- 1Department of Microbiology, Faculty of Biochemistry, Biophysics and Biotechnology, Jagiellonian University, Krakow, Poland
- 2Department of Oral Immunology and Infectious Diseases, School of Dentistry, University of Louisville, Louisville, KY, United States
The pathogenesis of the chronic periodontal disease is associated with a skewed host inflammatory response to periodontal pathogens, such as Porphyromonas gingivalis, that accounts for the majority of periodontal tissue damage. Neutrophils are the most abundant leukocytes in periodontal pockets and depending on the stage of the disease, also plentiful PMNs are present in the inflamed gingival tissue and the gingival crevice. They are the most efficient phagocytes and eliminate pathogens by a variety of means, which are either oxygen-dependent or -independent. However, these secretory lethal weapons do not strictly discriminate between pathogens and host tissue. Current studies describe conflicting findings about neutrophil involvement in periodontal disease. On one hand literature indicate that hyper-reactive neutrophils are the main immune cell type responsible for this observed tissue damage and disease progression. Deregulation of neutrophil survival and functions, such as chemotaxis, migration, secretion of antimicrobial peptides or enzymes, and production of reactive oxygen species, contribute to observed tissue injury and the clinical signs of periodontal disease. On the other hand neutrophils deficiencies in patients and mice also result in periodontal phenotype. Therefore, P. gingivalis represents a periodontal pathogen that manipulates the immune responses of PMNs, employing several virulence factors, such as gingipains, serine proteases, lipid phosphatases, or fimbriae. This review will sum up studies devoted to understanding different strategies utilized by P. gingivalis to manipulate PMNs survival and functions in order to inhibit killing by a granular content, prolong inflammation, and gain access to nutrient resources.
Periodontitis
Periodontitis is an inflammatory disease, which affects tissues surrounding and supporting the teeth. It occurs in response to dysbiotic periodontal microbiota accumulating as a bacterial plaque on the tooth surface below the gum line that triggers a chronic inflammatory reaction (Graves et al., 2008). Dysbiotic bacteria, also called pahtobionts, are commensal microorganisms that under conditions of disrupted homeostasis have a potential to deregulate inflammatory responses and cause disease (Hajishengallis and Lamont, 2012). Chronic periodontitis is one of the most prevalent inflammatory diseases of humans and in its most severe form it is the sixth most prevalent disease affecting 11.2% of the global population and representing a substantial public health burden (Kassebaum et al., 2014). Risk factors for developing periodontal disease (PD) are genetic factors, cigarette smoking, diabetes, osteoporosis, age, and infections with red complex bacteria. Red complex bacteria is a group of three species including Porphyromonas gingivalis, Treponema denticola, and Tannerella forsythia, which presence is strongly associated with each other and disease sites (Socransky et al., 1998; Van Dyke and Sheilesh, 2005).
According to the well-accepted polymicrobial synergy and dysbiosis model, the host immune response is initially manipulated by keystone pathogens (e.g., P. gingivalis) with the help of accessory pathogens and is subsequently over-activated by pathobionts (Hajishengallis et al., 2015a). This concept of keystone species is derived from basic ecological studies. A keystone pathogen is a microorganism that exerts a huge impact on the microbiotic community, while being present at very small quantity (Darveau et al., 2012). Another hypothesis on the development of the oral diseases is based on the imbalance in the oral microflora caused by ecological stress, causing an enrichment of some periodontal pathogens (Marsh, 1994, 2003). This “Ecological Plaque Hypothesis” is supported by the fact that expressed by P. gingivalis gingipains degrade collagen (Houle et al., 2003) and therefore enrich the growth environment in peptides favoring growth of the red complex bacteria. A futile attempt of the host to eradicate periodontopathogens fuels a chronic inflammatory reaction in the infected periodontium. In genetically susceptible hosts, this leads to a dissolution of periodontal ligament, alveolar bone resorption, deep periodontal pocket formation, and eventual tooth loss (Reynolds and Meikle, 1997). The presence of keystone pathogens can cause deregulated inflammation and disease without apparent predispositions (Hajishengallis, 2014).
Neutrophils' Functions and Survival
Neutrophils, also called polymorphonuclear leukocytes or, in short, PMNs, are the most abundant white blood cells in the gingival crevice and periodontal pocket, where they play a crucial role in the innate immunity response against bacterial infection and thus are responsible for the maintenance of homeostasis in periodontal tissues. PMNs are produced in the bone marrow in large amounts, meaning 5−10 × 1010 cells per day, and are released into the peripheral blood as terminally differentiated and fully competent effector cells (Borregaard, 2010). This is in contrast to adaptive immunity, where T and B lymphocytes require activation and proliferation steps in secondary lymphatic organs in order to become effector cells (Segal, 2005; Nathan, 2006).
Neutrophils are the most efficient phagocytes and they eliminate pathogens by a variety of means, which are either oxygen-dependent (oxidative burst) or oxygen-independent (anti-microbial peptides and lytic or proteolytic enzymes; Figure 1). Neutrophil priming by pro-inflammatory signals recruits the cytosolic NADPH oxidase complex to the phagosome membrane which leads to the generation of reactive oxygen species (ROS). The respiratory burst can disrupt bacterial phospholipid bilayers, degrade or inactivate proteins, and trigger DNA damage (Segal, 2005; Nauseef, 2007). Importantly, these processes can occur in hypoxic periodontal pockets, where oxygen concentration is as low as 1–3% (Loesche et al., 1988). In order to meet high-energy requirements, neutrophils engage glycolysis, which is a huge advantage under hypoxic conditions present in periodontal pockets. This unique strategy is in contrast to ATP production mechanisms in most cells in the human body (Borregaard and Herlin, 1982). Non-oxidative microbial killing relies on the contents of three types of cytoplasmic granules, namely: azurophilic (primary) granules, specific (secondary) granules, and gelatinase granules. Neutrophil activation triggers granule fusion with phagosomes. These granules deliver antimicrobial proteins and peptides, such as azurocidin, cathelicidin, α-defensins, lysozyme, lactoferrin, elastase, and cathepsin G, that disrupt bacterial cell envelope, destroy peptydoglican, degrade proteolytic bacterial virulence factors, or sequester iron (Soehnlein, 2009). Beside this antimicrobial arsenal, PMNs can additionally form neutrophil extracellular traps (NETs), which are composed of decondensed nuclear or mitochondrial DNA associated with antibacterial (granule) enzymes, peptides, and histones. These extracellular structures are designed to disable invading pathogens and elicit proinflammatory responses (White P. C. et al., 2016). PMNs have the shortest lifespan of all immune cells, i.e., around 24 h under the steady state, while for example T lymphocytes may stay alive for weeks. Normally, neutrophils circulate in the blood for 6–12 h and then home to the bone marrow, spleen or liver where they undergo apoptosis. Subsequently, they are phagocytosed by Kupffer cells in the liver or by red pulp macrophages in the spleen (Summers et al., 2010; Vier et al., 2016). This short life-span of neutrophils is tightly controlled by apoptosis, which is a form of programmed cell death relying on enzymes of the Caspase family of endopeptidases. It is a critical process involved in embryonic development or the maintenance of tissue homeostasis in the adult organism. Its deregulation is implicated in different pathologies, including cancerogenesis or disorders of the immune system (Sochalska et al., 2016; Tuzlak et al., 2016). Apoptosis is a very precise process controlled by the Bcl-2 family proteins, which encompasses many pro- and anti-apoptotic proteins that form homo- or heterodimers in order to promote or prevent apoptosis (Sochalska et al., 2015). The pro-survival family members, i.e., Bcl-2, Bcl-xL, Bcl-w, Mcl-1, and A1, share four BH (Bcl-2 homology) domains and beside A1, they possess a transmembrane domain at the C-terminal end. They prevent apoptosis by sequestering (inhibiting) pro-apoptotic BH3-only proteins, such as Bim, Bmf, Noxa, Puma, Bid, Bad, Bmf, and HRK. The BH3-only proteins act as sentinels for various stress stimuli, such as DNA damage, growth factor deprivation, ER-stress or oncogenic transformation (Tuzlak et al., 2016). Moreover, after successful phagocytosis of invading bacteria, neutrophils undergo apoptosis, a very important step for the resolution of inflammation, which is called phagocytosis-induced cell death (PICD). Exposure of the cell to an apoptotic stimulus frequently engages BH3-only proteins, either transcriptionally or translationally, which allows them to either directly (Bim and tBid) or indirectly (all BH3-only) activate the pro-apoptotic effector proteins Bax/Bak (Czabotar et al., 2014; Garcia Saez and Villunger, 2016).
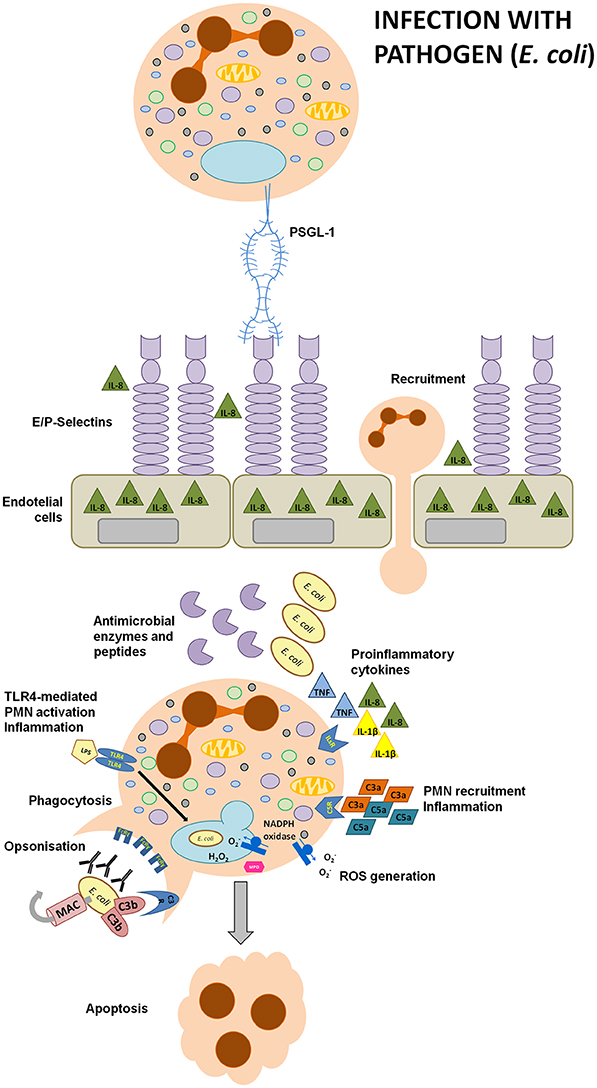
Figure 1. Immune responses to pathogens. During an infection with pathogens, for example E. coli, lipopolisaccharide enhances the secretion of chemotactic IL-8 and stimulates the upregulation of E- or P-selectins expression on gingival endothelial cells (GECs). Selectins facilitate neutrophils adhesion during transmigration as they interact with PSGL-1 expressed on PMNs. Moreover, the presence of microbes and their particles activates the complement cascade. C3a and C5a are anaphylatoxins with a strong chemotactic and pro-inflammatory potential. IgG and IgM antibodies or C3b recognize bacterial antigens and opsonize invading pathogens thus facilitating bacterial phagocytosis. LPS activates the TLR4 signaling pathway in recruited neutrophils, eliciting strong inflammatory responses designed to inactivate the pathogen. Inflammatory responses include the production of reactive oxygen species, secretion of pro-inflammatory cytokines and antimicrobial enzymes or peptides, such as cathepsin G, elastase, cathelicidins or defensins. After a successful bacterial clearance, neutrophils undergo apoptosis, an essential process triggering the resolution of inflammation.
