteins: HSP70, PFOR, G/FBPA, and GAPDH; inhibition of cytotoxicity, phagocytosis and alteration of the trophozoite migration (myosin II heavy chain, alpha-actinin, actin, and cortexillin II).
In vitro Studies and Molecular Targets
Catechin
Catechin has been isolated from plants that are used in traditional medicine for the treatment of gastrointestinal disorders. Plants with anti-amebic activity that have been used as sources of catechins and their derivatives include Helianthemum glomeratum, Osyris alba, Chiranthodendron pentadactylon, Geranium niveum, Geranium mexicanum, and Rubus coriifolius (Meckes et al., 1999; Alanis et al., 2003; Al-Jaber et al., 2010; Calzada et al., 2017c).
The (–)-epicatechin isoform in in vitro assays has shown the best IC50 value of 1.9 μg/ml in inhibiting amebic growth compared with that of other catechin derivatives [e.g., (–)-epigallocatechin, (+)-catechin-3-O-α-L-rhamnopyranoside, and geranins A, B, C, and D] (Meckes et al., 1999; Calzada et al., 2001). The differences in the anti-amebic activity of the epicatechin derivatives can be related with the presence of hydroxy groups in the phenolic ring and galloyl moieties [e.g., (–)-epicatechin and (–)-epigallocatechin]. In addition, the presence of hydroxy groups in position 3, 4, and 5 in the B ring enhances the anti-oxidant and scavenging activities (Mizushina et al., 2005; Braicu et al., 2011) (Figure 5). The (–)-epicatechin induced morphological changes in the trophozoites at the nuclear and cytoplasmic levels, causing programmed cell death in approximately 95% of amebas (Soto et al., 2010). Moreover, (–)-epicatechin caused alterations of cytoskeleton proteins from E. histolytica (myosin II heavy chain, actin and alpha-actinin), affecting adhesion, cytolysis, migration, and phagocytosis (Table 4). In addition, (–)-epicatechin caused the dysregulation of enzymes that are involved in energy metabolism, such as glyceraldehyde-phosphate dehydrogenase and fructose-1,6-bisphosphate aldolase (G/FBPA). The (–)-epicatechin concentration used did not promote cytotoxicity in intestinal mammalian cells (Caco-2) (Bolaños et al., 2014). Therefore, (–)-epicatechin can be considered the most promising alternative, safe flavonoid for treatment against amebiasis. It is necessary to evaluate in more detail the molecular mechanisms involved in the specific targets and their impact on the virulence of E. histolytica (Figures 4, 5).
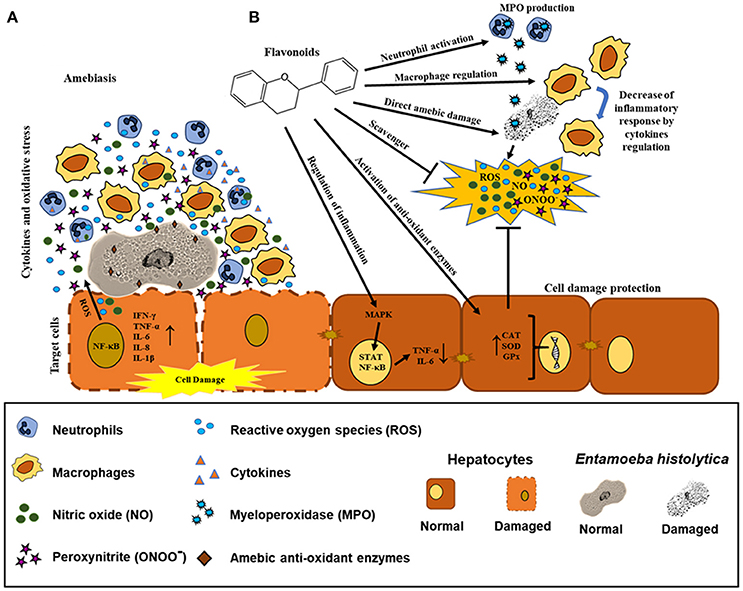
Figure 5. Possible effects of flavonoids in the regulation of biochemical and immunological responses against amebiasis. (A) Recruitment of neutrophils and macrophages by E. histolytica promoting the synthesis and production of pro-inflammatory cytokines and oxidative mediators. E. histolytica presents detoxifying enzymes. (B) Possible mechanisms of the flavonoids as direct scavenger of ROS, ONOO−, and NO, enhancer of CAT, SOD, and GPx enzymes and regulation of inflammation via STAT and NF-κB. Effect of the flavonoids in neutrophils (MPO production), macrophages (decrease of inflammatory mediators), and E. histolytica trophozoites (direct damage in different molecular targets).
Kaempferol
One of the first studies of the anti-amebic activity of kaempferol showed that the purified compound from the aerial parts of Cuphea pinetorum had an inhibitory effect on amebic culture proliferation. This work reported an IC50 value near 7.9 μg/ml (Calzada, 2005). Two later studies determined similar IC50 values against E. histolytica. The specific anti-amebic activity of kaempferol is independent of the plant species [Cnidoscolus chayamansa (Mc Vaugh) and Annona cherimola Miller] (Calzada et al., 2017b; Pérez-González et al., 2017). Some of the molecular mechanisms triggered by kaempferol in amebas include the dysregulation of actin, myosin II heavy chain, cortexillin II, heat shock protein 70, glyceraldehyde-phosphate dehydrogenase and G/FBPA. These results are similar to the results for (–)-epicatechin (Bolaños et al., 2014, 2015). The authors showed that at 27.7 μM, kaempferol inhibited 77.1% of ameba growth in comparison with trophozoites untreated with this flavonoid. In addition, they reported the inhibition of adhesion to Caco-2 cells in amebas co-incubated with kaempferol. (Bolaños et al., 2014, 2015; Table 4).
Kaempferol has a high affinity for pyruvate ferredoxin oxidoreductase (PFOR), an important therapeutic target in E. histolytica (Samarawickrema et al., 1997; Jeelani and Nozaki, 2016). By docking analysis, the authors found that the molecular interaction of kaempferol with the amebic PFOR included nine amino acid residues (Phe 665, Pro 666, Leu 667, Gly 845, Ala 846, Met 851, Tyr 853, Trp 864, and Asn, 866) compared with the four different binding sites of MTZ (Phe 174, His 178, Lys 435, Phe 453, and Tyr 455) (Calzada et al., 2017b).