However, in the context of P. gingivalis infection, neutrophils are unable to phagocyte this periodontopathogen present within the huge biofilm structure. During phagocytosis of something too big to be ingested, a process so-called “frustrated phagocytosis,” they generate reactive oxygen species and secrete enzymes in order to destroy the pathogen. Unfortunately, these secreted weapons concomitantly contribute to the inflammatory destruction of gingival tissues and alveolar bone (Scott and Krauss, 2012).
Neutrophils in Periodontitis
On one hand, functional neutrophils are indispensable for the maintenance of periodontal health, as illustrated by several neutrophil genetic defects, including neutropenias (Ryder, 2010; Wiesmeier et al., 2016), LAD-I Syndrome (Leukocyte Adhesion Deficiency Type I; Moutsopoulos et al., 2014) or Papillon-Lefevre syndrome (Eick et al., 2014), which are associated with periodontal phenotype. On the other hand, neutrophilia, which is the accumulation of neutrophils and their histotoxic cargo in the tissue, has been clinically linked to cystic fibrosis and meningitis and may also account for the currently unknown etiology of inflammatory bowel disease, Crohn's disease, ulcerative colitis, or periodontal disease (Hajishengallis et al., 2008a; Koedel et al., 2009; Levine and Segal, 2013; Gifford and Chalmers, 2014; Prat et al., 2014). Thus, tight control of neutrophil functions and survival after bacterial clearance by induction of apoptosis, immune tolerance and resolution of inflammation, exert protective effects toward the host tissues against inflammatory tissue damage. A failure to properly regulate neutrophil abundance and turnover directly contributes to the pathogenesis of periodontitis and current literature implicates neutrophils as the main immune cell type responsible for periodontal disease progression (Hajishengallis et al., 2008b, 2015a; Scott and Krauss, 2012).
A hyper-reactive neutrophil phenotype is considered to play a central function in the periodontal disease (Chapple and Matthews, 2007; Scott and Krauss, 2012; Hajishengallis and Lamont, 2016) and, importantly, many systemic inflammatory diseases are associated with periodontitis, including cardiovascular disease, rheumatoid arthritis and diabetes type 2. As such, “hyper-inflammation” may represent one casual mechanism by which periodontitis contributes to co-morbidity (Hatanaka et al., 2006; Yasunari et al., 2006; Koziel et al., 2014b).
Periodontitis Patient-Derived Neutrophils
A chronic type of the periodontal disease is frequently associated with P. gingivalis infection (Guentsch et al., 2009). As neutrophil influx into periodontal pockets is strongly induced during such infections, several studies analyzed the functions and survival of periodontitis patient-derived neutrophils. Patients suffering from PD showed overly increased numbers of neutrophils in the oral cavity (Bender et al., 2006). Accordingly, one microarray study characterized neutrophil transcriptome changes at the site of inflammation (the oral cavity) and compared the gene expression in the periphery in healthy and PD subjects. The only significant changes were found in the “apoptosis” cluster (Lakschevitz et al., 2013). Another study revealed that GM-CSF-dependent neutrophil apoptosis was diminished in periodontitis biopsies, as analyzed by TUNEL staining. Moreover, accumulation of GM-CSF and TNF-α in gingival tissue sections was noted in the majority of diseased sites (Gamonal et al., 2003). Furthermore, functionality of tissue-derived and peripheral blood neutrophils from PD patients was deregulated. These PMNs produced higher baseline levels of ROS and elastase (Matthews et al., 2007; Guentsch et al., 2009; Aboodi et al., 2011; Damgaard et al., 2017) and secreted more pro-inflammatory cytokines, namely IL-8, IL-6, IL-1β, and TNF-α (Ling et al., 2015). Additional challenge of patient-derived neutrophils with P. gingivalis augmented the secretion of elastase and pro-inflammatory cytokines (Guentsch et al., 2009; Ling et al., 2015). Moreover, further exposure of PMNs to either P. gingivalis- or E. coli-derived LPS enhanced ROS generation and cathelicidin (LL-37) secretion. Similar hyper-activation of neutrophils was noted upon FcɤR engagement and treatment with either GM-CSF or phorbol ester (PMA; Johnstone et al., 2007; Matthews et al., 2007; Guentsch et al., 2009; Mariano et al., 2012). In contrast, reduced production of nitric oxide (NO) by peripheral PMNs from PD patients was noted (Mariano et al., 2012), which may actually contribute to P. gingivalis persistence in the gingival tissue. Importantly, a potential oral-systemic inflammatory activation loop (a self-driving sequence of events) was suggested to operate in patient-derived neutrophils. Analysis of serum isolated from aggressive and chronic periodontitis patients revealed elevated systemic antibodies to P. gingivalis (Guentsch et al., 2009), while plasma from periodontal patients was more efficient in neutrophil priming to fMLP and directly induced the oxidative burst (Dias et al., 2008). Interestingly, this excessive neutrophil activation could be abolished by antibodies neutralizing IL-8, GM-CSF, or IFN-α (Dias et al., 2011). Interestingly, elevated NETs release was noted in patients suffering from gingivitis, but no differences were observed in peripheral neutrophils isolated from periodontitis patients. However, NETs degradation by plasma was significantly reduced in periodontitis patients, what might contribute to the chronic inflammation, exaggerated inflammatory responses and tissue destruction observed in PD patients. Importantly, the authors noted that a non-surgical periodontal treatment restored full plasma capacity (Cooper et al., 2013; White P. et al., 2016). These patient data demonstrated that chronic periodontitis is associated with accumulation in the gingiva of hyper-active neutrophils, which exhibit an elevated release of pro-inflammatory cytokines, ROS, and anti-bacterial enzymes and show defects in apoptosis. Strikingly, P. gingivalis-derived LPS might be found in the bloodstream in over 50% of periodontitis patients, what clearly might modulate neutrophil and other white blood cells responsiveness (Deleon-Pennell et al., 2013). This deregulation of leukocyte function could modulate not only local, but also systemic inflammatory-immune responses and influence the risk and severity of periodontitis-associated systemic inflammatory diseases. Therefore, many studies have been devoted to elucidate molecular mechanisms underlying such aberrant functions and prolonged survival of neutrophils in the context of P. gingivalis infection.
Immune Host Responses in Mouse Models of Periodontal Disease and Their Limitations
Keeping in mind that studies of periodontitis in humans are burdened with some limitations due to variables that are difficult to control among patients, animal models enable studying development, and progression of periodontal disease in a precise and controlled manner. Moreover, genetically modified mice allow to uncover defined molecular aspects of periodontitis for example involvement of specific immune cell types or signaling pathways in pathogenesis of periodontal disease.
The localized injection of periodontal pathogen P. gingivalis or its component into the palatal gingival tissue, oral gavage or ligature models are very convenient mice models of periodontitis. These models are characterized by periodontal tissue destruction as well as inflammation as measured by increased expression of inflammatory cytokines, such as IL-1β, IL-6, and TNF-α, which are typical hallmarks of periodontal disease observed in patients (Adamowicz et al., 2012; Saadi-Thiers et al., 2013; de Molon et al., 2013; Maekawa et al., 2014). Importantly, it was reported that the ligature model and injection of heat-killed P. gingivalis model were the most representative of periodontal disease in humans, whereas the oral gavage models were not effectively inducing periodontitis under the experimental conditions (de Molon et al., 2014). Thus, certainly some of these in vivo mouse models are burdened with limitations. Nevertheless, analysis of for example periodontitis induced by ligatures (either previously incubated with P. gingivalis or not) or oral gavage application of P. gingivalis strain ATCC 33277, revealed elevated serum levels of IL-1β and IL-6 (Saadi-Thiers et al., 2013). These results clearly indicated that P. gingivalis sustained periodontal inflammation. However, it is difficult to state which particular cells are responsible for this effects. Especially that pro-inflammatory cytokines such as IL-1β, IL-6, or TNF-α are produced by a variety of immune cell types (Arango Duque and Descoteaux, 2014).
The best mouse model, which allows analysis of specific immune cell types responsible for P. gingivalis-mediated inflammation is the mouse chamber model. This model involves injectable inoculation of bacteria into the lumen of a subcutaneously implanted titanium-coil chamber is better to pinpoint which particular cell types promote inflammation. Strikingly, over 95% of recruited cells to the chambers are Ly6G+ leukocytes (neutrophils). Therefore, reported by Maekawa and colleagues accumulation of pro-inflammatory mediators by in the fluid aspirated from chambers as measured by levels of IL-1β, IL-6, TNFα, or IL-17 was distinctly due to neutrophils activation (Maekawa et al., 2014). Thus, this chamber mouse model clearly confirmed that P. gingivalis triggers neutrophils inflammatory responses at the site of infection. Nevertheless, these in vivo results were validated using either ATRA differentiated HL-60 neutrophils or neutrophils isolated from human blood (Maekawa et al., 2014). Therefore, results obtained in vivo during analysis of mouse models of periodontitis need to be further verified ex vivo or in vitro.
Another possibility to overcome obstacles during analysis of actual cell types responsible for P. gingivalis-mediated inflammation is visualization by confocal microscopy. Adamowicz and colleagues quantified co-localization of infiltrating Ly6G+ neutrophils and pro-inflammatory IL-17 cytokine expression in periodontal tissues in P. gingivalis–induced periodontal bone loss model, clearly showing neutrophil contribution to periodontal inflammation (Adamowicz et al., 2012).
In order to pinpoint contribution of the specific immune cells in development and progression of periodontitis genetically modified mice deficient in leukocytes or lymphocytes were analyzed. For example it was shown that mice, which failed to develop MHC class II-reactive CD4+ T cells, presented with significantly reduced bone loss. Of note, analysis of mice devoid of NK T cells or CD8+ T cells did not protect mice from periodontitis (Baker et al., 1999). However, to our best knowledge, impact of neutrophils ablation on periodontal disease development and progression was not analyzed in any of mouse models of periodontitis. Instead, it was reported by Hajishengallis that loss of neutrophil infiltration inhibited disease development (Eskan et al., 2012). Mice deficient in Del-1, a negative regulator of neutrophil LFA-1-dependent recruitment to periodontium, exhibited enhanced neutrophil infiltration and IL-17-mediated inflammatory bone loss. In opposite, other authors described that IL-17-mediated neutrophil recruitment was critical in protection against P. gingivalis-induced alveolar bone loss in a mouse model (Yu et al., 2007). Along the same lines, other authors noted that neutrophil infiltration as well as CD45+ B lymphocytes was beneficial in mice infected with P. gingivalis (Settem et al., 2014). Importantly, IL-17 produced by Th17 cells themselves can drive periodontal bone loss in chronic P. gingivalis infections (Moutsopoulos et al., 2012). In chronic settings neutrophils themselves can also become a source of IL-17, leading to IL-17-dependent bone loss (Hajishengallis and Hajishengallis, 2014). Therefore, the complex role of leukocytes, lympohocytes, and IL-17 (Th17) in periodontitis remind to be determined.
Nevertheless, these results indicated that mouse models are certainly very useful tools in analysis of P. gingivalis-mediated periodontal inflammation and in vivo analysis contribute to development targeted therapeutics modulating inflammatory cascades regulated by complement (Hajishengallis and Lambris, 2013), GSK-3 (Adamowicz et al., 2012), regulatory T cells (Glowacki et al., 2013), and neutrophils (Eskan et al., 2012).
Corruption of Neutrophils by Porphyromonas gingivalis
P. gingivalis has developed a variety of mechanisms in order to overcome neutrophil-mediated killing and sustain inflammation, which allows colonization and contributes to tissue damage. This periodontopathogen utilizes several virulence factors that manipulate neutrophils recruitment, survival, and functions at the site of infections. This review will focus on virulence factors involved in the subversion of neutrophils, namely serine phosphatase (SerB), fimbriae, gingipains (RgpA, RgpB, and Kgp), P. gingivalis peptidyl arginine deiminase (PPAD), lipopolysaccharide (LPS), and its component (lipid A) (summarized in Table 1).