Kaempferol derivatives also exhibit considerable anti-amebic activity. In particular, tiliroside showed an IC50 of 7.45 μg/ml against E. histolytica trophozoites. In a similar manner to kaempferol, tiliroside can interact directly with PFOR and G/FBPA according to docking studies, showing an inhibition constant (KI) of 53.57 and 55.5 μM, respectively. These data are comparable with the values of the KI for MTZ (47.64 and 44.01 μM, respectively) (Calzada and Alanis, 2007; Calzada et al., 2017a) (Figure 4 and Table 4). It is important to remark that the differences in IC values and the molecular mechanisms of action between kaempferol and its derivatives may be due to the presence of glucosyl moieties on position C3 of the principal structure or the number of hydroxyl groups in ring B, which probably limits the correct activity of tiliroside (Cimanga et al., 2006; Singh et al., 2009) (Table 3).
Based on previous reports, kaempferol, (–)-epicatechin, and (–)-epigallocatechin present different IC50 for anti-amebic activity and presented the best IC50 values that showed good activity against E. histolytica. These natural compounds reduced the amebic viability by 50% (1.9, 6.89, and 7.93 μg/ml respectively). Due to the low IC50, these flavonoids could be considered good candidates to evaluate their in vivo activity. Other important advantages of the use of flavonoids are their anti-oxidant and anti-inflammatory activity, which could participate in the resolution of amebiasis.
Quercetin
This flavonoid showed a slight anti-amebic effect in vitro (IC50 114.3 μg/ml) compared with that of G. lamblia (IC50 26.6 μg/ml). However, isoquercitin, a quercetin derivative, inhibited the viability and growth of E. histolytica trophozoites after 48 h of incubation (IC50 14.7 μg/ml) (Calzada and Alanis, 2007). Although there are no reports of a specific target of isoquercetin in the ameba, it is imperative to mention that this flavonoid is metabolized in the bowel and the liver (Valentova et al., 2014); therefore, its administration in in vivo models could diminish the tissue damage induced by E. histolytica.
Other Polyphenol Compounds With Anti-amebic Effects
Other flavonoids that have anti-amebic activity are luteolin and apigenin. These compounds, isolated from the total extract of Morinda morindoides, have shown IC50 values of 17.8 and 10 μg/ml, respectively, against the ameba. The authors suggested that glycosylation on the C-7 position decreased the anti-amebic activity of these flavonoids (Cimanga et al., 2006) (Table 3). Nevertheless, it is important to mention that the anti-amebic mechanisms of these flavonoids and their real potential activities have not been determined. Other compounds with anti-amebic effects are the chalcones. Leeza Zaidi et al. (2015) synthetized modified chalcones (N-substituted ethanamine). In vitro assays showed that their derivatives displayed a better anti-amebic activity than the MTZ reference drug (Leeza Zaidi et al., 2015).
In vivo Studies
The flavonoids have shown extensive benefits and no side effects in in vivo models of different types of cancer, liver cirrhosis, neurodegenerative diseases and metabolic disorders (e.g., obesity and diabetes) (Goto et al., 2012; Nabavi et al., 2015; Panche et al., 2016; Hernández-Aquino et al., 2017).
In contrast, there are few reports of flavonoids in parasitic diseases using in vivo models. The efficacy of intragastric treatment with (–)-epicatechin at 0.072 μmol/kg was demonstrated in CD-1 mice infected with G. lamblia trophozoites (1 × 106). The (–)-epicatechin administered 6 days post-infection decreased the number of parasites in the small intestine. Moreover, this natural compound displayed a higher activity than that of MTZ and emetine (Barbosa et al., 2007). The epigallocatechin was effective in BALB/c mice infected with Leishmania amazonensis. The results showed that the animals treated orally with 30 mg/kg/day of epigallocatechin for 5 days presented a reduction in the size of the ear lesion and a low parasite burden compared with that of the control group (Inacio et al., 2013).
It is important to mention that, until now, there have been no studies of amebiasis using flavonoids in in vivo models. Nevertheless, a polyphenol, resveratrol, has presented anti-amebic properties both in vitro and in vivo. In vitro incubation with resveratrol (72 μM) for 48 h induced cell growth arrest, production of ROS, damage to membrane lipids, increased intracellular Ca2+, calpain activation, and decreased superoxide dismutase activity, leading to apoptosis in E. histolytica. Moreover, in hamsters with ALA, the pre-treatment for 2 or 10 days with 30 mg/kg of resveratrol by oral gavage prevented liver damage by the trophozoites, whereas non-pretreated animals developed extensive ALA (80% of the total liver). Histopathological analysis showed that resveratrol-treated hamsters presented a healthy liver parenchyma with retraction of the granulomatous reaction, whereas the untreated animals displayed liver necrosis. Based on these results, the authors suggested that resveratrol could be used as alternative treatments for amebiasis (Pais-Morales et al., 2016). Although resveratrol is a polyphenol, it is necessary to perform in vivo studies to demonstrate the potential anti-amebic effect of flavonoids before their use as an alternative or complementary treatment.
Closing Remarks
In recent years, the use of natural compounds against infectious diseases caused by protozoan parasites has gained popularity among pharmaceutical corporations (Newman and Cragg, 2016). In 2015, the Nobel Prize in Medicine was awarded to Professor Youyou Tu for her valuable contributions to the discovery of artemisinin as a natural malaria treatment (Su and Miller, 2015). These advances have been a consequence of the various reports on drug resistance in microorganisms as well as the toxicity and side effects of many drugs on humans. The field of amebiasis is no exception. Promising advances have been made using different secondary metabolites from plant extracts (Procházková et al., 2011; Herrera-Martínez et al., 2016; Bashyal et al., 2017). The use of plants rich in flavonoids (grapes, cacao and flowers such as Geranium mexicanum) have been shown to exert anti-amebic activity, having cytoskeletal proteins and enzymes related to the glycolytic metabolism of E. histolytica as molecular targets and leading to alterations in DNA replication. These changes suggest that the ameba lost their virulence factors (Bolaños et al., 2014, 2015) (Figure 4). It is well known that during the establishment of amebiasis in the intestine and in the liver, the inflammatory reaction can promote the participation of pro-inflammatory cytokines. In this milieu, there are a highly oxidative stresses, constituted by NO, peroxynitrites (ONOO−), ROS, and hypochlorous acid. All these oxidant metabolites caused tissue damage. Flavonoids can regulate inflammation-activating anti-oxidants enzymes (CAT, SOD, and GPx). Additionally, flavonoids participate as scavengers that can remove these free radicals. We cannot discard that flavonoids act directly with neutrophils and macrophages to kill amebas (Figures 5A,B).