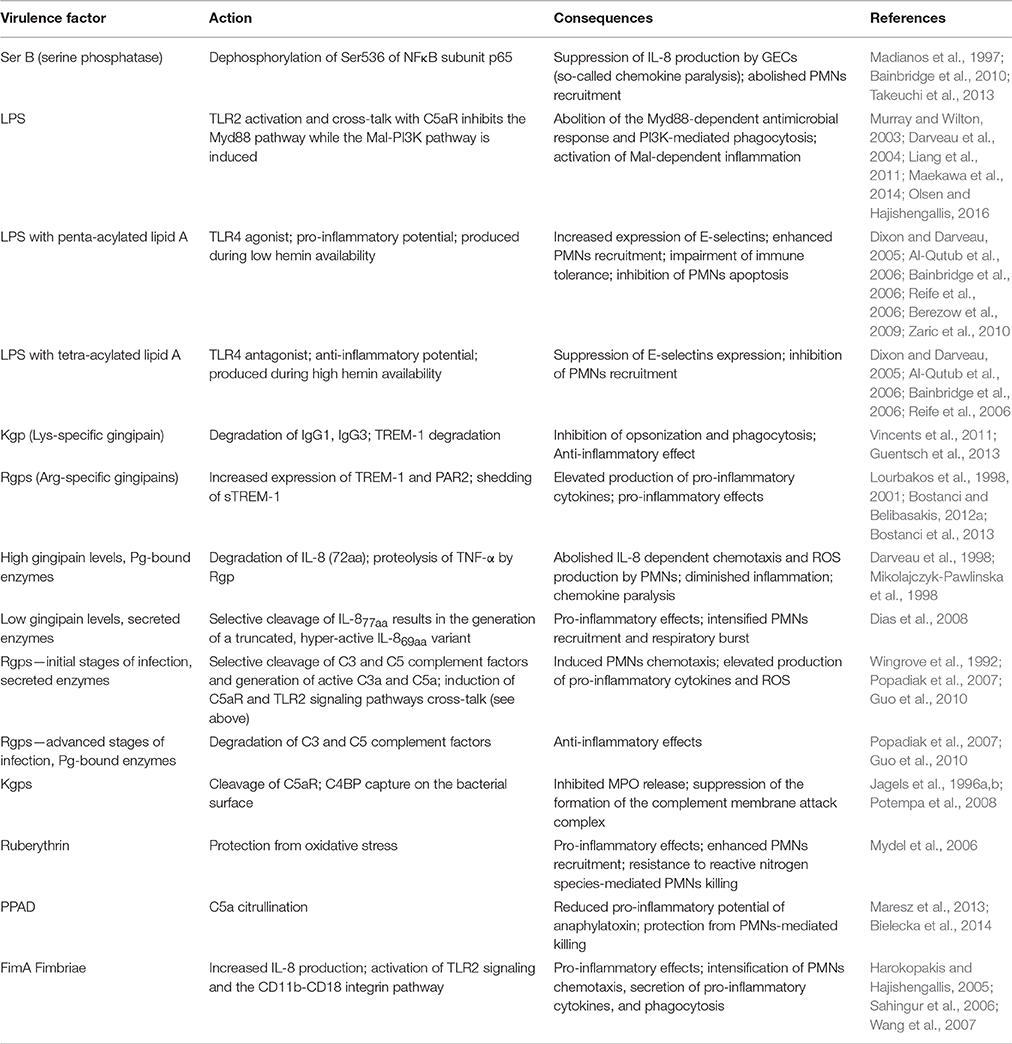
Table 1. P. gingivalis virulence factors involved in the manipulation of neutrophil immune responses.
PMNs Deregulation by SerB
Serine phosphatase is an enzyme secreted by P. gingivalis that modulates inflammatory responses, as it was shown to inhibit IL-8 production by gingival epithelial cells through the dephosphorylation of serine S536 of p65 NF-κB subunit. This subsequently abolished PMNs recruitment and gave P. gingivalis enough time for colonization of periodontal pockets (Figure 2). Such insidious manipulation of cell signaling protected this periodontopathogen and bystander bacteria from neutrophil killing during the initial stages of infection. Consequently, SerB expression enhanced alveolar bone resorption in experimentally induced periodontitis in rats (Madianos et al., 1997; Bainbridge et al., 2010; Takeuchi et al., 2013). This implies that manipulation of NF-κB signaling by SerB hinders PMNs recruitment and bacterial clearance in the periodontium and therefore is involved in a pathogenic mechanism referred to as a local chemokine paralysis.
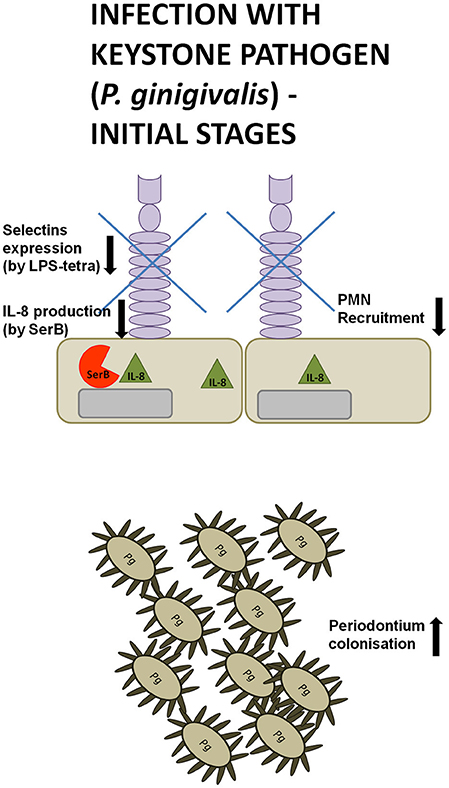
Figure 2. Manipulation strategies of neutrophils and the immune system by P. gingivalis at the initial phase of infection. During the initial phase of an infection with the keystone pathogen P. gingivalis (Pg) secretes serine phosphatase (SerB), inhibiting IL-8 production. At the same time, a tetra-acylated lipid A variant of P. gingivalis LPS suppresses the expression of L- and P-selectins on gingival epithelial cells. These manipulation strategies hinder neutrophil recruitment, giving periodontal pathogens enough time for colonization of periodontal pockets.
PMNs Deregulation by LPS
Toll-like receptors (TLR) engagement, neutrophil recruitment to the site of inflammation, pro-inflammatory cytokine release, and subsequent elimination of bacteria by PMNs are essential mechanisms of the host immune response against infection. However, after the bacterial clearance, several processes need to be engaged to prevent inflammatory tissue destruction, including the induction of immune tolerance and removal of apoptotic neutrophils from the site of infection. They contribute to the control of the host's defense and trigger resolution of inflammation (Bratton and Henson, 2011; McCracken and Allen, 2014). Lipopolysaccharide (LPS), also known as endotoxin, is a fundamental structural element of the cell envelope of gram-negative bacteria and it is able to elicit appropriate host innate responses. LPS is composed of three elements, namely O-antigen, core polysaccharide, and lipid A. The latter is responsible for the toxicity of Gram-negative bacteria and consists of di-glucosamine with two phosphate moieties at both 1′- and 4′-positions of the disaccharide backbone, where acyl chains are attached (Kumada et al., 1995; Raetz et al., 2007, 2009). However, P. gingivalis can deceitfully modify its lipid A structure due to both dephosphorylation and deacylation in order to manipulate host immune responses and promote chronic inflammation (Dixon and Darveau, 2005; Al-Qutub et al., 2006; Reife et al., 2006). When considering the heterogeneous acylation patterns of P. gingivalis lipid A, two variants are predominant, namely tetra-acylated and penta-acylated. Strikingly, these two variants were described to induce opposing host responses. The penta-acylated lipid A activated TLR4 (Toll-like receptor 4) signaling, whereas tetra-acylated lipid A variant had antagonistic effects against TLR4. Importantly, these changes in acylation were dependent on microenvironmental conditions. In particular, when bacteria were grown in low hemin conditions, LPS consisted of phosphorylated, penta-acylated lipid A structure, which exerted weak LPS agonistic effects. Whereas, during high hemin availability, mimicking an inflammatory condition, phosphorylated penta-acylated lipid A was converted into non-phosphorylated tetra-acylated lipid A, and exhibited an antagonistic activity (Al-Qutub et al., 2006). Moreover, tetra-acylated version of P. gingivalis lipid A was shown to inhibit E-selectin expression, while penta-acylated lipid A variant had a stimulatory effect (Reife et al., 2006). Therefore, P. gingivalis can exert opposing effects on the expression of this adhesion molecule expressed on endothelial cells, which impairs neutrophil transmigration during inflammation (Figures 2, 3).
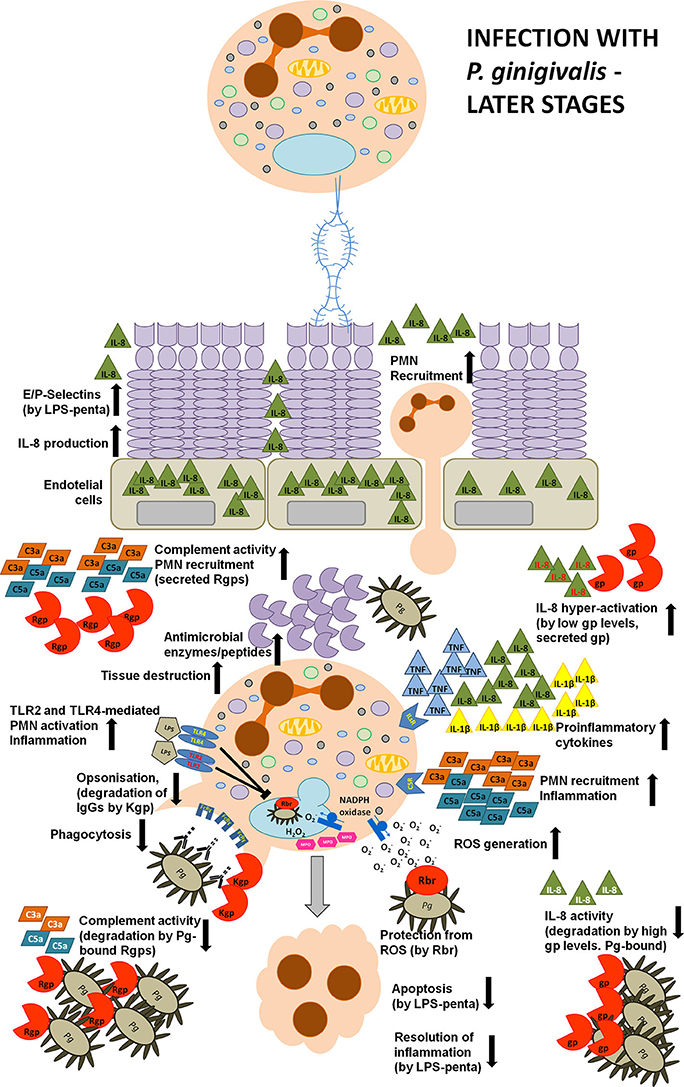
Figure 3. Manipulation strategies of neutrophils and the immune system by P. gingivalis at the later phase of infection. During a later phase of the infection P. gingivalis releases a penta-acylated LPS variant leading to the increased expression of L- and P-selectins on GECs and enhanced production of IL-8. This strongly stimulates neutrophil chemotaxis and transmigration to the site of infection. Moreover, P.g.-derived LPS and fimbriae strongly stimulate neutrophil pro-inflammatory and anti-bacterial responses, such as the secretion of reactive oxygen species and pro-inflammatory cytokines, and the production of anti-microbial peptides and enzymes. An elevated secretion of these anti-bacterial molecules results in gingival tissue destruction, while many virulence factors secreted by P. gingivalis protect this periodontopathogen from the consequences of hyper-inflammation. The keystone pathogen is protected from oxidative stress, as it expresses ruberythrin (Rbr) protein. Additionally, the expression of Lys-specific gingipains degrades immunoglobulins at the hinge region, and, coupled with the activation of the TLR2 signaling pathway (by LPS), abolishes bacterial opsonization and phagocytosis. Also, gingipains manipulate anti-bacterial responses by deregulating the complement cascade and IL-8-mediated neutrophil chemotaxis. In particular, depending on the concentration and the position of gingipains within the biofilm, these enzymes can exert opposing effects. C3, C5, and IL-8 are degraded at high gingipain concentration or by gingipains associated with bacterial cells or vesicles thus inhibiting pro-inflammatory responses and protecting bacteria from elimination. In contrast, low levels of soluble Arg-specific gingipains activate C5 and C3 by limited proteolysis that results in the generation of C5a and C3a anaphylatoxins. Furthermore, under such circumstances, gingipains can selectively cleave IL-8 generating a truncated, hyperactive IL-869aa variant (written in red) acting in concert with C5a and C3a in order to excessively activate neutrophil pro-inflammatory responses. Additionally, the secreted penta-acylated LPS variant diminishes neutrophil apoptosis which delays the resolution of inflammation Together, these events strongly contribute to the inflammatory tissue destruction observed in periodontitis and to excessive bleeding, providing P.g. and bystander bacteria with access to nutritional resources.
On top of acylation, P. gingivalis can change the phosphorylation status of lipid A (Rangarajan et al., 2008). Similarly to other Gram-negative bacteria, P. gingivalis synthesizes lipid A that initially contains two phosphate groups at the di-glucosamine backbone. However, dephosphorylation of P. gingivalis lipid A can facilitate its deacylation and subsequently manipulate neutrophil responses.
It is a well-documented innate immunity paradigm that LPS is recognized by Toll-like receptor 4 of the host innate immune system, which triggers pro-inflammatory cytokine production and promotes bacteria elimination (Murdock and Nunez, 2016; Figure 1). While it is true for the LPS of most Gram-negative species, P. gingivalis-derived LPS is also a potent activator of TLR2 (Darveau et al., 2004). Importantly, this degenerative manipulation of TLR signaling separates pathogen phagocytosis from neutrophil inflammatory response (Figure 3). This pathologic effect was achieved by the induction of a cross-talk between the complement receptor C5aR and TLR2, which inhibited the TLR-2-MyD88 signaling pathway and activated an alternative TLR2-Mal-PI3K pathway in vivo (Liang et al., 2011; Maekawa et al., 2014). The authors reported that the ability to activate TLR2 signaling had a protective effect not only toward P. gingivalis, but also toward bystander bacteria, otherwise susceptible to bacterial killing. These studies revealed the molecular mechanism of TLR signaling manipulation by P. gingivalis, which clearly contributes to the persistence of dysbiotic microbial communities and drives chronic inflammation in periodontal disease (Hajishengallis and Lambris, 2011).
Neutrophil apoptosis and subsequent uptake by macrophages, referred to as efferocytosis, is among the key processes leading to the resolution of inflammation. Therefore, neutrophil resistance to cell death and diminished efferocytosis may additionally contribute to the chronic inflammation and collateral tissue damage observed in periodontal disease (Hiroi et al., 1998; Zaric et al., 2010). Indeed, neutrophils exhibited an impaired immune tolerance to P. gingivalis endotoxin. Stimulation with penta-acylated dephosphorylated LPS, the most potent and prevalent endotoxin isoform of P. gingivalis, led to continued IL-8 secretion upon secondary exposure, but inhibited TNF-α production. Interestingly, observed results were in contrast to the effect elicited by E. coli-derived lipopolisaccharide, which abolished the production of both cytokines upon repeated treatment (Bainbridge et al., 2006; Berezow et al., 2009). This deregulated inflammatory response to P. gingivalis LPS resulted in enhanced neutrophil migratory potential while apoptosis was inhibited as identified by reduced caspase-3 activity (Bainbridge and Darveau, 2001; Reife et al., 2006; Zaric et al., 2010; Figure 3). Additionally, not only LPS and lipid A, but also capsular polysaccharide isolated from three different P. gingivalis strains (HG-184, A7A1–28, and 381) diminished human or murine PMNs cell death in a time- and dose-dependent manner (Hiroi et al., 1998; Preshaw et al., 1999) that was not influenced by IL-10 treatment (Murray and Wilton, 2003). However, taking into account that P. gingivalis endotoxin may engage TLR2 instead of TLR4, this may account for its inhibitory effect on apoptosis (Bainbridge and Darveau, 2001; Murray and Wilton, 2003). This is particularly important due to the fact that P. gingivalis LPS can penetrate gingival tissues and consequently can exert a broad impact toward all immune cells in the periodontium and affect their responses to all bacteria present within the biofilm (Schwartz et al., 1972). Therefore, manipulation of PMN apoptosis results in an accumulation of long-lived and fully active neutrophils in the inflamed periodontium, which may contribute to the development and progression of periodontal disease.
These studies provided the molecular evidence that changes in the P. gingivalis endotoxin structure influence its binding affinity to TLR4. This selectively affects downstream signaling in order to evade antibacterial responses and sustain inflammation. Collectively, LPS modifications depend on the microenvironment and exert opposing actions on the host immune system. This ensures access to nutrient resources and can potentially protect the entire microbial community from TLR-mediated immune responses.
PMNs Deregulation by Gingipains
Gingipains are major cysteine proteases, which are either membrane-bound or secreted and they account for 85% of the total proteolytic activity of P. gingivalis (Potempa et al., 1997). Based on their specificity, they are divided in two groups, namely arginine-specific (RgpA and RgpB) and lysine-specific (Kgp; Potempa et al., 2003). Working in concert, gingipains exhibit trypsin-like activity and can cleave many host components, such as the extracellular matrix, cytokines, immunoglobulins, or complement factors (Guo et al., 2010). However, these proteases are not merely responsible for an indiscriminate degradation of proteins, but show a large degree of specificity in action that accounts for much of P. gingivalis resistance to host antibacterial strategies (Bostanci and Belibasakis, 2012b). Gingipains are able to override host defense mechanisms, such as antibody opsonization, complement system activation, or pro-inflammatory signaling, which ensures this periodontopathogen's exceptional resistance to the bactericidal activity of the human serum and the killing potential of neutrophils (Figure 3).
Opsonization by natural or acquired antibodies is an important protective feature of innate and adaptive immune response and facilitates phagocytosis of invading bacteria. However, gingipain K (Kgp) has the unique ability to cleave IgG1 and IgG3 at the hinge region, thus separating the antigen binding Fab fragment from the effector Fc fragment of immunoglobulins. This activity was observed not only in vitro using isolated IgGs or human plasma, but it was also detected in vivo in gingival crevicular fluid (GCF). Consequently, treatment of patient serum samples with Kgp inhibited P. gingivalis opsonization and subsequent phagocytosis by neutrophils (Kobayashi et al., 2001; Guentsch et al., 2011; Vincents et al., 2011). Therefore, lysine-specific gingipain suppresses IgG-mediated opsonization and P. gingivalis phagocytosis, which contributes to pathogen persistence.
Gingipains can also influence the host innate immune response by a manipulation of signaling dependent on the TREM (Triggering Receptor Expressed on Myeloid cells) or PAR (Protease-Activated Receptors) families of cell receptors. TREM-1 receptor belongs to the immunoglobulin family and is involved in the amplification of pro-inflammatory cytokines production as well as regulation of apoptosis. It was reported that gingipains increased the expression of TREM-1 on neutrophils, which resulted in an elevated production of IL-8. Interestingly, depending on gingipain specificity, authors noted opposing biological effects. Arg-specific gingipains were capable of shedding a soluble TREM-1 (sTREM-1) from the PMNs surface, while Lys-specific gingipains were degrading TREM-1 (Bostanci and Belibasakis, 2012a; Bostanci et al., 2013).
Gingipains were also shown to deregulate PAR signaling in neutrophils, gingival fibroblasts, epithelial cells, and T lymphocytes. In particular, Rgps were able to activate PAR2, a G-protein-coupled transmembrane receptor expressed, among others, on neutrophils. Rgps-mediated PAR2 activation enhanced the biosynthesis of prostaglandin E2, IFN-ɤ, IL-1β, and IL-6, which led to accelerated alveolar bone loss in experimentally-induced periodontitis in mice. Therefore, induction of PAR expression by gingipains is designed to sustain inflammation in P. gingivalis-infected periodontium (Lourbakos et al., 1998, 2001; Bostanci and Belibasakis, 2012b). Importantly, authors also reported an elevated expression of PAR2 on neutrophils from periodontitis patients, which indicated that deregulated PAR2 expression may contribute to periodontal inflammation severity (Holzhausen et al., 2006, 2010). Therefore, differential regulation of TREM-1 or PAR2 signaling by gingipains is designed to manipulate the host innate immune responses and contributes to chronic periodontal inflammation.
Additionally, gingipains were also shown to exploit IL-8 signaling in order to sustain inflammation, deregulate neutrophil chemotaxis and eventually inhibit bacterial elimination. Interleukin 8 (IL-8) is a chemokine known as a neutrophil chemotactic factor orchestrating many PMNs bactericidal activities, such as phagocytosis, respiratory burst, production of anti-bacterial enzymes, and pro-inflammatory cytokines. P. gingivalis LPS is able to induce the secretion of IL-872aa and IL-877aa variants from immune and non-immune cells, respectively. While the first variant is very potent in priming neutrophils, the latter needs to be truncated on the N-terminus to elicit full activity. This functional and structural diversity of IL-8 is exploited by P. gingivalis, which can manipulate IL-8 signaling depending on the stage of infection, gingipain concentration as well as the proximity of the cytokine to the bacterial plaque (Figure 3). Specifically, Arg- or Lys-gingipains can diminish IL-872aa activity and inhibit neutrophil chemotaxis as well as ROS production induced by fMLP during initial stages of infection, thus significantly contributing to a local chemokine paralysis. On the contrary, during later stages of P. gingivalis infection, the limited proteolysis of IL-877aa by R- and K-gingipains in the presence of fMLP resulted in the generation of truncated hyperactive IL-869aa variant, which enhanced PMNs recruitment and oxygen-dependent bacterial elimination (Darveau et al., 1998; Dias et al., 2008). Additionally, it was shown that enzymes associated with the bacterial outer-membrane blebs (vesicles) degrade and inactivate this chemokine, whereas soluble gingipains were able to process IL-877aa into the hyper-active variant (Mikolajczyk-Pawlinska et al., 1998). Of note, Arg-specific gingipains interfere also with TNF-α signaling by degrading the soluble as well as membrane-bound forms of this cytokine (Mezyk-Kopec et al., 2005), which attenuated host immune response and neutrophil recruitment, both especially important during initial stages of P. gingivalis infection.
Also, various complement components strongly regulate PMNs activity as these cells constitutively express several complement receptors, such as CR1 (CD35), CR3 (CD11b/CD18, Mac-1), CR4 (CD11c/CD18, Mac-1), or CR5 receptors. Engagement of complement receptors activates many different signaling pathways and triggers neutrophil migration, phagocytosis, degranulation, intracellular killing, or ROS production (Krauss et al., 2010; Hajishengallis et al., 2015b). Not surprisingly, another strategy of immune evasion employed by P. gingivalis is the inactivation of complement factors (Figure 3). This periodontopathogen produces high quantities of gingipains targeting these fundamental complement-mediated innate immune responses (Popadiak et al., 2007). In particular, RgpA can precisely cleave C3 and C5 to produce active C3b and C5a, the latter being an anaphilatixin, strongly promoting neutrophil recruitment to the gingiva (Wingrove et al., 1992). Actually, gingipains have been shown to have complex, “biphasic” effects on the complement system. At low concentrations of gingipains, which mimic early infection stages, they precisely cleave C3 and C5 and generate active C3a and C5a, respectively. In turn, at high concentrations or at deeper gingival localization, where the biofilm resides, they inhibit the complement pathway. This indicates that at the beginning of bacterial invasion, gingipains enhance inflammation in order to increase nutrients supply, while at advanced stages of periodontal disease they inactivate the complement cascade, which is designed to protect P. gingivalis and bystander bacteria from the bactericidal activity of the human serum and neutrophil killing (Popadiak et al., 2007; Potempa and Pike, 2009).