Considering all the benefits and probable therapeutic targets of flavonoids in the treatment of E. histolytica infection, it is important to investigate these natural compounds in basic research studies that will establish the doses, administration routes, bioavailability, and metabolic biotransformation (glycosidation, glucuronidation, sulfation, and O-methylation) as well as effects on the tissue microenvironment of these natural compounds in in vivo models of amebiasis. Considering all of these remarks, flavonoids can be considered good alternatives for the effective treatment of amebiasis.
Author Contributions
Authors that contributed to writing the manuscript: MS (introduction and closing remarks), JP-Y and RC-R (flavonoids structure and classification), RJ-L (flavonoids and anti-oxidant properties), NF-H and LC-J (flavonoids and their molecular targets in protozoa), MM-C and PG-T (flavonoids with anti-amebic activity; in vitro and in vivo studies and molecular targets). MS organize and revise the manuscript. All the authors prepared and edited the figures and tables. All authors contributed to manuscript revision, read and approved the submitted version.
Funding
This work was supported by CONACyT grant number 237523 assigned to MS.
Conflict of Interest Statement
The authors declare that the research was conducted in the absence of any commercial or financial relationships that could be construed as a potential conflict of interest.
Acknowledgments
We would like to thank MSc Daniel Coronado-Velázquez for his valuable assistance in the performance of the schematic representations (Figures 4, 5). English grammar editing was done by the American Journal Experts (https://www.aje.com/).
References
Agarwal, A., Kanekar, S., Sabat, S., and Thamburaj, K. (2016). Metronidazole-induced cerebellar toxicity. Neurol. Int. 8:6365. doi: 10.4081/ni.2016.6365
Akbar, M. A., Chatterjee, N. S., Sen, P., Debnath, A., Pal, A., Bera, T., et al. (2004). Genes induced by a high-oxygen environment in Entamoeba histolytica. Mol. Biochem. Parasitol. 133, 187–196. doi: 10.1016/j.molbiopara.2003.10.006
Alanis, A. D., Calzada, F., Cedillo-Rivera, R., and Meckes, M. (2003). Antiprotozoal activity of the constituents of Rubus coriifolius. Phytother. Res. 17, 681–682. doi: 10.1002/ptr.1150
Al-Jaber, H. I., Mosleh, I. M., Mallouh, A., Abu Salim, O. M., and Abu Zarga, M. H. (2010). Chemical constituents of Osyris alba and their antiparasitic activities. J. Asian Nat. Prod. Res. 12, 814–820. doi: 10.1080/10286020.2010.502892
Andersen, Ø. M., and Markham, K. R. (2006). Flavonoids: Chemistry, Biochemistry and Applications. Boca Raton, FL; London; New York, NY: CRC press; Taylor & Francis.
Ansari, M. F., Siddiqui, S. M., Agarwal, S. M., Vikramdeo, K. S., Mondal, N., and Azam, A. (2015). Metronidazole hydrazone conjugates: design, synthesis, antiamoebic and molecular docking studies. Bioorg. Med. Chem. Lett. 25, 3545–3549. doi: 10.1016/j.bmcl.2015.06.091
Arias, D. G., Carranza, P. G., Lujan, H. D., Iglesias, A. A., and Guerrero, S. A. (2008). Immunolocalization and enzymatic functional characterization of the thioredoxin system in Entamoeba histolytica. Free Radic. Biol. Med. 45, 32–39. doi: 10.1016/j.freeradbiomed.2008.03.008
Arias, D. G., Gutíerrez, C. E., Iglesias, A. A., and Guerrero, S. A. (2007). Thioredoxin-linked metabolism in Entamoeba histolytica. Free Radic. Biol. Med. 42, 1496–1505. doi: 10.1016/j.freeradbiomed.2007.02.012
Arias, D. G., Regner, E. L., Iglesias, A. A., and Guerrero, S. A. (2012). Entamoeba histolytica thioredoxin reductase: molecular and functional characterization of its atypical properties. Biochim. Biophys. Acta 1820, 1859–1866. doi: 10.1016/j.bbagen.2012.08.020
Azzini, E., Giacometti, J., and Russo, G. L. (2017). Antiobesity effects of anthocyanins in preclinical and clinical studies. Oxid. Med. Cell. Longev. 2017:2740364. doi: 10.1155/2017/2740364
Bai, Y., Xia, B., Xie, W., Zhou, Y., Xie, J., Li, H., et al. (2016). Phytochemistry and pharmacological activities of the genus Prunella. Food Chem. 204, 483–496. doi: 10.1016/j.foodchem.2016.02.047
Barbosa, E., Calzada, F., and Campos, R. (2007). In vivo antigiardial activity of three flavonoids isolated of some medicinal plants used in Mexican traditional medicine for the treatment of diarrhea. J. Ethnopharmacol. 109, 552–554. doi: 10.1016/j.jep.2006.09.009
Bashyal, B., Li, L., Bains, T., Debnath, A., and LaBarbera, D. V. (2017). Larrea tridentata: a novel source for anti-parasitic agents active against Entamoeba histolytica, Giardia lamblia and Naegleria fowleri. PLoS Negl. Trop. Dis. 11:e0005832. doi: 10.1371/journal.pntd.0005832
Boechat, N., Carvalho, A. S., Salomão, K., Castro, S. L., Araujo-Lima, C. F., Mello, F. V., et al. (2015). Studies of genotoxicity and mutagenicity of nitroimidazoles: demystifying this critical relationship with the nitro group. Mem. Inst. Oswaldo Cruz 110, 492–499. doi: 10.1590/0074-02760140248
Bolaños, V., Díaz-Martínez, A., Soto, J., Marchat, L. A., Sánchez-Monroy, V., and Ramírez-Moreno, E. (2015). Kaempferol inhibits Entamoeba histolytica growth by altering cytoskeletal functions. Mol. Biochem. Parasitol. 204, 16–25. doi: 10.1016/j.molbiopara.2015.11.004
Bolaños, V., Díaz-Martínez, A., Soto, J., Rodríguez, M. A., López-Camarillo, C., Marchat, L. A., et al. (2014). The flavonoid (-)-epicatechin affects cytoskeleton proteins and functions in Entamoeba histolytica. J. Proteomics 111, 74–85. doi: 10.1016/j.jprot.2014.05.017
Borges, G., Ottaviani, J. I., van der Hooft, J. J. J., Schroeter, H., and Crozier, A. (2017). Absorption, metabolism, distribution and excretion of (–)-epicatechin: a review of recent findings. Mol. Aspects Med. doi: 10.1016/j.mam.2017.11.002. [Epub ahead of print].