Moreover, Lys-specific gingipain was shown to block C5a-mediated anti-microbial activity by cleaving C5aR and abolishing myeloperoxidase release by neutrophils (Jagels et al., 1996a,b). Interestingly, membrane-bound RgpA can capture complement inhibitor C4BP on P. gingivalis surface, which abolishes membrane complement attack (MAC; Potempa et al., 2008). Furthermore, gingipains were also shown to interfere with the clotting cascade by degradation of fibrinogen. Especially Kgp increased bleeding tendency at the site of infection and prolonged inflammation (Imamura et al., 1995, 1997; Rapala-Kozik et al., 2011).
This data proved that P. gingivalis represents a periodontal pathogen, which employs different strategies to gain access to nutrient resources and overcome neutrophil-mediated killing. Not only endogenous host enzymes, but also gingipains mediate periodontal tissue breakdown. Moreover, gingipains, which are found at very high concentrations in periodontal pockets, deregulate the complement cascade, opsonization by immunoglobulins and receptor signaling, collectively leading to increased vascular permeability and bleeding. These effects increase the availability of hemin required for bacterial growth and facilitate the spreading of the pathogen. Depending on the stage of infection, position within the biofilm and specific circumstances, this periodontopathogen can either promote or prevent such activities as complement activation, neutrophil recruitment and inflammatory response. However, observed in vitro suppression of inflammatory responses, which manipulate neutrophils infiltration to the periodontium at the very early stages of P. gingivalis colonization was not observed in vivo during analysis of mouse models of periodontitis. However, it is difficult to establish in vivo relevant in vitro systems as either cell lines or isolated primary neutrophils are separated from their normal environment. On one hand, in vitro approaches allow to study molecular mechanisms in a very precise and controlled manner. On the other hand, in vitro work simplifies the system under study. Therefore, conclusions based on in vitro approaches or mouse models will be ultimately verified by clinical trials involving periodontitis patients.
Collectively, this compartmentalization of pro- and anti-inflammatory responses protects P. gingivalis from elimination and fuels the inflammation in distal positions from bacterial plaque, which clearly contributes to inflammatory tissue damage observed in patients suffering from the periodontal disease.
PMNs Deregulation by RBR
P. gingivalis expresses a non-haem iron protein called rubrerythrin (Rbr) in order to avoid oxidative stress and gain protection from reactive oxygen and nitrogen species. Rbr-positive P. gingivalis was shown to be more resistant to neutrophil killing, which enabled colonization of the periodontal tissues. Moreover, this virulence factor increased the mobilization and activation of neutrophils which was indispensable for the establishment of inflammation and contributed to greater tissue damage in vivo (Mydel et al., 2006). These results suggest that the host respiratory burst is manipulated by rubrerythrin in order to provoke inflammation which, paradoxically, promotes P. gingivalis survival.
PMNs Deregulation by PPAD
P. gingivalis peptidyl arginine deiminase (PPAD) is another enzyme unique to P. gingivalis, which converts proteins Arg residues, usually present at the carboxy terminus, into citrulline (McGraw et al., 1999). This modification is implicated in the development of rheumatoid arthritis. However, it was also shown that C5a citrullination reduced the chemotactic and proinflammatory potential of this anaphylatoxin (Maresz et al., 2013; Bielecka et al., 2014; Koziel et al., 2014a). Therefore, modifications of host proteins by PPAD represent another manipulative strategy designed to protect P. gingivalis and bystander bacteria from neutrophil-mediated killing.
PMNs Deregulation by Fimbriae
Fimbriae, also called pili, are proteinaceous appendages on the bacterial outer surface, which promote both adhesion to and invasion of the host cells (Enersen et al., 2013). In order to colonize the subgingival region, P. gingivalis utilizes two types of fimbria, namely long, composed of the FimA protein, and short, or minor, which consists of the Mfa protein. However, fimbriae do not only facilitate bacterial invasion, but also manipulate neutrophil responses. Challenge of PMNs with FimA, a main structural protein of the P. gingivalis long fimbriae, resulted in an increased release of the pro-inflammatory cytokine IL-8 and enhanced fibrinogen binding, which could be further augmented by additional stimulation with fMLP (Sahingur et al., 2006). Detailed analysis of neutrophil signaling revealed that P. gingivalis fimbriae activated TLR2 signaling by employing CD14 and PI3K. This, in turn, induced activation in neutrophils of CD11b-CD18 (also called Mac-1 or CR3) integrins (Harokopakis and Hajishengallis, 2005), which are the most abundant integrin type. Usually, Mac-1 engagement trigger inside-out signaling, resulting in inflammatory and antibacterial responses, such as leukocyte adhesion, transmigration, production of inflammatory cytokines, and phagocytosis (Ehlers, 2000). However, activation of CR3 by P. gingivalis was reported to facilitate the invasion and colonization of macrophages, which subsequently served as an intracellular bacterial reservoir. Therefore, it would be interesting to investigate whether similar mechanisms are employed to corrupt PMNs. Collectively, already published results undoubtedly show that P. gingivalis fimbriae strongly manipulate neutrophil signaling in order to prolong a nutritionally favorable inflammatory response and promote bacterial persistence.
Conclusions and Future Directions
Neutrophil homeostasis in the periodontium is ensured by a balance between neutrophil migration to the site of infection, anti-bacterial and pro-inflammatory response, and finally the clearance of apoptotic PMNs during the resolution of inflammation. However, excessive neutrophil recruitment to the periodontium and their hyper-activation arises as a novel mechanism strongly contributing to the development and progression of periodontal disease. Different P. gingivalis virulence factors corrupt many neutrophil functions in order to sustain inflammation, gain access to nutrient resources and ensure protection from killing. This suggests that neutrophil contribution to the progression of periodontitis might not only be related to defective elimination of bacteria, but additionally involve a deregulation of immune tolerance, neutrophil apoptosis and mechanisms driving resolution of inflammation. However, depending on the specific circumstances and the stage of bacterial infection, the manipulation of PMNs might actually lead to opposing effects, either pro- or anti-inflammatory. Therefore, future research might discover the link between regulatory defects in anti-bacterial or effector neutrophil functions and the progression of chronic periodontal disease. A better molecular understanding of periodontitis-associated neutrophil dysfunctions due to their manipulation by P. gingivalis could be used as a potential targeted therapy in patients.
Author Contributions
MS wrote the manuscript, prepared figures, and the table; JP corrected the manuscript.
Funding
This work was supported by funding grants Foundation for Polish Science (Homing/2016-1/9) to MS, US NIH/NIDCR (DE 022597 and DE 023207), and National Science Center (2012/04/A/NZ1/00051, NCN, Krakow, Poland) to JP.
Conflict of Interest Statement
The authors declare that the research was conducted in the absence of any commercial or financial relationships that could be construed as a potential conflict of interest.
Acknowledgments
We would like to acknowledge Professor dr hab. Andreas Villunger from the Department of Developmental Immunology, Medical University of Innsbruck, Austria and Tyrolean Cancer Research Institute, Austria for the critical review of this manuscript. Additionally, we acknowledge that Faculty of Biochemistry, Biophysics and Biotechnology of Jagiellonian University is a partner of the Leading National Research Center (KNOW) supported by the Ministry of Science and Higher Education. The authors acknowledge the financial support from the Foundation of Polish Science grant HOMING. The HOMING programme is co-financed by the European Union under the European Regional Development Fund.
References
Aboodi, G. M., Goldberg, M. B., and Glogauer, M. (2011). Refractory periodontitis population characterized by a hyperactive oral neutrophil phenotype. J. Periodontol. 82, 726–733. doi: 10.1902/jop.2010.100508
Adamowicz, K., Wang, H., Jotwani, R., Zeller, I., Potempa, J., and Scott, D. A. (2012). Inhibition of GSK3 abolishes bacterial-induced periodontal bone loss in mice. Mol. Med. 18, 1190–1196. doi: 10.2119/molmed.2012.00180
Al-Qutub, M. N., Braham, P. H., Karimi-Naser, L. M., Liu, X., Genco, C. A., and Darveau, R. P. (2006). Hemin-dependent modulation of the lipid A structure of Porphyromonas gingivalis lipopolysaccharide. Infect. Immun. 74, 4474–4485. doi: 10.1128/IAI.01924-05
Arango Duque, G., and Descoteaux, A. (2014). Macrophage cytokines: involvement in immunity and infectious diseases. Front. Immunol. 5:491. doi: 10.3389/fimmu.2014.00491
Bainbridge, B. W., and Darveau, R. P. (2001). Porphyromonas gingivalis lipopolysaccharide: an unusual pattern recognition receptor ligand for the innate host defense system. Acta Odontol. Scand. 59, 131–138. doi: 10.1080/000163501750266710
Bainbridge, B. W., Coats, S. R., Pham, T. T., Reife, R. A., and Darveau, R. P. (2006). Expression of a Porphyromonas gingivalis lipid A palmitylacyltransferase in Escherichia coli yields a chimeric lipid A with altered ability to stimulate interleukin-8 secretion. Cell. Microbiol. 8, 120–129. doi: 10.1111/j.1462-5822.2005.00605.x
Bainbridge, B., Verma, R. K., Eastman, C., Yehia, B., Rivera, M., Moffatt, C., et al. (2010). Role of Porphyromonas gingivalis phosphoserine phosphatase enzyme SerB in inflammation, immune response, and induction of alveolar bone resorption in rats. Infect. Immun. 78, 4560–4569. doi: 10.1128/IAI.00703-10
Baker, P. J., Dixon, M., Evans, R. T., Dufour, L., Johnson, E., and Roopenian, D. C. (1999). CD4(+) T cells and the proinflammatory cytokines gamma interferon and interleukin-6 contribute to alveolar bone loss in mice. Infect. Immun. 67, 2804–2809.