Bors, W., Heller, W., Michel, C., and Saran, M. (1990). Flavonoids as antioxidants - determination of radical-scavenging efficiencies. Meth. Enzymol. 186, 343–355. doi: 10.1016/0076-6879(90)86128-I
Braicu, C., Pilecki, V., Balacescu, O., Irimie, A., and Neagoe, I. B. (2011). The relationships between biological activities and structure of flavan-3-ols. Int. J. Mol. Sci. 12, 9342–9353. doi: 10.3390/ijms12129342
Bruchhaus, I., Richter, S., and Tannich, E. (1997). Removal of hydrogen peroxide by the 29 kDa protein of Entamoeba histolytica. Biochem. J. 326, 785–789. doi: 10.1042/bj3260785
Calleja Bello, M., and Colin Abarranco, M. (1979). Amebic liver abscess. treatment with metronidazole by the intravenous route. Prensa Med. Mex. 44, 112–114.
Calzada, F. (2005). Additional antiprotozoal constituents from Cuphea pinetorum, a plant used in Mayan traditional medicine to treat diarrhoea. Phytother. Res. 19, 725–727. doi: 10.1002/ptr.1717
Calzada, F., Alanis, A. D., Meckes, M., Tapia-Contreras, A., and Cedillo-Rivera, R. (1998). In vitro susceptibility of Entamoeba histolytica and Giardia lamblia to some medicinal plants used by the people of Southern Mexico. Phytother. Res. 12, 70–72. doi: 10.1002/(SICI)1099-1573(19980201)12:1<70::AID-PTR189>3.0.CO;2-V
Calzada, F., and Alanís, A. D. (2007). Additional antiprotozoal flavonol glycosides of the aerial parts of Helianthemum glomeratum. Phytother. Res. 21, 78–80. doi: 10.1002/ptr.2031
Calzada, F., Basurto, J. C., Barbosa, E., Velázquez, C., Hernández, N. G., Ordoñez Razo, R. M., et al. (2017a). Antiprotozoal activities of tiliroside and other compounds from Sphaeralcea angustifolia (Cav.) G. Don. Pharmacognosy Res. 9, 133–137. doi: 10.4103/0974-8490.204644
Calzada, F., Cedillo-Rivera, R., Bye, R., and Mata, R. (2001). Geranins C and D additional new antiprotozoal A-type proanthocyanidins from Geranium niveum. Planta Med. 67, 677–680. doi: 10.1055/s-2001-17358
Calzada, F., Cervantes-Martínez, J. A., and Yépez-Mulia, L. (2005). In vitro antiprotozoal activity from the roots of Geranium mexicanum and its constituents on Entamoeba histolytica and Giardia lamblia. J. Ethnopharmacol. 98, 191–193. doi: 10.1016/j.jep.2005.01.019
Calzada, F., Correa-Basurto, J., Barbosa, E., Méndez-Luna, D., and Yepez-Mulia, L. (2017b). Antiprotozoal constituents from Annona cherimola miller, a plant used in mexican traditional medicine for the treatment of diarrhea and dysentery. Pharmacogn. Mag. 13, 148–152. doi: 10.4103/0973-1296.197636
Calzada, F., Juárez, T., García-Hernández, N., Valdes, M., Ávila, O., Mulia, L. Y., et al. (2017c). Antiprotozoal, Antibacterial and antidiarrheal properties from the flowers of Chiranthodendron pentadactylon and isolated flavonoids. Pharmacogn. Mag. 13, 240–244. doi: 10.4103/0973-1296.204564
Calzada, F., Meckes, M., and Cedillo-Rivera, R. (1999). Antiamoebic and antigiardial activity of plant flavonoids. Planta Med. 65, 78–80. doi: 10.1055/s-2006-960445
Cao, G., Sofic, E., and Prior, R. L. (1997). Antioxidant and prooxidant behavior of flavonoids: structure-activity relationships. Free Radic. Biol. Med. 22, 749–760. doi: 10.1016/S0891-5849(96)00351-6
Choi, M. H., Sajed, D., Poole, L., Hirata, K., Herdman, S., Torian, B. E., et al. (2005). An unusual surface peroxiredoxin protects invasive Entamoeba histolytica from oxidant attack. Mol. Biochem. Parasitol. 143, 80–89. doi: 10.1016/j.molbiopara.2005.04.014
Cimanga, R. K., Kambu, K., Tona, L., Hermans, N., Apers, S., Totté, J., et al. (2006). Cytotoxicity and in vitro susceptibility of Entamoeba histolytica to Morinda morindoides leaf extracts and its isolated constituents. J. Ethnopharmacol. 107, 83–90. doi: 10.1016/j.jep.2006.02.010
Cornelio, V. E., Maluf, F. V., Fernandes, J. B., da Silva, M. F. G. F., Oliva, G., Guido, R. V. C., et al. (2017). Isolation of tiliroside from Spiranthera odoratissima as inhibitor of Trypanosoma cruzi glyceraldehyde-3-phosphate dehydrogenase by using bioactivity-guided fractionation. J. Braz. Chem. Soc. 28, 512–519. doi: 10.21577/0103-5053.20160315
Cos, P., Ying, L., Calomme, M., Hu, J. P., Cimanga, K., Van Poel, B., et al. (1998). Structure-activity relationship and classification of flavonoids as inhibitors of xanthine oxidase and superoxide scavengers. J. Nat. Prod. 61, 71–76. doi: 10.1021/np970237h
Costa, G. M., Endo, E. H., Cortez, D. A. G., Nakamura, T. U., Nakamura, C. V., and Dias, B. P. (2016). Antimicrobial effects of Piper hispidum extract, fractions and chalcones against Candida albicans and Staphylococcus aureus. J. Mycol. Med. 26, 217–226. doi: 10.1016/j.mycmed.2016.03.002
de Groot, H., and Rauen, U. (1998). Tissue injury by reactive oxygen species and the protective effects of flavonoids. Fundam. Clin. Pharmacol. 12, 249–255. doi: 10.1111/j.1472-8206.1998.tb00951.x
Deponte, M. (2013). Glutathione catalysis and the reaction mechanisms of glutathione-dependent enzymes. Biochim. Biophys. Acta Gen. Sub. 1830, 3217–3266. doi: 10.1016/j.bbagen.2012.09.018
Dobbin, C. A., Smith, N. C., and Johnson, A. M. (2002). Heat shock protein 70 is a potential virulence factor in murine Toxoplasma infection via immunomodulation of host NF-kappa B and nitric oxide. J. Immunol. 169, 958–965. doi: 10.4049/jimmunol.169.2.958
Dodson, H. C., Lyda, T. A., Chambers, J. W., Morris, M. T., Christensen, K. A., and Morris, J. C. (2011). Quercetin, a fluorescent bioflavanoid, inhibits Trypanosoma brucei hexokinase 1. Exp. Parasitol. 127, 423–428. doi: 10.1016/j.exppara.2010.10.011
Elizondo, G., Gonsebatt, M. E., Salazar, A. M., Lares, I., Santiago, P., Herrera, J., et al. (1996). Genotoxic effects of metronidazole. Mutat. Res. 370, 75–80. doi: 10.1016/0165-1218(96)00022-5
Goto, T., Teraminami, A., Lee, J. Y., Ohyama, K., Funakoshi, K., Kim, Y. I., et al. (2012). Tiliroside, a glycosidic flavonoid, ameliorates obesity-induced metabolic disorders via activation of adiponectin signaling followed by enhancement of fatty acid oxidation in liver and skeletal muscle in obese-diabetic mice. J. Nutr. Biochem. 23, 768–776. doi: 10.1016/j.jnutbio.2011.04.001
Grayer, R. J., and Veitch, N. C. (2006). “Flavonones and dihydroflavonols in flavonoids chemistry,” in Flavonoids: Chemistry, Biochemistry and Applications, ed Ø. M. Andersen (Boca Raton, FL: CRC Press), 918–995.
Haenen, G. R., Paquay, J. B., Korthouwer, R. E., and Bast, A. (1997). Peroxynitrite scavenging by flavonoids. Biochem. Biophys. Res. Commun. 236, 591–593. doi: 10.1006/bbrc.1997.7016
Halliwell, B., and Gutteridge, J. M. C. (2015). Free Radicals in Biology and Medicine. New York, NY: Oxford University Press.
Hanasaki, Y., Ogawa, S., and Fukui, S. (1994). The correlation between active oxygens scavenging and antioxidative effects of flavonoids. Free Radic. Biol. Med. 16, 845–850. doi: 10.1016/0891-5849(94)90202-X
Haque, R., Faruque, A. S., Hahn, P., Lyerly, D. M., and Petri, W. A. Jr. (1997). Entamoeba histolytica and Entamoeba dispar infection in children in Bangladesh. J. Infect. Dis. 175, 734–736. doi: 10.1093/infdis/175.3.734
Haque, R., Huston, C. D., Hughes, M., Houpt, E., and Petri, W. A. Jr. (2003). Amebiasis. N. Engl. J. Med. 348, 1565–1573. doi: 10.1056/NEJMra022710
Havsteen, B. (1983). Flavonoids, a class of natural products of high pharmacological potency. Biochem. Pharmacol. 32, 1141–1148. doi: 10.1016/0006-2952(83)90262-9
Heijnen, C. G., Haenen, G. R., van Acker, F., van der Vijgh, W., and Bast, A. (2001). Flavonoids as peroxynitrite scavengers: the role of the hydroxyl groups. Toxicol. In vitro 15, 3–6. doi: 10.1016/S0887-2333(00)00053-9
Hernández-Aquino, E., Zarco, N., Casas-Grajales, S., Ramos-Tovar, E., Flores-Beltrán, R. E., Arauz, J., et al. (2017). Naringenin prevents experimental liver fibrosis by blocking TGFbeta-Smad3 and JNK-Smad3 pathways. World J. Gastroenterol. 23, 4354–4368. doi: 10.3748/wjg.v23.i24.4354
Herrera-Martínez, M., Hernández-Ramírez, V. I., Hernández-Carlos, B., Chávez-Munguía, B., Calderón-Oropeza, M. A., and Talamás-Rohana, P. (2016). Antiamoebic Activity of Adenophyllum aurantium (L.) strother and its effect on the actin cytoskeleton of Entamoeba histolytica. Front. Pharmacol. 7:169. doi: 10.3389/fphar.2016.00169
Hirano, R., Sasamoto, W., Matsumoto, A., Itakura, H., Igarashi, O., and Kondo, K. (2001). Antioxidant ability of various flavonoids against DPPH radicals and LDL oxidation. J. Nutr. Sci. Vitaminol. 47, 357–362. doi: 10.3177/jnsv.47.357
Inacio, J. D., Canto-Cavalheiro, M. M., and Almeida-Amaral, E. E. (2013). In vitro and in vivo effects of (-)-epigallocatechin 3-O-gallate on Leishmania amazonensis. J. Nat. Prod. 76, 1993–1996. doi: 10.1021/np400624d
Jeelani, G., and Nozaki, T. (2016). Entamoeba thiol-based redox metabolism: a potential target for drug development. Mol. Biochem. Parasitol. 206, 39–45. doi: 10.1016/j.molbiopara.2016.01.004
Jiang, N., Doseff, A. I., and Grotewold, E. (2016). Flavones: from biosynthesis to health benefits. Plants 5:E27. doi: 10.3390/plants5020027
Jin, H., Xu, Z., Cui, K., Zhang, T., Lu, W., and Huang, J. (2014). Dietary flavonoids fisetin and myricetin: dual inhibitors of Plasmodium falciparum falcipain-2 and plasmepsin II. Fitoterapia 94, 55–61. doi: 10.1016/j.fitote.2014.01.017
Kerboeuf, D., Riou, M., and Guégnard, F. (2008). Flavonoids and related compounds in parasitic disease control. Mini Rev. Med. Chem. 8, 116–128. doi: 10.2174/138955708783498168
Khoo, H. E., Azlan, A., Tang, S. T., and Lim, S. M. (2017). Anthocyanidins and anthocyanins: colored pigments as food, pharmaceutical ingredients, and the potential health benefits. Food Nutr. Res. 61, 1–21. doi: 10.1080/16546628.2017.1361779
Kim, H. P., Mani, I., Iversen, L., and Ziboh, V. A. (1998). Effects of naturally-occurring flavonoids and biflavonoids on epidermal cyclooxygenase and lipoxygenase from guinea-pigs. Prostaglandins Leukot. Essent. Fatty Acids 58, 17–24. doi: 10.1016/S0952-3278(98)90125-9
Krauth-Siegel, R. L., and Comini, M. A. (2008). Redox control in trypanosomatids, parasitic protozoa with trypanothione-based thiol metabolism. Biochim. Biophys. Acta Gen. Sub. 1780, 1236–1248. doi: 10.1016/j.bbagen.2008.03.006
Krauth-Siegel, R. L., and Leroux, A. E. (2012). Low-molecular-mass antioxidants in parasites. Antioxid. Redox Signal. 17, 583–607. doi: 10.1089/ars.2011.4392
Kuhnle, G. G. C. (2017). Nutrition epidemiology of flavan-3-ols: the known unknowns. Mol Aspects Med. doi: 10.1016/j.mam.2017.10.003. [Epub ahead of print].