Bender, J. S., Thang, H., and Glogauer, M. (2006). Novel rinse assay for the quantification of oral neutrophils and the monitoring of chronic periodontal disease. J. Periodont. Res. 41, 214–220. doi: 10.1111/j.1600-0765.2005.00861.x
Berezow, A. B., Ernst, R. K., Coats, S. R., Braham, P. H., Karimi-Naser, L. M., and Darveau, R. P. (2009). The structurally similar, penta-acylated lipopolysaccharides of Porphyromonas gingivalis and Bacteroides elicit strikingly different innate immune responses. Microb. Pathog. 47, 68–77. doi: 10.1016/j.micpath.2009.04.015
Bielecka, E., Scavenius, C., Kantyka, T., Jusko, M., Mizgalska, D., Szmigielski, B., et al. (2014). Peptidyl arginine deiminase from Porphyromonas gingivalis abolishes anaphylatoxin C5a activity. J. Biol. Chem. 289, 32481–32487. doi: 10.1074/jbc.C114.617142
Borregaard, N. (2010). Neutrophils, from marrow to microbes. Immunity 33, 657–670. doi: 10.1016/j.immuni.2010.11.011
Borregaard, N., and Herlin, T. (1982). Energy metabolism of human neutrophils during phagocytosis. J. Clin. Invest. 70, 550–557. doi: 10.1172/JCI110647
Bostanci, N., and Belibasakis, G. N. (2012a). Doxycycline inhibits TREM-1 induction by Porphyromonas gingivalis. FEMS Immunol. Med. Microbiol. 66, 37–44. doi: 10.1111/j.1574-695X.2012.00982.x
Bostanci, N., and Belibasakis, G. N. (2012b). Porphyromonas gingivalis: an invasive and evasive opportunistic oral pathogen. FEMS Microbiol. Lett. 333, 1–9. doi: 10.1111/j.1574-695X.2012.00982.x
Bostanci, N., Thurnheer, T., Aduse-Opoku, J., Curtis, M. A., Zinkernagel, A. S., and Belibasakis, G. N. (2013). Porphyromonas gingivalis regulates TREM-1 in human polymorphonuclear neutrophils via its gingipains. PLoS ONE 8:e75784. doi: 10.1371/journal.pone.0075784
Bratton, D. L., and Henson, P. M. (2011). Neutrophil clearance: when the party is over, clean-up begins. Trends Immunol. 32, 350–357. doi: 10.1016/j.it.2011.04.009
Chapple, I. L., and Matthews, J. B. (2007). The role of reactive oxygen and antioxidant species in periodontal tissue destruction. Periodontol. 2000 43, 160–232. doi: 10.1111/j.1600-0757.2006.00178.x
Cooper, P. R., Palmer, L. J., and Chapple, I. L. (2013). Neutrophil extracellular traps as a new paradigm in innate immunity: friend or foe? Periodontol. 2000 63, 165–197. doi: 10.1111/prd.12025
Czabotar, P. E., Lessene, G., Strasser, A., and Adams, J. M. (2014). Control of apoptosis by the BCL-2 protein family: implications for physiology and therapy. Nat. Rev. Mol. Cell Biol. 15, 49–63. doi: 10.1038/nrm3722
Damgaard, C., Kantarci, A., Holmstrup, P., Hasturk, H., Nielsen, C. H., and Van Dyke, T. E. (2017). Porphyromonas gingivalis-induced production of reactive oxygen species, tumor necrosis factor-alpha, interleukin-6, CXCL8 and CCL2 by neutrophils from localized aggressive periodontitis and healthy donors: modulating actions of red blood cells and resolvin E1. J. Periodontal. Res. 52, 246–254. doi: 10.1111/jre.12388
Darveau, R. P., Belton, C. M., Reife, R. A., and Lamont, R. J. (1998). Local chemokine paralysis, a novel pathogenic mechanism for Porphyromonas gingivalis. Infect. Immun. 66, 1660–1665.
Darveau, R. P., Hajishengallis, G., and Curtis, M. A. (2012). Porphyromonas gingivalis as a potential community activist for disease. J. Dent. Res. 91, 816–820. doi: 10.1177/0022034512453589
Darveau, R. P., Pham, T. T., Lemley, K., Reife, R. A., Bainbridge, B. W., Coats, S. R., et al. (2004). Porphyromonas gingivalis lipopolysaccharide contains multiple lipid A species that functionally interact with both toll-like receptors 2 and 4. Infect. Immun. 72, 5041–5051. doi: 10.1128/IAI.72.9.5041-5051.2004
de Molon, R. S., de Avila, E. D., and Cirelli, J. A. (2013). Host responses induced by different animal models of periodontal disease: a literature review. J. Investig. Clin. Dent. 4, 211–218. doi: 10.1111/jicd.12018
de Molon, R. S., de Avila, E. D., Boas Nogueira, A. V., Chaves de Souza, J. A., Avila-Campos, M. J., de Andrade, C. R., et al. (2014). Evaluation of the host response in various models of induced periodontal disease in mice. J. Periodontol. 85, 465–477. doi: 10.1902/jop.2013.130225
Deleon-Pennell, K. Y., de Castro Bras, L. E., and Lindsey, M. L. (2013). Circulating Porphyromonas gingivalis lipopolysaccharide resets cardiac homeostasis in mice through a matrix metalloproteinase-9-dependent mechanism. Physiol. Rep. 1:e00079. doi: 10.1002/phy2.79
Dias, I. H., Marshall, L., Lambert, P. A., Chapple, I. L., Matthews, J. B., and Griffiths, H. R. (2008). Gingipains from Porphyromonas gingivalis increase the chemotactic and respiratory burst-priming properties of the 77-amino-acid interleukin-8 variant. Infect. Immun. 76, 317–323. doi: 10.1128/IAI.00618-07
Dias, I. H., Matthews, J. B., Chapple, I. L., Wright, H. J., Dunston, C. R., and Griffiths, H. R. (2011). Activation of the neutrophil respiratory burst by plasma from periodontitis patients is mediated by pro-inflammatory cytokines. J. Clin. Periodontol. 38, 1–7. doi: 10.1111/j.1600-051X.2010.01628.x
Dixon, D. R., and Darveau, R. P. (2005). Lipopolysaccharide heterogeneity: innate host responses to bacterial modification of lipid a structure. J. Dent. Res. 84, 584–595. doi: 10.1177/154405910508400702
Ehlers, M. R. (2000). CR3: a general purpose adhesion-recognition receptor essential for innate immunity. Microbes Infect. 2, 289–294. doi: 10.1016/S1286-4579(00)00299-9
Eick, S., Puklo, M., Adamowicz, K., Kantyka, T., Hiemstra, P., Stennicke, H., et al. (2014). Lack of cathelicidin processing in Papillon-Lefevre syndrome patients reveals essential role of LL-37 in periodontal homeostasis. Orphanet J. Rare Dis. 9:148. doi: 10.1186/s13023-014-0148-y
Enersen, M., Nakano, K., and Amano, A. (2013). Porphyromonas gingivalis fimbriae. J. Oral Microbiol. 5:20265. doi: 10.3402/jom.v5i0.20265
Eskan, M. A., Jotwani, R., Abe, T., Chmelar, J., Lim, J. H., Liang, S., et al. (2012). The leukocyte integrin antagonist Del-1 inhibits IL-17-mediated inflammatory bone loss. Nat. Immunol. 13, 465–473. doi: 10.1038/ni.2260
Gamonal, J., Sanz, M., O'Connor, A., Acevedo, A., Suarez, I., Sanz, A., et al. (2003). Delayed neutrophil apoptosis in chronic periodontitis patients. J. Clin. Periodontol. 30, 616–623. doi: 10.1034/j.1600-051X.2003.00350.x
Garcia Saez, A. J., and Villunger, A. (2016). MOMP in the absence of BH3-only proteins. Genes Dev. 30, 878–880. doi: 10.1101/gad.281519.116
Gifford, A. M., and Chalmers, J. D. (2014). The role of neutrophils in cystic fibrosis. Curr. Opin. Hematol. 21, 16–22. doi: 10.1097/MOH.0000000000000009
Glowacki, A. J., Yoshizawa, S., Jhunjhunwala, S., Vieira, A. E., Garlet, G. P., Sfeir, C., et al. (2013). Prevention of inflammation-mediated bone loss in murine and canine periodontal disease via recruitment of regulatory lymphocytes. Proc. Natl. Acad. Sci. U.S.A. 110, 18525–18530. doi: 10.1073/pnas.1302829110
Graves, D. T., Fine, D., Teng, Y. T., Van Dyke, T. E., and Hajishengallis, G. (2008). The use of rodent models to investigate host-bacteria interactions related to periodontal diseases. J. Clin. Periodontol. 35, 89–105. doi: 10.1111/j.1600-051X.2007.01172.x
Guentsch, A., Hirsch, C., Pfister, W., Vincents, B., Abrahamson, M., Sroka, A., et al. (2013). Cleavage of IgG1 in gingival crevicular fluid is associated with the presence of Porphyromonas gingivalis. J. Periodont. Res. 48, 458–465. doi: 10.1111/jre.12027
Guentsch, A., Kramesberger, M., Sroka, A., Pfister, W., Potempa, J., and Eick, S. (2011). Comparison of gingival crevicular fluid sampling methods in patients with severe chronic periodontitis. J. Periodontol. 82, 1051–1060. doi: 10.1902/jop.2011.100565
Guentsch, A., Puklo, M., Preshaw, P. M., Glockmann, E., Pfister, W., Potempa, J., et al. (2009). Neutrophils in chronic and aggressive periodontitis in interaction with Porphyromonas gingivalis and Aggregatibacter actinomycetemcomitans. J. Periodont. Res. 44, 368–377. doi: 10.1111/j.1600-0765.2008.01113.x
Guo, Y., Nguyen, K. A., and Potempa, J. (2010). Dichotomy of gingipains action as virulence factors: from cleaving substrates with the precision of a surgeon's knife to a meat chopper-like brutal degradation of proteins. Periodontol. 2000 54, 15–44. doi: 10.1111/j.1600-0757.2010.00377.x
Hajishengallis, E., and Hajishengallis, G. (2014). Neutrophil homeostasis and periodontal health in children and adults. J. Dent. Res. 93, 231–237. doi: 10.1177/0022034513507956
Hajishengallis, G. (2014). Immunomicrobial pathogenesis of periodontitis: keystones, pathobionts, and host response. Trends Immunol. 35, 3–11. doi: 10.1016/j.it.2013.09.001
Hajishengallis, G., and Lambris, J. D. (2011). Microbial manipulation of receptor crosstalk in innate immunity. Nat. Rev. Immunol. 11, 187–200. doi: 10.1038/nri2918
Hajishengallis, G., and Lambris, J. D. (2013). Complement-targeted therapeutics in periodontitis. Adv. Exp. Med. Biol. 735, 197–206. doi: 10.1007/978-1-4614-4118-2_13
Hajishengallis, G., and Lamont, R. J. (2012). Beyond the red complex and into more complexity: the polymicrobial synergy and dysbiosis (PSD) model of periodontal disease etiology. Mol. Oral Microbiol. 27, 409–419. doi: 10.1111/j.2041-1014.2012.00663.x
Hajishengallis, G., and Lamont, R. J. (2016). Dancing with the stars: how choreographed bacterial interactions dictate nososymbiocity and give rise to keystone pathogens, accessory pathogens, and pathobionts. Trends Microbiol. 24, 477–489. doi: 10.1016/j.tim.2016.02.010
Hajishengallis, G., Chavakis, T., Hajishengallis, E., and Lambris, J. D. (2015a). Neutrophil homeostasis and inflammation: novel paradigms from studying periodontitis. J. Leukoc. Biol. 98, 539–548. doi: 10.1189/jlb.3VMR1014-468R
Hajishengallis, G., Maekawa, T., Abe, T., Hajishengallis, E., and Lambris, J. D. (2015b). Complement involvement in periodontitis: molecular mechanisms and rational therapeutic approaches. Adv. Exp. Med. Biol. 865, 57–74. doi: 10.1007/978-3-319-18603-0_4
Hajishengallis, G., Wang, M., Bagby, G. J., and Nelson, S. (2008a). Importance of TLR2 in early innate immune response to acute pulmonary infection with Porphyromonas gingivalis in mice. J. Immunol. 181, 4141–4149. doi: 10.4049/jimmunol.181.6.4141
Hajishengallis, G., Wang, M., Liang, S., Shakhatreh, M. A., James, D., Nishiyama, S., et al. (2008b). Subversion of innate immunity by periodontopathic bacteria via exploitation of complement receptor-3. Adv. Exp. Med. Biol. 632, 203–219. doi: 10.1007/978-0-387-78952-1_15
Harokopakis, E., and Hajishengallis, G. (2005). Integrin activation by bacterial fimbriae through a pathway involving CD14, Toll-like receptor 2, and phosphatidylinositol-3-kinase. Eur. J. Immunol. 35, 1201–1210. doi: 10.1002/eji.200425883
Hatanaka, E., Monteagudo, P. T., Marrocos, M. S., and Campa, A. (2006). Neutrophils and monocytes as potentially important sources of proinflammatory cytokines in diabetes. Clin. Exp. Immunol. 146, 443–447. doi: 10.1111/j.1365-2249.2006.03229.x
Hiroi, M., Shimojima, T., Kashimata, M., Miyata, T., Takano, H., Takahama, M., et al. (1998). Inhibition by Porphyromonas gingivalis LPS of apoptosis induction in human peripheral blood polymorphonuclear leukocytes. Anticancer Res. 18, 3475–3479.