Kumar, S., and Pandey, A. K. (2013). Chemistry and biological activities of flavonoids: an overview. Sci. World J. 2013:162750. doi: 10.1155/2013/162750
Kumar, S., Mishra, A., and Pandey, A. K. (2013). Antioxidant mediated protective effect of Parthenium hysterophorus against oxidative damage using in vitro models. BMC Complement Altern Med. 13:120. doi: 10.1186/1472-6882-13-120
Lapcík, O., Hill, M., Hampl, R., Wahala, K., and Adlercreutz, H. (1998). Identification of isoflavonoids in beer. Steroids 63, 14–20. doi: 10.1016/S0039-128X(97)00104-9
Leeza Zaidi, S., Mittal, S., Rajala, M. S., Avecilla, F., Husain, M., and Azam, A. (2015). Synthesis, characterization and antiamoebic activity of chalcones bearing N-substituted ethanamine tail. Eur. J. Med. Chem. 98, 179–189. doi: 10.1016/j.ejmech.2015.05.013
Leitsch, D., Kolarich, D., Wilson, I. B., Altmann, F., and Duchêne, M. (2007). Nitroimidazole action in Entamoeba histolytica: a central role for thioredoxin reductase. PLoS Biol. 5:e211. doi: 10.1371/journal.pbio.0050211
Li, E., and Stanley, S. L. Jr. (1996). Protozoa. Amebiasis. Gastroenterol. Clin. North Am. 25, 471–492. doi: 10.1016/S0889-8553(05)70259-4
Losada-Barreiro, S., and Bravo-Díaz, C. (2017). Free radicals and polyphenols: the redox chemistry of neurodegenerative diseases. Eur. J. Med. Chem. 133, 379–402. doi: 10.1016/j.ejmech.2017.03.061
Mamani-Matsuda, M., Rambert, J., Malvy, D., Lejoly-Boisseau, H., Daulouède, S., Thiolat, D., et al. (2004). Quercetin induces apoptosis of Trypanosoma brucei gambiense and decreases the proinflammatory response of human macrophages. Antimicrob Agents Chemother. 48, 924–929. doi: 10.1128/AAC.48.3.924-929.2004
Marunaka, Y. (2017). Actions of quercetin, a flavonoid, on ion transporters: its physiological roles. Ann. N. Y. Acad. Sci. 1398, 142–151. doi: 10.1111/nyas.13361
Maya, J. D., Cassels, B. K., Iturriaga-Vásquez, P., Ferreira, J., Faúndez, M., Galanti, N., et al. (2007). Mode of action of natural and synthetic drugs against Trypanosoma cruzi and their interaction with the mammalian host. Comp. Biochem. Physiol. Mol. Integr. Physiol. 146, 601–620. doi: 10.1016/j.cbpa.2006.03.004
Mead, J. R., and McNair, N. (2006). Antiparasitic activity of flavonoids and isoflavones against Cryptosporidium parvum and Encephalitozoon intestinalis. FEMS Microbiol. Lett. 259, 153–157. doi: 10.1111/j.1574-6968.2006.00263.x
Meckes, M., Calzada, F., Tapia-Contreras, A., and Cedillo-Rivera, R. (1999). Antiprotozoal properties of Helianthemum glomeratum. Phytother. Res. 13, 102–105.
Middleton, E. Jr., Kandaswami, C., and Theoharides, T. C. (2000). The effects of plant flavonoids on mammalian cells: implications for inflammation, heart disease, and cancer. Pharmacol. Rev. 52, 673–751.
Mizushina, Y., Saito, A., Tanaka, A., Nakajima, N., Kuriyama, I., Takemura, M., et al. (2005). Structural analysis of catechin derivatives as mammalian DNA polymerase inhibitors. Biochem. Biophys. Res. Commun. 333, 101–109. doi: 10.1016/j.bbrc.2005.05.093
Mudry, M. D., Carballo, M., Labal de Vinuesa, M., Gonzalez Cid, M., and Larripa, I. (1994). Mutagenic bioassay of certain pharmacological drugs: III. Metronidazole (MTZ). Mutat. Res. 305, 127–132. doi: 10.1016/0027-5107(94)90230-5
Nabavi, S. F., Braidy, N., Gortzi, O., Sobarzo-Sanchez, E., Daglia, M., Skalicka-Wozniak, K., et al. (2015). Luteolin as an anti-inflammatory and neuroprotective agent: a brief review. Brain Res. Bull. 119, 1–11. doi: 10.1016/j.brainresbull.2015.09.002
Naczk, M., and Shahidi, F. (2004). Extraction and analysis of phenolics in food. J. Chromatogr. A 1054, 95–111. doi: 10.1016/S0021-9673(04)01409-8
Namiduru, E. S., Tarakçioglu, M., Namiduru, M., Kocabaş, R., Erbagci, B., Meram, I., et al. (2011). Increased serum nitric oxide and malondialdehyde levels in patients with acute intestinal amebiasis. Asian Pac. J. Trop. Biomed. 1, 478–481. doi: 10.1016/S2221-1691(11)60104-4
Newman, D. J., and Cragg, G. M. (2016). Natural products as sources of new drugs from 1981 to 2014. J. Nat. Prod. 79, 629–661. doi: 10.1021/acs.jnatprod.5b01055
Nicholas, C., Batra, S., Vargo, M. A., Voss, O. H., Gavrilin, M. A., Wewers, M. D., et al. (2007). Apigenin blocks lipopolysaccharide-induced lethality in vivo and proinflammatory cytokines expression by inactivating NF-kappaB through the suppression of p65 phosphorylation. J. Immunol. 179, 7121–7127. doi: 10.4049/jimmunol.179.10.7121
Nijveldt, R. J., van Nood, E., van Hoorn, D. E., Boelens, P. G., van Norren, K., and van Leeuwen, P. A. (2001). Flavonoids: a review of probable mechanisms of action and potential applications. Am. J. Clin. Nutr. 74, 418–425. doi: 10.1093/ajcn/74.4.418
Nowakowska, Z. (2007). A review of anti-infective and anti-inflammatory chalcones. Eur. J. Med. Chem. 42, 125–137. doi: 10.1016/j.ejmech.2006.09.019
Ondarza, R. N., Hurtado, G., Iturbe, A., Hernández, E., Tamayo, E., and Woolery, M. (2005). Identification of trypanothione from the human pathogen Entamoeba histolytica by mass spectrometry and chemical analysis. Biotechnol. Appl. Biochem. 42, 175–181. doi: 10.1042/BA20050023
Othman, A. I., El-Missiry, M. A., Amer, M. A., and Arafa, M. (2008). Melatonin controls oxidative stress and modulates iron, ferritin, and transferrin levels in adriamycin treated rats. Life Sci. 83, 563–568. doi: 10.1016/j.lfs.2008.08.004
Pacheco-Yépez, J., Campos-Rodríguez, R., Shibayama, M., Ventura-Juárez, J., Serrano-Luna, J., and Tsutsumi, V. (2001). Entamoeba histolytica: production of nitric oxide and in situ activity of NADPH diaphorase in amebic liver abscess of hamsters. Parasitol. Res. 87, 49–56. doi: 10.1007/s004360000287