Holzhausen, M., Cortelli, J. R., da Silva, V. A., Franco, G. C., Cortelli, S. C., and Vergnolle, N. (2010). Protease-activated receptor-2 (PAR(2)) in human periodontitis. J. Dent. Res. 89, 948–953. doi: 10.1177/0022034510373765
Holzhausen, M., Spolidorio, L. C., Ellen, R. P., Jobin, M. C., Steinhoff, M., Andrade-Gordon, P., et al. (2006). Protease-activated receptor-2 activation: a major role in the pathogenesis of Porphyromonas gingivalis infection. Am. J. Pathol. 168, 1189–1199. doi: 10.2353/ajpath.2006.050658
Houle, M. A., Grenier, D., Plamondon, P., and Nakayama, K. (2003). The collagenase activity of Porphyromonas gingivalis is due to Arg-gingipain. FEMS Microbiol. Lett. 221, 181–185. doi: 10.1016/S0378-1097(03)00178-2
Imamura, T., Potempa, J., Pike, R. N., Moore, J. N., Barton, M. H., and Travis, J. (1995). Effect of free and vesicle-bound cysteine proteinases of Porphyromonas gingivalis on plasma clot formation: implications for bleeding tendency at periodontitis sites. Infect. Immun. 63, 4877–4882.
Imamura, T., Potempa, J., Tanase, S., and Travis, J. (1997). Activation of blood coagulation factor X by arginine-specific cysteine proteinases (gingipain-Rs) from Porphyromonas gingivalis. J. Biol. Chem. 272, 16062–16067. doi: 10.1074/jbc.272.25.16062
Jagels, M. A., Ember, J. A., Travis, J., Potempa, J., Pike, R., and Hugli, T. E. (1996a). Cleavage of the human C5A receptor by proteinases derived from Porphyromonas gingivalis: cleavage of leukocyte C5a receptor. Adv. Exp. Med. Biol. 389, 155–164. doi: 10.1007/978-1-4613-0335-0_19
Jagels, M. A., Travis, J., Potempa, J., Pike, R., and Hugli, T. E. (1996b). Proteolytic inactivation of the leukocyte C5a receptor by proteinases derived from Porphyromonas gingivalis. Infect. Immun. 64, 1984–1991.
Johnstone, A. M., Koh, A., Goldberg, M. B., and Glogauer, M. (2007). A hyperactive neutrophil phenotype in patients with refractory periodontitis. J. Periodontol. 78, 1788–1794. doi: 10.1902/jop.2007.070107
Kassebaum, N. J., Bernabe, E., Dahiya, M., Bhandari, B., Murray, C. J., and Marcenes, W. (2014). Global burden of severe periodontitis in 1990-2010: a systematic review and meta-regression. J. Dent. Res. 93, 1045–1053. doi: 10.1177/0022034514552491
Kobayashi, T., Yamamoto, K., Sugita, N., van Spriel, A. B., Kaneko, S., van de Winkel, J. G., et al. (2001). Effective in vitro clearance of Porphyromonas gingivalis by Fc alpha receptor I (CD89) on gingival crevicular neutrophils. Infect. Immun. 69, 2935–2942. doi: 10.1128/IAI.69.5.2935-2942.2001
Koedel, U., Frankenberg, T., Kirschnek, S., Obermaier, B., Hacker, H., Paul, R., et al. (2009). Apoptosis is essential for neutrophil functional shutdown and determines tissue damage in experimental pneumococcal meningitis. PLoS Pathog. 5:e1000461. doi: 10.1371/journal.ppat.1000461
Koziel, J., Bryzek, D., Sroka, A., Maresz, K., Glowczyk, I., Bielecka, E., et al. (2014a). Citrullination alters immunomodulatory function of LL-37 essential for prevention of endotoxin-induced sepsis. J. Immunol. 192, 5363–5372. doi: 10.4049/jimmunol.1303062
Koziel, J., Mydel, P., and Potempa, J. (2014b). The link between periodontal disease and rheumatoid arthritis: an updated review. Curr. Rheumatol. Rep. 16:408. doi: 10.1007/s11926-014-0408-9
Krauss, J. L., Potempa, J., Lambris, J. D., and Hajishengallis, G. (2010). Complementary Tolls in the periodontium: how periodontal bacteria modify complement and Toll-like receptor responses to prevail in the host. Periodontol. 2000 52, 141–162. doi: 10.1111/j.1600-0757.2009.00324.x
Kumada, H., Haishima, Y., Umemoto, T., and Tanamoto, K. (1995). Structural study on the free lipid A isolated from lipopolysaccharide of Porphyromonas gingivalis. J. Bacteriol. 177, 2098–2106. doi: 10.1128/jb.177.8.2098-2106.1995
Lakschevitz, F. S., Aboodi, G. M., and Glogauer, M. (2013). Oral neutrophil transcriptome changes result in a pro-survival phenotype in periodontal diseases. PLoS ONE 8:e68983. doi: 10.1371/journal.pone.0068983
Levine, A. P., and Segal, A. W. (2013). What is wrong with granulocytes in inflammatory bowel diseases? Dig. Dis. 31, 321–327. doi: 10.1159/000354686
Liang, S., Krauss, J. L., Domon, H., McIntosh, M. L., Hosur, K. B., Qu, H., et al. (2011). The C5a receptor impairs IL-12-dependent clearance of Porphyromonas gingivalis and is required for induction of periodontal bone loss. J. Immunol. 186, 869–877. doi: 10.4049/jimmunol.1003252
Ling, M. R., Chapple, I. L., and Matthews, J. B. (2015). Peripheral blood neutrophil cytokine hyper-reactivity in chronic periodontitis. Innate Immun. 21, 714–725. doi: 10.1177/1753425915589387
Loesche, W. J., Robinson, J. P., Flynn, M., Hudson, J. L., and Duque, R. E. (1988). Reduced oxidative function in gingival crevicular neutrophils in periodontal disease. Infect. Immun. 56, 156–160.
Lourbakos, A., Chinni, C., Thompson, P., Potempa, J., Travis, J., Mackie, E. J., et al. (1998). Cleavage and activation of proteinase-activated receptor-2 on human neutrophils by gingipain-R from Porphyromonas gingivalis. FEBS Lett. 435, 45–48. doi: 10.1016/S0014-5793(98)01036-9
Lourbakos, A., Potempa, J., Travis, J., D'Andrea, M. R., Andrade-Gordon, P., Santulli, R., et al. (2001). Arginine-specific protease from Porphyromonas gingivalis activates protease-activated receptors on human oral epithelial cells and induces interleukin-6 secretion. Infect. Immun. 69, 5121–5130. doi: 10.1128/IAI.69.8.5121-5130.2001
Madianos, P. N., Papapanou, P. N., and Sandros, J. (1997). Porphyromonas gingivalis infection of oral epithelium inhibits neutrophil transepithelial migration. Infect. Immun. 65, 3983–3990.
Maekawa, T., Krauss, J. L., Abe, T., Jotwani, R., Triantafilou, M., Triantafilou, K., et al. (2014). Porphyromonas gingivalis manipulates complement and TLR signaling to uncouple bacterial clearance from inflammation and promote dysbiosis. Cell Host Microbe 15, 768–778. doi: 10.1016/j.chom.2014.05.012
Maresz, K. J., Hellvard, A., Sroka, A., Adamowicz, K., Bielecka, E., Koziel, J., et al. (2013). Porphyromonas gingivalis facilitates the development and progression of destructive arthritis through its unique bacterial peptidylarginine deiminase (PAD). PLoS Pathog. 9:e1003627. doi: 10.1371/journal.ppat.1003627
Mariano, F. S., Campanelli, A. P., Nociti, F. H. Jr., Mattos-Graner, R. O., and Goncalves, R. B. (2012). Antimicrobial peptides and nitric oxide production by neutrophils from periodontitis subjects. Braz. J. Med. Biol. Res. 45, 1017–1024. doi: 10.1590/S0100-879X2012007500123
Marsh, P. D. (1994). Microbial ecology of dental plaque and its significance in health and disease. Adv. Dent. Res. 8, 263–271. doi: 10.1177/08959374940080022001
Marsh, P. D. (2003). Are dental diseases examples of ecological catastrophes? Microbiology 149(Pt 2), 279–294. doi: 10.1099/mic.0.26082-0
Matthews, J. B., Wright, H. J., Roberts, A., Cooper, P. R., and Chapple, I. L. (2007). Hyperactivity and reactivity of peripheral blood neutrophils in chronic periodontitis. Clin. Exp. Immunol. 147, 255–264. doi: 10.1111/j.1365-2249.2006.03276.x
McCracken, J. M., and Allen, L. A. (2014). Regulation of human neutrophil apoptosis and lifespan in health and disease. J. Cell Death 7, 15–23. doi: 10.4137/JCD.S11038
McGraw, W. T., Potempa, J., Farley, D., and Travis, J. (1999). Purification, characterization, and sequence analysis of a potential virulence factor from Porphyromonas gingivalis, peptidylarginine deiminase. Infect. Immun. 67, 3248–3256.
Mezyk-Kopec, R., Bzowska, M., Potempa, J., Bzowska, M., Jura, N., Sroka, A., et al. (2005). Inactivation of membrane tumor necrosis factor alpha by gingipains from Porphyromonas gingivalis. Infect. Immun. 73, 1506–1514. doi: 10.1128/IAI.73.3.1506-1514.2005
Mikolajczyk-Pawlinska, J., Travis, J., and Potempa, J. (1998). Modulation of interleukin-8 activity by gingipains from Porphyromonas gingivalis: implications for pathogenicity of periodontal disease. FEBS Lett. 440, 282–286. doi: 10.1016/S0014-5793(98)01461-6
Moutsopoulos, N. M., Kling, H. M., Angelov, N., Jin, W., Palmer, R. J., Nares, S., et al. (2012). Porphyromonas gingivalis promotes Th17 inducing pathways in chronic periodontitis. J. Autoimmun. 39, 294–303. doi: 10.1016/j.jaut.2012.03.003
Moutsopoulos, N. M., Konkel, J., Sarmadi, M., Eskan, M. A., Wild, T., Dutzan, N., et al. (2014). Defective neutrophil recruitment in leukocyte adhesion deficiency type I disease causes local IL-17-driven inflammatory bone loss. Sci. Transl. Med. 6:229ra240. doi: 10.1126/scitranslmed.3007696
Murdock, J. L., and Nunez, G. (2016). TLR4: the winding road to the discovery of the LPS receptor. J. Immunol. 197, 2561–2562. doi: 10.4049/jimmunol.1601400
Murray, D. A., and Wilton, J. M. (2003). Lipopolysaccharide from the periodontal pathogen Porphyromonas gingivalis prevents apoptosis of HL60-derived neutrophils in vitro. Infect. Immun. 71, 7232–7235. doi: 10.1128/IAI.71.12.7232-7235.2003
Mydel, P., Takahashi, Y., Yumoto, H., Sztukowska, M., Kubica, M., Gibson, F. C. I. I. I., et al. (2006). Roles of the host oxidative immune response and bacterial antioxidant rubrerythrin during Porphyromonas gingivalis infection. PLoS Pathog. 2:e76. doi: 10.1371/journal.ppat.0020076
Nathan, C. (2006). Neutrophils and immunity: challenges and opportunities. Nat. Rev. Immunol. 6, 173–182. doi: 10.1038/nri1785
Nauseef, W. M. (2007). How human neutrophils kill and degrade microbes: an integrated view. Immunol. Rev. 219, 88–102. doi: 10.1111/j.1600-065X.2007.00550.x
Olsen, I., and Hajishengallis, G. (2016). Major neutrophil functions subverted by Porphyromonas gingivalis. J. Oral Microbiol. 8:30936. doi: 10.3402/jom.v8.30936
Popadiak, K., Potempa, J., Riesbeck, K., and Blom, A. M. (2007). Biphasic effect of gingipains from Porphyromonas gingivalis on the human complement system. J. Immunol. 178, 7242–7250. doi: 10.4049/jimmunol.178.11.7242
Potempa, J., and Pike, R. N. (2009). Corruption of innate immunity by bacterial proteases. J. Innate Immun. 1, 70–87. doi: 10.1159/000181144
Potempa, J., Pike, R., and Travis, J. (1997). Titration and mapping of the active site of cysteine proteinases from Porphyromonas gingivalis (gingipains) using peptidyl chloromethanes. Biol. Chem. 378, 223–230. doi: 10.1515/bchm.1997.378.3-4.223
Potempa, J., Sroka, A., Imamura, T., and Travis, J. (2003). Gingipains, the major cysteine proteinases and virulence factors of Porphyromonas gingivalis: structure, function and assembly of multidomain protein complexes. Curr. Protein Pept. Sci. 4, 397–407. doi: 10.2174/1389203033487036
Potempa, M., Potempa, J., Okroj, M., Popadiak, K., Eick, S., Nguyen, K. A., et al. (2008). Binding of complement inhibitor C4b-binding protein contributes to serum resistance of Porphyromonas gingivalis. J. Immunol. 181, 5537–5544. doi: 10.4049/jimmunol.181.8.5537
Prat, L., Bouaziz, J. D., Wallach, D., Vignon-Pennamen, M. D., and Bagot, M. (2014). Neutrophilic dermatoses as systemic diseases. Clin. Dermatol. 32, 376–388. doi: 10.1016/j.clindermatol.2013.11.004
Preshaw, P. M., Schifferle, R. E., and Walters, J. D. (1999). Porphyromonas gingivalis lipopolysaccharide delays human polymorphonuclear leukocyte apoptosis in vitro. J. Periodont. Res. 34, 197–202. doi: 10.1111/j.1600-0765.1999.tb02242.x
Raetz, C. R., Guan, Z., Ingram, B. O., Six, D. A., Song, F., Wang, X., et al. (2009). Discovery of new biosynthetic pathways: the lipid A story. J. Lipid Res. 50(Suppl.), S103–S108. doi: 10.1194/jlr.R800060-JLR200
Raetz, C. R., Reynolds, C. M., Trent, M. S., and Bishop, R. E. (2007). Lipid A modification systems in gram-negative bacteria. Annu. Rev. Biochem. 76, 295–329. doi: 10.1146/annurev.biochem.76.010307.145803
Rangarajan, M., Aduse-Opoku, J., Paramonov, N., Hashim, A., Bostanci, N., Fraser, O. P., et al. (2008). Identification of a second lipopolysaccharide in Porphyromonas gingivalis W50. J. Bacteriol. 190, 2920–2932. doi: 10.1128/JB.01868-07
Rapala-Kozik, M., Bras, G., Chruscicka, B., Karkowska-Kuleta, J., Sroka, A., Herwald, H., et al. (2011). Adsorption of components of the plasma kinin-forming system on the surface of Porphyromonas gingivalis involves gingipains as the major docking platforms. Infect. Immun. 79, 797–805. doi: 10.1128/IAI.00966-10
Reife, R. A., Coats, S. R., Al-Qutub, M., Dixon, D. M., Braham, P. A., Billharz, R. J., et al. (2006). Porphyromonas gingivalis lipopolysaccharide lipid A heterogeneity: differential activities of tetra- and penta-acylated lipid A structures on E-selectin expression and TLR4 recognition. Cell. Microbiol. 8, 857–868. doi: 10.1111/j.1462-5822.2005.00672.x
Reynolds, J. J., and Meikle, M. C. (1997). Mechanisms of connective tissue matrix destruction in periodontitis. Periodontol. 2000 14, 144–157. doi: 10.1111/j.1600-0757.1997.tb00195.x
Ryder, M. I. (2010). Comparison of neutrophil functions in aggressive and chronic periodontitis. Periodontol. 2000 53, 124–137. doi: 10.1111/j.1600-0757.2009.00327.x
Saadi-Thiers, K., Huck, O., Simonis, P., Tilly, P., Fabre, J. E., Tenenbaum, H., et al. (2013). Periodontal and systemic responses in various mice models of experimental periodontitis: respective roles of inflammation duration and Porphyromonas gingivalis infection. J. Periodontol. 84, 396–406. doi: 10.1902/jop.2012.110540
Sahingur, S. E., Boehm, T. K., Sojar, H. T., Sharma, A., and De Nardin, E. (2006). Fibrinogen-neutrophil interactions in response to fMLP and Porphyromonas gingivalis fimbrial peptides. Immunol. Invest. 35, 63–74. doi: 10.1080/08820130500496811
Schwartz, J., Stinson, F. L., and Parker, R. B. (1972). The passage of tritiated bacterial endotoxin across intact gingival crevicular epithelium. J. Periodontol. 43, 270–276. doi: 10.1902/jop.1972.43.5.270
Scott, D. A., and Krauss, J. (2012). Neutrophils in periodontal inflammation. Front. Oral Biol. 15, 56–83. doi: 10.1159/000329672
Segal, A. W. (2005). How neutrophils kill microbes. Annu. Rev. Immunol. 23, 197–223. doi: 10.1146/annurev.immunol.23.021704.115653
Settem, R. P., Honma, K., and Sharma, A. (2014). Neutrophil mobilization by surface-glycan altered Th17-skewing bacteria mitigates periodontal pathogen persistence and associated alveolar bone loss. PLoS ONE 9:e108030. doi: 10.1371/journal.pone.0108030
Sochalska, M., Ottina, E., Tuzlak, S., Herzog, S., Herold, M., and Villunger, A. (2016). Conditional knockdown of BCL2A1 reveals rate-limiting roles in BCR-dependent B-cell survival. Cell Death Differ. 23, 628–639. doi: 10.1038/cdd.2015.130
Sochalska, M., Tuzlak, S., Egle, A., and Villunger, A. (2015). Lessons from gain- and loss-of-function models of pro-survival Bcl2 family proteins: implications for targeted therapy. FEBS J. 282, 834–849. doi: 10.1111/febs.13188
Socransky, S. S., Haffajee, A. D., Cugini, M. A., Smith, C., and Kent, R. L. Jr. (1998). Microbial complexes in subgingival plaque. J. Clin. Periodontol. 25, 134–144. doi: 10.1111/j.1600-051X.1998.tb02419.x
Soehnlein, O. (2009). Direct and alternative antimicrobial mechanisms of neutrophil-derived granule proteins. J. Mol. Med. 87, 1157–1164. doi: 10.1007/s00109-009-0508-6
Summers, C., Rankin, S. M., Condliffe, A. M., Singh, N., Peters, A. M., and Chilvers, E. R. (2010). Neutrophil kinetics in health and disease. Trends Immunol. 31, 318–324. doi: 10.1016/j.it.2010.05.006
Takeuchi, H., Hirano, T., Whitmore, S. E., Morisaki, I., Amano, A., and Lamont, R. J. (2013). The serine phosphatase SerB of Porphyromonas gingivalis suppresses IL-8 production by dephosphorylation of NF-kappaB RelA/p65. PLoS Pathog. 9:e1003326. doi: 10.1371/journal.ppat.1003326
Tuzlak, S., Kaufmann, T., and Villunger, A. (2016). Interrogating the relevance of mitochondrial apoptosis for vertebrate development and postnatal tissue homeostasis. Genes Dev. 30, 2133–2151. doi: 10.1101/gad.289298.116
Van Dyke, T. E., and Sheilesh, D. (2005). Risk factors for periodontitis. J. Int. Acad. Periodontol. 7, 3–7.
Vier, J., Groth, M., Sochalska, M., and Kirschnek, S. (2016). The anti-apoptotic Bcl-2 family protein A1/Bfl-1 regulates neutrophil survival and homeostasis and is controlled via PI3K and JAK/STAT signaling. Cell Death Dis. 7:e2103. doi: 10.1038/cddis.2016.23
Vincents, B., Guentsch, A., Kostolowska, D., von Pawel-Rammingen, U., Eick, S., Potempa, J., et al. (2011). Cleavage of IgG1 and IgG3 by gingipain K from Porphyromonas gingivalis may compromise host defense in progressive periodontitis. FASEB J. 25, 3741–3750. doi: 10.1096/fj.11-187799
Wang, M., Shakhatreh, M. A., James, D., Liang, S., Nishiyama, S., Yoshimura, F., et al. (2007). Fimbrial proteins of porphyromonas gingivalis mediate in vivo virulence and exploit TLR2 and complement receptor 3 to persist in macrophages. J. Immunol. 179, 2349–2358. doi: 10.4049/jimmunol.179.4.2349
White, P. C., Chicca, I. J., Cooper, P. R., Milward, M. R., and Chapple, I. L. (2016). Neutrophil extracellular traps in periodontitis: a web of intrigue. J. Dent. Res. 95, 26–34. doi: 10.1177/0022034515609097
White, P., Sakellari, D., Roberts, H., Risafi, I., Ling, M., Cooper, P., et al. (2016). Peripheral blood neutrophil extracellular trap production and degradation in chronic periodontitis. J. Clin. Periodontol. 43, 1041–1049. doi: 10.1111/jcpe.12628
Wiesmeier, M., Gautam, S., Kirschnek, S., and Hacker, G. (2016). Characterisation of neutropenia-associated neutrophil elastase mutations in a murine differentiation model in vitro and in vivo. PLoS ONE 11:e0168055. doi: 10.1371/journal.pone.0168055
Wingrove, J. A., DiScipio, R. G., Chen, Z., Potempa, J., Travis, J., and Hugli, T. E. (1992). Activation of complement components C3 and C5 by a cysteine proteinase (gingipain-1) from Porphyromonas (Bacteroides) gingivalis. J. Biol. Chem. 267, 18902–18907.
Yasunari, K., Watanabe, T., and Nakamura, M. (2006). Reactive oxygen species formation by polymorphonuclear cells and mononuclear cells as a risk factor of cardiovascular diseases. Curr. Pharm. Biotechnol. 7, 73–80. doi: 10.2174/138920106776597612
Yu, J. J., Ruddy, M. J., Wong, G. C., Sfintescu, C., Baker, P. J., Smith, J. B., et al. (2007). An essential role for IL-17 in preventing pathogen-initiated bone destruction: recruitment of neutrophils to inflamed bone requires IL-17 receptor-dependent signals. Blood 109, 3794–3802. doi: 10.1182/blood-2005-09-010116
Keywords: periodontitis, neutrophils, Porphyromonas gingivalis, virulence factors, inflammation
Citation: Sochalska M and Potempa J (2017) Manipulation of Neutrophils by Porphyromonas gingivalis in the Development of Periodontitis. Front. Cell. Infect. Microbiol. 7:197. doi: 10.3389/fcimb.2017.00197
Received: 28 February 2017; Accepted: 04 May 2017;
Published: 23 May 2017.
Edited by:
Lee-Ann H. Allen, University of Iowa, United StatesReviewed by:
George Hajishengallis, University of Pennsylvania, United StatesThomas Elliott Van Dyke, The Forsyth Institute, United States
Marko Radic, University of Tennessee College of Medicine, United States
Copyright © 2017 Sochalska and Potempa. This is an open-access article distributed under the terms of the Creative Commons Attribution License (CC BY). The use, distribution or reproduction in other forums is permitted, provided the original author(s) or licensor are credited and that the original publication in this journal is cited, in accordance with accepted academic practice. No use, distribution or reproduction is permitted which does not comply with these terms.
*Correspondence: Maja Sochalska, bWFqYV9zb2NoYWxza2FAeWFob28uY29t