Pacheco-Yepez, J., Jarillo-Luna, R. A., Gutierrez-Meza, M., Abarca-Rojano, E., Larsen, B. A., and Campos-Rodriguez, R. (2014). Peroxynitrite and peroxiredoxin in the pathogenesis of experimental amebic liver abscess. Biomed Res. Int. 2014:324230. doi: 10.1155/2014/324230
Pacheco-Yépez, J., Rivera-Aguilar, V., Barbosa-Cabrera, E., Rojas Hernández, S., Jarillo-Luna, R. A., and Campos-Rodríguez, R. (2011). Myeloperoxidase binds to and kills Entamoeba histolytica trophozoites. Parasite Immunol. 33, 255–264. doi: 10.1111/j.1365-3024.2010.01275.x
Pais-Morales, J., Betanzos, A., García-Rivera, G., Chávez-Munguía, B., Shibayama, M., and Orozco, E. (2016). Resveratrol induces apoptosis-like death and prevents in vitro and in vivo virulence of Entamoeba histolytica. PLoS ONE 11:e0146287. doi: 10.1371/journal.pone.0146287
Pan, M. H., Lai, C. S., Wang, Y. J., and Ho, C. T. (2006). Acacetin suppressed LPS-induced up-expression of iNOS and COX-2 in murine macrophages and TPA-induced tumor promotion in mice. Biochem. Pharmacol. 72, 1293–1303. doi: 10.1016/j.bcp.2006.07.039
Panche, A. N., Diwan, A. D., and Chandra, S. R. (2016). Flavonoids: an overview. J. Nutr. Sci. 5:e47. doi: 10.1017/jns.2016.41
Paveto, C., Güida, M. C., Esteva, M. I., Martino, V., Coussio, J., Flawiá, M. M., et al. (2004). Anti-Trypanosoma cruzi activity of green tea (Camellia sinensis) catechins. Antimicrob. Agents Chemother. 48, 69–74. doi: 10.1128/AAC.48.1.69-74.2004
Pehrson, P., and Bengtsson, E. (1983). Treatment of non-invasive amoebiasis. A comparison between tinidazole alone and in combination with diloxanide furoate. Trans. R. Soc. Trop. Med. Hyg. 77, 845–846. doi: 10.1016/0035-9203(83)90302-4
Pérez-Fuentes, R., del Carmen Sánchez-Guillén, M., Velázquez-Rojas, M., Salgado-Rosas, H., Torres-Rasgado, E., and Talamás-Rohana, P. (2000). Increased nitric oxide levels in patients with acute intestinal amebiasis. Arch. Med. Res. 31, S87–S88. doi: 10.1016/S0188-4409(00)00179-X
Pérez-González, M. Z., Gutiérrez-Rebolledo, G. A., Yépez-Mulia, L., Rojas-Tomé, I. S., Luna-Herrera, J., and Jiménez-Arellanes, M. A. (2017). Antiprotozoal, antimycobacterial, and anti-inflammatory evaluation of Cnidoscolus chayamansa (Mc Vaugh) extract and the isolated compounds. Biomed. Pharmacother. 89, 89–97. doi: 10.1016/j.biopha.2017.02.021
Perron, N. R., García, C. R., Pinzón, J. R., Chaur, M. N., and Brumaghim, J. L. (2011). Antioxidant and prooxidant effects of polyphenol compounds on copper-mediated DNA damage. J. Inorg. Biochem. 105, 745–753. doi: 10.1016/j.jinorgbio.2011.02.009
Pietta, P. G. (2000). Flavonoids as antioxidants. J. Nat. Prod. 63, 1035–1042. doi: 10.1021/np9904509
Pizzino, G., Irrera, N., Cucinotta, M., Pallio, G., Mannino, F., Arcoraci, V., et al. (2017). Oxidative stress: harms and benefits for human health. Oxid. Med. Cell. Longev. 2017:8416763. doi: 10.1155/2017/8416763
Prior, R. L., and Cao, G. H. (2000). Antioxidant phytochemicals in fruits and vegetables: diet and health implications. HortScience 35, 588–592.
Procházková, D., Boušová, I., and Wilhelmová, N. (2011). Antioxidant and prooxidant properties of flavonoids. Fitoterapia 82, 513–523. doi: 10.1016/j.fitote.2011.01.018
Samarawickrema, N. A., Brown, D. M., Upcroft, J. A., Thammapalerd, N., and Upcroft, P. (1997). Involvement of superoxide dismutase and pyruvate:ferredoxin oxidoreductase in mechanisms of metronidazole resistance in Entamoeba histolytica. J. Antimicrob. Chemother. 40, 833–840. doi: 10.1093/jac/40.6.833
Scotti, L., Ferreira, E. I., da Silva, M. S., and Scotti, M. T. (2010). Chemometric studies on natural products as potential inhibitors of the NADH oxidase from Trypanosoma cruzi using the volsurf approach. Molecules 15, 7363–7377. doi: 10.3390/molecules15107363
Scotti, L., Ishiki, H., Mendonca Junior, F. J., Da Silva, M. S., and Scotti, M. T. (2015). In-silico analyses of natural products on Leishmania enzyme targets. Mini Rev. Med. Chem. 15, 253–269. doi: 10.2174/138955751503150312141854
Sehgal, D., Bhattacharya, A., and Bhattacharya, S. (1996). Pathogenesis of infection by Entamoeba histolytica. J. Biosci. 21, 423–432. doi: 10.1007/BF02703099
Sen, A., Chatterjee, N. S., Akbar, M. A., Nandi, N., and Das, P. (2007). The 29-kilodalton thiol-dependent peroxidase of Entamoeba histolytica is a factor involved in pathogenesis and survival of the parasite during oxidative stress. Eukaryotic Cell 6, 664–673. doi: 10.1128/EC.00308-06
Sen, G., Mukhopadhyay, S., Ray, M., and Biswas, T. (2008). Quercetin interferes with iron metabolism in Leishmania donovani and targets ribonucleotide reductase to exert leishmanicidal activity. J. Antimicrob. Chemother. 61, 1066–1075. doi: 10.1093/jac/dkn053
Sharma, S. K., Parasuraman, P., Kumar, G., Surolia, N., and Surolia, A. (2007). Green tea catechins potentiate triclosan binding to enoyl-ACP reductase from Plasmodium falciparum (PfENR). J. Med. Chem. 50, 765–775. doi: 10.1021/jm061154d
Singh, S., Bharti, N., and Mohapatra, P. P. (2009). Chemistry and biology of synthetic and naturally occurring antiamoebic agents. Chem. Rev. 109, 1900–1947. doi: 10.1021/cr068217k
Soto, J., Gómez, C., Calzada, F., and Ramírez, M. E. (2010). Ultrastructural changes on Entamoeba histolytica HM1-IMSS caused by the flavan-3-ol, (-)-epicatechin. Planta Med. 76, 611–612. doi: 10.1055/s-0029-1240599
Spanou, C., Veskoukis, A. S., Kerasioti, T., Kontou, M., Angelis, A., Aligiannis, N., et al. (2012). Flavonoid glycosides isolated from unique legume plant extracts as novel inhibitors of xanthine oxidase. PLoS ONE 7:e32214. doi: 10.1371/journal.pone.0032214
Spencer, J., Kuhnle, G., and Rice-Evans, C. (2003). Peroxynitrite scavenging by flavonoids and flavonoid metabolites. Free Radic. Biol. Med. 35, S48–S48.
Su, X. Z., and Miller, L. H. (2015). The discovery of artemisinin and the nobel prize in physiology or medicine. Sci. China Life Sci. 58, 1175–1179. doi: 10.1007/s11427-015-4948-7
Takahashi, Y., Odbayar, T. O., and Ide, T. (2009). A comparative analysis of genistein and daidzein in affecting lipid metabolism in rat liver. J. Clin. Biochem. Nutr. 44, 223–230. doi: 10.3164/jcbn.08-211
Tamayo, E. M., Iturbe, A., Hernández, E., Hurtado, G., de Lourdes Gutiérrez, X. M., Rosales, J. L., et al. (2005). Trypanothione reductase from the human parasite Entamoeba histolytica: a new drug target. Biotechnol. Appl. Biochem. 41, 105–115. doi: 10.1042/BA20040006
Tipoe, G. L., Leung, T. M., Hung, M. W., and Fung, M. L. (2007). Green tea polyphenols as an anti-oxidant and anti-inflammatory agent for cardiovascular protection. Cardiovasc. Hematol. Disord. Drug Targets 7, 135–144. doi: 10.2174/187152907780830905
Tiwari, K., Kumar, R., and Dubey, V. K. (2016). Biochemical characterization of dihydroorotase of Leishmania donovani: understanding pyrimidine metabolism through its inhibition. Biochimie 131, 45–53. doi: 10.1016/j.biochi.2016.09.009
Tsutsumi, V., Mena-López, R., Anaya-Velázquez, F., and Martínez Palomo, A. (1984). Cellular bases of experimental amebic liver-abscess formation. Am. J. Pathol. 117, 81–91.
Valentova, K., Vrba, J., Bancirova, M., Ulrichova, J., and Kren, V. (2014). Isoquercitrin: pharmacology, toxicology, and metabolism. Food Chem. Toxicol. 68, 267–282. doi: 10.1016/j.fct.2014.03.018
Valko, M., Leibfritz, D., Moncol, J., Cronin, M. T., Mazur, M., and Telser, J. (2007). Free radicals and antioxidants in normal physiological functions and human disease. Int. J. Biochem. Cell Biol. 39, 44–84. doi: 10.1016/j.biocel.2006.07.001
Vanacker, S. A., Tromp, M. N., Haenen, G. R., Vandervijgh, W. J., and Bast, A. (1995). Flavonoids as scavengers of nitric-oxide radical. Biochem. Biophys. Res. Commun. 214, 755–759. doi: 10.1006/bbrc.1995.2350
Veitch, N. C., and Grayer, R. J. (2006). “Chalcones, dihydrochalcones, and aurores,” in Flavonoids Chemistry, Biochemistry and Applications, ed Ø. M. Andersen (Boca Raton, FL: CRC Press), 1003–1071.
Wang, S., Yang, C., Tu, H., Zhou, J., Liu, X., Cheng, Y., et al. (2017). Characterization and metabolic diversity of flavonoids in Citrus species. Sci. Rep. 7:10549. doi: 10.1038/s41598-017-10970-2
Werner Apt, B. (2014). Infecciones por parásitos más frecuentes y su manejo. Rev. Méd. Clín. Condes 25, 485–528. doi: 10.1016/S0716-8640(14)70065-3
Wolfe, K. L., and Liu, R. H. (2008). Structure-activity relationships of flavonoids in the cellular antioxidant activity assay. J. Agric. Food Chem. 56, 8404–8411. doi: 10.1021/jf8013074
Yu, J., Bi, X., Yu, B., and Chen, D. (2016). Isoflavones: anti-inflammatory benefit and possible caveats. Nutrients 8:E361. doi: 10.3390/nu8060361
Keywords: Entamoeba histolytica, flavonoids, alternative treatment, anti-oxidants, anti-inflammatory response, metronidazole
Citation: Martínez-Castillo M, Pacheco-Yepez J, Flores-Huerta N, Guzmán-Téllez P, Jarillo-Luna RA, Cárdenas-Jaramillo LM, Campos-Rodríguez R and Shibayama M (2018) Flavonoids as a Natural Treatment Against Entamoeba histolytica. Front. Cell. Infect. Microbiol. 8:209. doi: 10.3389/fcimb.2018.00209
Received: 06 March 2018; Accepted: 05 June 2018;
Published: 22 June 2018.
Edited by:
Serge Ankri, Technion – Israel Institute of Technology, IsraelReviewed by:
Maryam Dadar, Razi Vaccine and Serum Research Institute, IranElisa Azuara-Liceaga, Universidad Autónoma de la Ciudad de México, Mexico
Copyright © 2018 Martínez-Castillo, Pacheco-Yepez, Flores-Huerta, Guzmán-Téllez, Jarillo-Luna, Cárdenas-Jaramillo, Campos-Rodríguez and Shibayama. This is an open-access article distributed under the terms of the Creative Commons Attribution License (CC BY). The use, distribution or reproduction in other forums is permitted, provided the original author(s) and the copyright owner are credited and that the original publication in this journal is cited, in accordance with accepted academic practice. No use, distribution or reproduction is permitted which does not comply with these terms.
*Correspondence: Mineko Shibayama, bWluZWtvQGNpbnZlc3Rhdi5teA==